- 1Servicios Especializados de Consultoría e Investigación Medioambiental, Madrid, Spain
- 2Terrestrial Ecology Group, Departamento de Ecología, Universidad Autónoma de Madrid, Madrid, Spain
- 3Jefatura de Producción, Gerencia de Servicios Comerciales Este, Renfe Viajeros, Madrid, Spain
- 4Servicios Comerciales, Dirección de Servicios Comerciales-Gerencia de Área de Operaciones, Renfe Viajeros, Madrid, Spain
Large high-speed railway (HSR) networks are planned for the near future to accomplish increased transport demand with low energy consumption. However, high-speed trains produce unknown avian mortality due to birds using the railway and being unable to avoid approaching trains. Safety and logistic difficulties have precluded until now mortality estimation in railways through carcass removal, but information technologies can overcome such problems. We present the results obtained with an experimental on-board system to record bird-train collisions composed by a frontal recording camera, a GPS navigation system and a data storage unit. An observer standing in the cabin behind the driver controlled the system and filled out a form with data of collisions and bird observations in front of the train. Photographs of the train front taken before and after each journey were used to improve the record of killed birds. Trains running the 321.7 km line between Madrid and Albacete (Spain) at speeds up to 250–300 km/h were equipped with the system during 66 journeys along a year, totaling approximately 14,700 km of effective recording. The review of videos produced 1,090 bird observations, 29.4% of them corresponding to birds crossing the infrastructure under the catenary and thus facing collision risk. Recordings also showed that 37.7% bird crossings were of animals resting on some element of the infrastructure moments before the train arrival, and that the flight initiation distance of birds (mean ± SD) was between 60 ± 33 m (passerines) and 136 ± 49 m (raptors). Mortality in the railway was estimated to be 60.5 birds/km year on a line section with 53 runs per day and 26.1 birds/km year in a section with 25 runs per day. Our results are the first published estimation of bird mortality in a HSR and show the potential of information technologies to yield useful data for monitoring the impact of trains on birds via on-board recording systems. Moreover, recordings point to the use of the infrastructure by birds as a key issue leading to bird train-kill.
Introduction
Railway has substantial growth potential as a mode of transportation given its energy efficiency, low emissions, and low perceived environmental impact relative to the other alternatives (Profillidis et al., 2014; Dorsey et al., 2015). Economic development is closely linked to increased mobility of people and goods, which can drive continued global growth of passengers, goods volumes, and length of transportation infrastructure (Chapman, 2007; Dritsaki and Dritsaki, 2014). However, to address environmental effects, especially energy consumption and greenhouse gas emissions, economic development strategies must define transportation plans that maximize benefits and minimize environmental costs (EC, 2011; Dulac, 2013; Liu et al., 2013). Railroad transportation is thus becoming an outstanding component of such strategies (Dulac, 2013; UIC, 2016).
High-speed railways (HSRs) are key to the development of high-capacity transportation networks that can compete successfully with air transport for medium distances. For instance, the European Union is planning to double the size of its HSR network by 2030, aiming for the majority of passenger travel to occur by rail by that time (EC, 2011). For routes in which high-speed trains compete directly with air travel, such as, between Madrid and Barcelona, Spain (624 km), once trains are operational, they partially absorb passengers from other modes of transportation (Castillo-Manzano et al., 2015). Medium term, supply and demand patterns for air travel and HSRs adapt to the new market conditions (Pellegrini and Rodriguez, 2013) and the disadvantages of each mode of transportation are reduced if demand is stable (Albalate et al., 2015; D'Alfonso et al., 2015). In this context construction of HSR networks is planned in different parts of the world as a priority mode of intercity connection (Campos and de Rus, 2009; USDOT, 2009; Todorovich et al., 2011; Fu et al., 2012).
However, HSR planning faces a key environmental challenge: practically nothing is known regarding the potential environmental effects of HSRs beyond pollution and climate change (Popp and Boyle, 2017). The destruction of habitats and their degradation close to the infrastructure (edge effect) is thought to be less important for railroads than for highways given the smaller breadth of its infrastructure and lower traffic intensity (Dorsey et al., 2015; Borda-de-Água et al., 2017). It has also been suggested that vertebrate mortality from collisions should not be a major problem because of the relatively low frequency of train circulation, although under certain conditions, it can become a relevant issue and perhaps a bigger problem than for roads running parallel to the railways (Waller and Servheen, 2005). In the case of birds, collision frequency with high speed trains is practically unknown (e.g., Loss et al., 2015), but could be high since (i) birds use areas surrounding railways and can even be attracted to them (e.g., for traditional rail lines Havlin, 1987; De la Pe-a and Llama, 1997; Mammen et al., 2002; Li et al., 2010; Wiaçek et al., 2015), (ii) slow flight birds are less able to avoid rapid trains, being then more often at risk of road or railroad-kill (Glue, 1971), and (iii) trains run at such high speeds that birds cannot evade an oncoming train (DeVault et al., 2015).
Because it's difficult to work within and around HSR networks, scientific knowledge of bird mortality from collisions is very scarce (see reviews by Dorsey et al., 2015 and Santos et al., 2017). The infrastructure is fenced and highly restricted to human access for safety reasons, making it very difficult to run field studies (Wells et al., 1999). Also, bird carcasses from train collisions are left in a condition which makes location and identification difficult, especially in the presence of scavenger animals around railways (Heske, 2015; Reyes et al., 2016). Besides, the sheer breadth of rail networks and cost of fieldwork make it practically impossible to conduct studies that cover even a small part of a network. Such work is thus feasible only along short stretches of the line. The preliminary results of an ongoing field study along 10.6 km of HSR in Spain (LIFE+ Impacto Cero, 2017) show bird mortality of about 91.3 birds/km/year, with species being affected in accordance to their abundance (Malo et al., 2016).
Information technologies may be critical to overcome the difficulties inherent in estimating HSR bird mortality, specifically the use of on-board systems that record collisions. Such systems should include (i) a cabin-front recording camera, (ii) image and data storage of the instant the events occur (location, speed, etc.), and (iii) data review and interpretation to enable extraction of only relevant data from the huge volume of data collected. Technology advances have already addressed the first two requirements and the emergence of an artificial intelligence system may soon enable automated analysis of the generated images (Yu et al., 2013; Longmore et al., 2017).
While awaiting technology advances, there is, thus far, no experience with applying extant technology to the problem of HSR bird mortality, although some illuminating work has been done in car-dragonfly collisions (Furness, 2014). For HSRs, on-board systems could solve safety, cost, and spatial extent issues that today preclude even a basic estimation of bird mortality.
The objectives of this article are (i) to demonstrate the use of a high-speed image recording system aboard high-speed trains for analyzing behavior and mortality of colliding birds, and to discuss the potential uses of such a system; and (ii) to present initial estimates of bird collision mortality and associated data on bird behavior during train approaches, which will be valuable for developing corrective measures.
Materials and Methods
We recorded bird collisions with trains using a portable system comprising a high-speed digital camera and a data logger with high-recording-frequency GPS navigation (Figure 1). The video camera was a commercial model (GoPro Hero 3+ Black Edition) that records in a super-wide format (170°) at 120 frames per second (FPS) and 1,280 × 720 pixel image resolution or 240 FPS with 848 × 480 pixel image resolution. After a few initial recordings at 240 FPS, we decided to use 120 FPS to achieve clearer images of the collision events. The small camera size enabled us to anchor it with a suction cup to the front windshield of the train without loss of visibility for the train driver. For recording and precise geolocation of the images, we used a professional data logger with GPS (Qstarz BT-Q1000eX) with a 10-Hz location-refreshing frequency. GPS data were processed with the QRacing™ v3.3.3 software (Qstarz International Company Ltd.) and they were synchronized with data from the camera with Dashware (GoPro Inc.). We installed the system in the front cabin of high-speed trains (GEC Alstom-series S100-and Talgo-Bombardier-series S112 and S130) from Renfe Viajeros in regular circulation on the line running at speeds up to 250–300 km/h.
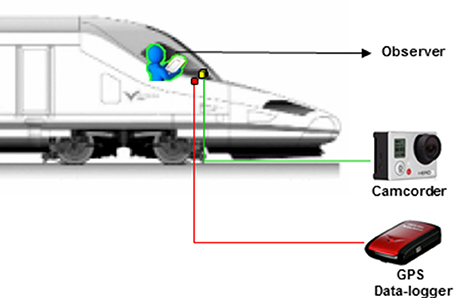
Figure 1. Basic diagram of the collision detection system for a high-speed train. In the train cabin, a high-speed camera was aimed forward, connected to a data logger that records GPS navigation data continuously. In parallel, an observer records on a form the crossing and collision of birds with the train.
In parallel with video recordings, an observer standing in the cabin behind the driver filled out a form to note trip data and observation of birds in front of the train (time, location, bird size, number of individuals, and behavior) as well as collisions detected from the cabin. We used these data to focus the review of each video on the points of the line where birds had been detected (see below). Also, for each trip, we photographed the front of the train before and after the trip, to study collision evidences (feather remains and blood) that are often found on the train bodywork in search of additional collisions not seen from the cabin.
All the filming was done between Madrid and Albacete stations of the high-speed line, 321.7 km apart (Supplementary Figure 1). The rail route crosses mostly rural areas with dryland crops (~60%; south of Madrid, Mesa de Ocaña-Tarancón, La Manchuela) and irrigated land (~10% near Albacete), as well as forest and scrubland areas (~20% around Cuenca). The line also crosses peri-urban and industrial zones (~8%) and river meadows (~2%), especially near the Tajo and Júcar river crossings. Part of the line passes near two Special Protection Areas of ornithological interest linked to steppe and rural habitats (Natura 2000 site ES0000170) and marshlands (Natura 2000 site ES0000119). The line also crosses several protected areas of pseudo-steppe habitat interest.
Most of the railway runs on the land surface and the design of the rail line permits speeds above 250 km/h. Because the slopes do not exceed a 2‰ (0.2%) grade, the rail line runs at the level of the land in the flattest parts, and on embankments, mostly 2- to 15-m high, where the terrain is uneven. The line includes 20 tunnels between 187 m and 3,128 m long, 7 of which are more than 1-km long. There are also 30 viaducts between 127 m and 1,600 m long. The HSR line runs 30 trains per day on working weekdays and 11 trains per day on holidays, with a journey duration between Madrid and Albacete of 1 h 30 min (average speed 214 km/h). On the same line, trains run between Madrid and Motilla del Palancar (km 251) toward Valencia with frequencies of 30 runs on work days and about 25 on holidays. Trains regularly run during daytime, though some circulations take place at nighttime short before dawn o after dusk, mainly in winter.
We conducted the recordings between July 2014 and May 2015, with a total of 66 recording journeys at daytime distributed across seasons (14–20 recordings per season). Two journeys were done every filming day, one early in the morning beginning around dawn from Madrid and the return trip from Albacete in the late evening. Our sampling was thus balanced between mornings and evenings, though logistic restrictions precluded a fully balanced sampling along the whole day. The system could not operate at nighttime with the front lights of the train, thus leaving a full gap of knowledge for this situation. All recordings (total recording time of 59 h and 55 min) were downloaded to a hard drive for analysis. The recordings covered about 14,700 km of cumulative travel (average 222.7 km per recording, range 78.3–288.7 km).
A single investigator carried out film data extraction by direct viewing each recording at all the points where the cabin observer had indicated the presence of birds (about 500–1,000 m in each case). In addition, in all the recordings, we reviewed the video for two stretches of the line at km 83–89 and 96–101 (total, 10.6 km), where there are ongoing field studies of bird behavior and mortality (Malo et al., 2016, 2017). This additional review, along with some review of other small portions of recordings, comprised about 5% of the total recording time.
From each bird observation, we extracted the following data: number of individuals, taxonomic identification as precise as possible, position (resting/flying) at the first frame in which the birds were visible, time, location (geographic, and kilometer position), train speed, surrounding habitat, typology of the rail line, and if birds flew across the line. For resting birds we noted if they were using elements of the infrastructure (wires, poles…) or not. In the case of crossings, we differentiated between those that occurred above the catenary or between the rails and the catenary located at a 5.3-m height. The gap between the rails and the catenary defines the bird-train collision risk area.
In recordings where the birds were at rest at first detection, we reviewed the image sequences to estimate the birds' distance from the train when they started their escape flight (FID, flight initiation distance). FID is a common measure of the bird response to human disturbance or predation risk (Weston et al., 2012), and it is directly related with the remaining time to escape from the approaching train. We used the poles supporting the catenary, which are 60 m apart, as a distance reference. Also, for each sequence, we determined whether or not the observation resulted in a bird collision with the train.
To estimate the mortality rate due to collision (colliding birds per km traveled), we counted the number of colliding birds directly recorded by the camera on each trip and added the number of collision marks on the front of the train that did not correspond to observed collisions. We used this estimate of mortality per km traveled to calculate the annual bird mortality for the two line sections, Madrid-Motilla del Palancar with about 53 runs per day and Motilla del Palancar and Albacete with 25 runs per day.
Temporal patterns of bird observation from the cabin were analyzed through a chi-squared test comparing the number of observations in morning vs. afternoon journeys. Temporal patterns were not further analyzed due to the time and spatial restrictions of our recordings (uneven in time and beginning every morning from Madrid). To better understand the underlying factors leading to mortality, FID variability among bird groups was analyzed via one-way ANOVA. For this analysis we considered only observations from bird groups with more than 10 records in order to work with more reliable estimates of this variable. Four bird groups were thus considered according to taxonomical and ecological criteria (passerines, pigeons and doves, corvids, and mid-sized raptors). Also, a t-test was carried out to compare the speed of the train in events of bird crossing under the catenary ending in collision vs. those in which the bird escaped. All analyses were carried out with STATISTICA 8.0 (StatSoft, Inc., 2007).
Results
For 66 journeys, we obtained a total of 1,090 recorded observations of birds from the cabin (Figure 2) distributed throughout the line (Supplementary Figure 1). Of these, 1,040 were seen directly by the observer and 50 (4.6%) were obtained during film review. Considering all of the raw data, we estimated an average frequency of 16.5 observations per journey (range 0–41) and 0.07 observations per km traveled (i.e., one observation every 13.9 km). Observations were more frequent in the morning journeys than in the afternoon ones (65.0 vs. 35.0%; chi-squared test = 48.8; d.f. = 1; p < 0.001), and they probably peak with the first lights of the morning.
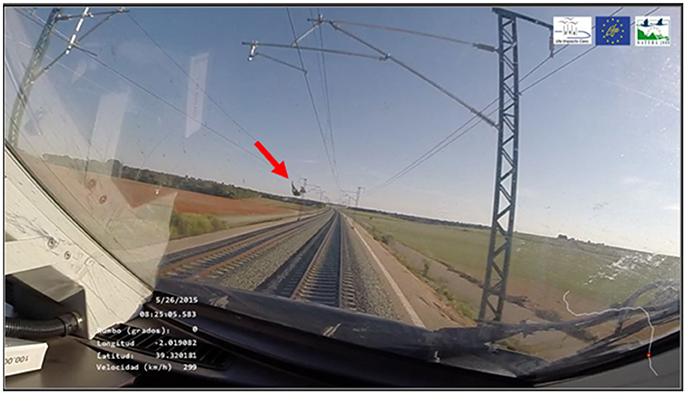
Figure 2. Image capture of a recording from a train running at high speed. Lower left quadrant, in white, shows data inserted by the GPS system. Red arrow shows bird position moments before collision with the train.
The recording quality enabled at least a partial taxonomic identification for most of the observations (Supplementary Table 1), at a species level in 45.5% of the cases and at a supra-species level in 52.0% of the cases. In 1.5% of the observations, we could not determine the type of bird. We identified a total of 32 different bird species of which 62.5% were non-passerines (especially daytime raptors, Falconiformes, including eight separate species) and the remaining 37.5% were passerines (especially corvids, Corvidae, including four species). Based on the number of observations, passerines predominated with 52.2% of recordings (30.5% corvids and 21.7% other passerines), followed by 27.6% of pigeons and doves (Columbiformes, especially the common wood pigeon, Columba palumbus). We also detected daytime raptors (Falconiformes; 16.4%) and other non-passerine species of various orders (2.3%; Supplementary Table 1).
In terms of flock size, most bird observations were of singletons (70.1%) and bird pairs (13.4%). We recorded flocks of 3–10 individuals in 12.8% of the cases and flocks of 11 to 50 individuals in 2.7% of the cases. Only in 1% of the cases did we observe more than 50 individuals (including a flock of spotless starlings, Sturnus unicolor, of ~350 individuals).
In terms of infrastructure crossing behavior, in most of the observations (80.5%), birds crossed the infrastructure, with 51.0% flying over the catenary and 29.4% flying under the catenary in the collision risk zone. The birds that did not cross the rail line mostly stood on the infrastructure (e.g., on power lines or poles) or escaped from the infrastructure with no risk of collision. Only 2.3% of the observations were of birds that did not cross the infrastructure nor used it for resting. A relevant feature is that 27.1% of the line crossings were of birds initially resting on some element of the infrastructure (rails, catenary, fencing, etc.); this portion reached 37.7% for birds crossing within the collision risk zone.
In 162 cases, we could determine the FID and found significant differences [ANOVA F(3, 158) = 31.55, p < 0.001] between different groups of birds (Table 1). Thus, there was a gradient in which the birds with lower FIDs were passerines (7 Galerida cristata, 1 Lanius meridionalis, and 4 indeterminate), followed by pigeons and doves (79 C. palumbus, 8 Columbia livia, and 2 Columba sp.), corvids (10 Corvus sp., 3 Corvus corone, 1 Corvus corax, 2 Corvus modenula, and 6 Pica pica), and finally midsize and large raptors (18 Buteo buteo, 1 Milvus milvus, 1 Milvus sp., 2 Aquila chrysaetos, and 18 indeterminate). In 11.2% of the recordings of pigeons and doves, the birds did not take flight as the train passed (i.e., FID = 0), but rather remained at rest on the catenary cables.
Finally, we counted a total of 42 bird collisions, 28 of them recorded from the cabin. Collisions were recorded in 47.0% of the journeys. As a whole, the data are equivalent to a mortality of 0.0029 birds per km traveled by a high-speed train (i.e., one collision every 349.8 km). With respect to average traffic on the HSR in this study, the mortality is estimated to be 60.5 birds/km year on the Madrid-Motilla del Palancar section and 26.1 birds/km year between Motilla del Palancar and Albacete.
For colliding species, among 28 cases recorded from the cabin, 53.6% were small passerines, 14.3% were pigeons and doves, 10.7% were corvids, and 10.7% were other non-passerines (Table 2). In 10.7% of the cases, we could not determine the species or group of the colliding bird. The percentage of observations from each species ending in collision was very variable (Table 2), though these features reflect a mix of both interspecific differences in flight behavior and size-related differences in detectability (see also Supplementary Table 1).
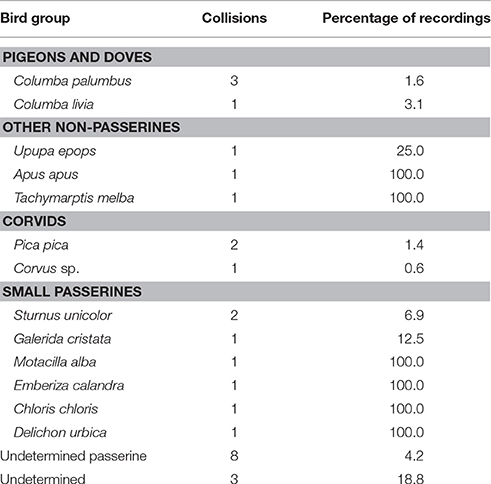
Table 2. Number of bird collisions by species recorded from the train cabin, and percentage of recordings ending in collision with the train.
In 12.1% of the observations of birds crossing under the catenary there was a collision. All collisions occurred in high-speed situations (mean ± standard deviation, 265.8 ± 39.2 km/h; range, 175–305 km/h; N = 20), with no significant differences (t-test = 1.06; d.f. = 201; p = 0.291) relative to the remainder of line crossings under the catenary (251.5 ± 58.9 km/h; range, 0–305 km/h; N = 183). Notably, the recordings showed that 17.8% of the birds that collided were individuals initially resting on an element of the infrastructure when they were first visible in the recording.
Discussion
The present results show the potential of information technology in analyzing the impact of high-speed trains on bird communities in the areas they cross. In addition, the data presented provide a first approximation to direct bird mortality from high-speed trains, and enable an initial understanding of some key aspects of bird behavior that lead to mortality risk.
It is important to note that the estimation of collision mortality seems reliable in the light of the available evidences (see below), even though it was obtained with a simple and portable system. The estimate of bird collisions obtained for the Madrid-Motilla del Palancar section (60.5 birds/km/year) was of the same order of magnitude as that measured in a simultaneous sampling over an entire year on two stretches totaling 10.6 km (91.3 birds/km/year; Malo et al., 2016). The difference could be due to the spatial variability in abundance of birds surrounding the rail lines (Supplementary Table 1) and the fact that field studies were carried out in an area adjacent to a Special Protection Area of ornithological interest (Natura 2000 site ES0000170). Moreover, the lack of a balanced sampling along the whole day and the absence of nighttime recordings add some uncertainties of unknown direction to the estimate. It is noticeable in this sense that owls are known to die in traditional railways (Glue, 1971; De la Pe-a and Llama, 1997), and HSR have some nighttime circulations that also lead to owl death (Malo et al., 2016). Such mortality associated to trains running at night could not be analyzed here, and it probably affects few but completely different species as those recorded here. In conclusion, further recordings including nighttime would be needed for a detailed assessment of bird mortality in HSR, but our data can be seen as a first reliable approach to it. In the scientific literature, there are only internal rail company documents recording bird mortality for high-speed trains, and published studies for traditional lines to date are based on very targeted field carcass samplings (Havlin, 1987; De la Pe-a and Llama, 1997; Mammen et al., 2002; Heske, 2015). The lack of data on the impact of trains on fauna extends to all types of rail lines and compromises their environmental evaluation (Wells et al., 1999; Rodríguez et al., 2008; Dorsey et al., 2015).
This is the first time that a system such as, ours has been used to study bird collisions with trains, although a similar system was used to study dragonfly-car collisions (Furness, 2014). To date, few studies have used data obtained in train cabins from direct observation (Wells et al., 1999) or driver surveys (SCV, 1996). Video recording observations combined with collection of GPS data improves the collection of precise data, both for identifying the species involved and for determining location and circumstances of the collisions (train speed, bird behavior, etc.). Techniques like video recording from fixed points, vehicles, or animal-borne cameras have been applied to study animal behavior and monitor wildlife (Whorff and Griffing, 1992; Thompson et al., 1999; Okuyama et al., 2015), but rarely to assess mortality risk (Desholm et al., 2006; Cryan et al., 2014; Furness, 2014; Doppler et al., 2015).
As for the types of birds crossing the lines and colliding with trains, our results showed the presence of birds common to the agrarian landscapes along the railway, and especially those birds that use the infrastructure as a habitat for feeding, resting, and even nesting (Havlin, 1987; Li et al., 2010; Morelli et al., 2014; Malo et al., 2017). Most observations were done early in the morning and corresponded to passerines, many of which could not be identified precisely. We recorded pigeons and doves, large corvids (most of them probably C. corone), and midsize corvids (e.g., P. pica), frequently. But we also observed raptors such as, B. buteo in several occasions as well as other species that might approach the railway looking for carcasses or prey, primarily rabbits and rodents (see for motorways Planillo et al., 2015). On the whole, the collision data are relatively worrisome from a biological perspective, involving a large number of individuals, some portion of which may be protected species (Mammen et al., 2002; see also Malo et al., 2016). From the point of view of train operation and safety, the possibility of collision with large birds or with large flocks is not insignificant, given the potential cost of stopping the trains and repairing the damage (Seiler et al., 2014; Renfe Viajeros, unpublished data).
Our mortality estimates via on-board camera systems are also useful for quantifying the time and cost savings achievable with the use of information technologies. To obtain cabin data, we invested the recording time (an observer for 33 days of round trips) and the time for review and assembly of the data (an ornithologist working for about 50 days). In comparison, field data collection in the two sub-stretches of rail line required a group of at least three ornithologists and one safety technician during 48 days (4 seasons * 6 surveys * 2 sub-stretches) as well as parallel installation of baited stations to estimate carcass removal rates and carcass detectability (Reyes et al., 2016). Added to these resources, would be preparation time for field material and datasets (total of around 24 person-days). In short, on-board recording systems can enable much more efficient data collection than traditional methods based on direct personal observation (83 vs. 216 person-days in this example), underscoring the current trend of increasing reliance on information technology for ecological studies (Tomkiewicz et al., 2010; Galliard et al., 2012; Gurarie et al., 2016; Price and Schmitz, 2016). In our case, automation not only saved labor costs but also enabled us to broaden the study beyond what is possible with direct fieldwork to the more than 300 km that comprise the entire rail line.
In addition, the on-board recordings enabled us to describe three factors of bird behavior that determine mortality. Thus, (i) we confirmed that a large percentage of observed birds cross the rail lines under the catenary and are thus at risk for collision. Slightly more than one of every three recorded crossings occurred in the risk zone, demonstrating that birds do not perceive the risk of crossing the gap between the rails and a power wire located at a 5.3-m height. Although this estimate may be subject to some bias, given that on-board recordings may underestimate events above the catenary, the results are informative, showing a high of collision risk during low flight, a routine characteristic in bird flight patterns (Shamoun-Baranes et al., 2006; Wulff et al., 2016). Also, (ii) we confirmed that the use of various infrastructure elements by birds (poles, catenary, embankments) strongly determines their risk of collision (Morelli et al., 2014; Mainwaring, 2015); almost 40% of the crossing events under the catenary occurred for birds resting on the infrastructure moments before the train arrival.
Lastly, (iii) the recordings showed that, frequently, the birds reacted to the train's approach when it was almost upon them. The escape distances for different groups of birds (60–140 m) correspond to flight initiation when the train traveling at 300 km/h is only 0.72 to 1.68 s away from the resting point. The data thus demonstrate the problem faced by many birds: too late response to avoid collision with the high-speed train. This is probably the general rule, even though recordings do not show average behavior of all birds since the on-board system only registers events relatively close to the train. Thus, it misses birds that react early and escape upon commencement of ground and catenary vibration, which occurs 5–10 s before train arrival (personal observation). The delayed reaction is in accordance with the limited capacity of the birds to react to objects approaching at high speed (Martin, 2011; DeVault et al., 2015), and depicts the reduced probability of wildlife to escape from an approaching high-speed train.
On technical grounds, the system used for this experiment is relatively low cost and available on the market. However, there are other technologies and equipment, which are more expensive and less accessible, that have enormous potential to advance this field of work. Our GPS navigation system often lost signal due to tunnels or cabin interference, but future systems could use the automated position controls present in the train itself. The recordings, which were carried out mostly at 120 FPS and 1,280 × 720 pixel resolution, enabled us to identify bird species in about 45% of the cases. The identification most often was only possible after a detailed review of the complete recording sequences and was based as much on details of the birds in the images with sufficient definition as on the flight patterns observed in the sequence as a whole. Modern cameras can record up to 4.4 trillion FPS (Nakagawa et al., 2014) and 7,580 × 4,320 pixel cameras also exist on the market (e.g., WEAPON 8K S35) for very specific applications, but they have limited recording capacity in terms of recording time due to the data volume generated. In this trade-off between recording speed and image quality, it is probable that, in the near future, cameras capable of 480 FPS and 4,096 × 2,160 pixels may be available at affordable prices for applications such as, our study. With such images, it might be possible to recognize at a species level most of the birds that cross in front of the train, especially in cases where images show them very close to the train, a brief instant before collision.
Additionally, artificial intelligence systems under development are expected to enable extraction of useful wildlife data from images taken in nature by automated cameras (e.g., Yu et al., 2013; Longmore et al., 2017). This extraction capacity will be a key advance to enable data analysis of high-speed recordings from train fronts, similar to the extraction of useful biological monitoring data from continuous recording webcams or sensor networks (Benson et al., 2010; Porter et al., 2010; Evans et al., 2015). In our case, review of the recordings was focused on the points at which the cabin observer detected birds in front of the train, and on a small complementary sampling of the recordings as a whole. Since review of the image sequences must be done at a slow speed (24 FPS) or even frame-to-frame, the time spent for reviewing is much longer than the length of the sequences of interest and it limits a generalized use on the trains (see also Longmore et al., 2017). In any case, automating data interpretation of the on-board recordings will be a challenge. The rails, posts, and catenary that comprise the infrastructure limit the analysis focus, but the constant (relative) movement of the background makes it difficult to distinguish the targets (birds) from the landscape elements in the recordings (Yu et al., 2013; Longmore et al., 2017). A solution for this would be to combine high-speed cameras with complementary sensors (e.g., sound/impact) in the front of the train, to precisely record collision impacts, and in combination with automated recording systems capture specifically the video fragments for the moments just before a collision occurs. Such a solution would also limit the volume of information to be stored in hardware. Future potential for improvement of the system is thus huge.
Anyhow, the system in our study shows the present and near-future potential for such high-speed recording systems boarded on trains to assess the environmental impact of HSRs. First, information gleaned from massive data on bird crossings and collisions with trains in different settings (landscapes, seasons, time of the day) would be of high interest for proper environmental assessment of new and planned rail infrastructure as a viable travel option in the future (USDOT, 2009; EC, 2011; UIC, 2015). Collision mortality data may command attention in railways in the same way it already does in roads (Benítez-López et al., 2010; Dorsey et al., 2015). Second, high-definition recordings will provide a better understanding of how, where, and why collisions occur, opening the door for corrective measures (Rodríguez et al., 2008; Gunson et al., 2011). Longer term, these onboard systems may also be able to feed data to the Environmental Monitoring Programs of these rail infrastructures, continuing to improve both prevention and mitigation (Williams and Brown, 2014). At present, our data imply that (i) mitigation should be focused on reducing the proportion of birds flying under the catenary for instance by use of barrier-like structures, at least in locations of high collision risk (see Rodríguez et al., 2008; LIFE+ Impacto Cero, 2017). Also, (ii) efforts should be aimed at reducing the attractiveness of rail surroundings to birds, not only structural elements (poles, catenary), but also the habitat of the embankments (Malo et al., 2017), as well as reducing the potential of dense populations of prey in embankments that can attract birds (Planillo et al., 2015). Unfortunately, addressing the small escape distance for the birds may not be possible given the sensory and behavioral limitations of birds (Martin, 2011; DeVault et al., 2015).
Author Contributions
EG, JM, IH, CM, SG, and JH contributed substantially to different parts of the study design, data acquisition, analysis and interpretation. First text was drafted by EG and JM with contributions from IH, CM, SG, and JH, and final paper was revised and approved by EG, JM, IH, CM, SG, and JH. EG, JM, IH, CM, SG, and JH are accountable for all aspects of the work regarding their accuracy and integrity.
Funding
This study has been carried out as part of research project LIFE+ Impacto 0 (LIFE 12 BIO/ES/000660) focused upon the mitigation of High Speed Railway impact on bird populations. The Comunidad de Madrid, together with the European Social Fund, supports the Terrestrial Ecology Group research group through the REMEDINAL-3 Research Network (S-2013/MAE-2719).
Conflict of Interest Statement
The authors declare that the research was conducted in the absence of any commercial or financial relationships that could be construed as a potential conflict of interest.
Acknowledgments
Staff from Adif (Administrador de Infraestructuras Ferroviarias) kindly helped with project logistics. The final manuscript was improved with comments from two reviewers.
Supplementary Material
The Supplementary Material for this article can be found online at: https://www.frontiersin.org/articles/10.3389/fevo.2017.00117/full#supplementary-material
References
Albalate, D., Bel, G., and Fageda, X. (2015). Competition and cooperation between high-speed rail and air transportation services in Europe. J. Transp. Geogr. 42, 166–174. doi: 10.1016/j.jtrangeo.2014.07.003
Benítez-López, A., Alkemade, R., and Verweij, P. A. (2010). The impacts of roads and other infrastructure on mammal and bird populations: a meta-analysis. Biol. Conserv. 143, 1307–1316. doi: 10.1016/j.biocon.2010.02.009
Benson, B. J., Bond, B. J., Hamilton, M. P., Monson, R. K., and Han, R. (2010). Perspectives on next-generation technology for environmental sensor networks. Front. Ecol. Environ. 8, 193–200. doi: 10.1890/080130
Borda-de-Água, L., Barrientos, R., Beja, P., and Pereira, H. M. (2017). “An introduction to railway ecology,” in Railway Ecology, eds L. Borda-de-Água, R. Barrientos, P. Beja, and H. M. Pereira (Cham: Springer), 3–10.
Campos, J., and de Rus, G. (2009). Some stylized facts about high-speed rail: a review of HSR experiences around the world. Transport. Policy 16, 19–28. doi: 10.1016/j.tranpol.2009.02.008
Castillo-Manzano, J. I., Pozo-Barajas, R., and Trapero, J. R. (2015). Measuring the substitution effects between High Speed Rail and air transport in Spain. J. Transp. Geogr. 43, 59–65. doi: 10.1016/j.jtrangeo.2015.01.008
Chapman, L. (2007). Transport and climate change: a review. J. Transp. Geogr. 15, 354–367. doi: 10.1016/j.jtrangeo.2006.11.008
Cryan, P. M., Gorresen, P. M., Hein, C. D., Schirmacher, M. R., Diehl, R. H., Huso, M. M., et al. (2014). Behavior of bats at wind turbines. Proc. Natl. Acad. Sci. U.S.A. 111, 15126–15131. doi: 10.1073/pnas.1406672111
D'Alfonso, T., Jiang, C., and Bracagli, V. (2015). Would competition between air transport and high-speed rail benefit environment and social welfare? Transport. Res. B Methodol. 74, 118–137. doi: 10.1016/j.trb.2015.01.007
De la Pe-a, R., and Llama, O. (1997). Mortalidad de Aves en un Tramo de Línea de Ferrocarril. Grupo local SEO-Sierra de Guadarrama. Available online at: http://www.actiweb.es/seosierradeguadarrama/archivo1.pdf (Accessed February 20, 2017).
Desholm, M., Fox, A. D., Beasley, P. D. L., and Kahlert, J. (2006). Remote techniques for counting and estimating the number of bird-wind turbine collisions at sea: a review. Ibis 148, 76–89. doi: 10.1111/j.1474-919X.2006.00509.x
DeVault, T. L., Blackwell, B. F., Seamans, T. W., Lima, S. L., and Fernández-Juricic, E. (2015). Speed kills: ineffective avian escape responses to oncoming vehicles. Proc. R. Soc. Lond. B. Bio. 282:20142188. doi: 10.1098/rspb.2014.2188
Doppler, M. S., Blackwell, B. F., DeVault, T. L., and Fernández-Juricic, E. (2015). Cowbird responses to aircraft with lights tuned to their eyes: implications for bird–aircraft collisions. Condor 117, 165–177. doi: 10.1650/CONDOR-14-157.1
Dorsey, B., Olsson, M., and Rew, L. J. (2015). “Ecological effects of railways on wildlife,” in Handbook of Road Ecology, eds R. van der Ree, D. Smith, and C. Grilo (Chichester, UK John Wiley & Sons, Ltd.), 219–227.
Dritsaki, C., and Dritsaki, M. (2014). Causal relationship between energy consumption, economic growth and CO2 emissions: a dynamic panel data approach. Int. J. Energ. Econ. Policy 4, 125–136. doi: 10.1016/j.ecolecon.2007.11.006
Dulac, J. (2013). Global Land Transport Infrastructure Requirements. Estimating Road and Railway Infrastructure Capacity and Costs to 2050. Paris: International Energy Agency Publications.
EC (2011). European Commission Directorate-General for Mobility and Transport. White Paper on Transport: Roadmap to a Single European Transport Area: Towards a Competitive and Resource-Efficient Transport System. Brussels: Publications Office of the European Union. 144 final.Available online at: https://ec.europa.eu/transport/themes/strategies/2011_white_paper_en (Accessesd February 16, 2017).
Evans, D. R., McArthur, S. L., Bailey, J. M., Church, J. S., and Reudink, M. W. (2015). A high-accuracy, time-saving method for extracting nest watch data from video recordings. J. Ornithol. 156, 1125–1129. doi: 10.1007/s10336-015-1267-5
Fu, X., Zhang, A., and Lei, Z. (2012). Will China's airline industry survive the entry of high-speed rail? Res. Transport. Econ. 35, 13–25. doi: 10.1016/j.retrec.2011.11.006
Furness, A. N. (2014). Why Shouldn't the Dragonfly cross the Road? the Influence of Roadway Construction and Vehicle Speed on the Behavior, Mortality, and Conservation of Adult Dragonflies (odonata: Anisoptera) (Order No. 1569990). Degree of Master of Science, University of South Dakota.
Galliard, J. F. L., Guarini, J. M., and Gaill, F. (2012). Sensors for Ecology. Towards Integrated Knowledge of Ecosystems. Paris: CNRS-IMEE. Available online at: http://www.cnrs.fr/fr/pdf/inee/sensors_for_ecology/
Glue, D. E. (1971). Ringing recovery circumstances of small birds of prey. Bird Study 18, 137–146. doi: 10.1080/00063657109476307
Gunson, K. E., Mountrakis, G., and Quackenbush, L. J. (2011). Spatial wildlife-vehicle collision models: a review of current work and its application to transportation mitigation projects. J. Environ. Manage. 92, 1074–1082. doi: 10.1016/j.jenvman.2010.11.027
Gurarie, E., Bracis, C., Delgado, M., Meckley, T. D., Kojola, I., and Wagner, C. M. (2016). What is the animal doing? Tools for exploring behavioural structure in 575 animal movements. J. Anim. Ecol. 85, 69–84. doi: 10.1111/1365-2656.12379
Havlin, J. (1987). On the importance of railway lines for the avifauna in agrocoenoses. Folia Zool. 364, 345–358.
Heske, E. J. (2015). Blood on the tracks: track mortality and scavenging rate in urban nature preserves. Urban Nat. 4, 1–13.
Li, Z., Ge, C., Li, J., Li, Y., Xu, A., Zhou, K., et al. (2010). Ground-dwelling birds near the Qinghai-Tibet highway and railway. Transp. Res. D 15, 525–528. doi: 10.1016/j.trd.2010.07.004
LIFE+ Impacto Cero (2017). Development and Demonstration of an Anti-Bird Strike Tubular Screen for High Speed Rail Lines. LIFE+ Biodiversity project (LIFE12 BIO/ES/000660). Available online at: http://www.lifeimpactocero.com (Accessed February 15, 2017).
Liu, W., Lund, H., and Mathiesen, B. V. (2013). Modelling the transport system in China and evaluating the current strategies towards the sustainable transport development. Energy Policy 58, 347–357. doi: 10.1016/j.enpol.2013.03.032
Longmore, S. N., Collins, R. P., Pfeifer, S., Fox, S. E., Mulero-Pázmány, M., Bezombes, F., et al. (2017). Adapting astronomical source detection software to help detect animals in thermal images obtained by unmanned aerial systems. Int. J. Remote Sens. 38, 2623–2638. doi: 10.1080/01431161.2017.1280639
Loss, S. R., Will, T., and Marra, P. P. (2015). Direct mortality of birds from anthropogenic causes. Annu. Rev. Ecol. Evol. Syst. 46, 99–120. doi: 10.1146/annurev-ecolsys-112414-054133
Mainwaring, M. C. (2015). The use of man-made structures as nesting sites by birds: a review of the costs and benefits. J. Nat. Conserv. 25, 17–22. doi: 10.1016/j.jnc.2015.02.007
Malo, J. E., Hervás, I., García de la Morena, E. L., Mata, C., and Herranz, J. (2016). “Large and non-specific bird mortality in a high-speed railway traversing a Spanish agrarian landscape,” in Proceedings of the 5th International Conference on Ecology and Transportation, Lyon: Integrating Transport Infrastructure with Living Landscapes.
Malo, J. E., García de la Morena, E. L., Hervás, I., Mata, C., and Herranz, J. (2017). “Cross-scale changes in bird behavior around a high speed railway: from landscape occupation to infrastructure use and collision risk,” in Railway Ecology, eds L. Borda-de-Água, R. Barrientos, P. Beja, and H. M. Pereira (Lyon: Springer), 61.
Mammen, U., Klammer, G., and Mammen, K. (2002). “Greifvogeltod an Eisenbahntrassen - ein unterschaetztes problem [Dead of raptors at railways – an underestimated problem], in 5 Internationales Symposium “Populationsökologie von Greifvogel- und Eulenarten” [“Population Ecology of Raptors and Owls”] (Meisdorf/Harz).
Martin, G. R. (2011). Understanding bird collisions with man-made objects: a sensory ecology approach. Ibis 153, 239–254. doi: 10.1111/j.1474-919X.2011.01117.x
Morelli, F., Beim, M., Jerzak, L., Jones, D., and Tryjanowski, P. (2014). Can roads, railways and related structures have positive effects on birds? - a review. Transport. Res. 30, 21–31. doi: 10.1016/j.trd.2014.05.006
Nakagawa, K., Iwasaki, A., Oishi, Y., Horisaki, R., Tsukamoto, A., Nakamura, A., et al. (2014). Sequentially timed all-optical mapping photography (STAMP). Nat. Photon. 8, 695–700. doi: 10.1038/nphoton.2014.163
Okuyama, J., Nakajima, K., Matsui, K., Nakamura, Y., Kondo, K., Koizumi, T., et al. (2015). Application of a computer vision technique to animal-borne video data: extraction of head movement to understand sea turtles' visual assessment of surroundings. Anim. Biotelem. 3, 35. doi: 10.1186/s40317-015-0079-y
Pellegrini, P., and Rodriguez, J. (2013). Single European sky and single European railway area: a system level analysis of air and rail. Transport. Res. A Pol. 57, 64–86. doi: 10.1016/j.tra.2013.09.004
Planillo, A., Kramer-Schadt, S., and Malo, J. E. (2015). Transport infrastructure shapes foraging habitat in a raptor community. PLoS ONE 10:e0118604. doi: 10.1371/journal.pone.0118604
Popp, J. N., and Boyle, S. P. (2017). Railway ecology: underrepresented in science? Basic Appl. Ecol. 19, 84–93. doi: 10.1016/j.baae.2016.11.006
Porter, J., Lin, C., Smith, D. E., and Lu, S. (2010). Ecological image databases: from the webcam to the researcher. Ecol. Inf. 5, 51–58. doi: 10.1016/j.ecoinf.2009.09.001
Price, S. A., and Schmitz, L. (2016). A promising future for integrative biodiversity research: an increased role of scale-dependency and functional biology. Philos. Trans. R. Soc. Lond., B, Biol. Sci. 371:20150228. doi: 10.1098/rstb.2015.0228
Profillidis, V. A., Botzoris, G. N., and Galanis, A. T. (2014). Environmental effects and externalities from the transport sector and sustainable transportation planning - a review. Int. J. Energ. Econ. Policy 4, 647–661.
Reyes, G. A., Rodriguez, M. J., Lindke, K. T., Ayres, K. L., Halterman, M. D., Boroski, B. B., et al. (2016). Searcher efficiency and survey coverage affect precision of fatality estimates. J. Wildl. Manage. 80, 1488–1496. doi: 10.1002/jwmg.21126
Rodríguez, J. J., García de la Morena, E. L., and González, D. (2008). Estudio de las Medidas Correctoras Para Reducir las Colisiones de Aves Con Ferrocarriles de Alta Velocidad. Madrid: Ministerio de Fomento; CEDEX.
Santos, S. M., Rui, L., and Carvalho, F. (2017). “Wildlife mortality in railways,” in Railway Ecology, eds L. Borda-de-Água, R. Barrientos, P. Beja, and H. M. Pereira (Cham: Springer), 11–22.
SCV (1996). Mortalidad de Vertebrados en Líneas de Ferrocarril. Documentos Técnicos de Conservación SCV. Sociedad Para la Conservación de los Vertebrados, Madrid.
Seiler, A., Söderström, P., Olsson, M., and Sjölund, A. (2014). “Costs and effects of deer-train collisions in Sweden,” in Proceedings of the 4th International Conference on Ecology and Transportation, Life for a Greener Transport Infrastructure (Malmö). Abstracts book: 112.
Shamoun-Baranes, J., van Loon, E., van Gasteren, H., van Belle, J., Bouten, W., and Buurma, L. (2006). A comparative analysis of the influence of weather on the flight altitudes of birds. Bull. Am. Meteorol. Soc. 87, 47–61. doi: 10.1175/BAMS-87-1-47
Thompson, F. R., Dijak, W., and Burhans, D. E. (1999). Video identification of predators at songbird nests in old fields. Auk 116, 259–264. doi: 10.2307/4089477
Todorovich, P., Schned, D., and Lane, R. (2011). High-Speed Rail. International Lessons for U.S. Policy Makers. Cambridge, MA: Lincoln Institute of Land Policy.
Tomkiewicz, S. M., Fuller, M. R., Kie, J. G., and Bates, K. K. (2010). Global positioning system and associated technologies in animal behaviour and ecological research. Philos. Trans. R. Soc. Lond. B. Biol. Sci. 365, 2163–2176. doi: 10.1098/rstb.2010.0090
UIC (2015). High Speed Rail. Fast Track to Sustainable Mobility. Paris: International Union of Railways (UIC); Passenger and High Speed Department.
UIC (2016). Railway Handbook 2016. Energy Consumption and CO2 Emissions. Focus on Sustainability Targets. Paris: International Union of Railways (UIC) – International Energy Agency.
USDOT (2009). Vision for High-Speed Rail in America. High-Speed Rail Strategic Plan. The American Recovery and Reinvestment Act. Washington, DC: Department of Transportation, Federal Railroad Administration.
Waller, J. S., and Servheen, C. (2005). Effects of transportation infrastructure on grizzly bears in northwestern Montana. J. Wildl. Manage. 69, 985–1000. doi: 10.2193/0022-541X(2005)069[0985:EOTIOG]2.0.CO;2
Wells, P., Woods, J. G., Bridgewater, G., and Morrison, H. (1999). “Wildlife mortalities on railways: monitoring methods and mitigation,” in Proceedings of the Third International Conference on Wildlife Ecology and Transportation, (Missoula, MT), 85–88.
Weston, M. A., McLeod, E. M., Blumstein, D. T., and Guay, P. J. (2012). A review of flight initiation distances and their application to managing disturbance to Australian birds. Emu 112, 269–286. doi: 10.1071/MU12026
Whorff, J. S., and Griffing, L. (1992). A video recording and analysis system used to sample intertidal communities. J. Exp. Mar. Biol. 160, 1–12. doi: 10.1016/0022-0981(92)90106-K
Wiaçek, J., Polak, M., Filipiuk, M., Kucharczyk, M., and Bohatkiewicz, J. (2015). Do birds avoid railroads as has been found for roads? Environ. Manage. 56, 643–652. doi: 10.1007/s00267-015-0528-7
Williams, B. K., and Brown, E. D. (2014). Adaptive management: from more talk to real action. Environ. Manage. 53, 465–479. doi: 10.1007/s00267-013-0205-7
Wulff, S. J., Butler, M. J., and Ballard, W. B. (2016). Assessment of diurnal wind turbine collision risk for grassland birds on the Southern Great Plains. J. Fish Wildlife Manage. 7, 129–140. doi: 10.3996/042015-JFWM-031
Keywords: agrarian birds, environmental impact assessment, flight initiation distance, high-speed railway, infrastructure, monitoring, camera, road kill
Citation: García de la Morena EL, Malo JE, Hervás I, Mata C, González S, Morales R and Herranz J (2017) On-Board Video Recording Unravels Bird Behavior and Mortality Produced by High-Speed Trains. Front. Ecol. Evol. 5:117. doi: 10.3389/fevo.2017.00117
Received: 17 March 2017; Accepted: 13 September 2017;
Published: 05 October 2017.
Edited by:
Diane E. Pataki, University of Utah, United StatesReviewed by:
Iryna Dronova, University of California, Berkeley, United StatesFederico Morelli, Czech University of Life Sciences Prague, Czechia
Copyright © 2017 García de la Morena, Malo, Hervás, Mata, González, Morales and Herranz. This is an open-access article distributed under the terms of the Creative Commons Attribution License (CC BY). The use, distribution or reproduction in other forums is permitted, provided the original author(s) or licensor are credited and that the original publication in this journal is cited, in accordance with accepted academic practice. No use, distribution or reproduction is permitted which does not comply with these terms.
*Correspondence: Juan E. Malo, amUubWFsb0B1YW0uZXM=