- 1Natural Resources and Environmental Sciences, University of Illinois at Urbana-Champaign, Urbana, IL, United States
- 2Department of Botany, University of Hawai‘i at Mānoa, Honolulu, HI, United States
- 3Department of Molecular, Cellular, and Biomedical Sciences, University of New Hampshire, Durham, NH, United States
Mutualistic interactions between species are crucial for the maintenance and functioning of the community. Current research has shown the importance of not only understanding the structure of these mutualistic interaction networks but also their temporal dynamics. Temporal changes in species abundance, such as those caused by vertebrate resource tracking, may create temporal variability in a network though neighborhood effects and influence the directedness of dispersal. While much research has been done on resource tracking, neighborhood effects, and directed dispersal individually, little research has been done into their interrelationships and, therefore, theory of how resource tracking can influence frugivory and seed dispersal networks remains poorly developed. We use the available literature to show the prevalence of resource tracking by vertebrate frugivores and hypothesize how, through neighborhood effects, resource tracking may influence short-term variation in network properties. We then discuss how resource tracking can influence long-term network properties by altering the dispersal of plant species. Lastly, we use this information to hypothesize how the introductions of new species into a community may alter the influence of resource tracking on frugivory and seed dispersal. While trait matching and links that are not possible between species in a community (i.e., forbidden links) play a large role in determining the structure of a network, temporal change in the abundance of species due to resource tracking may also affect the properties of networks. By increasing our understanding of the role resource tracking has in the temporal variability of frugivory and seed dispersal networks, we can better determine the full extent of species interactions and provide valuable information for the conservation of ecological communities.
Introduction
Mutualistic relationships between plants and animals play a major role in shaping and maintaining biodiversity (Fleming and Kress, 2013). Many recent studies have highlighted the need to incorporate temporal dynamics to better understand the assembly and maintenance of plant-animal mutualisms (Yang et al., 2013). Research into the temporal dynamics of plant-animal mutualisms, however, has largely focused on the relationship between plants and pollinators (see Olesen et al., 2008; Traveset et al., 2015), with seed dispersal (SD) mutualisms receiving less attention (see Carnicer et al., 2009; González-Castro et al., 2012; Yang et al., 2013). The complexity of community-level interactions complicates our understanding of frugivore-plant mutualisms (Olesen et al., 2010). At the community-level, frugivore-plant mutualisms are often viewed as a network of interacting species, which should lead to understanding their role in the maintenance of the community. Factors that influence the properties of SD networks has been the focus of much research in the past 15 years, particularly focusing on static networks, but a complete understanding of what causes these properties to vary temporally is still the focus of debate.
Multiple hypotheses have been suggested to explain the properties of mutualistic networks, with the “forbidden links” and “abundance” hypotheses receiving considerable attention. The forbidden links hypothesis states that not all links (i.e., interactions) in a network are realized due to constraints on the formation of some links (Vázquez, 2005). Constraints on potential links within plant-animal mutualistic networks include a wide variety of plant or animal traits, including morphological mismatch (e.g., gape width and fruit/seed size in SD mutualisms) and phenological uncoupling (e.g., asynchrony between disperser abundance and fruiting times), that prevent links between certain components of a network (Vázquez et al., 2009; Dehling et al., 2014; Muñoz et al., 2017). The influence of morphological mismatch on the temporal dynamics of SD networks should be negligible because significant change in morphology of a species on ecological timescales occurs only under extreme evolutionary pressures (see Thompson, 1998; Grant and Grant, 2006; Galetti et al., 2013). However, in some cases, natural, anthropogenic, and functional extinction and/or introduction of species can create temporal changes in the network created by morphological mismatches (Culliney et al., 2012; Galetti et al., 2013). Additionally, forbidden links created by phenological mismatches could explain temporal variation in network structure (Yang et al., 2013). These forbidden links may be strongly dependent on the temporal scale of the network (e.g., comparisons among seasons may differ from comparisons among years) and capable of acting in relatively short time frames. For example, in a study of two frugivory networks in southern Spain, phenological uncoupling influenced the cross-sectional structure of the networks—disregarding temporal variation—causing 22–25% of the forbidden links (Olesen et al., 2010). In addition, in Puerto Rico, bird species that had longer active periods were able to include more plant species in their diets, while those with shorter active periods had fewer species available to them, suggesting that phenology was the primary driver of temporal network patterns (Yang et al., 2013). Indeed, forbidden links provide a potential explanation of network properties, however, due to the complexity of ecological communities, it is unlikely that forbidden links can fully explain network properties.
The abundance hypothesis states that the degree (the number of interacting species) and strength of interspecific interactions (amount of interaction such as, the number of fruits eaten) for a species may be determined by that species' abundance (Carnicer et al., 2009). Several predictions can be derived from the abundance hypothesis. One is that the effect of an abundant species on a rare partner is greater than the effect of the reciprocal (i.e., asymmetric relationship; Vázquez et al., 2007). Asymmetric relationships within mutualistic networks are common both in terms of the number of species with which an individual species interacts (i.e., specialists interact with generalists but rarely with other specialists; Bascompte et al., 2006) and the strength of the interaction (i.e., a species with a large effect on a partner experiences a weaker reciprocal effect; Bascompte et al., 2006). Another prediction arising from the abundance hypothesis is that the most abundant species have encounters with more species than rare species do (i.e., a higher degree; Vázquez et al., 2007). However, this hypothesis does not fully account for some properties observed in networks, such as, the extent that species with a lower degree have interactions with a subset of the partners of species with a high degree, referred to as nestedness. For example, relative species abundance explains only 60–70% of the nestedness of two networks for plant-frugivore communities in southern Spain, with the 30–40% unexplained nestedness suggesting that the abundance and forbidden links hypotheses (and likely more) are not mutually exclusive and may work in concert to determine network structure and variability (Krishna et al., 2008).
The forbidden links hypothesis can explain temporal variation in network structure through phenological mismatch. Phenological mismatch would cause some potential interactions between species to be impossible at certain times. For example, SD networks early in a fruiting season could be very different from those later in the season in regard to the number of interactions for certain species and, to a lesser extent, the strength of interactions (González-Castro et al., 2012). The abundance hypothesis only explains the temporal variations of mutualistic networks when there are changes in the abundance and/or identity of species within the network through time. If species presence and/or abundance varies in time, then the number and strength of interactions could also vary in time, giving rise to temporal shifts in network structure (Carnicer et al., 2009; Yang et al., 2013). This idea clearly has important implications for mutualistic networks that occur in areas where species abundances vary seasonally as due to migration, climate, and/or other life history properties of the species involved. However, temporal shifts in species abundance can also occur through foraging behaviors, such as, resource tracking, exhibited by the animals within the network (Moegenberg and Levey, 2003).
Frugivores can track fruit resources, with abundance of frugivores strongly correlating with the availability of ripe fruit, although the extent and factors regulating this tracking are varied (Saracco et al., 2004; Hampe, 2008). Resource tracking can create scenarios where local abundances of frugivores increase as certain resources increase, which may, in turn, increase the likelihood of interactions with multiple plant species in the area that are fruiting at the same time; a scenario that is predicted by the abundance hypothesis. Multiple studies have suggested that resource tracking may play a role in the temporal dynamics of SD networks (Krishna et al., 2008; Carnicer et al., 2009; Yang et al., 2013). However, the influence resource tracking has on SD networks has not been explicitly explored, and, therefore, theory of how resource tracking may influence networks is still being developed (Figure 1). Here we use the available information in the literature to explore how vertebrates in SD networks may be tracking resources. We then propose how neighborhood effects may act as mechanisms through which fluctuations in abundance from resource tracking could influence the structure and small scale temporal variation of SD networks as predicted by the abundance hypothesis. We continue this discussion to determine how resource tracking may also influence the dispersal of seeds through creating scenarios where directed dispersal may be more prevalent and, therefore, influencing the large scale dynamics of SD networks. Lastly, we then apply this information to determine how the loss and/or introduction of species may alter SD networks through changing resource tracking behaviors and/or there outcome in terms of seed dispersal.
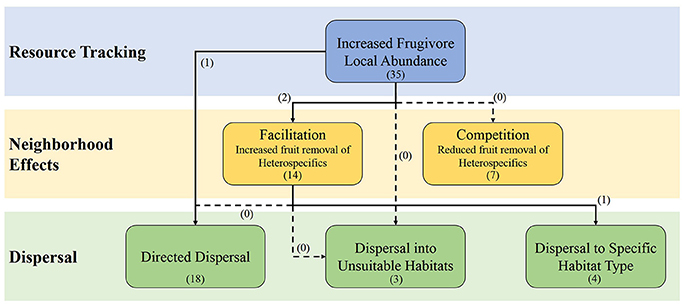
Figure 1. The influence of resource tracking on the fruit removal and potential seed dispersal through different types of neighborhood effects. The dashed arrows represent the links that where our literature search provided no studies investigating them. The numbers in parentheses represent the number of studies that investigate the process or link as determined by Web of Science topic searches (see Appendix 1 in Supplementary Materials for search terms).
Resource Tracking by Vertebrates
The temporal and spatial tracking of resources has been proposed as an explanation for the variability of animal abundance in many systems. Evidence for resource tracking has been found in both temperate (García and Ortiz-Pulido, 2004; Gleditsch and Carlo, 2010) and tropical systems (Johnson and Sherry, 2001; Moegenberg and Levey, 2003; McConkey and Drake, 2007; Blendinger et al., 2012; Heleno et al., 2013). The ubiquity of resource tracking across diverse habitats is likely due to the patchiness of resources in most ecosystems, irrespective of geographical and climatic zone. However, the extent to which resources vary in space and time can be a function of geography, climate, and the scale at which resource variation is observed (Fleming, 1992; Ting et al., 2008; Garcia et al., 2011; Côrtes and Uriarte, 2013). For example, in seasonal environments, fruits and other types of food resources have periods when they are unavailable on the landscape due to more extreme climate conditions, whereas in aseasonal environments, stable conditions result in many food types being available year-round with the relative amount of this resource varies across space and time (Jordano, 1992). However, the characteristics of the consumer can play a large role in their ability to track resources across the landscape.
Vertebrate species vary in their ability to track resources, and the factors that influence a species' ability to track resources are still largely unknown. However, tracking ability seems to be strongly related to the mode of locomotion. Species that fly, such as, birds, can track resources across broad landscapes. Levey (1988) found that frugivorous birds shift to secondary forest, where fruit is more abundant, in times of low fruit abundance in primary forests. In addition, foraging behaviors influence the daily movements and home range sizes for species and, therefore, their ability to track resources. Folivorous primates have smaller daily movements and smaller home ranges than frugivorous primates in both tropical America (Robinson and Janson, 1987) and Africa (Oates, 1987). Frugivorous birds in Panama were more likely to switch habitats than insectivorous birds (Martin and Karr, 1986), and many nectarivorous Australian honeyeaters switch habitats in response to changing flower distributions (Pyke, 1985). Tracking food resources determines much of daily and seasonal movements of frugivorous and nectarivorous birds (Karr, 1990). Together, mobility and foraging behaviors play large roles in determining a species' ability to track resources. However, the extent of resource tracking depends on the value of the resource to the consumer, availability of alternative resources, and degree of resource specialization. For example, Blendinger et al. (2015) demonstrated variation in species-specific responses to fruit abundances may be determined by factors such as, fruit nutrients and energy resources. We would expect resource tracking would be cost effective in terms of foraging efficiency for consumers.
Any resources that are commonly used by a species and vary through time and space can be tracked by animals. Food resources often vary greatly through space and time due to disturbance regimes, climatic variability, prey population fluctuations, and phenological patterns (Didham and Springate, 2003; Moegenberg and Levey, 2003). Temporal and spatial tracking of food resources has been observed in relation to animal prey (e.g., insectivores tracking prey abundance, Johnson and Sherry, 2001; Murakami, 2002; Mulwa et al., 2012), seed resources (e.g., granivores tracking seed production, Blendinger and Ojeda, 2001), and most commonly, flower and fruit resources (Moegenberg and Levey, 2003; Borghesio and Laiolo, 2004). Flower-pollinator systems, in particular, exhibit strong resource-tracking patterns due to the mutualistic relationship between plants and pollinators that can be highly specialized (Cotton, 2006). Similarly, fruit-frugivore systems can exhibit strong resource tracking since they are also largely mutualistic relationships in which the primary function of the fruit is to attract, and be eaten and dispersed by frugivores (Moegenberg and Levey, 2003). We included examples of tracked resources other than fruit in our discussion of resource tracking in part to show the commonness of resource tracking, but also because cross-resource type interactions may occur. Approximately 41% of bird families have mixed diets that include fruit to varying degrees (Jordano, 1992), which suggests that tracking food resources other than fruit can have implications that reach beyond the distribution and demographics of the consumer, since tracking a non-fruit resource may influence consumption of fruit in the area. For instance, ‘ōhi’a (Metrosideros polymorpha) flowers significantly predicted the abundance of Japanese white-eyes (Zosterops japonicus) in Hawai'i (Hart et al., 2011), where it has become an important frugivore (Foster and Robinson, 2007). However, these cross-resource type interactions have limited current data, therefore, we will focus on fruit tracking.
The diet composition of consumers also has important implications for the propensity of animals to track resources. Animals with generalized diets have the ability to switch to new food types during times when their preferred resource is limited. Diet switching may make tracking resources across the landscape or through time less necessary and, therefore, create a weak association between the abundance of resources and animals (Carnicer et al., 2009). On the other hand, dietary specialists may be constrained to track resources through space and time. Frugivores in particular often have mixed diets, with fruit as a supplemental resource to other diet items such as, arthropods (Jordano, 1992). Only 17 families of birds can be considered obligate frugivores (i.e., diet only comprising of fruit), and there are no obligate frugivores among mammals and reptiles (Jordano, 1992). Even though obligate frugivory is rare, tracking of fruit resources is exhibited by many species of birds, suggesting that dietary specialization is not needed for resource tracking to occur. Bird species with generalist diets, such as, gray catbird (Dumetella carolinensis) and song thrush (Turdus philomelos), can have strong relationships between their abundance and the abundance of common fruit species (Rey, 1995; Gleditsch and Carlo, 2010, respectively). However, bird species that rely more on fruit in their diet exhibit more fruit tracking behaviors (Blendinger et al., 2012). Variation in resource tracking over time can be related to the frugivore community and the differential ability of frugivores to switch seasonally between arthropod and fruit-based diets (Carnicer et al., 2009). When highly abundant fruit resources become available they may trigger certain species to shift their diets to include fruit, increasing the number and diversity of animals dispersing these plants. For example, two warblers (Sylvia spp.) that switch from being completely insectivorous to diets that include fruit, have strong relationships with fruit abundance when fruits become abundant on the landscape (Rey, 1995).
Frugivores exhibit resource tracking to high degree. Many studies have experimentally and correlatively shown resource tracking by frugivores (Blendinger et al., 2012 and citations within). However, some studies have found little evidence for fruit tracking (e.g., García and Ortiz-Pulido, 2004) and it has been suggested that these studies failed to find evidence for fruit tracking due to scale dependencies and broader ecological contexts (Hampe, 2008). Fruit tacking has been observed in tropical environments (Moegenberg and Levey, 2003) and in temperate environments (Rey, 1995). The prevalence of fruit tracking in the literature suggests that resource tracking by vertebrates may have implications for seed dispersal and thus properties of SD networks. We hypothesize that the mechanism through which resource tracking influences SD network properties is by altering the consumption of fruit in the local community and the deposition of seeds in certain habitats (Figure 1).
Neighborhood Effects
The aggregation and distribution of fruits in time and space can affect the number of potential dispersers in an area via resource tracking and, in turn, affect fruit removal and subsequent seed dispersal by vertebrates. In neighborhoods with many fruiting species, crop size is an important variable for predicting fruit removal—the larger the crop size the higher the fruit removal (Morales et al., 2012). There are two primary hypotheses to explain how crop size and the spatial aggregation of fruiting plants may influence fruit removal by vertebrates: the competition hypothesis and the facilitation hypothesis (Sargent, 1990). Under the competition hypothesis, high densities of fruits within a neighborhood attract dispersers; however, dispersers are a limiting resource and neighboring plants compete for their services. Alternatively, the facilitation hypothesis predicts that dispersers are not always limiting, and high fruit density in the area attracts more dispersers to the neighborhood, thereby increasing the number of total dispersal events for multiple species (Figure 1).
To date, research suggests that neighborhood effects are variable and likely species- and habitat-dependent. The competition hypothesis has been best supported within conspecific neighborhoods. Manasse and Howe (1983) found that increasing densities of conspecific fruits within a patch explained a decrease in fruit removal rates from individuals of the tropical shrub Virola surinamensis during peak fruiting seasons. In Puerto Rico, Schefflera morototoni trees also competed intraspecifically for dispersers, lowering overall visitation rates per individual (Saracco et al., 2005). The competition hypothesis has also been supported in temperate environments. Denslow (1987) found that high densities of conspecific fruits decreased the number of fruits removed per plant for the shrub Sambucus pubens.
In interspecific neighborhoods, the facilitation hypothesis has been most frequently supported; although, studies have also found evidence of competition and neutral effects (Carlo et al., 2007). When the mistletoe Phoradendron juniperinum was fruiting, removal of fruit from its host, Juniperus monosperma, was enhanced (van Ommeren and Whitham, 2002). Gleditsch and Carlo (2010) experimentally demonstrated increased fruit removal of Solanum americanum in areas that had higher shrub densities of Lonicera mackii and L. morrowii. Additionally, fruit removal of Actaea spicata was highest in sites where fruiting densities were highest, regardless of neighboring species (von Zeipel and Eriksson, 2007). The facilitation hypothesis is also one possible mechanism explaining maintenance of rare plant species in a Polish forest; rare species having greater phenological overlap with more abundant species benefit from the increase in potential dispersers attracted to a neighborhood by the high density of the fruit of the abundant species (Albrecht et al., 2015).
Both the competition and facilitation hypotheses predict that high densities of fruit attract more frugivores into an area and alter fruit removal rates from individual plants (Smith and McWilliams, 2014; Figure 1). However, differences in seed dispersal effectiveness caused by resource tracking and subsequent fruit removal can be less predictable, and can vary based on the number of frugivores and/or their foraging behavior and movement patterns in response to landscape characteristics (Aldrich and Hamrick, 1998). Generally, as fruit density and the number of potential dispersers increase, dispersal distances decrease (Carlo and Morales, 2008). However, a decrease in dispersal distance does not necessarily result in a decrease in seed dispersal effectiveness. High fruit densities of Cestrum diurnum resulted in the same quantity of S. americanum being dispersed when these fruiting plants were part of the same neighborhood; but, fewer seeds were dispersed per dispersal event, reducing possible density-dependent effects and increasing the number of sites seeds reached (Carlo, 2005). Additionally, possible facilitative neighborhood effects can cause rare species to be represented disproportionately in the seed rain to its abundance. In Puerto Rico, seed rain produced by generalist frugivores into pastures was more even across plant species than what was predicted by the abundance of plant species, which potentially maintains plant diversity and gives rise to frugivore based “rare species advantage” (Carlo and Morales, 2016). To add to this complexity, diverse frugivore characteristics such as, a range of foraging behaviors or morphologies, may influence seed dispersion (Jordano et al., 2007) causing the outcome of these neighborhood effects to depend on the characteristics of the frugivore that tracks the resource.
Resource tracking may have implications that reach beyond fruit removal to dispersal and environmental processes. Neighborhood effects likely alter the species that are effectively dispersed in a community, and thus the overall community structure. Since there is an abundance of evidence for facilitative neighborhood effects in heterospecific communities, one or many plant species near an abundant tracked resource may benefit with increased dispersal. A possible outcome of this would be directed dispersal.
Directed Dispersal
So far we have discussed how resource tracking can influence fruit removal. However, resource tracking can also influence the dispersal of seeds and plant recruitment. One way resource tracking and neighborhood effects can facilitate seed dispersal and plant recruitment is through directed dispersal. The directed dispersal hypothesis posits that natural selection will favor a dispersal interaction in which seeds are deposited into microclimates where they have a higher probability of germinating and surviving than they would in random sites (Howe and Smallwood, 1982). Successful dispersal events are important for altering local ecological characteristics of a site via changes in plant diversity (Salazar et al., 2013). In turn, these events affect the abundance of fruits within a neighborhood and the types of vertebrates within an area.
Historically, directed dispersal has been considered an uncommon process; but, this is most likely due to the inherent difficulties in studying dispersal (Wenny, 2001). To date, directed dispersal has been disproportionately studied for specialized and rare plant species (Wenny, 2001; Loayza et al., 2014). Classic examples of directed dispersal include the dispersal of mistletoes into suitable sites within its host trees and ant-plant mutualisms (Wenny, 2001; Green et al., 2008; Salazar et al., 2013). However, directed dispersal does occur in common plant species such as, the tropical tree Ocotea endresiana, where dispersal largely occurs into favorable sites for seedlings due to bird perching behavior (Wenny and Levey, 1998), or to specific advantageous growth sites when corvid bird species cache pine cones or acorns (Vander Wall, 1990).
Tropical examples of directed dispersal are becoming more frequent. Hirsch et al. (2012) found that Astrocaryum standleyanum seeds were more likely to be cached by agoutis in areas away from conspecifics, facilitating the escape from natural enemies (Janzen, 1970; Connell, 1971). While some dispersers contribute to plant fitness via directed dispersal, not all dispersers are created equal. In Madagascar, even though one lemur species was identified as directly dispersing seeds, seeds from lemur dispersers in general typically have lower recruitment levels than if dispersal were random (Razafindratsima and Dunham, 2015).
Resource tracking by vertebrates aggregates dispersers in areas where resources have established, which in turn may create seed deposition patterns that intensifies under fruiting canopies (Rodríguez-Pérez et al., 2014). Indeed, in a desert grassland and a temperate heterogeneous system seed deposition was higher under fruiting canopies (Carlo et al., 2013; Carlo and Tewksbury, 2014). Carlo et al. (2013) found that long distance dispersal events (distances of 150–700 m) were much more likely to occur under fruiting canopies than non-fruiting. Long distance dispersal events are important for plant community stability by maintaining gene flow. Additionally, Carlo and Tewksbury (2014) found that chiltepin pepper (Capscium annuum var. glabriusculum) seeds that were artificially added and arrived there via bird dispersal under dense fruiting shrubs exhibited increased recruitment, leading them to conclude that dispersal of these peppers was directed to favorable microhabitats. Therefore, resource tracking does have the potential to influence seed deposition patterns so that there is higher seed deposition under fruiting canopies potentially increasing the recruitment of plants depending on species-specific seed-seed interactions. This, in turn, can alter the long term population densities and the large scale dynamics of SD networks.
Effects of Human-Induced Rapid Environmental Change
Frugivory and seed dispersal interactions have not escaped the direct and indirect effects of human-induced rapid environmental change, and these effects can alter the influence of resource tracking on SD networks. Habitat loss, species extinctions, species introductions, and climate change are all having large impacts on frugivore communities, fruit communities, and, ultimately, seed dispersal (Fontúrbel et al., 2015). Habitat loss and fragmentation can decrease native species richness, increase the distance potential dispersers must travel between patches of fruiting plants, and decrease local fruit abundance within an area (Rey and Alcántara, 2014; Fontúrbel et al., 2015). In addition, the decline or loss of either once-abundant fruiting plant species and/or their vertebrate dispersers can drastically change the structure of a SD network and forest recruitment patterns (Meehan et al., 2002; Wotton and Kelly, 2011; Schleuning et al., 2014; Pejchar, 2015). However, understanding the detailed effects of species loss on an SD network would require an understanding of historic interactions within a community, which are often lacking (but see Pires et al., 2014). Compared to species loss, understanding the effects of species introductions on resource tracking and neighborhood effects can be studied in real time. However, more research is needed in order to understand the effect of introduced species on SD interactions in most systems.
Introduced frugivorous vertebrates and fruit bearing plants often assimilate into SD networks due to the general nature of SD interactions (Traveset and Richardson, 2006; Fleming and Kress, 2013). Indeed, native species often use introduced species for reproductive mutualisms like seed dispersal (Aslan and Rejmánek, 2010; Gleditsch and Carlo, 2010; Heleno et al., 2013). The effects of introduced species may be related to their position in the network (Figure 2). Introduced plants can become a dominant component of seed dispersal networks by creating dense patches of large, conspicuous fruit crops that are easily obtained by frugivores, making them easy to track across landscapes, and thus highly connected within the network (Traveset and Richardson, 2006). These highly-connected species act as hubs within the network, shaping frugivory and seed deposition patterns (Carlo et al., 2007). Indeed, this pattern appears to be occurring in our current study of SD networks in Hawai‘i, where plant species such as, Clidemia hirta, are dominating network interactions based on their abundance and ubiquity (Foster et al., unpubl. data). Because the fruits of introduced plants can be easily tracked, they can increase the local abundance, and potentially the diversity, of frugivores when fruiting. Since evidence suggests that neighborhood effects in interspecific neighborhoods are likely to be facilitative (Carlo et al., 2007), the introduction of plants may increase fruit removal of neighbors (Gleditsch and Carlo, 2010; Figure 2A). However, competition for dispersers can also occur (Aslan, 2011; Spotswood et al., 2012). Introduced plants can change habitat structure, thereby influencing disperser foraging behaviors (Traveset and Richardson, 2006). This can create instances in which dispersed seeds have a lower probability of escaping from the parent plant, increasing negative density-dependent effects and/or decreasing seed deposition in habitat that is suitable for germination (Lichstein et al., 2004). In addition, the tracking of an introduced fruiting plant can cause seeds of native species to be deposited under the canopies of the introduced plant where recruitment is often reduced (Traveset and Richardson, 2006; Figure 2A).
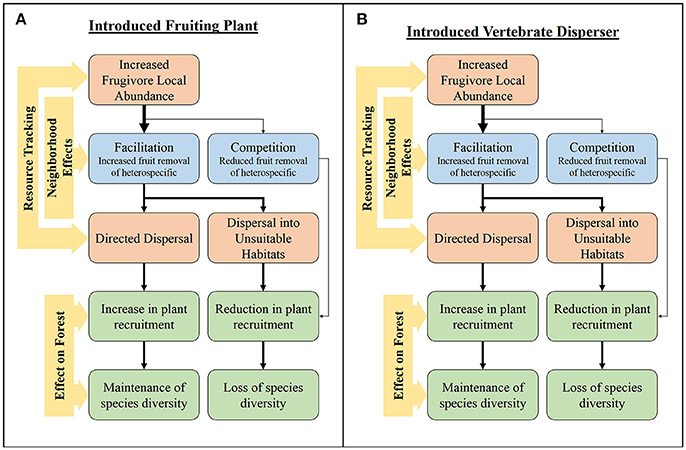
Figure 2. The influence of resource tracking on the fruit removal and potential seed dispersal through different types of neighborhood effects with the introduction of (A) a fruiting plant and (B) a frugivore.
The restructuring of habitat and the fruiting community through the loss and/or introduction of species can result in restructuring of the frugivore community (Traveset and Richardson, 2006). The resultant novel fruiting community would likely select for frugivores that are not neophobic, suggesting that they would be diet generalists. Introduced frugivores are often considered to be inferior dispersers of native plants compared to native frugivores (Chimera and Drake, 2010; Culliney et al., 2012; Spotswood et al., 2012; Wu et al., 2014). For example, the Hawaiian Islands have lost a majority of their native frugivorous birds, with only two thrush species remaining: the puaiohi (Myadestes palmeri) and the ‘ōma’o (M. obscurus; Pejchar, 2015). Thus, many native plant species must rely on introduced frugivorous birds for seed dispersal. However, the extent to which introduced birds compensate for the loss of dispersers remains unclear. Introduced species have been shown to disperse native plants, most likely due to their highly generalized diets (Foster and Robinson, 2007). However, differences in frugivore size and diet composition, suggest that introduced birds incompletely compensate for the loss of native dispersers (Chimera and Drake, 2010; Culliney et al., 2012; Wu et al., 2014). The overall effect of an introduced frugivore on the structure of a frugivory network is dependent on the species' characteristics, such as, their competitiveness, morphological attributes, and foraging behavior (Traveset and Richardson, 2006; Figure 2B). Even though introduced species are often generalists and have the capacity to switch their diets when certain resources are low, they may still track resources through space and time. This may create temporal variation in networks that could be influenced by the temporal changes in abundance of introduced frugivores due to resource tracking. Additionally, introduced frugivores often form relationships with introduced fruiting plants, furthering the creation of network hubs (Lafleur et al., 2007). Introduced species (both birds and plants) can affect the temporal dynamics of SD networks through highly connected hubs and determining the extent of how these interactions effect SD networks merits additional research.
Temporally explicit SD networks can help identify novel interactions that occur between introduced and native frugivore and plant species. These networks can be used to identify important interactions for conservation initiatives and when and where to focus conservation efforts. Additionally, continued monitoring of the networks can track success and community level impacts of conservation initiative (Kaiser-Bunbury and Blüthgen, 2015). Additionally, conservation initiatives that aim to promote rare plant species may benefit from information from temporally explicit SD networks (Carlo and Yang, 2011). If certain fruiting plant species are tracked by frugivores and act as hubs through facilitative neighborhood effects, managers can use them to promote the frugivory and seed dispersal of rare species by planting them in the vicinity of the rare species (or by planting the rare species in the vicinity of the common one). Alternatively, if abundant fruiting species that are tracked outcompete rare species for seed dispersers, then conservationists could remove the competitors and replace them with other fruiting plants that produce facilitative neighborhood effects. If combined with restoration programs, the effects of one fruiting species on the dispersal of others could be experimentally evaluated using replicated outplantings of different combinations of plants.
Conclusion
Changes in the abundance of frugivores and fruiting plants can affect the properties of SD networks (Carnicer et al., 2009; Gonzalez-Castro et al., 2015). Temporal variation in network properties, such as, momentary degree (the number of interactions a species has within a specific period) can be related to frugivore abundance when abundance varies through time (Yang et al., 2013). However, González-Castro et al. (2012) suggested that phenological matching is far more important than the abundance of species. While phenology is undoubtedly important in determining network structure, the relative weakness of species abundance in their network may be due to the fact that it was a simple, insular network (González-Castro et al., 2012). By reviewing the literature, we show that resource tracking is common and is important in determining the temporal variation in species' local abundances (see also Karr, 1990). Variation in local abundances can then influence frugivory and seed dispersal in the local fruiting community through neighborhood effects (facilitative or competitive) and directed dispersal (Figure 1). This in turn can have substantial implications for the conservation of ecosystem diversity, particularly in highly invaded regions with many endemic plant species.
Here we suggest a way in which the abundance hypothesis may apply to temporal dynamics of SD networks. The temporal variation in frugivore abundance may be largely created through resource tracking at varying scales (Carnicer et al., 2009). Through increased local abundance of frugivores, facilitative neighborhood effects can increase the probability of interaction and in turn the number of interactions for the species (both birds and plants) in the area of the tracked resource (Figure 3). Conversely, through competitive neighborhood effects, the plants in the area of the tracked resource would have a lower probability of interaction and a lower degree during the times when the tracked resource is fruiting (Figure 3). In addition to having an effect on the number of interactions for a species, the strength of interaction would also likely be influenced by resource tracking. Due to an increase in the abundance of dispersers, the strength of interactions in the area of the tracked resource would be greater than in areas without the tracked resource if facilitative neighborhood effects were present and lower for competitive neighborhood effects (Figure 3). However, predicting the influence resource tracking has on higher order network properties, like nestedness and modularity, is a little more difficult. For instance, the generalist dispersers may incorporate rare species in their diet if it is near a resource they track and the network would become more nested. This, in turn, would make the network more susceptible to extinction of the highly connected specie (Saavedra et al., 2011). On the other hand, because tracked resources can act as a hub in the network, the modularity (the level of isolation of sub networks in the overall network) of the network may increase which would decrease the nestedness of the network. Since nestedness can be reduced by higher connectance (the proportion of realized interactions) or modularity, the resulting effects on community properties is challenging to predict. Indeed, the stability-nestedness relationship of communities that has been theoretically shown (Thébault and Fontaine, 2010; Saavedra and Stouffer, 2013) has been called into question (James et al., 2012). Much uncertainty still exists in determining the relationship between network properties and the stability and resilience of communities. Even still, the ubiquity of resource tracking suggests its importance in contributing to the temporal dynamics of SD networks in many systems across the globe.
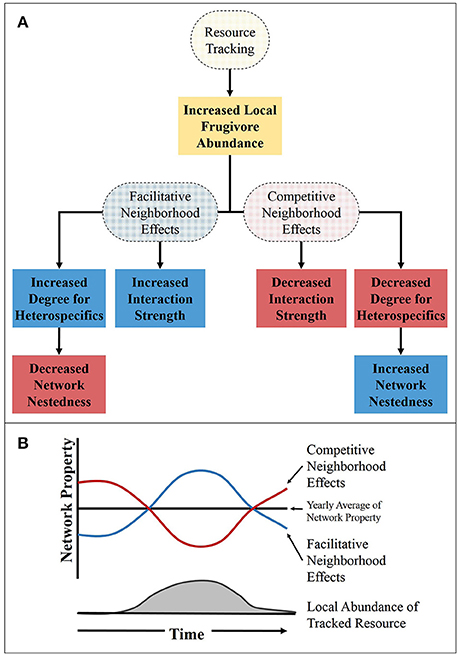
Figure 3. The influence of resource tracking on the network properties during the period of time in which the tracked resource is fruiting. (A) A flow chart showing how the resource tracking and neighborhood may influence network properties, with the dashed ovals representing processes and the boxes representing outcomes of those process. (B) The theoretical relationship between network properties (such as, momentary degree and strength) and the abundance of a tracked resource. The red and blue lines representing competitive and facilitative neighborhood effects, respectively, for momentary degree or interaction strength. For network nestedness the line colors are reversed, with the red line representing facilitative neighborhood effects and the blue line representing competitive.
Further research into the role resource tracking has in determining the short and long-term dynamics of SD networks is crucially needed. Many of the links between the different processes we have discussed have received little attention (Figure 1 and Appendix 1 in Supplementary Materials). For example, previous research into the relative contributions of the missing links and abundance hypotheses to network structure did not include resource tracking into their analyses. Resource tracking can influence the amount of support given to either hypothesis through altering the foraging behaviors and network properties at multiple scales. Simulations that alter the strength of resource tracking and the identity of the track resource and frugivore is a straightforward way of investigating the influence of resource tracking on network properties. However, field experiments can also be used to determine the role of resource tracking. The removal of a tracked resource on a large scale, though difficult to conduct, would show how network properties change without animals actively tracking the fruits. In addition, methods that involve labeling fruit around the tracked resource and combining this research with traditional SD methods will allow investigators to link the various processes in Figure 1 together and observe the relationships. DNA barcoding can be used to not only track the fate of seeds but also determine the vertebrate species that dispersed it (see González-Varo et al., 2014). Stable isotopes is a particularly appealing labeling method because with low cost they allow researchers to directly test if directed dispersal occurs, which is one of the most difficult aspects of seed dispersal to investigate, by sampling seedlings which would still contain the isotope (see Carlo et al., 2009). These methods allow researchers to link disperser behavior to the effectiveness of dispersal. Understanding the underlying causes of temporal variation within SD networks can further our comprehension of species interactions and provide valuable information for the conservation of ecological communities.
Author Contributions
JG, AH, and JF conceived the ideas and wrote the paper.
Funding
During the writing of this paper JG was supported by the department of Natural Resources and Environmental Sciences at the University of Illinois at Urbana-Champaign, AH was supported by the Department of Botany at the University of Hawai‘i at Mānoa, and JF was supported by the Department of Molecular, Cellular, and Biomedical Sciences at the University of New Hampshire. Further funding was provided by U.S. Department of Defense, Strategic Environmental Research & Development Program (SERDP) funding to JF.
Conflict of Interest Statement
The authors declare that the research was conducted in the absence of any commercial or financial relationships that could be construed as a potential conflict of interest.
Acknowledgments
We would like to thank the editors, Soumya Prasad and Tomás Carlo, for an invitation to this special issue. In addition, we would like to thank Donald R. Drake for his guidance throughout the writing of this manuscript, and Rebecca Wilcox, Jeferson Bugoni, Corey Tarwater, J. Patrick Kelley, and Jinelle Sperry for their comments the during conception of ideas and on drafts of this manuscript. We would also like to extend a thank you to the reviewers of this manuscript for giving us thorough and constructive feedback on the final drafts of this manuscript.
Supplementary Material
The Supplementary Material for this article can be found online at: http://journal.frontiersin.org/article/10.3389/fevo.2017.00098/full#supplementary-material
References
Albrecht, J., Bohle, V., Berens, D. G., Jaroszewicz, B., Selva, N., and Farwig, N. (2015). Variation in neighbourhood context shapes frugivore-mediated facilitation and competition among co-dispersed plant species. J. Ecol. 103, 526–536. doi: 10.1111/1365-2745.12375
Aldrich, P. R., and Hamrick, J. L. (1998). Reproductive dominance of pasture trees in a fragmented tropical forest mosaic. Science 281:103. doi: 10.1126/science.281.5373.103
Aslan, C. E. (2011). Implications of newly-formed seed-dispersal mutualisms between birds and introduced plants in northern California, USA. Biol. Invasions 13, 2829–2845. doi: 10.1007/s10530-011-9966-1
Aslan, C. E., and Rejmánek, M. (2010). Avian use of introduced plants: ornithologist records illuminate interspecific associations and research needs. Ecol. Appl. 20, 1005–1020. doi: 10.1890/08-2128.1
Bascompte, J., Jordano, P., and Olesen, J. M. (2006). Asymmetric coevolutionary networks facilitate biodiversity maintenance. Science 312, 431–433. doi: 10.1126/science.1123412
Blendinger, P. G., Giannini, N. P., Zampini, I. C., Ordo-ez, R., Torres, S., Sayago, J. E., et al. (2015). Nutrients in fruits as determinants of resource tracking by birds. Ibis 157, 480–495. doi: 10.1111/ibi.12274
Blendinger, P. G., and Ojeda, R. A. (2001). Seed supply as a limiting factor for granivorous bird assemblages in the Monte Desert, Argentina. Austral. Ecol. 26, 413–422. doi: 10.1046/j.1442-9993.2001.01125.x
Blendinger, P. G., Ruggera, R. A., Nú-ez Montellano, M. G., Macchi, L., Zelaya, P. V., Álvarez, M. E., et al. (2012). Fine-tuning the fruit-tracking hypothesis: spatiotemporal links between fruit availability and fruit consumption by birds in Andean mountain forests. J. Anim. Ecol. 81, 1298–1310. doi: 10.1111/j.1365-2656.2012.02011.x
Borghesio, L., and Laiolo, P. (2004). Seasonal foraging ecology in a forest avifauna of northern Kenya. J. Trop. Ecol. 20, 145–155. doi: 10.1017/S0266467403001159
Carlo, T. A. (2005). Interspecific neighbors change seed dispersal pattern of an avian-dispersed plant. Ecology 86, 2440–2449. doi: 10.1890/04-1479
Carlo, T. A., Aukema, J. E., and Morales, J. M. (2007). “Plant-frugivore interactions as spatially explicit networks: integrating frugivore foraging with fruiting plant spatial patterns,” in Seed Dispersal: Theory and Its Application in a Changing World, eds A. J. Dennis, E. W. Schupp, R. J. Green, and D. A. Westcott (Cambridge, MA: CABI Publishing), 369–390.
Carlo, T. A., García, D., Martínez, D., Gleditsch, J. M., and Morales, J. M. (2013). Where do seeds go when they go far? Integrating distance and directionality of avian seed dispersal in heterogeneous landscapes. Ecology 94, 301–307. doi: 10.1890/12-0913.1
Carlo, T. A., and Morales, J. M. (2008). Inequalities in fruit-removal and seed dispersal: consequences of bird behaviour, neighbourhood density and landscape aggregation. J. Ecol. 96, 609–618. doi: 10.1111/j.1365-2745.2008.01379.x
Carlo, T. A., and Morales, J. M. (2016). Generalist frugivores kick-start diverse tropical forest regeneration via anti-apostatic seed dispersal. Ecology 97, 1819–1831. doi: 10.1890/15-2147.1
Carlo, T. A., and Tewksbury, J. J. (2014). Directness and tempo of avian seed dispersal increases emergence of wild chiltepins in desert grasslands. J. Ecol. 102, 248–255. doi: 10.1111/1365-2745.12180
Carlo, T. A., Tewksbury, J. J., and Del Rio, C. M. (2009). A new method to track seed dispersal and recruitment using 15N isotope enrichment. Ecology 90, 3516–3525. doi: 10.1890/08-1313.1
Carlo, T. A., and Yang, S. (2011). Network models of frugivory and seed dispersal: challenges and opportunities. Acta Oecol. 37, 619–624. doi: 10.1016/j.actao.2011.08.001
Carnicer, J., Jordano, P., and Melián, C. J. (2009). The temporal dynamics of resource use by frugivorous birds: a network approach. Ecology 90, 1958–1970. doi: 10.1890/07-1939.1
Chimera, C. G., and Drake, D. R. (2010). Patterns of seed dispersal and dispersal failure in a Hawaiian dry forest having only introduced birds. Biotropica 42, 493–502. doi: 10.1111/j.1744-7429.2009.00610.x
Connell, J. H. (1971). “On the role of natural enemies in preventing competitive exclusion in some marine animals and in rain forest trees,” in Dynamics of Populations, eds P. J. Den Boer and G. R. Gradwell (Wageningen: Centre for Agricultural Publishing and Documentation), 298–330.
Côrtes, M. C., and Uriarte, M. (2013). Integrating frugivory and animal movement: a review of the evidence and implications for scaling seed dispersal. Biol. Rev. 88, 255–272. doi: 10.1111/j.1469-185X.2012.00250.x
Cotton, P. A. (2006). Seasonal resource tracking by Amazonian hummingbirds. Ibis 149, 135–142. doi: 10.1111/j.1474-919X.2006.00619.x
Culliney, S., Pejchar, L., Switzer, R., and Ruiz-Gutierrez, V. (2012). Seed dispersal by a captive corvid: the role of the 'Alalā (Corvus hawaiiensis) in shaping Hawai'i's plant communities. Ecol. Appl. 22, 1718–1732. doi: 10.1890/11-1613.1
Dehling, D. M., Töpfer, T., Schaefer, H. M., Jordano, P., Böhning-Gaese, K., and Schleuning, M. (2014). Functional relationships beyond species richness patterns: trait matching in plant-bird mutualisms across scales. Glob. Ecol. Biogeogr. 23, 1085–1093. doi: 10.1111/geb.12193
Denslow, J. S. (1987). Fruit removal rates from aggregated and isolated bushes of the red elderberry, Sambucus pubens. Can. J. Bot. 65, 1229–1235. doi: 10.1139/b87-170
Didham, R. K., and Springate, N. D. (2003). “Determinants of temporal variation in community structure,” in Arthropods of Tropical Forests: Spatio-Temporal Dynamics and Resource Use in the Canopy, eds Y. Basset, V. Novotny, S. E. Miller, and R. L. Kitching (Cambridge, UK: Cambridge University Press), 28–39.
Fleming, T. H. (1992). “How do fruit- and nectar-feeding birds and mammals track their food resources?” in Effects of Resource Distribution on Animal-Plant Interactions, eds M. D. Hunter, T. Ohgushi, and P. W. Price (San Diego, CA: Elsevier BV), 355–391.
Fleming, T. H., and Kress, W. J. (2013). “The frugivory mutualism,” in The Ornaments of Life: Coevolution and Conservation in the Tropics, eds T. H. Fleming and W. J. Kress (Chicago, IL: University of Chicago Press), 335–392.
Fontúrbel, F. E., Candia, A. B., Malebrán, J., Salazar, D. A., González-Browne, C., and Medel, R. (2015). Meta-analysis of anthropogenic habitat disturbance effects on animal-mediated seed dispersal. Glob. Change Biol. 21, 3951–3960. doi: 10.1111/gcb.13025
Foster, J. T., and Robinson, S. K. (2007). Introduced birds and the fate of Hawaiian rainforests. Conserv. Biol. 21, 1248–1257. doi: 10.1111/j.1523-1739.2007.00781.x
Galetti, M., Guevara, R., Cortes, M. C., Fadini, R., Von Matter, S., Leite, A. B., et al. (2013). Functional extinction of birds drives rapid evolutionary changes in seed size. Science 340, 1086–1090. doi: 10.1126/science.1233774
García, D., and Ortiz-Pulido, R. (2004). Patterns of resource tracking by avian frugivores at multiple spatial scales: two case studies on discordance among scales. Ecography 27, 187–196. doi: 10.1111/j.0906-7590.2004.03751.x
Garcia, D., Zamora, R., and Amico, G. C. (2011). The spatial scale of plant-animal interactions: effects of resource availability and habitat structure. Ecol. Monogr. 81, 103–121. doi: 10.1890/10-0470.1
Gleditsch, J. M., and Carlo, T. A. (2010). Fruit quantity of invasive shrubs predicts the abundance of common native avian frugivores in central Pennsylvania. Divers. Distrib. 17, 244–253. doi: 10.1111/j.1472-4642.2010.00733.x
Gonzalez-Castro, A., Yang, S., Nogales, M., and Carlo, T. A. (2015). Relative importance of phenotypic trait matching and species' abundances in determining plant-avian seed dispersal interactions in a small insular community. AoB Plants 7:plv017. doi: 10.1093/aobpla/plv017
González-Varo, J. P., Arroyo, J. M., and Jordano, P. (2014). Who dispersed the seeds? The use of DNA barcoding in frugivory and seed dispersal studies. Methods Ecol. Evol. 5, 806–814. doi: 10.1111/2041-210X.12212
González-Castro, A., Yang, S., Nogales, M., and Carlo, T. A. (2012). What determines the temporal changes of species degree and strength in an oceanic island plant-disperser network? PLoS ONE 7:e41385. doi: 10.1371/journal.pone.0041385
Grant, P. R., and Grant, B. R. (2006). Evolution of character displacement in Darwin's finches. Science 313, 224–226. doi: 10.1126/science.1128374
Green, A. K., Ward, D., and Griffiths, M. E. (2008). Directed dispersal of mistletoe (Plicosepalus acaciae) by Yellow-vented Bulbuls (Pycnonotus xanthopygos). J. Ornithol. 150, 167–173. doi: 10.1007/s10336-008-0331-9
Hampe, A. (2008). Fruit tracking, frugivore satiation, and their consequences for seed dispersal. Oecologia 156, 137–145. doi: 10.1007/s00442-008-0979-0
Hart, P. J., Woodworth, B. L., Camp, R. J., Turner, K., McClure, K., Goodall, K., et al. (2011). Temporal variation in bird and resource abundance across an elevational gradient in Hawaii. Auk 128, 113–126. doi: 10.1525/auk.2011.10031
Heleno, R. H., Olesen, J. M., Nogales, M., Vargas, P., and Traveset, A. (2013). Seed dispersal networks in the Galápagos and the consequences of alien plant invasions. Proc. R. Soc. B Biol. Sci. 280, 2012–2112. doi: 10.1098/rspb.2012.2112
Hirsch, B. T., Kays, R., Pereira, V. E., and Jansen, P. A. (2012). Directed seed dispersal towards areas with low conspecific tree density by a scatter-hoarding rodent. Ecol. Lett. 15, 1423–1429. doi: 10.1111/ele.12000
Howe, H. F., and Smallwood, J. (1982). Ecology of seed dispersal. Annu. Rev. Ecol. Syst. 13, 201–228. doi: 10.1146/annurev.es.13.110182.001221
James, A., Pitchford, J. W., and Plank, M. J. (2012). Disentangling nestedness from models of ecological complexity. Nature 487, 227–230. doi: 10.1038/nature11214
Janzen, D. H. (1970). Herbivores and the number of tree species in tropical forests. Am. Nat. 104, 501–528. doi: 10.1086/282687
Johnson, M. D., and Sherry, T. W. (2001). Effects of food availability on the distribution of migratory warblers among habitats in Jamaica. J. Anim. Ecol. 70, 546–560. doi: 10.1046/j.1365-2656.2001.00522.x
Jordano, P. (1992). “Fruits and frugivory. Seeds,” in The Ecology of Regeneration in Plant Communities, ed M. Fenner (Wallingford: CAB International), 105–156.
Jordano, P., García, C., Godoy, J. A., and García-Casta-o, J. L. (2007). Differential contribution of frugivores to complex seed dispersal patterns. Proc. Natl. Acad. Sci. U.S.A. 104, 3278–3282. doi: 10.1073/pnas.0606793104
Kaiser-Bunbury, C. N., and Blüthgen, N. (2015). Integrating network ecology with applied conservation: a synthesis and guide to implementation. AoB Plants 7:plv076. doi: 10.1093/aobpla/plv076
Karr, J. R. (1990). “Interactions between forest birds and their habitats: a comparative synthesis,” in Biogeography and Ecology of Forest Bird Communities, ed A. Keast (New York, NY: Academic Publishing), 379–386.
Krishna, A., Guimarães, P. R. Jr., Jordano, P., and Bascompte, J. (2008). A neutral-niche theory of nestedness in mutualistic networks. Oikos 117, 1609–1618. doi: 10.1111/j.1600-0706.2008.16540.x
Lafleur, N. E., Rubega, M. A., and Elphick, C. S. (2007). Invasive fruits, novel foods, and choice: an investigation of European starling and American robin frugivory. Wilson J. Ornithol. 119, 429–438. doi: 10.1676/05-115.1
Levey, D. J. (1988). Spatial and temporal variation in Costa Rican fruit and fruit-eating bird abundance. Ecol. Monogr. 58, 251–269. doi: 10.2307/1942539
Lichstein, J. W., Grau, H. R., and Aragón, R. (2004). Recruitment limitation in secondary forests dominated by an exotic tree. J. Veget. Sci. 15, 721–728. doi: 10.1111/j.1654-1103.2004.tb02314.x
Loayza, A. P., Carvajal, D. E., García-Guzmán, P., Gutierrez, J. R., and Squeo, F. A. (2014). Seed predation by rodents results in directed dispersal of viable seed fragments of an endangered desert shrub. Ecosphere 5, 43. doi: 10.1890/ES13-00283.1
Manasse, R. S., and Howe, H. F. (1983). Competition for dispersal agents among tropical trees: influences of neighbors. Oecologia 59, 185–190. doi: 10.1007/BF00378836
Martin, T. E., and Karr, J. R. (1986). Temporal dynamics of Neotropical birds with special reference to frugivores in second-growth woods. Wilson Bull. 98, 38–60.
McConkey, K. R., and Drake, D. R. (2007). Indirect evidence that flying foxes track food resources among islands in a Pacific archipelago. Biotropica 39, 436–440. doi: 10.1111/j.1744-7429.2007.00269.x
Meehan, H. J., McConkey, K. R., and Drake, D. R. (2002). Potential disruptions to seed dispersal mutualisms in Tonga, Western Polynesia. J. Biogeogr. 29, 695–712. doi: 10.1046/j.1365-2699.2002.00718.x
Moegenberg, S. M., and Levey, D. J. (2003). Do frugivores respond to fruit harvest? an experimental study of short-term responses. Ecology 84, 2600–2612. doi: 10.1890/02-0063
Morales, J. M., Rivarola, M. D., Amico, G., and Carlo, T. A. (2012). Neighborhood effects on seed dispersal by frugivores: testing theory with a mistletoe–marsupial system in Patagonia. Ecology 93, 741–748. doi: 10.1890/11-0935.1
Mulwa, R. K., Neuschulz, E. L., Böhning-Gaese, K., and Schleuning, M. (2012). Seasonal fluctuations of resource abundance and avian feeding guilds across forest-farmland boundaries in tropical Africa. Oikos 122, 524–532. doi: 10.1111/j.1600-0706.2012.20640.x
Muñoz, M. C., Schaefer, H. M., Böhning-Gaese, K., and Schleuning, M. (2017). Importance of animal and plant traits for fruit removal and seedling recruitment in a tropical forest. Oikos 126, 823–832. doi: 10.1111/oik.03547
Murakami, M. (2002). Foraging mode shifts of four insectivorous bird species under temporally varying resource distribution in a Japanese deciduous forest. Ornithol. Sci. 1, 63–69. doi: 10.2326/osj.1.63
Oates, J. F. (1987). “Food distribution and foraging behavior,” in Primate Societies, eds B. B. Smuts, Cheney D. L., R. M. Seyfarth, R. W. Wrangham, and T. T. Struhsaker (Chicago, IL: University of Chicago Press), 197–209.
Olesen, J. M., Bascompte, J., Dupont, Y. L., Elberling, H., Rasmussen, C., and Jordano, P. (2010). Missing and forbidden links in mutualistic networks. Proc. R. Soc. B Biol. Sci. 278, 725–732. doi: 10.1098/rspb.2010.1371
Olesen, J. M., Bascompte, J., Elberling, H., and Jordano, P. (2008). Temporal dynamics in a pollination network. Ecology 89, 1573–1582. doi: 10.1890/07-0451.1
Pejchar, L. (2015). Introduced birds incompletely replace seed dispersal by a native frugivore. AoB Plants 7:plv072. doi: 10.1093/aobpla/plv072
Pires, M. M., Galetti, M., Donatti, C. I., Pizo, M. A., Dirzo, R., and Guimarães, P. R. (2014). Reconstructing past ecological networks: the reconfiguration of seed-dispersal interactions after megafaunal extinction. Oecologia 175, 1247–1256. doi: 10.1007/s00442-014-2971-1
Pyke, G. H. (1985). “The relationship between abundances of honeyeaters and their food sources in open forest areas near Sydney,” in Birds of Eucalypt Forest and Woodland: Ecology, Conservation, Management, eds A. Keast, H. F. Recher, H. Ford, and D. Saunders (Sydney: Surrey Beatty & Sons), 65–77.
Razafindratsima, O. H., and Dunham, A. E. (2015). Assessing the impacts of nonrandom seed dispersal by multiple frugivore partners on plant recruitment. Ecology 96, 24–30. doi: 10.1890/14-0684.1
Rey, P. J. (1995). Spatio-temporal variation in fruit and frugivorous bird abundance in olive orchards. Ecology 76, 1625–1635. doi: 10.2307/1938163
Rey, P. J., and Alcántara, J. M. (2014). Effects of habitat alteration on the effectiveness of plant-avian seed dispersal mutualisms: consequences for plant regeneration. Perspect. Plant Ecol. Evol. Syst. 16, 21–31. doi: 10.1016/j.ppees.2013.11.001
Robinson, J. G., and Janson, C. H. (1987). “Capuchins, squirrel monkeys, and atelines: socioecological convergence with Old World monkeys,” in Primate Societies, eds B. B. Smuts, Cheney D. L., R. M. Seyfarth, R. W. Wrangham, and T. T. Struhsaker (Chicago, IL: University of Chicago Press), 197–209.
Rodríguez-Pérez, J., García, D., and Martínez, D. (2014). Spatial networks of fleshy-fruited trees drive the flow of avian seed dispersal through a landscape. Funct. Ecol. 28, 990–998. doi: 10.1111/1365-2435.12276
Saavedra, S., and Stouffer, D. B. (2013). “Disentangling nestedness” disentangled. Nature 500, E1–E2. doi: 10.1038/nature12380
Saavedra, S., Stouffer, D. B., Uzzi, B., and Bascompte, J. (2011). Strong contributors to network persistence are the most vulnerable to extinction. Nature 478, 233–235. doi: 10.1038/nature10433
Salazar, D., Kelm, D. H., and Marquis, R. J. (2013). Directed seed dispersal of Piper by Carollia perspicillata and its effect on understory plant diversity and folivory. Ecology 94, 2444–2453. doi: 10.1890/12-1172.1
Saracco, J. F., Collazo, J. A., and Groom, M. J. (2004). How do frugivores track resources? Insights from spatial analyses of bird foraging in a tropical forest. Oecologia 139, 235–245. doi: 10.1007/s00442-004-1493-7
Saracco, J. F., Collazo, J. A., Groom, M. J., and Carlo, T. (2005). Crop size and fruit neighborhood effects on bird visitation to fruiting Schefflera morototoni trees in puerto rico. Biotropica 37, 81–87. doi: 10.1111/j.1744-7429.2005.04040.x
Sargent, S. (1990). Neighborhood effects on fruit removal by birds: a field experiment with Viburnum dentatum (Caprifoliaceae). Ecology 71, 1289–1298. doi: 10.2307/1938266
Schleuning, M., Böhning-Gaese, K., Dehling, D. M., and Burns, K. C. (2014). At a loss for birds: insularity increases asymmetry in seed-dispersal networks. Glob. Ecol. Biogeogr. 23, 385–394. doi: 10.1111/geb.12134
Smith, A. D., and McWilliams, S. R. (2014). Fruit removal rate depends on neighborhood fruit density, frugivore abundance, and spatial context. Oecologia 174, 931–942. doi: 10.1007/s00442-013-2834-1
Spotswood, E. N., Meyer, J.-Y., and Bartolome, J. W. (2012). An invasive tree alters the structure of seed dispersal networks between birds and plants in French Polynesia. J. Biogeogr. 39, 2007–2020. doi: 10.1111/j.1365-2699.2012.02688.x
Thébault, E., and Fontaine, C. (2010). Stability of ecological communities and the architecture of mutualistic and trophic networks. Science 329, 853–856. doi: 10.1126/science.1188321
Thompson, J. N. (1998). Rapid evolution as an ecological process. Trends Ecol. Evol. 13, 329–332. doi: 10.1016/S0169-5347(98)01378-0
Ting, S., Hartley, S., and Burns, K. C. (2008). Global patterns in fruiting seasons. Glob. Ecol. Biogeogr. 17, 648–657. doi: 10.1111/j.1466-8238.2008.00408.x
Traveset, A., Chamorro, S., Olesen, J. M., and Heleno, R. (2015). Space, time and aliens: charting the dynamic structure of Galápagos pollination networks. AoB Plants 7:plv068. doi: 10.1093/aobpla/plv068
Traveset, A., and Richardson, D. (2006). Biological invasions as disruptors of plant reproductive mutualisms. Trends Ecol. Evol. 21, 208–216. doi: 10.1016/j.tree.2006.01.006
van Ommeren, R. J., and Whitham, T. G. (2002). Changes in interactions between juniper and mistletoe mediated by shared avian frugivores: parasitism to potential mutualism. Oecologia 130, 281–288. doi: 10.1007/s004420100792
Vázquez, D. P. (2005). Degree distribution in plant-animal mutualistic networks: forbidden links or random interactions? Oikos 108, 421–426. doi: 10.1111/j.0030-1299.2005.13619.x
Vázquez, D. P., Blüthgen, N., Cagnolo, L., and Chacoff, N. P. (2009). Uniting pattern and process in plant–animal mutualistic networks: a review. Ann. Bot. 103, 1445–1457. doi: 10.1093/aob/mcp057
Vázquez, D. P., Melián, C. J., Williams, N. M., Blüthgen, N., Krasnov, B. R., and Poulin, R. (2007). Species abundance and asymmetric interaction strength in ecological networks. Oikos 116, 1120–1127. doi: 10.1111/j.0030-1299.2007.15828.x
von Zeipel, H., and Eriksson, O. (2007). Fruit removal in the forest herb Actaea spicata depends on local context of fruits sharing the same dispersers. Int. J. Plant Sci. 168, 855–860. doi: 10.1086/518255
Wenny, D. G. (2001). Advantages of seed dispersal: A re-evaluation of directed dispersal. Evol. Ecol. Res. 3, 51–74.
Wenny, D. G., and Levey, D. J. (1998). Directed seed dispersal by bellbirds in a tropical cloud forest. Proc. Natl. Acad. Sci. U.S.A. 95, 6204–6207. doi: 10.1073/pnas.95.11.6204
Wotton, D. M., and Kelly, D. (2011). Frugivore loss limits recruitment of large-seeded trees. Proc. R. Soc. B Biol. Sci. 278, 3345–3354. doi: 10.1098/rspb.2011.0185
Wu, J. X., Delparte, D. M., and Hart, P. J. (2014). Movement patterns of a native and non-native frugivore in Hawaii and implications for seed dispersal. Biotropica 46, 175–182. doi: 10.1111/btp.12087
Keywords: seed dispersal, frugivory, resource tracking, neighborhood effects, temporal variation, networks
Citation: Gleditsch JM, Hruska AM and Foster JT (2017) Connecting Resource Tracking by Frugivores to Temporal Variation in Seed Dispersal Networks. Front. Ecol. Evol. 5:98. doi: 10.3389/fevo.2017.00098
Received: 02 February 2017; Accepted: 03 August 2017;
Published: 22 August 2017.
Edited by:
Tomás A. Carlo, Pennsylvania State University, United StatesReviewed by:
Pedro G. Blendinger, Consejo Nacional de Investigaciones Científicas y Técnicas (CONICET), ArgentinaMarcia Carolina Munoz Neyra, Senckenberg Biodiversity and Climate Research Centre (BiK-F), Germany
Copyright © 2017 Gleditsch, Hruska and Foster. This is an open-access article distributed under the terms of the Creative Commons Attribution License (CC BY). The use, distribution or reproduction in other forums is permitted, provided the original author(s) or licensor are credited and that the original publication in this journal is cited, in accordance with accepted academic practice. No use, distribution or reproduction is permitted which does not comply with these terms.
*Correspondence: Jason M. Gleditsch, Z2xlZGl0czJAaWxsaW5vaXMuZWR1