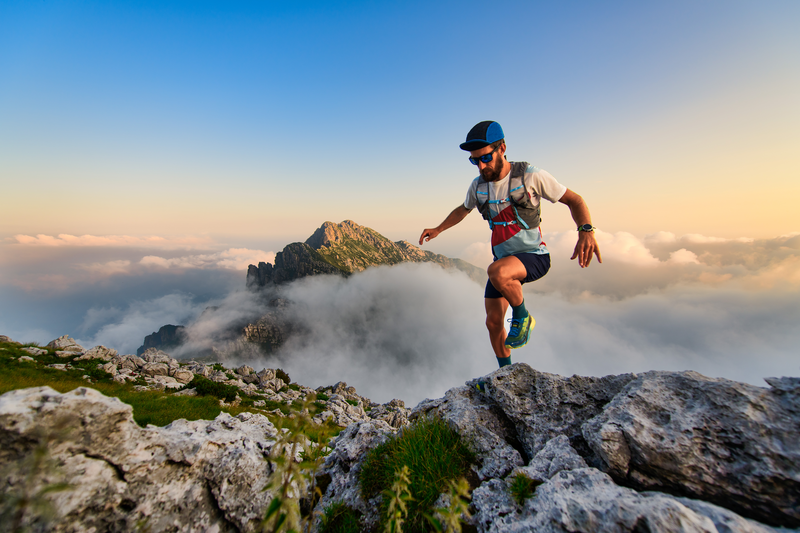
94% of researchers rate our articles as excellent or good
Learn more about the work of our research integrity team to safeguard the quality of each article we publish.
Find out more
REVIEW article
Front. Ecol. Evol. , 01 February 2017
Sec. Evolutionary Developmental Biology
Volume 5 - 2017 | https://doi.org/10.3389/fevo.2017.00003
Since ecomorphologists have started to use explicit and taxonomically-broad frameworks in studies on the relationships between form, behavior, ecology and phylogeny they have consistently reported—often against their expectations—(1) that phylogeny is usually a better predictor of anatomy than ecology is, and (2) many cases of etho-eco-morphological mismatches. It is puzzling that such mismatches occur frequently in an evolutionary process that often leads to macroevolutionary trends and in which organisms are said to be optimally/almost optimally “designed” for the habitats they inhabit. Organic Nonoptimal Constrained Evolution (ONCE), a new perspective on biological evolution that is proposed here, addresses this apparent paradox, based on an extensive compilation of empirical data and broader evolutionary ideas, from Aristotle to current Evo-Devo. According to ONCE, by taking behavioral choices, and subsequently due to their behavioral persistence related to behavioral/ecological inheritance, organisms as diverse as bacteria, plants and animals help to construct their own niches and are thus the central, active players in their evolutionary history. Darwinian (external) natural selection thus plays mainly a secondary - but still crucial - role in biological evolution, for instance helping to direct major evolutionary trends by selecting those random mutations that are advantageous within the context of the new, constructed niches. The highly constrained character of organic evolution, including developmental constraints as well as the crucial role played by behavioral persistence, can dramatically limit the occurrence of new behavioral shifts and thus the responses to external (e.g., environmental) changes, often resulting in etho-eco-morphological mismatches and eventually in evolutionary dead-ends that may lead to extinction.
In the last few years, numerous papers—most written by ecomorphologists to identify correlations between ecology and anatomy—consistently reveal eco-morphological mismatches in which form is, for instance, much more strongly related to phylogeny than to the current ecological habitats. The common occurrence of such mismatches was not at all expected in the light of the works of many Neo-Darwinists—particularly those subscribing to an adaptationist framework—and, for that matter, even of Darwin himself, who famously stressed how the morphology of the Galapagos finches seems to be beautifully optimized for the specific habitats in which they live. If natural selection by the external environment were almost always the key force in evolution, one would not expect the frequent occurrence of etho-ecological mismatches and/or eco-morphological mismatches. In contrast, the more important the role played by internal factors—including both internal constraints/selection and the behavioral choices made by organisms themselves—the more one would expect such mismatches to occur.
The occurrence of such mismatches goes against many of the ideas traditionally followed within the adaptationist program, including works by many functional morphologists and ecomorphologists that try to establish a “functional utility” for every single morphological and behavioral trait of every single organism. In fact, the terms “adaptation” and “adaptationism” continue to be mainly associated with the Neo-Darwinian view of evolution, which focused in great part on the fit between adult form and function. As recognized by Olson (2012, p. 283): “one of the most notorious aspects of the modern synthesis has been its elision of development, treating it as a trivial, more or less deterministic black box between the genome and the phenotype.” Olson also recognized that within this context, adaptationism was mainly a synonym of externalism, with natural selection, and in particular the external environment, playing a central role in morphological macroevolution, although Neo-Darwinists also stressed the importance of other factors in evolution, e.g., genetic drift and gene flow. As stated in Futuyma's book “Evolution” (Futuyma, 2013), natural selection has historically been seen as the only mechanism known to cause the evolution of adaptations, i.e., of the processes in which the members of a population become “better” suited to some features of their environment through changes in characteristics that affect their survival or reproduction in that environment. Many ecomorphologists have been, and in a way continue to be, inspired by this view, being interested in the links between morphological adaptations seen in adults and the ecology/external environment they occupy (e.g., Lloyd, 2015). However, as will be discussed in the Sections below, this is seemingly starting to slowly change with the use of explicit, broad phylogenetic methodologies and the inclusion of large taxonomical samples in ecomorphological studies in the last years.
The notion of “struggle” for existence and of optimality dates far back in time, including Aristotle, who famously stated that nature “does nothing in vain” (Leroi, 2014). In general, Baldwin, Wallace, Neo-Darwinists, and Lamarckians also emphasized the striking “fit” (match) between the phenotype of organisms and the external environment in which they live (e.g., Darwin, 1859; Darwin and Darwin, 1880; Baldwin, 1895, 1896a,b,c; Gould, 2002; Depew, 2003; Downes, 2003; Griffiths, 2003; Hall, 2003; Weber and Depew, 2003; West-Eberhard, 2003; Westneat and Fox, 2010; Young, 2013). Of course, authors such as Darwin, Wallace, Lamarck and Baldwin knew about nonsurvival of organisms and about known cases of mass extinctions, but mostly these were associated with phenomena such as relatively rapid and/or severe changes of the external—biotic or abiotic—environment. Moreover, they did not really explore in-depth the many examples of lineages, including those that are seemingly very “successful” in terms of taxonomic diversity, that are far from being optimal eco-morphological matches. The main reason for this oversight is that very few studies had focused on investigating and/or testing the frequency of such examples, because there was a strong historical bias toward a compelling adaptationist story of how each specific feature of each taxon conferred an advantage within the environments inhabited by the members of that taxon (e.g., Gould, 2002; Olson and Arroyo-Santos, 2015).
Moreover, this general bias was often related to and/or further influenced by teleological ideas about “progress” or “purpose” in evolution, e.g., toward an increase in “perfection” of the fit between the “design” of organisms and their environments (e.g., Bonner, 1988, 2013; McShea, 1991, 1996, 2012; Ruse, 1996, 2003, 2013; Turner, 2000, 2007, 2013, 2016; Rosslenbroich, 2006; Omland et al., 2008; Reiss, 2009; McShea and Brandon, 2010; Corning, 2013; Diogo et al., 2015c). Gould (2002) was particularly vocal about the occurrence of mismatches in living organisms, as he used them to emphasize the point that organisms are not designed by a supernatural entity but instead are the result of a complex, constrained, contingent and also random evolutionary history. His books provide numerous emblematic examples of such mismatches. Recently, my colleagues and I have focused on examples that relate directly to the anatomy of our own species, Homo sapiens (Diogo et al., 2015a,b, 2016; Diogo and Wood, 2016; Diogo and Molnar, 2016). Many other authors have also called attention to mismatches between some of the crucial functions of our internal organs, such as the heart, and our physiology. For instance, after providing various such examples, Noble (2006, p. 111) wrote: “now, by contrast, we can see that life is full of design faults, false trails, and imperfect compromise; we can still wonder at the intricate beauty of life on earth, but we no longer think that its logic is the best there could be.” Similarly, Lindholm's (2015) recently reviewed various illustrative cases of etho-ecological mismatches in other organisms, which he designated “maladaptive behavioral syndromes” because organisms maintain sub-optimal behaviors despite significant costs. These included, among others, high activity levels despite presence of predators, as in African springboks, newts opposing predatory fish, lemmings in northern alpine habitats, and exaggerated sexual cannibalism in certain spider species.
Wiens et al. (2011, pp. 2082–2083) provide an insightful empirical study that illustrates how sexual selection can lead to both long-term macroevolutionary trends and etho-ecological and/or eco-morphological mismatches. They used a phylogenetic approach to analyze the evolution of dorsal crests in European newts, a well-known sexually selected character system that was first noted by Darwin. Their phylogenetic results show a general relationship between the evolution of some male display behaviors and the evolution of crests, as well as that the accumulation of novel elements of the behavioral displays is related to the accumulation of modifications of the crests. They argue that the correlated addition of novel elements to both the morphological and behavioral displays might be seen as a trend toward increasing complexity in both signal types. Their results also suggest that phenotypically plastic traits such as the crests can be maintained for relatively long macroevolutionary timescales, i.e., for dozens of millions of years. Moreover, their results also show that while in some cases of long-term trends the plasticity seems to be lost, and both the behavior (via behavioral persistence) and form persist, in other cases the plasticity is not decreased. For instance, there is still enough plasticity for either behavioral shifts or morphological shifts (leading to etho-morphological and thus likely to etho-ecological and/or eco-morphological mismatches). The behavior might change while the form does not; females may no longer prefer males with crests, but crests continue to be present. Or form can change but the behavior not; females may continue to prefer males with crests, but for some reason crests are lost.
A widely discussed and beautifully illustrated example of a macroevolutionary trend that can be linked to etho-eco-morphological mismatches is given in Figure 1. Osborn (1929) reconstructed the skulls of different titanothere fossil taxa ranging from about 55–35 million years ago and argued that the evolutionary changes in the size of their horns were not random. According to him, they were instead biased in the direction of increasing horn size, because various different titanothere lineages experienced the same type of change in horn size. Osborn, a Neo-Lamarckian, reasoned that the horns were increasingly useful for fighting, but that the initial bumps were quite useless, so such a directional evolutionary change could not have been the result of natural selection (Beatty, 2008). That is, this directional change must have been due to some direction in the process of variation itself, which guaranteed the same evolutionary outcome time after time, although Osborn was silent on the process by which variation could be directed, stating that it might possibly even be “beyond human solution” (Beatty, 2008). Neo-Darwinists therefore used this example to criticize such Neo-Lamarckians, arguing that they were coming back to old vitalist ideas. They argued, for instance, that in this case even the most rudimentary of horns would have been better than nothing for fighting, so that the directionality in question might have been simply the result of natural selection of chance variations (Beatty, 2008).
Figure 1. From Osborn's 1929 monograph “The titanotheres of ancient Wyoming, Dakota, and Nebraska” (modified from Osborn, 1929). (A–H) (phylogenetically basal to derived), reconstructions of the titanothere genera Eotitanops, Manteoceras, Protitanotherium, and Brontotherium, which range from about 55 MYA (A,H), to 35 MYA (D,E).
Regarding the trend itself, many Neo-Darwinians argued that it could be simply a by-product of selection for increasing body size and/or the result of selection on horn size directly (e.g., by selecting males that tend to win fights, or by sexual selection by the females) (e.g., Hall, 2002; Beatty, 2008). One can see how these discussions frequently lead to broader evolutionary and philosophical discussions on teleology and purpose. In fact, Osborn's idea—so called “orthogenesis”—could relate evolution to a notion of design/purpose, as he saw progress in the evolutionary history of organisms toward an increasing perfection of form, function and beauty (Ruse, 1996). Therefore, Osborn's position on these issues was paradoxical: on the one hand he argued in favor of progress toward increasing perfection of form and function (eco-morphological match), but on the other hand he used titanothere nasal horns as an example of how evolutionary change can cease and stasis can prevail, because titanotheres may have evolved well beyond their adaptive optimum (eco-morphological mismatch). That is, the horns might have evolved to such a degree that they interfered with adaptation itself (Osborn, 1929; Hall, 2002; Beatty, 2008). It is clear that titanotheres did not reach orthogenetic “perfection,” in an evolutionary context, as they all became extinct (Osborn, 1929).
In such cases extinction is therefore probably associated with loss of plasticity and with stasis, as Osborn contended, although it is difficult to imagine that the existence of the horns themselves could lead to the extinction of the whole species by negative selection. As noted by Eldredge (2014, p. 172), one should be skeptical about such simplistic hypotheses. For instance, it was long thought that the “Irish elk” (a giant deer) evolved antlers so gigantic that they contributed directly to the demise of the species, but it now seems clear that the species actually became extinct along with an impressive and diverse array of other species living in all sorts of different settings. Be that as it may, the persistence of innovative features such as titanotheres' horns or the so-called panda's thumb that were advantageous within the context of, and subsequently to, the behavioral shifts/choices that the organisms made—e.g., fighting between males or females preferring males with big horns in titanotheres, eating bamboo in pandas—is very likely driven by behavioral persistence, possibly combined with phenomena such as genetic drift.
That is, the very instigators of evolutionary trends that first led to an increase in etho-eco-morphological matching—behavioral shifts/choices followed by persistence—are probably the major culprits of later etho-eco-morphological mismatches, together with internal factors. Due to the loss of the initial behavioral plasticity that allowed the animals to adopt the new behavior, the persistence of that behavior—and thus of the epigenetic/genetic anatomical features related to it—might become counterproductive when the external environment is changed, as is the case of pandas and the lack of available bamboo. At one moment in time, the ancestors of pandas did not eat bamboo, but then were able to perform a behavioral shift in order to eat it successfully. But, for some reason, extant pandas do no longer seem to have the ability to perform a new major behavioral shift/choice to substantially decrease their consumption of bamboo. In some cases, lack of behavioral plasticity may in turn depend reciprocally on the presence of, e.g., anatomical and/or genetic features that were selected within the context of the behavior acquired during the initial behavioral shift. For instance, the overspecialization of the “panda's thumb” might not allow pandas to revert to the more generalized type of diet that their ancestors had. Apart from natural selection (i.e. after the pandas started to mainly eat bamboo those random mutations that happened to be advantageous within that niche were selected), the lack of plasticity could also be enhanced by phenomena such as genetic drift, i.e., to a random sampling of those organisms that had those genetic features.
The ideas presented in the paragraphs above are supported by various types of empirical data presented in a well-documented review done by Morris (2014). For instance, benthic and limnetic three-spined sticklebacks are relatively nonplastic in the anatomical features that distinguish them. However, they occupy opposite ends of the morphological reaction norm—the range of forms that organisms with a single genotype can display across different environments and developmental conditions—of ancestral marine sticklebacks. This suggests that ancestral plasticity was required for this species pair to occupy their respective niches. That is, after the behavioral split (benthic vs. limnetic), the plasticity became decreased within the evolution of each group. Also, studies have shown that phenotypic change is greater in populations experiencing anthropogenic disturbance than in those that are not, mainly through plastic responses that may be adaptive. In other words, contrary to the case of pandas, some groups under stress still retain enough plasticity to change their behavior and related phenotype when that behavior puts them at risk within the context of anthropogenic disturbance.
Hone and Benton (2005) provided further examples linking the occurrence of evolutionary trends and of developmental constraints, which can further be linked to the incidence of eco-morphological mismatches and ultimately of extinctions. Specifically, Cope's rule refers to a tendency for organisms to increase in size over time, although this tendency is probably exaggerated in the literature due to the bias of biologists, including paleontologists, to provide narratives of “trends” and “progress,” as noted by Gould. But Cope's rule does seem to apply in at least some taxa, and according to Hone and Benton (2005) probably also occurred in other taxa in which it cannot be clearly seen. This is because mass extinctions often cap size increases, as organisms that are too large could be more vulnerable to environmental crises. In fact, in some cases the evolutionary trends toward size increase can lead to gigantism, and thus to overspecialized organisms that have difficulty subsisting, particularly with changes in the external environment. This stresses a major point noted above: evolutionary trends, often driven by behavioral shifts/persistence and then secondarily directed by natural selection, can often lead to overspecialization and loss of plasticity, and thus to eco-morphological mismatches and evolutionary dead ends, and potentially to extinction.
For example, our close relative, Gigantopithecus, is an extinct Asian ape that probably lived from nine million years to one hundred thousand years ago and could reach up to 3 m and 540 kg. Very likely, it could not cope with the changes during the Pleistocene era from forest to savanna that led to a decrease of its main food supply—e.g., fruits—and an increase in foods such as grass, roots and leaves that were dominant in the savanna and that it did not eat. According to Bocherens et al.'s (2016) recent study, even when open savannah environments were present in the landscape, Gigantopithecus foraging was limited to forested habitats. Therefore, the very large size of Gigantopithecus, combined with a relatively restricted dietary niche due to behavioral persistence, may explain its demise during the drastic forest reduction that characterized the glacial periods in Southeast Asia. On the other hand, as Hone and Benton (2005) pointed out, internal constraints also probably play a major role, in many cases, in preventing an increase in size toward phenomena such as gigantism, stressing another major point: the highly constrained nature of evolution.
A further, and particularly emblematic, example concerns the famous case of the peacock's tail that made Darwin particularly “sick” because it did not fit into the context of its external natural selection, as noted by West-Eberhard (2014, p. 502). This case illustrates well the difference between what Darwin considered to be (external) natural selection vs. phenomena such as sexual selection. That is, if the elaborate tail—which is mainly the product of sexual, and thus organic (sensu Baldwin), selection—made the male peacocks more vulnerable to a certain (external) predator, the negative selective force of natural selection would be opposed to the positive selective force of organic selection. There are many examples of such cases, and they often lead to etho-ecological and/or eco-morphological mismatches. However, in numerous other cases, natural selection secondarily reinforces the changes first driven by sexual and thus organic selection, often leading to directional evolution and eventually to long-term macroevolutionary trends. These trends can, in turn, later lead to cases of mismatch due to overspecialization and/or a change in the (external) natural selective pressures (see section above).
As helpful as such examples can be in stressing the importance of evolutionary and developmental constraints and our descent from other types of animals—and thus opposing views such as those defended by creationists—I will use different examples below when referring to eco-morphological mismatches. This is because the examples given by Gould, by me, and by the other authors mentioned just above mainly refer to single species. So, one could surely oppose these examples with an impressive list of “one-species” cases to argue that there is a beautiful, remarkable match between the form of the organisms of that species and the habitat where they live. Such cases have been historically emphasized over and over again since Aristotle (Leroi, 2014), and they were in fact the ones to which humans, who often want to find “positive narratives” as noted by Gould, paid more attention. Thence the teleological notions of “design” and “purpose” that have prevailed for a long time, and which not even Darwin could escape due to the philosophical context and historical constraints of his epoch.
However, as my close colleague and friend Virginia Abdala—who actually defines herself as an ecomorphologist—told me one day, the million-dollar question is: to which specific environments and/or behaviors are we referring to? During a single day a squirrel makes behavioral choices as diverse as climbing the main trunk of a tree, delicately moving within the thin high branches of that three, jumping from tree to tree, and running on the ground. Just outside my window right now, they also need to escape from cars when crossing the road, avoid being bitten by dogs, and so on. To which of these behaviors, and to which specific environment, is the form of squirrels “suited”? Is the morphology of the legs particularly “adapted” to run, to climb trees, or to jump between trees? That is why the adaptationist framework is, most of all, based on a profound simplification and trivialization of life: life is much more complex, fascinating and puzzling than that.
The main ideas of ONCE, the vast amount of multidisciplinary empirical data that strongly support these ideas, and the main differences between them and previous and current views proposed by other authors, have been described in detail in Diogo (in review), so readers interested in more details should refer to that paper. Here, I will just summarize those main ideas, as they are crucial for the discussions about the empirical ecomorphological data that will be presented in the next section and about their broader evolutionary and biological implications.
The main ideas of ONCE are shown in the scheme of Figure 2, which shows (in red) the major differences with a view of evolution that is currently defended by many authors within the field of Evo-Devo: the Extended Evolutionary Synthesis. ONCE is a new way of seeing biological evolution that is based on a multidisciplinary attempt to bridge the gap between internalists and externalists, Neo-Darwinists and Neo-Lamarckists, the ideas of authors such as Baldwin, Waddington and Goldschmidt, and current Evo-Devo thought including the physicalist, epigenetic plasticity and niche construction frameworks. In particular, ONCE aims to contribute to the explanation of an apparent paradox that has unfortunately not been often discussed in the literature: that eco-morphological mismatches commonly occur in an evolutionary process that often leads to macroevolutionary trends and in which organisms are supposedly “optimally,” or almost “optimally” “designed” for their habitats. In ONCE, the explanation of this paradox is deeply related to the crucial role played by behavioral persistence linked to behavioral/ecological inheritance. That is, organisms as diverse as bacteria, plants and animals help to construct their own niches and are thus the central, active players in their evolutionary history, as proposed in Baldwin's idea of Organic Selection. Darwinian natural selection then comes into play as a secondary, but crucial, player. That is, due to organismal behavioral persistence, the random mutations/epigenetic factors that happen to be advantageous within those constructed niches will be selected by the external environment, further directing evolution and increasing the match between behavior, phenotype, and that environment.
Figure 2. The structure of the Organic Nonoptimal Constrained Evolution (ONCE) idea; terms and arrows shown in red are those emphasized by ONCE but not in the Extended Evolutionary Synthesis (EES) scheme provided in Laland et al. (2015), shown in Figure 3. Following the style of the Laland et al.'s EES scheme shown in Figure 3, arrows represent causal influences (see caption of Figure 3 below for more details). Within the context of the present paper, the most crucial differences between ONCE and EES, emphasized in a central position of the scheme of this Figure, with larger fonts, is the fact that in ONCE organisms, and in particular their behavior (organismal behavior) are seen as the key active players of biological evolution, with Darwinian (external) natural selection playing mainly a secondary—but still crucial—evolutionary role. This “organic” (the “O” of ONCE; based on Baldwin's notion of “Organic selection”) view of evolution, together with the “nonoptimal,” “nonstruggling” view of evolution also defended in ONCE (its “N”), enables a natural, rational, nonteleological and nonvitalistic explanation for the common occurrence of long-term evolutionary trends, as well as of etho-ecological, etho-morphological and eco-morphological mismatches (for more details, see text and Diogo, in review).
One of the main differences between ONCE (Figure 2) and the Extended Evolutionary Synthesis (EES) recently proposed by authors such Laland et al. (2015) (Figure 3) is therefore that in ONCE organisms themselves, as a whole, and in particular their behavioral choices and persistence, are the key drivers of evolution, as noted above. Second among the differences between ONCE and the EES is that, in the scheme of Figure 3 and most publications about that synthesis, there is no special focus on long-term macroevolutionary trends, and even less on eco-morphological mismatches. In fact, apart from the recognition of strong developmental constraints and some randomness in, e.g., drift and possibly in gene flow, in a way that synthesis would predict a general tendency for organisms to display eco-morphological matches. This is because it proposes a direct link between environmental changes and developmental processes and gene expression and so on (Figure 3). These later aspects are also part of ONCE. However, ONCE focuses more on the importance of behavioral persistence as a main driver of evolution, and also attributes a more crucial role to constraints (Figure 2). Moreover, ONCE also emphasizes the important role of randomness—incorporating Gould's (e.g., 2002) thoughts on the subject -, which, together with all the other items mentioned above, can lead to a more comprehensive explanation of the frequent occurrence of both ecomorphological mismatches and macroevolutionary trends (Figure 2) than the EES does (Figure 3). This role includes phenomena such as drift/gene flow in particular, but also takes into account the particularly crucial role that behavioral choices play in ONCE. That is, even within the same external environment and in response to the same factor, organisms can make an impressive number of different behavioral choices. Therefore, they might not always make the theoretically most “logical” choice for the habitat in which they live, potentially resulting in etho-ecological mismatches.
Figure 3. The structure of the Extended Evolutionary Synthesis (EES) according to, and modified from, Laland et al. (2015), with arrows representing causal influences and processes shown in red being those emphasized by the EES but not by a more traditional Neo-Darwinist perspective. EED includes as evolutionary causes processes that create new variants, bias selection, change the frequency of heritable variation and contribute to inheritance. A diversity of ontogenetic processes (e.g., epigenetic effects, regulation of gene expression, construction of internal, and external developmental environments) contribute to the rise of new phenotypic variation, which may be viable and adaptive (e.g., “facilitated variation”). In addition to accepted evolutionary processes that directly change gene frequencies, processes that bias the outcome of natural selection, particularly ontogenetic bias and niche construction, are also recognized in EES. A broadened notion of inheritance encompasses genetic, epigenetic and ecological (including cultural) inheritance. “Mutation pressure” refers to the population-level consequences of repeated mutation, depicted as dashed because mutation is also shown in “processes that generate novel variation.” 1Developmental bias and niche construction can also affect other evolutionary processes, such as mutation, drift and gene flow. 2In EES, this category of processes will often need to be broadened to include processes that change the frequencies of other heritable resources.
Therefore, the term Organic Nonoptimal Constrained Evolution (ONCE) is explained as follows. Although one might argue that all biological evolution is in reality organic evolution, the word “Organic” is specifically meant to pay tribute to Baldwin's idea of Organic Selection, as discussed below. As Baldwin's organic selection is only one of the many forces/factors of evolution that ONCE integrates, I prefer to use “Organic Evolution.” Within the integrative view of evolution of ONCE, the term “Constrained” refers mainly to internal factors that constrain, help direct, and even catalyze evolutionary changes (“negative” and “positive” constraints sensu Gould, 2002). Such constraints were not emphasized in Baldwin's works—nor in the publications of most Neo-Darwinists for most of the last century—but have become more so in recent decades by authors such as Stephen Jay Gould and Pere Alberch, and even more recently by, e.g., many evolutionary developmental biologists (Evo-Devoists) (see Figure 3). These subjects were discussed in detail in Diogo (in review), and the readers should refer to that paper for more details about them. In this specific paper I will instead focus more on the use of the term “Nonoptimal”—and its contrast with Baldwin's and Darwin's views of evolution and with Neo-Darwinist and Evo-Devo ideas in general -, as will be explained in the sections below.
Therefore, in a brief summary of ONCE and as a complement to the scheme shown in Figure 2, it can be said that, basically, according to ONCE, during development, internal constraints related to, e.g., homeostasis and canalization tend to constrain the diversity of/morphospace used by adult phenotypes but can also allow the existence/increase of developmental plasticity/hidden variation, while internal selection tends to constrain/decrease both the used morphospace and plasticity/variation (Figure 2). In contrast, developmental epigenetic phenomena directly influenced by the external environment (e.g., related to hormonal regulation) tend to explore the available physiological, behavioral and anatomical plasticity within the context of the specific niches/behaviors/ways of life that the members of the population occupy/display, and therefore have the potential to help direct evolutionary change, as also has genetic drift, for instance.
Behavioral choices/shifts of organisms are possible due to the plasticity resulting from and interplay between both internal factors and externally-driven epigenetic events. Behavioral shifts are thus the main drivers of evolutionary changes such as those seen in macroevolutionary trends, in which the new behaviors are successful and followed by behavioral persistence through social heredity via phenomena such as teaching, learning and imitation. Moreover, behavioral persistence is also linked to less emphasized phenomena, such as those in which parents may directly or indirectly contribute to the abandonment, or even death, of their descendants if they fail to learn/imitate/perform the new behaviors. That is, organisms are active evolutionary players that directly contribute to driving their own evolution and build their own niches.
Natural selection thus often comes into play as a secondary but likewise crucial evolutionary player, as noted above. In the case of macroevolutionary trends, due to the behavioral persistence of the population, random mutations and/or epigenetic events leading to physiological/behavioral/anatomical/genetic features that turn out to be advantageous within the context of the new behavior and niche and the external environment would be selected. These phenomena further direct evolution and increases the match between behavior, phenotype, and external environment. This process can extend for long periods of time, thus resulting in the observed macroevolutionary trends and, at least in the earlier stages of these trends, in a further increase in etho-eco-morphological matching, leading for instance to cases of successful phenotypic overspecialization. However, even in these cases the existence of strong developmental internal factors causing phylogenetic inertia normally never allows an organism to be fully “optimized” to its ecological habitat/external environment, i.e., to reach an optimal etho-eco-morphological correlation.
Moreover, in many cases behavioral persistence, loss of plasticity due to natural selection or to sexual selection, genetic drift, internal constraints, and/or simple chance, separately or combined with each other and with many other factors typical of, e.g., overspecialization, can make it difficult for the organisms to respond to new changes of/challenges by the external environment. This in turn may lead to etho-ecological, eco-morphological and/or etho-morphological mismatches, and potentially to cases of extinction. In addition, because of internal developmental constraints resulting from the fact that organisms are composed of many developmentally closely interconnected parts, in at least some cases natural selection of a certain trait will often result in correlated changes in other traits, which may be detrimental with respect to the direction of that selection. Emblematic cases of both etho- and eco-morphological mismatches due to internal constraints are the presence of hindlimb elements in whales and the abnormal occurrence of tails in human adults.
On the other hand—in what may also seem to be a paradox but shows instead the profound and complex interconnection of all these phenomena—internal constraints can themselves be crucial for maintaining some of the original phenotypic (e.g., morphological or behavioral) plasticity. For instance, plasticity is present at least in earlier developmental stages as hidden variation, which might allow organisms to display new behavioral shifts or revert to the ancestral, less specialized, behaviors and thus to escape eco-etho-morphological mismatches and/or evolutionary dead ends. Moreover, as a further example of niche construction and the central, active role played by organisms in evolution, the likelihood of a taxon reaching an evolutionary dead-end is deeply related to their and/or their parents' initial behavioral choices that helped to construct the niche in which they now live. Furthermore, another major differences between ONCE and not only NeoDarwinism but also the EES (Figure 3) (e.g., Schlichting and Pigliucci, 1998; Laland and Galef, 2009; Pigliucci and Müller, 2010; Kull, 2014; Laland et al., 2014, 2015, 2016), concerns the crucial role played by randomness in ONCE (Figure 2). As emphasized above, this role includes phenomena such as drift/gene flow, but also takes into consideration the particularly crucial role that behavioral choices play in ONCE. As an example of the impressive number of, and randomness involved in, the different behavioral choices that organisms can take, if a group of birds migrates to a region that has dozens or hundreds of islands, the first island that they see will be a random one. It is not necessarily the “best” one, and they might decide not visit or stay on it. However, simply by being the first one the birds saw, this island can actually play a huge role in their behavioral choices and the future of the bird population. If they decide to visit/stay on the island, conditions might prove to be difficult over time and the birds might migrate again, but in many cases they might be able to stay on the first island, particularly through niche construction and the help of phenomena such as epigenetic factors directly related to the conditions/factors present in that island, and so on.
The crucial point here is that all of the mismatches—“eco-morpho,” “etho-morpho,” or “etho-eco” and all of the many gray areas between them—evidenced by the empirical data that will be provided in the next section are predicted by ONCE. The acronym ONCE incorporates elements strongly related to such mismatches, such as Baldwin's organic selection, Gould's/Alberch's more constrained view of evolution, and a nonoptimal, nonstruggling and more random concept of evolution. For instance, mismatches between behavior (“etho”) and the external environment (“eco”) are predicted under ONCE due to behavioral persistence of organisms and/or lack of behavioral/genetic/anatomical plasticity leading to a perseverance of behavior even when the environment changes. An emblematic example of this concerns the case of the pandas mentioned above: they continue to eat bamboo despite the huge decrease in bamboo in the habitats where they leave (Pilcher, 2004). The mismatches between form (“morpho”) and external environment (“eco”) can also be due to behavioral persistence. Probably because of behavioral persistence, pandas continue to have a “panda's thumb” especially useful for eating bamboo, despite the decrease in bamboo available. Or—and most likely in combination—mismatches can be due to lack of plasticity, in turn likely related to strong internal constraints. For example, whales normally still develop some hindlimb bones during their ontogeny. The case of pandas is an example of etho-ecological and eco-morphological mismatches and of etho-morphological match (morphology still accompanies persistent behavior, but both do not match the decrease in bamboo). In contrast, the famous example of whales having vestigial hindlimbs concerns etho-morphological and eco-morphological mismatches, and an etho-ecological match (pelvic morphology did not change completely to optimally match the swimming behavior of whales, which does match the current aquatic habitat in which they live).
In this section I will refer to empirical studies that combine the four following points. First, they are a completely random, unbiased sample of a larger number of works that were found using a Google Scholar search for terms such as “anatomy, ecology, phylogeny” anywhere in an article published in a journal in the last two decades, and then looking also for similar works on the list of references of each of those papers. Second, only works including several species—in some cases from different higher clades—were chosen. Third, among the chosen works only those that analyzed form-behavior-ecology correlations among these species/clades using quantitative tools and within a strict phylogenetic context were selected. Fourth, the subset of works that were finally selected were mainly written by ecomorphologists, who would not be not biased, a priori, to produce results that would support the nonadaptationist idea of ONCE—they would more likely be biased in the opposite direction. Of course, it will not be possible to describe here the scope and results of all the works that were finally included in that subset. Therefore, I selected studies that broadly represent the overall patterns found in those studies and that represent several major groups within a specific, selected group—the vertebrates—to show how these patterns apply to both higher (more inclusive) and lower (less inclusive) clades. I will also provide a few examples from invertebrates and plants, to show how they effectively do reveal the same general patterns—in terms of mismatches—seen in vertebrates, as I do not want the readers to just take my word for it, without presenting some sound empirical cases studies on nonvertebrate taxa, on a subject (mismatches) that is so crucial for this paper and for ONCE.
Within those ecomorphological studies, the “eco” refers to many different items, from more external (“ecological”) factors such as hot vs. cold environments to more internal (“ethological”) factors such as the type of locomotion of the organisms themselves, e.g., bipedal vs. quadrupedal. That is why one can actually talk, in the present paper, about etho-eco-morphological mismatches, which include mismatches between the behavior and the external habitats where the organisms live (etho-ecological mismatches), between these habitats and the form of organisms (eco-morphological mismatches) and between behavior and form (etho-morphological mismatches). Of course, because the behavior and ecology of organisms are often deeply interrelated, it is not easy to delimit what is strictly “etho” and what is strictly “eco.” For instance, ecomorphologists and functional morphologist are often interested in the correlations between “function” and form, so the “eco” of ecomorphology would seem to refer mostly to “function.” However, historically in the form vs. function debate the “function” was often seen as more associated with behavior, instead, as is also the case in the present paper.
I will start with Vidal-García et al.'s (2014) paper. As explained by them (2014, p. 182), Australian myobatrachid frogs include two major lineages that occupy a wide range of habitats, including rainforest, wood and grasslands, and extreme arid deserts. There are genera with many species, each with species that specialize in a broad variety of habitats, including some species that can borrow and spend extensive periods underground. According to them, these frogs thus present “an ideal group for looking at broad patterns in adaptive morphology, testing for repeated evolution of similar patterns within species-rich genera and investigating environmental correlates and phylogenetic constraints.” They inferred “the environmental niche and examined body size and shape variation displayed by all species and genera of myobatrachid frogs to test whether environmental factors determine their morphology.”
Specifically, they tested two hypotheses: “(1) is the rotund, short-limbed morphology of burrowing frog species an adaptation to aridity and (2) are frog species from wet environments more likely to have longer legs?” Based on these hypotheses, they specifically predicted that: “(1) the species occurring in arid habitats would display more squat bodies and short limbs, (2) species from wet habitats would display stream-lined bodies with long legs, and (3) species occurring in intermediate habitats would display intermediate or conservative anuran body shapes.” They tested each of these predictions with anatomical and environmental datasets for all species and in a phylogenetic context to investigate whether “the different morphological patterns are constrained by phylogeny, restricting directional selection.” Their results show that despite the differences among and within genera, there is no obvious climatic correlation with body size. They noted that “previous claims that rotund, short-limbed forms reduce surface area and therefore evaporative water loss in dry habitats, sound intuitively correct, but are not supported by the different geographic occurrence of certain body forms in our data” (Vidal-García et al., 2014, pp. 181, 188). They summarized their results as follows: “there was no clear relationship between body size and environmental niche, and this result persisted following phylogenetic correction; for most species, there was a better match between environment/habitat and body shape, but this relationship did not persist following phylogenetic correction; our results suggest that phylogenetic legacy is important in the evolution of body size and shape in Australian anurans.”
Fabrezi et al.'s (2014) paper also refers to anurans. The authors studied the anatomy of several muscles thought to be related to anuran locomotion and lifestyle. They then tested whether there was a correlation between locomotor modes (i.e., behaviors such as hopping, jumping, swimming and/or walking), lifestyles (i.e., life in different ecological habitats, e.g., aquatic, arboreal and/or terrestrial), and morphological traits. Their results reveal that there is not a unique combination of morphological (muscular) characters that is clearly related to any habitat (eco-morphological mismatches) or even to any locomotor mode (etho-morphological mismatches).
Still within anurans, Moen et al. (2016) recently tested the relative importance of convergence vs. history on frog morphology. In their first test, the best-fitting model was dominated by adaptive convergence. That is, each microhabitat had only one anatomical optimum independent of clade, including separate optima for aquatic, arboreal, burrowing, semi-aquatic, terrestrial, and torrent-dwelling species. However, the anatomical features included in this first test were mainly external/superficial ones such as the length of the limbs, which in theory are less subject to constraints and/or more directly influenced by the external environment, as will be discussed below. The only internal one was muscle mass, and more fine-grained features such as number or attachments of muscles were not included. However, even among those mainly external features analyzed by them there was a substantial contribution from phylogeny. That is, while the six microhabitat-related frog ecomorphs they studied were similar around the planet, no matter where or how many times they evolved, they did find that the species' phenotypes were generally not at the estimated phenotypic optimum for their microhabitat. They showed instead “an imprint of history” linked with a systematic bias toward the ancestral, terrestrial phenotype.
Continuing with amphibians, Heiss et al.'s (2016) empirical study on newts (urodele amphibians) stresses the importance of behavioral shifts and concerns eco-morphological mismatches, and at the same time challenges the way in which topics such as the occurrence of polymorphisms are seen in the literature. As noted by the authors, certain newt species change seasonally between an aquatic and a terrestrial life as adults, being time after time exposed to dissimilar physical conditions affecting a wide range of organismal functions. For instance, seasonally habitat-changing newts display obvious changes in skin texture and tail fin anatomy, having markedly distinct aquatic and terrestrial morphotypes and facing a major functional challenge to change between efficient aquatic and terrestrial modes of prey capture. Newts adapt quickly to such challenges because they have a high degree of behavioral flexibility, using suction feeding in their aquatic stage and tongue prehension in their terrestrial stage. By undertaking an anatomical examination of the musculoskeletal system of the prey capture apparatus in the two multiphasic newt species Ichthyosaura alpestris and Lissotriton vulgaris, the authors showed hypertrophy of the hyolingual musculoskeletal system in both the terrestrial morphotype of L. vulgaris and aquatic morphotype of I. alpestris. That is, they showed that the seasonal behavioral and habitat shifts are accompanied by a species-dependent muscular plasticity.
Strikingly, in such a case study where the morphological changes seem to be directly related to behavioral changes and to epigenetic factors directly influenced by the external environment, eco-morphological mismatches still occur. For instance, under natural conditions suction feeding is the dominant prey capture mode of the aquatic morphotype and tongue protraction the prevailing prey capture mode of the terrestrial morphotype in newts. Therefore, Heiss et al. predicted that the muscles subarcualis rectus and rectus cervicis (both muscles associated with the neck region) should become hypertrophied/atrophied in a reciprocal manner as a response to shifts in functional demands in the two morphotypes. Specifically, their eco-morphological prediction was that muscle hypertrophy of the muscle rectus cervicis and atrophy of the muscle subarcualis rectus would be found in the aquatic morphotype and that hypertrophy of the muscle subarcualis rectus and atrophy of the muscle cervicis would be found in the terrestrial morphotype. However, that adaptationist prediction was contradicted by their results. Both muscle volumes and PCSAs (“Physiological Cross Sectional Areas”) of the rectus cervicis and subarcualis rectus as well as the volumes of the hyobranchial skeletal elements (which, in humans, include structures such as our hyoid bone) were significantly higher in the terrestrial than in the aquatic morphotype in L. vulgaris. Conversely, in I. alpestris muscle volumes, PCSAs and the volumes of the hyobranchial elements were significantly higher in the aquatic than in the terrestrial morphotype. Accordingly, the changes of the hyobranchial system within morphotypes in the seasonally habitat changing newts were different, as predicted. That is, a similar pattern of quantitative morphological changes was expected in both species based on different functional demands in aquatic vs. terrestrial morphotypes. However, all tested musculoskeletal hyobranchial elements hypertrophied in the terrestrial morphotype in L. vulgaris and in the aquatic morphotype in I. alpestris, and the authors did not find any evidence for a function-based reciprocal change.
I will now move on to fishes to explain the term “many forms-to-one function,” as this term was mainly promoted by the fish studies of Peter Wainwright and his students and colleagues. Wainwright et al. (2005, pp. 259–261) state that “many-to-one mapping is a ubiquitous feature of biological design…genetic epistasis produces many-to-one mapping of genotypes to phenotypes and has long been recognized as a basic property of plant and animal genetic systems…many-to-one mapping occurs between genotype and RNA secondary structure…protein structure maps redundantly onto function.” They further note that “for many physiological properties of organisms there is redundant mapping of the underlying features to values of the physiological, mechanical or performance property…for example, at the level of whole-organism performance, lizards with many different combinations of hindlimb dimensions and leg muscles can have the same jumping ability.” Their empirical study of labrid teleost fishes supported this idea: “in the labrid 4-bar case it would not be possible to infer jaw morphology given Maxillary KT” (output rotation in the upper jaw per degree of lower jaw rotation). They stressed that “the weak correlations between morphological and mechanical diversity in our simulations that was caused by the many-to-one mapping of 4-bar form to Maxillary KT is, by extension, discouraging for attempts to infer patterns of niche diversity from variation in morphology.”
Wainwright et al. then state that “previous authors have noted that morphology may not map closely to ecology because of the nature of behavioral or performance filters that are imposed on this relationship; our observations…on the nonlinear mapping of form to mechanics in many systems, provide an intrinsic mechanism in the relationship between form and mechanics that also can weaken this relationship.” Importantly, they obtained similar results among other groups of fishes. For instance, in Collar and Wainwright's (2006, p. 2275) study on centrarchid teleost fishes, they wrote: “we show that many-to-one mapping leads to discordance between morphological and mechanical diversity…despite close associations between morphological changes and their mechanical effects.” Specifically, each of the five morphological variables on their model underlined “evolution of suction capacity…yet, the major centrarchid clades exhibit an order of magnitude range in diversity of suction mechanics in the absence of any clear difference in diversity of the morphological variables.” As explained above, the occurrence of “many forms-to-one function” and “many functions-to-one form” mappings is very important within the context of ONCE. This is because these phenomena help to explain the common occurrence of mismatches, of exaptations, and of “function/behavior before form” evolutionary changes, all central tenets of ONCE.
Moving now to amniotes (a clade including reptiles and mammals), Anolis lizards are often seen in the literature as an emblematic example of homoplasy due to adaptations to similar habitats, and are thus expected to display a high match between ecology and morphology. Strikingly, ecomorphological empirical studies revealed that even in these lizards form and phylogeny are deeply correlated, supporting the importance of evolutionary constraints. This is pointed out for instance by Poe (2005). He explains that several authors documented striking ecological and morphological convergence in Anolis lizards of the Greater Antilles. For instance, various authors have defended the existence of several specific “ecomorphs”—i.e., lizards with a supposed set of correlated ecological and morphological states associated with their particular niche. These comprise a “crown-giant” ecomorph including large species with long tails that live on the top of the trees, and a “twig” ecomorph including small species with short limbs that live on narrow perches. The existence of such ecomorphs would thus suggest that characters related to, for instance, forelimb length, hindlimb length, and tail length would not be good phylogenetic markers because they would be mainly related to, and predicted by, the habitats where the lizards live.
However, this prediction was not supported when Poe tested the phylogenetic signal of four features that are said to be ecomorph features in both the entire Anolis clade and only among the Greater Antillean species from which such concept of ecomorphs was initially postulated: length from snout to vent (the opening through which the lizard defecates), hindlimb length, tail length and number of subdigital lamellae. The null hypothesis of no phylogenetic association was strongly rejected for all features in both the entire Anolis clade and the Greater Antillean taxa only. In a common reaction seen in almost all the empirical studies reviewed in this Section, the author did not hide his surprise: “this latter result is especially surprising…the presence of a strong phylogenetic correlation in the very species for which convergence has been demonstrated (i.e., within the Greater Antillean taxa only) begs for explanation” (Poe, 2005, p. 340). He then gave possible explanations for these “surprising” results. A possible reason is “that the ecomorphs generally constitute mini-radiations within islands.” A second possible explanation “is the existence of several species that defy ecomorph characterization or occupy different ecomorphs but still share derived ecomorph characters and close relationship.” Another possible justification is that “some ecomorphs appear to be monophyletic across islands…in this case ecomorph characters are a better predictor of phylogeny than island locality.”
Another similar empirical example, also referring to lizards, was published just a few months ago by Olberding et al. (2016, p. 775). In brief, they analyzed the evolution of total hindlimb length and of thigh, crus (leg), pes (foot) and toe length and its correlation with habitat use and phylogeny within 46 species of phrynosomatids (a group that includes spiny and horned lizards). They took into account that sexes are usually behaviorally and morphologically dimorphic but still found that “overall, clade-level differences were more important than habitat as predictors of segment or total hindlimb length.” Such empirical studies on lizards emphasize a crucial problem that has been often neglected, creating the illusion—among scientists, as well as the general public—that there is almost always a very close match between behavior (“function”: “etho”), form (“morpho”) and the habitat where organisms live (“eco”), i.e., a “design” in nature. The problem, as noted above, is the cherry-picking of “one trait-to-one species/group of species” examples, in which, e.g., a certain morphological feature of a certain species/group of species seems to be “perfect” for a certain “habitat.” This was precisely what was done for lizards such as Anolis, and the cherry-picked examples were then used as “evidence” of design and of “optimality,” or near “optimality” in nature. However, only rigorous empirical eco-morphological phylogenetic studies, including not only that/those species/groups of species but also other species of the same clade or living in the same geographical region, can appropriately be used to test whether specific morphological features are more correlated with ecology than they are with phylogeny, in the overall. In both these two recent studies, they were not: the adaptationist predictions were contradicted by empirical evidence.
Remaining within lizards, Vitt and Pianka (2005, p. 7877) published a paper entitled “Deep history impacts present-day ecology and biodiversity.” They explained that squamates (a clade including lizards, snakes and tuatara) are an interesting case study for testing theories on the evolution of ecological features because “their evolutionary history dates back to the early Jurassic or late Triassic, they have diversified on all major continents, and they occupy a remarkable diversity of ecological niches.” They noted that one theory postulates that ecological dissimilarities result from recent factors such as shifts in accessibility of different prey types or interspecific competition, predicting that niche differences arose relatively recently (“shallow history hypothesis”). Another theory postulates that ecological differences arose early in the evolutionary history of major groups and that present-day assemblages may thus coexist mainly because of early preexisting differences (“deep history hypothesis”).
Vitt and Pianka integrated phylogenetic data with ecological information to test these theories in squamates, using data on diets of 184 lizard species in 12 families from four continents. On the one hand, their results revealed that there were major behavioral (dietary) changes at six major divergence points, with major macroevolutionary implications, as predicted by ONCE. For instance, the most remarkable dietary divergence occurred in the late Triassic (period from 252 to 201 million of years), when Iguania (including, e.g., iguanas and chameleons) and Scleroglossa (including, e.g., geckos) split, leading to their occupation of very different regions of dietary niche space. This included the acquisition of chemical prey discrimination, jaw prehension, and broad foraging by scleroglossans, which allowed them to access sedentary and hidden prey that are unavailable to iguanians. That is, as stressed by the authors, “this cladogenic event may have profoundly influenced subsequent evolutionary history and diversification,” reinforcing the idea that behavioral shifts are probably often related to speciation/cladogenesis and subsequently to major macroevolutionary divergences. On the other hand, and also as predicted by ONCE, their results indicated that “such ancient events in squamate cladogenesis, rather than present-day competition, caused dietary shifts in major clades such that some lizard clades gained access to new resources, which in turn led to much of the biodiversity observed today.” That is, new behaviors that become persistent, and are then further directed by natural selection, can lead to evolutionary trends and eventually to decreased plasticity. This process might finally lead to cases in which there is a much stronger correlation between form and phylogeny than between form and the current habitat where the organisms live, i.e., to ecomorphological mismatches (see Sections above).
A different type of phylogenetic ecomorphological study of lizards was conducted by Abdala et al. (2014). In this study, “form” does not refer exclusively to external and/or hard-tissue features or to more general and/or physiological traits of muscles, but to finer morphological and morphometric muscle traits. They explain, in their introduction, that “following the tenets of ecomorphology that hold that different ecological demands lead to different organismal “designs,” it is reasonable to expect that morphological differences in muscles, tendons, and bones of the hindlimb of lizards will reflect their ecological specializations” (Abdala et al., 2014, p. 398). Because the arboreal environment is one of most challenging microhabitats exploited by lizards, they analyzed pedal grasping in Neotropical iguanian lizards through a morphometric study of muscles and a morphological and morphometric study of tendons. They also examined the relative proportions of the skeletal elements involved in grasping. Specifically, they tested whether 55 hindlimb internal features are related to habitat and/or to the phylogenetic relationships of the 23 species they studied.
Their results showed that phylogeny was the major factor associated with the anatomy of the hindlimb skeletal characters. Interestingly, this correlation was not as clear for most of the variables concerning hindlimb muscle and tendon morphometric characters, indicating that variation of the soft tissues cannot be explained only by phylogeny. However, neither the osteological nor soft tissue hindlimb characters were entirely related to habitat. In fact, in the overall the correlations between form and phylogeny were stronger than those between form and habitats. The authors stated, “the prevalence of phylogeny in shaping internal morphological traits correlated with external ones (e.g., femur and tibia length) is striking when contrasted with the observation that, in general, changes in body size and limb and tail proportions have been demonstrated to be associated with the evolution of locomotor performance in different ecological settings for several clades of squamates” (Abdala et al., 2014, p. 404).
Again, their use of the word “demonstrated” in this latter sentence should be taken with caution, because as explained above such “demonstrations” are often performed with either smaller samples or without a strict, broad phylogenetic analysis. For instance, one of the works listed by Abdala and colleagues to be contrasted with their results was Herrel et al. (2008), which suggested that differences in the morphology, muscle mass, and muscle mass distribution of the limbs of Anolis valencienni and A. sagrei were correlated with locomotor performance and locomotor style. However, these are only two species of the same genus, so it is very hard to argue that this suggestion is really framed in a broad phylogenetic and comparative context. As noted above, several works on Anolis and other lizards that were framed in such a broad context actually showed a stronger correlation between form and phylogeny. Abdala et al. (2014) recognized this somewhat when they stated that “the overwhelming effect of phylogeny in shaping morphology has been repeatedly obtained in studies of various taxa” and of many different structures, such as the external anatomy of lacertids (Vanhooydonck and Van Damme, 1999) and skinks (Goodman et al., 2008) and liolaemids (Schulte et al., 2004; Tulli et al., 2009), thermal biology of liolaemids (Cruz et al., 2009), and internal morphology of geckos and liolaemids (Zaaf et al., 1999; Tulli et al., 2012). Moreover, similar results were obtained for tendinous tissue in iguanid lizards (Tulli et al., 2011), and forelimb traits in tropidurid lizards (Grizante et al., 2010). Abdala et al. (2014, p. 397) thus conclude that “it appears that the Bauplan of the lizard pes incorporates a morphological configuration that is sufficiently versatile to enable exploitation of almost all of the available habitats; as unexpected as conservation of internal gross morphology appears, it represents a means of accommodating to environmental challenges by apparently permitting adequacy for all situations examined.” This statement is highly relevant for the idea of ONCE defended in the present work, because it further contradicts the argument that only a previous change of form can enable a new/different behavior/function.
Moving now to birds, a recent empirical study analyzed the links between the relative proportions of wing components (humerus, ulna, and carpometacarpus), flight style, and phylogeny in waterbirds (a diverse group including birds such as albatrosses, diving petrels, penguins, loons and shags, among many others) (Wang and Clarke, 2014). These birds exhibit substantial diversity in flight style (e.g., flapping, flapping/soaring, dynamic soaring, flapping/gliding) and foraging ecology (e.g., feeding on the wing, from the water surface, pursuit underwater). The authors examined the phylogenic signal and used ancestral trait reconstruction to test for rate shifts in forelimb proportions. Their results revealed a nonadaptationist pattern that was—once again—surprising to the authors: “different waterbird clades are clearly separated based on forelimb component proportions, which are significantly correlated with phylogeny but not with flight style” (Wang and Clarke, 2014, pp. 2847–2857). Agreeing with the criticism made above about adaptationists cherry-picking one-species-one-function types of examples to support their views, they stated: “although changes in locomotor ecology in birds are often expected to be generally linked to changes in forelimb shape, detection of these patterns requires their consideration in a phylogenetic framework.”
Concerning mammals, I will start with marsupials, which are less studied in such ecomorphological phylogenetic studies than are placentals (monotremes are even less studied). In order to analyze whether the diversity of food consumed by didelphids (opossums)—e.g., fruits, small vertebrates, insects—is related to molar size and shape, Chemisquy et al. (2015) used a geometric morphometric methodology to map shape onto the phylogeny of 16 didelphid genera. Then, they statistically estimated the effect of diet, size, and phylogeny on molar shape. Their results were very much in line with those of other studies discussed in this Section. Using the authors' own words, “all the analyses indicated little correlation between diet and molar shape and a strong correlation between the position of each genus on the phylogeny and molar shape” (Chemisquy et al., 2015, p. 217). They noted: “we found a strong phylogenetic effect, but the data available make the real constraints behind this historical pattern difficult to distinguish; we believe that the broad ecological niche used by most of the groups/genera studied herein (at least regarding diet) did not generate enough selective pressure on molar morphology to override preexisting differences that occur among clades, subsequently confounding the relationship between diet and tooth shape” (Chemisquy et al., 2015, p. 232). According to the authors, “because a large proportion of shape variation is related to phylogeny and not to size or diet, it is possible that this part of the variation is related to genetic drift, which could be translated on a macroevolutionary scale to an evolutionary model similar to a random walk or Brownian motion or other models where the change occurred at speciation.”
Similar results were obtained by Magnus and Cáceres (2016), as explicitly stated in the title of their paper, “Phylogeny explains better than ecology or body size the variation of the first lower molar in didelphid marsupials.” Following the methods of Chemisquy et al.'s (2015), but using a larger sample, they analyzed how the first lower molar evolved among didelphids by examining the two sexes independently, the influence of body size on molar shape, whether different habitats influence molar shape, and the links between these factors and phylogeny. Their study included a large number of individuals (261) from 37 species representing 14 of the 19 didelphid genera, with a total of 130 female specimens from 29 species and of 131 male specimens from 36 species. Their results indicated that the shape variation of the didelphid molars is more strongly correlated to phylogeny than to body size or habitat. In their own words, “body size assumes a secondary role when influencing molar shape adaptation in didelphids, and habitat is apparently not meaningful in such a role” (Magnus and Cáceres, 2016, p. 10). However, they noted that, although form was more strongly correlated with phylogeny, there were detectable minor differences in molar shape variation due to habitat and body size. When considered together with phylogeny, habitat seemed to be somewhat important in the adaptive radiation of didelphid lineages. For instance, this radiation is accompanied by a general shift of habitat, from more ancestral and arboreal to more derived and terrestrial/scansorial environments. Accordingly, molar shape configuration evolved from a relatively small to a large trigonid, an evolutionary trend modulated by phylogeny, according to them.
Importantly for the idea of ONCE, Magnus and Cáceres (2016, p. 11) link these trends to mass extinction events followed by environmental changes and behavioral changes in response to those shifts. Specifically, they argue that “following the probable Miocene mass extinction, the reduction of the forest environments allowed the development of open habitats giving more chances for diversification of the surviving Miocene terrestrial marsupials via a rapid cladogenesis (or adaptive radiation).” For them, “this also had other evolutionary consequences by inducing a major shift in feeding habits of the family (from frugivory related to a larger talonid, to insectivory related to a larger trigonid), with lineages becoming more insectivorous with time.” This change in feeding habits may be related to the behavioral tendency toward terrestriality in didelphid evolution that occurred independently in various taxa, e.g., Monodelphis (short-tailed-opossum) in Marmosini and Thylamys (fat-tailed mouse opossums) and Cryptonanus (gracile opoissums) in Thylamyini. The authors also point out that the strong association between molar shape variation and phylogeny does not necessarily mean that the shape of the first molar is a conservative feature within didelphids. That is, molar shape configuration changed substantially in didelphid evolution but kept a strong phylogenetic signal, with the current environment playing a minor role in its macroevolution but an intrinsic role in the adaptive radiation of certain lineages.
So, there is—again—a link between phylogenetic constraints and behavioral shifts in response to environmental changes; i.e., form is not conservative within the whole didelphid clade. However, after a certain behavioral shift is completed and a subclade follows a certain evolutionary path, then its ancestors often “get stuck” in that path by a combination of behavioral persistence (e.g., being terrestrial) and natural selection (Figure 2). This leads to the maintenance of a new form or the occurrence of a certain specific evolutionary trend from that form within the subclade. This can happen even if the habitat is changed and certain other behavioral traits are changed along with it (e.g., eating food item B instead of food item A), leading to an overall scenario in which there is a stronger correlation between form and phylogeny than between form and habitats and even between form and certain behaviors, including dietary preferences.
In a study that also included marsupials, Narita and Kuratani (2005) examined the distribution pattern of mammalian vertebral formulae as a case study to investigate the contribution of developmental constraints in vertebrate evolution. Their results indicated that the changes in the vertebral formulae in eutherian mammals (which include placentals) seem to be lineage-specific; e.g., most Carnivora species have 20 instead of 19 thoracolumbar vertebrae. Such lineage-specific vertebral formulae are different from the estimated distribution pattern that they calculated on the assumption of evolution exclusively related to selective pressures. Therefore, they concluded that “developmental constraints played an important role in the evolution of mammalian vertebral formulae” (Narita and Kuratani, 2005, p. 91). They further noted that in some cases the lineage-specific ontogenetic constraints seemingly are not easy to surmount because members of a clade radiate but still keep the same basic bodyplan shared by all taxa in the clade. The patterns of vertebral formulae seen in marsupials, in particular, indicate a strong influence of developmental constraints. In these mammals—which are often seen as an emblematic illustration of convergence or parallelism because they have radiated into many forms and habitats in a way somewhat similar to that seen in placentals—there is a constant vertebral formulae. The authors therefore state “if the evolution of vertebral formulae tends to change under taxon-specific selection toward the taxon-specific habitat and morphology, the constant vertebral formulae in marsupials and variable vertebral formulae in placentals could not be reconciled (Narita and Kuratani, 2005, p. 104). So, they conclude, “it is again much more appropriate to consider that the stasis has occurred as a result of marsupial specific (and mammalian primitive) developmental constraints that could not be overcome in the radiation of marsupial lineages.”
Moving on to placentals, we find one of the very few ecomorphological studies based on an explicit, broad phylogenetic analysis in which the correlation between morphology and phylogeny is not stronger than that between morphology and ecological and/or behavioral traits. However, even in this study—by Fabre et al. (2015)—the latter correlations are not stronger than the one between form and phylogeny, either. That is, the evolution of forelimb shape in the placentals studied by the authors, the musteloid carnivorans (a group including, e.g., red pandas, otters, raccoons, and skunks) was strongly influenced by other anatomical features such as body mass as well as by both locomotion and phylogeny (Figure 4). As noted by them, musteloids form a very diverse group: they display a broad variation in body mass and live in an array of habitats that is more varied than that of any other carnivoran clade, so they are exceptionally interesting for ecomorphological studies. The authors explain that phylogeny—one of the three major factors explaining forelimb shape is musteloids, according to their results, as explained above—was also found to be a key factor in their previous study (Fabre et al., 2013) of musleloid shape data.
Figure 4. Phylogenetic relationships of the musteloid species used in Fabre et al.'s study (modified from Fabre et al., 2015). Time scale is in millions of years; outlines used as symbols scaled relatively to body size and colors are: green for arboreal; yellow for semi-arboreal; red for terrestrial; brown for semi-fossorial; and blue for aquatic species.
For Fabre et al. (2015, p. 603), such a strong signal “is not too surprising as, for example, all the aquatic species included in the study belong to a single clade (Lutrinae)” (which includes otters). “thus, shared ancestry, body mass and locomotor habitat are all important factors in explaining variation in limb shape across species.” This strong phylogenetic signal is clearly seen in Figure 4. Another key point stressed by them is that this study also showed that each bone has its own ecological/functional signal, e.g., “the signal provided by the shape of the humerus tends to separate arboreal and semi-arboreal species, whereas the analyses on the shape of the ulna and radius tend to separate the aquatic from the semi-fossorial species.” This result reinforces the criticism made above that the adaptationist framework is often too simplistic, not only because each organism might display several behaviors/functions (e.g., squirrels climb trees, run on the ground, and so on), but also because in macroevolution there is often mosaic morphological evolution. Different parts of the body change in different ways and might be adapted for different behaviors/functions and/or ecological habitats (e.g., our hindlimbs have many features related to bipedalism, our forelimbs with tool use, our head with facial communication, and so on).
The same team that published the study on lizard muscles and tendons described above (Abdala et al., 2014) also recently published an ecomorphological phylogenetic analysis on rodents (the clade including, e.g., mice and rats) and compared the patterns seen in rodents vs. lizards (Carrizo et al., 2014). As they did in their study of lizards, the authors noted, in their introduction, that previous investigations on the external and internal architecture of muscles had suggested that muscle anatomy is linked to particular functional demands and locomotory types in mammals. For instance, it has been said that fossorial taxa (i.e., tetrapods that often live underground, e.g., through digging) have enlarged forelimb extensor muscles, and that the form of the tendon of the forearm muscle flexor carpi ulnaris is particularly related to life in a specific habitat in, e.g., didelphid marsupials. However, once again, those suggestions were often mainly based on functional morphology analyses or on phylogenetic analyses that included only a few taxa and/or characters. The authors' aim was thus to test whether the morphology of forelimb muscles and tendons of 97 adult specimens belonging to 26 species of sigmodontines (the rodent subfamily that includes mice and rats)—coded as 32 phylogenetic characters—is correlated with different types of locomotion (e.g., ambulatory, fossorial, saltatorial and natatorial), with phylogeny, and/or with other factors.
Specifically, they predicted that fossorial sigmodontines have forelimb muscles that are shorter, and have greater cross-sectional areas, than those of other locomotory groups. This is because muscles with short fibers that attach to long tendons in a pennate pattern (i.e., obliquely) are said to evolve to act as force generators for elastic strain energy storage and recovery within the tendon. They also predicted that forelimb extensor muscles used by natatorial (swimming) species should have large cross-sectional areas in order to generate a higher force to thrust. Their results showed that tendon variables seem to be more correlated with locomotory types than muscle variables, but—once again—phylogeny is the best predictor of morphology, overall. Specifically, they stated, “twelve tendon variables of the forelimb exhibit distinct differences between fossorial and scansorial sigmodontines,” but “no particular morphological variables are associated with ambulatory, saltatorial, and natatorial taxa…this phylogenetic inertia could be responsible for the homogeneity in the overall muscle forelimb morphology in this group” (Carrizo et al., 2014, pp. 843, 849).
We will now consider the organisms that are more closely related to us, primates. As explained in the beginning of this Section, we recently compiled a series of examples showing how many of the structures of our body do not make sense unless they are seen as highly influenced by phylogenetic and developmental constraints. Many of the examples that Gould (e.g., 2002) famously used to stress the importance of constraints and to criticize both the adaptationist framework and the “argument for design” are in fact related to features that can be observed in humans, such as the so-called “blind spot” in our eyes (see Diogo and Molnar, 2016 for more details). So, what about other primates? A recent phylogenetic study about cranial morphology in lemurs by Baab et al. (2014) addressed this question. In that study, phylogeny explained, once again, the greatest amount of variation; much smaller amounts of variation were explained by diet and even smaller amounts with activity pattern. As is usually the case, the authors noted that previous researchers had suggested that there is a positive relationship between diet, biomechanical function, and cranial shape in some vertebrate groups, but correctly pointed out: “however, some of these studies failed to explicitly incorporate phylogeny into their analyses” (Baab et al., 2014, p. 1444). Furthermore, they noted that some explicit phylogenetic studies—e.g., of primates such as Old and New World monkeys—actually “did fail to recover or recovered only a weak relationship between ecological variation, including differences in diet, and cranial form (e.g., McBrayer, 2004; Cardini and Elton, 2008; Jones and Goswami, 2010; Perez et al., 2011).” In addition, in some studies dietary variables could predict a great proportion of variation of cranial shape, but only when phylogeny was ignored, because when it was taken into account many fewer anatomical features remained associated to ecology (e.g., Perez-Barberia and Gordon, 1999; Marroig and Cheverud, 2005). Based on the results of their lemur phylogenetic study, Baab et al. (2014, p. 1472) thus stated that “overall, lemur cranial morphology retains a strong phylogenetic signal…the correlation between diet and cranial form is weak when the underlying phylogeny is taken into account, a pattern also documented in other vertebrate lineages.” According to the authors, “this may mean that diet has not strongly impacted cranial form, but may also be the result of an evolutionary history characterized by a relatively small number of dietary (behavioral) shifts that occurred in conjunction with the divergence of major clades and few instances of dietary convergences between these clades.”
In another recent study that also comprised strepsirrhines—i.e., the sister-group of all other extant primates, which includes lemurs and lorisoids such as lorises and galagos -, as well as rodents and marsupials, Ruth et al. (2016) tested the usually accepted assumption that the foramen magnum position is an indicator of bipedalism because it is supposed to favor a more “balanced” position of the skull. They examined the connection between the angle of this foramen and locomotion in these three clades including bipedal or orthograde species and quadrupedal or pronograde species. Their results revealed that in marsupials and strepsirrhines there is no association between this angle and locomotor pattern. They did find a significant difference in this angle between quadrupedal and bipedal rodents, but when these taxa were analyzed in the context of enlarged auditory bullae, this relationship was no longer significant. Using their own words, they concluded that “taken together, these data indicate that several developmental modules of the cranium influence FMA (foramen magnum angle), but that locomotion does not; we caution that basicranial evolution is a complex phenomenon that must be explored in the context of each taxon's unique evolutionary and developmental history” (Ruth et al., 2016, p. 45).
To show that many of the results found in the works on the Vertebrata that were mentioned in the above chapters also apply more broadly, I will now refer to one of the numerous empirical phylogenetic ecomorphological studies on invertebrates that reveals patterns essentially similar to those usually found in the other invertebrate studies I reviewed. The study—by Law et al. (2014)—is specifically focused on the polychaete family Opheliidae (a family of small annelid worms). The authors explain that there are considerable anatomical and behavioral differences between two major groups within this family, e.g., some animals burrow by peristalsis (i.e., through wave-like muscle contractions) while others display undulatory burrowing. They therefore analyzed the anatomical differences that might be related to these distinct burrowing behaviors and undertook a DNA-based phylogenetic analysis to test the connection between behavior, form, and the habitats where these worms live.
The mapping of the anatomical features onto the phylogeny revealed close links between morphology (namely the musculature) and behavior, and a lower correlation between form and habitat/ecology. They state that “even though the mechanical responses of muds and sands to burrowers are substantially different—muds are elastic materials through which most worms extend burrows by fracture, whereas sands are noncohesive granular materials, suggesting that morphologies and behaviors of burrowing animals might be distinct between these two habitats—our data showed that habitat distribution is variable and did not coincide well with burrowing mode, musculature, or presence of septa” (Law et al., 2014, p. 557). They further note that ”the nearly identical morphologies, musculature, and undulatory burrowing behavior within Ophelininae did not coincide with a single sediment distribution; even generalizations based on similar morphologies and musculature that appear to be convergent seem to be an unreliable indicator of habitat distribution.” Various phylogenetic studies of several clades of herbivorous insects also point out that insect host-use evolution is often quite conservative regarding host taxon, being often better predicted by phylogeny than by apparent ecological opportunity (Kelley and Farrell, 1998).
Lastly, I will refer to one example from plants that illustrates the pattern commonly found among the numerous plant ecomorphological studies found in the literature, as documented for instance in the exceptional book Phenotypic Evolution—A Reaction Norm Perspective by Schlichting and Pigliucci (1998). As stated by these authors (pp. 182–183), there are many examples “in the literature (of plants) of cases in which patterns usually interpreted as the results of natural selection are in fact reinterpreted to be due to some sort of constraint; again, in these cases phylogenetic analyses are pivotal.” For instance, they review a large study of 910 angiosperms that tested the assumption that features of fleshy fruits such as length, diameter, mass, or energy and protein content evolved in response to the animals that disperse those fruits. The study showed that within the 16 traits examined 61% of the overall variance was explained by phylogeny, and that most correlations between fruit traits and type of disperser were nonsignificant after considering phylogenetic effects. As they note, “the overall conclusion is that historical contingencies are generally responsible for the observed macroevolutionary scenario, while selection played a comparative minor role.”
A common feature among all the empirical studies reviewed above is that their authors—particularly those that are ecomorphologists—often admitted that they were profoundly surprised by their own results. This is because they were often searching for clear, positive eco-morphological correlations that should, according to their assumptions, be stronger than phylo-morphological correlations. The authors' surprise sends us an important message: lack of clear eco-morphological correlations is probably even much more common that these studies indicate. That is, these authors probably represent a small group of researchers that admit not only that their predictions/a priori ideas were wrong, but also that they were genuinely surprised by this fact. It is possible that many authors who obtained similar results—or, in particular, “worse” results such as a complete lack of any type of correlation or even negative correlation between morphology and ecology—simply opted to not publish their results. Such “negative results” may be ignored because there is no “story at all to be told,” a phenomenon that is well known in science and was strongly criticized by Gould in his famous “Cordelia's dilemma” metaphor (e.g., Gould, 1993, 2002; see also Diogo, 2006). Therefore, based on the results of the ecomorphological empirical studies reviewed here and on the similar results of others that I did not have the space to review, and on this bias against the publication of such “negative” results, I think that the common occurrence of eco-morphological mismatches is probably one of the most crucial untold stories in evolutionary biology. The story remains untold because it goes against the main ideas defended by followers of Darwinism, of Lamarckism, of Neo-Darwinism, and even of the Evo-Devo Extended Evolutionary Synthesis (see Figure 3), as explained above. In fact, it needs to be emphasized that, as noted above, the methodology used for this paper explicitely avoided cherry-picking. That is, all the publications that were found within the dates that were searched and that included the searched keywords—e.g., ecomorphology and phylogeny, or ecology and anatomy and phylogeny, and so on (see above)—were read and analyzed for the present work, and the ones included in this review represent the pattern that was consistently seen in all those publications. Therefore, the clear pattern seen in all these publications retrieved using this methodology does seem to indicate that this pattern is the norm in nature, and not the exception.
By placing behavioral choices, shifts and persistence at the very center, and as the primary drivers, of evolution, thus considering organisms to be key active players in their evolutionary history and the evolutionary history of other organisms as well, one can explain this pattern because one can account for evolutionary trials-and-errors, “mistakes,” and mismatches. If organisms as a whole were mainly passive players, and everything was “programmed” (gene-centered view) in the genome, decided by external forces (externalist view: e.g., by the external environment or a supernatural being), or related to vitalistic forces within the cells/atoms/tissues forming the organisms (vitalism), then in theory we should not expect such a high frequency of mismatches. Computers do not often make “bad decisions” because they normally do not decide anything at all by themselves. But organisms are active players that can potentially make an almost endless number of behavioral choices. Many of these choices are constrained by phenomena such as teaching/learning/imitating, leading to behavioral persistence, but there are also numerous cases of behavioral changes, including unexpected and maladaptive ones, in nature.
Darwin did care a lot about extinction, and was much more aware of evolutionary mismatches than were many Neo-Darwinists; extinction is included in the last, and most famous, sentence of his 1959 book: “a struggle for life, and as a consequence to natural selection, entailing divergence of character and the extinction of less-improved forms…thus, from the war of nature, from famine and death, the most exalted object which we are capable of conceiving, namely, the production of the higher animals, directly follows. there is grandeur in this view of life, with its several powers, having been originally breathed into a few forms or into one; and that, whilst this planet has gone cycling on according to the fixed law of gravity, from so simple a beginning endless forms most beautiful and most wonderful have been, and are being, evolved.” I completely agree with Darwin's emphasis on extinction and “less-improved forms” to refer to evolutionary mismatches, and in some ways some of the tenets of the idea of ONCE are also not too different from those of Aristotle, who is one of my personal heroes. However, unlike Darwin I would not use the teleological word “improved” in this context. Above all, I think that the new discoveries in evolutionary biology - which were only possible because of Darwin's brilliant ideas - have shown us that life is not only an intense, never ending, unbreakable “struggle, war, famine and death,” where forms have to optimally “fit” to their habitats, and any nonoptimality, any behavior that is not directly related to survival and/or reproduction and does not “improve” fitness is purged from existence. So long as overspecialized humans do not put in danger the ecosystems of this beautiful planet, and the globe continues to display such an amazing quality and diversity of resources, life it much richer and varied than that. In fact, as recently noted by Gailer et al. (2016), more and more studies are emphasizing the large plasticity between the so-called “optimal” morphology of a structure and the potential function of that structure, underscoring the need to appreciate apparently “maladaptive” structures in biological evolution as nevertheless effective functioning units. That is, such structures and the function they perform are “good enough” to allow the organisms that display them to survive and reproduce, in the nonstruggling view of life defended in ONCE. Not every single structure needs to be “perfectly designed,” or “optimal,” or ”optimally fit” for each function it performs and/or for each habitat occupied or behavior exhibited by the organisms displaying it, at every single developmental stage and geological time during their evolutionary history: in fact, many structures, such as vestigial rudimentary ones, may well not perform any function at all.
Another example concerns the fact that analyses of the yeast genome have shown that 70% of its genes were actually unnecessary in a rich medium. If this is really so, even if we follow an “utilitarian” framework and speculate that all those 70% may eventually become useful for subsequent particular environmental changes (Schlichting and Pigliucci, 1998), the fact is that when yeast lives in such a rich medium, more than two thirds of their genes are “dispensable” or at least “redundant.” This does not match at all with the narrative idea of a continuous, implacable struggle in evolution, in which “nature does nothing in vain.” This planet has seen, since about four billion years ago, zillions of fascinating organisms displaying an almost infinite array of behaviors—from bacteria's adaptive comportments to suicidal or self-harming acts by birds, from selfish attitudes of cuckoos to profoundly altruistic warning calls of squirrels, from remarkable phenotypic features to expandable genes. And this is precisely what led to what Darwin beautifully referred to as “endless forms most beautiful and most wonderful.”
The author confirms being the sole contributor of this work and approved it for publication.
The author declares that the research was conducted in the absence of any commercial or financial relationships that could be construed as a potential conflict of interest.
Abdala, V., Tulli, M. J., Russell, A. P., Powell, G. L., and Cruz, F. B. (2014). Anatomy of the crus and pes of Neotropical iguanian lizards in relation to habitat use and digitally based grasping capabilities. Anat. Rec. 297, 397–409. doi: 10.1002/ar.22851
Baab, K. L., Perry, J. M. G., Rohlf, F. J., and Jungers, W. L. (2014). Phylogenetic, ecological, and allometric correlates of cranial shape in Malagasy lemuriforms. Evolution 68, 1450–1468. doi: 10.1111/evo.12361
Baldwin, J. M. (1895). Mental Development in the Child and Race: Methods and Processes. New York, NY: MacMillan.
Baldwin, J. M. (1896b). A new factor in evolution (continued). Ame. Nat. 30, 536–553. doi: 10.1086/276428
Baldwin, J. M. (1896c). On criticisms of organic selection. Science 4, 724–727. doi: 10.1126/science.4.98.724-a
Beatty, J. (2008). “Chance variation and evolutionary contingency: Darwin, Simpson (The Simpsons) and Gould,” in The Oxford Handbook of Philosophy of Biology, eds M. Ruse (Oxford: Oxford University Press), 189–210.
Bocherens, H., Schrenk, F., Chaimanee, Y., Kullmer, O., Mörike, D., Pushkina, D., et al. (2016). Flexibility of diet and habitat in Pleistocene South Asian mammals: implication for the fate of the giant fossil ape Gigantopithecus. Quat. Int. doi: 10.1016/j.quaint.2015.11.059
Bonner, J. T. (1988). The Evolution of Complexity by Means of Natural Selection. Princeton: Princeton University Press.
Cardini, A., and Elton, S. (2008). Variation in guenon skulls (I): species divergence, ecological and genetic differences. J. Hum. Evol. 54, 615–637. doi: 10.1016/j.jhevol.2007.09.022
Carrizo, L. V., Tulli, M. J., and Abdala, V. (2014). An ecomorphological analysis of forelimb musculotendinous system in sigmodontine rodents (Rodentia, Cricetidae, Sigmodontinae). J. Mammal. 95, 843–854. doi: 10.1644/13-MAMM-A-232
Chemisquy, M. A., Prevosti, F. J., Martin, G., and Flores, D. A. (2015). Evolution of molar shape in didelphid marsupials (Marsupialia: Didelphidae): analysis of the influence of ecological factors and phylogenetic legacy. Zool. J. Linn. Soc. 173, 217–235. doi: 10.1111/zoj.12205
Collar, D., and Wainwright, P. (2006). Discordance between morphological and mechanical diversity in the feeding mechanism of centrarchid fishes. Evolution 60, 2575–2584. doi: 10.1111/j.0014-3820.2006.tb01891.x
Corning, P. A. (2013). Evolution 'on purpose': how behavior has shaped the evolutionary process. Biol. J. Linn. Soc. 112, 242–260. doi: 10.1111/bij.12061
Cruz, F. B., Belver, L., Acosta, J. C., Villavicencio, H. J., Blanco, G., and Canovas, M. G. (2009). Thermal biology of Phymaturus lizards: evolutionary constraints or lacks of environmental variation. Zoology 112, 425–432. doi: 10.1016/j.zool.2009.03.004
Darwin, C. (1859). On the Origin of Species by Means of Natural Selection, or, the Preservation of Favored Races in the Struggle for Life. London: J. Murray.
Depew, D. J. (2003). “Baldwin and his many effects,” in Evolution and Learning: The Baldwin Effect Reconsidered, eds B. H. Weber and D. J. Depew (Cambridge: MIT Press), 3–31.
Diogo, R. (2006). Cordelia's dilemma, historical bias, and general evolutionary trends: catfishes as a case study for general discussions on phylogeny and macroevolution. Int. J. Morphol. 24, 607–618. doi: 10.4067/S0717-95022006000500016
Diogo, R., Esteve-Altava, B., Smith, C., Boughner, J. C., and Rasskin-Gutman, D. (2015b). Anatomical network comparison of human upper and lower, newborn and adult, and normal and abnormal limbs, with notes on development, pathology and limb serial homology vs. homoplasy. PLoS ONE 10:e0140030. doi: 10.1371/journal.pone.0140030
Diogo, R., and Molnar, J. L. (2016). Links between evolution, development, human anatomy, pathology and medicine, with a proposition of a re-defined anatomical position and notes on constraints and morphological 'imperfections'. J. Exp. Zool. B Mol. Dev. Evol. 316, 215–224. doi: 10.1002/jez.b.22679
Diogo, R., Noden, D., Smith, C. M., Molnar, J. L., Boughner, J., Barrocas, C., et al. (2016). Learning and Understanding Human Anatomy and Pathology: An Evolutionary and Developmental Guide for Medical Students. Oxford: Taylor and Francis.
Diogo, R., Smith, C., and Ziermann, J. M. (2015a). Evolutionary Developmental Pathology and Anthropology: a new area linking development, comparative anatomy, human evolution, morphological variations and defects, and medicine. Dev. Dyn. 244, 1357–1374. doi: 10.1002/dvdy.24336
Diogo, R., and Wood, B. (2016). “Origin, development and evolution of primate muscles, with notes on human anatomical variations and anomalies,” in Developmental Approaches to Human Evolution, eds J. Boughner and C. Rolian (Hoboken: John Wiley and Sons), 167–2014.
Diogo, R., Ziermann, J. M., and Linde-Medina, M. (2015c). Is evolutionary biology becoming too politically correct? A reflection on the scala naturae, phylogenetically basal clades, anatomically plesiomorphic taxa, and “lower” animals. Biol. Rev. 90, 502–521. doi: 10.1111/brv.12121
Downes, S. M. (2003). “Baldwin effects and the expansion of the explanatory repertoire in evolutionary biology,” in Evolution and Learning: The Baldwin Effect Reconsidered, eds B. H. Weber and D. J. Depew (Cambridge: MIT Press), 33–52.
Eldredge, N. (2014). Extinction and Evolution: What Fossils Reveal about the History of Life. Toronto, ON: Firefly Books.
Fabre, A.-C., Cornette, R., Goswami, A., and Peigné, S. (2015). Do constraints associated with the locomotor habitat drive the evolution of forelimb shape? A case study in musteloid carnivorans. J. Anat. 226, 596–610. doi: 10.1111/joa.12315
Fabre, A.-C., Cornette, R., Peigné, S., and Goswami, A. (2013). Influence of body mass on the shape of forelimb in musteloid carnivorans. Biol. J. Linn. Soc. 110, 91–103. doi: 10.1111/bij.12103
Fabrezi, M., Manzano, A. S., Abdala, A., and Lobo, F. (2014). Anuran locomotion: ontogeny and morphological variation of a distinctive set of muscles. Evol. Biol. 41, 308–326. doi: 10.1007/s11692-014-9270-y
Gailer, J. P., Calandra, I., Schulz-Kornas, E., and Kaiser, T. M. (2016). Morphology is not destiny: discrepancy between form, function and dietary adaptation in bovid cheek teeth. J. Mammal. Evol. 23, 369–383. doi: 10.1007/s10914-016-9325-1
Goodman, B. A., Miles, D. B., and Schwarzkopf, L. (2008). Life on the rocks: habitat use drives morphological and performance evolution in lizards. Ecology 89, 3462–3471. doi: 10.1890/07-2093.1
Griffiths, P. E. (2003). “Beyond the Baldwin effect: James Mark Baldwin's 'social heredity', epigenetic inheritance and niche-construction,” in Evolution and Learning: The Baldwin Effect Reconsidered, eds B. H. Weber and D. J. Depew (Cambridge: MIT Press), 193–215.
Grizante, M. B., Navas, C. A., Garland, T. Jr., and Kohlsdorf, T. (2010). Morphological evolution in Tropidurinae squamates: an integrated view along a continuum of ecological settings. J. Evol. Biol. 3, 98–111. doi: 10.1111/j.1420-9101.2009.01868.x
Hall, B. K. (2002). Palaeontology and Evolutionary Developmental Biology: a science of the nineteenth and twenty–first centuries. Palaeontology 45, 647–669. doi: 10.1111/1475-4983.00253
Hall, B. K. (2003). “Baldwin and beyond: organic selection and genetic assimilation,” in Evolution and Learning: The Baldwin Effect Reconsidered, eds B. H. Weber and D. J. Depew (Cambridge: MIT Press), 141–167.
Heiss, E., Handschuh, S., Aerts, P., and Van Wassenbergh, S. (2016). Musculoskeletal architecture of the prey capture apparatus in salamandrid newts with multiphasic lifestyle: does anatomy change during the seasonal habitat switches? J. Anat. 228, 757–770. doi: 10.1111/joa.12445
Herrel, A., Vanhooydonck, B., Porck, J., and Irschick, D. (2008). Anatomical basis of differences in locomotor behavior in Anolis lizards: a comparison between two ecomorphs. Bull. Mus. Comp. Zool. 159, 213–238. doi: 10.3099/0027-4100-159.4.213
Hone, D. W. E., and Benton, M. J. B. (2005). The evolution of large size: how does Cope's Rule work? Trends Ecol. Evol. 20, 4–6. doi: 10.1016/j.tree.2004.10.012
Jones, K. E., and Goswami, A. (2010). Quantitative analysis of the influences of phylogeny and ecology on phocid and otariid pinniped (Mammalia; Carnivora) cranial morphology. J. Zool. 280, 297–308. doi: 10.1111/j.1469-7998.2009.00662.x
Kelley, S. T., and Farrell, B. D. (1998). Is specialization a dead end? The phylogeny of host use in Dendroctonus bark beetles (Scolytidae). Evolution 52, 1731–1743.
Kull, K. (2014). Adaptive evolution without natural selection. Biol. J. Linn. Soc. 112, 287–294. doi: 10.1111/bij.12124
Laland, K., Matthews., B., and Feldman, M. W. (2016). An introduction to niche construction theory. Evol. Ecol. 30, 191–202. doi: 10.1007/s10682-016-9821-z
Laland, K. N., Odling-Smee, J., and Turner, S. (2014). The role of internal and external constructive processes in evolution. J. Phys. 592, 2413–2422. doi: 10.1113/jphysiol.2014.272070
Laland, K. N., Uller, T., Feldman, M. W., Sterelny, K., Müller, B., Moczek, A., et al. (2015). The extended evolutionary synthesis: its structure, assumptions and predictions. Proc. R. Soc. Lond. B 282. doi: 10.1098/rspb.2015.1019
Law, C. J., Dorgan, K. M., and Rouse, G. W. (2014). Relating divergence in polychaete musculature to different burrowing behaviors: a study using Opheliidae (Annelida). J. Morphol. 275, 548–571. doi: 10.1002/jmor.20237
Lindholm, M. (2015). DNA dispose, but subjects decide -learning and the extended synthesis. Biosemiotics 8, 4431–4461. doi: 10.1007/s12304-015-9242-3
Lloyd, E. A. (2015). Adaptationism and the logic of research questions: how to think clearly about evolutionary causes. Biol. Theory 10, 343–362. doi: 10.1007/s13752-015-0214-2
Magnus, L. Z., and Cáceres, N. (2016). Phylogeny explains better than ecology or body size the variation of the first lower molar in didelphid marsupials. Mammalia. doi: 10.1515/mammalia-2015-0070
Marroig, G., and Cheverud, J. M. (2005). Size as a line of least evolutionary resistance: diet and adaptive morphological radiation in new world monkeys. Evolution 59, 1128–1142. doi: 10.1111/j.0014-3820.2005.tb01049.x
McBrayer, L. D. (2004). The relationship between skull morphology, biting performance and foraging mode in Kalahari lacertid lizards. Zool. J. Linn. Soc. 140, 403–416. doi: 10.1111/j.1096-3642.2003.00106.x
McShea, D. W. (1991). Complexity and evolution: what everybody knows. Biol. Philos. 6, 303–324. doi: 10.1007/BF00132234
McShea, D. W. (1996). Metazoan complexity and evolution: is there a trend? Evolution 50, 477–492. doi: 10.2307/2410824
McShea, D. W. (2012). Upper-directed systems: a new approach to teleology in biology. Biol. Philos. 27, 663–684. doi: 10.1007/s10539-012-9326-2
McShea, D. W., and Brandon, R. N. (2010). Biology's First Law: The Tendency for Diversity and Complexity to Increase in Evolutionary Systems. Chicago: The University of Chicago Press.
Moen, D. S., Morlon, H., and Wiens, J. J. (2016). Testing convergence vs. history: convergence dominates phenotypic evolution for over 150 million years in frogs. Syst. Biol. 65, 146–160. doi: 10.1093/sysbio/syv073
Morris, M. R. J. (2014). Plasticity-mediated persistence in new and changing environments. Int. J. Evol. Biol. 2014, 1–18. doi: 10.1155/2014/416497
Narita, Y., and Kuratani, S. (2005). Evolution of the vertebral formulae in mammals: a perspective on developmental constraints. J. Exp. Zool. B Mol. Dev. Evol. 304B, 91–106. doi: 10.1002/jez.b.21029
Olberding, J. P., Herrel, A., Higham, T. E., and Garland, T. (2016). Limb segment contributions to the evolution of hind limb length in phrynosomatid lizards. Biol. J. Linn. Soc. 117, 775–795. doi: 10.1111/bij.12709
Olson, M. E. (2012). The developmental renaissance in adaptationism. TREE 27, 278–287. doi: 10.1016/j.tree.2011.12.005
Olson, M. E., and Arroyo-Santos, A. (2015). How to study adaptations (and why to do it that way). Quat. Rev. Biol. 90, 167–191. doi: 10.1086/681438
Omland, K. E., Cook, L. G., and Crisp, M. D. (2008). Tree thinking for all biology: the problem with reading phylogenies as ladders of progress. Bioessays 30, 854–867. doi: 10.1002/bies.20794
Osborn, H. F. (1929). The titanotheres of ancient wyoming, Dakota, and Nebraska, United States. Geolog. Survey Monogr. 55.
Perez, S. I., Klaczko, J., Rocatti, G., and Dos Reis, S. F. (2011). Patterns of cranial shape diversification during the phylogenetic branching process of New World monkeys (Primates: Platyrrhini). J. Evol. Biol. 24, 1826–1835. doi: 10.1111/j.1420-9101.2011.02309.x
Perez-Barberia, F. J., and Gordon, I. J. (1999). The functional relationship between feeding type and jaw and cranial morphology in ungulates. Oecologia 118, 157–165. doi: 10.1007/s004420050714
Pigliucci, M., and Müller, G. B. (eds.). (2010). Evolution—the Extended Synthesis. Cambridge: MIT Press.
Poe, S. (2005). A study of the utility of convergent characters for phylogeny reconstruction: do ecomorphological characters track evolutionary history in Anolis lizards? Zoology 108, 337–343. doi: 10.1016/j.zool.2005.08.002
Reiss, J. O. (2009). Not by Design: Retiring Darwin's Watchmaker. Berkeley: University of California Press.
Rosslenbroich, B. (2006). The notion of progress in evolutionary biology—the unresolved problem and an empirical suggestion. Biol. Philos. 21, 41–70. doi: 10.1007/s10539-005-0957-4
Ruse, M. (1996). Monad to Man: The Concept of Progress in Evolutionary Biology. Cambridge: Harvard University Press.
Ruse, M. (2003). Darwin and Design—Does Evolution Have a Purpose? Cambridge: Harvard University Press.
Ruse, M. (2013). “From organisms to mechanisms—and halfway back?” in Beyond Mechanism: Putting Life Back into Biology, eds B. G. Henning and A. C. Scarfe. (Lexington: Lexington Books), 409–430.
Ruth, A., Meindl., R. S., Raghanti., M. A., and Lovejoy, C. O. (2016). Locomotor pattern fails to predict foramen magnum angle in marsupials, rodents, and strepsirrhine primates. J. Hum. Evol. 94, 45–52. doi: 10.1016/j.jhevol.2016.01.003
Schlichting, C. D., and Pigliucci, M. (1998). Phenotypic Evolution: A Reaction Norm Perspective. Sunserland: Sinauer Associates.
Schulte, J. A. I. I., Losos, J. B., Cruz, F. B., and Nú-ez, H. (2004). The relationship between morphology, escape behavior, and microhabitat occupation in the iguanid lizard genus Liolaemus. J. Evol. Biol. 17, 48–420. doi: 10.1046/j.1420-9101.2003.00659.x
Tulli, M. J., Abdala, V., and Cruz, F. B. (2011). Relationships among morphology, clinging performance and habitat use in Liolaemini lizards. J. Evol. Biol. 24, 843–855. doi: 10.1111/j.1420-9101.2010.02218.x
Tulli, M. J., Cruz, F. B., Herrel, A., Vanhooydonck, B., and Abdala, V. (2009). The interplay between claw morphology and habitat use in neotropical iguanian lizards. Zoology 112, 379–392. doi: 10.1016/j.zool.2009.02.001
Tulli, M. J., Herrel, A., Vanhooydonck, B., and Abdala, V. (2012). Is phylogeny driving tendon length in lizards? Acta Zool. 93, 319–329. doi: 10.1111/j.1463-6395.2011.00505.x
Turner, J. S. (2000). The Extended Organism—the Physiology of Animal-Built Structures. Cambridge: Harvard University Press.
Turner, J. S. (2007). The Tinkerer's Accomplice: How Design Emerges from Life Itself. Cambridge: Harvard University Press.
Turner, J. S. (2013). “Biology's second law: homeostasis, purpose and desire,” in Beyond Mechanism: Putting Life Back into Biology, eds B. G. Henning and A. C. Scarfe (Lexington: Lexington Books), 183–204.
Turner, J. S. (2016). Homeostasis and the physiological dimension of niche construction theory in ecology and evolution. Evol. Ecol. 30, 203–219. doi: 10.1007/s10682-015-9795-2
Vanhooydonck, B., and Van Damme, R. (1999). Evolutionary relationships between body shape and habitat use in lacertid lizards. Evol. Ecol. Res. 1, 785–805.
Vidal-García, M., Byrne, P. G., Roberts, J. D., and Keogh, J. S. (2014). The role of phylogeny and ecology in shaping morphology in 21 genera and 127 species of Australo-Papuan myobatrachid frogs. J. Evol. Biol. 27, 181–192. doi: 10.1111/jeb.12292
Vitt, L. J., and Pianka, E. R. (2005). Deep history impacts present-day ecology and biodiversity. Proc. Natl. Acad. Sci. U.S.A. 102, 7877–7881. doi: 10.1073/pnas.0501104102
Wainwright, P. C., Alfaro, M. E., Bolnick, D. I., and Hulsey, D. (2005). Many-to-one mapping of form to function: a general principle in organismal design. Integr. Comp. Biol. 45, 256–262. doi: 10.1093/icb/45.2.256
Wang, X., and Clarke, J. A. (2014). Phylogeny and forelimb disparity in waterbirds. Evolution 68, 2847–2860. doi: 10.1111/evo.12486
Weber, B. H., and Depew, D. J. (eds.). (2003). Evolution and Learning: The Baldwin Effect Reconsidered. Cambridge: MIT Press.
West-Eberhard, M. J. (2003). Developmental Plasticity and Evolution. Oxford: Oxford University Press.
West-Eberhard, M. J. (2014). Darwin's forgotten idea: the social essence of sexual selection. Neurosci. Biobehav. Rev. 46, 501–508. doi: 10.1016/j.neubiorev.2014.06.015
Westneat, D. F., and Fox, C. W. (eds.). (2010). Evolutionary Behavioral Ecology. New York, NY: Oxford University Press.
Wiens, J. J., Sparreboom, M., and Arntzen, J. W. (2011). Crest evolution in newts: implications for reconstruction methods, sexual selection, phenotypic plasticity and the origin of novelties. J. Evol. Biol. 24, 2073–2086. doi: 10.1111/j.1420-9101.2011.02340.x
Young, J. L. (2013). The Baldwin effect and the persistent problem of preformation vs. epigenesis. New Ideas Psychol. 31, 356–362. doi: 10.1016/j.newideapsych.2012.07.002
Keywords: developmental constraints, phylogeny and ecology, ecomorphology, behavior, organic selection, natura selection, evolution, niche construction
Citation: Diogo R (2017) Etho-Eco-Morphological Mismatches, an Overlooked Phenomenon in Ecology, Evolution and Evo-Devo That Supports ONCE (Organic Nonoptimal Constrained Evolution) and the Key Evolutionary Role of Organismal Behavior. Front. Ecol. Evol. 5:3. doi: 10.3389/fevo.2017.00003
Received: 18 October 2016; Accepted: 16 January 2017;
Published: 01 February 2017.
Edited by:
Giuseppe Fusco, University of Padua, ItalyCopyright © 2017 Diogo. This is an open-access article distributed under the terms of the Creative Commons Attribution License (CC BY). The use, distribution or reproduction in other forums is permitted, provided the original author(s) or licensor are credited and that the original publication in this journal is cited, in accordance with accepted academic practice. No use, distribution or reproduction is permitted which does not comply with these terms.
*Correspondence: Rui Diogo, cnVpLmRpb2dvQGhvd2FyZC5lZHU=
Disclaimer: All claims expressed in this article are solely those of the authors and do not necessarily represent those of their affiliated organizations, or those of the publisher, the editors and the reviewers. Any product that may be evaluated in this article or claim that may be made by its manufacturer is not guaranteed or endorsed by the publisher.
Research integrity at Frontiers
Learn more about the work of our research integrity team to safeguard the quality of each article we publish.