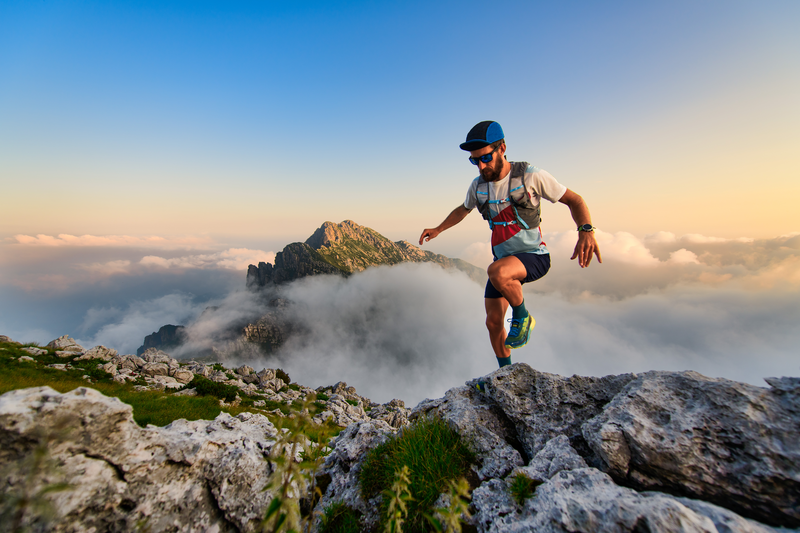
94% of researchers rate our articles as excellent or good
Learn more about the work of our research integrity team to safeguard the quality of each article we publish.
Find out more
HYPOTHESIS AND THEORY article
Front. Ecol. Evol. , 26 October 2016
Sec. Behavioral and Evolutionary Ecology
Volume 4 - 2016 | https://doi.org/10.3389/fevo.2016.00124
This article is part of the Research Topic The Importance of Olfaction in Intra- and Interspecific Communication View all 7 articles
Non-lethal management of wildlife, both “problem wildlife” and pest species, to protect crops and threatened species is becoming increasingly important as non-human animals and humans come into closer proximity. A particularly promising approach is to apply predator scents to manipulate the cost/benefit ratio that influences the behavioral decisions made by prey and other predators about where to forage or rest. However, such olfactory manipulations are not always successful. Using insights from size-structured food webs, we develop a novel integrative model of the information that animals acquire from eavesdropping on predator and conspecific scents. We show how animals can use the information content in predator scents to derive knowledge of other predators and competitors and thus influence their decision to stay in or leave an area. This model framework clarifies how predator scents can influence all trophic levels, from interference competition directed at smaller predators, to predation and herbivory, and exploits direct and indirect pathways to promote landscapes of fear that influence spatial and temporal patch use in target animals. We illustrate how the application of this conceptual model can focus future research to enhance the use of predator scent-based deterrents in conservation and management. This integrated model shows great promise for addressing wildlife management concerns and for eventually improving the success and efficacy of traditional management techniques.
Most mammals are richly endowed with olfactory receptors that enable them to perceive the complex, multidimensional chemical environment of the natural world (Buck and Axel, 1991). Scents are deposited continuously over space and time by conspecific and heterospecific competitors and predators, both by deliberate marking and incidentally while traveling through the environment. This effectively creates an “odorama” through which animals perceive much potential information about their surroundings (e.g., see Banks et al., 2016). Animals often use this information to make biologically important decisions such as where to feed and move (Hughes et al., 2010), a trait exploited in wildlife management to alter animal decision making.
Traditionally, over-abundant populations are managed by targeted removal, contraception or fencing to protect specific areas. Focusing on individual target species can have unintended—and often unwanted—consequences for other species and ecological processes in the broader community. This is particularly true in complex assemblages of invasive species, which often occur through indirect suppression or promotion of competition (Ruscoe et al., 2011). Because of the issues arising from numerical disruption of target populations and increasing pressure to adopt non-lethal means to manage mammalian pests and other “problematic” wildlife (Rose, 2011), there is considerable interest in using olfactory cues, which we will refer to as “scents” (Maynard Smith and Harper, 1995), to modify herbivore or predator behavior and thereby reduce deleterious impacts of wildlife. For example, predator scents can be used to repel prey from crops and other high-value sites as well as to modify predator patch use and habitat selection. One specific example is where browsing of seedlings by snowshoe hares (Lepus americanus) can be suppressed by applying compounds from the anal glands of large mustelid predators to the seedlings (Sullivan and Crump, 1984). There have been some successes, but many failures, in developing agents to transmit biologically meaningful predator cues in a predictable context (Banks et al., 2014). Failure is more likely when isolating a single target molecule from complex scents (Apfelbach et al., 2015) or when using synthetic mimics that approximate, but do not fully replicate, the composite signal within scent derivatives (Burwash et al., 1998). Constituent failures can be beneficial as they help identify the stages when a scent is least active (Parsons et al., 2012). As we will demonstrate in this paper, however, failure could also arise from the trophic context of the scent that is interpreted by the target species. And in this case, it is not the integrity of the scent that matters, but rather the context.
Here, we develop several models that integrate knowledge of the information content of predator scents with information about the food web that animals occupy. We then use these models to generate novel predictions about how information from scents affects patch choice decisions. We demonstrate how our models will be useful to both conservation biologists and wildlife managers to present biologically meaningful predator cues to target species in a predictable and meaningful way.
Predators produce an array of scents that potential prey may “eavesdrop” (Goodale et al., 2010; Stevens, 2013). Most research on predator scents has focused on urine and feces as scent marks that may convey information about sex, social status, territory ownership, and even individual signatures (Scordato et al., 2007) to conspecifics as well as to potential heterospecific predators (Apfelbach et al., 2005). Scent marks can also be created by depositing oily secretions on objects (such as rocks or vegetation) from specific scent-producing glands. But predators also leave other clues to their current presence or previous visitations. This information, such as the time of last visitation of the signaling predator, is most likely conveyed by the dynamic aging and degrading of chemical constituents within complex scents (Apps et al., 2013). Many species have scent-producing glands on their feet that leave trace trails of their movements, and others shed fur that contains odorous compounds from skin glands (Stumpf et al., 2004; May et al., 2012). Predator nesting or resting points also accumulate body scents and secretions that signal their local presence (Macdonald, 1985).
The potential meaning that prey infer from these chemical cues depends upon the spatial and temporal association they have with predator presence (Dickman, 1992) and the strength of selection favoring their perception. For prey to perceive a signal from a predator scent, perceiving meaning detection with subsequent modification of behavior, the predation costs of not perceiving the signal must be greater than the opportunity costs of changing behavior. This in turn may inform the best strategies to reduce predation risk during the different stages of the sequence of predator-prey encounter (Endler, 1991). Urine and feces produce concentrated scents, and these excrements and their associated odors persist in the environment for some time. In the short term, they could signal immediate risk of an encounter with the predator that left the scent, although this is most likely when they are fresh. Aged fecal or urinary scents are physically substantive and may entice animals to approach them to extract further information (Osada et al., 2008), while aged integumentary scents may dissipate more rapidly and completely and lead to less inspection. Over the life of their presence in the environment, urine and feces have low spatial and temporal association with predator presence, and hence signal a lower level of risk of an encounter relative to other predatory cues (e.g., integumentary scents), because they mostly provide information about where an animal was, not where it currently is Hughes et al. (2012). However, urine and feces are often patchily deposited in the landscape, frequently with the purpose of attracting the interest of other passing predators (e.g., as seen in the classic study of Peters and Mech, 1975). Such deposits therefore signal some increased likelihood of encountering any predator, more when they are freshly deposited, but are less likely to favor a change in behavior in prey. These cues might function best for prey early in the predator detection and evasion phases, and the information obtained will then have the greatest influence on reducing the risk of predation (Bytheway et al., 2013). By contrast, integumentary scents from the body, feathers, or fur indicate that there is either a higher risk of immediate predator encounter or that a predator is present nearby, either active or resting. Thus, prey are more likely to perceive the signal and change their behavior. For example, integumentary scents from cat fur and skin elicit a more complete pattern of defensive and emotional behaviors in rats than do scents derived from feces, suggesting that they have greater predictive value for the presence of the predator (Blanchard et al., 2003). Such cues are likely to function later in the predation sequence—during the capture and subjugation phases—and invoke immediate but transient responses that might not be as useful in reducing risk of predator encounter as they are in reducing risk of subjugation and consumption by a predator (Bytheway et al., 2013).
But predator scents contain more than potential information about the presence of a given species of predator; they may contain information about the size of that predator (Cox et al., 2010; Sharp et al., 2015), the presence of other species of predators that are attracted to eavesdrop on the odor of heterospecifics, as well as the likelihood of encountering competitors (Hughes et al., 2012; Banks et al., 2016; Garvey et al., 2016), their parasites (Sharp et al., 2015), and information on resource distribution (Lee et al., 2016). For example, predators are attracted to the scent left by heterospecifics, particularly those of a larger predator, and may benefit from exploitative eavesdropping both for assessing risk of predation and for locating resources (Garvey et al., 2016). Likewise macrosmatic prey species (Apfelbach et al., 2005), those having a good sense of smell, can benefit from eavesdropping on the scent cues of conspecifics and heterospecifics, although this does not mean that they can perceive all scents left behind by predators or prey. For example field voles (Microtus agrestis) use scent cues of competing sibling voles (M. rossiameridionalis) to assess their risk from a shared predator, least weasels (Mustela nivalis; Hughes et al., 2010) and cattle lower their vigilance when they perceive deer scents (Kluever et al., 2009).
Such transtrophic flows of information have been proposed to influence food webs mainly via alterations in the abundance of predators and prey (Vos et al., 2006). However, because olfactory information contains general odors such as carnivore metabolic breakdown products as well as more specific odors (e.g., Cooper et al., 1990) they are open to eavesdropping by a wide range of community members. Thus, there are more subtle but much broader implications for the influence of semiochemicals (i.e., chemicals that have a signaling quality and can modify the behavior of the receiver) contained in scents (Vet, 1999; Goodale et al., 2010). In size-structured communities, for example, the elimination of a large dominant predator such as a wolf or dingo may result in increases of medium-sized predators, such as coyotes or foxes, that then persecute smaller predators and prey (Ripple et al., 2014). Thus, a prey individual that has information about the presence of a larger predator also has potential information about the likelihood that it will encounter a medium-sized or smaller predator. Furthermore, prey may also derive information about competitors from predator scent since changes in predator numbers influence prey abundance and thereby the potential for competitive interactions among prey. This suggests that greater understanding of the ecological relationships within communities is essential to enable us to predict the outcome of using predator scents to manipulate the behaviors of both predators and prey.
Olfactory cues are already used in a variety of situations to either modify problem behaviors (Sparrow et al., 2016) or manipulate the presence of a targeted prey species. These include aversive conditioning, which may be reinforced by sensitization (Blumstein, 2016), and may also benefit through the multimodal use of olfactory as well as acoustic and visual stimuli (Munoz and Blumstein, 2012) to modify space use or lure animals to specific locations. For mammals, most of which have highly developed olfactory abilities (Box 1) and live in environments that are replete with complex and ever-changing arrays of semiochemicals (Box 2), this approach is especially promising. As we elaborate below, intriguingly powerful and complex suites of interactions may be unleashed by the use of stable and time-enduring predator scents (urine and feces), because of how the information they contain is likely to be interpreted by other players in the system.
Box 1. The main and accessory olfactory systems and animal responses to heterospecific scents.
Most mammals have a main olfactory system for the perception of scents and flavors, and an accessory olfactory system that detects specialized conspecific and heterospecific chemical cues (i.e., pheromones and kairomones) that have special relevance to survival and reproduction (Ma, 2012). The main olfactory receptors are located at the roof of the nasal cavity and are most accessible to volatile compounds. The accessory olfactory receptors are located in the vomeronasal organ (VNO), a specialized tubular sensory organ that can aspirate liquids (Døving and Trotier, 1998), enabling non-volatiles such as peptides to be detected in solution (Brennan and Kendrick, 2006). Recent research indicates that the two systems overlap to some extent and act synergistically to provide a complex chemosensory picture (Mucignat-Caretta et al., 2012). In rodents, the main olfactory system involves an array of more than 1200 different types of olfactory receptors located on sensory neurons in the main olfactory epithelium. This sensor array feeds a complex glomerular system in the main olfactory bulb (MOB), which then projects to cortical regions that perform integration, recognition and learning-related functions involving scents. The accessory olfactory system, by comparison, is much simpler. The vomeronasal organ expresses receptors that have evolved narrow sensitivity to chemical cues of species-specific importance. The VNO contains pheromone and kairomone receptors on its sensory neurons that project to the accessory olfactory bulb (AOB), which is located toward the rear and top of the MOB. There is little if any complex cortical processing of VNO and AOB outputs: rather, direct projections to the amygdala and hypothalamus from the AOB allow pheromones and kairomones to rapidly release stereotyped behavioral responses. The kairomone hypothesis of predator scent (Ben-Shaul et al., 2010) suggests that predator scents are preferentially processed by the accessory olfactory system rather than the main olfactory system. This is supported by observations that specific VNO receptors (known as V2Rs) are highly tuned to detect heterospecific predator-related cues (Isogai et al., 2011), and by observations that such cues activate the AOB to a greater extent than the MOB. On the other hand, there is evidence that urine and feces-derived predator-related stimuli activate the MOB as well as the AOB. It may be then that both the main and accessory olfactory systems are necessary for full processing of such cues, and for the presence and identity of local predators to be perceived. Future research will better establish the molecular components of predator-derived stimuli (from fur, skin, urine, feces, anal glands etc.) that activate V2Rs and elicit defensive responses. The development of in vitro model systems, where specific V2Rs are expressed in recombinant cells and interrogated with predator-derived stimuli, may hasten this. Identification of the relevant kairomone compounds may allow commercial development of repellent compounds that could have great utility in wildlife management.
Box 2. Prospects for pharmacological interventions in scent reception: can sensitivity to predator and prey scent be antagonized?
Scents are molecules that bind to and activate receptors on sensory neurons in the olfactory epithelia. In mammals, these receptors are located in the main olfactory system and the vomeronasal organ (Box 1). Each sensory neuron expresses one receptor type, which can bind any molecule which fits the receptor. Each activated receptor transmits an electrical signal to glomeruli in the olfactory bulb. Initial information processing takes place in the olfactory bulb. The scent of one or more scent molecules is identified by a characteristic pattern of activity in the glomeruli (Buck and Axel, 1991). In nature there are many scent molecules, each of which may bind more than one receptor, and the perceived scent is the result of this combination. Scent receptors are beginning to be molecularly characterized. Our emerging knowledge of them creates the opportunity to design novel chemicals that can reversibly modify scent perception. Importantly, this will avoid the toxicity and permanent damage created by existing methods of olfactory ablation (e.g., Rehn et al., 1982). Lectins have been used to selectively and reversibly block scent receptors (Kirner et al., 2003). Such new drugs could be designed for selective or non-selective effects, as well as for short or long-term effects. Blocking one or more scent receptors in a predator could seriously impair its ability to find and even recognize prey. For example, night hunters, such as polecats (Mustela putorius), are particularly dependent on olfactory cues (Apfelbach, 1986). Similarly, black-footed ferrets (Mustela nigripes) are prey specialists that must learn to accept food types during early development, and there is evidence that olfaction is critical to this imprinting (Vargas and Anderson, 1996; Liman and Dulac, 2007). There is tremendous unrealized potential to capitalize on these developments to manipulate the olfactory experiences of both predators and prey and in turn influence both direct and indirect ecological relationships with other species in the food web.
Our model (Figure 1) links the information that prey acquire from predators with the ecological relationships that link predators with their prey, and predators with other predators. Animals base patch choice decisions on perceived predatory cues (Lima and Dill, 1990) and occasionally parasitism cues (Sharp et al., 2015). Individuals must then discriminate between stimuli that derive from predatory and non-predatory animals, as well as potentially distinguish different predators that represent different levels of risk of predation to create an informational state which is the individual's assessment of its predation risk (Blumstein and Bouskila, 1996).
Figure 1. The information that prey gain about the fear-based ecological relationships in a food web when the odor of a predator is applied as a management tool to influence the behavior of, or to protect, prey. Solid black lines indicate the direction of negative direct effects of all natural predatory and competitive relationships in the food web. Information derived from predator scents is indicated by colored lines. These can include information about direct effects (solid lines) and also indirect effects (presented as dashed lines), the latter which can be both positive (green) and negative (red). Indirect effects, in which the target scent changes the local abundance or behavior of another species which then results in altered risk for a third species, can cascade through several trophic interactions and can even come full circle to influence the source. Black squares indicate the source of the scent used in a management context, blue circles the intended recipient, green hexagons small predators, and medium prey which indirectly and possibly unintentionally profit from the application of scents, and finally red hexagons which show potential unintended negative consequences of management action. Predator scents are applied to influence the behavior of (A) large prey (i) of a large predator (I); (B) small prey (iii) of a small predator (III); (C) medium-sized prey (ii) of a medium-sized predator (II); (D) a small predator (III), by applying the scent of a medium-size predator (II), to protect small prey (iii).
Upon detecting a predator's scent, an individual could assess its relative risk of predation from that predator. But other potential information is contained in that scent as well. Prey could assess potential information about the competitive relationships between predators and possibly the effects that predators have on other prey species with which they directly compete. For instance, small mammals commonly show aversive responses to the fecal or urinary scents from house cats (Felis catus) when cats are the sole or dominant predator (Takahashi et al., 2005), but may show no response if cats occur with larger predators that suppress cat activity and also pose a lesser threat to small mammals (Lazenby and Dickman, 2013). Thus, the response of a macrosmatic prey species to a predator's scent will vary with first, its ability to perceive that scent, and if it can detect and respond then with both with the (perceived) relative abundance of this prey species, the relative abundance of other prey species, the strength of competitive interactions between different prey species, and also their relative attractiveness to the predator. We elaborate on these links below.
The same information thus does not lead inevitably to the same behavioral response, and differences in informational state may or may not lead to effectively different antipredator behaviors (Blumstein and Bouskila, 1996; Owen et al., 2016). Variation in response to the same informational state could arise by several means. For instance, individuals may detect a predator's odor, classify it as predatory and increase their assessment of risk, yet not respond overtly by leaving a patch if there are other compelling reasons to remain there (Shimoda and Dicke, 2000). Alternatively, they may be in poor body condition and thus accept a higher risk of predation by remaining in the patch and trade this off against the risk of starvation.
Our model, however, suggests another mechanism that could lead to variable responses: indirect trophic effects suppress or enhance an overt response by modifying the informational state. We illustrate this by considering several different scenarios that focus on the potential consequences of presenting a predator scent to prey in different positions in size-structured guilds. We also illustrate the consequences of directly presenting predatory scents to predators in these size-structured guilds.
The first scenario we consider is the consequence of presenting the scent of a large and dominant predator (I) to a large prey species (i) such as a large ungulate or macropodid marsupial (Figure 1A). In this case, the ecological effects are relatively straightforward—the scent should directly trigger antipredator behavior by the prey (Shrader et al., 2008). However, additional information about risk may be inferred by an individual of a large prey species that detects a predator's odor (Fishman, 1999; Apfelbach et al., 2015). Our model facilitates identification of the consequences of this information.
If the presence of a large predator (I) competitively suppresses medium-sized predators (II), medium-sized prey (ii), which are hunted by the medium-sized predator, may experience predatory release. Their consequent increase in numbers could, in turn, increase resource competition with the large prey (I). In this indirect pathway that affects multiple levels of trophic interactions (Figure 1A), a prey individual that detects a predator's scent could infer information about the competitive relationships within the prey guild. This additional information may influence the patch choice response of both large (i) and medium-sized (ii) prey. In the case of the large prey, both of these effects, direct and indirect, are negative and should increase the informational state about the costs of remaining in a patch and hence induce patch abandonment.
In the second scenario, where the predator (III) and prey (iii) are the smallest species in a food web, interactions that affect the prey are more complex. The smallest prey species (iii) will experience greater overall risk of predation than the largest prey species (i) because it is at risk from both its dominant small predator (III) and the next largest predator (II) (Figure 1B). Thus, it would be advantageous for the smallest prey species (iii) to eavesdrop on information about the threats of predation and competition from their dominant (III) and next larger predator (II) as well as competitors. If scents from the smallest predator are prevalent in the landscape, they provides information about high direct predation risk from the dominant small predator (III) but indirectly lower predation risk from the medium-sized predator (II); the latter is likely to be rare because the small predator (III) is not being suppressed. The reverse is also likely to be true, with small prey (iii) potentially perceiving indirect net benefits to remaining in a patch if scents from the mid-sized predator (II) are most prevalent in the landscape. Thus, there are competing trade-offs in the information on overall and specific predation risk from the small and medium-sized predators to be derived from the scents from the small predator. As is the case for the large predator (I), prevalence of the scents from the small predator (III) will contain relevant information on the likely reduced suppressive effect of the medium-sized predator (II) on the medium-sized prey (ii), and thus increased competition from other herbivores. In more complex situations such as this and subsequent scenarios, there is an assumption implicit in the effect that prevalence of a predator scent might have on the target species; that there is a reasonably tight relationship between the amount of scent and the number of predators, or that receivers can identify individuals from their scent (true at least for mice, see Hurst and Beynon, 2004) and therefore assess the number of different predators, or both.
Now consider a medium-sized prey and a medium-sized predator. Both are positioned in the middle of a food web, and both experience competition from larger and smaller guild members as well as other medium sized predators and prey, respectively, with the prey experiencing predation from all three-sized predators (Figure 1C). In this scenario, the medium-sized prey (ii) will receive direct information on predation risk from the scents from its major medium-sized predator/s (II). In addition, it will gain indirect information about both the larger (I) and the smaller (III) predators which influence the total predatory pressure it experiences, as well as information about the larger (i), other medium-size (ii), and smaller (iii) prey with which it competes. This indirect information can be derived both from assessing the prevalence of the scent of the larger predator and potentially also from meeting other eavesdroppers at the point of contact with the predator scent, as all wildlife interested in eavesdropping will be attracted to investigate the scent (Hughes et al., 2012; Banks et al., 2016). Thus, if the medium-sized predator (II) is common, then the larger predator (I) is likely rare since it is not suppressing the middle predator (II), and the smaller predator (III) is also rare as the middle predator (II) is sufficiently abundant to suppress its populations or activity. Lower abundances of the larger (I) and smaller (III) predator will reduce secondary predation pressure on the medium-sized prey (ii) and will also have lower suppressive effects on the larger (i) and smaller (iii) herbivores, their dominant prey, respectively. Thus, individuals from the medium-sized prey species (ii) are likely to experience lower secondary predation from other predators but greater overall competition from other herbivores, both larger (i) and smaller (iii) that are released from predation pressure. This information will result in complex trade-offs in patch selection decisions in the vicinity of the point of contact with the predator scents.
Our approach suggests that, compared with larger and smaller species, predators, and prey positioned in the middle of a food web live in a more complex olfactory landscape in which eavesdropping information from the major predator potentially contains a complex web of information about other predator and competitor states in the system. The management implications of this are clear: managers working with species in simple food webs, or with species that lie on the periphery of the web, are likely to have most success in directly manipulating prey behavior with predator scents.
Predator scents should also influence the patch choice decisions of other predators and, via indirect food web interactions such as trophic cascades, protect even smaller predators or prey species. This process represents an interaction of trophic and informational cascades. In one example of how this could work, the scent of a large predator (I) can influence the patch choice of a mid-sized predator (II) and, by eliciting the cascading effects of competition and predation of this predator on even smaller predators (III) and its major mid-sized prey (ii), protect small predators (III) and mid-sized prey species (ii) for conservation (Figure 1D). Managers could thus alter the patch use decisions of the mid-sized predator (II) by providing information indicating an increased level of risk of interference competition from the large predator (I), which should influence its decision to vacate a habitat patch (Mitchell and Banks, 2005; Leo et al., 2015). This will release the smallest predator (III) and mid-sized prey (ii) from competition and predation, respectively, by the mid-sized predator (II) without increasing secondary predation pressure from the larger predator (I). It could, however, result in increased predation from the small predator (III) on both the mid-sized (ii) and small prey (iii), and increased competition from the mid-sized prey (ii) on the small prey (iii); using our model can alert managers to these types of unintended consequences of management actions.
This trophic model can be used to identify potential unintended consequences of predator odor manipulations that result from olfactory eavesdropping among species to gain information about indirect effects in complex webs of ecological interactions. Further, novel insights for management can be gained from integrating informational and ecological networks.
Bottom-up influences such as resource variability, specifically of food, and the structural complexity of vegetation that provides refuge from predators, will influence interaction strengths. Thus, management actions should be timed to exploit temporal and spatial weaknesses in the system when animals already assess high risk or when they are seeking a place to settle following migration and where interventions that increase assessment of risk might be particularly effective. This could potentially allow managers to engineer situations where the cost of staying in a patch that has been treated with a repellent scent is outweighed by the benefits of leaving the patch. This approach may improve the efficacy of management. For example, using scent to camouflage prey produces the strongest effects when alternative prey are available (Carthey et al., 2011).
Food web complexity may be an unintended management ally if it removes the predictability of cues from the system, because predictability accelerates habituation to scent cues and reduces the efficacy of management (Bytheway et al., 2013). In complex food webs, the greater number of scents in the landscape and possibilities for direct and indirect interactions could make it difficult to reliably interpret olfactory cues (Vos et al., 2006). Indeed, some larger felids seemingly account for this information when they modify the composition (Burger et al., 2006), or deposition of scents (Vogt et al., 2016) in response to prey activity.
Predator sociality may influence risk perception. With solitary predators, use of scent from multiple individuals is predicted to increase signal strength and enhance both direct effects and information about indirect trophic interactions. Conversely, while increasing the amount or freshness of a scent from a single individual predator may be interpreted as a higher immediate risk of predation, it is less likely to influence perceptions about other predators and prey in the web. The presence of multiple individual predators should create a riskier situation for prey than the presence of a single predator, and prey often respond by increasing apprehension or reducing activity when they detect multiple predators (Martin et al., 2010). This is a particularly important consideration when attempting to synthetize a predator scent, because a synthetic analog should attempt to mimic the intensity of multiple predators.
This effect is mediated by predator sociality. Thus, the same information may alter signal strength in the context of a social predator if multiple individuals hunt together and predation risk is concentrated spatially. Therefore, to optimize the perceived risk, managers should use scents from several different individuals unrelated to a resident predator or group of predators, and routinely refresh the scent with new voids of natural rather than synthetic analogs of scent if possible, as these will more closely mimic natural predator scents. If by doing so, predators are attracted to the scent, both direct and indirect effects would be enhanced.
Prey sociality may also influence risk perception. Prey foraging in groups rather than alone may experience reduced risk perception (Carthey and Banks, 2015). Indeed, a conserved behavioral response of prey species to a predator scent is huddling (defensive aggregation) which serves to dilute individual risk of predation (Bowen et al., 2013). Alternatively, prey may transmit information on risk collectively (e.g., by alarm calling), and thus amplify the perception of risk. Understanding the net polarity and strength of these orthogonal effects mediated through both direct and indirect effects may influence the perception of risk interpreted from the signal.
We believe that by adopting this new odorama-based framework, practitioners will enhance the success rate and efficacy of managing wildlife using predator scents. Several avenues of inquiry will further develop the utility of this framework.
To improve the targeting of scents and the precision of their effects, we suggest further research into the role of semiochemicals at the biological, chemical, and bioassay levels (e.g., Apps et al., 2013). We also need to ensure that we have sufficiently sensitive bioassays to reveal the true information content and extent of inferences that animals can make across ecological networks.
For effective large-scale wildlife management, the production of synthetic scents is likely to be required, and these need to be created to contain the context-specific chemical components of signals that provide appropriate information targeted to the specific predator and prey system. This will be a difficult challenge, since mammalian scents are typically complex mixtures (Burger, 2005) and the behavioral responses to all or some of the constituent odorants have generally yet to be studied. To maximize testing and implementation of new management tools, all experiments should be conducted under an adaptive management framework—“learning by doing” (Walters and Holling, 1990). Hand-held odor instruments can be used to detect whether a signal is changing during a course of treatment and will provide managers with a mechanism to understand net polarity and strength of the scent signal.
Our framework opens up several new and intriguing lines of inquiry. In identifying the need to develop a more nuanced view of the information contained in potential signals, we also recognize the need to better understand the neurophysiological mechanisms mediating behavioral responses. We also recognize that while a model is by definition broadly generic, species vary in their reliance on olfactory cues, and we need to understand how ecology influences the sensitivity of different species to predator scents.
We need to explore the distinction between chemicals that target the primary olfactory system and the vomeronasal organ (VNO). Do chemicals that differentially target the primary olfactory system and the VNO vary in their efficacy? Do they create different perceptions of risk? And, do chemicals that target one vs. the other differentially influence the strength of the direct and indirect relationships? Can we block the VNO or olfactory system (Box 2) and, by doing so, specifically manipulate the information prey have about predators and conspecifics?
An open question is how many indirect ecological links animals can deduce from a predator's odor. We know that animals acquire information about more than simply the presence of a predator, and that this information can change decisions about patch use (Shimoda and Dicke, 2000). But what is the strength of indirect effects potentiated by acquiring olfactory predator information, and how does the strength of these effects vary based on the specific information acquired? Elegant experiments in controlled but natural environments are required to test this.
Vital to the method's success as a management tool across ecological networks at a variety of scales is to test how information acquired through multiple trophic steps varies with spatial and temporal scale of scent application, and how quickly prey habituate to predator scents in the absence (or sufficient abundance) of other predator cues.
Grounding wildlife management within a framework of integrated ecological and informational networks holds much promise for improving success rate of olfactory repellent and deterrent use and efficacy over traditional management techniques. Working in nature, with a detailed knowledge of system linkages, allows exploitation of leverage points, those places within a system where a small shift in one place can result in larger shifts overall (Meadows, 1999). This is a cost-effective and potentially more humane way of managing over-abundant or problem-causing species or, in other cases, conserving threatened ones.
All authors substantially contributed to the conception of the work during a workshop; MJ, DB, CD, MP, AF, DM, PB, SM, IM substantially drafted the manuscript; all authors contributed to revision; MJ, DB edited the manuscript; all authors approved the final version.
The authors declare that the research was conducted in the absence of any commercial or financial relationships that could be construed as a potential conflict of interest.
The authors acknowledge the support of a University of Tasmania Group Career Development Scholarship to EC that funded the international workshop during which the manuscript was conceived and drafted. We also thank the two reviewers who provided insightful and constructive comments helping us to improve the message.
Apfelbach, R. (1986). Imprinting on prey odours in ferrets (Mustela putorius F. furo L.) and its neural correlates. Behav. Process. 12, 363–381. doi: 10.1016/0376-6357(86)90005-7
Apfelbach, R., Blanchard, C. D., Blanchard, R. J., Hayes, R. A., and McGregor, I. S. (2005). The effects of predator odors in mammalian prey species: a review of field and laboratory studies. Neurosci. Biobehav. Rev. 29, 1123–1144. doi: 10.1016/j.neubiorev.2005.05.005
Apfelbach, R., Parsons, M. H., Soini, H. A., and Novotny, M. V. (2015). Are single odorous components of a predator sufficient to elicit defensive behaviors in prey species? Front. Neurosci. 9:263. doi: 10.3389/fnins.2015.00263
Apps, P., Mmualefe, L., and Mcnutt, J. W. (2013). “A reverse-engineering approach to identifying which compounds to bioassay for signalling activity in the scent marks of African wild dogs (Lycaon pictus),” in Chemical Signals in Vertebrates 12 eds M. L. East and M. Dehnhard (New York, NY: Springer), 417–432.
Banks, P. B., Bytheway, J. P., Carthey, A. J., Hughes, N. K., and Price, C. J. (2014). “Olfaction and predator-prey interactions amongst mammals in Australia,” in Carnivores of Australia: Past, Present and Future, eds A. S. Glen and C. R. Dickman (Collingwood, VIC: CSIRO Publishing), 389.
Banks, P. B., Daly, A., and Bytheway, J. P. (2016). Predator odours attract other predators creating an olfactory web of information. Biol. Lett. 12:20151053 doi: 10.1098/rsbl.2015.1053
Ben-Shaul, Y., Katz, L. C., Mooney, R., and Dulac, C. (2010). In vivo vomeronasal stimulation reveals sensory encoding of conspecific and allospecific cues by the mouse accessory olfactory bulb. Proc. Natl. Acad. Sci. U.S.A. 107, 5172–5177. doi: 10.1073/pnas.0915147107
Blanchard, D. C., Griebel, G., and Blanchard, R. J. (2003). Conditioning and residual emotionality effects of predator stimuli: some reflections on stress and emotion. Prog. NeuroPsychopharmacol. Biol. Psychiatry 27, 1177–1185. doi: 10.1016/j.pnpbp.2003.09.012
Blumstein, D. T. (2016). Habituation and sensitization: new thoughts about old ideas. Anim. Behav. 120, 255–262. doi: 10.1016/j.anbehav.2016.05.012
Blumstein, D. T., and Bouskila, A. (1996). Assessment and decision making in animals: a mechanistic model underlying behavioral flexibility can prevent ambiguity. Oikos 77, 569–576. doi: 10.2307/3545948
Bowen, M. T., Kevin, R. C., May, M., Staples, L. G., Hunt, G. E., and McGregor, I. S. (2013). Defensive aggregation (huddling) in Rattus norvegicus toward predator odor: individual differences, social buffering effects and neural correlates. PLoS ONE 8:e68483. doi: 10.1371/journal.pone.0068483
Brennan, P. A., and Kendrick, K. M. (2006). Mammalian social odours: attraction and individual recognition. Philos. Trans. R. Soc. B Biol. Sci. 361, 2061–2078. doi: 10.1098/rstb.2006.1931
Buck, L., and Axel, R. (1991). A novel multigene family may encode odorant receptors: a molecular basis for odor recognition. Cell 65, 175–187. doi: 10.1016/0092-8674(91)90418-X
Burger, B. V. (2005). “Mammalian semiochemicals,” in The Chemistry of Pheromones and Other Semiochemicals II, ed S. Schulz (Berlin: Springer Science & Business Media), 231–278.
Burger, B. V., Visser, R., Moses, A., and Le Roux, M. (2006). Elemental sulfur identified in urine of cheetah, Acinonyx jubatus. J. Chem. Ecol. 32, 1347–1352. doi: 10.1007/s10886-006-9056-5
Burwash, M. D., Tobin, M. E., Woolhouse, A. D., and Sullivan, T. P. (1998). Field testing synthetic predator odors for roof rats (Rattus rattus) in Hawaiian macadamia nut orchards. J. Chem. Ecol. 24, 603–630. doi: 10.1023/A:1022304917652
Bytheway, J. P., Carthey, A. J. R., and Banks, P. B. (2013). Risk vs. reward: how predators and prey respond to aging olfactory cues. Behav. Ecol. Sociobiol. 67, 715–725. doi: 10.1007/s00265-013-1494-9
Carthey, A. J. R., and Banks, P. B. (2015). Foraging in groups affects giving-up densities: solo foragers quit sooner. Oecologia 178, 707–713. doi: 10.1007/s00442-015-3274-x
Carthey, A. J., Bytheway, J. P., and Banks, P. B. (2011). Negotiating a noisy, information-rich environment in search of cryptic prey: olfactory predators need patchiness in prey cues. J. Anim. Ecol. 80, 742–752. doi: 10.1111/j.1365-2656.2011.01817.x
Cooper, W. E., Buth, D. G., and Vitt, L. J. (1990). Prey odor discrimination by ingestively naive coachwhip snakes (Masticophis flagellum). Chemoecology 1, 86–91. doi: 10.1007/BF01241648
Cox, T. E., Murray, P. J., Hall, G. P., and Li, X. (2010). Pest responses to odors from predators fed a diet of target species conspecifics and heterospecifics. J. Wildl. Manage. 74, 1737–1744. doi: 10.2193/2009-410
Dickman, C. R. (1992). Predation and habitat shift in the house mouse, Mus domesticus. Ecology 73, 313–322. doi: 10.2307/1938742
Døving, K. B., and Trotier, D. (1998). Structure and function of the vomeronasal organ. J. Exp. Biol. 201, 2913–2925.
Endler, J. A. (1991). Interactions between predators and prey. Behav. Ecol. Evol. Approach 3, 169–196.
Fishman, M. A. (1999). Predator inspection: closer approach as a way to improve assessment of potential threats. J. Theor. Biol. 196, 225–235. doi: 10.1006/jtbi.1998.0834
Garvey, P. M., Glen, A. S., and Pech, R. P. (2016). Dominant predator odour triggers caution and eavesdropping behaviour in a mammalian mesopredator. Behav. Ecol. Sociobiol. 70, 481–492. doi: 10.1007/s00265-016-2063-9
Goodale, E., Beauchamp, G., Magrath, R. D., Nieh, J. C., and Ruxton, G. D. (2010). Interspecific information transfer influences animal community structure. Trends Ecol. Evol. 25, 354–361. doi: 10.1016/j.tree.2010.01.002
Hughes, N. K., Kelley, J. L., and Banks, P. B. (2012). Dangerous liaisons: the predation risks of receiving social signals. Ecol. Lett. 15, 1326–1339. doi: 10.1111/j.1461-0248.2012.01856.x
Hughes, N. K., Korpimäki, E., and Banks, P. B. (2010). The predation risks of interspecific eavesdropping: weasel–vole interactions. Oikos 119, 1210–1216. doi: 10.1111/j.1600-0706.2010.18006.x
Hurst, J. L., and Beynon, R. J. (2004). Scent wars: the chemobiology of competitive signalling in mice. Bioessays 26, 1288–1298. doi: 10.1002/bies.20147
Isogai, Y., Si, S., Pont-Lezica, L., Tan, T., Kapoor, V., Murthy, V. N., et al. (2011). Molecular organization of vomeronasal chemoreception. Nature 478, 241–245. doi: 10.1038/nature10437
Kirner, A., Deutsch, S., Weiler, E., Polak, E. H., and Apfelbach, R. (2003). Concanavalin A application to the olfactory epithelium reveals different sensory neuron populations for the odour pair D- and L-carvone. Behav. Brain Res. 138, 201–206. doi: 10.1016/S0166-4328(02)00242-5
Kluever, B. M., Howery, L. D., Breck, S. W., and Bergman, D. L. (2009). Predator and heterospecific stimuli alter behaviour in cattle. Behav. Process. 81, 85–91. doi: 10.1016/j.beproc.2009.02.004
Lazenby, B. T., and Dickman, C. R. (2013). Patterns of detection and capture are associated with cohabiting predators and prey. PLoS ONE 8:e59846. doi: 10.1371/journal.pone.0059846
Lee, A. E. G., Ounsley, J. P., Coulson, T., Marcus Rowcliffe, J., and Cowlishaw, G. (2016). Information use and resource competition: an integrative framework. Proc. R. Soc. B Biol. Sci. 283:20152550. doi: 10.1098/rspb.2015.2550
Leo, V., Reading, R. P., and Letnic, M. (2015). Interference competition: odours of an apex predator and conspecifics influence resource acquisition by red foxes. Oecologia 179, 1033–1040. doi: 10.1007/s00442-015-3423-2
Lima, S. L., and Dill, L. M. (1990). Behavioral decisions made under the risk of predation: a review and prospectus. Can. J. Zool. 68, 619–640. doi: 10.1139/z90-092
Liman, E. R., and Dulac, C. (2007). “TRPC2 and the molecular biology of pheromone detection in mammals,” in TRP Ion Channel Function in Sensory Transduction and Cellular Signaling Cascades, eds W. B. Liedtke and S. Heller (Boca Raton, FL: CRC Press, Taylor and Francis Group), 45–53.
Ma, M. (2012). Odor and pheromone sensing via chemoreceptors. Adv. Exp. Med. Biol. 739, 93–106. doi: 10.1007/978-1-4614-1704-0_6
Macdonald, D. W. (1985). “The carnivores: order Carnivora,” in Social Odours in Mammals, eds R. E. Brown and D. W. Macdonald (Oxford: Clarendon), 619–722.
Martin, C. W., Fodrie, F. J., Heck, K. L. Jr., and Mattila, J. (2010). Differential habitat use and antipredator response of juvenile roach (Rutilus rutilus) to olfactory and visual cues from multiple predators. Oecologia 162, 893–902. doi: 10.1007/s00442-010-1564-x
May, M. D., Bowen, M. T., McGregor, I. S., and Timberlake, W. (2012). Rubbings deposited by cats elicit defensive behavior in rats. Physiol. Behav. 107, 711–718. doi: 10.1016/j.physbeh.2012.03.007
Maynard Smith, J., and Harper, D. G. (1995). Animal signals: models and terminology. J. Theor. Biol. 177, 305–311. doi: 10.1006/jtbi.1995.0248
Meadows, D. H. (1999). Leverage Points: Places to Intervene in a System. Vermont: The Sustainability Institute).
Mitchell, B. D., and Banks, P. B. (2005). Do wild dogs exclude foxes? Evidence for competition from dietary and spatial overlaps. Aust. Ecol. 30, 581–591. doi: 10.1111/j.1442-9993.2005.01473.x
Mucignat-Caretta, C., Redaelli, M., and Caretta, A. (2012). One nose, one brain: contribution of the main and accessory olfactory system to chemosensation. Front. Neuroanat. 6:46. doi: 10.3389/fnana.2012.00046
Munoz, N. E., and Blumstein, D. T. (2012). Multisensory perception in uncertain environments. Behav. Ecol. 23, 457–462. doi: 10.1093/beheco/arr220
Osada, K., Curran, M., Kurachi, M., Tsunoda, K., and Yamazaki, K. (2008). Effect of an orally ingested mugwort and mushroom extract mixture on urine odor from aged mice. Biosci. Biotechnol. Biochem. 72, 1249–1256. doi: 10.1271/bbb.70718
Owen, M. A., Swaisgood, R. R., and Blumstein, D. T. (2016). Contextual influences on animal decision-making: significance for behavior-based wildlife conservation and management. Integr. Zool. doi: 10.1111/1749-4877.12235. [Epub ahead of print].
Parsons, M. H., Blumstein, D. T., and Dods, K. C. (2012). An in situ vertebrate bioassay helps identify potential matrices for a predator-based synthetic management tool. Wildl. Soc. Bull. 36, 383–388. doi: 10.1002/wsb.144
Rehn, B., Breipohl, W., Naguro, T., and Schmidt, U. (1982). Effect of N-methyl-formimino-methylester on the vomeronasal neuroepithelium of mice. Cell Tissue Res. 225, 465–468. doi: 10.1007/BF00214699
Ripple, W. J., Estes, J. A., Beschta, R. L., Wilmers, C. C., Ritchie, E. G., Hebblewhite, M., et al. (2014). Status and ecological effects of the world's largest carnivores. Science 343:1241484. doi: 10.1126/science.1241484
Rose, M. (2011). Challenges to the development and implementation of public policies to achieve animal welfare outcomes. Animals 1, 69–82. doi: 10.3390/ani1010069
Ruscoe, W. A., Ramsey, D. S. L., Pech, R. P., Sweetapple, P. J., Yockney, I., Barron, M. C., et al. (2011). Unexpected consequences of control: Competitive vs. predator release in a four-species assemblage of invasive mammals. Ecol. Lett. 14, 1035–1042. doi: 10.1111/j.1461-0248.2011.01673.x
Scordato, E. S., Dubay, G., and Drea, C. M. (2007). Chemical composition of scent marks in the ringtailed lemur (Lemur catta): glandular differences, seasonal variation, and individual signatures. Chem. Senses 32, 493–504. doi: 10.1093/chemse/bjm018
Sharp, J. G., Garnick, S., Elgar, M. A., and Coulson, G. (2015). Parasite and predator risk assessment: nuanced use of olfactory cues. Proc. R. Soc. B 282, 20151941. doi: 10.1098/rspb.2015.1941
Shimoda, T., and Dicke, M. (2000). Attraction of a predator to chemical information related to nonprey: when can it be adaptive? Behav. Ecol. 11, 606–613. doi: 10.1093/beheco/11.6.606
Shrader, A. M., Brown, J. S., Kerley, G. I., and Kotler, B. P. (2008). Do free-ranging domestic goats show ‘landscapes of fear’? Patch use in response to habitat features and predator cues. J. Arid Environ. 72, 1811–1819. doi: 10.1016/j.jaridenv.2008.05.004
Sparrow, E., Parsons, M., and Blumstein, D. (2016). Novel use for a predator scent: preliminary data suggest that wombats avoid recolonize collapsed burrows following application of dingo scent. Aust. J. Zool. 64, 192–197. doi: 10.1071/ZO15068
Stumpf, P., Künzle, H., and Welsch, U. (2004). Cutaneous eccrine glands of the foot pads of the small Madagascan tenrec (Echinops telfairi, Insectivora, Tenrecidae): skin glands in a primitive mammal. Cell Tissue Res. 315, 59–70. doi: 10.1007/s00441-003-0815-0
Sullivan, T. P., and Crump, D. R. (1984). Influence of mustelid scent-gland compounds on suppression of feeding by snowshoe hares (Lepus americanus). J. Chem. Ecol. 10, 1809–1821. doi: 10.1007/BF00987363
Takahashi, L. K., Nakashima, B. R., Hong, H., and Watanabe, K. (2005). The smell of danger: a behavioral and neural analysis of predator odor-induced fear. Neurosci. Biobehav. Rev. 29, 1157–1167. doi: 10.1016/j.neubiorev.2005.04.008
Vargas, A., and Anderson, S. H. (1996). Effects of diet on captive black-footed ferret (Mustela nigripes) food preference. Zoo Biol. 15, 105–113.
Vet, L. E. (1999). From chemical to population ecology: infochemical use in an evolutionary context. J. Chem. Ecol. 25, 31–49. doi: 10.1023/A:1020833015559
Vogt, K., Hofer, E., Ryser, A., Kölliker, M., and Breitenmoser, U. (2016). Is there a trade-off between scent marking and hunting behaviour in a stalking predator, the Eurasian lynx, Lynx lynx? Anim. Behav. 117, 59–68. doi: 10.1016/j.anbehav.2016.04.004
Vos, M., Vet, L. E., Wäckers, F. L., Middelburg, J. J., Van Der Putten, W. H., Mooij, W. M., et al. (2006). Infochemicals structure marine, terrestrial and freshwater food webs: implications for ecological informatics. Ecol. Inform. 1, 23–32. doi: 10.1016/j.ecoinf.2005.06.001
Keywords: non-lethal management, predator odor, scent-based deterrents, chemical communication, eavesdropping, food webs
Citation: Jones ME, Apfelbach R, Banks PB, Cameron EZ, Dickman CR, Frank A, McLean S, McGregor IS, Müller-Schwarze D, Parsons MH, Sparrow E and Blumstein DT (2016) A Nose for Death: Integrating Trophic and Informational Networks for Conservation and Management. Front. Ecol. Evol. 4:124. doi: 10.3389/fevo.2016.00124
Received: 28 April 2016; Accepted: 10 October 2016;
Published: 26 October 2016.
Edited by:
Luisa Amo, Spanish National Research Council, SpainReviewed by:
Mark A. Elgar, University of Melbourne, AustraliaCopyright © 2016 Jones, Apfelbach, Banks, Cameron, Dickman, Frank, McLean, McGregor, Müller-Schwarze, Parsons, Sparrow and Blumstein. This is an open-access article distributed under the terms of the Creative Commons Attribution License (CC BY). The use, distribution or reproduction in other forums is permitted, provided the original author(s) or licensor are credited and that the original publication in this journal is cited, in accordance with accepted academic practice. No use, distribution or reproduction is permitted which does not comply with these terms.
*Correspondence: Menna E. Jones, menna.jones@utas.edu.au
Daniel T. Blumstein, marmots@ucla.edu
Disclaimer: All claims expressed in this article are solely those of the authors and do not necessarily represent those of their affiliated organizations, or those of the publisher, the editors and the reviewers. Any product that may be evaluated in this article or claim that may be made by its manufacturer is not guaranteed or endorsed by the publisher.
Research integrity at Frontiers
Learn more about the work of our research integrity team to safeguard the quality of each article we publish.