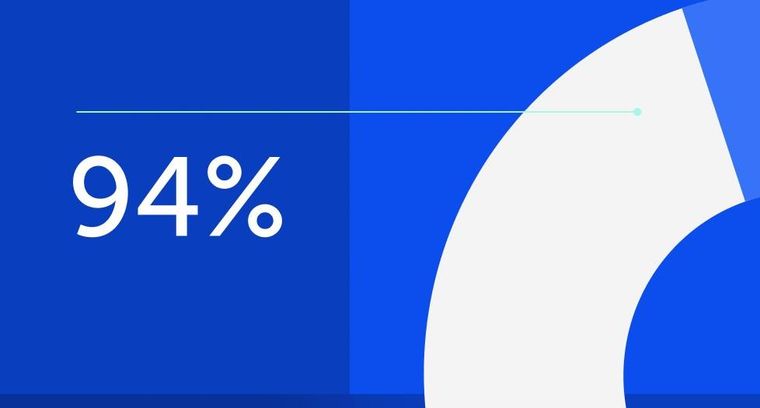
94% of researchers rate our articles as excellent or good
Learn more about the work of our research integrity team to safeguard the quality of each article we publish.
Find out more
MINI REVIEW article
Front. Ecol. Evol., 04 August 2016
Sec. Behavioral and Evolutionary Ecology
Volume 4 - 2016 | https://doi.org/10.3389/fevo.2016.00095
This article is part of the Research TopicOxidative stress and signal honestyView all 7 articles
The capacity to communicate effectively with other individuals plays a critical role in the daily life of an individual and can have important fitness consequences. Animals rely on a number of visual and non-visual signals, whose production brings costs to the individual. The theory of honest signaling states that these costs are higher for low than for high-quality individuals, which prevents cheating and makes signals, such as skin and plumage coloration, indicators of individual's quality or condition. The condition-dependent nature of signals makes them ideally suited as indicators of environmental quality, implying that signal production might be affected by contaminants. In this mini-review article, we have made the point that oxidative stress (OS) is one overlooked mechanism linking exposure to contaminants to signaling because (i) many contaminants can influence the individual's oxidative balance, and (ii) generation of both visual and non-visual signals is sensitive to OS. To this end, we have provided the first comprehensive review on the way both non-organic (heavy metals, especially mercury) and organic [persistent organic pollutants (POPs)] contaminants may influence either OS or sexual signaling. We have also paid special attention to emerging classes of pollutants like brominated flame-retardants (BFRs) and perfluoroalkoxy alkanes (PFAs) in order to stimulate research in this area. We have finally provided suggestions and warnings for future work on the links among OS, sexual signaling, and contaminant exposure.
Work on honest signaling has been a major area of research in animal behavior and evolutionary ecology in recent decades. Honest signals reflect the individual quality, which depends on various interacting factors, such as foraging capability, functionality of the hormonal and immune systems, or oxidative balance (Hill, 2011). In 1999, Von Schantz et al. proposed that the association between oxidative stress (OS) and health might be a primary mechanism linking the expression of sexual ornaments to genetic variation in fitness-related traits, promoting the evolution of female's mate choice and male's sexual ornamentation (von Schantz et al., 1999). OS is a biochemical condition of cells, which occurs when there is an increase in oxidative molecular damage and oxidation of non-protein and protein thiols that regulate the cell oxidative balance. It is now increasingly recognized that OS may have an impact on the whole organism, for example as a modulator of some key life-history trade-offs (Costantini, 2014).
It is sometimes forgotten in ecological studies that some among individuals or species variation in life histories or physiological condition may simply be the by-product of contaminant exposure (Carere et al., 2010). In this context, the role of contaminants in influencing the production of honest signals and, consequently, sexual selection might be relevant. Pioneering work from Møller (1993), Manning and Chamberlain (1994), or Hill (1995) suggested that the condition-dependent nature of ornaments and armaments makes them suited indicators of environmental quality. The majority of the work in this area has been focused on the expression of colorations in birds. Both non-organic and organic contaminants may alter various, often inter-linked, physiological processes, causing changes in endocrine and neuroendocrine pathways (Frye et al., 2011), and oxidative balance (Metodiewa and Dunford, 1990; Whysner and Wang, 2001; Isaksson, 2010). Contaminants might either exacerbate costs of or constrain on physiological pathways underlying the production of honest signals.
In this review we have made the point that OS is an additional but overlooked mechanism linking contaminant accumulation in the body to the expression of sexual signals. OS has been extensively studied in ecotoxicology and this background knowledge can be used as a strong basis to establish mechanisms of action for signaling. We have provided the first comprehensive review of potential pathways through which both non-organic and organic contaminants would influence signaling through the induction of OS. We have also relied on studies of emerging pollutants of global concern for wildlife in order to stimulate research in this area. Although most of the work has been on birds, the implications of the examples provided are not taxon-specific.
Non-organic contaminants can disrupt body colorations (Figure 1A) and induce OS through various mechanisms. For example, metal ions can induce cleavage of peroxides and organic hydroperoxides through the Fenton reaction, leading to the generation of new free radicals that can fuel OS. Metals can also bind to thiol groups, such as the endogenous antioxidant glutathione (Chan et al., 1992; Bridges and Zalups, 2006), inducing oxidation of the thiol without the generation of free radicals. These metals include, for example, cadmium, mercury, lead, and arsenic. For example, mercury has a strong propensity to react with cysteine and reduced glutathione (Stricks and Kolthoff, 1953; Mah and Jalilehvand, 2010) and animals have evolved adaptive mechanisms, in some species confined during specific stages of the life cycle, to actively eliminate such damaging contaminant from the body. For example, in birds, mercury is excreted through its deposition in the feathers during the molt (Monteiro and Furness, 1995). In this pathway, mercury is bound to keratin sulfhydryl groups during synthesis of feathers. Seabirds with slow molt cycles may have, however, a limited capacity for mercury detoxification (Thompson and Furness, 1989). Mercury may also be excreted from the body through the glutathione-pathway. Mercury binds to the thiol group of glutathione, forming a complex that is excreted in the feces (Ballatori and Clarkson, 1985). Sulfhydryl groups like thiols are important molecules that regulate the oxidative balance and any depletion leads to disruption of redox signaling and control, and increase in OS (Jones, 2006). Thus, high contamination with mercury might lead to a high depletion of thiols regardless of the pathway of excretion, which may compromise defenses against oxidation. Depletion of glutathione due to mercury may, for example, compromise the activity of the enzyme glutathione peroxidase (Hoffman and Heinz, 1998), which uses glutathione as a cofactor to detoxify the organism from peroxides and organic hydroperoxides, which is an important pathway through which organisms protect themselves against OS. The activity of selenium-dependent glutathione peroxidase may be further compromised by depletion of selenium that is being used to biosynthesize mineral granules containing mercury and selenium (Nigro and Leonzio, 1996).
Figure 1. (A) Wild male mallards (Anas platyrhynchos) exposed to high concentrations of lead (Pb) had paler carotenoid-based colorations in both legs and beak. Reprinted from Vallverdú-Coll et al. (2016) with permission from John Wiley and Sons; (B) Wild female black-legged kittiwakes (Rissa tridactyla) with higher blood concentrations of persistent organic pollutants (POPs) had paler carotenoid-based colorations in the gapes. Modified from Blévin et al. (2014). Photographs were courteously provided by Frédéric Angelier.
The interaction between mercury and glutathione offers a potential way through which mercury might influence melanin-based colorations (Figures 2A,B). Melanins are pigments that animals synthesize from the aromatic amino acids phenylalanine and tyrosine (Hearing, 1993). Melanins occur in two chemically distinct forms: eumelanin is a brown-black polymer of dihydroxyindole carboxylic acids and their reduced forms; pheomelanin is a cysteine-containing red-brown polymer of benzothiazine units. Melanocyte-stimulating hormones and the adrenocorticotropic hormone regulate the activity of the enzyme tyrosinase, which favors eumelanogenesis to pheomelanogenesis when its activity is high (Ozeki et al., 1997; Benathan et al., 1999). Melanogenesis is also regulated by the availability of thiol groups, such as free cysteine and cysteine-containing peptides (Ozeki et al., 1997; Benathan et al., 1999). Dopaquinone (produced from hydroxylation of the amino acid tyrosine) can react with thiol groups to synthesize pheomelanin or, in the absence of thiol groups, undergo a cyclisation that leads to the synthesis of eumelanin (García-Borrón and Olivares Sαnchez, 2011). Given that thiol compounds like glutathione play an important role in the regulation of cell redox status and protection against oxidative damage, melanin-based ornaments might signal the individual OS level (Galván and Alonso-Alvarez, 2008; Galván and Solano, 2009; Grunst et al., 2014). The property of melanic traits to signal individual OS would be favored by the differentiation in color between eumelanic (black and gray) and pheomelanic (yellowish, reddish, chestnut, and brown) traits (Galván and Alonso-Alvarez, 2008; Galván and Solano, 2009). Production of pheomelanin requires cysteine, which is also needed for the synthesis of glutathione (Galván and Solano, 2009). Thus, there should be a trade-off between the use of cysteine for glutathione and for pheomelanin synthesis. Cysteine-containing peptides like glutathione can also be used in melanogenesis. Thus, when the synthesis of glutathione is not constrained, the organism can maintain high glutathione concentration, while using it for the production of pheomelanins. Using glutathione in melanogensis might indicate that the individual has sufficient alternative antioxidant resources to counteract the decrease in glutathione itself or is not exposed to pro-oxidants (Galván and Alonso-Alvarez, 2008; Galván and Solano, 2009). On the other hand, a decrease in glutathione and, consequently, in cysteine availability would result in increased production of eumelanins. If mercury decreases glutathione availability, we should expect an increase in eumelanin coloration, but this has never been tested experimentally in free-living nor captive animals.
Figure 2. (A) Simplistic diagram of the hypothetical links among heavy metals, oxidative stress, and coloration via the glutathione pathway; (B) Schema of the catalytic cycle for the depletion of the cellular glutathione pool by mercury, proposed on the basis of mass spectrometric behavior, and biochemical evidence (reprinted from (Rubino et al., 2006) with permission from Elsevier); GSH, glutathione; GS-Hg+, mono-S-glutathionyl-mercury(II); Hg, mercury; CSCyGlyOH, oxidized cysteinyl-glycine; Hg+-SCyGlyOH, mercury(II)-cysteinyl-glycine conjugate; Pyr, pyroglutamic acid; RSH, thiols; (C) Simplistic diagram of the hypothetical links among persistent organic pollutants (POPs), oxidative stress, and coloration. Different classes of POPs can generate oxidative stress via binding with specific cellular receptors, including the aryl hydrocarbon receptor (AhR), and proliferator-activated receptor-alpha (PPAR-α); (D) Schema of the effects of dioxin-like pollutants (e.g., co-planar PCBs, PBDEs) via induction of the cytochrome P450 pathway (adapted from Regoli and Giuliani, 2014) which induces uncoupling of the catalytic cycle of the cytochrome P450 1A subfamily allowing the heme iron within the active site of this enzyme complex to undergo cycles of oxidation and reduction, and act as a Fenton catalyst generating free radicals—AhR, aryl hydrocarbon receptor; Ah, aryl hydrocarbon genes (e.g., CYP1A, CYP1B); HSP 90, heat shock protein 90; ARNT, aryl hydrocarbon receptor nuclear translocator; DRE, dioxin responsive element. Different classes of pollutants can act on the same cellular targets altering the cell oxidative machinery, see Regoli and Giuliani (2014) for a full review on these aspects.
Persistent organic pollutants (POPs) are a large variety of compounds, including polychlorinated biphenyls (PCBs), organochlorine pesticides, or dioxins. Although industrial production of some POPs is now banned in most countries, they still represent a major health hazard due to their high persistence in the environment and their lipophilic properties that facilitate bio-accumulation and bio-magnification via the food chain (Beyer and Biziuk, 2009). Exposure to PCBs has been associated with dramatic adverse effects in many species (e.g., eggshell thinning in birds of prey; Wiemeyer and Porter, 1970; Ross, 2004; Frye et al., 2011). Several studies have shown that increased OS may be an additional mechanism underlying the negative effects of PCBs on the organism (e.g., Oakley et al., 1996; Zhu et al., 2009).
One important mechanism of action of PCBs is the binding capacity of some “dioxin-like” congers, such as PCBs 77 and 126, with the aryl hydrocarbon receptor (AhR), activating the enzyme cytochrome P450 1A subfamily (Hennig et al., 1999, 2002; Figures 2C,D). These interactions can uncouple the catalytic cycle of the enzyme CYP1A, allowing the heme iron within the active site of this enzyme complex to undergo cycles of oxidation and reduction, and act as a Fenton catalyst, generating free radicals (Schlezinger et al., 1999). Therefore exposure to PCBs might increase production of pro-oxidants that, if not counteracted by antioxidants, may cause OS. PCBs might also cause OS through the de-regulation of specific antioxidants. Administration of PCB 77 to rats suppressed both transcription and enzymatic activity of selenium-dependent glutathione peroxidases and total selenium in liver (Twaroski et al., 2001), which are important components of the antioxidant machinery. Although this review specifically focuses on OS, we note that several PCBs are also known to influence hormonal systems that regulate signaling (Ottinger et al., 2002).
Exposure to PCBs can also disrupt body coloration in birds, and, therefore, potentially influence sexual communication (Figure 1B). Pioneering work by Bortolotti et al. (2003) demonstrated that oral exposure to environmentally relevant dose of a PCB mixture (i.e., 1:1:1 Aroclor 1254, 1248, and 1260) in American kestrels (Falco sparverius) affected both plasma carotenoids and carotenoid-dependent coloration in a complex sex- and season-dependent manner. Differences in coloration were also found in the juveniles produced by the PCB-exposed parents, a presumed long-term effect of exposure to PCBs in ovo (Bortolotti et al., 2003). The effects of PCBs on the adult colorations might have been the consequence of changes in the oxidative balance. PCB-exposed individuals may have diverted carotenoids from colorations to protection against PCB-induced OS (Bortolotti et al., 2003); this would imply a trade-off for allocation of carotenoids between sexual signaling and self-maintenance (Pérez-Rodriguez et al., 2010). Indeed, during the courtship period, PCB-exposed males were duller than control males, while PCB-exposed females maintained their plumage coloration conversely to the expected loss in color observed in control females when carotenoids are diverted towards the developing ovaries (Surai, 2002). Moreover, the same PCB-treated females as in Bortolotti et al. (2003) delayed egg laying (Fernie et al., 2001). Although the antioxidant role of carotenoids appears to be minor in birds (Costantini and Møller, 2008), carotenoids might be more important when individuals are exposed to environmental (and oxidative) challenges. It might also be that depletion of other antioxidants would cause increased passive oxidation of carotenoids (Hartley and Kennedy, 2004; “protection hypothesis” in Pérez et al., 2008). Therefore, depletion of carotenoids might still confer honesty to the signal as long as it conveys other individual quality- and fitness-dependent functions.
Changes in coloration in response to elevated PCBs have also been found in ornaments independent from carotenoids and other pigments. McCarty and Secord (2000) examined plumage color in sub-adult female tree swallows (Tachycineta bicolor) breeding in an area highly contaminated by PCBs in the Hudson River. Females from contaminated areas had significantly more adult-like structurally colored blue-green feathers than females from control areas. This result was interpreted as an effect of PCBs on plumage through endocrine disruption, however, mechanisms were not directly investigated.
Emerging POPs can be broadly defined as contaminants that are suspect of negative effects for the environment and wildlife, either not yet or recently regulated. These can include compounds that have been recently discovered (“true” or “new” emerging contaminants), or contaminants that have been in the environment since decades but for which concerns have been raised more recently (Sauvé and Desrosiers, 2014).
Brominated flame-retardants (BFRs) include various compounds, such as emerging BFRs [e.g., 2,3-dibromopropyl phosphate (TDBPP)], polybrominated diphenyl ethers (PBDEs), tetrabromibishenol-A (TBBPA), hexabromocyclodecane (HBCD), and polybrominated biphenyls (PBBs), present in plastics, textiles, and commonly used in electronic equipment from catching fire. Several BFRs have raised environmental health concerns since they are highly persistent in the environment and can bio-accumulate in the food chains (de Wit, 2002). Most work has mainly focused on PBDEs, which are structurally similar to PCBs, and are now recognized as global pollutants (de Wit, 2002). The adverse effects of PBDEs may involve neurotoxic action during brain development or disruption of the thyroid endocrine system (Dingemans et al., 2011). Both these two mechanisms are likely to be associated with perturbation of homeostasis and generation of OS. In mammals, commercially available mixtures of PBDEs can enhance production of free radicals among different tissues, including the brain (e.g., Cheng et al., 2009; Bellés et al., 2010), liver (Fernie et al., 2005), and kidney (Albina et al., 2010).
Although free-living organisms are exposed to PBDEs since the start of life (Sellström et al., 1992), only a handful of studies have examined the consequences of PBDEs exposure for the organism. In captive nestling American kestrels (F. sparverius), exposure to environmentally relevant doses of a commercial mixture of PBDEs led to alterations in the thyroid system as well as hepatic OS, with marginal increases in oxidative damage (lipid peroxidation), and increased oxidized glutathione and retinol (Fernie et al., 2005). Exposure to environmentally relevant doses of DE-71 (a commercially available PBDE congener) caused changes in reproductive performance during the pre-nesting period, reducing copulatory activities and altering frequency and timing of pair-bonding behaviors (Fernie et al., 2008). Given that PBDEs are structurally similar to PCBs and appear to induce similar effects on the individuals' oxidative balance, and given that they can alter social and sexual signaling, it is likely that PBDEs might affect signaling through OS. We need experimental work that aims to elucidating such potential functional links among PBDEs, OS, and honest signals.
Perfluoroalkylated substances (PFAs) have been widely used since 1950s for industrial applications, such as impregnating agents for carpets and textiles, fire-fighting foam (Jensen and Leffers, 2008). Perfluorooctane sulfonic acid (PFOS) and perfluorooctanoic acid (PFOA) have been the most widely used PFAs. Both PFOS and PFOA are highly persistent in the environment and have been detected globally in wildlife and humans (Lau et al., 2007). Vertebrates do not seem to be able to metabolize and excrete PFAs (Muir and de Wit, 2010). Thus, PFAs bio-accumulate via the food chain (Tittlemier et al., 2007) and high levels of PFOS have been recorded in Arctic top predators, such as polar bears (Bossi et al., 2005).
A suspected mechanism involved in the toxic effects of PFAs is the peroxisome proliferator-activated receptor-alpha (PPAR-alpha; Figure 2C). Both PFOS and PFOA are weak activators of this receptor (Abbott, 2009). The PPAR is considered an important POP-related pathway involved in cell differentiation and hormone homeostasis (Kennedy et al., 2004). In rodents, activation of the PPAR-α increases production of hydrogen peroxide, which may lead to generation of hydroxyl radicals, which can boost OS (Klaunig et al., 2003). Experimental evidence shows that PFAs may trigger OS through the stimulation of ROS production (Eriksen et al., 2010), increased oxidative damage to DNA (Yao and Zhong, 2005), and cell apoptosis (Panaretakis et al., 2001).
In agreement with mammalian studies (e.g., Lau et al., 2006), Yanai et al. (2008) reported that exposure to PFOA in ovo in chicken reduced hatching success. Intriguingly, the latter study also showed that the surviving individuals exposed to the higher doses of PFOA were partially or completely missing their typical yellow pigmentation post-hatching (Yanai et al., 2008). Although neither antioxidant nor oxidative damage markers were measured, the results provided by Yanai et al. (2008) suggest that pre-natal exposure to PFOA might have interfered with the metabolism of carotenoids (Surai, 1998). The latter work offers preliminary evidence that PFAs can alter carotenoid-dependent colorations, similarly as the exposure to PCBs.
An important point that is often forgotten in research on the impacts of contaminants on wildlife is that low dose exposure may stimulate organism's defense mechanisms. This phenomenon, called “hormesis,” is increasingly recognized to be strongly associated with exposure to contaminants (Calabrese and Blain, 2005) and OS (Costantini, 2014). For example, sublethal lead exposure may increase male coloration (Vallverdú-Coll et al., 2015). Male starlings exposed to environmentally relevant levels of synthetic and natural estrogen mimics developed longer and more complex songs, and had larger brain areas controlling song complexity than controls (Markman et al., 2008). Moreover, females preferred the song of males which had higher pollutant exposure, despite experimentally dosed males showed reduced immune function (Markman et al., 2008). The low dose stimulation of traits involved in signaling may therefore have serious implications at the population level whether contaminants paradoxically enhance a signal of male quality biasing female choice towards contaminated males.
Many contaminants can affect either oxidative balance or expression of signals. We now need experimental work that seeks to assess the extent to which contamination-induced OS impacts on sexual signaling. We also need more studies to fully understand whether sexual signals could reliably be applied to monitor contaminant exposure in natural animal populations (Blévin et al., 2014; Giraudeau et al., 2015). Although in our mini-review we have not included literature on the impact of pesticides on OS and signaling, we highlight that our argumentations also apply to this important class of contaminants. Future work should pay special attention to the many factors that likely influence the impact of contaminant-induced OS on signaling. For instance, we might expect differences between males and females in those species where their investment into reproduction differs largely. We would also expect differences among age classes because both reproductive investment and resistance to OS vary with age (Costantini et al., 2014).
Most of the work about either OS or honest signals to date has focused on melanin and carotenoid-based colorations. Contaminants can also influence other components of signaling, such as vocalizations (DeLeon et al., 2013), or olfactory detection (Olsén, 2011). More research on these sexual communication components would be useful to deeper our understanding of the links among contaminants, OS, and individual's quality. We also stress that very little is known on the effects of emerging POPs such as BFRs and PFAs on honest signaling and OS in wild populations. Experimental work on these emerging POPs is a fruitful area for future research. We finally highlight that acknowledging the significance of hormesis will be fundamental for the interpretation of results.
Design of review protocol: DC and VM; wrote the manuscript: DC and VM.
Preparation of this article was supported by a postdoctoral fellowship from FWO-Flanders (DC) and by the College of Medical, Veterinary and Life Sciences of the University of Glasgow (VM).
The authors declare that the research was conducted in the absence of any commercial or financial relationships that could be construed as a potential conflict of interest.
We thank the reviewers for the constructive and useful comments on previous drafts of the manuscript. DC thanks very much Jordi Figuerola for inviting him to organize the research topic “Oxidative stress and signal honesty.”
Abbott, B. D. (2009). Review of the expression of peroxisome proliferator-activated receptors alpha (PPARα), beta (PPARβ) and gamma (PPARγ) in rodent and human development. Reprod. Toxicol. 27, 246–257. doi: 10.1016/j.reprotox.2008.10.001
Albina, M. L., Alonso, V., Linares, V., Bellés, M., Sirvent, J. J., Domingo, J. L., et al. (2010). Effects of exposure to BDE-99 on oxidative status of liver and kidney in adult rats. Toxicology 271, 51–56. doi: 10.1016/j.tox.2010.03.006
Ballatori, N., and Clarkson, T. W. (1985). Biliary secretion of glutathione and glutathione-metal complexes. Fundam. Appl. Toxicol. 5, 816–831. doi: 10.1016/0272-0590(85)90165-4
Bellés, M., Alonso, V., Linares, V., Albina, M. L., Sirvent, J. J., Domingo, J. L., et al. (2010). Behavioral effects and oxidative status in brain regions of adult rats exposed to BDE-99. Toxicol. Lett. 194, 1–7. doi: 10.1016/j.toxlet.2010.01.010
Benathan, M., Virador, V., Furumura, M., Kobayashi, N., Panizzon, R. G., and Hearing, V. J. (1999). Co-regulation of melanin precursors and tyrosinase in human pigment cells: roles of cysteine and glutathione. Cell. Mol. Biol. 45, 981–990.
Beyer, A., and Biziuk, M. (2009). “Environmental fate and global distribution of polychlorinated biphenyls,” in Reviews of Environmental Contamination and Toxicology, Vol. 201, ed D. M. Whitacre (New York, NY: Springer US), 137–158.
Blévin, P., Tartu, S., Angelier, F., Leclaire, S., Bustnes, J. O., Moe, B., et al. (2014). Integument colouration in relation to persistent organic pollutants and body condition in arctic breeding black-legged kittiwakes (Rissa tridactyla). Sci. Total Environ. 470–471, 248–254. doi: 10.1016/j.scitotenv.2013.09.049
Bortolotti, G. R., Fernie, K. Y., and Smiths, J. E. (2003). Carotenoid concentration and coloration of American Kestrels (Falco sparverius) disrupted by experimental exposure to PCBs. Funct. Ecol. 17, 651–657. doi: 10.1046/j.1365-2435.2003.00778.x
Bossi, R., Riget, F. F., Dietz, R., Sonne, C., Fauser, P., Dam, M., et al. (2005). Preliminary screening of perfluoroctane sulfonate (PFOS) and other fluorochemicals in fish, birds and marine mammals from Greenland and the Faroe Islands. Environ. Pollut. 136, 323–329. doi: 10.1016/j.envpol.2004.12.020
Bridges, C. C., and Zalups, R. K. (2006). Molecular mimicry as a mechanism for the uptake of cysteine S-conjugates of methylmercury and inorganic mercury. Chem. Res. Toxicol. 19, 1117–1118. doi: 10.1021/tx060158i
Calabrese, E. J., and Blain, R. (2005). The occurrence of hormetic dose responses in the toxicological literature, the hormesis database: an overview. Toxicol. Appl. Pharmacol. 202, 289–301. doi: 10.1016/j.taap.2004.06.023
Carere, C., Costantini, D., Fusani, L., Alleva, E., and Cardinale, M. (2010). Hypothermic abilities of migratory songbirds at a stopover site. Rendiconti Lincei 21, 323–334. doi: 10.1007/s12210-010-0094-0
Chan, H. M., Satoh, M., Zalups, R. K., and Cherian, M. G. (1992). Exogenous metallothionein and renal toxicity of cadmium and mercury in rats. Toxicology 76, 15–26. doi: 10.1016/0300-483X(92)90014-6
Cheng, J., Gu, J., Ma, J., Chen, X., Zhang, M., and Wang, W. (2009). Neurobehavioural effects, redox responses and tissue distribution in rat offspring developmental exposure to BDE-99. Chemosphere 75, 963–968. doi: 10.1016/j.chemosphere.2009.01.004
Costantini, D. (2014). Oxidative Stress and Hormesis in Evolutionary Ecology and Physiology: a Marriage between Mechanistic and Evolutionary Approaches. Berlin; Heidelberg: Springer-Verlag.
Costantini, D., Meillere, A., Carravieri, A., Lecomte, V., Sorci, G., Faivre, B., et al. (2014). Oxidative stress in relation to reproduction, contaminants, gender and age in a long-lived seabird. Oecologia 175, 1107–1116. doi: 10.1007/s00442-014-2975-x
Costantini, D., and Møller, A. P. (2008). Carotenoids are minor antioxidants for birds. Funct. Ecol. 22, 367–370. doi: 10.1111/j.1365-2435.2007.01366.x
DeLeon, S., Halitschke, R., Hames, R. S., Kessler, A., DeVoogd, T. J., and Dhondt, A. A. (2013). The effect of polychlorinated biphenyls on the song of two passerine species. PLoS ONE 8:e73471. doi: 10.1371/journal.pone.0073471
de Wit, C. A. (2002). An overview of brominated flame retardants in the environment. Chemosphere 46, 583–624. doi: 10.1016/S0045-6535(01)00225-9
Dingemans, M. M., van den Berg, M., and Westerink, R. H. (2011). Neurotoxicity of brominated flame retardants: (in)direct effects of parent and hydroxylated polybrominated diphenyl ethers on the (developing) nervous system. Environ. Health Perspect. 119, 900–907. doi: 10.1289/ehp.1003035
Eriksen, K. T., Raaschou-Nielsen, O., Sorensen, M., Roursgaard, M., Loft, S., and Moller, P. (2010). Genotoxic potential of the perfluorinated chemicals PFOA, PFOS, PFBS, PFNA and PFHxA in human HepG2 cells. Mutat. Res.Genet. Toxicol. Environ. Mutagen. 700, 39–43. doi: 10.1016/j.mrgentox.2010.04.024
Fernie, K. J., Shutt, J. L., Letcher, R. J., Ritchie, J. I., Sullivan, K., and Bird, D. M. (2008). Changes in reproductive courtship behaviors of adult american kestrels (Falco sparverius) exposed to environmentally relevant levels of the polybrominated diphenyl ether mixture, DE-71. Toxicol. Sci. 102, 171–178. doi: 10.1093/toxsci/kfm295
Fernie, K. J., Shutt, L., Mayne, G., Hoffman, D., Letcher, R. J., Drouillard, K. G., et al. (2005). Exposure to polybrominated diphenyl ethers (PBDEs): changes in thyroid, vitamin A, glutathione homeostasis, and oxidative stress in American kestrels (Falco sparverius). Toxicol. Sci. 88, 375–383. doi: 10.1093/toxsci/kfi295
Fernie, K. J., Smits, J. E., Bortolotti, G. R., and Bird, D. M. (2001). In ovo exposure to polychlorinated biphenyls: reproductive effects on second generation American kestrels. Arch. Environ. Contam. Toxicol. 40, 544–550. doi: 10.1007/s002440010208
Frye, C., Bo, E., Calamandrei, G., Calza, L., Dessì-Fulgheri, F., Fernandez, M., et al. (2011). Endocrine disrupters: a review of some sources, effects, and mechanisms of actions on behaviour and neuroendocrine systems. J. Neuroendocrinol. 24, 144–159. doi: 10.1111/j.1365-2826.2011.02229.x
Galván, I., and Alonso-Alvarez, C. (2008). An intracellular antioxidant determines the expression of a melanin-based signal in a bird. PLoS ONE 3:e3335. doi: 10.1371/journal.pone.0003335
Galván, I., and Solano, F. (2009). The evolution of eu- and pheomelanic traits may respond to an economy of pigments related to environmental oxidative stress. Pigment Cell. Melan. Res. 22, 339–342. doi: 10.1111/j.1755-148X.2009.00559.x
García-Borrón, J. C., and Olivares Sαnchez, M. C. (2011). “Biosynthesis of melanins,” in Melanins and Melanosomes: Biosynthesis, Biogenesis, Physiological, and Pathological Functions, eds J. Borovanský and P. A. Riley (Weinheim: Wiley-Black-well), 87–116.
Giraudeau, M., Mateos-Gonzalez, F., Cotín, J., Pagani-Nuñez, E., Torné-Noguera, A., and Senar, J. C. (2015). Metal exposure influences the melanin and carotenoid-based colorations in great tits. Sci. Total Environ. 532, 512–516.
Grunst, A. S., Salgado-Ortiz, J., Rotenberry, J. T., and Grunst, M. L. (2014). Phaeomelanin- and carotenoid-based pigmentation reflect oxidative status in two populations of the yellow warbler (Setophaga petechia). Behav. Ecol. Sociobiol. 68, 669–680. doi: 10.1007/s00265-013-1681-8
Hartley, R. C., and Kennedy, M. W. (2004). Are carotenoids a red herring in sexual display? Trends Ecol. Evol. 19, 353–354. doi: 10.1016/j.tree.2004.04.002
Hennig, B., Meerarani, P., Slim, R., Toborek, M., Daugherty, A., Silverstone, A. E., et al. (2002). Proinflammatory properties of coplanar PCBs: in vitro and in vivo evidence. Toxicol. Appl. Pharmacol. 181, 174–183. doi: 10.1006/taap.2002.9408
Hennig, B., Slim, R., Toborek, M., and Robertson, L. W. (1999). Linoleic acid amplifies polychlorinated biphenyl-mediated dysfunction of endothelial cells. J. Biochem. Mol. Toxicol. 13, 83–91.
Hill, G. E. (1995). Ornamental traits as indicators of environmental health. BioScience 45, 25–31. doi: 10.2307/1312532
Hill, G. E. (2011). Condition-dependent traits as signals of the functionality of vital cellular processes. Ecol. Lett. 14, 625–634. doi: 10.1111/j.1461-0248.2011.01622.x
Hoffman, D. J., and Heinz, G. H. (1998). Effects of mercury and selenium on glutathione metabolism and oxidative stress in mallard ducks. Environ. Toxicol. Chem. 17, 161–166. doi: 10.1002/etc.5620170204
Isaksson, C. (2010). Pollution and its impact on wild animals: a meta-analysis on oxidative stress. Ecohealth 7, 342–350. doi: 10.1007/s10393-010-0345-7
Jensen, A. A., and Leffers, H. (2008). Emerging endocrine disrupters: perfluoroalkylated substances. Int. J. Androl. 31, 161–169. doi: 10.1111/j.1365-2605.2008.00870.x
Jones, D. P. (2006). Redefining oxidative stress. Antioxid. Redox Signal. 8, 1865–1879. doi: 10.1089/ars.2006.8.1865
Kennedy, G. L., Butenhoff, J. L., Olsen, G. W., O'Connor, J. C., Seacat, A. M., Perkins, R. G., et al. (2004). The toxicology of perfluorooctanoate. Crit. Rev. Toxicol. 34, 351–384. doi: 10.1080/10408440490464705
Klaunig, J. E., Babich, M. A., Baetcke, K. P., Cook, J. C., Corton, J. C., David, R. M., et al. (2003). PPARα agonist-induced rodent tumors: modes of action and human relevance. Crit. Rev. Toxicol. 33, 655–780. doi: 10.1080/713608372
Lau, C., Anitole, K., Hodes, C., Lai, D., Pfahles-Hutchens, A., and Seed, J. (2007). Perfluoroalkyl acids: a review of monitoring and toxicological findings. Toxicol. Sci. 99, 366–394. doi: 10.1093/toxsci/kfm128
Lau, C., Thibodeaux, J. R., Hanson, R. G., Narotsky, M. G., Rogers, J. M., Lindstrom, A. B., et al. (2006). Effects of perfluorooctanoic acid exposure during pregnancy in the mouse. Toxicol. Sci. 90, 510–518. doi: 10.1093/toxsci/kfj105
Mah, V., and Jalilehvand, F. (2010). Glutathione complex formation with mercury(II) in aquaeous solution at physiological pH. Chem. Res. Toxicol. 23, 1815–1823. doi: 10.1021/tx100260e
Manning, J. T., and Chamberlain, A. T. (1994). Fluctuating asymmetry in gorilla canines: a sensitive indicator of environmental stress. Proc. Biol. Sci. 255, 189–193. doi: 10.1098/rspb.1994.0027
Markman, S., Leitner, S., Catchpole, C., Barnsley, S., Müller, C. T., Pascoe, D., et al. (2008). Pollutants increase song complexity and the volume of the brain area HVC in a songbird. PLoS ONE 3:e1674. doi: 10.1371/journal.pone.0001674
McCarty, J. P., and Secord, A. L. (2000). Possible effects of PCB contamination on female plumage color and reproductive success in Hudson River tree swallows. Auk 117, 987–995. doi: 10.1642/0004-8038(2000)117[0987:PEOPCO]2.0.CO;2
Metodiewa, D., and Dunford, H. B. (1990). The role of myeloperoxidase in the oxidation of biologically active polyhydroxyphenols substituted catechols. Eur. J. Biochem. 193, 445–448. doi: 10.1111/j.1432-1033.1990.tb19358.x
Møller, A. P. (1993). Morphology and sexual selection in the barn swallow Hirundo rustica in Chernobyl, Ukraine. Proc. Biol. Sci. 252, 51–57. doi: 10.1098/rspb.1993.0045
Monteiro, L. R., and Furness, R. W. (1995). Seabirds as monitors of mercury in the marine environment. Water Air Soil Pollut. 80, 851–870. doi: 10.1007/BF01189736
Muir, D. C. G., and de Wit, C. A. (2010). Trends of legacy and new persistent organic pollutants in the circumpolar arctic: overview, conclusions, and recommendations. Sci. Total Environ. 408, 3044–3051. doi: 10.1016/j.scitotenv.2009.11.032
Nigro, M., and Leonzio, C. (1996). Intracellular storage of mercury and selenium in different marine vertebrates. Mar. Ecol. Progr. Ser. 135, 137–143. doi: 10.3354/meps135137
Oakley, G. G., Devanaboyina, U., Robertson, L. W., and Gupta, R. C. (1996). Oxidative DNA damage induced by activation of polychlorinated biphenyls (PCBs): implications for PCB-induced oxidative stress in breast cancer. Chem. Res. Toxicol. 9, 1285–1292. doi: 10.1021/tx960103o
Olsén, K. H. (2011). “Effects of pollutants on olfactory mediated behaviours in fish and crustaceans,” in Chemical Communication in Crustaceans, eds T. Breithaupt and M. Thiel (New York, NY: Springer), 507–529.
Ottinger, M. A., Abdelnabi, M., Quinn, M., Golden, N., Wu, J., and Thompson, N. (2002). Reproductive consequences of EDCs in birds What do aboratory effects mean in field species? Neurotoxicol. Teratol. 24, 17–28. doi: 10.1016/S0892-0362(01)00195-7
Ozeki, H., Ito, S., Wakamatsu, K., and Ishiguro, I. (1997). Chemical characterization of pheomelanogenesis starting from dihydroxyphenylalanine or tyrosine and cysteine. Effects of tyrosinase and cysteine concentrations and reaction time. Biochim. Biophys. Acta 1336, 539–548. doi: 10.1016/S0304-4165(97)00068-8
Panaretakis, T., Shabalina, I. G., Grandér, D., Shoshan, M. C., and DePierre, J. W. (2001). Reactive oxygen species and mitochondria mediate the induction of apoptosis in human hepatoma HepG2 cells by the rodent peroxisome proliferator and hepatocarcinogen, perfluorooctanoic acid. Toxicol. Appl. Pharmacol. 173, 56–64. doi: 10.1006/taap.2001.9159
Pérez, C., Lores, M., and Velando, A. (2008). Availability of nonpigmentary antioxidant affects red coloration in gulls. Behav. Ecol. 19, 967–973. doi: 10.1093/beheco/arn053
Pérez-Rodriguez, L., Mougeot, F., and Alonso-Alvarez, C. (2010). Carotenoid-based coloration predicts resistance to oxidative damage during immune challenge. J. Exp. Biol. 213, 1685–1690. doi: 10.1242/jeb.039982
Regoli, F., and Giuliani, M. E. (2014). Oxidative pathways of chemical toxicity and oxidative stress biomarkers in marine organisms. Mar. Environ. Res. 93, 106–117. doi: 10.1016/j.marenvres.2013.07.006
Ross, G. (2004). The public health implications of polychlorinated biphenyls (PCBs) in the environment. Ecotoxicol. Environ. Saf. 59, 275–291. doi: 10.1016/j.ecoenv.2004.06.003
Rubino, F. M., Pitton, M., Brambilla, G., and Colombi, A. (2006). A study of the glutathione metaboloma peptides by energy-resolved mass spectrometry as a tool to investigate into the interference of toxic heavy metals with their metabolic processes. J. Mass Spectrom. 41, 1578–1593. doi: 10.1002/jms.1143
Sauvé, S., and Desrosiers, M. (2014). A review of what is an emerging contaminant. Chem. Cent. J. 8:15. doi: 10.1186/1752-153X-8-15
Schlezinger, J. J., White, R. D., and Stegeman, J. J. (1999). Oxidative inactivation of cytochrome P-450 1A (CYP1A) stimulated by 3,3′, 4,4′-tetrachlorobiphenyl: production of reactive oxygen by vertebrate CYP1As. Mol. Pharmacol. 56, 588–597.
Sellström, U., Jansson, B., Kierkegaard, A., and de Wit, D. (1992). Polybrominated diphenyl ethers (PBDE) in biological samples from the Swedish environment. Chemosphere 26, 1703–1718. doi: 10.1016/0045-6535(93)90114-K
Stricks, W., and Kolthoff, I. M. (1953). Reactions between mercuric mercury and cysteine and glutathione. Apparent dissociation constants, heats and entropies of formation of various forms of mercuric mercapto-cysteine and -glutathione. J. Am. Chem. Soc. 75, 5673–5681. doi: 10.1021/ja01118a060
Surai, P. F. (1998). Distribution of carotenoids from the yolk to the tissues of the chick embryo. J. Nutr. Biochem. 9, 645–651. doi: 10.1016/S0955-2863(98)00068-0
Surai, P. F. (2002). Natural Antioxidants in Avian Nutrition and Reproduction. Nottingham: Nottingham University Press.
Thompson, D. R., and Furness, R. W. (1989). The chemical form of mercury stored in south Atlantic seabirds. Environ. Pollut. 60, 305–317. doi: 10.1016/0269-7491(89)90111-5
Tittlemier, S. A., Pepper, K., Seymour, C., Moisey, J., Bronson, R., Cao, X. L., et al. (2007). Dietary exposure of Canadians to perfluorinated carboxylates and perfluorooctane sulfonate via consumption of meat, fish, fast foods, and food items prepared in their packaging. J. Agric. Food Chem. 55, 3203–3210. doi: 10.1021/jf0634045
Twaroski, T. P., O'Brien, M. L., and Robertson, L. W. (2001). Effects of selected polychlorinated biphenyl (PCB) congeners on hepatic glutathione, glutathione-related enzymes, and selenium status: implications for oxidative stress. Biochem. Pharmacol. 62, 273–281. doi: 10.1016/S0006-2952(01)00668-2
Vallverdú-Coll, N., Mougeot, F., Ortiz-Santaliestra, M. E., Rodriguez-Estival, J., López-Antia, A., and Mateo, R. (2016). Lead exposure reduces carotenoid-based coloration and constitutive immunity in wild mallards. Environ. Toxicol. Chem. 35, 1516–1525. doi: 10.1002/etc.3301.
Vallverdú-Coll, N., Ortiz-Santaliestra, M. E., Mougeot, F., Vidal, D., and Mateo, R. (2015). Sublethal Pb exposure produces season-dependent effects on immune response, oxidative balance and investment in carotenoid-based coloration in red-legged partridges. Environ. Sci. Technol. 49, 3839–3850. doi: 10.1021/es505148d
von Schantz, T., Bensch, S., Grahn, M., Hasselquist, D., and Wittzell, H. (1999). Good genes, oxidative stress and condition-dependent sexual signals. Proc. Biol. Sci. 266, 1–12. doi: 10.1098/rspb.1999.0597
Whysner, J., and Wang, C. X. (2001). Hepatocellular iron accumulation and increased cell proliferation in polychlorinated biphenyl-exposed Sprague-Dawley rats and the development of hepatocarcinogenesis. Toxicol. Sci. 62, 36–45. doi: 10.1093/toxsci/62.1.36
Wiemeyer, S. N., and Porter, R. D. (1970). DDE thins eggshells of captive American Kestrels. Nature 227, 737–738. doi: 10.1038/227737a0
Yanai, J., Dotan, S., Goz, R., Pinkas, A., Seidler, F. J., Slotkin, T. A., et al. (2008). Exposure of developing chicks to perfluorooctanoic acid induces defects in prehatch and early posthatch development. J. Toxicol. Environ. Health Part A 71, 131–133. doi: 10.1080/15287390701613280
Yao, X., and Zhong, L. (2005). Genotoxic risk and oxidative DNA damage in HepG2 cells exposed to perfluorooctanoic acid. Mutat. Res. Genet. Toxicol. Environ. Mutagen. 587, 38–44. doi: 10.1016/j.mrgentox.2005.07.010
Keywords: contaminants, POPs, oxidative stress, honest signals, heavy metals, hormesis
Citation: Marasco V and Costantini D (2016) Signaling in a Polluted World: Oxidative Stress as an Overlooked Mechanism Linking Contaminants to Animal Communication. Front. Ecol. Evol. 4:95. doi: 10.3389/fevo.2016.00095
Received: 21 January 2016; Accepted: 21 July 2016;
Published: 04 August 2016.
Edited by:
Carlos Alonso Alvarez, Spanish National Research Council, SpainReviewed by:
Rafael Mateo, Instituto de Investigación en Recursos Cinegéticos, Consejo Superior de Investigaciones Científicas (CSIC), la Universidad de Castilla–La Mancha (UCLM), SpainCopyright © 2016 Marasco and Costantini. This is an open-access article distributed under the terms of the Creative Commons Attribution License (CC BY). The use, distribution or reproduction in other forums is permitted, provided the original author(s) or licensor are credited and that the original publication in this journal is cited, in accordance with accepted academic practice. No use, distribution or reproduction is permitted which does not comply with these terms.
*Correspondence: Valeria Marasco, dmFsZXJpYS5tYXJhc2NvQGdsYXNnb3cuYWMudWs=
David Costantini, ZGF2aWQuY29zdGFudGluaUB1YW50d2VycGVuLmJl
Disclaimer: All claims expressed in this article are solely those of the authors and do not necessarily represent those of their affiliated organizations, or those of the publisher, the editors and the reviewers. Any product that may be evaluated in this article or claim that may be made by its manufacturer is not guaranteed or endorsed by the publisher.
Research integrity at Frontiers
Learn more about the work of our research integrity team to safeguard the quality of each article we publish.