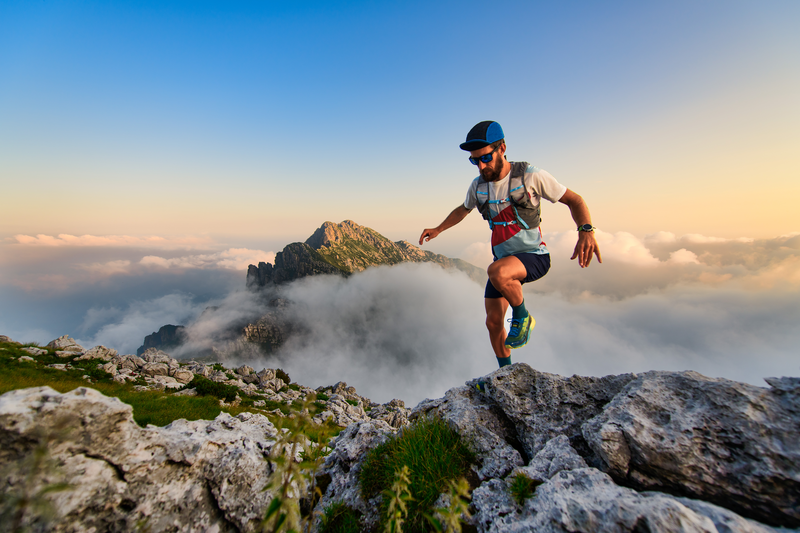
94% of researchers rate our articles as excellent or good
Learn more about the work of our research integrity team to safeguard the quality of each article we publish.
Find out more
ORIGINAL RESEARCH article
Front. Ecol. Evol. , 21 July 2016
Sec. Agroecology
Volume 4 - 2016 | https://doi.org/10.3389/fevo.2016.00085
This article is part of the Research Topic Compositional data analysis in ecology and agronomy: Looking at an unbiased future View all 3 articles
Sugar maple (Acer saccharum Marshall, SM) is believed to be more sensitive to acidic and nutrient-poor soils associated with conifer-dominated stands than red maple (Acer rubrum L., RM). Greater foliar nutrient use efficiency (FNUE) of RM is likely the cause for this difference. In the context of climate change, this greater FNUE could be key in favoring northward migration of RM over SM. We used the concept of foliar nutrient balances to study the nutrition of SM and RM seedlings along an increasing gradient in forest floor acidity conditioned by increasing proportions of conifers (pH values ranging from 4.39 under hardwoods, to 4.29 under mixed hardwood-conifer stands and 4.05 under conifer-dominated stands). Nutrients were subjected to isometric log-ratio (ilr) transformation, which views the leaf as one closed system and considers interactions between nutrients. The ilr method eliminates numerical biases and weak statistical inferences based on raw or “operationally” log-transformed data. We analyzed foliar nutrients of SM and RM seedlings and found that the [Ca,Mg,K| P,N] and [Ca,Mg| K] balances of SM seedlings were significantly different among soil acidity levels, whereas they did not vary for RM seedlings. For SM seedlings, these differences among soil acidity levels were due to a significant decrease in foliar Ca and Mg concentrations with increasing forest floor acidity. Similar differences in foliar balances were also found between healthy and declining SM stands estimated from literature values. Conversely, foliar balances of RM seedlings did not differ among soil acidity levels, even though untransformed foliar nutrient concentrations were significantly different. This result highlights the importance of using ilr transformation, since it provides more sensitive results than standard testing of untransformed nutrient concentrations. The lower nutrient requirements of RM and its greater capacity to maintain nutrient equilibrium are factors that could explain its competitive success and recent northward expansion. This study underscores the importance of using nutrient balances to study the redistribution of plant species in natural ecosystems under climate change.
Climate, soil properties and biotic interactions are key factors controlling the geographical distribution of plant species (Whittaker, 1970). However, rapid ongoing climatic changes are creating new physiological constraints that force plant species to find new ecological optima by shifting their ranges to higher elevations and latitudes (Parmesan and Yohe, 2003; Rosenzweig et al., 2008; Chen et al., 2011). Scientists have paid greater attention to climate, as it is presumed to be the leading factor that results in changing plant distributions, but observed shifts of plant species have occurred at slower rates than that of climate change itself (Parmesan and Yohe, 2003; Loarie et al., 2009; Chen et al., 2011; Zhu et al., 2012). Recent evidence suggests that non-climatic factors such as soil properties and biotic interactions are slowing the migration of plant species (McMahon et al., 2011; Brown and Vellend, 2014; Graignic et al., 2014). Therefore, more research is needed regarding the role of non-climatic factors, such as soil properties, in affecting the northward migration of trees in the early stages of establishment (i.e., tree recruitment, including seed germination and seedling survival and growth) (Cleavitt et al., 2014).
Recruitment of sugar maple (Acer saccharum Marshall, SM) is of particular interest in eastern North America, given that this tree species is dominant over a large geographical area. Sugar maple is sensitive to acidic and nutrient-poor soils (St. Clair et al., 2008), and this sensitivity was proposed as an explanation for its limited capacity in migrating northward into the boreal forest (Graignic et al., 2014). Acidic, Ca-poor soils that have thick litter layers, and which are characterized by low N-mineralization rates, generally support boreal forests (Binkley and Giardina, 1998). These soils may negatively affect the early stages of SM seedling establishment (Graignic et al., 2014), as has been demonstrated in various mature SM stands in eastern North America (Kobe et al., 2002; Juice et al., 2006; Long et al., 2009). The effects of soil acidity and soil base-cation depletion have resulted in foliar Ca and Mg deficiency, leading to tree decline.
Unlike SM, the range of red maple (Acer rubrum L., RM) has expanded more successfully over the last 100 years in North American forests that are supported by acidic and nutrient-poor soils (Abrams, 1998). Recently, Zhang et al. (2015) reported that RM establishment and growth were facilitated at its northern distributional limit in Quebec by increasing fire frequency. A survey of Allegheny National Forest (northwest Pennsylvania) revealed that decline symptoms and mortality rates in SM were three times greater than those of RM (McWilliams et al., 1996). These declines were linked to soil nutrient status (i.e., low Ca and Mg saturation) and severe insect defoliation events (Bailey et al., 2004). Variation in foliar nutrient use efficiency (FNUE) and tolerance to nutrient limitations were proposed as an explanation for the greater success of RM compared to SM on acidic and nutrient-poor soils (Abrams, 1998; St. Clair and Lynch, 2005b).
Relationships between soil nutrient availability, foliar nutrition and forest stand composition must be better understood to predict the recruitment and establishment of tree species that would need to migrate under climate change. Foliar nutrient status of forest tree species have been most often compared using mass nutrient content, nutrient percentages or dual ratios. However, foliar nutrients (as is the case of any other variables that are expressed in the same units, as proportions, percentages, ppm or ppb) belong to the class of compositional data, which are quantitative descriptions of the parts of a closed system, i.e., a composition or simplex, within the leaf tissue (Aitchison, 1986). Such data consist of “closed” or bounded variables, since they sum to a constant value for a given sample. Thus, the manner in which foliar nutrients are reported has some consequences for their interpretation. First, the use of ratios can lead to spurious correlations caused by scaling as it was demonstrated that if the foliar nutrients X, Y, and Z are uncorrelated, then X/Z and Y/Z will not be uncorrelated (Pearson, 1897; Chayes, 1960). Second, nutrients in the leaf are not only related physiologically through interactions (Marschner, 2011), but they are also numerically related given that all nutrients are constrained to sum to 100%; when one nutrient increases, then the others or some of the other nutrients must decrease concomitantly, and vice versa (Aitchison, 1986). Third, a D-part composition (D is the number of nutrients that have been measured) has only D-1 degrees-of-freedom because one component can be calculated as the difference between the unit and the sum of the other components (Claringbold, 1955; Schrevens and Cornell, 1993; Aitchison and Greenacre, 2002). Using raw compositional data or dual ratios to conduct linear statistical analysis therefore leads to methodological biases and analytical incoherence caused by: (1) spurious correlations; (2) neglecting to account for nutrient interactions; and (3) redundancy of information that is carried by excess degrees-of-freedom (Wilkinson et al., 2000; Bacon-Shone, 2011). To avoid bias, the isometric log-ratio (ilr) transformation technique that was developed by Egozcue et al. (2003) allows D – 1 orthogonal contrasts to be produced, which are interpreted as balances between two groups of nutrients in an Euclidean space. The ilr transformation also illustrates nutrient relationships according to partitions that decompose the composition into hierarchically arranged or nested groups of nutrients, i.e., subcompositions (Parent S. E. et al., 2012). Further, ilr is the most appropriate transformation and is more easily interpreted for plant nutrient diagnosis (Parent, 2011; Parent L. E. et al., 2012; Parent et al., 2013) compared to additive log-ratios or centered log-ratios. Our objective was to use ilr-transformed foliar nutrients to study the nutrition of SM and RM seedlings along a gradient of soil acidity conditioned by increasing proportions of conifers. We hypothesized that while nutrient imbalances in SM foliage are exacerbated due to increasing soil acidity, nutrients in RM foliage are more balanced because this species can better adapt to environments with greater nutrient limitations.
The study site is located at the Station de Biologie des Laurentides (SBL) of the University de Montréal in St. Hippolyte, Quebec (45°59′N; 74°00′O). The SBL lies within the SM-yellow birch (Betula alleghaniensis Britton) domain of the lower Laurentians, near its northern limit and at the transition with the boreal forest (Saucier et al., 2009). Sugar maple is found concurrently with RM, yellow birch, poplars (Populus spp.), balsam fir (Abies balsamea (L.) Mill.), white spruce (Picea glauca [Moench] Voss) and eastern white cedar (Thuja occidentalis L.). Due to the mosaic of tree species that formed the site, it was possible to find SM and RM seedlings growing concomitantly along an increasing gradient of soil acidity conditioned by increasing proportions of coniferous tree species.
Plots that were used for this experiment are the same as those previously studied by Collin et al. (submitted). Four plots (50 × 50 m) were delineated under each of the following forest canopies: (1) hardwood stands of SM and birch species; (2) mixed hardwood-conifer stands with SM, Betula spp. and conifers; and (3) conifer-dominated stands with SM seedlings and saplings (3 sites × 3 species composition × 4 repetitions = 36 plots). In each of these plots, SM and RM seedlings were present. Foliage from five SM and RM seedlings (between 20 and 30 cm in height) were collected within each plot for subsequent chemical analysis. Total height and diameter at the ground level were measured on all SM and RM seedlings that were sampled. We did not measure the age of SM and RM seedlings, but we assume that SM seedlings were a little bit older than RM seedlings, on average, due to their greater shade-tolerance and slower growth rate. However, nutrient concentrations in plant parts are mostly affected by their physiological age (Marschner, 2011). Therefore, comparisons of nutrient concentrations and ratios should ideally be done between plants of similar growth stages and not necessarily of similar ages. In this respect, the bias that was incurred by comparing SM and RM of similar heights and growth stages, despite possible differences in ages, is likely small.
The sandy loam soils at St. Hippolyte are Orthic Humo-Ferric Podzols (Soil Classification Working Group, 1998) developed from well-drained rocky glacial tills composed of a mixture of rocks from the small underlying anorthosite pluton (deep-seated intrusive igneous rock) of the Morin Complex (Doig, 1991) and of more felsic rocks (e.g., mangerite, syenite, charnockite) in the surroundings of the anorthosite pluton (Bélanger et al., 2012). The forest floor is a moder humus form with a thickness of 5–10 cm. Details on soil sampling, analysis and chemical properties are provided in Collin et al. (submitted). We simply confirm here that conifer-dominated stands have a significantly lower forest floor (i.e., FH horizons) pH (4.05 ± 0.07) compared to forest floors supporting mixed hardwood-conifer stands (4.29 ± 0.11) and hardwoods (4.39 ± 0.09). On the whole, nothing indicates that variations in forest floor pH are due to intrinsic site factors (e.g., parent material and topographic position, slope or aspect) other than forest type. For example, mineral soil texture is similar under each of the forest canopies and composed of about 2–3% of clay, 38–42% of silt and 55–60% of sand.
Leaf samples were oven-dried for 72 h at 65°C upon arrival in the laboratory. They were then pulverized using a ball mill prior to total C and N determination by high-temperature combustion and thermal conductivity detection (EA 1108 CHNS-O Analyzer, Thermo Fisons, Waltham, MA, USA). A 0.2 g subsample was also digested in 2 ml of 15 N HNO3 for 4 h at 100°C in glass test tubes. Base cation concentrations (Ca, Mg, and K) of the digests were determined using atomic adsorption/emission (Model AA-1475, Varian, Palo Alta, CA, USA), whereas P concentrations were determined colorimetrically using the molybdenum blue method (Technicon Autoanalyzer, Technicon Instruments Corporation, Tarrytown, NY, USA).
Computing ilr implies that the compositional data are numerically constrained to a compositional data space. Given that not all elements of the leaf are typically measured, it is therefore a necessity to first compute a filling value (Fv), which replaces the elements that were not measured. This Fv was calculated as the difference between the unit of measurement (i.e., 100%) and the sum of all nutrients that had been measured. The compositional data matrix was then constrained as described by Aitchison (1986), i.e., the closure operation computes the constant sum of components and closes it to some whole such as 100%. Once the composition data space was closed, the ilr-transformation was applied (Egozcue et al., 2003). This ilr system of balances is first defined by a nested, binary contrasts that are implemented during the sequential binary partition (SBP) steps, in which components are hierarchically arranged into functional sub-compositions (Parent S. E. et al., 2012). This hierarchical arrangement of balances needs to be constructed based on prior knowledge or hypotheses as a means to produce interpretable results (Parent S. E. et al., 2012). In this study, the SBP (Table 1) was designed based on prior knowledge of nutrient interactions in higher plants (Marschner, 2011). We first contrasted all measured nutrients (i.e., N, P, K, Ca, and Mg) with Fv, i.e., [Fv∣Elements]. We then partitioned measured nutrients to reflect the charge balance in plant cells (Marschner, 2011). Thus, N and P were contrasted with K, Ca and Mg, i.e., the two-part amalgamated ratio [Mg,Ca,K∣P,N]. Sub-compositions were further divided into an anionic Redfield [P∣N] balance (Loladze and Elser, 2011) and cationic [Mg,Ca∣K] and [Mg∣Ca] balances (Marschner, 2011).
Table 1. Sequential binary partition (SBP) of foliar nutrients of sugar and red maple seedlings based on prior knowledge of nutrient interactions in higher plants (Marschner, 2011).
The ilr balances defined during the SBP steps (Table 1) were then computed according to the equation by Egozcue and Pawlowsky-Glahn (2005):
where, in the jth row of the SBP, and are the numbers of components in the plus (+) and minus (−) groups, and g() and g() are the geometric means of components in the “+” and “−” group, respectively. Balances are generally noted [−1 group | +1 group], with denominator on the left because log ratios become more negative as values at denominator increase, hence leaning to the left as in algebra. For example, the ilr equation of the [Mg|Ca] partition is . If Ca concentration increases, then the ilr coordinate will also increase in value. In addition, an increase in Ca concentration will change the [Mg|Ca] and [Mg,Ca|K] balances, without affecting the [P|N] balance. This technique produces D−1 balances in a D-part composition, avoiding matrix singularity and redundancy. The transformation also assures that ilr balances are orthogonal to each other, thus linearly independent, making this technique totally appropriate for conducting multivariate and multiple regression analyses.
Because growth rates of maple seedlings were not measured in this study, we could not directly assess their health and vigor in relation to foliar nutrients. Therefore, we compared foliar data obtained from the literature to foliar data from SM seedlings at St. Hippolyte to assess their nutritional status. First, foliar nutrient concentrations that were measured in this study were compared to foliar nutrient concentrations from a small set of recent literature reports that included data on SM and RM seedlings that were growing on similar acidic soils (forest floor pH ranging from 3.4 to 5.5). The data were used to construct ranges of foliar N, P, K, Ca, and Mg concentrations. Second, we attempted to develop specific ranges for foliar ilr balances in healthy and declining maple seedlings from the literature. However, data on foliar nutrients for SM and RM seedlings are sparse. Many studies do not report all foliar nutrients that are required to compute ilr balances, nor do they relate foliar nutrients to seedling performance. Foliar nutrient concentrations of SM trees are more widely documented and relationships to tree fitness are often provided. A substantial SM data sets comprised of trees growing on soils (forest floors) with pH values ranging from 3.14 to 7.04 was assembled, which allowed us to construct ranges in foliar ilr balances for healthy and declining SM stands. This data set was later used as a proxy for the nutritional status of SM seedlings that were growing under three different forest types (hardwood, mixed hardwood-conifer and conifer-dominated forests) at St. Hippolyte. Conversely, foliar nutrient data for RM are sparse for both trees and seedlings and, in turn, it was not possible to compute foliar ilr balances for RM.
Data were analyzed using R software (version 3.0.0, R Core Team, 2013). The ilr-transformations were performed with the functions acomp and ilr of the compositions package (van den Boogaart et al., 2013). The function acomp is used for the closure operation, and the function ilr is used on this closed data space to perform the ilr transformation equation described above. To assess whether forest types have specific foliar nutrition signatures, linear discriminant analysis combined with a posteriori classification was performed with the function lda available in the MASS package (Venables and Ripley, 2002). Foliar ilr balances were employed as the response variables, and the multivariate homogeneity of variance-covariance matrices (permutation-based, multivariate extension of Levene's test) between species was tested using the betadisper function in the vegan package (Oksanen et al., 2013). Multivariate regressions between foliar signatures as the response variables (i.e., all balances considered) and forest floor pH as the explanatory variable were computed using data from all forest types to determine if foliar balances of SM or RM seedlings are controlled by forest species composition. Simple linear regressions between each foliar ilr balance and forest floor pH were then performed to quantify the relative contributions of each balance to the main multivariable SM and RM regressions if the latter were found significant. One-way analysis of variance and Tukey's HSD (honest significant difference) post-hoc tests were also used to separate means of forest types or species based on their respective mean foliar nutrient concentrations and balances. Testing nutrient concentrations aided our interpretation of nutrient balance results. Foliar nutrient concentrations that were used in the analysis were occasionally log-transformed to meet the assumptions of normality and homoscedasticity of the residuals.
Concentrations of foliar N, P, K, Ca, and Mg of SM and RM seedlings among the three forest types at St. Hippolyte are presented in Table 2. Sugar maple seedlings that were sampled in hardwood stands have significantly higher foliar Ca and Mg concentrations than seedlings in conifer-dominated stands. Foliar K concentrations of RM seedlings in hardwood stands are significantly higher than seedlings in conifer-dominated stands. Also, RM seedlings in mixed hardwood-conifer stands have significantly higher foliar N concentrations than seedlings in conifer-dominated stands. Finally, RM seedlings have significantly lower foliar N and higher foliar Mg concentrations than SM seedlings (Table 2).
Table 2. Mean foliar nutrient concentrations (%) of sugar maple and red maple seedlings between forest types.
Foliar nutrient concentrations in SM and RM seedlings that were measured in St. Hippolyte are generally within the range of concentrations that have been reported in the recent literature (Figure 1). Only foliar P concentrations of both SM and RM seedlings are at the lower limit of literature ranges.
Figure 1. Ranges of foliar N, P, K, Ca, and Mg concentrations in sugar maple (SM) and red maple (RM) seedlings that were measured in this study and were taken from the literature, i.e., based on St. Clair and Lynch (2005a,b), Park and Yanai (2009), Fahey and Blum (2011), and Momen et al. (2015).
Foliar ilr balances of SM and RM seedlings at St. Hippolyte were computed for each forest type (Table 3). Considering the influence of forest type on soil chemical properties (notably forest floor pH, see Collin et al., submitted), it was necessary to analyze the data using a mixed-effects model to separate the plot and forest type effects on the foliar balances of SM and RM. Analysis revealed that SM seedlings have significantly higher foliar [Mg,Ca,K|P,N], [Mg,Ca|K], and [Mg|Ca] balances, and a significantly lower foliar [Fv|Elements] balance compared to RM (Figure 2). It is important to note that these balances do not behave in the same manner as traditional ratios. A decreasing foliar ilr balance means that either the left part of the balance (the numerator of the ratio) is increasing or that the right part (the denominator of the ratio) is decreasing. Linear discriminant analysis suggests that the foliar [Mg|Ca] balance, followed by the [Mg,Ca,K|P,N] balance, can discriminate most RM seedlings from SM seedlings (Figure 3). The resulting classification matrix indicated 91.6% and 85% correct classifications of SM and RM seedlings, respectively, using foliar balances. This indicates that foliage of SM and RM seedlings possess distinct nutritional signatures.
Figure 2. Tukey tests determined differences in foliar ilr balances between sugar maple (SM) and red maple (RM) seedlings, as analyzed using a mixed model with plot and forest type as random factors. Differences are significant (P < 0.05) when they do not include zero. Differences on the left side of the origin indicate that concentrations of nutrients in the left part of the balance are higher (or concentrations in right part lower) in SM than RM seedlings, and vice-versa.
Figure 3. Results of linear discriminant function analysis (DFA) between foliar balances of sugar maple (SM) and red maple (RM) seedlings. The 1st axis explains all of the variation. Subsequent classification analysis reports that 91.6 and 85% of responses were correctly classified for SM and RM seedlings, respectively. The higher the absolute value of the coefficient, the higher its ability to discriminate between groups.
Foliar [Mg,Ca,K|P,N] and [Mg,Ca|K] balances of SM seedlings rise with increasing proportions of conifers (and thus, increasing forest floor acidity) (Figure 4). These balances are significantly higher for seedlings in conifer-dominated stands than for seedlings in hardwood stands. Conversely, the foliar [Fv|Elements] balance of seedlings in conifer-dominated stands is significantly lower than that for seedlings in hardwood stands. No difference was found among forest types in the foliar balances that were computed for RM. A high degree of variability was found for the balances of RM seedlings in hardwood stands (Table 3, Figure 4).
Figure 4. Comparison of foliar ilr balances of sugar maple (SM) and red maple (RM) seedlings among forest types (H, hardwood stands; M, mixed hardwood-conifer stands; C, conifer-dominated stands). Rectangles on the left of each panel are foliar ilr balances based on literature data for healthy and declining SM stands (see Table 5 for details of references and values). Significant differences (P < 0.05) in foliar balances between forest types (not between maple species) are indicated with different letters. A significantly lower balance indicates that concentrations of nutrients in the left part of the balance are significantly higher (or concentrations in right part lower), and vice-versa.
A significant linear relationship was found between foliar signatures (i.e., all balances considered) of SM seedlings and forest floor pH which varied with forest types (adjusted R2 = 0.262, P < 0.01, Table 4). However, this relationship is only due to variations in foliar [Mg,Ca|K] balances (adjusted R2 = 0.494, P < 0.01). There is no relationship between foliar signatures of RM seedlings and forest floor pH (adjusted R2 = −0.056, P = 0.83, Table 4). This is in agreement with the observation that foliar balances of RM seedlings do not vary significantly among forest types.
Table 4. Linear model comparisons between foliar ilr balances (response variables) of sugar and red maple seedlings and forest floor pH.
Foliar ilr balances for healthy and declining SM stands that were computed from literature foliar nutrient data are presented in Table 5. The ranges of those foliar balances are represented in Figure 4, together with foliar ilr balances of SM seedlings at St. Hippolyte. Comparisons between data sets can be used to partially assess SM seedling fitness. On the one hand, foliar [Mg,Ca,K|P,N] and [Mg,Ca|K] balances of declining SM stands are higher than foliar balances of healthy stands. On the other hand, foliar [Fv|Elements] balances are lower for declining SM stands than healthy stands. Among these three balances diverging between healthy and declining stands, foliar [Fv|Elements] of SM seedlings in mixed hardwood-conifer and conifer-dominated stands at St. Hippolyte fall within the upper limit of declining stands, whereas foliar [Fv|Elements] of SM seedlings in hardwood stands fall within the range of healthy stands. Foliar [Mg,Ca|K] and [Mg,Ca,K|P,N] balances of SM seedlings in hardwood and mixed hardwood-conifer stands fall within the upper limit of the ranges of healthy stands, whereas the balances of seedlings in conifer-dominated stands fall within or lean toward the lower limit of declining stands for [Mg,Ca|K] and [Mg,Ca,K|P,N], respectively.
Table 5. Foliar ilr balances that were computed based on nutrient concentrations found in the literature for healthy and declining sugar maple trees.
We tested the hypothesis that while nutrient imbalances in SM seedling foliage increase with increasing soil acidity, nutrients in RM seedling foliage are more balanced because this species can better adapt to environments with greater nutrient limitations. Thus, ilr balances were computed from foliar nutrient concentrations of SM and RM seedlings that were growing naturally on forest floors with increasing acidity as conditioned by varying proportions of conifers. Our results support both parts of the hypothesis.
Differences in foliar [Mg|Ca] and [Mg,Ca,K|P,N] balances between SM and RM seedlings directly reflect foliar concentrations of N and Mg in RM seedlings, which are respectively lower and higher than those of SM seedlings (Table 2). Higher foliar Mg concentrations tend to lower the [Mg|Ca] balance, whereas lower N concentrations tend to lower the [Mg,Ca,K|P,N] balance. Concentrations of foliar nutrients in RM seedlings were previously reported as being lower than those of SM seedlings in ecosystems that are characterized by acidic soils, according to St. Clair and Lynch (2005b). These authors explained that lower nutrients in RM foliage could be due, in part, to lower nutrient requirements of RM. However, at St. Hippolyte, foliar nutrients of RM seedlings were not necessarily lower than those of SM seedlings. Moreover, foliar nutrients of SM and RM seedlings at our study site were generally in the same range as values that have been reported in other recent studies for SM and RM seedlings (Figure 1), reinforcing the idea that seedlings of various ages can be compared relatively well as long as they have similar physiological ages (Marschner, 2011).
Back-transformations of foliar [P|N] balances to N:P ratios at St. Hippolyte yielded values averaging 16.6 (±0.6) for SM seedlings and 17.5 (±4.5) for RM seedlings (data not shown). A meta-analysis of Redfield ratios of terrestrial plant species in natural field sites revealed an average value of 12–13 (Güsewell, 2004). N:P ratios vary widely among and within species, with intraspecific variation being greater than interspecific variation. Ratios < 10 and >20 generally correspond respectively to N- and P-limited biomass production (Güsewell, 2004). The higher average N:P ratio in St. Hippolyte compared to the mean ratio that was reported by Güsewell (2004) is most likely the consequence of the low foliar P concentrations at our study site. Foliar P concentrations of both SM and RM seedlings at St. Hippolyte are at the lower limit of values that are reported in the literature (Figure 1). This could be due to the fact that both species are at the northern limits of their distributions. Lower soil temperatures that are found at the northern limit tend to decrease nutrient turnover and availability (Zak et al., 1999), and ion uptake by plant roots (Dong et al., 2001; Pregitzer and King, 2005). However, we believe that low foliar P concentrations at St. Hippolyte are more likely related to its very specific bedrock and parent material geochemistry (see Materials and Methods section for details), which in turn affect soil P availability and plant uptake. As a whole, the podzolic B horizons have very low Mehlich III extractable P concentrations (average of 0.92 mg P kg−1, Collin et al. submitted) and total P2O5 contents (~0.02%) (Bélanger et al., 2012).
Increasing proportions of conifers and soil acidity resulted in modifications of foliar ilr balances only for SM seedlings (Figure 4). A significant decline in the foliar [Fv|Elements] balance with an increasing proportion of conifers indicates a general decrease in nutrients or an increase in Fv. The Fv is composed of elements that are not often measured, particularly in forest studies, viz., C, H, O, other macronutrients (e.g., S) and micronutrients (e.g., Cu, Zn, B). An increase in foliar Al and Mn concentrations in SM seedlings under an increasing proportion of conifers may be one factor explaining Fv variation. Increases in foliar Al and Mn have been recorded in SM due to decreasing soil pH (Hallett et al., 2006; Kogelmann and Sharpe, 2006; Schaberg et al., 2006; Long et al., 2009; Park and Yanai, 2009; Beauregard et al., 2010). High ionic activity of Al and Mn in acidic soil solution is common and this tends to increase their uptake by tree roots at the expense of Ca and Mg, including SM (Cronan and Grigal, 1995; Likens et al., 1998). A significant increase in the foliar [Mg,Ca,K|P,N] balance can be due to a decrease in Mg, Ca or K concentrations, or an increase in P and N concentrations. However, our data suggest that the latter explanation is compromised, as foliar N and P concentrations of SM seedlings did not vary between forest types (Table 2). Thus, the significantly higher foliar [Mg,Ca|K] balance of SM seedlings in conifer-dominated stands compared to SM seedlings in hardwood stands, together with stability of the foliar [Mg|Ca] balance between forest types, could mean that Ca and Mg are deficient in conifer-dominated stands compared to hardwood stands. The foliar [Mg,Ca|K] balance was also the main factor explaining foliar ilr balance discrimination among forest types (Table 4). Differences in foliar balances, therefore, are clearly related to decreasing soil pH and most probably increased Al and Mn mobility/availability. The latter are both conditioned by increasing proportions of conifers. In turn, there is an antagonistic effect on Ca and Mg uptake by SM seedlings that subsequently translates into foliar imbalances. These results support the first part of our hypothesis that nutrient imbalances in SM foliage increase due to increasing soil acidity as conditioned by increasing proportions of conifers.
It is well known that SM trees are particularly sensitive to low Ca and Mg availability compared to other tree species that are growing on acidic soils (Kobe et al., 2002; Duchesne and Ouimet, 2009; Long et al., 2009). Most declining SM stands in southern Quebec were attributed to low soil Ca and Mg availability, in part, due to imbalances in Al and Mn that were induced by atmospheric acid deposition. Imbalances could also be attributed to exports of Ca and Mg in harvested biomass in excess of natural inputs, resulting in low foliar Ca and Mg (Duchesne et al., 2002; Ouimet et al., 2006; Duchesne and Ouimet, 2009). The health and vigor of SM trees is constrained by the low Ca and Mg availability of acidic soils, and accompanied by foliar Ca and Mg concentrations below deficiency thresholds (Drohan et al., 2002; Bailey et al., 2004; Houle et al., 2007; St. Clair et al., 2008; Long et al., 2009). Foliar Mg concentrations in declining SM stands are often very low and correlated with a decline in canopy health (Horsley et al., 2000). The benefits of liming declining stands of SM on rates of photosynthesis or growth were demonstrated through field studies (Liu et al., 1997; Duchesne et al., 2003; Moore and Ouimet, 2006; Schaberg et al., 2006; Moore et al., 2014). Calcium and Mg deficiencies of SM seedlings in conifer-dominated stands compared to hardwood stands at St. Hippolyte suggests that conifer-dominated boreal forests could limit the ability of SM to migrate northward to find a more adequate climate in a rapidly warming world. Our results only reflect the best-case scenario as the SM seedlings that were measured were those capable of naturally growing even under more stressful conditions that were found in conifer-dominated stands. Many conifer-dominated stands, for example, were not selected for the study because SM seedlings were not present. Similarly, the only foliar balances of SM seedlings that were significantly different among forest types at St. Hippolyte, i.e., [Fv|Elements], [Mg,Ca,K|P,N], [Mg,Ca|K] (Table 3, Figure 2), were also those differing between healthy and declining SM stands found in the literature (Table 4, Figure 4). Because the data linking yield, fitness and foliar nutrition are unfortunately limited to SM trees, not to SM seedlings, it is likely more appropriate to refer to samples in conifer-dominated stands as poorly acclimating seedlings rather than declining seedlings.
Foliar balances of RM seedlings at St. Hippolyte do not show any significant difference among forest types (Figure 4), nor are foliar balances related to forest floor pH (Table 5). These results indicate a greater capacity of RM seedlings to maintain their foliar balances within a certain range of soil acidity (i.e., forest floor pH on the study site ranges from 3.73 to 4.94) than SM seedlings. Greater variation in foliar balances of RM seedlings in the hardwood stands may be the reason that we failed to detect significant differences among forest types. However, the large variation may also reflect higher nutritional plasticity in RM to contrasting environmental conditions. In the past few decades, RM populations in eastern North America have been expanding more rapidly than those of other tree species (Alderman et al., 2005; Fei and Steiner, 2007). Expansion of RM was related to factors as diverse as fire regime, climate, biotic interactions and physiological characteristics. For example, high recruitment capacity that is associated with high seed banking (and, therefore, fast colonization capacity) was proposed as an explanation for its current expansion (Warren et al., 2004; Lambers and Clark, 2005). Lower energy and resource requirements for biomass production and maintenance were also suggested to explain its competitive success (Nagel et al., 2002). As a whole, RM is characterized as a competitive generalist possessing functional traits belonging to both early and late-successional tree species (Abrams, 1998). It is able to thrive on sites with greatly contrasting soil conditions due to its lower water and nutrient requirements for growth and survival compared to many other hardwood species in North America. Our results support the second part of our hypothesis that the lower nutrient requirements of RM seedlings allow the species to adapt nutritionally to a wide range of acid soils compared to SM seedlings. This lower nutrient requirement is another possible factor explaining the recent expansion of RM relative to SM on acidic and nutrient-poor soils.
First, our study indicates that forest floor acidity under conifer-dominated forests negatively affects foliar nutrition of SM seedlings through reduced foliar Ca and Mg concentrations. This leads to alteration of the equilibrium of foliar nutrient balances. In this case, analysis of foliar nutrient concentrations and balances led to similar conclusions. Second, results support our hypothesis that RM seedlings can better adapt its foliar nutrient balances than can SM seedlings through greater tolerance of more acidic soil environments with lower nutrient availability. Interestingly, while RM balances were not significantly different between forest types, foliar nutrient concentrations showed statistically significant decreases with traditional mean comparison (i.e., N and K). Hence, it demonstrates the usefulness of ilr transformations for studying plant nutrition. It should be emphasized that the ilr method is complementary to studying untransformed nutrient concentrations—it does not always provide further information but rather removes any possible bias of working with untransformed data. In this respect, this study demonstrates that the ilr method provides a different perspective for explaining the ecology of RM seedlings. Finally, our study also provides a valuable set of foliar nutrient data for SM and RM seedlings, which are currently sparse in the literature, together with ilr balances of SM that were computed from a comprehensive list of studies. More studies should focus on foliar nutrition of tree seedlings as a means to elucidate their potential for establishing and surviving in new environments under climate change.
AC, designed study, performed research, analyzed data, wrote the paper. NB, contributions to the conception and design of the work, contributions to the acquisition and interpretation of data, contributed to the redaction of parts of the paper, contributed intellectual content in revising the paper. CM, BC, contributions to the conception and design of the work, contributed intellectual content in revising the paper. MF, contributions to the acquisition and interpretation of data, contributed intellectual content in revising the paper.
Financial support was provided through Natural Sciences and Engineering Research Council of Canada Discovery (NSERC) grants (RGPIN 312369-2010 and 2015-03699) to NB.
The authors declare that the research was conducted in the absence of any commercial or financial relationships that could be construed as a potential conflict of interest.
We thank Jacinthe Ricard-Piché and Florence Bélanger for their help in the field and laboratory. We are also grateful to Marie-Claude Turmel and Dominic Bélanger for laboratory analysis, William F. J. Parsons for careful language editing and Serge-Etienne Parent for providing R codes for computing ilr transformations at the initial stage of the project. Finally, we thank the Station de biologie des Laurentides of the Université de Montréal for providing access to the research sites at St. Hippolyte.
Aitchison, J. (1986). The Statistical Analysis of Compositional Data. London: The Blackburn Press; Chapman and Hall.
Aitchison, J., and Greenacre, M. (2002). Biplots of compositional data. J. R. Stat. Soc. Ser. C (Appl. Stat.) 51, 375–392. doi: 10.1111/1467-9876.00275
Alderman, J., Delton, R., Bumgardner, M. S., and Baumgras, J. E. (2005). An assessment of the red maple resource in the northeastern United States. North. J. Appl. Forest. 22, 181–189.
Bacon-Shone, J. (2011). “A short history of compositional data analysis,” in Compositional Data Analysis: Theory and Applications, eds V. Pawlowsky-Glahn and A. Buccianti (New York, NY: John Wiley and Sons), 3–11.
Bailey, S., Horsley, S., Long, R., and Hallett, R. (2004). Influence of edaphic factors on sugar maple nutrition and health on the Allegheny Plateau. Soil Sci. Soc. Am. J. 68, 243–252. doi: 10.2136/sssaj2004.2430
Beauregard, S. L., Côté, B., and Houle, D. (2010). Application of compositional nutrient diagnosis (CND) to the dendrochemistry of three hardwoods in three geological regions of southern Quebec. Dendrochronologia 28, 23–36. doi: 10.1016/j.dendro.2009.01.003
Bélanger, N., Holmden, C., Courchesne, F., Côté, B., and Hendershot, W. H. (2012). Constraining soil mineral weathering 87Sr/86Sr for calcium apportionment studies of a deciduous forest growing on soils developed from granitoid igneous rocks. Geoderma 185–186, 84–96. doi: 10.1016/j.geoderma.2012.03.024
Binkley, D., and Giardina, C. (1998). Why do tree species affect soils? The warp and woof of tree-soil interactions. Biogeochemistry 42, 89–106. doi: 10.1023/A:1005948126251
Brown, C. D., and Vellend, M. (2014). Non-climatic constraints on upper elevational plant range expansion under climate change. Proc. R. Soc. B 281:20141779. doi: 10.1098/rspb.2014.1779
Chayes, F. (1960). On correlation between variables of constant sum. J. Geophys. Res. 65, 4185–4193. doi: 10.1029/JZ065i012p04185
Chen, I.-C., Hill, J. K., Ohlemüller, R., Roy, D. B., and Thomas, C. D. (2011). Rapid range shifts of species associated with high levels of climate warming. Science 333, 1024–1026. doi: 10.1126/science.1206432
Claringbold, P. (1955). Use of the simplex design in the study of joint action of related hormones. Biometrics 11, 174–185. doi: 10.2307/3001794
Cleavitt, N. L., Battles, J. J., Fahey, T. J., and Blum, J. D. (2014). Determinants of survival over 7 years for a natural cohort of sugar maple seedlings in a northern hardwood forest. Can. J. Forest Res. 44, 1112–1121. doi: 10.1139/cjfr-2014-0177
Côté, B., and Camire, C. (1995). Application of leaf, soil, and tree-ring chemistry to determine the nutritional-status of sugar maple on sites of different levels of decline. Water Air Soil Pollut. 83, 363–373. doi: 10.1007/BF00477363
Cronan, C. S., and Grigal, D. F. (1995). Use of calcium/aluminum ratios as indicators of stress in forest ecosystems. J. Environ. Qual. 24, 209–226. doi: 10.2134/jeq1995.00472425002400020002x
Doig, R. (1991). U-Pb zircon dates of Morin anorthosite suite rocks, Grenville Province, Quebec. J. Geol. 99, 729–738. doi: 10.1086/629535
Dong, S., Scagel, C. F., Cheng, L., Fuchigami, L. H., and Rygiewicz, P. T. (2001). Soil temperature and plant growth stage influence nitrogen uptake and amino acid concentration of apple during early spring growth. Tree Physiol. 21, 541–547. doi: 10.1093/treephys/21.8.541
Drohan, P., Stout, S., and Petersen, G. (2002). Sugar maple (Acer saccharum Marsh.) decline during 1979–1989 in northern Pennsylvania. Forest Ecol. Manag. 170, 1–17. doi: 10.1016/S0378-1127(01)00688-0
Duchesne, L., and Ouimet, R. (2009). Present-day expansion of American beech in northeastern hardwood forests: does soil base status matter? Can. J. Forest Res. 39, 2273–2282. doi: 10.1139/X09-172
Duchesne, L., Ouimet, R., and Houle, D. (2002). Basal area growth of sugar maple in relation to acid deposition, stand health, and soil nutrients. J. Environ. Qual. 31, 1676–1683. doi: 10.2134/jeq2002.1676
Duchesne, L., Ouimet, R., and Morneau, C. (2003). Assessment of sugar maple health based on basal area growth pattern. Can. J. Forest Res. 33, 2074–2080. doi: 10.1139/x03-141
Egozcue, J. J., and Pawlowsky-Glahn, V. (2005). Groups of parts and their balances in compositional data analysis. Math. Geol. 37, 795–828. doi: 10.1007/s11004-005-7381-9
Egozcue, J. J., Pawlowsky-Glahn, V., Mateu-Figueras, G., and Barcelo-Vidal, C. (2003). Isometric logratio transformations for compositional data analysis. Math. Geol. 35, 279–300. doi: 10.1023/A:1023818214614
Fahey, T. J., and Blum, J. D. (2011). Litter layers (Oie) as a calcium source of sugar maple seedlings in a northern hardwood forest. Can. J. Forest Res. 41, 898–901. doi: 10.1139/x11-023
Fei, S., and Steiner, K. C. (2007). Evidence for increasing red maple abundance in the eastern United States. For. Sci. 53, 473–477.
Graignic, N., Tremblay, F., and Bergeron, Y. (2014). Geographical variation in reproductive capacity of sugar maple (Acer saccharum Marshall) northern peripheral populations. J. Biogeogr. 41, 145–157. doi: 10.1111/jbi.12187
Güsewell, S. (2004). N:P ratios in terrestrial plants: variation and functional significance. New Phytol. 164, 243–266. doi: 10.1111/j.1469-8137.2004.01192.x
Hallett, R. A., Bailey, S. W., Horsley, S. B., and Long, R. P. (2006). Influence of nutrition and stress on sugar maple at a regional scale. Can. J. Forest Res. 36, 2235–2246. doi: 10.1139/x06-120
Horsley, S. B., Long, R. P., Bailey, S. W., Hallett, R. A., and Hall, T. J. (2000). Factors associated with the decline disease of sugar maple on the Allegheny Plateau. Can. J. Forest Res. 30, 1365–1378. doi: 10.1139/x00-057
Houle, D., Tremblay, S., and Ouimet, R. (2007). Foliar and wood chemistry of sugar maple along a gradient of soil acidity and stand health. Plant Soil 300, 173–183. doi: 10.1007/s11104-007-9401-7
Juice, S. M., Fahey, T. J., Siccama, T. G., Driscoll, C. T., Denny, E. G., Eagar, C., et al. (2006). Response of sugar maple to calcium addition to northern hardwood forest. Ecology 87, 1267–1280. doi: 10.1890/0012-9658(2006)87[1267:ROSMTC]2.0.CO;2
Kobe, R. K., Likens, G. E., and Eagar, C. (2002). Tree seedling growth and mortality responses to manipulations of calcium and aluminum in a northern hardwood forest. Can. J. Forest Res. 32, 954–966. doi: 10.1139/x02-018
Kogelmann, W. J., and Sharpe, W. E. (2006). Soil acidity and manganese in declining and nondeclining sugar maple stands in Pennsylvania. J. Environ. Qual. 35, 433–441. doi: 10.2134/jeq2004.0347
Kolb, T., and McCormick, L. (1993). Etiology of sugar maple decline in four Pennsylvania stands. Can. J. Forest Res. 23, 2395–2402. doi: 10.1139/x93-296
Lambers, J. H. R., and Clark, J. S. (2005). The benefits of seed banking for red maple (Acer rubrum): maximizing seedling recruitment. Can. J. Forest Res. 35, 806–813. doi: 10.1139/x05-017
Likens, G., Driscoll, C., Buso, D., Siccama, T., Johnson, C., Lovett, G., et al. (1998). The biogeochemistry of calcium at Hubbard Brook. Biogeochemistry 41, 89–173. doi: 10.1023/A:1005984620681
Liu, X., Ellsworth, D. S., and Tyree, M. T. (1997). Leaf nutrition and photosynthetic performance of sugar maple (Acer saccharum) in stands with contrasting health conditions. Tree Physiol. 17, 169–178. doi: 10.1093/treephys/17.3.169
Loarie, S. R., Duffy, P. B., Hamilton, H., Asner, G. P., Field, C. B., and Ackerly, D. D. (2009). The velocity of climate change. Nature 462, 1052–1055. doi: 10.1038/nature08649
Loladze, I., and Elser, J. J. (2011). The origins of the Redfield nitrogen-to-phosphorus ratio are in a homoeostatic protein-to-rRNA ratio. Ecol. Lett 14, 244–250. doi: 10.1111/j.1461-0248.2010.01577.x
Long, R. P., Horsley, S. B., Hallett, R. A., and Bailey, S. W. (2009). Sugar maple growth in relation to nutrition and stress in the northeastern United States. Ecol. Appl. 19, 1454–1466. doi: 10.1890/08-1535.1
Lozano, F. C., and Huynh, K. D. (1989). Foliar diagnosis of sugar maple decline by DRIS. Commun. Soil Sci. Plant Anal. 20, 1895–1914. doi: 10.1080/00103628909368191
Marschner, H. (2011). Marschner's Mineral Nutrition of Higher Plants, 3rd Edn. London: Academic Press.
McMahon, S. M., Harrison, S. P., Armbruster, W. S., Bartlein, P. J., Beale, C. M., Edwards, M. E., et al. (2011). Improving assessment and modelling of climate change impacts on global terrestrial biodiversity. Trends Ecol. Evol. 26, 249–259. doi: 10.1016/j.tree.2011.02.012
McWilliams, W. H., White, R., Arner, S. L., Nowak, C. A., and Stout, S. L. (1996). Characteristics of Declining Forest Stands on the Allegheny National Forest. Newtown Square, PA: Northeastern Forest Experiment Station. USDA, Forest Service Research Note NE-360.
Momen, B., Behling, S. J., Lawrence, G. B., and Sullivan, J. H. (2015). Photosynthetic and growth response of sugar maple (Acer saccharum Marsh.) mature trees and seedlings to calcium, magnesium, and nitrogen additions in the Catskill Mountains, NY, USA. PLoS ONE 10:e0136148. doi: 10.1371/journal.pone.0136148
Moore, J.-D., Duchesne, L., and Ouimet, R. (2008). Soil properties and maple–beech regeneration a decade after liming in a northern hardwood stand. Forest Ecol. Manag. 255, 3460–3468. doi: 10.1016/j.foreco.2008.02.026
Moore, J.-D., and Ouimet, R. (2006). Ten-year effect of dolomitic lime on the nutrition, crown vigor, and growth of sugar maple. Can. J. Forest Res 36, 1834–1841. doi: 10.1139/x06-081
Moore, J.-D., Ouimet, R., Long, R. P., and Bukaveckas, P. A. (2014). Ecological benefits and risks arising from liming sugar maple dominated forests in northeastern North America. Environ. Rev. 23, 66–77. doi: 10.1139/er-2014-0048
Nagel, J. M., Griffin, K. L., Schuster, W. S., Tissue, D. T., Turnbull, M. H., Brown, K. J., et al. (2002). Energy investment in leaves of red maple and co-occurring oaks within a forested watershed. Tree Physiol. 22, 859–867. doi: 10.1093/treephys/22.12.859
Oksanen, J., Blanchet, F. G., Kindt, R., Legendre, P., Minchin, P. R., O'Hara, R., et al. (2013). Package ‘vegan’. R Packag version 2.3–3.
Ouimet, R., Arp, P. A., Watmough, S. A., Aherne, J., and DeMerchant, I. (2006). Determination and mapping critical loads of acidity and exceedances for upland forest soils in Eastern Canada. Water Air Soil Poll. 172, 57–66. doi: 10.1007/s11270-005-9050-5
Ouimet, R., and Camiré, C. (1995). Foliar deficiencies of sugar maple stands associated with soil cation imbalances in the Quebec Appalachians. Can. J. Soil Sci. 75, 169–175. doi: 10.4141/cjss95-024
Parent, L.-E. (2011). Diagnosis of the nutrient compositional space of fruit crops. Rev. Bras. Frutic. 33, 321–334. doi: 10.1590/S0100-29452011000100041
Parent, S. E., Parent, S.-É., Hébert-Gentile, V., Naess, K., and Lapointe, L. (2013). Mineral balance plasticity of cloudberry (Rubus chamaemorus) in Quebec-Labrador bogs. Am. J.Plant Sci. 4, 1508–1520. doi: 10.4236/ajps.2013.47183
Parent, L. E., Parent, S.-É., Rozane, D., Amorim, D., Hernandes, A., and Natale, W. (2012). “Unbiased approach to diagnose the nutrient status of red guava (Psidium guajava),” in III International Symposium on Guava and other Myrtaceae, Vol. 959, eds C. A. F. Santos, S. K. Mitra, and J. L. Griffis Jr. (Petronlina), 145–159.
Parent, S.E, Parent, L., Rozane, D., Hernandes, A., and Natale, W. (2012). “Nutrient balance as paradigm of plant and soil chemometrics,” in Soil Fertility, ed R. N. Issaka (New York, NY:InTech Publ), 83–114.
Park, B. B., and Yanai, R. D. (2009). Nutrient concentrations in roots, leaves and wood of seedling and mature sugar maple and American beech at two contrasting sites. Forest Ecol. Manag. 258, 1153–1160. doi: 10.1016/j.foreco.2009.06.003
Parmesan, C., and Yohe, G. (2003). A globally coherent fingerprint of climate change impacts across natural systems. Nature 421, 37–42. doi: 10.1038/nature01286
Pearson, K. (1897). Mathematical contributions to the theory of evolution. On a form of spurious correlation which may arise when indices are used in the measurement of organs. Proc. R. Soc. Lond. 60, 489–502. doi: 10.1098/rspl.1896.0076
Pregitzer, K. S., and King, J. S. (2005). “Effects of soil temperature on nutrient uptake,” in Nutrient Acquisition by Plants, ed H. BassiriRad (Berlin; Heidelberg: Springer), 277–310.
R Core Team (2013). R: A Language and Environment for Statistical Computing. Vienna: R Foundation for Statistical Computing. Available online at: http://www.R-project.org/
Rosenzweig, C., Karoly, D., Vicarelli, M., Neofotis, P., Wu, Q., Casassa, G., et al. (2008). Attributing physical and biological impacts to anthropogenic climate change. Nature 453, 353–357. doi: 10.1038/nature06937
Saucier, J., Robitaille, A., and Grondin, P. (2009). Cadre Bioclimatique du Québec. Écologie Forestière. Manuel de foresterie, 2nd Edn. Québec: Ordre des Ingénieurs Forestiers du Québec, 186–205.
Schaberg, P. G., Tilley, J. W., Hawley, G. J., DeHayes, D. H., and Bailey, S. W. (2006). Associations of calcium and aluminum with the growth and health of sugar maple trees in Vermont. Forest Ecol. Manag. 223, 159–169. doi: 10.1016/j.foreco.2005.10.067
Schrevens, E., and Cornell, J. (1993). Design and analysis of mixture systems: applications in hydroponic, plant nutrition research. Plant Soil 154, 45–52. doi: 10.1007/BF00011070
Soil Classification Working Group (1998). The Canadian System of Soil Classification. 3rd Edn. Agriculture and Agri-Food Canada Publication 1646. Ottawa: NRC Research Press, 187.
St. Clair, S. B., and Lynch, J. P. (2005a). Base cation stimulation of mycorrhization and photosynthesis of sugar maple on acid soils are coupled by foliar nutrient dynamics. New Phytol. 165, 581–590. doi: 10.1111/j.1469-8137.2004.01249.x
St. Clair, S. B., and Lynch, J. P. (2005b). Differences in the success of sugar maple and red maple seedlings on acid soils are influenced by nutrient dynamics and light environment. Plant Cell Environ. 28, 874–885. doi: 10.1111/j.1365-3040.2005.01337.x
St. Clair, S. B., Sharpe, W. E., and Lynch, J. P. (2008). Key interactions between nutrient limitation and climatic factors in temperate forests: a synthesis of the sugar maple literature. Can. J. Forest Res. 38, 401–414. doi: 10.1139/X07-161
van den Boogaart, K. G., Tolosana, R., and Bren, M. (2013). Compositions: Compositional Data Analysis. R package version 1.30–31.
Venables, W. N., and Ripley, B. D. (2002). Modern Applied Statistics with S, 4th Edn. New York, NY: Springer.
Warren, R. J., Rossell, I. M., and Moorhead, K. K. (2004). Colonization and establishment of red maple (Acer rubrum) in a southern Appalachian wetland. Wetlands 24, 364–374. doi: 10.1672/0277-5212(2004)024[0364:CAEORM]2.0.CO;2
Wilkinson, S., Grunes, D., and Sumner, M. (2000). “Nutrient interactions in soil and plant nutrition,” in Handbook of Soil Science, ed M. E. Sumner (Boca Raton, FL: CRC Press), 89–112.
Zak, D. R., Holmes, W. E., MacDonald, N. W., and Pregitzer, K. S. (1999). Soil temperature, matric potential, and the kinetics of microbial respiration and nitrogen mineralization. Soil Sci. Soc. Am. J. 63, 575–584. doi: 10.2136/sssaj1999.03615995006300030021x
Zhang, Y., Bergeron, Y., Zhao, X.-H., and Drobyshev, I. (2015). Stand history is more important than climate in controlling red maple (Acer rubrum L.) growth at its northern distribution limit in western Quebec, Canada. J. Plant Ecol. 8, 368–379. doi: 10.1093/jpe/rtu029
Keywords: seedlings, isometric log-ratio, conifers, soil acidity, ecological gradient, foliar nutrients
Citation: Collin A, Messier C, Côté B, Fontana M and Bélanger N (2016) Contrasting Nutritional Acclimation of Sugar Maple (Acer saccharum Marsh.) and Red Maple (Acer rubrum L.) to Increasing Conifers and Soil Acidity as Demonstrated by Foliar Nutrient Balances. Front. Ecol. Evol. 4:85. doi: 10.3389/fevo.2016.00085
Received: 04 March 2016; Accepted: 08 July 2016;
Published: 21 July 2016.
Edited by:
Leon Etienne Parent, Laval University, CanadaCopyright © 2016 Collin, Messier, Côté, Fontana and Bélanger. This is an open-access article distributed under the terms of the Creative Commons Attribution License (CC BY). The use, distribution or reproduction in other forums is permitted, provided the original author(s) or licensor are credited and that the original publication in this journal is cited, in accordance with accepted academic practice. No use, distribution or reproduction is permitted which does not comply with these terms.
*Correspondence: Nicolas Bélanger, YmVsYW5nZXIubmljb2xhc0B0ZWx1cS5jYQ==
Disclaimer: All claims expressed in this article are solely those of the authors and do not necessarily represent those of their affiliated organizations, or those of the publisher, the editors and the reviewers. Any product that may be evaluated in this article or claim that may be made by its manufacturer is not guaranteed or endorsed by the publisher.
Research integrity at Frontiers
Learn more about the work of our research integrity team to safeguard the quality of each article we publish.