- 1Evolutionary Physiology Group, Max Planck Institute for Ornithology, Seewiesen, Germany
- 2Behavioural Ecology and Ecophysiology Group, Department of Biology, University of Antwerp, Antwerp, Belgium
- 3Didactica Research Unit, Antwerp School of Education, University of Antwerp, Antwerp, Belgium
Much research on animal communication has addressed how costs or constraints determined by the oxidative status of an individual can assure the honesty of visual signals, such as sexually selected color ornaments. However, acoustic communication has been largely overlooked in this respect. Here, we describe the few available studies that have considered the role of oxidative status in mediating vocal behavior in adult and nestling birds. Further, we discuss the theoretical principles of how the honesty of avian acoustic signals may be maintained by an organism's oxidative status. We here distinguish between studies that considered songs and begging calls as indicators of oxidative status and studies where vocalizations were assumed to be the source of oxidative costs. We outline experimental and methodological issues related to the study of bird vocalizations and oxidative stress and describe opportunities for future work in this field of research. Investigating the interactions between acoustic signals and redox state may help address some unresolved questions in avian vocalization, thereby increasing our understanding of the evolutionary pressures shaping animal communication. Finally, we argue that it will be important to extend this line of research beyond birds and include other taxa as well.
Introduction
Acoustic signals are a major mode of communication that is used to repel rivals, attract mates, or signal to parents and relatives in many animal taxa. Among arthropods, sound communication is limited to certain insect species such as orthopterans, cicadas, a few decapod species, arachnids, and millipedes (Bradbury and Vehrencamp, 1998). These invertebrates produce their acoustic signals in many ways, such as by rubbing one part of the body against the other, by vibrating appendages, and other structures or by tapping on specific substrates. By contrast, the majority of vertebrates that communicate vocally (mainly anurans, birds and mammals) generate acoustic signals by utilizing the air inhaled when breathing. The characteristic that renders birdsong a “special case” among vertebrates is that, contrary to mammals (except for human speech and few other species) and anurans for which vocalizations are innate traits, songbirds (oscines) learn their acoustic signals from conspecifics, accurately memorizing them for their entire life and even inventing new parts of song (Miller et al., 2008). These features makes birdsong a cognitive performance in every respect (Buchanan et al., 2013; Sewall et al., 2013), as well as a unique example of complex acoustic communication that has often been paralleled to human speech because of the neuronal development, control, and learning processes involved (Catchpole and Slater, 2008; Miller et al., 2008; Buchanan et al., 2013). In general, brain function and congnitive performance can be severely impaired by conditions of oxidative stress (i.e., impairment of the redox homeostasis; Halliwell and Gutteridge, 2007), with important implications for physiological, ecological, and evolutionary processes. Here we will briefly review the state of knowledge of the relationships between bird vocalizations and oxidative stress. Since we are not aware of any study that linked redox homeostasis to acoustic communication in vertebrates other than birds, we will focus this review on the “avian case.”
From an evolutionary perspective, except for arbitrary traits evolved by Fisherian runaway processes (Fisher, 1930), the game between emitters and receivers must be played by reliable counterparts that honestly signal their state (Polnaszek and Stephens, 2014), even in the case of nestlings emitting calls to communicate their state of demand (Johnstone, 1999). Signals may be honest either because they are costly to produce and only individuals in an optimal condition can sustain these costs (handicap principle; Zahavi, 1975) or because they reflect the condition of the signaler (Andersson, 1994; Hill, 2011; Garratt and Brooks, 2012), and thus its capacity to maintain optimal cellular functioning in spite of environmental challenges, as defined in Hill (2011). Note that the latter hypothesis does not invoke a cost of signaling to ensure signal honesty (Hill, 2011).
The oxidative status (or redox state) of an organism is a major component of body condition in all aerobic species, which use oxygen to efficiently produce energy. Reactive Oxygen Species (ROS) are potentially toxic by-products of cellular catabolism that are able to impair organismal function at several levels (Halliwell and Gutteridge, 2007). To avoid the risk of entering a state of oxidative stress, the organism has to counterbalance the oxidative actions of ROS with an appropriate anti-oxidant defense. One current hypothesis proposes that the balance between oxidants and antioxidants (i.e., the redox state) can mediate life-history trade-offs and affect the fitness of an individual (Costantini, 2008; Monaghan et al., 2009; Metcalfe and Alonso-Alvarez, 2010; Garratt and Brooks, 2012). Birdsong can be viewed as the expression of a cognitive trait that involves several brain functions such as perception, learning, and memory (Buchanan et al., 2013). Birdsong is also the consequence of a careful synchronization of well-defined brain nuclei that can be vulnerable to oxidative damage (von Schantz et al., 1999; Hill, 2011). Although, a role of the redox state in assuring the honesty of birdsong has been proposed 17 years ago (von Schantz et al., 1999), it has only been investigated recently and in a few studies (Van Hout et al., 2011; Casagrande et al., 2014; Baldo et al., 2015; Costantini et al., 2015). This strongly contrasts with the extensive literature on the mediation of visual signal by oxidative status (reviewed e.g., Blount et al., 2001; McGraw, 2008; Svensson and Wong, 2011; Hill and Johnson, 2012). Here we provide an overview of what it is known about the relationship between bird vocalizations and oxidative stress, considering both songs produced by adults and calls emitted by nestlings. We outline some physiological processes that may be the reason why the production of vocal signals is vulnerable to oxidative stress, thus potentially reflecting the oxidative status of an individual. We also discuss evidence for bird vocalizations being costly in terms of generating oxidative stress. Finally, we indicate gaps in our knowledge that should be addressed to better understand the complex interactions between the redox state and the production of acoustic signals in birds but also in other taxa.
Oxidative Status and Song Activity
Birdsong is a signal that functions mainly in the context of sexual selection, specifically in territory defense and mate attraction (Gil and Gahr, 2002; Naguib and Riebel, 2014). Field studies have consistently demonstrated that song traits (e.g., singing activity, song structure, or song repertoire) are linked to mating success and paternity (reviewed in Naguib and Riebel, 2014). Likewise, laboratory studies have shown that females can select males based on song traits (Nowicki and Searcy, 2004; Catchpole and Slater, 2008). Birds possess specific interconnected brain nuclei that are designated to coordinate the syringeal, respiratory, and beak gape motor systems necessary for the production of acoustic signals (Goller et al., 2004; Nottebohm, 2005). Birdsong is a cognitive performance that is prone to suffer from oxidative insult. Brain tissue is both particularly active in producing ROS and especially vulnerable to the damage caused by ROS because of a low content of antioxidants (Sorce and Krause, 2009; Costantini and Marasco, 2011; Nayernia et al., 2014). Once redox homeostasis is altered even to a small extent, ROS can damage mitochondrial DNA, altering the synthesis of enzymes and proteins required for oxidative phosphorylation, impairing ATP generation and perturbing electron transfer through further leakage of ROS, thus impairing the neural function (Uttara et al., 2009; Hill, 2014). This condition of oxidative stress is interesting for eco-physiologists, which aim at understanding the evolutionary pressures acting on individuals that are not pathologically impaired in their function. However, a prolonged state of redox imbalance (oxidative stress) can lead to cell apoptosis, neuronal damage, and decreased brain performance (Gilgun-Sherki et al., 2001; Salganik, 2001), thus also to an impaired vocal signals.
In the last decades several studies have investigated the role of the redox state in mediating the expression of visual secondary sexual traits (Alonso-Alvarez et al., 2007; McGraw, 2008; Svensson and Wong, 2011; Garratt and Brooks, 2012; Hill and Johnson, 2012; Simons et al., 2012), but until now only few of them focused on birdsong (Van Hout et al., 2011; Casagrande et al., 2014, 2015; Baldo et al., 2015; Costantini et al., 2015; Table 1). Studies carried out in captive male European starlings (Sturnus vulgaris) to investigate whether song activity can honestly convey information about the redox state, reported that the administration of dietary lutein through the diet was associated with an increase in song rate (Van Hout et al., 2011; Casagrande et al., 2014, 2015). Lutein is a hydroxy-carotenoid synthetized by autotrophic organisms as an essential photosynthetic pigment. Animals have to acquire lutein through their diet. Besides acting as a pigment (Svensson and Wong, 2011; Hill and Johnson, 2012) and as an enhancer of the immune system (Chew and Park, 2004), lutein is mostly known for its antioxidant properties (Zhang et al., 1992; Ben-Dor et al., 2005; Pérez-Rodríguez, 2009; Hill and Johnson, 2012). Specifically, Van Hout et al. (2011) revealed that dietary lutein boosts the rate of undirected song of males housed in large outdoor aviaries. The effects of lutein persisted even when these males were treated with testosterone and shifted their songs toward nestbox-oriented song, a type of directed song used in reproductive contexts (Van Hout et al., 2011). Furthermore, captive male starlings undergoing an experimentally induced inflammation were able to maintain song activity when being provided with dietary lutein, while controls birds without access to extra antioxidants significantly decreased their song activity (Casagrande et al., 2015). The first physiological evidence explaining the positive effect of lutein on song indicated that non-reproducing male starlings supplied with dietary lutein had increased endogenous thiols and decreased circulating pro-oxidants (ROMs), suggesting that an amelioration of the redox state was associated with an increase in song activity (Casagrande et al., 2014). The latter study also demonstrated a negative relationship between oxidative damage and the rate of directed and undirected song, suggesting that the song rate of male starlings may be constrained by oxidative status. From an ecological perspective, an experimental manipulation of the diet simulates natural conditions where the quality of individuals is determined by their interaction with the environment (e.g., because they are better foragers or occupy territories richer in lutein) and their redox state.
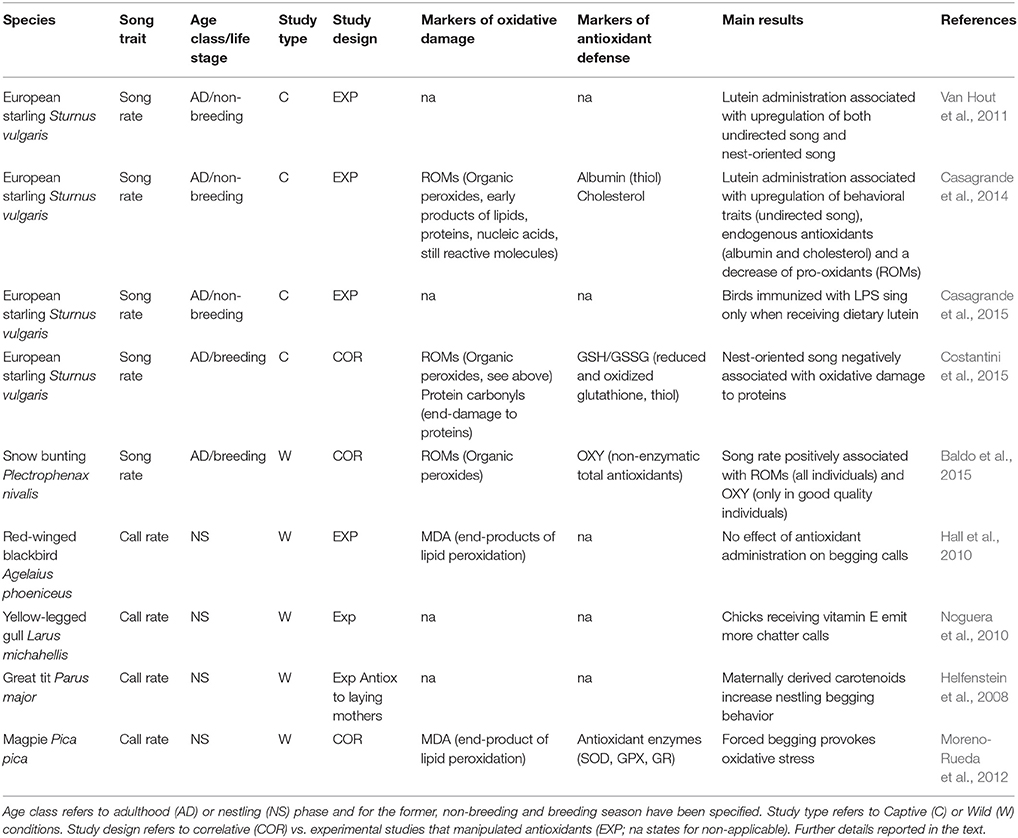
Table 1. Review of existing literature on the relationships between song traits and oxidative stress.
In a follow-up study, the relationship between oxidative status and song in male European starlings was investigated during the breeding season (Costantini et al., 2015). In this experiment, undirected song was not affected by an individual's redox state, while the amount of nest-oriented song, which is the predominant song produced at this time of year, was negatively associated with the level of oxidative damage to proteins. The negative correlation between reactive oxygen metabolites (biomarkers of ongoing oxidative damage to lipids, proteins, and nucleic acids) or protein carbonyls (irreversible damage to proteins) and song rate in captive starlings (Casagrande et al., 2014; Costantini et al., 2015) may suggest that singing activity is not a source of oxidative stress per se and thus does not seem to support the hypothesis that singing performance must entangle costs to be honest, as postulated by the principle of handicap (Zahavi, 1975). However, the seminal paper by Van Noordwijk and de Jong (1986) has nicely demonstrated that heterogeneity in individual quality, and in particular variation in acquisition and allocation of resources, can mask trade-offs. Hence, we cannot exclude that, for example, individuals better able to forage for high quality resources (rich in energy and antioxidants), or better able to monopolize a higher amount of resources, or that are more efficient at up taking antioxidant and other nutrients from their diets, may be able to invest more in singing and in defending themselves against oxidative damage. Experimental manipulation of singing rate is therefore needed to demonstrate whether singing rate is a source, or not, of oxidative stress per se.
A different perspective emerged when free-living birds were studied, as in the case of snow buntings (Plectrophenax nivalis) breeding in a challenging environment such as the Arctic tundra (Baldo et al., 2015). Male snow buntings were sampled upon their arrival on the breeding grounds and were followed through the late egg-laying/early incubation stages. Individuals that sang at a higher rate and that were in better body condition showed a higher level of plasma reactive oxygen metabolites (the same parameter as in the starling study) and a concomitant increase in non-enzymatic plasma antioxidants, indicating that song activity is a pro-oxidant but that individuals in good condition can sustain the oxidative cost of singing by up-regulating their antioxidant defenses. This correlative relationship suggests that oxidative costs arising from song prevent low-quality males from singing at a higher rate. To the best of our knowledge, this is the only study thus far suggesting that song activity is costly in terms of oxidative stress (Baldo et al., 2015). However, from such a correlative approach it is impossible to discern whether the effect was directly caused by singing activity or indirectly induced by other trade-offs that individuals have to face when singing in the wild. Trade-offs with other behaviors such as foraging or self-maintenance processes can expose singing males to increased oxidative risk. For example, a decrease in forage time can limit an individual's capacity to select high-quality food while increasing predation risk. Likewise, decreased investment in self-maintenance processes like immune function exposes individuals to a greater risk of infections. All of these limitations create conditions that could potentially impair the redox state (Casagrande and Groothuis, 2011; Guerra et al., 2013). Hence, further experimental studies are needed to assess whether singing can directly affect the oxidative status. Furthermore, evidence that birdsong can affect metabolic rate is controversial (Oberweger and Goller, 2001; Ward, 2004) and not easy to determine (Gil and Gahr, 2002). Even if a clear relationship between song and energy expenditure would exist, a high metabolic rate does not necessarily equate oxidative stress (Speakman et al., 2015). This is because mitochondrial function during ATP production can vary greatly both among- and within-individuals, affecting the relationship between energy expenditure and oxidative stress levels such that ROS production can be high at times of lowest energy expenditure (Selman et al., 2012; Royle et al., 2015; Salin et al., 2015; Speakman et al., 2015).
Song activity in adulthood depends on the development of its neurological structures during early stages of ontogeny (Nowicki et al., 2002; Buchanan et al., 2003; London and Clayton, 2008; Kubli and MacDougall-Shackleton, 2014). Through such developmental effects, birdsong offers another way of honestly signaling individual condition, even if in this case it would refer to conditions experienced during the early phases of ontogeny (Nowicki and Searcy, 2004; Peters et al., 2014; Schmidt et al., 2014). A key characteristic of the song-learning process is the occurrence of sensitive periods for song memorization, which coincide with the growth of song nuclei (Brenowitz et al., 1997; Nottebohm, 2005) and occur when young birds are likely to face nutritional restrictions (Nowicki et al., 1998). However, nutritional conditions are not the only way in which food can limit the development of song. An experimental study on starling nestlings exposed birds to unpredictable fluctuations in food availability (without a reduction in overall food amount), which are conditions commonly experienced in the wild. Unpredictable food availability during development resulted in reduced song output, shorter song bouts, and reduced repertoire sizes of adults (Spencer et al., 2004). An unpredictable food supply has also been shown to activate the hypothalamus-pituitary-adrenal (HPA) axis that releases glucocorticoids hormones (Fokidis et al., 2012; Marasco et al., 2015), which in turn can have pro-oxidant actions (Costantini et al., 2011a). For example, elevated plasma glucocorticoid concentrations increase the metabolic production of reactive molecule species, decrease the antioxidant protection, and disrupt the repair mechanisms of oxidative damage (Haussmann and Marchetto, 2010). Thus, the rapid increases in glucocorticoid concentrations observed during an endocrine stress response are often associated with a condition of oxidative stress both in the short and in the long term (Haussmann et al., 2012). Further studies are needed to understand the effects of oxidative stress during song development, the role of glucocorticoids during development and adulthood, and whether song nuclei are indeed particularly sensitive to ROS action.
Oxidative Stress and Begging Calls
During the nestling phase, young birds solicit food provisioning by their parents through the repetitive emission of loud calls, characterized by simple non-structured vocalizations. These begging calls are often accompanied by specific behaviors such as wing movements to jostle competitive siblings and certain postures such as stretching the neck and exposing the gape to get closer to the source of food (Johnstone, 1999; Saino et al., 2000; Jacob et al., 2011). Begging calls signal the nutritional state of the nestling and become more intense with increasing hunger. However, in general calls are also related to the condition of the bearer with individuals in better condition able to emit longer and more intense vocalizations (Kilner and Johnstone, 1997; Royle et al., 2002). Although it is tempting to assume that the physical and neurological effort of begging requires a certain amount of energy, empirical evidence for specific metabolic costs for begging is, again, controversial (Chappell and Bachman, 2002). However, studies that evaluated the existence of trade-offs between begging calls and immune function or growth rate are in favor of viewing begging as a costly performance (Kilner, 2001; Moreno-Rueda et al., 2012). Furthermore, there is good evidence that a trade-off exists between oxidative status and begging behavior (Table 1). Chicks of yellow-legged gulls (Larus michahellis) that were food-intake restricted, emitted more chatter calls when receiving vitamin E (Noguera et al., 2010), indicating that these vocalizations (but not other types of calls) were constrained by the redox state (Noguera et al., 2010). Likewise, great tits (Parus major) supplemented with carotenoids during egg-laying produced nestlings that were able to beg more intensively than nestlings hatched from control females (Helfenstein et al., 2008). Magpie (Pica pica) nestlings forced to beg longer than controls by experimentally withholding parental provisioning showed a greater oxidative damage to lipids, indicating that protracted vocalizations are a source of oxidative damage (Moreno-Rueda et al., 2012). However, it cannot be excluded that, among other possible explanations, the stress of waiting for food could have induced the activation of the HPA axis in these nestlings, which can alter the redox state of the organism (Haussmann et al., 2012). Finally, it is important to mention that in red-winged blackbird nestlings (Agelaius phoeniceus) supplementary antioxidants did not have an effect on begging behavior (Hall et al., 2010). A major challenge for future studies is to employ experimental designs that induce birds to call or sing at a higher rate without inflicting stressful conditions and quantifying the effect on the oxidative state.
Future Challenges and Study Directions
Conceptual and Experimental Approaches
As outlined in the Introduction, the honesty of birdsong as signal could be maintained either because it conveys an individual's redox state (i.e., song activity is promoted by increased antioxidant availability, and oxidative damage can impair song characteristics) or because only individuals in good condition can sustain the oxidative costs produced by singing. Although these hypotheses are not mutually exclusive, it is important to clearly distinguish between them from the outset, as they lead to divergent predictions as to how the redox state and birdsong may be related (Figure 1).
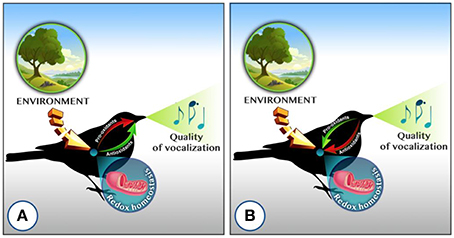
Figure 1. Simplified relationships between an acoustic signal (song or call) and the redox state in relation to two hypotheses on the maintenance of honesty of this behavior: (A) Vocalizations are honest because they convey information about the state of an individual's oxidative status, i.e., oxidative stress limits vocal performance. The main predictions are negative associations between the acoustic signal and circulating pro-oxidants (reactive molecules able to oxidize molecules -ROS), oxidative damage (damage to mainly proteins, nucleic acids and lipids) and enzymatic antioxidants, which are costly to upregulate and increase only when needed. Levels of non-enzymatic endogenous antioxidants (e.g., albumin, glutathione, haptoglobin, uric acid) and dietary antioxidants are expected to be positively related to the acoustic signal (Casagrande et al., 2014). (B) Acoustic signals are honest because they are costly, by producing oxidative damage. In this case an up-regulation of enzymatic antioxidants is expected together with a perturbation of other antioxidants processes that mainly depend on the quality of the individual or of their territory (Baldo et al., 2015). In both cases, the environment (and its interaction with the physiological state) is an important factor that needs to be taken into account. The two scenarios are not mutually exclusive. Red arrows are for down-regulating processes and green arrows for up-regulating ones (Concept of illustration: Lucia Mentesana; graphic realization: Alessandro Candelari).
Moreover, disentangling the direct effects of song from indirect processes caused by trade-offs with behavior or self-maintenance will be crucial for understanding the nature of the costs associated with song, especially in the wild. Similar to the hypothesis suggested for the role of antioxidant pigments in producing honest coloration (i.e., carotenoids have to be traded-off with health functions like immune responses to produce intense coloration; McGraw, 2008; Pike et al., 2010; Vinkler and Albrecht, 2010; Svensson and Wong, 2011), songs or calls may be honest because they have to be traded-off with activities that could ameliorate the redox state (e.g., eating or resting).
Future studies should try to manipulate the oxidative status of singing males (Koch and Hill, 2016). Providing additional antioxidants turned out to be an effective way to manipulate birdsong (see Discussion above), but the mechanisms underlying these effects are still far from being clear. One valuable approach could be to impair the antioxidant defense of an individual to induce a state of oxidative stress. This has recently successfully been done in captivity by reducing glutathione production through a treatment of individuals with butathione sulfoximine (Costantini et al., 2016). An alternative approach could be to assess song performance in relation to external and internal challenges known to produce oxidative stress such as senescence, detrimental developmental conditions, activation of the immune system, or the presence of environmental contaminants. For instance, song has been shown to be sensitive to chemical pollutants, such as heavy metals (Gorissen et al., 2005) and 2,2′,4,4′,5-pentabromodiphenyl ether (BDE-99) (Eng et al., 2012). The involvement of sex steroid hormones in birdsong represents another possible mechanism for oxidative costs associated with singing and it is related to the possible role of pro-oxidant sexual steroids (Alonso-Alvarez et al., 2007), such as testosterone, in mediating birdsong. Two recent studies tested this hypothesis but could not support it (Casagrande et al., 2014; Baldo et al., 2015). At present, we do not consider it likely that testosterone causes the oxidative costs of birdsong, first because the evidence for pro-oxidant effects of testosterone is controversial, and second birdsong is not always testosterone dependent. For example, some species can sing year-round, independent of their testosterone levels (Pinxten et al., 2002; Casagrande et al., 2014). However, further work that considers different contexts, species, and sex steroids is needed to obtain a clearer picture about oxidative costs of song behavior mediated by steroids.
Other approaches that could help to disentangle the oxidative costs of birdsong vs. the limitations imposed by oxidative stress on song would be comparative methods. For example, one could compare individuals of artificially selected lines for singing behavior (e.g., Mundinger, 2010). A general prediction would be that individuals from high-singing lines would show more oxidative damage than those from low-singing ones, which would document that singing is indeed costly in terms of oxidative status. Conversely, the potential limiting effects of the redox state would be expected to be similar for the two lines, provided that they do not differ in their redox state for other reasons. Similar predictions could be made for a comparison among species that vary in the duration of song or the size of repertoires (Garamszegi and Eens, 2004). Another possibility to examine the relationship between oxidative stress and birdsong within species would be to experimentally manipulate song repertoire and activity by tutoring young birds in the laboratory with high- or low-quality models (Gil and Gahr, 2002).
Disentangling the Complexity of Birdsong in Relation to Oxidative Stress
There is still much to understand and know about the role of the redox state in the expression of birdsong. Studies carried out so far have assessed the role of the redox state in influencing singing activity (expressed as song rate) and vice versa. However, song behavior is a multi-faceted activity encompassing multiple traits that are not equally important in different species, across life-history stages or in divergent environments (Gil and Gahr, 2002). For example, species and individuals can differ in their repertoire size (the number of different elements or song types), which in turn can be related to the redox state. Most songbird species sing several types of songs in a redundant modality, i.e., one song type is serially repeated several times in a bout before switching to another type (Catchpole and Slater, 2008). Redundancy can help ensure the correct reception of the signal, while a switching from one song type to another may serve to limit neuromuscular fatigue, which can occur when a muscle is contracted in the same way for a protracted amount of time (“anti-exhaustion hypothesis”; Lambrechts and Dhondt, 1988). It has been observed that great tit males sing for a shorter total amount of time when a single song type was repeated for a long time (“drift” effect; Lambrechts and Dhondt, 1988). Even if the phenomenon of drift is not universally observed across species and individuals (Brumm and Slater, 2006), it suggests that prolonged vocal performance can cause muscular fatigue. This phenomenon is well known in humans where antioxidant supplementation can help avoid the voice impairment and muscle pain that often afflicts professional voice performers (Asher, 2013). The prolonged use of the muscles involved in song production could be a primary source of ROS production, leading to oxidative damage (Reid et al., 1992). In turn, oxidative stress can impair muscle force production (Powers and Jackson, 2008), thereby generating among and within individual variation in song characteristics.
More work is needed to relate different performance related song traits to oxidative stress measurements. However, the association between oxidative damage and song performance may be related to the song repertoire of the individual or the species. For example, individuals with limited repertoires, due to e.g., developmental constraints (Pfaff et al., 2007; Peters et al., 2014; Schmidt et al., 2014) may consistently repeat the same motif thereby inducing muscular fatigue and oxidative damage. In such a case it is possible that oxidative damage experienced during development and manifested for example by telomere shortening or other extensive oxidative damage, overlaps with currently ongoing oxidative stress. The latter may be expressed by circulating levels of pro-oxidants rather than the occurrence of oxidative damage (see below for an explanation about the importance of disentangling these two measures of oxidative status). This is only one possible relationship between redox state and song repertoire. Song is a complex behavior, with different song traits having diverse costs and constrains (Gil and Gahr, 2002). However, at present, studies investigating the effect the oxidative status on song repertoire, accuracy of song production, and structure of motives, amplitude and frequency of the sound and vice versa are completely lacking, while they could greatly contribute in understanding potential oxidative costs and constraints related to expression.
Choosing the Right Parameters to Describe Oxidative Status
This issue has been thoroughly addressed by excellent recent reviews aimed at clarifying which measures ecologists should use to study the redox system (Cohen and McGraw, 2009; Monaghan et al., 2009; Hõrak and Cohen, 2010; Metcalfe and Alonso-Alvarez, 2010; Selman et al., 2012; Speakman et al., 2015). Here we would like to emphasize the importance of carefully evaluating the biological implications that a particular biomarker can have. For example, the antioxidant system is represented by three levels of defense, each having distinctive characteristics and functions (Halliwell and Gutteridge, 2007). The first level is represented by enzymatic antioxidants (mainly glutathione peroxidase and superoxide dismutase), which have the role of detoxifying the organism from ROS before they can damage molecules such as proteins, lipids, or DNA. Their upregulation implies that cells are experiencing an increase in ROS and that they are trying to cope with it (Costantini et al., 2011b; Royle et al., 2015). As an example, the muscle fibers that are activated to produce song can upregulate the concentration of antioxidant enzymes to counteract increased intra-cellular production of ROS (Powers and Jackson, 2008). Thus, an upregulation of the endogenously produced antioxidant barrier can be expected in good quality individuals. However, even though an up-regulation of enzymes may help containing the oxidative insult, it is a costly process (Alonso-Alvarez et al., 2004; Wiersma et al., 2004; Cohen et al., 2008; Monaghan et al., 2009), with possible repercussions both in the short- and in the long-term. A second level of antioxidant defense is represented by non-enzymatic antioxidants synthetized by the organism such as thiols (e.g., glutathione and albumin). These antioxidants have other functions compared to enzymes, which is to scavenge the ROS that have escaped the controlled mitochondrial environment. Plasma concentrations of non-enzymatic antioxidants are difficult to interpret without concomitant quantification of biomarkers of oxidative damage (Costantini and Verhulst, 2009). The third level of antioxidant protection is represented by dietary (and hence exogenous) antioxidants, such as carotenoids, vitamins, and polyphenols. The levels of exogenous anti-oxidants in the body largely depend on their environmental availability and on the capacity of the individual to acquire them. An assessment of total antioxidant protection, which includes both endogenous and exogenous antioxidants, is probably a too general biomarker for studying oxidative stress and birdsong because it cannot assess the relative importance of endogenous and abundant antioxidants from that of essential and potentially limiting dietary antioxidants. Thus, a separate quantification of these antioxidant elements should be considered good practice for assessing their effects on song behavior (Figure 1). Moreover, even if individuals can modulate their capacity to uptake dietary antioxidants, this regulation can be affected by spatial-temporal availability of antioxidants and foraging quality.
Similarly, the assessment of actual oxidative damage to lipids, proteins, and nucleic acids to quantify impairment of vital macromolecules should be separate from those of active pro-oxidants, such as organic hydroperoxides. Organic hydroperoxides are early products of oxidative cascades of an array of substrates including proteins, nucleic acids, and lipids. These cascades end with the production of protein carbonyls, DNA adducts and products of lipid peroxidation (malondialdehyde or MDA; Costantini, 2016). While the latter compounds are markers of oxidative damage, the former are both markers of oxidative damage (Costantini, 2016), and of active pro-oxidants (e.g., Trotta et al., 1981; Runge-Morris et al., 1989; Zavodnik et al., 2013). In addition, organic hydroperoxides have clearly been associated with a non-selective permeabilization of the mitochondrial membrane. Such damages can impair the phosphorylation process and ultimately cell respiration (Zavodnik et al., 2013). Cell respiration is a crucial physiological process that has been proposed to be a unifying mechanism of honest signaling across different species in the animal kingdom (Hill, 2014). The redox biomarker should be based on the song trait of interest. For example, when studying traits related to repertoire size, biomarkers reflecting the redox state of the brain should be selected. The most common biomarkers of oxidative damage that are generated in the brain and measurable in the blood are the end-products of lipid peroxidation, such as isoprostanes (Skoumalova and Hort, 2012). These biomarkers are considered to be enriched in brain tissue that is oxidatively stressed because of its high PUFA (polyunsaturated fatty acids) content. An increase in isoprostanes should be detectable in the blood because these small lipophilic molecules can easily pass the blood-brain barrier. Isoprostanes are also more stable than MDA, which is also an end-product of lipid peroxidation, but one that can still react with other molecules or undergo fast metabolic conversions (Skoumalova and Hort, 2012). Damages to proteins are also common in an oxidatively stressed brain and the quantification of protein carbonyls in the blood can be important (Skoumalova and Hort, 2012). With respect to antioxidants, small lipophilic molecules such as carotenoids and vitamin E are good candidates that cross the blood-brain barrier and therefore can be measured in the circulation (Gilgun-Sherki et al., 2001).
Conclusions
Although, the study of vocalizations in relation to an individual's oxidative status is still at its infancy, some interesting patterns are already emerging. As mentioned above, supplementary antioxidants can boost vocalizations both in adults (song rate) and nestlings (begging intensity; Noguera et al., 2010; Van Hout et al., 2011; Casagrande et al., 2014). However, the overall picture appears to be complex because the same redox biomarker may be up- or down-regulated depending on an individual's condition and environmental quality (Figure 1). In general, in light of the complex relationship of avian communication with the redox state we suggest that studies more strongly focus on the individual level. Like many other behaviors, song is a plastic trait that can be performed depending on individual, social, and environmental conditions. An important step forward in understanding the relationship between oxidative status and birdsong could be the study of within-individual variation across environmental gradients, i.e., a reaction norm approach (Dingemanse et al., 2010; Dingemanse and Wolf, 2013). Individuals could be stimulated to sing more to increase the costs of song, for example by prolonging song duration with conspecific playback, or by inducing louder songs through exposure to noise (Zollinger et al., 2011). Likewise, nestling begging calls could be manipulated by exposing nestlings to stimuli able to solicit their calls but without delaying the consumption of food. Thus, understanding the physiological mechanisms of the interaction of birdsong and oxidative status still presents a formidable challenge for eco-physiologists. Given that the efficiency of cell respiration has been proposed to be a general mechanism of honest signaling across vertebrate species (Hill, 2014), there is an urgent need to study the relationship between oxidative status and vocalizations in taxa other than songbirds. Birds produce their sounds in peculiar ways compared to anurans and mammals. First, birds do not use the larynx but the syrinx, a unique avian organ entirely enclosed in an inter-clavicular resonant air sac. The syrinx is composed of several sets of vibrating membranes that are finely controlled by extrinsic and intrinsic muscles. The muscular control of avian song is highly sophisticated as the two sides of the syrinx can be moved independently and two structured sounds can be produced at once. Secondly, the respiration of birds allows them to maximize their vocalization output, as contrast to anurans and mammals. In birds, the relaxed position during respiration is with the lungs partially filled. Moreover, avian lungs are connected to an extended series of air sacs that guarantee a constant provision of air, suggesting that birds can vocalize during breathing without suffering from hypoxia (Bradbury and Vehrencamp, 1998). Consequently, it is possible that prolonged vocalizations are more costly for mammals and anurans than for birds because the latter have a more efficient respiratory system. Hypoxia is a state of low oxygen levels that can alter mitochondrial function such that the rate of electron-transport is decreased, which usually is associated with an overproduction of ROS (Solaini et al., 2010). As far as we know studies assessing the role of short-repeated hypoxia on redox homeostasis in vocalizing vertebrates still lacking, indicating that more research is needed in this field of ecology.
Author Contributions
SC, RP, ME, Outlined the structure of the review. SC, Wrote the first draft of the manuscript. SC, RP, ME, Contributed to the writing and editing of the manuscript.
Conflict of Interest Statement
The authors declare that the research was conducted in the absence of any commercial or financial relationships that could be construed as a potential conflict of interest.
Acknowledgments
We thank the Subject Editors for inviting us to contribute to this special issue and the reviewers for their constructive comments that substantially improved the manuscript. Lucia Mentesana provided valuable comments on a previous draft of the manuscript. SC is grateful to the Max Planck Institute for Ornithology for the financial support provided during the writing and the editorial processes and in particular to Michaela Hau, for her fundamental backing of this work. RP and ME acknowledge support from the University of Antwerp and FWO-Flanders.
References
Alonso-Alvarez, C., Bertrand, S., Devevey, G., Prost, J., Faivre, B., and Sorci, G. (2004). Increased susceptibility to oxidative stress as a proximate cost of reproduction. Ecol. Lett. 7, 363–368. doi: 10.1111/j.1461-0248.2004.00594.x
Alonso-Alvarez, C., Bertrand, S., Faivre, B., Chastel, O., and Sorci, G. (2007). Testosterone and oxidative stress: the oxidation handicap hypothesis. Proc. R. Soc. Lond. B Biol. Sci. 274, 819–825. doi: 10.1098/rspb.2006.3764
Asher, B. F. (2013). Complementary and integrative treatments. Otolaryngol. Clin. North Am. 46, 437–445. doi: 10.1016/j.otc.2013.02.008
Baldo, S., Mennill, D. J., Guindre-Parker, S., Gilchrist, H. G., and Love, O. P. (2015). The oxidative cost of acoustic signals: examining steroid versus aerobic activity hypotheses in a wild bird. Ethology 121, 1081–1090. doi: 10.1111/eth.12424
Ben-Dor, A., Steiner, M., Gheber, L., Danilenko, M., Dubi, N., Linnewiel, K., et al. (2005). Carotenoids activate the antioxidant response element transcription system. Mol. Cancer Ther. 4, 177–186.
Blount, J. D., Moller, A. P., and Houston, D. C. (2001). Antioxidants, showy males and sperm quality. Ecol. Lett. 4, 393–396. doi: 10.1046/j.1461-0248.2001.00255.x
Bradbury, J. W., and Vehrencamp, S. L. (1998). Principles of Animal Communication, 1st Edn. Sunderland, MA: Sinauer Associates, Inc.
Brenowitz, E. A., Margoliash, D., and Nordeen, K. W. (1997). An introduction to birdsong and the avian song system. J. Neurobiol. 33, 495–500.
Brumm, H., and Slater, P. J. B. (2006). Ambient noise, motor fatigue, and serial redundancy in chaffinch song. Behav. Ecol. Sociobiol. 60, 475–481. doi: 10.1007/s00265-006-0188-y
Buchanan, K. L., Grindstaff, J. L., and Pravosudov, V. V. (2013). Condition dependence, developmental plasticity, and cognition: implications for ecology and evolution. Trends Ecol. Evol. 28, 290–296. doi: 10.1016/j.tree.2013.02.004
Buchanan, K. L., Spencer, K. A., Goldsmith, A. R., and Catchpole, C. K. (2003). Song as an honest signal of past developmental stress in the European starling (Sturnus vulgaris). Proc. Biol. Sci. 270, 1149–1156. doi: 10.1098/rspb.2003.2330
Casagrande, S., and Groothuis, T. G. G. (2011). The interplay between gonadal steroids and immune defence in affecting a carotenoid-dependent trait. Behav. Ecol. Sociobiol. 65, 2007–2019. doi: 10.1007/s00265-011-1210-6
Casagrande, S., Pinxten, R., Zaid, E., and Eens, M. (2014). Carotenoids, birdsong and oxidative status: administration of dietary lutein is associated with an increase in song rate and circulating antioxidants (albumin and cholesterol) and a decrease in oxidative damage. PLoS ONE 9:e115899. doi: 10.1371/journal.pone.0115899
Casagrande, S., Pinxten, R., Zaid, E., and Eens, M. (2015). Birds receiving extra carotenoids keep singing during the sickness phase induced by inflammation. Behav. Ecol. Sociobiol. 69, 1029–1037. doi: 10.1007/s00265-015-1916-y
Catchpole, C. K., and Slater, P. J. B. (2008). Bird Song: Biological Themes and Variations, 2nd Edn. Cambridge, U.K: Cambridge University Press.
Chappell, M. A., and Bachman, G. C. (2002). “Energetic cost of begging behavior,” in The Evolution of Begging: Competition, Cooperation and Communication, eds J. Wright and M. L. Leonard (Dordrecht: Kluwer Academic Press), 143–162.
Chew, B. P., and Park, J. S. (2004). Functions and actions of retinoids and carotenoids: building on the vision of James Allen Olson carotenoid action on the immune response. J. Nutr. 134, 257S–261S.
Cohen, A. A., McGraw, K. J., Wiersma, P., Williams, J. B., Robinson, W. D., Robinson, T. R., et al. (2008). Interspecific associations between circulating antioxidant levels and life-history variation in birds. Am. Nat. 172, 178–193. doi: 10.1086/589456
Cohen, A. A., and McGraw, K. J. (2009). No simple measures for antioxidant status in birds: complexity in inter- and intraspecific correlations among circulating antioxidant types. Funct. Ecol. 23, 310–320. doi: 10.1111/j.1365-2435.2009.01540.x
Costantini, D. (2008). Oxidative stress in ecology and evolution: lessons from avian studies. Ecol. Lett. 11, 1238–1251. doi: 10.1111/j.1461-0248.2008.01246.x
Costantini, D. (2016). Oxidative stress ecology and the d-ROMs test: facts, misfacts and an appraisal of a decade's work. Behav. Ecol. Sociobiol. 70, 809–820. doi: 10.1007/s00265-016-2091-5
Costantini, D., and Marasco, V. (2011). The unsaturated brain: an evolutionary compromise? J. Cell. Biochem. 112, 2201–2202. doi: 10.1002/jcb.23152
Costantini, D., and Verhulst, S. (2009). Does high antioxidant capacity indicate low oxidative stress? Funct. Ecol. 23, 506–509. doi: 10.1111/j.1365-2435.2009.01546.x
Costantini, D., Casagrande, S., Casasole, G., AbdElgawad, H., Asard, H., Pinxten, R., et al. (2015). Immunization reduces vocal communication but does not increase oxidative stress in a songbird species. Behav. Ecol. Sociobiol. 69, 829–839. doi: 10.1007/s00265-015-1899-8
Costantini, D., Casasole, G., AbdElgawad, H., Asard, H., and Eens, M. (2016). Experimental evidence that oxidative stress influences reproductive decisions. Funct. Ecol. doi: 10.1111/1365-2435.12608
Costantini, D., Marasco, V., and Møller, A. P. (2011a). A meta-analysis of glucocorticoids as modulators of oxidative stress in vertebrates. J. Comp. Physiol. B 181, 447–456. doi: 10.1007/s00360-011-0566-2
Costantini, D., Monaghan, P., and Metcalfe, N. B. (2011b). Biochemical integration of blood redox state in captive zebra finches (Taeniopygia guttata). J. Exp. Biol. 214, 1148–1152. doi: 10.1242/jeb.053496
Dingemanse, N. J., and Wolf, M. (2013). Between-individual differences in behavioural plasticity within populations: causes and consequences. Anim. Behav. 85, 1031–1039. doi: 10.1016/j.anbehav.2012.12.032
Dingemanse, N. J., Kazem, A. J. N., Réale, D., and Wright, J. (2010). Behavioural reaction norms: animal personality meets individual plasticity. Trends Ecol. Evol. 25, 81–89. doi: 10.1016/j.tree.2009.07.013
Eng, M. L., Elliot, J. E., MacDougall-Shackleton, S., Letcher, R. J., and Williams, T. D. (2012). Early exposure to 2,2′,4,4′,5-pentabromodiphenyl ether (BDE-99) affects mating behavior of zebra finches. Toxicol. Sci. 127, 269–276. doi: 10.1093/toxsci/kfs076
Fokidis, H. B., des Roziers, M. B., Sparr, R., Rogowski, C., Sweazea, K., and Deviche, P. (2012). Unpredictable food availability induces metabolic and hormonal changes independent of food intake in a sedentary songbird. J. Exp. Biol. 215, 2920–2930. doi: 10.1242/jeb.071043
Garamszegi, L. Z., and Eens, M. (2004). Brain space for a learned task: strong intraspecific evidence for neural correlates of singing behavior in songbirds. Brain Res. Rev. 44, 187–193. doi: 10.1016/j.brainresrev.2003.12.001
Garratt, M., and Brooks, R. C. (2012). Oxidative stress and condition-dependent sexual signals : more than just seeing red. Proc. R. Soc. Lond. B Biol. Sci. 279, 3121–3130. doi: 10.1098/rspb.2012.0568
Gil, D., and Gahr, M. (2002). The honesty of bird song: multiple constraints for multiple traits. Trends Ecol. Evol. 17, 133–141. doi: 10.1016/S0169-5347(02)02410-2
Gilgun-Sherki, Y., Melamed, E., and Offen, D. (2001). Oxidative stress induced-neurodegenerative diseases: The need for antioxidants that penetrate the blood brain barrier. Neuropharmacology 40, 959–975. doi: 10.1016/S0028-3908(01)00019-3
Goller, F., Mallinckrodt, M. J., and Torti, S. D. (2004). Beak gape dynamics during song in the zebra finch. J. Neurobiol. 59, 289–303. doi: 10.1002/neu.10327
Gorissen, L., Snoeijs, T., Van Duyse, E., and Eens, M. (2005). Heavy metal pollution affects dawn singing behaviour in a small passerine bird. Oecologia 145, 504–509. doi: 10.1007/s00442-005-0091-7
Guerra, C., Zenteno-Savín, T., Maeda-Martínez, A. N., Abele, D., and Philipp, E. E. R. (2013). The effect of predator exposure and reproduction on oxidative stress parameters in the Catarina scallop Argopecten ventricosus. Comp. Biochem. Physiol. A Mol. Integr. Physiol. 165, 89–96. doi: 10.1016/j.cbpa.2013.02.006
Hall, M. E., Blount, J. D., Forbes, S., and Royle, N. J. (2010). Does oxidative stress mediate the trade-off between growth and self-maintenance in structured families? Funct. Ecol. 24, 365–373. doi: 10.1111/j.1365-2435.2009.01635.x
Halliwell, B. H., and Gutteridge, J. M. C. (2007). Free Radicals in Biology and Medicine. 4th Edn. Oxford: Oxford University Press.
Haussmann, M. F., Longenecker, A. S., Marchetto, N. M., Juliano, S. A., and Bowden, R. M. (2012). Embryonic exposure to corticosterone modifies the juvenile stress response, oxidative stress and telomere length. Proc. Biol. Sci. 279, 1447–1456. doi: 10.1098/rspb.2011.1913
Haussmann, M. F., and Marchetto, N. M. (2010). Telomeres : linking stress and survival, ecology and evolution. Curr. Zool. 56, 714–727.
Helfenstein, F., Berthouly, A., Tanner, M., Karadas, F., and Richner, H. (2008). Nestling begging intensity and parental effort in relation to prelaying carotenoid availability. Behav. Ecol. 19, 108–115. doi: 10.1093/beheco/arm103
Hill, G. E. (2011). Condition-dependent traits as signals of the functionality of vital cellular processes. Ecol. Lett. 14, 625–634. doi: 10.1111/j.1461-0248.2011.01622.x
Hill, G. E. (2014). Cellular respiration: the nexus of stress, condition, and ornamentation. Integr. Comp. Biol. 54, 645–657. doi: 10.1093/icb/icu029
Hill, G. E., and Johnson, J. D. (2012). The vitamin A-redox hypothesis: a biochemical basis for honest signaling via carotenoid pigmentation. Am. Nat. 180, E127–E150. doi: 10.1086/667861
Hõrak, P., and Cohen, A. (2010). How to measure oxidative stress in an ecological context: methodological and statistical issues. Funct. Ecol. 24, 960–970. doi: 10.1111/j.1365-2435.2010.01755.x
Jacob, S., Rieucau, G., and Heeb, P. (2011). Multimodal begging signals reflect independent indices of nestling condition in European starlings. Behav. Ecol. 22, 1249–1255. doi: 10.1093/beheco/arr121
Johnstone, R. A. (1999). Signaling of need, sibling competition, and the cost of honesty. Proc. Natl. Acad. Sci. U.S.A. 96, 12644–12649.
Kilner, R. M. (2001). A growth cost of begging in captive canary chicks. Proc. Natl. Acad. Sci. U.S.A. 98, 11394–11398. doi: 10.1073/pnas.191221798
Kilner, R., and Johnstone, R. A. (1997). Begging the question: are offspring solicitation behaviours signals of need? Trends Ecol. Evol. 12, 11–15. doi: 10.1016/S0169-5347(96)10061-6
Koch, R. E., and Hill, G. E. (2016). An assessment of techniques to manipulate oxidative stress in animals. Funct. Ecol. doi: 10.1111/1365-2435.12664. [Epub ahead of print].
Kubli, S. P., and MacDougall-Shackleton, E. (2014). Developmental timing of signals affects information content: song complexity but not consistency reflects innate immune strategy in male song sparrows. Am. Nat. 183, 660–670. doi: 10.1086/675757
Lambrechts, M., and Dhondt, A. A. (1988). The anti-exhaustion hypothesis: a new hypothesis to explain song performance and song switching in the great tit. Anim. Behav. 36, 327–334. doi: 10.1016/S0003-3472(88)80002-2
London, S. E., and Clayton, D. F. (2008). Functional identification of sensory mechanisms required for developmental song learning. Nat. Neurosci. 11, 579–586. doi: 10.1038/nn.2103
Marasco, V., Boner, W., Heidinger, B., Griffiths, K., and Monaghan, P. (2015). Repeated exposure to stressful conditions can have beneficial effects on survival. Exp. Gerontol. 69, 170–175. doi: 10.1016/j.exger.2015.06.011
McGraw, K. J. (2008). An update on the honesty of melanin-based color signals in birds. Pigment Cell Melanoma Res. 21, 133–138. doi: 10.1111/j.1755-148X.2008.00454.x
Metcalfe, N. B., and Alonso-Alvarez, C. (2010). Oxidative stress as a life-history constraint: the role of reactive oxygen species in shaping phenotypes from conception to death. Funct. Ecol. 24, 984–996. doi: 10.1111/j.1365-2435.2010.01750.x
Miller, J. E., Spiteri, E., Condro, M. C., Dosumu-johnson, R. T., Geschwind, D. H., and White, S. A. (2008). Birdsong decreases protein levels of foxP2, a molecule required for human speech. J. Neurophysiol. 100, 2015–2025. doi: 10.1152/jn.90415.2008
Monaghan, P., Metcalfe, N. B., and Torres, R. (2009). Oxidative stress as a mediator of life history trade-offs: mechanisms, measurements and interpretation. Ecol. Lett. 12, 75–92. doi: 10.1111/j.1461-0248.2008.01258.x
Moreno-Rueda, G., Redondo, T., Trenzado, C. E., Sanz, A., and Zúñiga, J. M. (2012). Oxidative stress mediates physiological costs of begging in magpie (Pica pica) nestlings. PLoS ONE 7:e40367. doi: 10.1371/journal.pone.0040367
Mundinger, P. C. (2010). Behaviour genetic analysis of selective song learning in three inbred canary strains. Behaviour 147, 705–723. doi: 10.1016/0003-3472(95)80006-9
Naguib, M., and Riebel, K. (2014). “Singing in space and time: the biology of birdsong,” in Biocommunication of Animals, ed E. G. Witzany (Dordrecht: Springer), 233–247. doi: 10.1007/978-94-007-7414-8
Nayernia, Z., Jaquet, V., and Krause, K.-H. (2014). New insights on NOX enzymes in the central nervous system. Antioxid. Redox Signal. 20, 2815–2837. doi: 10.1089/ars.2013.5703
Noguera, J. C., Morales, J., Pérez, C., and Velando, A. (2010). On the oxidative cost of begging: antioxidants enhance vocalizations in gull chicks. Behav. Ecol. 21, 479–484. doi: 10.1093/beheco/arq005
Nottebohm, F. (2005). The neural basis of birdsong. PLoS Biol. 3:0759–0761. doi: 10.1371/journal.pbio.0030164
Nowicki, S., and Searcy, W. A. (2004). Song function and the evolution of female preferences: why birds sing, why brains matter. Ann. N.Y. Acad. Sci. 1016, 704–723. doi: 10.1196/annals.1298.012
Nowicki, S., Peters, S., and Podos, J. (1998). Song learning, early nutrition and sexual selection in songbirds. Am. Zool. Feb. 38, 179–190. doi: 10.1093/icb/38.1.179
Nowicki, S., and Searcy, W. A. Peters, S. (2002). Brain development, song learning and mate choice in birds: A review and experimental test of the “nutritional stress hypothesis.” J. Comp. Physiol. A Neuroethol. Sens. Neural Behav. Physiol. 188, 1003–1014. doi: 10.1007/s00359-002-0361-3
Oberweger, K., and Goller, F. (2001). The metabolic cost of birdsong production. J. Exp. Biol. 204, 3379–3388.
Pérez-Rodríguez, L. (2009). Carotenoids in evolutionary ecology: re-evaluating the antioxidant role. BioEssays 31, 1116–1126. doi: 10.1002/bies.200900070
Peters, S., Searcy, W. A., and Nowicki, S. (2014). Developmental stress, song-learning, and cognition. Integr. Comp. Biol. 54, 555–567. doi: 10.1093/icb/icu020
Pfaff, J. A., Zanette, L., MacDougall-Shackleton, S. A., and MacDougall-Shackleton, E. A. (2007). Song repertoire size varies with HVC volume and is indicative of male quality in song sparrows (Melospiza melodia). Proc. Biol. Sci. 274, 2035–2040. doi: 10.1098/rspb.2007.0170
Pike, T. W., Blount, J. D., Lindström, J., and Metcalfe, N. B. (2010). Dietary carotenoid availability, sexual signalling and functional fertility in sticklebacks. Biol. Lett. 6, 191–193. doi: 10.1098/rsbl.2009.0815
Pinxten, R., De Ridder, E., Balthazart, J., and Eens, M. (2002). Context-dependent effects of castration and testosterone treatment on song in male European starlings. Horm. Behav. 42, 307–318. doi: 10.1006/hbeh.2002.1824
Polnaszek, T. J., and Stephens, D. W. (2014). Why not lie? Costs enforce honesty in an experimental signalling game. Proc. Biol. Sci. 281, 20132457. doi: 10.1098/rspb.2013.2457
Powers, S. K., and Jackson, M. J. (2008). Exercise-induced oxidative stress : cellular mechanisms and impact on muscle force production. Physiol. Rev. 88, 1243–1276. doi: 10.1152/physrev.00031.2007
Reid, M., Shoji, T., Moody, M., and Entman, M. (1992). Reactive oxygen in skeletal muscle. II. Extracellular release of free radicals. J Appl Physiol 73, 1805–1809.
Royle, N. J., Hartley, I. R., and Parker, G. A. (2002). Begging for control: when are offspring solicitation behaviours honest? Trends Ecol. Evol. 17, 434–440. doi: 10.1016/S0169-5347(02)02565-X
Royle, N. J., Orledge, J. M., and Blount, J. D. (2015). “Life-history effects, oxidative stress, and the evolution and expression of animal aignals,” in Animal Signaling and Function, eds J. I. Duncan, M. Briffa, and J. Podos (Hoboken, NJ: John Wiley & Sons, Inc.), 11–46.
Runge-Morris, M., Frank, P., and Novak, R. F. (1989). Differential effects of organic hydroperoxides and hydrogen peroxide on proteolysis in human erythrocytes. Chem. Res. Toxicol. 2, 76–83.
Saino, N., Ninni, P., Incagli, M., Calza, S., Sacchi, R., and Møller, A. P. (2000). Begging and parental care in realtion to offspring need and condition in the barn swallow (Hirundo rustica). Am. Nat. 156, 637–649.
Salganik, R. I. (2001). The benefits and hazards of antioxidants: controlling apoptosis and other protective mechanisms in cancer patients and the human population. J. Am. Coll. Nutr. 20, 464S–472S. discussion: 473S–475S. doi: 10.1080/07315724.2001.10719185
Salin, K., Auer, S. K., Rudolf, A. M., Anderson, G. J., Cairns, A. G., Mullen, W., et al. (2015). Individuals with higher metabolic rates have lower levels of reactive oxygen species in vivo. Biol. Lett. 11, 20150538. doi: 10.1098/rsbl.2015.0538
Schmidt, K. L., MacDougall-Shackleton, E., Kubli, S. P., and MacDougall-Shackleton, S. (2014). Developmental stress, condition, and birdsong: a case study in song sparrows. Integr. Comp. Biol. 54, 1–10. doi: 10.1093/icb/icu090
Selman, C., Blount, J. D., Nussey, D. H., and Speakman, J. R. (2012). Oxidative damage, ageing, and life-history evolution: where now? Trends Ecol. Evol. 27, 570–577. doi: 10.1016/j.tree.2012.06.006
Sewall, K. B., Soha, J. A., Peters, S., and Nowicki, S. (2013). Potential trade-off between vocal ornamentation and spatial ability in a songbird. Biol. Lett. 9, 16–18. doi: 10.1098/rsbl.2013.0344
Simons, M. J. P., Cohen, A. A., and Verhulst, S. (2012). What does carotenoid-dependent coloration tell ? Plasma carotenoid level signals immunocompetence and oxidative stress state in birds – A Meta-Analysis. PLoS ONE 7:e43088. doi: 10.1371/journal.pone.0043088
Skoumalova, A., and Hort, J. (2012). Blood markers of oxidative stress in Alzheimer's disease. J. Cell. Mol. Med. 16, 2291–2300. doi: 10.1111/j.1582-4934.2012.01585.x
Solaini, G., Baracca, A., Lenaz, G., and Sgarbi, G. (2010). Hypoxia and mitochondrial oxidative metabolism. Biochim. Biophys. Acta 1797, 1171–1177. doi: 10.1016/j.bbabio.2010.02.011
Sorce, S., and Krause, K.-H. (2009). NOX enzymes in the central nervous system: from signaling to disease. Antioxid. Redox Signal. 11, 2481–2504. doi: 10.1089/ARS.2009.2578
Speakman, J. R., Blount, J. D., Bronikowski, A. M., Buffenstein, R., Isaksson, C., Kirkwood, T. B. L., et al. (2015). Oxidative stress and life histories: unresolved issues and current needs. Evol. Ecol. 5, 5745–5757. doi: 10.1002/ece3.1790
Spencer, K. A., Buchanan, K. L., Goldsmith, A. R., and Catchpole, C. K. (2004). Developmental stress, social rank and song complexity in the European starling (Sturnus vulgaris). Proc. Biol. Sci. 271(Suppl. 3), S121–S123. doi: 10.1098/rsbl.2003.0122
Svensson, P. A., and Wong, B. B. M. (2011). Carotenoid-based signals in behavioural ecology: a review. Behaviour 148, 131–189. doi: 10.1163/000579510X548673
Trotta, R. S., Sullivan, S. G., and Stern, A. (1981). Lipid peroxidation and hemoglobin degradation in red blood cells exposed to t-butyl hydroperoxide. Biochem. Biophys. Acta 679, 230–237.
Uttara, B., Singh, A. V., Zamboni, P., and Mahajan, R. T. (2009). Oxidative stress and neurodegenerative diseases: a review of upstream and downstream antioxidant therapeutic options. Curr. Neuropharmacol. 7, 65–74. doi: 10.2174/157015909787602823
Van Hout, A. J. M., Eens, M., and Pinxten, R. (2011). Carotenoid supplementation positively affects the expression of a non-visual sexual signal. PLoS ONE 6:e16326. doi: 10.1371/journal.pone.0016326
Van Noordwijk, A. J., and de Jong, G. (1986). Acquisition and allocation of resources: their influence on variation in life history tactics. Am. Nat. 128, 137–142.
Vinkler, M., and Albrecht, T. (2010). Carotenoid maintenance handicap and the physiology of carotenoid-based signalisation of health. Naturwissenschaften 97, 19–28. doi: 10.1007/s00114-009-0595-9
von Schantz, T., Bensch, S., Hasselquist, D., and Wittzell, H. (1999). Good genes, oxidative stress and condition-dependent sexual signals. Proc. R. Soc. B Biol. Sci. 266, 1–12.
Ward, S. (2004). Singing is not energetically demanding for pied flycatchers, Ficedula hypoleuca. Behav. Ecol. 15, 477–484. doi: 10.1093/beheco/arh038
Wiersma, P., Selman, C., Speakman, J. R., and Verhulst, S. (2004). Birds sacrifice oxidative protection for reproduction. Proc. Biol. Sci. 271(Suppl.), S360–S363. doi: 10.1098/rsbl.2004.0171
Zahavi, A. (1975). Mate selection-a selection for a handicap. J. Theor. Biol. 53, 205–214. doi: 10.1016/0022-5193(75)90111-3
Zavodnik, I. B., Dremza, I. K., Cheshchevik, V. T., Lapshina, E. A., and Zamaraewa, M. (2013). Oxidative damage of rat liver mitochondria during exposure to t-butyl hydroperoxide. Role of Ca2 + ions in oxidative processes. Life Sci. 92, 1110–1117. doi: 10.1016/j.lfs.2013.04.009
Zhang, L., Cooney, R. V., and Bertram, J. S. (1992). Carotenoids up-regulate connexin43 gene expression independent of their provitamin A or antioxidant properties. Cancer Res. 52, 5707–5712.
Keywords: birdsong, calls, honest signaling, redox system, condition trait, oxidative damage, antioxidants
Citation: Casagrande S, Pinxten R and Eens M (2016) Honest Signaling and Oxidative Stress: The Special Case of Avian Acoustic Communication. Front. Ecol. Evol. 4:52. doi: 10.3389/fevo.2016.00052
Received: 04 February 2016; Accepted: 26 April 2016;
Published: 11 May 2016.
Edited by:
Fabrice Helfenstein, University of Neuchâtel, SwitzerlandCopyright © 2016 Casagrande, Pinxten and Eens. This is an open-access article distributed under the terms of the Creative Commons Attribution License (CC BY). The use, distribution or reproduction in other forums is permitted, provided the original author(s) or licensor are credited and that the original publication in this journal is cited, in accordance with accepted academic practice. No use, distribution or reproduction is permitted which does not comply with these terms.
*Correspondence: Stefania Casagrande, c2Nhc2FncmFuZGVAb3JuLm1wZy5kZQ==