Re-evaluating the environment in developmental evolution
- Department for Evolutionary Biology, Max-Planck-Institute for Developmental Biology, Tübingen, Germany
Integrative research at the interphase between ecology, developmental, and evolutionary biology increasingly highlights the importance of phenotypic plasticity, the property of a single genotype to produce different phenotypes depending on environmental conditions. Plasticity occurs in multiple forms at the morphological, physiological, and behavioral levels. It can be reversible or irreversible, continuous or discrete, the latter also known as “polyphenism”. While plasticity has long been discussed as a concept of both, ecological and evolutionary significance, only recent experimental studies have begun providing insights into the associated molecular mechanisms. One promising system for genetic and molecular analyses of phenotypic plasticity is a feeding polyphenism in the nematode model organism Pristionchus pacificus. In this species, genetically identical nematodes can express two alternative mouth-forms, which are advantageous under different environmental conditions. Although the expression of these mouth-forms can be influenced by environment, even under fixed environmental conditions, genetically identical individuals of P. pacificus form both morphs. Thus, in addition to conditional regulation, mouth dimorphism in P. pacificus is regulated stochastically. Here, we discuss the importance of the stochastic regulation of the switch between alternative phenotypes and show that this characteristic provides a unique advantage for genetic, molecular, and experimental analyses. We then relate this stochasticity in mouth-form regulation to a similar phenomenon seen in bacteria, bistability, and finally discuss stochasticity as a bet-hedging mechanism for living in unpredictable environments.
Introduction
The notion of phenotypic plasticity (the ability of one genotype to produce different phenotypes) has a long history in evolutionary thought (Nicoglou, 2015). In the last two decades especially, there has been a growing interest in plasticity as an important factor in evolution contributing to rapid adaptive change and having the potential to explain two mysteries in evolutionary theory: the evolution of novelty and large-scale evolutionary transitions (Schlichting and Pigliucci, 1998; Pigliucci, 2001; West-Eberhard, 2003). Does phenotypic plasticity direct evolutionary processes? Or does it hold evolution back? This remains a matter of debate. It is clear, however, that empirical studies are needed to test the mechanisms predicted by different evolutionary theories. The greatest advances in this attempt to understand evolution can be achieved by focusing on systems that permit the integration of mechanistic (molecular) studies with microevolutionary, macroevolutionary, and ecological observations. Here, we discuss one promising model system for looking at different aspects of plasticity and its role in evolution. The feeding dimorphism in the nematode Pristionchus pacificus not only provides ecological and historical contexts for studying the role of plasticity in evolution but also allows unprecedented insights into the molecular mechanisms underlying the regulation of plasticity. We show that one unusual characteristic of this dimorphism, its stochastic regulation, which can be further influenced by environmental conditions, is a major asset for genetic, molecular, and experimental studies. We place the stochasticity in regulation of developmental switches into a broader context of plasticity and discuss its widespread occurrence in bacteria and importance as a bet-hedging strategy.
P. pacificus and its Mouth-Form Dimorphism
The nematode P. pacificus shares many of its features with C. elegans, and it is a useful model system in comparative evolutionary biology (Sommer et al., 1996; Sommer, 2015). An array of genetic tools available for P. pacificus as well as the nematode's small size, short generation time, self-fertilizing mode of reproduction, and easy culturing on E. coli bacteria make this organism ideal for functional investigations. Two traits found in P. pacificus, the mouth dimorphism and predatory feeding, have gained special attention in recent years (Hartenstein and Jacobs, 2013; Nijhout, 2015).
P. pacificus, other Pristionchus nematodes, and many other species of the family Diplogastridae, to which Pristionchus belongs, express two alternative mouth phenotypes: “narrow-mouthed” stenostomatous (St) and “wide-mouthed” eurystomatous (Eu) mouth-forms (Ragsdale, 2015). Both forms differ in size, shape and the number of teeth, and in the sclerotization of mouth structures (Figures 1A,B). Specifically, Eu animals of P. pacificus have a broad mouth, a claw-like dorsal tooth, and a large subventral tooth (Figure 1B). In contrast, St animals have a narrow mouth, a small flint-like dorsal tooth, and lack the subventral tooth (Figure 1A). In all species with mouth dimorphism, mouth-forms are discrete with no intermediate forms observed (Susoy et al., 2015).
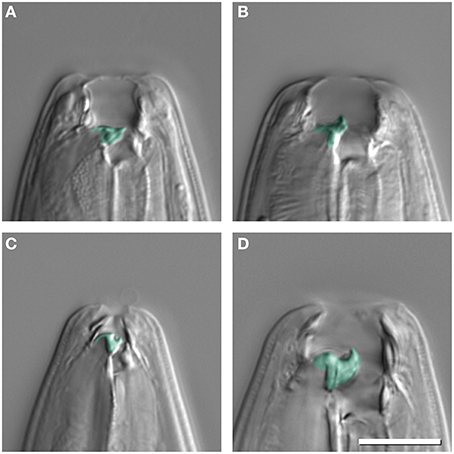
Figure 1. Mouth dimorphism of Pristionchus pacificus (A,B) and Micoletzky japonica (C,D). Stenostomatous animals (St) (A,C) have a narrow buccal cavity and in the case of P. pacificus only a dorsal tooth, whereas eurystomatous animals (Eu) (B,D) have a dorsal and subventral tooth. Discrete mouth-form differences are false colored. Scale bar 10 μm.
This intraspecific phenotypic variation in mouth-forms could in theory be based on either discrete phenotypic plasticity or genetic polymorphisms. Self-fertilization of P. pacificus hermaphrodites, which ultimately results in isogenic clones, makes the contribution of genetic polymorphisms unlikely. Furthermore, in additional selection-line experiments, propagating single Eu or St animals for 10 generations did not result in the fixation of a single phenotype. Even after 10 generations of such selection, both Eu and St animals produced progeny of both mouth-forms with a ratio similar to that observed in the starting population (Bento et al., 2010). All this evidence indicates that mouth dimorphism in P. pacificus is an example of discrete plasticity and not of genetic polymorphism.
Facultatively expressed phenotypic alternatives are often assumed to have adaptive advantages (Pigliucci, 2001). In P. pacificus, experimental studies showed striking differences between the two forms with respect to behavior and resource use (Serobyan et al., 2013, 2014; Wilecki et al., 2015). While Eu P. pacificus adults can predate on nematodes of other species exploiting them as a food source, St individuals show no predatory behavior. However, the St form is advantageous when microbial food is abundant. Provided with plenty of bacteria as food, St individuals complete their development faster than Eu individuals, an observation that can best be explained by St animals demanding fewer resources. Thus, both Eu and St phenotypes confer an adaptive advantage, but under different environmental conditions. When bacterial food is abundant, expression of the St mouth-form allows faster development, whereas when microbial food is exhausted, expression of the Eu form enables alternative foods to be exploited.
Based on these original studies, the last 5 years have brought major insights into the molecular mechanisms underlying the mouth-form decision in P. pacificus. In short, an endocrine signaling module consisting of the nuclear-hormone-receptor DAF-12 and steroid hormones related to dafachronic acid (Bento et al., 2010), the Ser/Thr kinase egl-4 (Kroetz et al., 2012), and the sulfatase eud-1 (Ragsdale et al., 2013) were shown to be involved in the regulation of P. pacificus mouth-form expression. Also, it was shown that the mouth-form decision happens during larval development and it is irreversible (Bento et al., 2010). So, how do individual nematode larvae decide which phenotype to express?
Conditional Mouth-Form Regulation in P. pacificus
Induction of the two mouth phenotypes in P. pacificus is influenced by environmental factors. Under standard laboratory conditions (i.e., nematodes cultured using the E. coli strain OP50; no overcrowding or food deprivation are allowed), wild isolates of P. pacificus consist of 10–100% St individuals (Ragsdale et al., 2013). For example, the wild type reference strain PS312 originally isolated in Pasadena (CA, USA) has 70–90% of Eu and 10–30% of St individuals. Many other wild isolates show an abundance of Eu animals as well when fed with OP50 bacteria. In contrast, some wild isolates from La Réunion Island are largely St under these growth conditions with less than 20% of animals expressing the Eu form (Ragsdale et al., 2013).
Focusing on the reference strain PS312, it was shown that under starved conditions the mouth-form ratio shifts further toward Eu animals (Bento et al., 2010). In addition, population density has an impact on mouth phenotype. Isolated individuals produce progeny that is more likely to express the St phenotype, whereas crowded conditions result in a high fraction of Eu individuals (Serobyan et al., 2013). This effect is attributed to pheromones, a blend of small molecules secreted by nematodes into their environment (Bento et al., 2010). A 2D NMR-spectroscopic screen of the P. pacificus exometabolome identified several of these small molecules that influence the mouth-form decision (Bose et al., 2012). Specifically, ascaroside derivatives dasc#1, pasc#9, nucleoside derivative npar#1, and ascaroside ascr#1 strongly induce expression of the Eu form. For a detailed account on the current knowledge of the molecular aspects of P. pacificus mouth-form regulation, we refer the interested reader to recent reviews (Sommer and Ogawa, 2011; Ragsdale, 2015).
Stochastic Mouth-Form Regulation
The experiments summarized above clearly indicate that the expression of the alternative mouth-forms is at least partly condition-dependent, with environmental factors, such as starvation, population density, and diet playing important roles in the regulation of the mouth-form switch. In addition, it might well be that additional unknown factors and conditions direct the development of this polyphenism even under standardized laboratory conditions (Ragsdale et al., 2013). Nevertheless, even in a common environment, strains of P. pacificus show high levels of intragenotypic variability. When cultured on agar plates with E. coli bacteria as food and all abiotic conditions being constant, genetically identical individuals of P. pacificus PS312 still express both Eu and St mouth-forms (Ragsdale et al., 2013; Serobyan et al., 2013). While the exact ratio differs in other isogenic strains of P. pacificus, they also form both morphs when cultured under these standard laboratory conditions. This observation suggests that stochasticity also plays a role in the developmental regulation of the mouth-form decision. Stochasticity and phenotypic heterogeneity in invariable environments are of great interest in contemporary biology with important case studies in developmental biology and neurobiology (Olivia Casanueva et al., 2012; Ayroles et al., 2015; Gordus et al., 2015).
In the following, we discuss four aspects associated with stochastic regulation of plasticity in a broader, evolutionary context. First, we show that in the nematode family Diplogastridae, mouth-form expression is usually strongly induced by the environment; considering the phylogenetic context, phenotypic heterogeneity in stable environments, as seen in P. pacificus, represents an evolutionary derived state. Second, we show the experimental advantage of stochasticity for genetic and mechanistic studies of developmental plasticity in P. pacificus. Third, we relate stochasticity in expression of discrete mouth phenotypes to related phenomena in bacteria. And finally, we discuss the potential importance of the stochastic regulation as a bet-hedging strategy for organisms living in unpredictable environments.
The Evolutionary Context: Stochastic vs. Conditional Dimorphism?
The systematics and phylogeny of the family Diplogastridae have recently been reviewed (Kanzaki and Giblin-Davis, 2015). Currently, 37 genera are recognized, 28 of which have been used for a detailed molecular phylogenetic analysis (Susoy et al., 2015). Susoy and co-workers combined their molecular phylogenetic study, which was based on 14 genes that had more than 6300 parsimony-informative sites with a detailed analysis of mouth morphology (Figure 2). Of 54 species of Diplogastridae included in this study, 23 were dimorphic and 31 were monomorphic. In contrast to P. pacificus and many other members of the genus Pristionchus, the majority of dimorphic diplogastrids species occur exclusively in the St morph when fed on a bacterial diet (Susoy et al., 2015). The expression of the Eu form can be induced experimentally by starvation or a switch to a prey-diet. In most experiments, more than 90% of the treated animals expressed the Eu mouth-form. For example, the bark beetle associated nematode Micoletzkya japonica formed 0% Eu animals when fed on E. coli bacteria, and 92% Eu animals on a C. elegans larval diet (Figures 1C,D; Table 1; Susoy et al., 2015). These findings result in two major conclusions. First, the majority of dimorphic diplogastrids show inducible plasticity similar to what is known from many other animal and plant systems. Second, the phenotypic heterogeneity under stable conditions observed in P. pacificus and other species of Pristionchus is unique to this genus and, based on the phylogeny of Diplogastridae, it can be considered to represent a secondary evolutionary innovation. Finally, it should be noted that the 31 species of monomorphic Diplogastridae analyzed in the study mentioned above (Susoy et al., 2015) were considered to be monomorphic as they could not be induced to form an alternative mouth-form in any tested condition. However, it may be possible that some of these species can be induced to generate an alternative mouth-form by yet unknown environmental conditions. Taken together, the phylogenetic comparison of the environmental regulation of mouth dimorphisms in Diplogastridae indicates that the phenotypic heterogeneity observed in Pristionchus represents a derived state specific for this genus. We will next discuss the unique experimental advantages of this stochasticity for looking at mechanisms of mouth-form regulation.
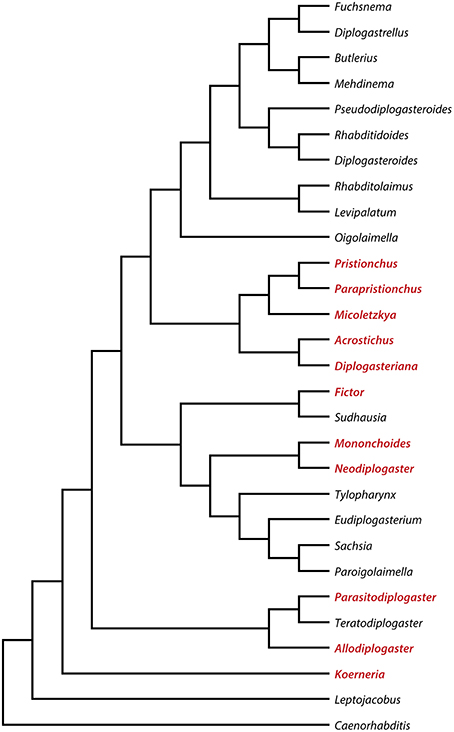
Figure 2. Molecular phylogeny of selected diplogastrid genera based on 14 genes. Genera that have species with mouth dimorphism are highlighted in red. For a full phylogeny and methodology see Susoy et al. (2015).
Stochasticity and Experimental Manipulation
Experimental analysis profits from laboratory settings in which environmental conditions can be kept constant. This results in inherent limitations for the functional investigation of the majority of cases of phenotypic plasticity. If environmental changes induce plasticity, laboratory conditions must also mimic these environmental changes if the underlying molecular mechanisms are to be investigated. However, one can overcome these limitations using the stochastic regulation of the mouth dimorphism in P. pacificus as a model system. Several experiments in the past showed the power of this approach. For example, to identify genetic players involved in mouth-form regulation, wild type P. pacificus can be mutagenized, and its progeny can be analyzed for mutations that alter the wild type mouth-form ratio. Using this logic, Ragsdale and co-workers have identified a switch gene that plays a key role in mouth-form regulation. Mutations in the sulfatase-encoding gene eud-1 resulted in the absence of Eu animals, shifting the complete culture to the St mouth-form (Table 2; Ragsdale et al., 2013). Similarly, transgenic experiments were performed and transgenic lines carrying extrachromosomal copies of eud-1 were shown to have the opposite effect resulting in 100% Eu strains (Table 2; Ragsdale et al., 2013). This simple regime is currently expanded by reverse genetic engineering applying the recently adopted CRISPR/Cas9 technology in P. pacificus (Witte et al., 2015). Thus, phenotypic heterogeneity allows the identification of genetic regulators of plasticity using forward and reverse genetics tools without the need to interfere with complex environmental conditions in laboratory experiments. Therefore, while stochasticity as observed in P. pacificus mouth-form regulation is seemingly rare, it represents unique advantages for experimentation. But is stochasticity so rare in nature? Expanding the discussion to the microbial world tells us a different story, which we briefly summarize below.
Bistability in Bacteria
While the majority of examples of plasticity indicate conditional regulation, some examples of stochastic regulation are known from plants and animals, such as sex ratio of offspring in Drosophila (Cline, 1993) and heterophylly in tropical plants of the genus Cyanea (Givnish et al., 1994), but controversial discussion about such examples are still ongoing (West-Eberhard, 2003, pp. 424–426). In contrast, stochasticity in the expression of phenotypes has long been noticed in microbes, and it has been the subject of study in both applied and basic microbial research. Microbiologists studying the influence of rapidly changing environmental conditions on bacteria have described a plethora of adaptive responses, some of which involve population heterogeneity. Dubnau and Losick (2006) suggested the term “bistability” for phenotypic heterogeneity that results in phenotypically distinct subpopulations of cells, and other authors followed this terminology (Dubnau and Losick, 2006; Smits et al., 2006; de Jong et al., 2011). The first example of bistability was described by Bigger in 1944. He found that when cultures of Staphylococcus aureus were treated with antibiotics, most bacterial cells died, but there were always some cells that survived (Bigger, 1944). These surviving (persister) cells were not mutated; cultures established from the persister cells did not show increased antibiotic resistance. Instead, these cultures had the usual sensitivity to the antibiotic, each time forming a few surviving persister cells. It was later shown that persister cells result from stochastic changes in gene expression, which leads to very slow or no growth of some cells, thereby eliminating the effect of the antibiotic (Balaban et al., 2004). Other examples of bistability are known from Bacillus subtilis, including spore formation and cannibalism, swimming and chaining, and genetic competence to take up foreign DNA (Dubnau and Losick, 2006). Many of these phenomena have been studied in great detail and supported by molecular and mechanistic insights.
Adaptive Stochastic Regulation
What are the conditions that could favor the origin of stochastic regulation of initially condition-dependent discrete plasticity? For bistability in bacteria, one important consideration is that most natural environments change over time with different degrees of predictability. Even if an environmental change is predictable by means of reliable cues, significant and sometimes variable lag times might exist. Therefore, randomness as observed in microbial bistability is considered to represent a bet-hedging strategy, a risk spreading strategy that can be displayed by isogenic populations in unpredictably changing environments (Veening et al., 2008). Indeed, microorganisms are usually subject to environmental changes that are not only frequent but also unpredictable. It is important to note that not all cases of phenotypic heterogeneity can be considered a bet-hedging strategy; a recent commentary provides an overview on the need for a proper definition of the term (de Jong et al., 2011).
In multicellular organisms with irreversible developmental decisions, similar reasons can explain the evolution of the stochastic regulation of plasticity. First, cues indicating environmental change might not be reliable. Second, environmental changes may occur over short timescales relative to the lifespan of individuals. Specifically, if the developmental lag time is larger than the time period over which the environmental change occurs, frequent changes in the environment can lower or even eliminate fitness benefits of plasticity. Multiple authors have argued for stochastic regulation as a mechanism that enables risk-spreading strategies under such conditions (Kaplan and Cooper, 1984; Clauss and Venable, 2000; Menu et al., 2000). Pigliucci (2001) called this type of plasticity “non-anticipatory” and highlighted the fact that such non-anticipatory plasticity can increase fitness, dependent on the exact circumstances.
We argue that the stochastic regulation of plasticity observed in the expression of Pristionchus mouth phenotypes represents an example of non-anticipatory plasticity. Indeed, habitats in which Pristionchus is commonly found are characterized by unpredictable changes. First, Pristionchus occurs in soil, and the patchy distribution of microbes in soil and their influence on nematode distribution has been discussed in great detail (Ragsdale, 2015). Second, Pristionchus nematodes often have a necromenic association with scarab beetles (Herrmann et al., 2006, 2007). Dispersal (dauer) juveniles of Pristionchus can attach themselves to beetle bodies, resuming their development only after the beetle's death, when bacteria and other microbes start proliferating on the beetle's carcass (Sommer and Mayer, 2015). Indeed, preliminary experimental studies on the decay of beetle carcasses in soil indicate that the decay period can be fast, and it is highly variable between carcasses. Most importantly, the nematode life cycle is probably too slow in relation to the environmental fluctuations to allow effective anticipation of the future environment.
In contrast to the facultative Pristionchus-beetle association, some other diplogastrid-insect associations represent examples of obligate symbioses. In such cases, nematodes often live in microhabitats constructed by their insect hosts, and the nematodes' life cycle is tightly linked to that of the insects. For example, species of the diplogastrid genus Micoletzkya have stable and highly specific associations with bark beetles. They are vertically transmitted within populations of their hosts from parents to offspring, and they reproduce in the breeding galleries of bark beetles, feeding on bark-beetle-associated microbes and other nematodes (Susoy and Herrmann, 2014). Although such microecosystems also change over time, the changes that occur in these systems are probably highly predictable because they happen in cycles that coincide with the nematode host life cycle. Furthermore, such microhabitats are sheltered from fluctuations of abiotic factors, and are usually inhabited by stable ecological communities consisting of relatively few coevolving organisms. All this could allow reliable anticipation of the environmental change, decreasing the need to bet-hedge. Consistently with this line of thought, mouth-form plasticity in species of Micoletzkya and some other insect-associated diplogastrids was shown to be readily inducible by environment, with no or little stochastic variation in the mouth-form expression under test conditions (Susoy et al., 2015). Whether or not there is a strong link between symbioses and the conditional expression of phenotypic alternatives, such systems can still provide a valuable ecological context for studies on plasticity. A small number of ecological interactions, compared to free-living nematodes, and a predictable environment, make it possible to grasp most of the environmental complexity of such systems.
A different reason for bet-hedging being so common in Pristionchus, but rare in other dimorphic genera of predatory diplogastrids might be the inability of the St Pristionchus to be predators. In most species of dimorphic diplogastrids, both Eu and St animals show predatory behavior, although the efficiency of predation can be different between different forms. When Wilecki et al. (2015) looked at the predatory behavior across fifteen different species of Pristionchus, they found that in none of them St individuals were able to kill nematode prey. This is probably because St Pristionchus, unlike many other St diplogastrids, lack the subventral tooth or this tooth is very small, which makes predation impossible (Figure 1). When the microbial food becomes depleted, these animals are not able to switch to alternative food sources, such as other nematodes. In these circumstances, it might be beneficial to always maintain a fraction of Eu animals in the population, such that if the conditions suddenly change from favorable to unfavorable for the St form, there are animals that are able to switch to a different diet, decreasing the chance of population collapse and lineage extinction. It should be noted that such a group selection argument, might well apply to hermaphroditic species such as P. pacificus. Future research will reveal if similar patterns are seen in gonochoristic Pristionchus species and current sampling efforts aim at identifying sufficient wild isolates to address such questions.
Finally, it is important to note that stochastic and conditional modes of regulation of the trait expression do not have to be mutually exclusive. The availability of cues that can reliably predict the future environment can favor the evolution of developmental switches, which match the phenotype expression to the anticipated environment. In dimorphisms, the outcomes of developmental processes are binary. It is possible then that near the neutrality point, when the environment does not strongly favor either of the phenotypic alternatives, external and internal noise (which result from stochastic variation in gene expression, environmental fluctuation, etc.) will play roles in determining which of the alternative phenotypes is expressed. If the reliable anticipation of environmental change by means of integration of external cues becomes impossible, and if the cost of expressing a phenotype that does not match the environment is high, as in the case of St Pristionchus, stochastic determination of the phenotype might be favorable because it can serve as a mechanism of bet-hedging. In contrast, when the future environment can be anticipated reliably, conditional control might be preferable. The degree of developmental sensitivity to both external and internal environments is likely to be under the control of complex polygenic regulatory networks, and thus it can be a subject of selection. The evolution of a stochastic conditional mode of regulation of the switch between phenotypic alternatives thus can enable the randomization of phenotypes when the future environment cannot be predicted while still maintaining the ability to respond to strong environmental signals in an adaptive manner, offering an adaptive solution to living in a fluctuating environment. Current studies are directed at pinpointing the molecular mechanisms of integration of external and internal inputs relevant in the stochastic conditional regulation of binary developmental switches. Also, ongoing studies try to identify how mouth-form ratios are kept stable over generations.
In conclusion, we argue that the mouth-form polyphenism in diplogastrid nematodes is adaptive and evolutionarily stable. In P. pacificus, the St and Eu forms have adaptive values (Serobyan et al., 2013, 2014; Wilecki et al., 2015), and comparative phylogenetic studies on Pristionchus and other diplogastrids show that plasticity can lead to large-scale evolutionary diversifications. A recently identified group of fig and fig-wasp associated species of Pristionchus has expanded mouth-form polyphenism from two to five distinct morphs. This multiplication of the ancestral dimorphism was associated with exaggerated divergence between morphs; some of them showing unusual structures that have no analogs in Pristionchus and other known nematodes (Susoy et al., 2016). Remarkably, this macroevolutionary-scale diversification occurred without genetic divergence, and instead has been based on discrete plasticity. In a separate study, a comprehensive analysis of mouth-forms in 90 nematode species of Diplogastridae and Rhabditidae showed that the mouth-form polyphenism in this group of nematodes evolved only once, but was lost multiple times (Susoy et al., 2015). Looking at evolutionary dynamics associated with the gain and loss of plasticity revealed that phenotypic complexity increased sharply following the origin of plasticity, and then decreased in lineages where plasticity was secondarily lost. The rates of mouth form evolution, however, became even higher after the loss of dimorphism. These studies show the advantage of microscopic nematodes for both genetic and evolutionary analyses.
Author Contributions
All authors listed, have made substantial, direct and intellectual contribution to the work, and approved it for publication.
Funding
This work was funded by the Max-Planck Society.
Conflict of Interest Statement
The authors declare that the research was conducted in the absence of any commercial or financial relationships that could be construed as a potential conflict of interest.
References
Ayroles, J. F., Buchanan, S. M., O'Leary, C., Skutt-Kakaria, K., Grenier, J. K., Clark, A. G., et al. (2015). Behavioral idiosyncrasy reveals genetic control of phenotypic variability. Proc. Natl. Acad. Sci. U.S.A. 112, 6706–6711. doi: 10.1073/pnas.1503830112
Balaban, N. Q., Merrin, J., Chait, R., Kowalik, L., and Leibler, S. (2004). Bacterial persistence as a phenotypic switch. Science 305, 1622–1625. doi: 10.1126/science.1099390
Bento, G., Ogawa, A., and Sommer, R. J. (2010). Co-option of the endocrine signaling module Dafachronic Acid-DAF-12 in nematode evolution. Nature 466, 494–497. doi: 10.1038/nature09164
Bigger, J. W. (1944). Treatment of staphylococcal infections with penicillin by intermittent sterilisation. Lancet 244, 497–499. doi: 10.1016/S0140-6736(00)74210-3
Bose, N., Ogawa, A., von Reuss, S. H., Yim, J. J., Ragsdale, E. J., Sommer, R. J., et al. (2012). Complex small molecular architectures regulate phenotypic plasticity in a nematode. Angew. Chem. Int. Ed. Engl. 51, 12438–12443. doi: 10.1002/anie.201206797
Clauss, M. J., and Venable, D. L. (2000). Seed germination in desert annuals: an empirical test of adaptive bet hedging. Am. Nat. 155, 168–186. doi: 10.1086/303314
Cline, T. W. (1993). The Drosophila sex determination signal: how do flies count to two? Trends Genet. 9, 385–390. doi: 10.1016/0168-9525(93)90138-8
de Jong, I. G., Haccou, P., and Kuipers, O. P. (2011). Bet hedging or not? A guide to proper classification of microbial survival strategies. Bioessays 33, 215–223. doi: 10.1002/bies.201000127
Dubnau, D., and Losick, R. (2006). Bistability in bacteria. Mol. Microbiol. 61, 564–572. doi: 10.1111/j.1365-2958.2006.05249.x
Givnish, T. J., Sytsma, K. J., Smith, J. F., and Hanh, W. J. (1994). Thorn-like prickles and heterophylly in Cyanea: adaptations to extinct avian browsers on Hawaii? Proc. Natl. Acad. Sci. U.S.A. 91, 2810–2814.
Gordus, A., Pokala, N., Levy, S., Flavell, S., and Bargmann, C. I. (2015). Feedback from network states generates variability in a probabilistic olfactory circuit. Cell 161, 215–227. doi: 10.1016/j.cell.2015.02.018
Hartenstein, V., and Jacobs, D. (2013). Developmental plasticity, straight from the worm's mouth. Cell 155, 742–743. doi: 10.1016/j.cell.2013.10.038
Herrmann, M., Mayer, E. W., and Sommer, R. J. (2006). Nematodes of the genus Pristionchus are closely associated with scarab beetles and the Colorado potato beetle in western Europe. Zoology 109, 96–108. doi: 10.1016/j.zool.2006.03.001
Herrmann, M., Mayer, W., Hong, R., Kienle, S., Minasaki, R., and Sommer, R. J. (2007). The nematode Pristionchus pacificus (Nematoda: Diplogastridae) is associated with the Oriental beetle Exomala orientalis (Coleoptera: Scarabaeidae) in Japan. Zool. Sci. 24, 883–889. doi: 10.2108/zsj.24.883
Kanzaki, N., and Giblin-Davis, R. M. (2015). “Diplogastrid systematics and phylogeny,” in Pristionchus pacificus: A Nematode Model for Comparative and Evolutionary Biology, ed R. J. Sommer (Leiden: Brill), 43–76.
Kaplan, R. H., and Cooper, W. S. (1984). The evolution of developmental plasticity in reproductive characteristics: an application of the adaptive coin-flipping principle. Am. Nat. 123, 393–410. doi: 10.1086/284211
Kroetz, S. M., Srinivasan, J., Yaghoobian, J., Sternberg, P. W., and Hong, R. (2012). The cGMP signaling pathway affects feeding behavior in the necromenic nematode Pristionchus pacificus. PLoS ONE 4:e34464. doi: 10.1371/journal.pone.0034464
Menu, F., Roebuck, J.-P., and Viala, M. (2000). Bet-hedging diapause strategies in stochastic environments. Am. Nat. 155, 724–734. doi: 10.1086/303355
Nicoglou, A. (2015). “Phenotypic plasticity: from microevolution to macroevolution,” in Handbook of Evolutionary Thinking in the Sciences, eds T. Heams, P. Huneman, G. Lecointre, and M. Silberstein (Heidelberg: Springer), 285–318.
Olivia Casanueva, M., Burga, A., and Lehner, B. (2012). Fitness trade-offs and environmentally induced mutation buffering in isogenic C. elegans. Science 335, 82–86. doi: 10.1126/science.1213491
Pigliucci, M. (2001). Phenotypic Plasticity: Beyond Nature and Nurture. Baltimore, MD: John Hopkins University Press.
Ragsdale, E., Müller, M., Roedelsperger, C., and Sommer, R. J. (2013). A developmental switch coupled to the evolution of plasticity acts through a sulfatase. Cell 155, 922–933. doi: 10.1016/j.cell.2013.09.054
Ragsdale, E. J. (2015). “Mouth dimorphis and the evolution of novelty and diversity,” in Pristionchus Pacificus. A Nematode Model for Comparative and Evolutionary Biology, ed R. J. Sommer (Leiden: Brill), 301–329.
Schlichting, C., and Pigliucci, M. (1998). Phenotypic Evolution. Sunderland, MA: Sinauer Associates Inc.
Serobyan, V., Ragsdale, E. J., Müller, M. R., and Sommer, R. J. (2013). Feeding plasticity in the nematode Pristionchus pacificus is influenced by gender and social context and is linked to developmental speed. Evol. Dev. 15, 173–182. doi: 10.1111/ede.12030
Serobyan, V., Ragsdale, E. J., and Sommer, R. J. (2014). Adaptive value of a predatory mouth-form in a dimorphic nematode. Proc. Biol. Sci. 281:20141334. doi: 10.1098/rspb.2014.1334
Smits, W. K., Kuipers, O. P., and Veening, J.-W. (2006). Phenotypic variation in bacteria: the role of feedback regulation. Nat. Rev. Microbiol. 4, 259–271. doi: 10.1038/nrmicro1381
Sommer, R. J. (ed.). (2015). Pristionchus Pacificus. A Nematode Model for Comparative and Evolutionary Biology. Leiden: Brill.
Sommer, R. J., Carta, L. K., Kim, S.-Y., and Sternberg, P. W. (1996). Morphological, genetic and molecular description of Pristionchus pacificus sp. n. (Nematoda, Diplogastridae). Fundam. Appl. Nematol. 19, 511–521.
Sommer, R. J., and Mayer, M. G. (2015). Towards a synthesis of developmental biology with evolutionary theory and ecology. Anun. Rev. Cell Dev. Biol. 31, 453–471. doi: 10.1146/annurev-cellbio-102314-112451
Sommer, R. J., and Ogawa, A. (2011). Hormone signaling and phenotypic plasticity in nematode development and evolution. Curr. Biol. 21, R758–R766. doi: 10.1016/j.cub.2011.06.034
Susoy, V., and Herrmann, M. (2014). Preferential host switching and codivergence shaped radiation of bark beetle symbionts, nematodes of Micoletzkya (Nematoda: Diplogastridae). J. Evol. Biol. 27, 889–898. doi: 10.1111/jeb.12367
Susoy, V., Herrmann, M., Kanzaki, N., Kruger, M., Nguyen, C. N., Rödelsperger, C., et al. (2016). Large-scale diversification without genetic isolation in nematode symbionts of figs. Sci. Adv. 2:e1501031. doi: 10.1126/sciadv.1501031
Susoy, V., Ragsdale, E. J., Kanzaki, N., and Sommer, R. J. (2015). Rapid diversification associated with a macroevolutionary pulse of developmental plasticity. Elife 4:e05463. doi: 10.7554/elife.05463
Veening, J.-W., Smits, W. K., and Kuipers, O. P. (2008). Bistability, epigenetics and bet-hedging in bacteria. Annu. Rev. Microbiol. 62, 193–210. doi: 10.1146/annurev.micro.62.081307.163002
West-Eberhard, M. J. (2003). Developmental Plasticity and Evolution. Oxford: Oxford University Press.
Wilecki, M., Lightfoot, J., Susoy, V., and Sommer, R. J. (2015). Predatory feeding behavior in Pristionchus nematodes is dependent on a phenotypic plasticity and induced by serotonin. J. Exp. Biol. 218, 1306–1313. doi: 10.1242/jeb.118620
Keywords: developmental plasticity, Pristionchus pacificus, stochastic regulation, conditional regulation, mouth-form polyhenism
Citation: Susoy V and Sommer RJ (2016) Stochastic and Conditional Regulation of Nematode Mouth-Form Dimorphisms. Front. Ecol. Evol. 4:23. doi: 10.3389/fevo.2016.00023
Received: 28 January 2016; Accepted: 29 February 2016;
Published: 11 March 2016.
Edited by:
Angelika Stollewerk, Queen Mary University of Lodon, UKReviewed by:
John P. Masly, University of Oklahoma, USAPedro Martinez, Universitat de Barcelona, Spain
Copyright © 2016 Susoy and Sommer. This is an open-access article distributed under the terms of the Creative Commons Attribution License (CC BY). The use, distribution or reproduction in other forums is permitted, provided the original author(s) or licensor are credited and that the original publication in this journal is cited, in accordance with accepted academic practice. No use, distribution or reproduction is permitted which does not comply with these terms.
*Correspondence: Ralf J. Sommer, ralf.sommer@tuebingen.mpg.de