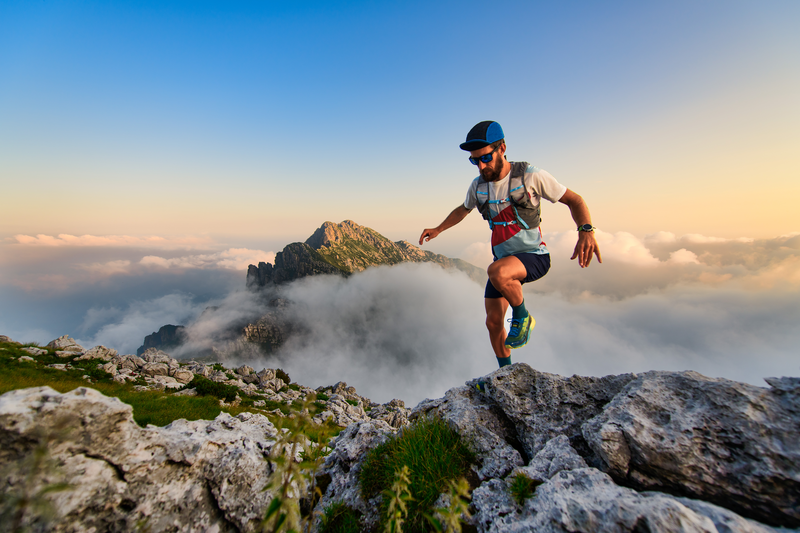
94% of researchers rate our articles as excellent or good
Learn more about the work of our research integrity team to safeguard the quality of each article we publish.
Find out more
REVIEW article
Front. Ecol. Evol. , 12 January 2016
Sec. Conservation and Restoration Ecology
Volume 3 - 2015 | https://doi.org/10.3389/fevo.2015.00155
A commentary has been posted on this article:
Commentary: Linking Movement Ecology with Wildlife Management and Conservation
A common challenge in species conservation and management is how to incorporate species movements into management objectives. There often is a lack of knowledge of where, when, and why species move. The field of movement ecology has grown rapidly in the last decade and is now providing the knowledge needed to incorporate movements of species into management planning. This knowledge can also be used to develop management strategies that are flexible in time and space and may improve the effectiveness of management actions. Therefore, wildlife management and conservation may benefit by strengthening the link with movement ecology. We present a framework that illustrates how animal movement can be used to enhance conservation planning and identify management actions that are complementary to existing strategies. The framework contains five steps that identify (1) the movement attributes of a species, (2) their impacts on ecosystems, (3) how this knowledge can be used to guide the scale and type of management, (4) the implementation, and (5) the evaluation of management actions. We discuss these five steps in detail, highlighting why the step is important and how the information can be obtained. We illustrate the framework through a case study of managing a highly mobile species, the Atlantic salmon (Salmo salar), a harvested species of conservation concern. We believe that the movement-management framework provides an important, and timely, link between movement ecology and wildlife management and conservation, and highlights the potential for complementary, dynamic solutions for managing wildlife.
The field of movement ecology has grown rapidly in the last decade due to a number of recent technological and analytical advances in tracking animal movement (Tomkiewicz et al., 2010). Alongside the growth in technological advances have been advances in conceptual frameworks that aim to unify research in animal movement (Nathan et al., 2008) and incorporate movement into biodiversity research (Jeltsch et al., 2013). This growth has provided a number of benefits for conservation and management, such as improving our understanding of habitats important for wildlife and the area traversed by wide-ranging species (Hebblewhite and Haydon, 2010). However, it has also highlighted a number of challenges for conservation, such as maintaining connectivity, both within the landscape and for species with wide-ranging movements like nomadic or migratory species (Sanderson et al., 2002; Martin et al., 2007; Runge et al., 2014). At the same time, studies have revealed that traditional approaches to conservation, such as protected areas, may be inadequate due to reasons like the spatial scale of a species movements (e.g., Thirgood et al., 2004), conflicts with stakeholders (e.g., Symes et al., 2015), or available finances (e.g., Carwardine et al., 2008; Chadés et al., 2015). Traditional approaches will continue to have a vital role in conservation planning, however their effectiveness may be improved if they are combined with strategies that are flexible in time and/or space (Runge et al., 2014; Chadés et al., 2015; Tulloch et al., 2015).
Research in movement ecology is generating knowledge of species movements that enables managers to implement actions that are flexible in space and time. As a result, managers have begun to link the movement ecology of a species with management planning, resulting in targeted management actions that incorporate species movements or specific areas where threats are located (Table 1). An example is how the results of a tracking study for leatherback turtles (Dermochelys coriacea) were used to identify new potential conservation strategies to reduce leatherback-fisheries interactions that included targeted spatial actions and dynamic time-area closures along the migration corridor (Table 1; Shillinger et al., 2008). Knowledge of species movements is also being used to prioritize management actions and achieve maximum benefit from the limited funds available (Martin et al., 2007; Carwardine et al., 2008, 2012). An example is focusing management actions on bottleneck sites, which are particular areas that species rely upon like stopover sites (Iwamura et al., 2014), or where landscape connectivity is being constrained by physical barriers (Table 1; Sawyer et al., 2013; Seidler et al., 2015). These studies highlight the potential for linking management planning with movement ecology. However, the examples are few and greater emphasis is needed to link the fields of movement ecology and conservation if we are to improve upon the existing model.
We formulated a conceptual Movement-management framework (hereon described as framework) to illustrate how knowledge of animal movement may enhance management planning. The workflow described in the framework is applicable across taxa, as many species exhibit movements that vary from being sedentary year round, to migration, nomadism, and dispersal (Table 1). Furthermore, these movements share common attributes across taxa, such as the use of pathways, stopover sites, seasonal ranges, or breeding sites. We outline the steps used in the framework, their rationale and highlight some aspects that require important considerations. We discuss its implementation, how it links to existing practices and identify potential challenges for its implementation. Through a case study of the Atlantic salmon (Salmo salar), we show how knowledge of movement has been used and can be used further to guide management planning.
The framework is organized into five interlinked steps, whereby baseline information on species movements are used to guide management decisions (Figure 1). The first three steps include understanding species' movements, their ecosystem impacts and how these are linked to the scale of management required (Figure 1). A fourth step considers the implementation of management actions whilst the final step incorporates an adaptive management component of evaluation (Figure 1).
Figure 1. Movement-management framework. Movement-Management framework that provides a workflow for incorporating movement ecology into decision-making processes. Before applying the framework, one must consider the quality of the movement data and whether it is appropriate for achieving the management goal. This includes questions like resolution, sample size, and the type of methods that will be used. Data may also be available from alternative sources, such as expert opinion or presence data, which can be combined with predictive modeling. Once appropriate movement data is available, the first step of the framework concerns understanding the movement attributes occurring in a study population or system. This includes the movement types that exist in a population that include “migration,” “dispersal,” “sedentary,” and “nomadism.” It is important to understand the characteristics of these movements, for example, movement pathways, home ranging patterns, or the timing of movements. The second step is to determine the ecosystem impacts and services resulting from movements. The knowledge gained from the first two steps guides the decision making process and identifies potential management actions that complement existing management plans. These may include actions that are flexible in space and time, such as time-area closures, which require detailed knowledge of species movements. The fourth step considers the implementation of the proposed actions, including considerations like available knowledge, cost, stakeholder interests and how these influence the effectiveness and feasibility of proposed actions. The final step evaluates the effectiveness of management actions, thereby creating an adaptive management cyclical process whereby the outcomes of the evaluation guide management objectives and future actions.
The primary requirement for the framework is the availability of animal movement data that is appropriate for the management objective(s). The movement data required will depend upon the ecological or conservation questions underlying the management objectives, and may involve considerations like whether the time period of observation is long enough to make general conclusions on movement patterns, or whether the sample size is large enough to make population level inferences (Figure 1). In addition, quantitative methods for analysing movements have progressed rapidly with time (Patterson et al., 2008; Kranstauber et al., 2012; Fleming et al., 2015). These methods have specific requirements regarding the quality of data needed, as few as 10 locations per month may be sufficient to estimate a home range but this would not be sufficient for understanding resource use (Marzluff et al., 2004; Börger et al., 2006). However, it should be noted that detailed movement data is not always available. Instead, informed decisions can be made based on predictive modeling that are performed in conjunction with alternative sources of data, such as expert opinion or presence data (Low Choy et al., 2009; Iwamura et al., 2014). These data sources may guide initial decision making processes whilst more detailed data are acquired (Grantham et al., 2009), and may also benefit study design by identifying existing knowledge gaps. Collecting new movement data may be limited by the study species, available time, or money. Nevertheless, the improved knowledge that movement data provides may lead to more effective management actions as opposed to costly mistakes (Carwardine et al., 2008; Grantham et al., 2009).
The target for managers is to develop an understanding of how individual movements affect a species survival and reproduction and therefore population dynamics. An individual's decision to move is influenced by several factors that include food resource availability and/or quality, predator avoidance and environmental conditions, which will enhance its capacity to survive and reproduce (Morales et al., 2010; van Moorter et al., 2013). Movement attributes, like the timing of spring migration, may have direct effects on the fitness of individuals (Winkler et al., 2014). A number of bird species have not advanced the timing of their spring migrations in response to climate change, and appear to be declining because the timing of breeding has become mismatched with peak food availability (Møller et al., 2008). Furthermore, the performance of a population may also be influenced by the ability of individuals to adapt their movements to environmental change, such as adapting foraging movements to habitat loss (McNamara et al., 2011; Winkler et al., 2014).
Applying the first step of the framework allows managers to identify how animal movements influence demography and subsequent population dynamics (Figure 1). Animal movement can be described according to three major population-level distribution strategies that include being sedentary in annual ranges, migration and nomadism (Mueller and Fagan, 2008). Being sedentary on an annual scale involves having stable home ranges or territories, where an individual occupies a relatively small area compared to the population distribution (Mueller and Fagan, 2008). Migration consists of seasonal, round-trip movements between spatially disjunct areas (Mueller and Fagan, 2008; Harris et al., 2009). Nomadism differs from being sedentary or migratory as individuals move across the landscape using routes that do not repeat across years (Mueller and Fagan, 2008). A fourth movement type that managers need to consider is dispersal. Dispersal is the movement to a site of reproduction and includes movements away from the site of birth (natal dispersal) and movements between successive reproductive sites (breeding dispersal; Matthysen, 2012). The types of movements present in the population will influence the type of management actions needed, such as preserving connectivity for dispersing and nomadic movements, setting aside reserves for species which are sedentary in their annual home ranges or adopting a flyway approach for conserving migratory species (Klaassen et al., 2008; Howard and Davis, 2009; Minor and Lookingbill, 2010; Hodgson et al., 2011). Previous research has shown that management interventions have been less effective when management actions have not matched the spatial, or temporal, scale of species movements (Thirgood et al., 2004; Moffitt et al., 2009).
In addition to the types of movement present in the population, it is important to understand the characteristics of movements. These comprise of movement pathways, distance and timing of movements, shapes and sizes of home ranges, habitat selection along movement paths and the use of stopover sites by migratory species (Figure 1). Understanding the type of movement in the population determines the types of management actions needed, but understanding the characteristics of movements is necessary for planning, designing, and implementing management actions. For example, understanding movement pathways is particularly important when managers aim to maintain connectivity, particularly as the loss of some sites can lead to sudden population decline (Webster et al., 2002; Iwamura et al., 2013). Movement pathways also indicate whether species use matrix habitats (Fischer et al., 2005), are restricted to specific habitat types (Hagen et al., 2012), or barriers prevent movements (Sawyer et al., 2013). Other movement characteristics, such as the size and shape of home ranges, are often used to guide the scale of management (Schwartz, 1999) and to determine habitat preference and subsequent habitat suitability (Chetkiewicz and Boyce, 2009; Lu et al., 2012). Knowledge of movement characteristics may also identify potential threats, such as the increased risk of exploitation due to the predictable and aggregating nature of some migratory species (Bolger et al., 2007; Harris et al., 2009). We expand upon how knowledge of the types and characteristics of species movements can be used to guide the scale of management in Section Scale of Management.
Animal movement is a core component of an ecosystem and maintaining movement patterns may be vital for sustaining ecosystem processes like trophic and species interactions (Lundberg and Moberg, 2003; Massol et al., 2011). Movement provides links between ecosystems and these links may be classified as either resource, genetic or process links (reviewed in Jeltsch et al., 2013). The frequency and type of link will be affected by the spatiotemporal scale of movement, such as foraging movements within the home range or the less frequent but larger scale movements of migration (Jeltsch et al., 2013). Movement is also important for maintaining interaction networks in both antagonist (e.g., predator-prey) and mutualist (e.g., plant-pollinator) networks (Tylianakis et al., 2010; Hagen et al., 2012). The loss of species interaction networks may have cascading effects on for example food web dynamics resulting in secondary extinctions (Hagen et al., 2012). Managers also need to consider how movement may disturb ecosystems. The aggregating nature, and long-range movements, of many migratory species may impact ecosystems through exploitation of habitats, disease transmission and nutrient loading (Kerbes et al., 1990; Post et al., 2008).
Animal movement may provide important ecosystem services and these services have been termed mobile-agent based ecosystem services (MABES; Kremen et al., 2007). These services are provided at a local scale by species moving within or among habitats (Kremen et al., 2007). For example, the movements of bees pollinate both wild and agricultural plants (Kremen et al., 2007), seeds may be dispersed long distances by birds and mammals (Nathan and Muller-landau, 2000) and nutrients are transferred between marine and terrestrial environments by the foraging movements of seabirds (Ellis et al., 2006). The ongoing modification of the landscape by humans is altering landscape connectivity and threatens the future provision of MABES (Mitchell et al., 2013). Linking animal movement with management planning allows managers to identify the ecosystem functions and services that movement provides and thus improve landscape management (Jeltsch et al., 2013; Mitchell et al., 2013).
A challenge for management is identifying the scale of management required for effective species conservation. The scale of management may be guided by, amongst others, the distance of movements like migrations (Klaassen et al., 2008), by the size of home ranges (Schwartz, 1999), or by the habitat requirements of a species (Angelstam et al., 2004). Habitat selection studies have commonly been used to identify the habitat requirements of a species, in particular using the four orders of habitat selection described by Johnson (1980). The first-order of selection identifies the geographical range of the species and has commonly been used to map species distributions and develop habitat suitability models, which are used to guide the scale of conservation planning (Johnson, 1980; Angelstam et al., 2004; Guisan et al., 2013). The second, third and fourth orders of selection concern the selection of the home range, habitat patches within the home range and microhabitats within used patches respectively (Johnson, 1980; Meyer and Thuiller, 2006). Meyer and Thuiller (2006) introduce a fifth order of selection, which are areas used by populations within the geographical range. These orders of selection inform managers about local reserve site selection (Aldridge and Boyce, 2007; Guisan et al., 2013), provide indications of habitat quality and improvements needed (e.g., Dickson and Beier, 2002; Zeale et al., 2012) and how human disturbance may be influencing species movements (e.g., Hornseth and Rempel, 2015). However, an ongoing challenge in conservation is how to achieve the scale of management required. Knowing species movements answers important management questions like where, when, how and why animals move, which can be used to develop management actions that increase the dynamism and scale of wildlife management, and are complementary to existing plans. Alternative management actions may include ecological networks, time-area closures or threat management, and we expand upon these alternative management actions below.
The first approach is concerned with incorporating localized management actions, such as protected areas or reserves, into a network of areas and thus increasing the scale of management. Ecological networks should maintain ecosystem processes, which includes the movement of organisms and subsequent species interactions networks (Opdam et al., 2006; Hagen et al., 2012). Ecological networks also incorporate multiple objectives into the spatial planning process, such as conservation goals and different land uses by stakeholders (Opdam et al., 2006). A principal concept of ecological networks is connectivity, whereby a set of areas or ecosystems are linked to maintain or enhance population viability by facilitating movement (Beier, 1998; Opdam et al., 2006). Management tools used to improve connectivity of two or more areas are matrix management and corridors (Beier, 1998; Fischer et al., 2005). Identifying which habitats are important for species helps managers create a “soft,” more permeable matrix. For example, a matrix containing scattered trees facilitated the movement of birds in Australia (Fischer et al., 2005). Habitat patches may also act as important stepping stones for long-distance dispersal and range expansions, following climate-driven shifts in habitat suitability (Saura et al., 2014). Strengthening the link between corridor design and species movements improves connectivity in the landscape, for instance, corridors that were identified through tracking studies were more effective than those identified through modeling approaches (LaPoint et al., 2013). Corridors may be beneficial for species moving at larger scales like the Mule deer Odocoileus hemionus R., for maintaining connectivity within a species home range, and to preserve dispersal events and maintain functional connectivity (Table 1; Baguette and Van Dyck, 2007; LaPoint et al., 2013; Sawyer et al., 2013).
The second approach is the use of time-area closures. Time-area closures may be dynamic in time only, i.e., excluding unwanted practices during a specific time of year, such as a stopover site for migratory waterfowl (O'Neal et al., 2008). Time-area closures may also be dynamic in space and time, i.e., the closure tracks the species' movements like the movements of pelagic species (Hobday and Hartmann, 2006). Time-area closures provide a viable alternative for managing species' with predictable movements. They can be implemented when species are most vulnerable, such as aggregation or spawning areas and during critical movement phases (Table 1; Hunter et al., 2006; Shillinger et al., 2008; Bull et al., 2013). Time-area closures may also achieve conservation targets whilst incorporating stakeholder interests, for example by maintaining alternative land-use or harvesting practices, and are being increasingly utilized in marine and freshwater ecosystems (Hobday and Hartmann, 2006; O'Neal et al., 2008; Shillinger et al., 2008).
When it is not possible to spatially delineate large areas, it may be more appropriate to manage the primary threats to a species instead (Carwardine et al., 2012). Understanding a species threats enables managers to prioritize management actions that achieve the greatest impact (Auerbach et al., 2014; Tulloch et al., 2015). Identifying threats to a species may also indicate which movement attributes increase species vulnerability. Traits of some species, such as the predictability of routes, timing of movements, or reliance upon particular sites may increase the risk of exploitation (Bolger et al., 2007). This knowledge can be used to guide management decisions, for instance threat management actions may involve time-specific interventions like anti-poaching activities at important locations and times of the year (Berger et al., 2008; Murgui, 2014). Recent advances in tracking technologies also provide the opportunity to incorporate real-time monitoring data into threat management schemes, thus justifying the cost of tracking animal movements (Wall et al., 2014). Real-time tracking data from African elephants Loxodonta africana in Kenya was used to notify wildlife managers when elephants were about to move into community areas (Wall et al., 2014). Wildlife managers were able to intervene and prevent the elephants causing crop damage and reduce human-wildlife conflicts, a major threat to elephants in Africa (Wall et al., 2014).
The feasibility of management actions described above may be limited at the implementation phase by considerations like costs, stakeholder interests, and enforcement. Incorporating animal movement into management planning may entail a number of costs, such as the costs of acquiring land and the costs associated with establishing and maintaining a network of managed areas (Naidoo et al., 2006). Emphasis is now being placed on identifying the most cost effective action that maximizes benefits (Naidoo et al., 2006; Carwardine et al., 2012; Auerbach et al., 2014). The interests of local stakeholders may also influence the implementation of management actions due to conflicts between involved parties, for example conflicts arising over land-use, resettlement, policies or legislation, and human-wildlife conflicts (Davies et al., 2013; Symes et al., 2015). In addition, limitations may arise due to available manpower, either in relation to monitoring the outcomes of management actions or enforcing them (Keane et al., 2008). These challenges are especially pertinent when a species movements takes them across several country borders (Iwamura et al., 2014; Kark et al., 2015). Cross-boundary collaborations may provide a number of benefits, such as improving the cost effectiveness of management actions and increasing the scale of threat management, however it also presents a number of challenges like political instability, increased costs related to establishing the collaboration, conflicting national goals, and potential delays in implementing actions (Kark et al., 2009, 2015). However, incorporating the above challenges into the decision making process allows managers to identify management strategies that are implementable and attainable.
A number of approaches have been developed to identify which management actions will maximize benefits, such as decision theoretic approaches and systematic conservation planning (e.g., Margules and Pressey, 2000; Wilson et al., 2009). Decision theoretic approaches consist of an objective or desired outcome, a description of our knowledge of the system, state variables in the area of interest, such as species populations or habitats, control variables which represent possible management strategies, model constraints and an equation that describes the relationship between the benefit of actions and the potential management strategies in the area of interest (Wilson et al., 2009). Understanding species movements enables managers to improve their understanding of the threats, use their knowledge of where and when a species will be to identify alternative management actions, and also recognize the challenges of achieving the management objectives which may not be apparent if a species' movements are unknown. Decision theoretic approaches enable managers to prioritize management actions that will better achieve the management objectives (Tulloch et al., 2015). Previously, actions were prioritized on areas with the highest threats or species richness, but now the management actions themselves are being prioritized depending upon whether they are cost-effective, have the greatest impact and are achievable (Auerbach et al., 2014; Tulloch et al., 2015). Multiple-action prioritization schemes are also being considered, whereby a combined set of strategies may be more cost effective and have greater impact than any one strategy alone (Wilson et al., 2009; Chadés et al., 2015). Therefore, it is vital for managers to evaluate all possible management scenarios to improve the effectiveness of the decision-making process.
The importance of evaluating management actions has been highlighted in recent decades to avoid implementing management actions that do not achieve management goals and thus waste limited funds (e.g., Ferraro and Pattanayak, 2006; Walsh et al., 2012). Therefore, management strategy evaluation (MSE) is vital for determining whether actions have either succeeded or failed in achieving the management objective, and using this knowledge to inform future decisions and actions (Pullin et al., 2013). Evaluation is an integral part of adaptive management and is thus applicable to any form of wildlife management. With regards to our framework, evaluation may be important for determining the effectiveness of actions implemented with incomplete knowledge of species movements, and may help prioritize the type of knowledge needed to improve future management. Evaluation is also important for tracking future uncertainties, such as how variation in the timing of movement may affect management strategies like time-area closures, which rely upon knowledge of where and when a species will be.
The effectiveness of management actions can be simulated prior to their implementation, through frameworks like the MSE framework (Smith et al., 1999), which has thus far been used mostly in commercial fisheries but also has relevance for the management and conservation of terrestrial species (Bunnefeld et al., 2011; Milner-Gulland, 2011). MSE compares multiple management strategies prior to their implementation, allowing managers to identify their effectiveness and understand the varying forms of uncertainty, such as incomplete knowledge of species movements (Bunnefeld et al., 2011). The outcomes of MSE are used to inform the management objectives, study design, and implementation of management actions (Bunnefeld et al., 2011; Milner-Gulland, 2011). For example, comparative analyses could be performed to determine whether management actions should be implemented with limited knowledge of movement or whether priority should instead be placed on acquiring data that can be used to develop management actions that are more tailored to species movements and consequently have greater impact, such as those developed for the leatherback turtle discussed earlier (Shillinger et al., 2008).
We illustrate the potential for linking movement ecology with management through a case study of the Atlantic salmon. By following the steps of the framework, we show how existing knowledge of salmon movement ecology has been used to develop management actions and how further knowledge could be used to identify alternative management actions.
Atlantic salmon have had large population declines in the Baltic Sea (Karlsson and Karlstrårn, 1994; Klemetsen et al., 2003) so it is an important issue for management and conservation alike. Overfishing and the loss of connectivity in river systems, due to hydroelectric dams, has been a driving cause of salmon declines and in the 1990s only 12 of the 44 naturally reproducing salmon stocks in the Gulf of Bothnia remained (Karlsson and Karlstrårn, 1994). A number of actions have been taken to restore these populations but several rivers are not self-sustaining and many salmon rivers are below 50% of potential smolt production (ICES, 2012). In this case study, we focus on the management goals of the Swedish government, through the Swedish Agency for Marine and Water Management (SwAM). Their aim is to reverse the decline of salmon stocks whilst maintaining activities like recreational and commercial fishing. Multiple techniques have been used to monitor the movements of salmon that include observational and trapping data (Lundqvist et al., 2010), tagging methods (Payne et al., 2010), acoustic or radio telemetry (Serrano et al., 2009), stable isotopes, and genetics (Barnett-Johnson et al., 2008). Therefore, several sources of movement data are available to continue with the movement-management framework.
Salmon can exhibit all four types of movement (sedentary, dispersal, nomadism, and migration) during its life cycle. Salmon hatch in freshwater rivers, where they are solitary and defend territories for food, thus exhibiting sedentary movements (Lundqvist, 1983). During this phase they may also disperse to nearby tributaries (McCormick et al., 1998). After 1 to 5 years the salmon migrates downstream to enter the sea (Lundqvist, 1983; Otero et al., 2014). Salmon movements in the sea remain difficult to study but this phase can be described as nomadic where the distribution of salmon is largely influenced by environmental factors like sea temperature, surface currents, and food availability (Klemetsen et al., 2003; Trudel et al., 2011). Salmon from multiple river systems mix in the Main Basin of the Baltic Sea, leading to mixed-stock fisheries (Karlsson and Karlstrårn, 1994). Salmon normally migrate back to their natal river systems to spawn (Lundqvist, 1983). The movements of hatchery-reared salmon may differ from wild salmon in their extent, timing, and fidelity (McKinnell et al., 1994; Jutila et al., 2003).
The stage-structured life cycle of salmon means that juveniles and adults occupy and connect different ecosystems (Schreiber and Rudolf, 2008; Miller and Rudolf, 2011). Species with complex life cycles may cause abrupt changes in different ecosystems, such as how changes in juvenile abundance may lead to trophic cascades across ecosystems to the adult habitat (Knight et al., 2005; Schreiber and Rudolf, 2008). The salmon's life cycle influences food web dynamics by preying on aquatic species and being preyed upon by aquatic, terrestrial, and avian species (Holmlund and Hammer, 1999, 2004). The Atlantic salmon is iteroparous, meaning it can spawn repeatedly as opposed to dying after spawning like the semelparous Pacific salmon (Oncorhynchus spp.; Klemetsen et al., 2003). Salmon deaths, and repeated migrations, link ecosystems and transfer nutrients and carbon between marine and freshwater ecosystems (Holmlund and Hammer, 1999, 2004). Salmon provide several ecosystem services. Spawning salmon regulate sediment processes whilst their movements between marine and freshwater ecosystems support aquatic/terrestrial food webs and nutrient cycling (Bottom et al., 2009; Kulmala et al., 2012). Salmon populations provide a highly valued food source for both commercial, personal-use, and recreational fisheries (Kulmala et al., 2012).
The life cycle of the salmon illustrates the importance of understanding their movements due to the direct influence that salmon movement has on their survival and reproduction. As a consequence, the scale of management may vary in accordance to the specific life cycle stage and process that is being targeted by management. For instance, management may consider the implementation of more localized management actions during the sedentary phases of the salmon's life cycle. These include the development phase after hatching salmon and spawning phase of adults. Management actions have therefore focused on either preserving existing habitats used by salmon, or alternatively, restoration actions have been taken to improve habitat suitability for spawning and recently hatched salmon (Nilsson et al., 2005).
A key aspect of salmon movements is the migration from the natal/spawning areas to the sea and their return to rivers. Lundqvist et al. (2008) indicate that a salmon population could increase by 500% if connectivity was improved along the migration path. The severe implications resulting from loss of connectivity has resulted in several management actions that target connectivity between river systems and the sea (McKinnell et al., 1994; Lundqvist et al., 2008; Serrano et al., 2009). Knowledge of migration timing has enabled managers to adopt actions that are flexible in time, which may increase their acceptance by impacted stakeholders like the hydroelectric power industry. These have included diverting water from hydroelectric dams to a bypass during the migratory period only, or transporting salmon 88 km upstream in trucks from the first barrier to the spawning grounds (Lundqvist et al., 2008; Serrano et al., 2009; Hagelin et al., 2015).
Meanwhile, a key aim should be to reduce the amount of fishing effort in the Main Basin, due to stock mixing, which includes threatened stocks. Instead, fishing efforts could be focused in coastal areas or river estuaries where the stock status is known. Understanding the movements of hatchery-reared salmon may also identify alternative management actions for implementation. Research indicates that hatchery-reared salmon are more likely to be caught in the Bothnian Sea compared to wild stocks because hatchery-reared salmon are less likely to migrate to the Main Basin of the Baltic Sea (Jutila et al., 2003). Hatchery-reared salmon may also arrive in the northern Baltic later than wild salmon stocks (McKinnell et al., 1994). This knowledge may be used to implement a time-area based approach, providing a potentially viable management option that maintains recreational and commercial harvesting whilst simultaneously increasing the harvest of hatchery-reared salmon.
The management of salmon influences a number of stakeholders like commercial fisheries, the power industry, general public, and conservation community. Understanding the movement ecology of salmon has enabled managers to identify actions that have a higher likelihood of acceptance by stakeholders and thus improved effectiveness. An example includes the time-area based approach for fisheries, whereby the coastal fishery is opened later in the season meaning that most wild salmon have already entered the river system and the fisheries harvest is dominated by hatchery-reared salmon (ICES, 2012). Such an approach maintains the livelihoods of fisherman whilst reducing harvest pressure on wild salmon and thus achieving conservation targets. Another example is how the knowledge of timing of salmon migrations enables hydroelectric dams to regulate discharge rates, which target conservation goals during the salmon migration and electricity production at other times of the year (Lundqvist et al., 2008; Håkansson, 2009). Achieving management targets increasingly relies upon identifying trade-offs that are acceptable to all stakeholders (Redpath et al., 2013). By linking movement ecology with management, alternative management actions can be identified that allow managers to explore trade-off scenarios with stakeholders, which increase the implementation potential of proposed management actions.
Several management actions for salmon are executed within national boundaries, such as maintaining connectivity in freshwater systems, improving breeding habitats, and managing coastal fisheries. However, during the marine phase of the salmon life cycle, the nomadic movements of adults need transboundary collaborations for effective management. The Main Basin of the Baltic Sea is an offshore fishery that is exploited by many countries in the region. Therefore, international measures are needed to effectively manage the mixed-stock fishery and knowledge of movements and genetics is vital to achieve this goal. Harvest quotas are provided by the Common Fisheries Policy but the Atlantic salmon would benefit further from the re-establishment of an International Baltic Treaty. Transboundary collaborations provide a number of challenges that influence the implementation potential of management actions (Kark et al., 2015). These may include increased financial costs, delayed conservation planning or countries “free-riding” on the assumption that management actions will be implemented by other countries (Kark et al., 2009, 2015). However, transboundary collaborations may also improve the cost effectiveness of management and increase the scale of management to more effectively manage a highly mobile species (Kark et al., 2015).
Mathematical and statistical models have been developed that link biological and economic data to identify management actions for salmon in the Baltic (Kulmala et al., 2008). Kulmala et al. (2008) explicitly incorporate the migratory movements of salmon to determine optimal harvest solutions, and identify that driftnet fisheries should be excluded as a harvest method. Several studies have also evaluated the effectiveness of management plans prior to their implementation, which include both national plans and international plans (Haapasaari and Karjalainen, 2010; Levontin et al., 2011). Bayesian network analyses have been used to incorporate several sources of data that include the biology of the species, expert knowledge, and sociological data to evaluate alternative management options (Haapasaari and Karjalainen, 2010; Levontin et al., 2011). These studies have determined the commitment of stakeholders to management options and how this may influence the effectiveness of proposed management actions (Haapasaari and Karjalainen, 2010; Levontin et al., 2011).
With regards to movement, management interventions like fish ladders may not guarantee connectivity, as illustrated by Lundqvist et al. (2008). The project evaluation found that salmon migrating upstream were not drawn to the bypass containing the fish ladder, due to differing discharge rates from the hydroelectric dam and the bypass. Salmon survival was also reduced the following spring as salmon were not drawn to the fish ladder during the seawards migration, but traveled through the turbines instead. Improved knowledge of the movement ecology of salmon, such as how they move in the river and what cues they are drawn to are needed to improve the designs of existing and future connectivity measures (Lundqvist et al., 2008). In addition, management actions are not being implemented for the full movement cycle. In the example of transporting salmon upstream by truck, no actions are being taken for the downstream migration of salmon, resulting in very low survival rates (Bergman et al., 2014; Hagelin et al., 2015). The recovery of this river's stock is therefore limited by not considering the migratory connectivity of both upstream and downstream migrations. In this instance it would be important to evaluate both the effectiveness of translocating individuals to spawning areas (Fischer and Lindenmayer, 2000), and subsequently whether the action is meeting management objectives of population recovery.
Studies continue to highlight that management actions focused in one area, such as protected areas, are not sufficient for effectively conserving a species (Thirgood et al., 2004; Martin et al., 2007; Runge et al., 2014). Shortfalls include the scale of management, conflicts with stakeholders, alternative land-uses and limited space available (Sanderson et al., 2002; Runge et al., 2014; Symes et al., 2015). Management actions focused in one area also fail to account for species movements (Thirgood et al., 2004; Runge et al., 2014). Linking movement ecology to conservation has several implications for the future management of wildlife. Understanding a species movements enable managers to implement actions along the entire movement path, such as a flyway approach for birds and butterflies (Klaassen et al., 2008; Howard and Davis, 2009) or dynamic protected areas in the ocean (Shillinger et al., 2008; Game et al., 2009) and on land (Singh and Milner-Gulland, 2011; Bull et al., 2013). Understanding movement also enables managers to identify threats, such as the loss of important sites (Iwamura et al., 2013) or barriers to movement (Seidler et al., 2015), and therefore prioritize the most effective management actions that have the highest chance of success (Game et al., 2013; Auerbach et al., 2014; Tulloch et al., 2015). Knowledge of species movements allows managers to identify alternative management actions that are flexible in space and time, such as time-area based approaches that are used to flood wetlands for migratory waterfowl (O'Neal et al., 2008) or time-restricted harvesting practices (Hunter et al., 2006). Knowing where and when a species will be is a pre-requisite for the successful implementation of time-area approaches, which allow managers to develop trade-off scenarios that balance conservation needs with alternative land-use practices (O'Neal et al., 2008; Shillinger et al., 2008; Game et al., 2009; Redpath et al., 2013).
We have highlighted how knowledge gained from movement ecology can be used to identify alternative, complementary strategies in wildlife management. Our conceptual framework provides a step by step workflow that aims to understand the movement patterns of a species and use this knowledge to guide management actions. As the field of movement ecology continues to grow, it is important to strengthen the link with wildlife management to further improve the decision-making capabilities of practitioners and managers.
AA and NS conceived the idea. AA wrote the majority of the manuscript with input from NS
The study was funded by the thematic programme in Wildlife and Forestry at the Swedish University of Agricultural Sciences, the Swedish Environmental Protection Agency (Naturvårdsverket, 802-0102-11) and their committee for Wildlife Research, the Swedish Association for Hunting Wildlife Management.
The authors declare that the research was conducted in the absence of any commercial or financial relationships that could be construed as a potential conflict of interest.
We thank Ascelin Gordon, Anouschka Hof, Göran Spong, and Nils Bunnefeld, and the two reviewers, Robert A. Montgomery and Johannes Signer, for their comments that improved the manuscript.
Aldridge, C. L., and Boyce, M. S. (2007). Linking occurrence and fitness to persistence: habitat-based approach for endangered Greater Sage-Grouse. Ecol. Appl. 17, 508–526. doi: 10.1890/05-1871
Altizer, S., Bartel, R., and Han, B. A. (2011). Animal migration and infectious disease risk. Science 331, 296–302. doi: 10.1126/science.1194694
Angelstam, P., Roberge, J., Lõhmus, A., Bergmanis, M., Brazaitis, G., Edenius, L., et al. (2004). Habitat modelling as a tool for landscape-scale conservation – a review of parameters for focal forest birds. Ecol. Bull. 51, 427–453. Available online at: http://www.jstor.org/stable/20113327
Armsworth, P. R., Block, B. A., Eagle, J., and Roughgarden, J. E. (2010). The economic efficiency of a time-area closure to protect spawning bluefin tuna. J. Appl. Ecol. 47, 36–46. doi: 10.1111/j.1365-2664.2009.01738.x
Auerbach, N. A., Tulloch, A. I. T., and Possingham, H. P. (2014). Informed actions: where to cost-effectively manage multiple threats to species to maximize return on investment. Ecol. Appl. 24, 1357–1373. doi: 10.1890/13-0711.1
Baguette, M., and Van Dyck, H. (2007). Landscape connectivity and animal behavior: functional grain as a key determinant for dispersal. Landsc. Ecol. 22, 1117–1129. doi: 10.1007/s10980-007-9108-4
Barkin, D. (2003). Alleviating poverty through ecotourism: promises and reality in the Monarch butterfly reserve of Mexico. Environ. Dev. Sustain. 5, 371–382. doi: 10.1023/A:1025725012903
Barnett-Johnson, R., Pearson, T. E., Ramos, F. C., Grimes, C. B., and MacFarlane, R. B. (2008). Tracking natal origins of salmon using isotopes, otoliths, and landscape geology. Limnol. Oceanogr. 53, 1633–1642. doi: 10.4319/lo.2008.53.4.1633
Berger, J., Young, J. K., and Berger, K. M. (2008). Protecting Migration Corridors: challenges and optimism for mongolian saiga. PLoS Biol. 6:e165. doi: 10.1371/journal.pbio.0060165
Bergman, E., Norrgård, J. R., Piccolo, J. J., Gustaffson, P., Nilsson, F., and Hart, P. J. B. (2014). Atlantic Salmon and Brown Trout in Lake Vänern: a proposal for a co-management system. Aquat. Ecosyst. Heal. Manag. 17, 365–373. doi: 10.1080/14634988.2014.965119
Bolger, D. T., Newmark, W. D., Morrison, T. A., and Doak, D. F. (2007). The need for integrative approaches to understand and conserve migratory ungulates. Ecol. Lett. 11, 63–77. doi: 10.1111/j.1461-0248.2007.01109.x
Börger, L., Franconi, N., De Michele, G., Gantz, A., Meschi, F., Manica, A., et al. (2006). Effects of sampling regime on the mean and variance of home range size estimates. J. Anim. Ecol. 75, 1393–1405. doi: 10.1111/j.1365-2656.2006.01164.x
Bottom, D. L., Jones, K. K., Simenstad, C. A., and Smith, C. L. (2009). Reconnecting social and ecological resilience in salmon ecosystems. Ecol. Soc. 14:5. Available online at: http://www.ecologyandsociety.org/vol14/iss1/art5/
Brower, L. P., Taylor, O. R., Williams, E. H., Slayback, D. A., Zubieta, R. R., and Ramírez, M. I. (2012). Decline of monarch butterflies overwintering in Mexico: is the migratory phenomenon at risk? Insect Conserv. Divers. 5, 95–100. doi: 10.1111/j.1752-4598.2011.00142
Bull, J. W., Suttle, K. B., Singh, N. J., and Milner-Gulland, E. (2013). Conservation when nothing stands still: moving targets and biodiversity offsets. Front. Ecol. Environ. 11, 203–210. doi: 10.1111/j.1752-4598.2011.00142.x
Bunnefeld, N., Hoshino, E., and Milner-Gulland, E. J. (2011). Management strategy evaluation: a powerful tool for conservation? Trends Ecol. Evol. 26, 441–447. doi: 10.1016/j.tree.2011.05.003
Carwardine, J., O'Connor, T., Legge, S., Mackey, B., Possingham, H. P., and Martin, T. G. (2012). Prioritizing threat management for biodiversity conservation. Conserv. Lett. 5, 196–204. doi: 10.1111/j.1755-263X.2012.00228.x
Carwardine, J., Wilson, K. A., Watts, M., Etter, A., Klein, C. J., and Possingham, H. P. (2008). Avoiding costly conservation mistakes: the importance of defining actions and costs in spatial priority setting. PLoS ONE 3:e2586. doi: 10.1371/journal.pone.0002586
Chadés, I., Nicol, S., van Leeuwen, S., Walters, B., Firn, J., Reeson, A., et al. (2015). Benefits of integrating complementarity into priority threat management. Conserv. Biol. 29, 525–536. doi: 10.1111/cobi.12413
Chetkiewicz, C.-L. B., and Boyce, M. S. (2009). Use of resource selection functions to identify conservation corridors. J. Appl. Ecol. 46, 1036–1047. doi: 10.1111/j.1365-2664.2009.01686.x
Davies, A. L., Bryce, R., and Redpath, S. M. (2013). Use of multicriteria decision analysis to address conservation conflicts. Conserv. Biol. 27, 936–944. doi: 10.1111/cobi.12090
Dickson, B. G., and Beier, P. (2002). Home-range and habitat selection by adult cougars in southern california. J. Wildl. Manage. 66, 1235–1245. doi: 10.2307/3802956
Dzialak, M. R., Olson, C. V., Harju, S. M., Webb, S. L., Mudd, J. P., Winstead, J. B., et al. (2011). Identifying and prioritizing greater sage-grouse nesting and brood-rearing habitat for conservation in human-modified landscapes. PLoS ONE 6:e26273. doi: 10.1371/journal.pone.0026273
Ellis, J. C., Fariña, J. M., and Witman, J. D. (2006). Nutrient transfer from sea to land: the case of gulls and cormorants in the Gulf of Maine. J. Anim. Ecol. 75, 565–574. doi: 10.1111/j.1365-2656.2006.01077.x
Faaborg, J., Holmes, R. T., Anders, A. D., Bildstein, K. L., Dugger, K. M., Gauthreaux, S. A., et al. (2010). Conserving migratory land birds in the New World: do we know enough? Ecol. Appl. 20, 398–418. doi: 10.1890/09-0397.1
Fedy, B. C., Aldridge, C. L., Doherty, K. E., O'Donnell, M., Beck, J. L., Bedrosian, B., et al. (2012). Interseasonal movements of greater sage-grouse, migratory behavior, and an assessment of the core regions concept in Wyoming. J. Wildl. Manage. 76, 1062–1071. doi: 10.1002/jwmg.337
Ferguson, S. H., and Elkie, P. C. (2004). Seasonal movement patterns of woodland caribou (Rangifer tarandus caribou). J. Zool. Lond. 262, 125–134. doi: 10.1017/S0952836903004552
Ferraro, P. J., and Pattanayak, S. K. (2006). Money for nothing? A call for empirical evaluation of biodiversity conservation investments. PLoS Biol. 4: 482–488. doi: 10.1371/journal.pbio.0040105
Figuerola, J., and Green, A. J. (2002). Dispersal of aquatic organisms by waterbirds: a review of past research and priorities for future studies. Freshw. Biol. 47, 483–494. doi: 10.1046/j.1365-2427.2002.00829.x
Fischer, J., Fazey, I., Briese, R., and Lindenmayer, D. B. (2005). Making the matrix matter: challenges in Australian grazing landscapes. Biodivers. Conserv. 14, 561–578. doi: 10.1007/s10531-004-3916-5
Fischer, J., and Lindenmayer, D. B. (2000). An assessment of the published results of animal relocations. Biol. Conserv. 96, 1–11. doi: 10.1016/S0006-3207(00)00048-3
Fleming, C. H., Fagan, W. F., Mueller, T., Olson, K. A., Leimgruber, P., and Calabrese, J. M. (2015). Rigorous home range estimation with movement data: a new autocorrelated kernel density estimator. Ecology 96, 1182–1188. doi: 10.1890/14-2010.1
Fossette, S., Hobson, V. J., Girard, C., Calmettes, B., Gaspar, P., Georges, J.-Y., et al. (2010). Spatio-temporal foraging patterns of a giant zooplanktivore, the leatherback turtle. J. Mar. Syst. 81, 225–234. doi: 10.1016/j.jmarsys.2009.12.002
Gaines, S. D., White, C., Carr, M. H., and Palumbi, S. R. (2010). Designing marine reserve networks for both conservation and fisheries management. Proc. Natl. Acad. Sci. U.S.A. 107, 18286–18293. doi: 10.1073/pnas.0906473107
Game, E. T., Grantham, H. S., Hobday, A. J., Pressey, R. L., Lombard, A. T., Beckley, L. E., et al. (2009). Pelagic protected areas: the missing dimension in ocean conservation. Trends Ecol. Evol. 24, 360–369. doi: 10.1016/j.tree.2009.01.011
Game, E. T., Kareiva, P., and Possingham, H. P. (2013). Six common mistakes in conservation priority setting. Conserv. Biol. 27, 480–485. doi: 10.1111/cobi.12051
Grantham, H. S., Wilson, K. A., Moilanen, A., Rebelo, T., and Possingham, H. P. (2009). Delaying conservation actions for improved knowledge: how long should we wait? Ecol. Lett. 12, 293–301. doi: 10.1111/j.1461-0248.2009.01287.x
Guisan, A., Tingley, R., Baumgartner, J. B., Naujokaitis-Lewis, I., Sutcliffe, P. R., Tulloch, A. I. T., et al. (2013). Predicting species distributions for conservation decisions. Ecol. Lett. 16, 1424–1435. doi: 10.1111/ele.12189
Haapasaari, P., and Karjalainen, T. P. (2010). Formalizing expert knowledge to compare alternative management plans: sociological perspective to the future management of Baltic salmon stocks. Mar. Policy 34, 477–486. doi: 10.1016/j.marpol.2009.10.002
Hagelin, A., Calles, O., Greenberg, L., Piccolo, J., and Bergman, E. (2015). Spawning migration of wild and supplementary stocked landlocked Atlantic salmon (Salmo salar). River Res. Appl. doi: 10.1002/rra.2870. [Epub ahead of print].
Hagen, M., Kissling, W. D., Rasmussen, C., De Aguiar, M. A. M., Brown, L. E., Carstensen, D. W., et al. (2012). Biodiversity, species interactions and ecological networks in a fragmented world. Adv. Ecol. Res. 46, 89–120. doi: 10.1016/B978-0-12-396992-7.00002-2
Håkansson, C. (2009). Costs and benefits of improving wild salmon passage in a regulated river. J. Environ. Plan. Manag. 52, 345–363. doi: 10.1080/09640560802703249
Harris, G., Thirgood, S., Hopcraft, J., Cromsight, J., and Berger, J. (2009). Global decline in aggregated migrations of large terrestrial mammals. Endanger. Species Res. 7, 55–76. doi: 10.3354/esr00173
Hebblewhite, M., and Haydon, D. T. (2010). Distinguishing technology from biology: a critical review of the use of GPS telemetry data in ecology. Philos. Trans. R. Soc. Lond. B Biol. Sci. 365, 2303–2312. doi: 10.1098/rstb.2010.0087
Hobday, A. J., and Hartmann, K. (2006). Near real-time spatial management based on habitat predictions for a longline bycatch species. Fish. Manag. Ecol. 13, 365–380. doi: 10.1111/j.1365-2400.2006.00515.x
Hobson, K. A., Soto, D. X., Paulson, D. R., Wassenaar, L. I., and Matthews, J. H. (2012). A dragonfly (δ2H) isoscape for North America: a new tool for determining natal origins of migratory aquatic emergent insects. Methods Ecol. Evol. 3, 766–772. doi: 10.1111/j.2041-210X.2012.00202.x
Hodgson, J. A., Moilanen, A., Wintle, B. A., and Thomas, C. D. (2011). Habitat area, quality and connectivity: striking the balance for efficient conservation. J. Appl. Ecol. 48, 148–152. doi: 10.1111/j.1365-2664.2010.01919.x
Holdo, R. M., Galvin, K. A., Knapp, E. L. I., Polasky, S., Hilborn, R. A. Y, and Holt, R. D. (2010). Responses to alternative rainfall regimes and antipoaching in a migratory system. Ecol. Appl. 20, 381–397. doi: 10.1890/08-0780.1
Holmlund, C. M., and Hammer, M. (1999). Ecosystem services generated by fish populations. Ecol. Econ. 29, 253–268. doi: 10.1016/S0921-8009(99)00015-4
Holmlund, C. M., and Hammer, M. (2004). Effects of fish stocking on ecosystem services: an overview and case study using the stockholm archipelago. Environ. Manage. 33, 799–820. doi: 10.1007/s00267-004-0051-8
Hornseth, M. L., and Rempel, R. S. (2015). Seasonal resource selection of woodland caribou (Rangifer tarandus caribou) across a gradient of anthropogenic disturbance. Can. J. Zool. doi: 10.1139/cjz-2015-0101. [Epub ahead of print].
Howard, E., and Davis, A. K. (2009). The fall migration flyways of monarch butterflies in eastern North America revealed by citizen scientists. J. Insect Conserv. 13, 279–286. doi: 10.1007/s10841-008-9169-y
Hunter, E., Berry, F., Buckley, A. A., Stewart, C., and Metcalfe, J. D. (2006). Seasonal migration of thornback rays and implications for closure management. J. Appl. Ecol. 43, 710–720. doi: 10.1111/j.1365-2664.2006.01194.x
ICES. (2012). Report of the Baltic salmon and trout assessment working group (WGBAST), Vol. 353, Uppsala, 15–23.
Iwamura, T., Fuller, R. A., and Possingham, H. P. (2014). Optimal management of a multispecies shorebird flyway under sea-level rise. Conserv. Biol. 28, 1710–1720. doi: 10.1111/cobi.12319
Iwamura, T., Possingham, H. P., Chadès, I., Minton, C., Murray, N. J., Rogers, D. I., et al. (2013). Migratory connectivity magnifies the consequences of habitat loss from sea-level rise for shorebird populations. Proc. R. Soc. B Biol. Sci. 280:20130325. doi: 10.1098/rspb.2013.0325
Jeltsch, F., Bonte, D., Pe'er, G., Reineking, B., Leimgruber, P., Balkenhol, N., et al. (2013). Integrating movement ecology with biodiversity research - exploring new avenues to address spatiotemporal biodiversity dynamics. Mov. Ecol. 1:6. doi: 10.1186/2051-3933-1-6
Johnson, D. H. (1980). The comparison of usage and availability measurements for evaluating resource preference. Ecology 61, 65–71. doi: 10.2307/1937156
Johnson, C. J., Seip, D. R., and Boyce, M. S. (2004). A quantitative approach to conservation planning: using resource selection functions to map the distribution of mountain caribou at multiple spatial scales. J. Appl. Ecol. 41, 238–251. doi: 10.1111/j.0021-8901.2004.00899.x
Jutila, E., Jokikokko, E., Kallio-Nyberg, I., Saloniemi, I., and Pasanen, P. (2003). Differences in sea migration between wild and reared Atlantic salmon (Salmo salar L.) in the Baltic Sea. Fish. Res. 60, 333–343. doi: 10.1016/S0165-7836(02)00169-8
Kark, S., Levin, N., Grantham, H. S., and Possingham, H. P. (2009). Between-country collaboration and consideration of costs increase conservation planning efficiency in the Mediterranean Basin. Proc. Natl. Acad. Sci. U.S.A. 106, 15368–15373. doi: 10.1073/pnas.0901001106
Kark, S., Tulloch, A., Gordon, A., Mazor, T., Bunnefeld, N., and Levin, N. (2015). Cross-boundary collaboration: key to the conservation puzzle. Curr. Opin. Environ. Sustain. 12, 12–24. doi: 10.1016/j.cosust.2014.08.005
Karlsson, L., and Karlstrårn, Ö. (1994). The Baltic salmon (Salmo salar L.): its history, present situation and future. Dana 10, 61–85.
Keane, A., Jones, J. P. G., Edwards-Jones, G., and Milner-Gulland, E. J. (2008). The sleeping policeman: understanding issues of enforcement and compliance in conservation. Anim. Conserv. 11, 75–82. doi: 10.1111/j.1469-1795.2008.00170.x
Kellermann, J. L., Johnson, M. D., Stercho, A. M., and Hackett, S. C. (2008). Ecological and economic services provided by birds on jamaican blue mountain coffee farms. Conserv. Biol. 22, 1177–1185. doi: 10.1111/j.1523-1739.2008.00968.x
Kerbes, R. H., Kotanen, P. M., and Jefferies, R. L. (1990). Destruction of wetland habitats by lesser snow geese: a keystone species on the West Coast of Hudson Bay. J. Appl. Ecol. 27, 242–258. doi: 10.2307/2403582. Available online at: http://www.jstor.org/stable/10.2307/2403582 (Accessed October 17, 2013).
Klaassen, M., Bauer, S., Madsen, J., and Possingham, H. (2008). Optimal management of a goose flyway: migrant management at minimum cost. J. Appl. Ecol. 45, 1446–1452. doi: 10.1111/j.1365-2664.2008.01532.x
Klemetsen, A., Amundsen, P. A., Dempson, J. B., Jonsson, B., Jonsson, N., O'Connell, M. F., et al. (2003). Atlantic salmon Salmo salar L., brown trout Salmo trutta L. and Arctic charr Salvelinus alpinus (L.): a review of aspects of their life histories. Ecol. Freshw. Fish 12, 1–59. doi: 10.1034/j.1600-0633.2003.00010.x
Knight, T. M., McCoy, M. W., Chase, J. M., McCoy, K. A., and Holt, R. D. (2005). Trophic cascades across ecosystems. Nature 437, 880–883. doi: 10.1038/nature03962
Kranstauber, B., Kays, R., Lapoint, S. D., Wikelski, M., and Safi, K. (2012). A dynamic Brownian bridge movement model to estimate utilization distributions for heterogeneous animal movement. J. Anim. Ecol. 81, 738–746. doi: 10.1111/j.1365-2656.2012.01955.x
Kremen, C., Williams, N. M., Aizen, M. A., Gemmill-Herren, B., LeBuhn, G., Minckley, R., et al. (2007). Pollination and other ecosystem services produced by mobile organisms: a conceptual framework for the effects of land-use change. Ecol. Lett. 10, 299–314. doi: 10.1111/j.1461-0248.2007.01018.x
Kulmala, S., Haapasaari, P., Karjalainen, T. P., Kuikka, S., Pakarinen, T., Parkkila, K., et al. (2012). “Ecosystem services provided by Baltic salmon – a regional perspective to the socio-economic benefits associated with a keystone migratory species,” in Synthesis in the Context of The Economics of Ecosystems and Biodiversity (TEEB)2 (TemaNord 2012:559, Nordic Council of Ministers), ed E. Watkins (Copenhagen: Nordic Council of Ministers).
Kulmala, S., Laukkanen, M., and Michielsens, C. (2008). Reconciling economic and biological modeling of migratory fish stocks: optimal management of the Atlantic salmon fishery in the Baltic Sea. Ecol. Econ. 64, 716–728. doi: 10.1016/j.ecolecon.2007.08.002
LaPoint, S., Gallery, P., Wikelski, M., and Kays, R. (2013). Animal behavior, cost-based corridor models, and real corridors. Landsc. Ecol. 28, 1615–1630. doi: 10.1007/s10980-013-9910-0
Levontin, P., Kulmala, S., Haapasaari, P., and Kuikka, S. (2011). Integration of biological, economic, and sociological knowledge by Bayesian belief networks: the interdisciplinary evaluation of potential management plans for Baltic salmon. ICES J. Mar. Sci. 68, 632–638. doi: 10.1093/icesjms/fsr004
López-Hoffman, L., Varady, R. G., Flessa, K. W., and Balvanera, P. (2010). Ecosystem services across borders: a framework for transboundary conservation policy. Front. Ecol. Environ. 8, 84–91. doi: 10.1890/070216
Low Choy, S., O'Leary, R., and Mengersen, K. (2009). Elicitation by design in ecology: using expert opinion to inform priors for Bayesian statistical models. Ecology 90, 265–277. doi: 10.1890/07-1886.1
Lu, N., Jia, C. X., Lloyd, H., and Sun, Y. H. (2012). Species-specific habitat fragmentation assessment, considering the ecological niche requirements and dispersal capability. Biol. Conserv. 152, 102–109. doi: 10.1016/j.biocon.2012.04.004
Lundberg, J., and Moberg, F. (2003). Mobile link organisms and ecosystem functioning: implications for ecosystem resilience and management. Ecosystems 6, 0087–0098. doi: 10.1007/s10021-002-0150-4
Lundqvist, H. (1983). Precocious Sexual Maturation and Smolting in Baltic Salmon (Salmo salar): Photoperiodic Synchronisation and Adaptive Significance of Annual Biological Cycles. Ph.D. thesis, University of Umeå, Sweden.
Lundqvist, H., Leonardsson, K., Carlsson, U., Larsson, S., Nilsson, J., Östergren, J., et al. (2010). “Monitoring juvenile Atlantic Salmon and Sea Trout in the River Sävarån, Northern Sweden,” in Biological Monitoring in Freshwater Habitats (Dordrecht: Springer), 207–218.
Lundqvist, H., Rivinoja, P., Leonardsson, K., and McKinnell, S. (2008). Upstream passage problems for wild Atlantic salmon (Salmo salar L.) in a regulated river and its effect on the population. Hydrobiologia 602, 111–127. doi: 10.1007/s10750-008-9282-7
Margules, C. R., and Pressey, R. L. (2000). Systematic conservation planning. Nature 405, 243–253. doi: 10.1038/35012251
Martin, T., Chadès, I., Arcese, P., Marra, P. P., Possingham, H. P., and Norris, D. R. (2007). Optimal conservation of migratory species. PLoS ONE 2:e751. doi: 10.1371/journal.pone.0000751
Marzluff, J. M., Millspaugh, J. J., Hurvitz, P., and Handcock, M. S. (2004). Relating resources to a probabilistic measure of space use: forest fragments and Steller's Jays. Ecology 85, 1411–1427. doi: 10.1890/03-0114
Massol, F., Gravel, D., Mouquet, N., Cadotte, M. W., Fukami, T., and Leibold, M. A. (2011). Linking community and ecosystem dynamics through spatial ecology. Ecol. Lett. 14, 313–323. doi: 10.1111/j.1461-0248.2011.01588.x
Matthysen, E. (2012). “Multicausality of dispersal,” in Dispersal Ecology and Evolution, eds J. Clobert, M. Baguette, T. G. Benton, and J. M. Bullock (Oxford: Oxford University Press), 3–18.
McCormick, S. D., Hansen, L. P., Quinn, T. P., and Saunders, R. L. (1998). Movement, migration, and smolting of Atlantic salmon (Salmo salar). Can. J. Fish. Aquat. Sci. 55, 77–92. doi: 10.1139/d98-011
McKinnell, S., Lundqvist, H., and Johansson, H. (1994). Biological characteristics of the upstream migration of naturally and hatchery-reared Baltic salmon, Salmo salar L. Aquac. Fish. Manag. 25, 45–63.
McNamara, J. M., Barta, Z., Klaassen, M., and Bauer, S. (2011). Cues and the optimal timing of activities under environmental changes. Ecol. Lett. 14, 1183–1190. doi: 10.1111/j.1461-0248.2011.01686.x
Meyer, C. B., and Thuiller, W. (2006). Accuracy of resource selection functions across spatial scales. Divers. Distrib. 12, 288–297. doi: 10.1111/j.1366-9516.2006.00241.x
Miller, T. E. X., and Rudolf, V. H. W. (2011). Thinking inside the box: community-level consequences of stage-structured populations. Trends Ecol. Evol. 26, 457–466. doi: 10.1016/j.tree.2011.05.005
Milner-Gulland, E. J. (1997). A stochastic dynamic programming model for the management of the Saiga antelope. Ecol. Appl. 7, 130–142. doi: 10.1890/1051-0761(1997)007[0130:ASDPMF]2.0.CO;2
Milner-Gulland, E. J. (2011). Integrating fisheries approaches and household utility models for improved resource management. Proc. Natl. Acad. Sci. U.S.A. 108, 1741–1746. doi: 10.1073/pnas.1010533108
Minor, E. S., and Lookingbill, T. R. (2010). A multiscale network analysis of protected-area connectivity for mammals in the United States. Conserv. Biol. 24, 1549–1558. doi: 10.1111/j.1523-1739.2010.01558.x
Mitchell, M. G. E., Bennett, E. M., and Gonzalez, A. (2013). Linking Landscape Connectivity and Ecosystem Service Provision: current knowledge and research gaps. Ecosystems 16, 894–908. doi: 10.1007/s10021-013-9647-2
Moffitt, E. A., Botsford, L. W., Kaplan, D. M., and O'Farrell, M. R. (2009). Marine reserve networks for species that move within a home range. Ecol. Appl. 19, 1835–1847. doi: 10.1890/08-1101.1
Møller, A. P., Rubolini, D., and Lehikoinen, E. (2008). Populations of migratory bird species that did not show a phenological response to climate change are declining. Proc. Natl. Acad. Sci. U.S.A. 105, 16195–16200. doi: 10.1073/pnas.0803825105
Monteith, K. L., Bleich, V. C., Stephenson, T. R., Pierce, B. M., Conner, M. M., Klaver, R. W., et al. (2011). Timing of seasonal migration in mule deer: effects of climate, plant phenology, and life-history characteristics. Ecosphere 2:art47. doi: 10.1890/ES10-00096.1
Morales, J. M., Moorcroft, P. R., Matthiopoulos, J., Frair, J. L., Kie, J. G., Powell, R. A., et al. (2010). Building the bridge between animal movement and population dynamics. Philos. Trans. R. Soc. Lond. B Biol. Sci. 365, 2289–2301. doi: 10.1098/rstb.2010.0082
Mueller, T., and Fagan, W. F. (2008). Search and navgation in dynamic environments - from individual behaviours to population distributions. Oikos 117, 654–664. doi: 10.1111/j.0030-1299.2008.16291.x
Mueller, T., Olson, K. A., Fuller, T. K., Schaller, G. B., Murray, M. G., and Leimgruber, P. (2008). In search of forage: predicting dynamic habitats of Mongolian gazelles using satellite-based estimates of vegetation productivity. J. Appl. Ecol. 45, 649–658. doi: 10.1111/j.1365-2664.2007.01371.x
Murgui, E. (2014). When governments support poaching: a review of the illegal trapping of thrushes Turdus spp. in the parany of Comunidad Valenciana, Spain. Bird Conserv. Int. 24, 127–137. doi: 10.1017/S095927091300052X
Myers, J. A., Vellend, M., Gardescu, S., and Marks, P. L. (2004). Seed dispersal by white-tailed deer: implications for long-distance dispersal, invasion, and migration of plants in eastern North America. Oecologia 139, 35–44. doi: 10.1007/s00442-003-1474-2
Naidoo, R., Balmford, A., Ferraro, P., Polasky, S., Ricketts, T., and Rouglet, M. (2006). Integrating economic costs into conservation planning. Trends Ecol. Evol. 21, 681–687. doi: 10.1016/j.tree.2006.10.003
Nathan, R., Getz, W. M., Revilla, E., Holyoak, M., Kadmon, R., Saltz, D., et al. (2008). A movement ecology paradigm for unifying organismal movement research. Proc. Natl. Acad. Sci. U.S.A. 105, 19052–19059. doi: 10.1073/pnas.0800375105
Nathan, R., and Muller-landau, H. C. (2000). Spatial patterns of seed dispersal, their determinants and consequences for recruitment. Trends Ecol. Evol. 15, 278–285. doi: 10.1016/S0169-5347(00)01874-7
Nilsson, C., Lepori, F., Malmqvist, B., Törnlund, E., Hjerdt, N., Helfield, J. M., et al. (2005). Forecasting environmental responses to restoration of rivers used as log floatways: an interdisciplinary challenge. Ecosystems 8, 779–800. doi: 10.1007/s10021-005-0030-9
O'Neal, B. J., Heske, E. J., and Stafford, J. D. (2008). Waterbird response to wetlands restored through the conservation reserve enhancement program. J. Wildl. Manage. 72, 654–664. doi: 10.2193/2007-165
Opdam, P., Steingröver, E., Rooij, S., and van (2006). Ecological networks: a spatial concept for multi-actor planning of sustainable landscapes. Landsc. Urban Plan. 75, 322–332. doi: 10.1016/j.landurbplan.2005.02.015
Otero, J., L'Abée-Lund, J. H., Castro-Santos, T., Leonardsson, K., Storvik, G. O., Jonsson, B., et al. (2014). Basin-scale phenology and effects of climate variability on global timing of initial seaward migration of Atlantic salmon (Salmo salar). Glob. Chang. Biol. 20, 61–75. doi: 10.1111/gcb.12363
Patterson, T., Thomas, L., Wilcox, C., Ovaskainen, O., and Matthiopoulos, J. (2008). State–space models of individual animal movement. Trends Ecol. Evol. 23, 87–94. doi: 10.1016/j.tree.2007.10.009
Payne, J., Andrews, K., Chittenden, C., Crossin, G., Goetz, F., Hinch, S., et al. (2010). “Tracking fish movements and survival on the northeast Pacific shelf,” in Life in the World's Oceans: Diversity, Distribution, and Abundance, ed A. MacIntyre (Oxford: Blackwell Publishing Ltd), 267–290.
Pinard, V., Dussault, C., Ouellet, J.-P., Fortin, D., and Courtois, R. (2012). Calving rate, calf survival rate, and habitat selection of forest-dwelling caribou in a highly managed landscape. J. Wild. Manange. 76, 189–199. doi: 10.1002/jwmg.217
Post, D. M., Taylor, J. P., Kitchell, J. F., Olson, M. H., Schindler, D. E., and Herwig, B. R. (2008). The role of migratory waterfowl as nutrient vectors in a managed wetland. Conserv. Biol. 12, 910–920. doi: 10.1111/j.1523-1739.1998.97112.x
Pullin, A. S., Sutherland, W., Gardner, T., Kapos, V., and Fa, J. E. (2013). “Conservation priorities: identifying need, taking action and evaluating success,” in Key Topics in Conservation Biology 2, eds D. W. MacDonald and K. J. Willis (Oxford: John Wiley & Sons), 3–22.
Redpath, S. M., Young, J., Evely, A., Adams, W. M., Sutherland, W. J., Whitehouse, A., et al. (2013). Understanding and managing conservation conflicts. Trends Ecol. Evol. 28, 100–109. doi: 10.1016/j.tree.2012.08.021
Rowland, M. M., Wisdom, M. J., Suring, L. H., and Meinke, C. W. (2006). Greater sage-grouse as an umbrella species for sagebrush-associated vertebrates. Biol. Conserv. 129, 323–335. doi: 10.1016/j.biocon.2005.10.048
Runge, C. A., Martin, T. G., Possingham, H. P., Willis, S. G., and Fuller, R. A. (2014). Conserving mobile species. Front. Ecol. Environ. 12, 395–402. doi: 10.1890/130237
Saher, D. J., and Schmieglow, F. K. A. (2004). Movement pathways and habitat selection by woodland caribou during spring migration. Rangifer 25, 143–154. doi: 10.7557/2.25.4.1779
Sanderson, E. W., Redford, K. H., Vedder, A., Coppolillo, P. B., and Ward, S. E. (2002). A conceptual model for conservation planning based on landscape species requirements. Landsc. Urban Plan. 58, 41–56. doi: 10.1016/S0169-2046(01)00231-6
Saura, S., Bodin, Ö., and Fortin, M.-J. (2014). Stepping stones are crucial for species' long-distance dispersal and range expansion through habitat networks. J. Appl. Ecol. 51, 171–182. doi: 10.1111/1365-2664.12179
Sawyer, H., and Kauffman, M. J. (2011). Stopover ecology of a migratory ungulate. J. Anim. Ecol. 80, 1078–1087. doi: 10.1111/j.1365-2656.2011.01845.x
Sawyer, H., Kauffman, M. J., Middleton, A. D., Morrison, T. A., Nielson, R. M., and Wyckoff, T. B. (2013). A framework for understanding semi-permeable barrier effects on migratory ungulates. J. Appl. Ecol. 50, 68–78. doi: 10.1111/1365-2664.12013
Schreiber, S., and Rudolf, V. H. W. (2008). Crossing habitat boundaries: coupling dynamics of ecosystems through complex life cycles. Ecol. Lett. 11, 576–587. doi: 10.1111/j.1461-0248.2008.01171.x
Schwartz, M. W. (1999). Choosing the appropriate scale of reserves for conservation. Annu. Rev. Ecol. Syst. 30, 83–108. doi: 10.1146/annurev.ecolsys.30.1.83
Seidler, R. G., Long, R. A., Berger, J., Bergen, S., and Beckmann, J. P. (2015). Identifying impediments to long-distance mammal migrations. Conserv. Biol. 29, 99–109. doi: 10.1111/cobi.12376
Serrano, I., Rivinoja, P., Karlsson, L., and Larsson, S. (2009). Riverine and early marine survival of stocked salmon smolts, Salmo salar L., descending the Testebo River, Sweden. Fish. Manag. Ecol. 16, 386–394. doi: 10.1111/j.1365-2400.2009.00688.x
Sherrill-Mix, S. A., James, M. C., and Myers, R. A. (2008). Migration cues and timing in leatherback sea turtles. Behav. Ecol. 19, 231–236. doi: 10.1093/beheco/arm104
Shillinger, G. L., Palacios, D. M., Bailey, H., Bograd, S. J., Swithenbank, A. M., Gaspar, P., et al. (2008). Persistent leatherback turtle migrations present opportunities for conservation. PLoS Biol. 6:e171. doi: 10.1371/journal.pbio.0060171
Singh, N. J., and Milner-Gulland, E. J. (2011). Conserving a moving target: planning protection for a migratory species as its distribution changes. J. Appl. Ecol. 48, 35–46. doi: 10.1111/j.1365-2664.2010.01905.x
Smith, A. D. M., Sainsbury, K. J., and Stevens, R. A. (1999). Implementing effective fisheries-management systems - management strategy evaluation and the Australian partnership approach. ICES J. Mar. Sci. 56, 967–979. doi: 10.1006/jmsc.1999.0540
Symes, W. S., Rao, M., Mascia, M. B., and Carrasco, R. L. (2015). Why do we lose protected areas? Factors influencing protected area downgrading, downsizing and degazettment (PADDD) in the tropics and sub-tropics. Glob. Chang. Biol. doi: 10.1111/gcb.13089. [Epub ahead of print].
Thirgood, S., Mosser, A., Tham, S., Hopcraft, G., Mwangomo, E., Mlengeya, T., et al. (2004). Can parks protect migratory ungulates? The case of the Serengeti wildebeest. Anim. Conserv. 7, 113–120. doi: 10.1017/S1367943004001404
Tomkiewicz, S. M., Fuller, M. R., Kie, J. G., and Bates, K. K. (2010). Global positioning system and associated technologies in animal behaviour and ecological research. Philos. Trans. R. Soc. Lond. B Biol. Sci. 365, 2163–2176. doi: 10.1098/rstb.2010.0090
Trudel, M., Jensen, A. J., Fiske, P., Hansen, L. P., Johnsen, B. O., Mork, K. A., et al. (2011). Synchrony in marine growth among Atlantic salmon (Salmo salar) populations. Can. J. Fish. Aquat. Sci. 68, 444–457. doi: 10.1139/F10-156
Tulloch, V. J. D., Tulloch, A. I. T., Visconti, P., Halpern, B. S., Watson, J. E. M., Evans, M. C., et al. (2015). Why do we map threats? Linking threat mapping with actions to make better conservation decisions. Front. Ecol. Environ. 13, 91–99. doi: 10.1890/140022
Tylianakis, J. M., Laliberté, E., Nielsen, A., and Bascompte, J. (2010). Conservation of species interaction networks. Biol. Conserv. 143, 2270–2279. doi: 10.1016/j.biocon.2009.12.004
van Moorter, B., Bunnefeld, N., Panzacchi, M., Rolandsen, C. M., Solberg, E. J., and Saether, B.-E. (2013). Understanding scales of movement: animals ride waves and ripples of environmental change. J. Anim. Ecol. 82, 770–780. doi: 10.1111/1365-2656.12045
Wall, J., Wittemyer, G., Klinkenberg, B., and Douglas-Hamilton, I. (2014). Novel opportunities for wildlife conservation and research with real-time monitoring. Ecol. Appl. 24, 593–601. doi: 10.1890/13-1971.1
Walsh, J. C., Wilson, K. A., Benshemesh, J., and Possingham, H. P. (2012). Unexpected outcomes of invasive predator control: the importance of evaluating conservation management actions. Anim. Conserv. 15, 319–328. doi: 10.1111/j.1469-1795.2012.00537.x
Webster, M. S., Marra, P. P., Haig, S. M., Bensch, S., and Holmes, R. T. (2002). Links between worlds: unraveling migratory connectivity. Trends Ecol. Evol. 17, 76–83. doi: 10.1016/S0169-5347(01)02380-1
Wikelski, M., Moskowitz, D., Adelman, J. S., Cochran, J., Wilcove, D. S., and May, M. L. (2006). Simple rules guide dragonfly migration. Biol. Lett. 2, 325–329. doi: 10.1098/rsbl.2006.0487
Wilson, K. A., Carwardine, J., and Possingham, H. P. (2009). Setting conservation priorities. Ann. N Y. Acad. Sci. 1162, 237–264. doi: 10.1111/j.1749-6632.2009.04149.x
Winkler, D. W., Jørgensen, C., Both, C., Houston, A. I., McNamara, J. M., Levey, D. J., et al. (2014). Cues, strategies, and outcomes: how migrating vertebrates track environmental change. Mov. Ecol. 2, 10. doi: 10.1186/2051-3933-2-10
Keywords: adaptive management, animal movement, conservation, movement ecology, wildlife management
Citation: Allen AM and Singh NJ (2016) Linking Movement Ecology with Wildlife Management and Conservation. Front. Ecol. Evol. 3:155. doi: 10.3389/fevo.2015.00155
Received: 08 October 2015; Accepted: 22 December 2015;
Published: 12 January 2016.
Edited by:
Matt W. Hayward, Bangor University, UKReviewed by:
Robert A. Montgomery, Michigan State University, USACopyright © 2016 Allen and Singh. This is an open-access article distributed under the terms of the Creative Commons Attribution License (CC BY). The use, distribution or reproduction in other forums is permitted, provided the original author(s) or licensor are credited and that the original publication in this journal is cited, in accordance with accepted academic practice. No use, distribution or reproduction is permitted which does not comply with these terms.
*Correspondence: Andrew M. Allen, YW5kcmV3LmFsbGVuQHNsdS5zZQ==
Disclaimer: All claims expressed in this article are solely those of the authors and do not necessarily represent those of their affiliated organizations, or those of the publisher, the editors and the reviewers. Any product that may be evaluated in this article or claim that may be made by its manufacturer is not guaranteed or endorsed by the publisher.
Research integrity at Frontiers
Learn more about the work of our research integrity team to safeguard the quality of each article we publish.