- 1Research Department, Phillip Island Nature Parks, Cowes, VIC, Australia
- 2Departamento de Biología de la Conservación, Estación Biológica de Doñana (Consejo Superior de Investigaciones Científicas), Seville, Spain
- 3Environment Canada, Saskatoon, SK, Canada
Marine top and meso predators like seabirds are limited by the need to breed on land but forage on limited or patchily distributed resources at sea. Constraints imposed by such central-place foraging behavior change during breeding or even disappear outside the breeding period when there is no immediate pressure to return to a central place. However, central place foraging is usually factored as an unchanging condition in life history studies. Here we used little penguin Eudyptula minor, a resident bird with one of the smallest foraging range among seabirds, to examine the different degree of pressure/constraints of being a central-place forager. We combined data on isotopic composition (δ13C and δ15N), conventional stomach contents and body mass of little penguins breeding at Phillip Island, Australia over 9 years (2003–2011). We explored relationships between diet and body mass in each stage of the breeding season (pre-laying, incubation, guard, and post-guard) in years of “high” and “low” reproductive success. Values of δ13C and δ15N as well as isotopic niche width had similar patterns among years, with less variability later in the season when little penguins shorten their foraging range at the expected peak of their central-place foraging limitation. Body mass peaked before laying and hatching in preparation for the energetically demanding periods of egg production and chick provisioning. An increase of anchovy and barracouta in the diet, two major prey for little penguins, occurred at the critical stage of chick rearing. These intra-annual trends could be a response to imposed foraging constraints as reproduction progresses, while inter-annual trends could reflect their ability to match or mismatch the high energy demanding chick rearing period with the peak in availability of high-quality prey such as anchovy. Our findings underline the key advantages of using a stable isotope approach combined with conventional dietary reconstruction to reveal an otherwise intractable ecological issue of different constrains of being a central-place forager, such as the little penguin, which could be applied to other marine species.
Introduction
Energy budgets of animals can vary throughout their annual cycle with higher energy-demands occurring during reproduction (Weimerskirch et al., 1993; Shaffer et al., 2003). In highly dynamic marine systems, marine top, and meso predators such as seabirds have developed an array of behavioral responses to spatiotemporal heterogeneity in food availability (e.g., Tremblay and Cherel, 2003; Chiaradia and Nisbet, 2006; Zimmer et al., 2011). Seabird responses to environmental heterogeneity are likely driven by spatiotemporal changes in prey distribution and therefore availability (Kato et al., 2003; Zimmer et al., 2011) and also by intrinsic individual traits like sex, age, or body condition (Kato et al., 2000; Limmer and Becker, 2009; Zimmer et al., 2011).
Energy budgets of seabirds can vary depending on the stage of their life cycle, which likely influences their foraging distribution and consequently prey selection. Seabirds face an increase in energy and nutritional requirements over the breeding season (Gales and Green, 1990), resulting in increased foraging effort (e.g., Williams and Rothery, 1990; Bethge et al., 1997; Zimmer et al., 2011). As reproduction progresses they could also shift their diets toward high-quality prey types to fulfill the energetic and nutritional requirements to breed (Kowalczyk et al., 2014). Further constraints imposed during the chick-rearing period force parents to reduce the length of their foraging trips, thus reducing foraging ranges and likely access to habitats and food resources (Collins et al., 1999; Kato et al., 2003, 2008).
Most of these adaptations are thought to derive from the fundamental constraint of foraging at sea with a need to return to land for breeding (Bried and Jouventin, 2001; Gaston, 2004) called “central place foraging.” Since the term was coined by Orians and Pearson (1979), most studies have considered central place foraging as an unchanging condition in life history studies. However, the pressure of central-place foraging is expected to change during breeding and could even disappear outside the breeding period when there is no immediate pressure to return to a central breeding place. Our ability to measure the ecological response of seabirds to the different constraints imposed over the life cycle of being a central place forager is crucial to understand the evolution of this behavior.
For central place foraging, the foraging area can be in a two-dimension scale that changes at different stages of breeding (Shealer, 2001). If food depletion occurs, individuals would increase their foraging zone as breeding progresses, as theorized by Ashmole (1971). In practice, however, the “Ashmole's halo” of prey depletion around colonies is not enough to explain foraging behavior of penguins and other deep-diving seabirds as they use a three dimensional foraging zone (Chiaradia et al., 2007; Halsey, 2011; Houston, 2011). Perhaps a better approach to study fluctuations in prey and foraging area of deep-diving seabirds is to understand their diet, which can offer insights into changes in resource use of central place foragers.
The little penguin (Eudyptula minor) is a good model to study changes in foraging strategies and different pressures experienced by central place foragers. It is a resident species, forage in a highly unpredictable and heterogeneous environment (Gales and Pemberton, 1990), have one of the smallest foraging ranges among seabirds (Collins et al., 1999), and so are particularly sensitive to changes in food availability and accessibility near their colonies (Chiaradia et al., 2003; Chiaradia and Nisbet, 2006). Moreover, there is evidence that little penguin body mass also varies significantly in response to variations in breeding onset (Ramírez et al., 2015; Salton et al., 2015) and foraging decisions (Saraux et al., 2011b). Little penguin diet can change dramatically between breeding stages (Chiaradia et al., 2003, 2010, 2012). As result, the events that drive success or failure within each breeding stage is independent of each other (Saraux et al. unpublished). Thus, we expect that constrains of each breeding stage would result in changing conditions of little penguin central place status over the breeding cycle.
Here, we studied penguins from Phillip Island (Australia) where two separated datasets with different timespan were available for the analysis. We combined data from nine seasons of stable isotope values (blood δ13C and δ15N, 2003–2011) with a longer dataset on 17 years of penguin stomach contents (1985–2012 not continuous) to unravel the feeding response of this seabird species to the various constraints imposed by its central-place foraging behavior throughout the entire reproductive cycle. The combination of conventional diet analysis with more recent isotopic techniques has been proposed as the best way to address dietary reconstructions in seabirds (Karnovsky et al., 2008; Ramos et al., 2009; Chiaradia et al., 2012, 2014). We explored trends in isotopic and conventional diet and body mass along the breeding season (prelaying, incubation, guard, and post-guard breeding stages) for years of “high,” “average,” and “low” breeding success, a proxy to food resource availability (Chiaradia and Nisbet, 2006). We expected little Penguins to adjust their feeding strategies at different breeding stages and years of high and low food availability. Despite the fact that both parents typically share costs of reproduction in this species (Chiaradia and Nisbet, 2006; Saraux et al., 2011a), reproductive requirements may differ quantitatively and qualitatively between the sexes (Saraux et al., 2011a). Accordingly, the sex factor was included in our analyses to explore variations in feeding strategies according to gender.
Methods
Study Area and Fieldwork Procedures
We studied little Penguins at the Summerland Peninsula on the western end of Phillip Island, Victoria, Australia (38°15′S, 145°30′E). The study was conducted during nine breeding seasons (from 2003 to 2011, where 2003 refers to the breeding season 2003–2004, etc.). Additional conventional diet dataset from 1985 to 2011 (not continuous) were used (Table 1). The breeding stages, i.e., pre-laying, incubation, chick guard (chicks up to 3 weeks old), and chick post-guard (chicks between 3 and 8 weeks old) were determined during three-times a week visits to the colonies (details in Chiaradia and Kerry, 1999). Penguins were marked individually with transponders (Allflex and Trovan, Australia).
For stable isotope analysis, adult blood samples were collected for each stage of the breeding season (Table 1) and stored in 70% ethanol in a freezer (−18°C) until laboratory analysis (see details in Chiaradia et al., 2010, 2014). Birds were sexed by bill measurements and weighted with spring scale to the nearest 10 g. Stomach contents were sampled by stomach flushing according to Chiaradia et al. (2003, 2010, 2012). Samples were frozen at −18°C for later analysis.
Stable Isotope Analyses
Before stable-isotope analysis, ethanol was removed from the samples by decanting superfluous ethanol, then freeze-drying the balance. Whole blood was freeze-dried, then powdered. Subsamples of powdered materials were weighed to the nearest μg and placed into tin capsules for δ13C and δ15N determinations. Isotopic analyses for blood samples collected during the 2003, 2004, and 2006 breeding seasons were performed at the National Hydrology Research Centre (Environment Canada, Saskatoon, Canada) facilities by means of a Robo-Prep elemental analyzer coupled to a Europa 20:20 continuous-flow isotope ratio mass spectrometer (see details in Chiaradia et al., 2010). The remaining samples were analyzed at the Laboratory of Stable Isotopes of the Estación Biológica de Doñana (LIE-EBD, www.ebd.csic.es/lie/index.html) using a continuous flow isotope-ratio mass spectrometry system (Thermo Electron) consisting of a Flash HT Plus elemental analyzer interfaced with a Delta V Advantage mass spectrometer. Stable isotope ratios are expressed in the standard δ-notation (‰) relative to Vienna Pee Dee Belemnite (δ13C) and atmospheric N2 (δ15N). Based on replicate measurements on within-run standards at the National Hydrology Research Centre (albumen) and the LIE-EBD (queratines) facilities, measurement errors were estimated to be ±0.1 and ±0.2‰ for δ13C and δ15N, respectively.
Parameters Estimation and Statistical Testing
Breeding stages were categorized as pre-laying, incubation, chick guard, and post guard. Breeding success (bs) was measured according to the number of chicks fledged per pair (cpp) per year, grouped in three categories: high (bs > 1.2 cpp), average (1.2 cpp ≥ bs > 0.7 cpp) and low breeding success (bs ≤ 0.7 cpp). Some categories were under-represented in the dataset so we have grouped them to prevent from an unbalanced analysis (Table 1).
In the conventional diet analysis, we used frequency of occurrence (FOO), i.e., the presence or absence of a particular prey for each sampled stomach. Detailed diet results have been published elsewhere (Chiaradia et al., 2003, 2010, 2012). Four main prey were included in the statistical analysis: barracouta (Thyrsites atun), anchovy (Engraulis australis), Gould's squid (Nototodarus gouldi), and pilchard (Sardinops sagax). Each prey species was analyzed as binomial variable (presence/absence) per sex and breeding stage (Table 1). In addition, we used information on stomach contents to calculate the Shannon-Weaver biodiversity indexes (H', Shannon and Weaver, 1949) for each stomach sampled.
Throughout the breeding cycle, we used isotopic data and a Bayesian framework to derive multivariate ellipse-based metrics, i.e., isotopic niches (area of the multivariate ellipses, hereafter referred as SEAB, with unit expressed as ‰2). To explore variations in isotopic niche width and overlap, we used metrics based on standard ellipses to measure niche area and overlap among groups, following methods in SIBER from Jackson et al. (2011) and the R package SIAR from Parnell et al. (2008).
Body mass was recorded when penguins were caught for blood sampling. We used direct body mass instead of calculating body condition (body mass adjusted for body size). We have followed Salton et al. (2015) who suggested that the addition of a linear measure required for calculating body condition has the potential to inflate estimated variance, while body mass alone can be used as measure of an individual's physical condition on little penguins.
Dietary shifts among breeding stages, along with variations in metrics informing on isotopic niche widths were explored by testing for variations among breeding stages in key prey occurrences, raw blood δ13C and δ15N measurements, adult body mass, SEAB estimates provided by 100 posterior draws, and diversity index (H') values. Sex and its interaction with breeding stage were introduced in the models to account for potential differences according to gender, whereas calendar year (factor) and annual breeding success, an index of prey availability (Chiaradia and Nisbet, 2006) were also included to account for potential differences among breeding seasons. We used model selection starting from a prior model assumption, the full model:
FOO of prey and SEAB estimates were analyzed using a Generalized Linear Model (GLM) with binomial and negative binomial family distribution, respectively. In turn, GLMs were used to fit raw isotopic data, body mass, and H' values. Our research questions focused on whether the effect of sex, breeding success, and breeding stage were additive to that for the season, namely whether sex-specific differences in little penguin feeding strategies occurred consistently for all seasons. Accordingly, we used likelihood-ratio and F-tests (for Generalized and General Linear Models, respectively) to establish the significance of the full models by comparing its deviance with that for the null model. For model selection, we used the function dredge in the MuMin R package (Barton, 2013). We selected the best fit model(s) based on the Akaike weights with a sum value ≥ 0.9. Finally, we estimated the relative importance of Akaike weights—RIW (Burnham and Anderson, 2004) of each response variable (j) by the sum of Akaike weights of models where explanatory variable (j) was present. RIW ranges from 0 to 1. A strong explanatory variable has a RIW > 0.9, moderate effect between 0.6 and 0.9, very weak effect between 0.5 and 0.6 and no effect below 0.5. For interactions, a strong effect is >0.7 and moderate >0.5.
We validated all models by checking for homogeneity, over or under dispersion and independence using residual analysis, influential values (outliers), normality histogram (when applicable), and balanced sample size among factors (see Supplementary Material). Generalized and General linear Models were fitted in R (version 3.1.1; R Core Team, 2015). The analyses are full reproducible with all steps of the statistical analysis in the Supplementary Material.
Results
Stable Isotopes
Fitted models for blood δ13C and δ15N values revealed significant differences in the isotopic composition of penguins among the different breeding stages and years (Table 2). The isotope stable values showed a slight and gradual decreasing trend along the breeding period. The variation in values was slightly higher in δ13C values for males whereas no differences were found in δ15N values according to gender (Tables 2, 3, Figure 1, Supplementary Material).
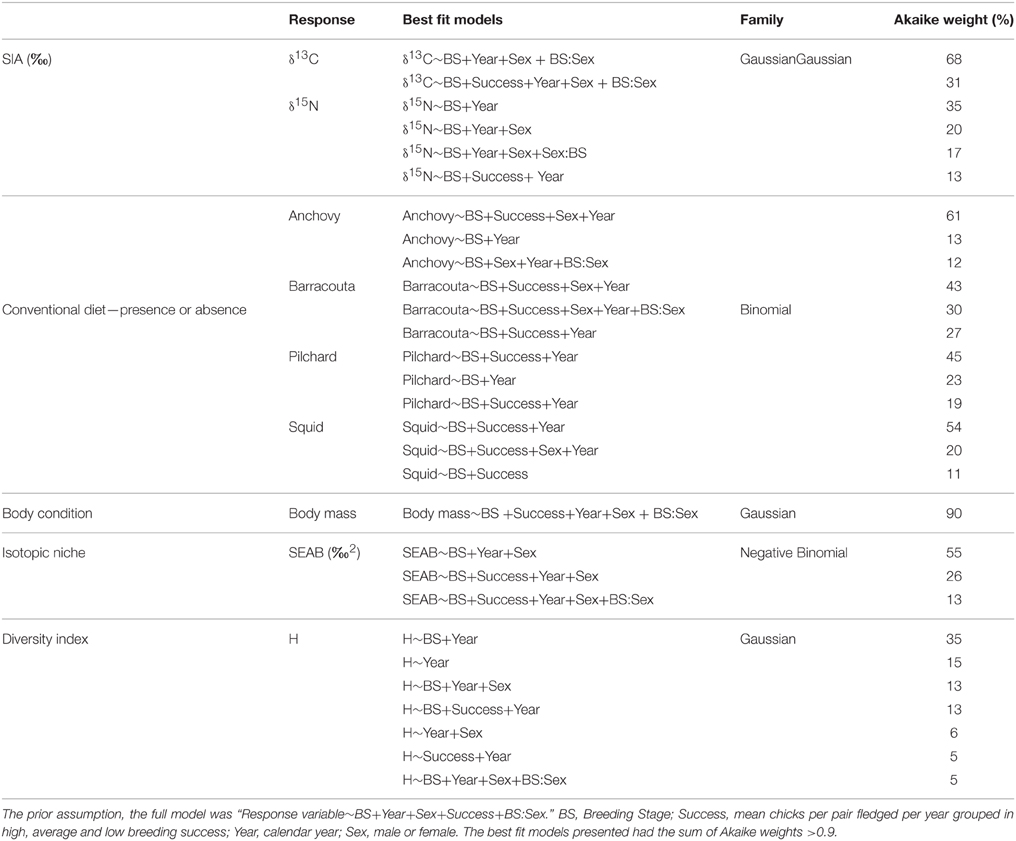
Table 2. Model selection using response variables from stable isotope analysis (δ13C, δ15N), conventional diet from stomach contents, body condition, isotopic niche analysis, and diversity index.
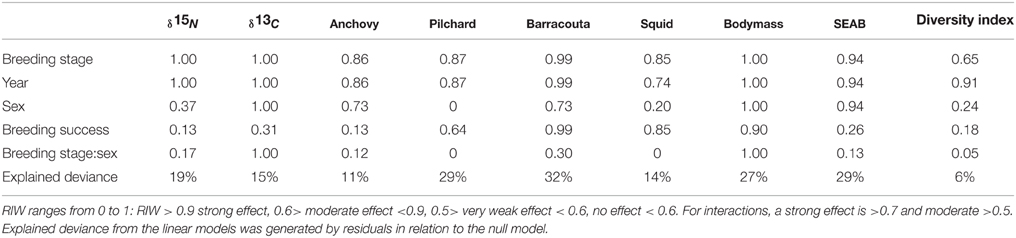
Table 3. Estimates of Relative Importance of Weights (RIW) for each explanatory variable (j) calculated by the sum of Akaike weights of models where explanatory variable (j) was present.
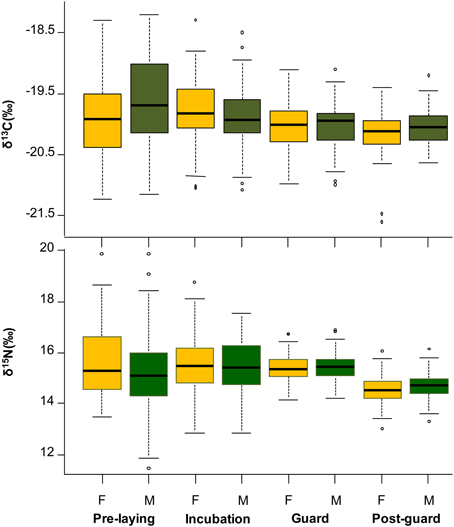
Figure 1. Blood isotopic composition (δ13C and δ15N, ‰) for little penguins sampling during the 2003–2011 period. Isotopic values are summarized by sex and breeding stage.
Conventional Diet
Little penguins mainly foraged on fish, with the relative contribution of fish in the penguins' diet averaging 73% (±SD 0.15%) over the study period (Table 1) regardless of the sex and breeding stage considered (Figure 2). Estimated relative prey abundances indicated that anchovy (19 ± 0.3%, mean ± SD), barracouta (17 ± 0.3%), and pilchard (8 ± 0.2%) were the main fish species included in penguins' diet. In addition to fish species, squid (N. gouldi, 15 ± 0.3%) also showed a relevant contribution to penguin diet all through the breeding cycle. Fitted models for key prey occurrences (anchovy, barracouta, pilchard, and squid) strongly suggested a significant dietary shift among the different breeding stages and years (Table 2, Supplementary Material). The effect of both covariates, estimated by their Akaike weights were moderate to strong (Table 3). In addition, sex showed a moderate effect for anchovy and barracouta while breeding success had a moderate to strong effect for barracouta, pilchard, and squid (Table 3). Barracouta provided the most parsimonious model, explaining 32% of the deviance (Tables 2, 3, Supplementary Material). There was no interaction effect of the breeding stage and sex (Table 3) for any of prey items. Observed dietary shifts, which mainly consisted of a gradual increase in the occurrence of anchovy and barracouta were therefore similar for both sexes (see Table 2 and Figure 3, Supplementary Material).
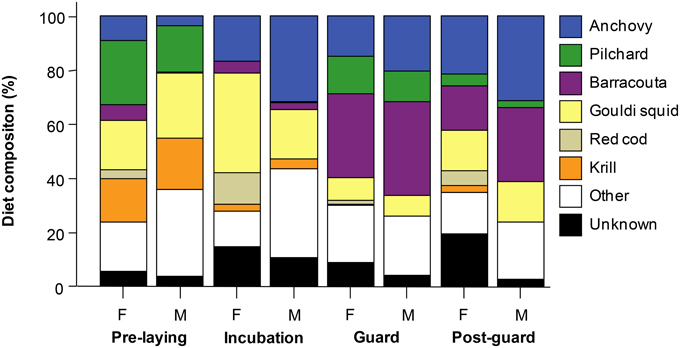
Figure 2. Mean relative prey abundance (combining data on stomach contents for the 1995–2012 not continuously—see Table 1) in the diet of little penguins split by sex and breeding stage. Prey types with relative abundances < 3% have been grouped within “Other” category.
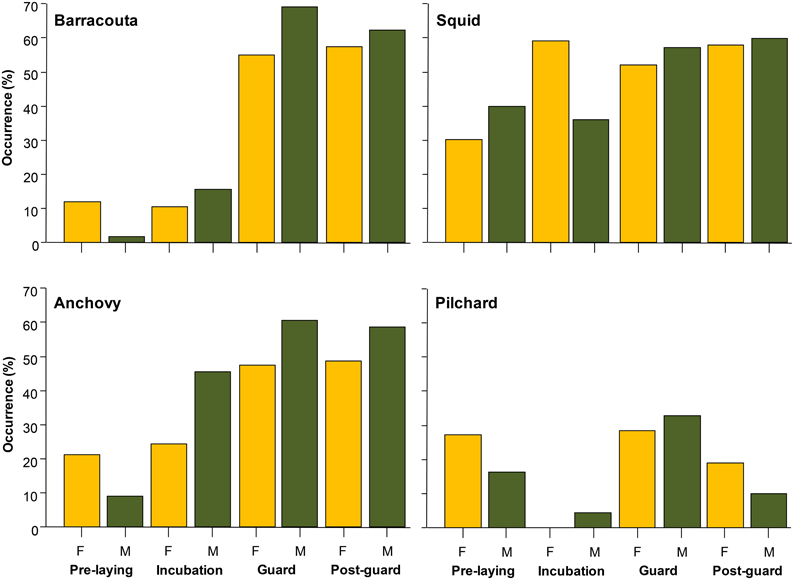
Figure 3. Relative occurrences (%) of key prey types (most important prey species in terms of biomass within stomach contents, i.e., barracouta, squid, anchovy, and pilchard; see Figure 2) split per sex and breeding stage. Relative occurrences were estimated from stomach contents collected during the 1995–2012 (not continuously see Table 1).
Body Mass
The full model with interaction (Breeding Stage:Sex) was the most parsimonious fit for body mass (Table 2, Supplementary Material). Although it is difficult to discern which variables had more relative importance (Table 3) in the 27% explained deviance of this full model, it highlights how body mass varies among all covariates. Males were always heavier than females (Figure 4), except at pre-laying when there was no difference between sexes (Figure 4), in agreement with Ramírez et al. (2015) who used a much large body mass dataset.
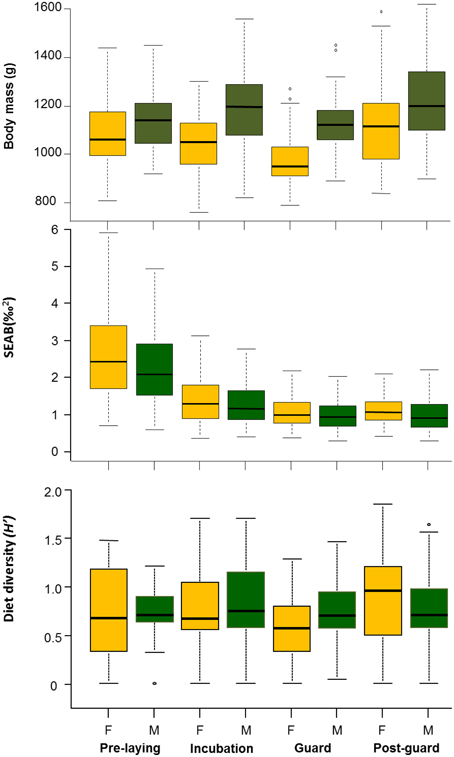
Figure 4. Body mass from birds sample for blood and niche analysis [SEAB (‰2)] estimated from 2003 to 2011 stable isotope data (δ13C and δ15N, ‰). Diversity (H′) values are from conventional data from 1985 to 2012 (not continuously see Table 1). Values are summarized by sex and breeding stage.
Niche Analysis
The isotopic niche widths (SEAB) varied among breeding stages, year, and sex, being clearly narrower during the chick rearing period (Figure 4). Although the significant effect of the sex factor pointed to slightly but consistent wider isotopic niches for females, the absence of a significant interaction with the breeding stage suggested that the observed pattern was similar for both sexes (Tables 2, 3, Figure 4, Supplementary Material).
Diversity Index
The diet diversity from derived H' values varied, with a moderate effect along the breeding period, regardless of the sex but with strong effect between years (Tables 2, 3, Figure 4), although the total explained deviance was low (6%, Table 3).
Discussion
This long-term study revealed shifts in the diet, body mass and isotopic niche changes of an inshore resident top predator—the little penguin. Our insights could only be derived using stable isotopes as ideal and relevant indicators of penguin feeding ecology throughout time. Dietary variability was strongly influenced by the stages of breeding, likely associated with the different requirements that must be met during their breeding cycle, ultimately reflecting different restrictions imposed by their central-place foraging behavior. Variation in the annual breeding success (index of prey availability, Chiaradia and Nisbet, 2006) and sex difference had a lesser effect. Whether or not the observed changes would be the result of (i) active decisions directed to face the higher energy and nutritional requirements associated to the chick rearing period or (ii) passive consequences of the ability of penguins to couple their reproduction to marine productivity patterns, our findings highlight that constraints imposed on central-place foraging is not an unchanging condition in the life history of this resident seabird.
On What and Where Penguins are Feeding While Breeding
Little penguin foraging ranges vary throughout the breeding cycle, with individuals feeding over broader areas during the first stages of the reproductive cycle (Chiaradia et al., 2012). In particular, foraging ranges during the non-breeding period and early in the reproductive cycle typically include Port Phillip Bay (a large bay of 1930 km2 in area with waters no deeper than 14 m and about 70 Km away from Phillip Island; Collins et al., 1999; McCutcheon et al., 2011; Chiaradia et al., 2012). As the breeding season progresses, the degree of linkage between parents and their nests increases, thus reducing foraging trip distances (Collins et al., 1999) and forcing individuals to feed over the deeper open Bass Strait waters surrounding the Summerland Peninsula (Chiaradia et al., 2007, 2012). Differences in isotopic baselines for the contrastingly different foraging areas exploited by individuals throughout the reproductive cycle (see Hobson et al., 1994, for isotopic differences between inshore and offshore marine systems), could explain the consistent annual trends in δ13C values for little penguins. Further, our derived estimates for isotopic niches supported the consistency of this general intra-annual trend. In particular, the narrower isotopic niches for later breeding stages, along with the constant values for estimated biodiversity indexes (H') from stomach contents, point to changes in foraging ranges (and not to decreasing diversity in dietary intakes) as the most likely explanation for the consistent decreasing patterns in isotopic variability throughout the reproductive cycle.
Our results from nine breeding seasons agreed with previous studies at this colony showing a consistent increase throughout the breeding cycle in the occurrence of anchovies within the stomach contents of little penguins (Chiaradia et al., 2012). We also detected a consistent increase in barracouta occurrences that became a major prey type during guard and post-guard stages of the chick rearing period. The higher occurrences of these isotopically enriched prey types (Chiaradia et al., 2012) in the stomach contents of little penguins contrasted with the observed decrease in their blood δ15N values as reproduction progress. However, this apparent mismatch could be explained by a diet segregation between parents and their chicks (Chiaradia et al., 2014). Indeed, the blood isotopic values for adult penguins, along with information derived from stomach contents from the pre-laying and incubation periods, likely informed on the diet of parents, whereas stomach contents from the chick rearing reported on what parents were provisioning to their chicks as previously reported for little penguins (Chiaradia et al., 2010, 2012).
Nutritional and energy requirements often differ between adults and their progeny (Murphy, 1996) as parents may adjust their feeding strategies to simultaneously cope with requirements of reproduction and self-maintenance (Saraux et al., 2011b). Little penguins may attempt to maximize the rate of energy provisioning to their offspring by selecting high-quality, isotopically enriched prey items, such as anchovy or barracouta, to feed their young (see Orians and Pearson, 1979; Chiaradia et al., 2010; Saraux et al., 2011b). Similar to what occurs in many other seabirds (e.g., Weimerskirch et al., 1993; Chaurand and Weimerskirch, 1994; Shaffer et al., 2003), little penguins rearing chicks are known to alternate between short and long foraging trips for regular provisioning of chicks and rebuilding of their own reserves (Saraux et al., 2011b). Owing to the spatial heterogeneity in marine productivity patterns in this area (Hobday, 1992), and the fact that trip duration and distance traveled are highly correlated in this species (Collins et al., 1999), food may also come from different prey patches when parents are foraging for their young or for themselves. This highlights the advantages of using a compound-specific stable isotope approach to reconstructing diets of marine animals as the key advantage here would be the ability to control for varying isotopic baselines through the measurement of source (e.g., phenylalanine) and trophic (glutamic acid) tracers (e.g., Karnovsky et al., 2008; Seminoff et al., 2012).
Proactive Decisions vs. Passive Consequences
Consistent dietary shifts incurred by penguins during reproduction could be the consequence of proactive decisions due to the increasing energy and nutritional requirements associated with chick-rearing. In other words, adult penguins may cope with the higher energy requirements related to the chick-rearing period by deliberately shifting their diets toward high-quality prey types. Such decisions could be taken by intentionally searching for these food resources or by selecting occasionally encountered high-quality prey items for provisioning their chicks. Given that these obligate swimmers are restricted in their ability to cover large distances when foraging (Collins et al., 1999; Chiaradia et al., 2007; McCutcheon et al., 2011), it is unlikely that individuals have been able to successfully implement the strategy of intentionally searching for such unpredictable food resources. Indeed, little penguins are widely considered as generalist and opportunistic predators, with diet compositions typically reflecting food resource availability and variations in the marine community composition (Chiaradia et al., 2003); an argument against individuals intentionally shifting their diets according to reproductive requirements. The clear segregation between foraging trips to meet parent and chick needs makes therefore unfeasible the hypothesis of selecting high-quality, occasionally encountered prey items for provisioning the chicks.
In contrast, it may be possible that penguins were able to match time of breeding to marine productivity patterns (Afán et al., 2015). As expected for a generalist/opportunistic predator, observed changes in diet composition with a progressing season could be “simply” a passive reflection of changes in the availability of the main prey types. Indeed, reproduction takes place at the most favorable time of the year, and the onset of breeding is often dependent upon food availability (Meijer and Drent, 1999; Afán et al., 2015). Among the different breeding stages, chick rearing is a crucial phase for seabirds, in terms of reproductive requirements, as parents have to frequently return to their nest to provide their offspring with an adequate supply of food in order to be successful (Takahashi et al., 2003). In breeding little penguins, this constraint is even further aggravated because birds rely on the food supply directly in the vicinity of the colony and may not be able to undergo long trips in order to target more profitable distant prey patches a they can do during the pre-laying and incubation periods (Kato et al., 2008). Thus, penguins may couple the high demanding phase of chick rearing with periods of increasing availability and accessibility of penguins main prey types (e.g., anchovies and barracoutas, Figure 3).
Little penguins typically lay at the end of October (average laying date for the 2000–2011 time period was October the 20th). However, mean laying date may range from early September (September the 7th in 2002) to mid-November (November the 17th in 2007). Age and individual quality can influence the onset of reproduction (Chiaradia and Nisbet, 2006; Nisbet and Dann, 2009). But at population level, little penguins respond to inter-annual variations in relevant oceanographic features and marine productivity patterns, probably driving patterns in the availability and distribution of prey (Afán et al., 2015). Very little information is available on the phenology of barracouta. However, and given that this species grows quickly (reaching 23–30 cm in the first year, Kailola et al., 1993), we can deduce little penguins are feeding on very young individuals (fish consumed range from 3 to 5 cm long Cullen et al., 1992; Chiaradia et al., 2003). Similarly, it is post-larval anchovy that are eaten at the times of year when this species is most frequent in the diet of penguins (Montague and Cullen, 1988). Anchovy typically spawns from November to April and the resulting post-larval fish form shoals from early January to July (Montague and Cullen, 1988; Collins et al., 1999), thus matching with little penguin chick rearing. Fluctuations in anchovy stocks have been attributed to changes in water temperature (Hoedt et al., 1996). So it is possible that similar ecological mechanisms are forcing the breeding dynamics of the little penguin colony at Phillip Island (Afán et al., 2015). Indeed, the unexplained deviance of our models could well be due to influence of environment factors, which was beyond of the purpose of this paper.
Conclusions
Central place foraging occurs when individuals have to return to a central place/location to mate, incubate or feed their offspring. During each of these stages of the life cycle, birds are placed under differing degrees of pressure/constraints through their central-place foraging behavior on a continuum ranging from no pressure (pre-breeding) to high pressure (incubation and chick rearing) to return to a central place (Kato et al., 2008; Pelletier et al., 2014). Our results illustrated this continuum and plastic change in the degree of central place foraging pressure. By combining methods from independent sources on stomach contents and stable isotope analysis from blood, we could determine the dietary shifts throughout the breeding cycle, a pattern repeated over several years of this study. However, stable isotope analysis has allowed us to further trace consistent changes in the feeding ecology of little penguins. In particular, the isotopic niche analysis informed on the spatiotemporal component of the foraging ecology of deep-diving birds like little penguins. Importantly, our work underlines the key advantages of using stable isotope approaches combined with conventional dietary reconstructions to investigate an otherwise intractable ecological question.
Author Contributions
Design—KH, MF, AC, FR, Data collection—AC, Lab analysis—KH, MF, AC, Data analysis—FR, AC, Writing up—FR, AC. All authors contributed equally on the review of several versions.
Conflict of Interest Statement
The authors declare that the research was conducted in the absence of any commercial or financial relationships that could be construed as a potential conflict of interest.
Acknowledgments
We are thankful for several grants received to support this collaborative research: Penguin Foundation, Junta Andalucía, European Union Research Fund and Ministerio de Educación y Ciencia de España. We also thank the Phillip Island Nature Parks for their continued support, in particular Peter Dann, Marcus Salton, Leanne Renwick. Paula Wasiak and all people who helped in the field. The project was approved by the Phillip Island Nature Park Animal Experimentation Ethics Committee and by the Department of Sustainability and Environment of Victoria, Australia. Thanks to Ricardo Álvarez for his help during stable isotope analysis.
Supplementary Material
The Supplementary Material for this article can be found online at: http://journal.frontiersin.org/article/10.3389/fevo.2015.00154
References
Afán, I., Chiaradia, A., Forero, M. G., Dann, P., and Ramírez, F. (2015). A novel spatio-temporal scale based on ocean currents unravels environmental drivers of reproductive timing in a marine predator. Proc. R. Soc. B 282:20150721. doi: 10.1098/rspb.2015.0721
Ashmole, N. P. (1971). “Sea bird ecology and the marine environment,” in Avian Biology, Vol. 1., eds D. S. Farner and J. R. King (London: Academic Press), 223–286.
Barton, K. (2013). MuMIn: Multi-Model Inference, R Package Version 1.9.13. Available online at: http://CRAN.R-project.org/package=MuMIn
Bethge, P., Nicol, S., Culik, B. M., and Wilson, R. P. (1997). Diving behaviour and energetics in breeding little penguins (Eudyptula minor). J. Zool. 242, 483–502. doi: 10.1111/j.1469-7998.1997.tb03851.x
Bried, J., and Jouventin, P. (2001). “Site and mate choice in seabirds: an evolutionary approach,” in Biology of Marine Birds, eds E. A. Schreiber and J. Burger (Boca Raton, FL: CRC Press), 263–305.
Burnham, K. P., and Anderson, D. R. (2004). Multimodel inference: understanding AIC and BIC in model selection. Sociol. Methods Res. 33, 261–304. doi: 10.1177/0049124104268644
Chaurand, T., and Weimerskirch, H. (1994). The regular alternation of short and long foraging trips in the blue petrel halobaena caerulea: a previously undescribed strategy of food provisioning in a pelagic seabird. J. Anim. Ecol. 63, 275–282. doi: 10.2307/5546
Chiaradia, A., Costalunga, A., and Kerry, K. R. (2003). The diet of little penguins Eudyptula minor at phillip island, victoria, following the 1995 mass mortality of one of their main prey, the pilchard Sardinops sagax. Emu 103, 43–48. doi: 10.1071/MU02020
Chiaradia, A., Forero, M. G., Hobson, K. A., and Cullen, J. M. (2010). Changes in diet and trophic position of a top predator 10 years after a mass mortality of a key prey. ICES J. Mar. Sci. 67, 1720. doi: 10.1093/icesjms/fsq067
Chiaradia, A., Forero, M. G., Hobson, K. A., Swearer, S. E., Hume, F., Renwick, L., et al. (2012). Diet segregation between two colonies of little penguins Eudyptula minor in southeast australia. Austral Ecol. 37, 610–619. doi: 10.1111/j.1442-9993.2011.02323.x
Chiaradia, A., Forero, M. G., McInnes, J. C., and Ramírez, F. (2014). Searching for the true diet of marine predators: incorporating bayesian priors into stable isotope mixing models. PLoS ONE 9:e92665. doi: 10.1371/journal.pone.0092665
Chiaradia, A. F., and Kerry, K. R. (1999). Daily nest attendance and breeding performance in the little penguin Eudyptula minor at phillip island, Australia. Mar. Ornithol. 27, 13–20.
Chiaradia, A., and Nisbet, I. C. T. (2006). Plasticity in parental provisioning and chick growth in little penguins in years of high and low breeding success. Ardea 94, 257–270.
Chiaradia, A., Ropert-Coudert, Y., Kato, A., Mattern, T., and Yorke, J. (2007). Diving behaviour of little penguins from four colonies across their whole distribution range: bathymetry affecting diving effort and fledging success. Mar. Biol. 151, 1535–1542. doi: 10.1007/s00227-006-0593-9
Collins, M., Cullen, J. M., and Dann, P. (1999). Seasonal and annual foraging movements of little penguins from phillip island, victoria. Wildl. Res. 26, 705–721. doi: 10.1071/WR98003
Cullen, J. M., Montague, T. L., and Hull, C. (1992). Food of little penguins Eudyptula minor in victoria: comparison of three localities between 1985 and 1988. Emu 91, 318–341. doi: 10.1071/MU9910318
Gales, R., and Green, B. (1990). The annual energetics cycle of little penguins. Ecology 71, 2297–2312. doi: 10.2307/1938641
Gales, R. P., and Pemberton, D. (1990). Seasonal and local variation in the diet of the little penguin, Eudyptula minor, in Tasmania. Aust. J. Wildl. Res. 17, 231–259. doi: 10.1071/WR9900231
Halsey, L. G. (2011). Optimal diving models: their development and critique requires accurate physiological understanding. Trends Ecol. Evol. 26, 437–438. doi: 10.1016/j.tree.2011.05.001
Hobday, D. K. (1992). Abundance and distribution of pilchard and Australian anchovy as prey species for the little penguin Eudyptula minor at Phillip Island, Victoria. Emu 91, 342–354. doi: 10.1071/MU9910342
Hobson, K. A., Piatt, J. F., and Pitocchelli, J. (1994). Using stable isotopes to determine seabird trophic relationships. J. Anim. Ecol. 63, 786–798. doi: 10.2307/5256
Hoedt, F. E., Jones, K., Jackson, G., and Dimmlich, W. F. (1996). “Studies on the relationship between sea surface temperature and the distribution of pilchards (Sarinops sagax neopilchardus steindachner) in gulf and shelf waters of south australia,” in Proceedings of the Ocean and atmosphere Pacific International Conference, ed T. H. Aung (Adelaide, SA: National Tidal Facility, The Flinders Univeristy of South Australia), 103–108.
Houston, A. I. (2011). Assessing models of optimal diving. Trends Ecol. Evol. 26, 292–297. doi: 10.1016/j.tree.2011.03.003
Jackson, A. L., Inger, R., Parnell, A. C., and Bearhop, S. (2011). Comparing isotopic niche widths among and within communities: SIBER – stable isotope bayesian ellipses in R. J. Anim. Ecol. 80, 595–602. doi: 10.1111/j.1365-2656.2011.01806.x
Kailola, P. J., Williams, M. J., Stewart, P. C., Reichelt, R. E., McNee, A., and Grieve, C. (1993). Australian Fisheries Resources. Canberra, ACT: Fisheries Research and Development Corporation.
Karnovsky, N. J., Hobson, K. A., Iverson, S., and Hunt, G. L. (2008). Seasonal changes in diets of seabirds in the north water polynya: a multiple-indicator approach. Mar. Ecol. Prog. Ser. 357, 291–299. doi: 10.3354/meps07295
Kato, A., Ropert-Coudert, Y., and Chiaradia, A. (2008). Regulation of trip duration by an inshore forager, the little penguin (Eudyptula minor), during incubation. Auk 125, 588–593. doi: 10.1525/auk.2008.06273
Kato, A., Watanuki, Y., and Naito, Y. (2003). Annual and seasonal changes in foraging site and diving behavior in adélie penguins. Polar Biol. 26, 389–395. doi: 10.1007/s00300-003-0493-0
Kato, A., Watanuki, Y., Nishiumi, I., Kuroki, M., Shaughnessy, P., and Naito, Y. (2000). Variation in foraging and parental behavior of king cormorants. Auk 117, 718–730. doi: 10.1642/0004-8038(2000)117[0718:VIFAPB]2.0.CO;2
Kowalczyk, N. D., Chiaradia, A., Preston, T. J., and Reina, R. D. (2014). Linking dietary shifts and reproductive failure in seabirds: a stable isotope approach. Funct. Ecol. 28, 755–765. doi: 10.1111/1365-2435.12216
Limmer, B., and Becker, P. H. (2009). Improvement in chick provisioning with parental experience in a seabird. Anim. Behav. 77, 1095–1101. doi: 10.1016/j.anbehav.2009.01.015
McCutcheon, C., Dann, P., Salton, M., Renwick, L., Hoskins, A. J., Gormley, A. M., et al. (2011). The foraging range of little penguins (Eudyptula minor) during winter. Emu 111, 321–329. doi: 10.1071/MU10078
Meijer, T., and Drent, R. (1999). Re-examination of the capital and income dichotomy in breeding birds. Ibis 141, 399–414. doi: 10.1111/j.1474-919X.1999.tb04409.x
Montague, T. L., and Cullen, J. M. (1988). The diet of little penguin Eudyptula minor at phillip island, victoria. Emu 88, 138–149. doi: 10.1071/MU9880138
Murphy, M. E. (1996). “Nutrition and metabolism,” in Avian Energetics and Nutritional Ecology, ed C. Carey (New York, NY: Chapman and Hall), 31–60.
Nisbet, I. C. T., and Dann, P. (2009). Reproductive performance of little penguins in relation to year, age, pair-bond duration, breeding date and individual quality. J. Avian Biol. 40, 296–308. doi: 10.1111/j.1600-048X.2008.04563.x
Orians, G. H., and Pearson, N. E. (1979). “On the theory of central place foraging,” eds D. J. Horn, B. R. Stairs, and R. D. Mitchell (Columbus, OH: Ohio State University Press), 155–177.
Parnell, A., Inger, R., Bearhop, S., and Jackson, A. L. (2008). Stable Isotope Analysis in R (SIAR) Available online at: http://cran.r-project.org/web/packages/siar/index.html
Pelletier, L., Chiaradia, A., Kato, A., and Ropert-Coudert, Y. (2014). Fine-scale spatial age segregation in the limited foraging area of an inshore seabird species, the little penguin. Oecologia 176, 399–408. doi: 10.1007/s00442-014-3018-3
Ramírez, F., Forero, M. G., Hobson, K. A., and Chiaradia, A. (2015). Older female little penguins Eudyptula minor adjust nutrient allocations to both eggs. J. Exp. Mar. Biol. Ecol. 468, 91–96. doi: 10.1016/j.jembe.2015.03.020
Ramos, R., Ramírez, F., Sanpera, C., Jover, L., and Ruiz, X. (2009). Feeding ecology of yellow-legged gulls larus michahellis in the western mediterranean: a comparative assessment using conventional and isotopic methods. Mar. Ecol. Prog. Ser. 377, 289–297. doi: 10.3354/meps07792
R Core Team (2015). R: A Language and Environment for Statistical Computing, Version 3.1.1. Available online at: http://www.R-project.org
Salton, M., Saraux, C., Dann, P., and Chiaradia, A. (2015). Carry-over body mass effect from winter to breeding in a resident seabird, the little penguin. R. Soc. Open Sci. 2:140390. doi: 10.1098/rsos.140390
Saraux, C. A., Chiaradia, A., Maho, Y. L., and Ropert-Coudert, Y. (2011a). Everybody needs somebody: Unequal parental effort in little penguins. Behav. Ecol. 22, 837–845. doi: 10.1093/beheco/arr049
Saraux, C. A., Robinson-Laverick, Y., Maho, Y. L., Ropert-Coudert, Y., and Chiaradia, A. (2011b). Plasticity in foraging strategies of inshore birds: how little penguins maintain body reserves while feeding the chicks. Ecology 92, 1909–1916. doi: 10.1890/11-0407.1
Seminoff, J. A., Benson, S. R., Arthur, K. E., Eguchi, T., Dutton, P. H., Tapilatu, R. F., et al. (2012). Stable isotope tracking of endangered sea turtles: validation with satellite telemetry and δ15N analysis of amino acids. PLoS ONE 7:e37403. doi: 10.1371/journal.pone.0037403
Shaffer, S. A., Costa, D. P., and Weimerskirch, H. (2003). Foraging effort in relation to the constraints of reproduction in free-living albatross. Funct. Ecol. 17, 66–74. doi: 10.1046/j.1365-2435.2003.00705.x
Shannon, C. E., and Weaver, W. (1949). The Mathematical Theory of Communication. Urbana, IL: University of Illinois Press.
Shealer, D. A. (2001). “Foraging behavior and food of seabirds,” in Biology of Marine Birds, eds E. A. Schreiber and J. Burger (Boca Raton, FL: CRC Press), 137–177.
Takahashi, A., Watanuki, Y., Sato, K., Kato, A., Arai, N., Nishikawa, J., et al. (2003). Parental foraging effort and offspring growth in adélie penguins: does working hard improve reproductive success? Funct. Ecol. 17, 590–597. doi: 10.1046/j.1365-2435.2003.00772.x
Tremblay, Y., and Cherel, Y. (2003). Geographic variation in the foraging behaviour, diet and chick growth of rockhopper penguins. Mar. Ecol. Prog. Ser. 251, 279–297. doi: 10.3354/meps251279
Weimerskirch, H., Salamolard, M., Sarrazin, F., and Jouventin, P. (1993). Foraging strategy of wandering albatrosses through the breeding season: a study using satellite telemetry. Auk 110, 325–342.
Williams, T. D., and Rothery, P. (1990). Factors affecting variation in foraging and activity patterns of gentoo penguins (Pygoscelis papua) during the breeding season at bird island, south georgia. J. Appl. Ecol. 27, 1042–1054. doi: 10.2307/2404394
Keywords: carbon-13, central-place forager, little penguin, Eudyptula minor, nitrogen-15, stable isotope, stomach content, body mass
Citation: Chiaradia A, Ramírez F, Forero MG and Hobson KA (2016) Stable Isotopes (δ13C, δ15N) Combined with Conventional Dietary Approaches Reveal Plasticity in Central-Place Foraging Behavior of Little Penguins Eudyptula minor. Front. Ecol. Evol. 3:154. doi: 10.3389/fevo.2015.00154
Received: 28 October 2015; Accepted: 22 December 2015;
Published: 11 January 2016.
Edited by:
Richard Inger, University of Exeter, UKReviewed by:
Jaime Resano Mayor, University of Bern, SwitzerlandRichard Brian Sherley, University of Exeter, UK
Copyright © 2016 Chiaradia, Ramírez, Forero and Hobson. This is an open-access article distributed under the terms of the Creative Commons Attribution License (CC BY). The use, distribution or reproduction in other forums is permitted, provided the original author(s) or licensor are credited and that the original publication in this journal is cited, in accordance with accepted academic practice. No use, distribution or reproduction is permitted which does not comply with these terms.
*Correspondence: André Chiaradia, achiaradia@penguins.org.au