- 1Department of Environmental Sciences, University of Helsinki, Helsinki, Finland
- 2Nanjing Institute of Geography and Limnology, Chinese Academy of Sciences, Nanjing, China
- 3Department of Bioscience, Aarhus University, Århus, Denmark
- 4Geological Survey of Finland, Kuopio, Finland
- 5Department of Biology, University of Turku, Turku, Finland
- 6Department of Marine Geology and Glaciology, Geological Survey of Denmark and Greenland, Copenhagen, Denmark
- 7Pyhäjärvi Institute, Kauttua, Finland
The concern for the state of global freshwater reservoirs has increased due to deterioration of the water quality during the last decades. This has prompted monitoring and restoration efforts such as the European Water Framework Directive and the national-scale 2nd-investigation and monitoring of the water quality, water volume and biota resources in China. The challenge so far has been the determination of the “natural” state (reference conditions) of freshwater ecosystems. We used the sediment archives of five lakes and one brackish water embayment in Finland and China to assess the impact of selected variables of climatology, hydrology, nutrients, and changes in human population on these ecosystems during the last few centuries. The study sites represent catchment areas with varying land use. Despite the long distance between the sites and their different land-use characteristics, the direction and timing of changes during the last few centuries are well comparable between the high latitudes of Finland and the mid-low latitudes of China. This study reinforces the sensitivity of aquatic ecosystems to environmental change and underlines the usefulness of the palaeolimnological approach as a tool for determining reference conditions.
Introduction
Water is essential to all life forms and thus its availability and quality are of first order importance. Although it is estimated that freshwaters cover only slightly over 3% of the earth's surface area, the estimated number of lakes is ca. 304 million (Downing et al., 2006). Freshwater lakes are of great value in water storage, flood prevention, and maintenance of biodiversity, in addition to which they are vital resources for settlement pattern, food production, recreation, and tourism (Henriksen et al., 1997). Lakes are dynamic systems that can vary on different time scales (e.g., inter-annual to millennial) due to autogenic processes and natural exogenic stress (e.g., Deevey, 1984). Anthropogenic impact has increased the magnitude and speed of change in freshwater ecosystems deteriorating the water quality drastically. Although humans have affected water quality of lakes for thousands of years (e.g., Renberg, 1990; Bradshaw et al., 2006; Räsänen et al., 2006; Guilizzoni et al., 2011), the most distinctive and intensive changes have happened since ca. 1850 after the period of major industrialization and increased agricultural activities (e.g., Bennion et al., 2004; Battarbee et al., 2011) and at ca. 1950 after the intensified use of artificial fertilizers (e.g., Räsänen et al., 2006). Moreover, it is expected that the growth of global population and the predicted climate warming will increase the pressure on lakes and their ecological state (Millennium Ecosystem Assessment, 2005). These changes will further challenge the protection of aquatic ecosystems and their use for ecosystem services (e.g., Moss et al., 2011; Jeppesen et al., 2012, 2014).
During the last decades, the concern for the state of global freshwater reservoirs has increased significantly resulting in Europe in the Water Framework Directive (WFD), a legislation to secure a good water quality for both surface and groundwater by the year 2015 (European Union, 2000). One of the key issues of the WFD has been the determination of “reference,” “pristine,” or “baseline” conditions for aquatic ecosystems against which the effects of anthropogenic activities can be measured to achieve the good ecological status required (e.g., European Union, 2000; Bennion and Battarbee, 2007; Bennion et al., 2011). A comparable action has also been undertaken in China, where a national-scale 2nd-investigation and monitoring of the water quality, water volume and biota resources was launched in 2002 including 2693 Chinese lakes within a framework of the Chinese Ministry of Science and Technology (Yang et al., 2010). The aim of this program is to understand the status of Chinese lakes and to improve the Chinese lake database. However, since aquatic ecosystems have been influenced by various anthropogenic pressures for already thousands of years (e.g., Battarbee and Bennion, 2011; Bouleau and Pont, 2015), the defining of the “reference state” can be very challenging. A number of approaches can be used to estimate “reference” conditions including, e.g., expert judgment, modeling, historical data or spatial surveys (Anderson et al., 1995; Bennion et al., 2011). According to the WFD, in the absence of long-term monitoring data, “reference” conditions can be derived using the palaeolimnological approach (Pollard and Huxham, 1998; European Union, 2000).
Palaeolimnological methods offer a powerful tool to reconstruct past environmental conditions and to provide information of the timing, magnitude, rate and direction of ecological change using sediment records (e.g., Smol, 2008). Although the palaeolimnological approach has been used extensively in the context of WFD (Bennion and Battarbee, 2007) it is still too infrequently used in aquatic and wildlife management practices (Saulnier-Talbot, 2015). A wide variety of biological proxies, palaeobioindicators, exist, including the physical remains of biota (e.g., diatoms, dinoflagellate cysts, cladoceran, chironomids, and plant macrofossils) and biochemical markers (e.g., plant pigments and lipids). Diatoms (Bacillariophyceae) are probably the most commonly used group in palaeolimnological analyses (Moser et al., 1996) as they can be identified to species level, they are usually present in diverse, numerically abundant assemblages (Charles et al., 1994), they have a short life cycle reacting fast to changes in their environment, and many species have narrow optima along different environmental gradients (Dixit et al., 1992). Thus, diatoms provide a wealth of environmental information of the environments they live in. They have proven to be a highly useful proxy for research questions such as presented in our study.
In this study we used the sediment archives of five lakes and one brackish water embayment in Finland and China (Figure 1) representing catchment areas with varying land use (forests, agriculture, and urban area) to assess the impact of selected variables of climatology (air temperature, precipitation), hydrology (lake-level), nutrient concentrations (total phosphorus, total nitrogen), chlorophyll-a, and changes in population on these ecosystems during the last few centuries. The sites comprise large environmental gradients spanning from the Chinese lowland temperate zone to the Finnish northern-boreal zone and from almost pristine catchments to heavily urbanized catchments with 40 million inhabitants (Lake Taihu, Qin et al., 2010). However, we hypothesize that regardless of the geographical location, the impact of similar catchment changes is comparable in the initial timing of lake change, but differs in magnitude and speed. We also hypothesize that the impact of an urban catchment on lake ecosystems is more severe and faster than that of agricultural catchments, the latter being more gradual and long-lasting, and that the impact of global climate warming is stronger at higher latitudes. The main questions to be answered by our study are (1) Are there any detectable changes in the aquatic ecosystems during the last ca. 150 years? If changes can be detected, what was the timing, magnitude, direction and speed of change? (2) Are the possible impacts of changes in agricultural practize, urban pressure and climate change on aquatic ecosystems comparable in Finland and China? (3) Is palaeolimnology well suited for defining “reference” conditions for aquatic ecosystems regardless of the geographical and environmental settings?
Excluding Jerisjärvi and partly Pyhäjärvi, the diatom assemblage data and sediment dates from all other sites have already been published elsewhere Weckström et al. (2004), Dong et al. (2006, 2008), Xiao et al. (2013), Chen et al. (2014), Wang et al. (2014), Cao et al. (2014), Deng et al. (2014), and Ventelä et al. (2011, 2015). In this study, however, the data of single sites is combined and compared in order to answer the research questions described above. This study is part of the Sino-Finnish project “Lakes in Trouble,” funded by the Academy of Finland and the Chinese Academy of Sciences, aiming to elucidate the effect of climate change on threatened ecosystem services of eutrophied aquatic ecosystems.
Materials and Methods
Pairs of freshwater lakes from Finland and China representing forest catchments, agricultural catchments, and urban catchments (Figure 1) were selected for palaeolimnological analyses. The definition of catchment type is based on the main land-use activity of the catchment area. The three catchment types were selected in order to get a larger gradient in the timing, speed and magnitude of change as natural change (forest catchment) is often subtle occurring over centuries or millennia, whereas cultural impact (agricultural catchment) may be seen within decades or even years (urban catchment). The widely applied thresholds for the most distinctive and intensive human-induced changes on lakes (i.e., 1850 and 1950, see above) have been marked as dashed lines in Figures 2, 3 for comparison with our lake data.
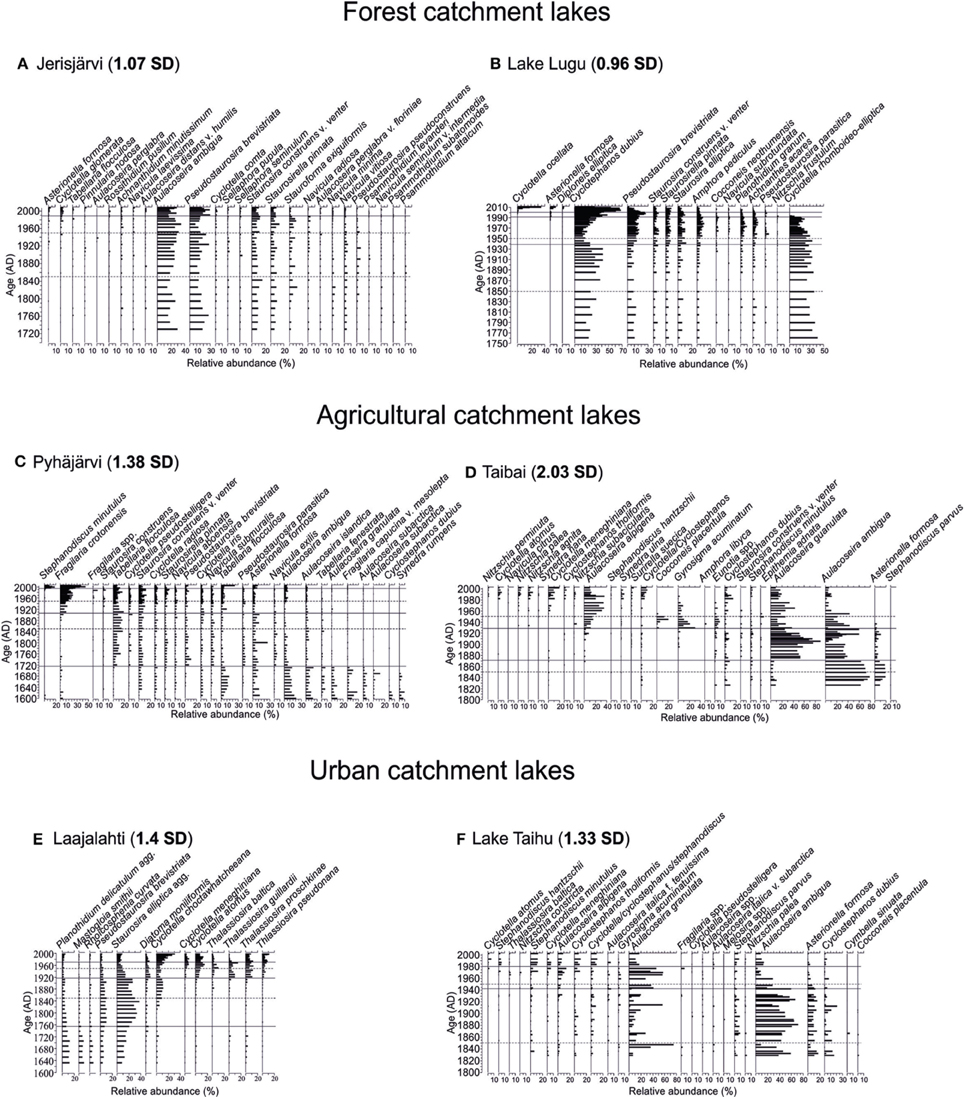
Figure 2. Stratigraphic diagrams of the most common diatom taxa. The years 1850 and 1950, usually referred to as the timing of most intensive change of aquatic ecosystems (e.g., Bennion et al., 2004; Räsänen et al., 2006) are marked with a dashed line. The statistically significant diatom zones are marked with a solid line. Beta-diversity values (in SD units) are shown in bold next to each site's name. The sites are arranged according to their catchment type: Forested catchments (A) Jerisjärvi, (B) Lake Lugu; Agricultural catchments, (C) Pyhäjärvi, (D) Lake Taibai; and Urban catchments, (E) Laajalahti, (F) Lake Taihu.
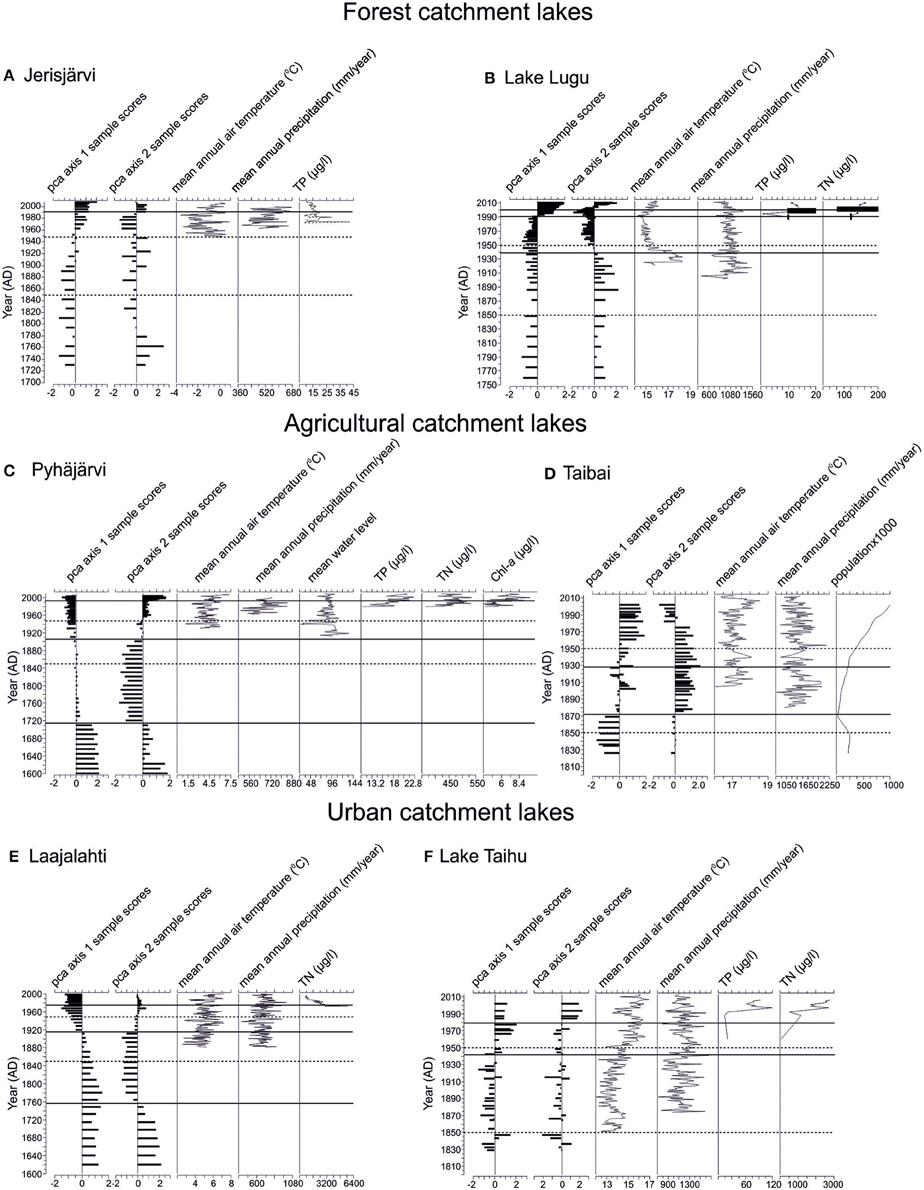
Figure 3. Summary of the PCA 1 and 2 axis sample scores and documented values for annual mean air temperature, annual mean precipitation, water level changes, TP, TN, Chl-a, and human population, if available. The years 1850 and 1950, usually referred to as the timing of most intensive change of aquatic ecosystems (e.g., Bennion et al., 2004; Räsänen et al., 2006) are marked with a dashed line. The statistically significant diatom zones are marked with a solid line. The black bars indicate roughly the approximate variability of TP and TN values of Lake Lugu according to Whitmore et al. (1997), Wang and Dou (1998), Wu et al. (2008), and Yu et al. (2010). The sites are arranged according to their catchment type: Forested catchments (A) Jerisjärvi, (B) Lake Lugu; Agricultural catchments, (C) Pyhäjärvi, (D) Lake Taibai; and Urban catchments, (E) Laajalahti, (F) Lake Taihu.
Forest Sites
Jerisjärvi (Finland)
Jerisjärvi (67.94°N, 24.02°E, 258 m a.s.l.) is located in northwestern Finland in the northern boreal zone (Figure 1). The lake has a surface area of 23.5 km2, a maximum depth of 12 m and a mean depth of 3.4 m (OIVA database, 2015). Its catchment area is ca. 109 km2 of which 83% constitutes of coniferous forests (Corine land cover data from European Environmental Agency). The mean annual average temperature (measured since 1947) decreased between 1947 and 1960 by ca. 2°C, but started to increase again from 1987 toward the present being currently −1.4°C (Figure 3A). The mean annual precipitation (measured since 1962) has increased from the beginning of the 1970s toward the present being currently 540 mm (Figure 3A; Supplementary 1). Autumnal (mainly August and September) total phosphorus (TP, measured since 1972) has decreased since the peak values of the 1970's, whereas summer TP (July) has slightly increased between 1972 and 1990 to similar levels than the present-day autumnal TP (Figure 3A). The lake is ice-covered for up to 8 months of the year (Supplementary 2). A sediment core of 10 cm was derived at a water depth of 4 m using a HTH-corer (Renberg and Hansson, 2008) in April 2007 and subsampled at 0.25 cm intervals representing a temporal resolution of ca. 1–15 years (Supplementary 3).
Lake Lugu (China)
Lake Lugu (27.27°N, 100.78° E, 2691 m a.s.l) is located in Ninglang County, Yunnan Province, southwestern China (Figure 1). The lake has a surface area of 48.25 km2, a maximum depth of 93.5 m and a mean depth of 40.3 m. Its catchment area is ca. 246 km2 of which 86% constitutes of forest. The mean annual average temperature (measured since 1921) was high between the mid-1920s and the beginning of 1940s, after which it decreased by almost 3°C until 1990. Since then, mean annual temperature has increased toward the present by ca. 1° C being at present 12.7°C (Figure 3B). The mean annual precipitation (measured since 1902) has decreased slightly since the 1920s, but more clearly during the last decade being at present 920 mm (Figure 3B) (Wang and Dou, 1998; Supplementary 1). Mean annual TP and total nitrogen (TN, measured since 1988) have increased since 1995 from < 10 μg/l to 10–20 μg/l, and from ca. 120 μg/l to 120–200 μg/l, respectively (Figure 3B) (Wu et al., 2008; Chen et al., 2014) (Supplementary 2). The lake is seasonally strongly stratified. A sediment core of 27.5 cm was derived near the central part of the southern Lake Lugu at a water depth of 41 m using a piston gravity corer in August 2010 and subsampled at 0.5-cm intervals representing a temporal resolution of 1–10 years (Chen et al., 2014).
Agricultural Sites
Pyhäjärvi (Finland)
Pyhäjärvi (61.00°N, 22.18°E, 45 m a.s.l.) is located in southwestern Finland (Figure 1) and is one of Finland's most extensively studied lakes due to its important role in supporting local ecosystem services. The lake has a surface area of 155 km2, a maximum depth of 26 m and a mean depth of 5.5 m (OIVA database, 2015). Its catchment area is ca. 616 km2 of which 22% constitutes of cultivated fields (Corine land cover data from European Environmental Agency). This area is one of the main food production areas in Finland, and agriculture as well as food processing industry is very intensive. Thus, the impact of cultivated fields on the lake is significant, although the forested and peatland areas cover a larger proportion of the catchment area than the agricultural area. The mean annual average temperature (measured since 1931) was relatively stable until the end of 1980s after which it started to increase being at present 4.8°C (Figure 3C). The mean annual precipitation (measured since 1931) has increased linearly toward the present from ca. 450 mm/year to ca. 650 mm/year (Figure 3C) (Supplementary 1). Annual water level measurements (measured since 1914) show distinct annual variability, but no clear trend throughout the measured series. Mean annual TP (measured since 1980) has increased from 1980 to ca. 2001 with some decline and stabilization since then. Mean annual TN (measured since 1980) slightly increased through the 1980s with relatively stable values or some decline since 1987, whereas the amount of chlorophyll a (Chl-a) increased through the 1990s with some decline and notable annual variations during the latest years (Figure 3C). The lake is ice-covered for ca. 5 months of the year (Supplementary 2). Two sediment cores were derived through the ice from the deepest part of the lake in March 2004 using a HON-Kajak-type corer (Renberg, 1991) for the most recent sediments (0-30 cm) and a modified Kullenberg corer (PP corer; Putkinen and Saarelainen, 1998) for the deeper sections (0–687 cm of which the uppermost 130 cm were used here). The HON-Kajak core was subsampled at 1 cm intervals representing a temporal resolution of 1–10 years (Ventelä et al., 2015), whereas the PP core was subsampled at intervals widening deeper down.
Lake Taibai (China)
Lake Taibai (29.13°N, 115.8°E, 16 m a.s.l) is located in Huanggang city, Hubei Province, in eastern China (Figure 1). The lake has a surface area of 25.1 km2, a maximum depth of 3.9 m and a mean depth of 3.2 m. Its catchment area is 960 km2 of which 70.4% constitutes of agricultural land. The mean annual average temperature (measured since 1905) has been relatively stable until ca. the mid 1990s after which temperature increased by ca 1°C until ca. 2007, but decreased toward the present being currently 16.7°C (Figure 3D). The mean annual precipitation (measured since 1880) has been relatively stable with a slight decrease since the 1970s toward present being currently 1273 mm (Wang and Dou, 1998; Supplementary 1). The water level is significantly impacted by precipitation. No measured nutrient data were available. However, according to Liu et al. (unpublished data) in Cao et al. (2014) the sedimentary TP values have increased since ca. 1650 and increased sharply again at ca. 1970, whereas according to Cao et al. (2014) a major increase in chironomid-inferred limnic TP values occurred since ca. 1940. The population in Huangmei County has grown between ca. 1350 and 1860 from ca. 100,000 to 400,000 inhabitants, after which a sharp decline occurred due to a series of peasant uprising by the Taiping Heavenly Kingdom. Since then the population has increased fast, especially since ca. 1950, being around 1 million at present (Cao et al., 2014) (Supplementary 2). A sediment core of 1.5 m was derived at the deepest part of the lake using a UWITEC sampling system in April 2004 and subsampled at 0.5 cm intervals for the topmost 50 cm representing a temporal resolution of 2–11 years and at 1 cm intervals for the sediment sequence below 50 cm (Dong et al., 2006).
Urban Sites
Laajalahti (Finland)
Laajalahti (60.18°N, 24.87°E, 0 m a.s.l) is a semi-enclosed urban embayment located in Helsinki, southern Finland (Figure 1). The embayment has a surface area of 5.3 km2, a maximum depth of 4 m and a mean depth of 2.4 m. The water exchange of the embayment is restricted, as it is connected to the open archipelago only by two narrow straits and its average salinity is low (4.6 o). Its catchment area is ca. 44 km2 of which 66% constitutes of constructed areas (Corine land cover data from European Environmental Agency). The mean annual average temperature (measured since 1882) is 5.9°C and has increased toward the present from ca. 4.5°C (Figure 3E). The mean annual precipitation (measured since 1882) has been relatively stable and is currently 655 mm (Supplementary 1). Mean annual TN (measured since 1972) has decreased drastically toward the present from its peak values at 1972 (Figure 3E). The embayment is ice-covered for ca. 4 months of the year. In the 1960s, Laajalahti was one of the most polluted coastal areas of southern Finland, receiving ca. 60 t yr−1 of total phosphorus, 300 t yr−1 of total nitrogen and 1000 t yr−1 of organic matter (measured as BOD7) from a sewage treatment plant built in 1957. At present, the embayment receives only diffuse loading from two brooks discharging 11.6 t yr−1 of TN and 0.9 t yr−1 of TP (Clarke et al., 2006) (Supplementary 2). A sediment core of 90 cm was derived from the deepest part of the embayment using a Mackereth corer (Mackereth, 1969) in September 1998 and subsampled at 1 cm intervals representing a temporal resolution of 1–20 years (Weckström, 2006).
Lake Taihu (China)
Lake Taihu (30.27°N, 120.7°E, 3.1 m a.s.l) is located in the middle and lower reaches of the Yangtze River, Jiangsu Province, in the eastern China (Figure 1). The lake has a surface area of 2338 km2, a maximum depth of 3.3 m and a mean depth of 2.1 m. Its catchment area is ca. 36500 km2 of which 28.1% constitutes of constructed area. Although the constructed area does not comprise the major part of the catchment's surface area, the catchment area is heavily populated with estimated inhabitants of 40 million people (Qin et al., 2010). The mean annual average temperature (measured since 1850) has increased from ca. 1920 to 1960 but was lower between ca. 1985 and 1995, after which temperature has increased again toward the present being currently 16°C (Figure 3F). The mean annual precipitation (measured since 1873) has been relatively stable with higher values between ca. 1900 and 1920, lower values between 1920 and 1930, after which precipitation increased until the mid-1950s. Since then mean annual precipitation has decreased toward the present being currently ca. 1084 mm (Figure 3F; Supplementary 1). Depending on the reference (e.g., Zhang and Qin, 2001; Mao et al., 2009; Deng et al., 2014), the mean annual TP (measured since 1960) has varied substantially between >20 μg/l to as high as 250 μg/l. In general, the values have increased until 1997, after which they have fluctuated, but decreased to ca. 100 μg/l at present. The differences in the measured TP values between the different references are most likely caused by the different water sampling points, as the northern part of lake Taihu is the most eutrophied part of the lake (e.g., Deng et al., 2014). The mean annual TN follows very closely the trend of TP described in Mao et al. (2009) with a drastic increase between 1992 and 1997, a decrease until 2002, after which it has increased again toward present (Figure 3F) (Supplementary 2). The lake is not stratified due its shallowness, large size and strong impact of wind. A sediment core of 50 cm was derived at a water depth of 2.5 m using a gravity corer in November 2002 and subsampled at 0.5 cm intervals representing a temporal resolution of 2–18 years (Dong et al., 2008).
Sediment Dating
Sediment samples were analysed for 210Pb, 226Ra, and 137Cs by gamma spectrometry in the Environmental Radioactivity Laboratory of Liverpool University (Jerisjärvi, Laajalahti), the State Key Laboratory of Lake Science and Environment, Nanjing Institute of Geography and Limnology, Chinese Academy of Sciences (Lake Lugu, Lake Taibai), the Institute of Geological Sciences, Polish Academy of Sciences, Poland (Pyhäjärvi) and in the Bloomsbury Environmental Isotope Facility at the University College London (Lake Taihu). The radiometric dating chronologies were calculated using the Constant Rate of Supply (CRS) and the Constant Initial Concentration (CIC) 210Pb dating models (Appleby and Oldfield, 1978).
Diatom Analyses
Diatoms were prepared using H2O2 digestion and HCl-treatment, and cleaned diatoms were mounted in Naphrax®. A minimum of 300 diatom valves from each sample were identified and counted along random transects at 1000x magnification for Jerisjärvi, Pyhäjärvi and Lake Taibai, whereas 600, 500, and 350 diatom valves were counted for Lake Lugu, Laajalahti, and Lake Taihu, respectively. However, due to dissolution of diatom valves in the northern parts of Lake Taihu only 100–200 valves from samples with dissolved and poorly preserved diatoms were counted. Diatom identification was based mainly on Krammer and Lange-Bertalot (1986, 1988, 1991a,b), Witkowski (1994), Qi (1995), Snoeijs (1993), Snoeijs and Vilbaste (1994), Snoeijs and Potapova (1995), Snoeijs and Kasperovièiene (1996), Snoeijs and Balashova (1998), and Witkowski et al. (2000). For more details concerning the procedures and taxonomic literature used, see Weckström et al. (1997a,b, 2004), Dong et al. (2006, 2008), Xiao et al. (2013), Chen et al. (2014), Wang et al. (2014), Cao et al. (2014), Deng et al. (2014), and Ventelä et al. (2015).
Data Analyses
All diatom counts were converted into percentages and plotted as a stratigraphical frequency diagram including only the most common diatom taxa (Figure 2), using the program C2 (version 1.7.2; Juggins, 2007). In order to identify periods of the most significant shifts in diatom assemblages constrained optimal sum of squares partitioning was used (Birks and Gordon, 1985). The number of statistically significant zones was calculated using the broken-stick model and the associated approach described in Bennett (1996). Optimal partitioning was implemented using the program ZONE 1.2 (Lotter and Juggins, 1991). Detrended canonical correspondence analysis (DCCA) was used to develop quantitative estimates of compositional turnover as beta-diversity, scaled in SD units (ter Braak and Verdonschot, 1995). The sample ages were added as the sole constraint in DCCA to estimate the total amount of compositional change in each biostratigraphic record. Differences in down-core DCCA sample scores provide an estimate of the amount of compositional change between samples along environmental or temporal gradients (Smol et al., 2005). Principal component analysis (PCA) was used in order to reveal the compositional changes in the biological data. DCCA and PCA were performed using CANOCO for Windows, version 5.01 (ter Braak and Šmilauer, 2007-2012). Time series of annual mean temperature, annual mean precipitation, annual mean water level, TP, TP, Chl-a, and population history, if available, were qualitatively compared with the first and second PCA axis sample scores. For more information on the methods used for deriving the environmental variables, see Supplementary 2.
Results
Forest Sites
Lake Jerisjärvi
The diatom stratigraphy of Jerisjärvi consisted of 160 taxa comprising both planktonic and benthic diatoms. The diatom assemblages were divided into two statistically significant zones at ca. 1990 (Figure 2A). The diatom assemblages were relatively stable throughout the core dominated by Aulacoseira ambigua and Pseudostaurosira brevistriata (Figure 2A). The first subtle changes can be observed around 1970, when the relative occurrence of Asterionella formosa and Cyclotella glomerata increased and the proportion of Aulacoseira ambigua decreased. The statistically significant change, however, occurred around 1990, where also the proportion of Pseudostaurosira brevistriata increased and Stauroforma exiquiformis decreased. This change is also demonstrated by the PCA axis 1 and PCA axis 2 sample scores (Figure 3A). No distinctive changes can be seen in the diatom assemblages around 1850 or 1950.
Lake Lugu
The diatom stratigraphy of Lake Lugu consisted of 145 taxa comprising both planktonic and benthic diatoms. The diatom assemblages were divided into four statistically significant zones at ca. 2001, 1991, and 1939 (Figure 2B). Overall, the planktonic component was dominated by Cyclostephanos dubius, Cyclotella rhomboideo-elliptica, and Cyclotella ocellata, whereas the benthic assemblages constituted mainly of Pseudostaurosira brevistriata and Staurosira elliptica (Figure 2B). The first statistically significant change around 1939 is mainly driven by the decrease of Cyclostephanos dubius. The clearest change occurred around 1991, when Cyclotella rhomboideo-elliptica disappeared from the diatom record. Around 2001, the relative abundance of Cyclotella ocellata, Asterionella formosa and Cyclostephanos dubius increased clearly, whereas the abundance of Pseudostaurosira brevistriata decreased (Figure 2B). These changes are also clearly demonstrated by the PCA axis 1 and axis 2 sample scores (Figure 3B). No distinctive changes in the diatom composition can be seen around 1850 or 1950.
Agricultural Sites
Pyhäjärvi
The diatom stratigraphy of Pyhäjärvi consisted of 295 taxa comprising both planktonic and benthic diatoms (Figure 2C). The diatom assemblages were divided into four statistically significant zones at ca. 1994, 1905, and 1715 (Figure 2C). The first clear change occurred around 1715, where the amount of periphytic diatoms such as Tabellaria flocculosa, Pseudostaurosira brevistriata, Navicula exilis, and Cyclotella pseudostelligera increased and, e.g., Aulacoseira ambigua, A. islandica, A. granulata, and A. subarctica decreased (Figure 2C). A second clear change occurred around 1905, when Fragilaria crotonensis increased rapidly and Tabellaria flocculosa and Staurosira construens f. venter maintained high abundances despite the increase in planktonic species. Around 1994 the relative abundance of Stephanodiscus minutulus and Fragilaria crotonensis increased markedly, but their abundance decreased again during the last decade (Figure 2C). These changes are also clearly demonstrated by the PCA axis 1 and 2 sample scores (Figure 3C). No distinctive changes in the diatom composition can be seen around 1850 or 1950.
Lake Taibai
The diatom stratigraphy of Lake Taibai consisted of 98 taxa (Dong et al., 2006). The diatom assemblages were divided into three statistically significant zones at ca. 1928 and 1872 (Figure 2D). Planktonic species dominated throughout the core and benthic species were rare (Figure 2D). Before ca. 1872 the diatom assemblage was dominated by Aulacoseira ambigua, Asterionella formosa, Aulacoseira granulata, Cyclotella meneghiniana, and Cyclostephanos dubius. At ca. 1872 a sudden increase of Aulacoseira granulata occurred with maximum abundances up to 90%. Around 1928 a second clear change occurred in the diatom assemblages as epiphytic species like Gyrosigma acuminatum and Cocconeis placentula increased rapidly together with the planktonic Aulacoseira alpigena (Figure 2D). Since the 1970s small planktonic Cyclotella spp., which are favored by nutrient-enriched waters increased and epiphytic species decreased. The change in diatom composition can also be seen in the PCA axis 1 and 2 sample scores (Figure 3D). No distinctive changes can be seen in the diatom assemblages around 1850 or 1950.
Urban Sites
Laajalahti
The diatom stratigraphy of Laajalahti consisted of 226 taxa (Figure 2E). The diatom assemblages were divided into four statistically significant zones at ca. 1975, 1915, and 1756 (Figure 2E). The lower part of the core (ca. 1600–1915) was dominated by benthic diatom assemblages, characterized by small Fragilaria spp., but also included several other benthic taxa such as Mastogloia smithii, Rhoicosphenia curvata, and Planothidium delicatulum agg. (Figure 2E). The first clear change occurred around 1756 when the abundance of Staurosira elliptica increased. The second significant change at 1915 can be seen when the proportion of planktonic diatoms doubled to >40%. At the start of this phase the dominant Fragilaria spp. declined and several small planktonic diatoms, such as Thalassiosira spp. and Cyclotella spp. increased in abundance. There was a further increase in planktonic diatoms in the mid-1950s, characterized by an increase in the common eutrophic taxa Cyclotella atomus and C. meneghiniana (EDDI, 2001; MOLTEN, 2004). Around 1975 a decline in the eutrophic Cyclotella meneghiniana and C. atomus and a rise in the less eutrophic taxon C. choctawhatcheeana can be observed (Figure 2E). These changes are also clearly revealed by PCA axis 1 and axis 2 sample scores (Figure 3E). No changes in the diatom composition can be seen around 1850 and 1950.
Lake Taihu
The diatom stratigraphy of Lake Taihu consisted of 81 taxa (Figure 2F). The diatom assemblages were divided into three statistically significant zones at ca. 1980 and 1943 (Figure 2F). The core was dominated throughout by planktonic diatoms such as Aulacoseira ambigua, Aulacoseira granulata, Asterionella formosa, Cyclostephanos spp., and Stephanodiscus spp. with only a few benthic species. The first clear change in the diatom assemblages occurred around 1943 as the relative abundance of Aulacoseira ambigua decreased and that of A. granulata increased (Figure 2F). A second major shift occurred around 1980 as the proportion of A. granulata decreased and, e.g., Cyclotella atomus, C. meneghiniana, Stephanodiscus hantzschii, S. minutulus, and Cyclostephanos tholiformis increased. These changes can also be observed in the PCA axis 1 and 2 sample scores (Figure 3F). No changes in the diatom composition can be seen around 1850 and 1950.
Species Compositional Turnover
The compositional change of the diatom assemblages of the study sites was estimated as beta-diversity. Higher value indicates greater taxonomical change. The total range of the beta-diversity varies from 0.96 SD (Lake Lugu) to 2.3 SD (Lake Taibai) (Figure 2). Overall, the forested catchment lakes had the lowest beta-diversity values, whereas, except for Lake Taibai, the values for the agricultural catchment sites and the urban catchment sites were relatively similar.
Discussion
Forest Sites
Jerisjärvi
The remote location of Jerisjärvi in the far north of Fennoscandia, an area with one of the lowest levels of atmospheric deposition in Europe for most environmental contaminants (e.g., sulfur and heavy metals, Hettelingh et al., 1991) and with low anthropogenic impact, has ensured that the lake has remained remarkably stable during the last few centuries (Figures 2A, 3A). Only very minor changes in the diatom composition have occurred highlighting the stability of the aquatic ecosystem and environmental settings except for the last few decades. The relatively subtle change in the diatom composition but clearer change in the PCA axis 1 sample scores around 1990 can mainly be seen as an increase in the abundances of Asterionella formosa and Cyclotella glomerata. Since the beginning of the 1970s the mean annual precipitation has increased and the duration of the ice cover decreased by ca. 2 weeks (OIVA database, 2015). Asterionella formosa is favored by slightly elevated nutrient concentrations (e.g., Anderson et al., 1995), whereas C. glomerata is thriving in low TP concentrations (Anderson et al., 1997). The discrepancy between our data and these known ecological preferences could be explained by the observation that A. formosa and C. glomerata are favored by moderate N enrichment in oligotrophic alpine lakes (Saros et al., 2005; Saros and Anderson, 2015), suggesting that increased TN could have been the reason behind their increase in Jerisjärvi instead of TP. Also, the increase in air temperature (Figure 3A) may have strengthened the stratification, thus reducing the mixing of the water column favoring small and/or lightly silicified diatoms (like C. glomerata and A. formosa) with very slow sinking velocities. Another explanation might be a decrease in silica content as A. formosa and C. glomerata are lightly silicified species (Olsson et al., 1992).
Lake Lugu
The diatom assemblages of Lake Lugu consisted mainly of oligotrophic taxa before 1990 (Figure 2B). Since 1991 the relative abundance of Cyclotella ocellata and Asterionella formosa increased and Cyclotella rhomboideo-elliptica disappeared from the diatom record. Cyclotella ocellata is usually found in nutrient poor waters (e.g., Cremer and Wagner, 2003), whereas Asterionella formosa thrives in lakes with elevated nutrient concentrations (e.g., Anderson et al., 1995). Cyclotella rhomboideo-elliptica is favored by clear and deep-oligo-mesotrophic conditions (Li et al., 2007). This change in the diatom composition is likely caused by the increased mean annual air temperature as the increase in nutrients has been modest before 1995 due to the remote location of the lake, and has been more substantial only after 2003 due to increased tourism (Chen et al., 2014). Increased air temperature will strengthen the stratification, thus reducing the mixing of the water column. This, in turn, enables the increase of the relative abundance of C. ocellata and A. formosa as they are small diatoms with very slow sinking velocities (e.g., Canter and Lund, 1948) compared to the much bigger and heavier C. rhomboideo-elliptica. The effect of the increased nutrient concentrations since 2005 can be seen as a decrease in the abundance of Pseudostaurosira brevistriata and an increase in Cyclostephanus dubius (e.g., Wu et al., 2008; Chen et al., 2014). As the anthropogenic impact on Lake Lugu has been very modest before the last two decades, climate warming has most probably been the most important environmental variable affecting the lake (Chen et al., 2014).
Agricultural Sites
Pyhäjärvi
The planktonic and meroplanktonic diatom assemblages suggest that Pyhäjärvi was meso-eutrophic before the late 1600s. Since then anthropogenic water level decrease related to the establishment of an iron mill to the outlet river in the 1600s and to a major drawdown in the 1850s had a drastic impact on Pyhäjärvi resulting in lake oligotrophication between ca. 1700 and 1930 (Ventelä et al., 2015). This is supported by an increase of periphytic diatoms and a decline in the proportion of planktonic diatoms, which suggests a reduction in phytoplankton production and improved water clarity. Moreover, a connection of Pyhäjärvi with an aquifer (Rautio and Korkka-Niemi, 2011, 2015) might also have contributed to the oligotrophication. Between ca. 1930 and 1994 major changes in the environmental setting occurred: vendace (Coregonus albula) was introduced in the 1950s culminating in high vendace abundances since the 1960s (Sarvala et al., 1998), agriculture intensified due to the increased use of artificial fertilizers after the Second World War (Valkama et al., 2009), and the first signs of climatic change appeared in the early 1990s (Ventelä et al., 2011). Between 1980 and 1994, the measured TP increased from 11 μg/l to 19.3 μg/l. The increase in TP was reflected in a rapid increase of Fragilaria crotonensis, a species indicating eutrophic conditions. In 1995, the Pyhäjärvi Restoration Programme was initiated (Kirkkala, 2014) and the Finnish agri-environmental system was established, introducing new resources and tools aimed to reduce external loading. The vendace stock was weaker than earlier and commercial fishermen were (as from 1995) paid to fish also commercially less valuable fish species (smelt, roach, ruffe). Biomanipulation was further intensified after 2002 (Ventelä et al., 2007). These lake management efforts can be seen as decreased abundances of diatoms, which are favored by elevated nutrient levels.
Lake Taibai
Since the beginning of the 1600s the diatom composition in Lake Taibai has been fluctuating (Dong et al., 2006). The quantitatively inferred diatom-based TP values have varied between ca. 50 and 160 μg/l during the last four centuries, and a TP concentration of 50 μg/l has been suggested as a reference/background level of Lake Taibai (Dong et al., 2006; Cao et al., 2014). These values imply that the lake has not been truly oligotrophic for centuries, further supported by the fact that planktonic species have dominated throughout the core and benthic species have been rare (Figure 2D). The first change in the diatom assemblages occurred at ca. 1872 as Aulacoseira granulata increased suddenly with a relative occurrence up to 90% and A. ambigua decreased (Figure 2D). This might have been caused by a slight increase in nutrient concentrations or due to increased turbulence as A. granulata is more heavily silicified than A. ambigua and is more dependent on the mixing of the water column than A. ambigua. At ca. 1928 a second clear change occurred in the diatom assemblages as epiphytic species like Gyrosigma acuminata, Eunotia pectinalis and Cocconeis placentula increased rapidly together with the planktonic Aulacoseira alpigena (Figure 2D). As the epiphytic taxa thrive in shallower environments and A. alpigena is distinctly smaller than A. granulata and A. ambigua, this change might have been due to a decrease in water level. Indeed, according to Liu et al. (2007) and Xiao et al. (2013) the water level has decreased significantly in the 1950s due to land reclamation. Another clear change occurred in the 1970s as small planktonic Cyclotella spp., Cyclostephanos spp., and Stephanodiscus spp., which are favored by nutrient-enriched waters increased and epiphytic species decreased (Figure 2D). This change is highlighted also by the significant drop in the PCA axis 2 sample scores (Figure 3D). According to Dong et al. (2006), Xiao et al. (2013), and Cao et al. (2014), the nutrient levels of Lake Taibai have increased significantly after the 1950s, and increased even more drastically during the 1970s, reaching values up to 120 μg/l (Dong et al., 2006). Since the 1950s the mean annual air temperature has increase slightly, but the mean annual precipitation instead decreased. The impact of climate change on the lake has been larger before 1900, but after the 1950s has been overridden by the anthropogenic impact. The increase of inhabitants in the catchment area of Lake Taibai during the last 60 years from ca. 400,000 to over a million (Cao et al., 2014) and the intensified agriculture accompanied with large-scale irrigation systems (Xiao et al., 2013) has had a negative impact on the development of the water quality of Lake Taibai.
Urban Sites
Laajalahti
The first notable change in the diatom assemblages occurred in the mid-1700s (Figures 2E, 3E), which can be seen as an increase in Pseudostaurosira elliptica agg., P. brevistriata and a decrease in the abundances of Mastogloia smithii and Planothidium delicatulum agg. The predominance of Fragilaria spp. is typical for shallow and semi-enclosed coastal embayments as well as recently isolated lakes (Stabell, 1985). The water depth of Laajalahti has decreased over time due to post-glacial isostatic land uplift (at present ca. 0.3 cm yr-1 in the study area) and it may have reached a threshold during the mid-18th century, after which conditions were favorable for small, benthic Fragilaria taxa. The largest change in diatom assemblages occurred around 1915, when the proportion of planktonic diatoms doubled to >40% coinciding with a rapid expansion of the urban area (Laakkonen and Lehtonen, 1999). In the mid-1950s, two waste water treatment plants were installed in the watershed of Laajalahti. However, these treatment plants only removed organic matter from sewage (Weckström et al., 2004), which resulted in an increase of the relative abundance of the common eutrophic taxa Cyclotella atomus and C. meneghiniana (EDDI, 2001; MOLTEN, 2004). In the early 1970s a clear response to improved waste water purification can be seen as a decreased abundance of meso-eutrophic planktonic taxa. In 1986 a further marked reduction in the nutrient load occurred, when the last treatment plant was closed. In response, the eutrophic Cyclotella meneghiniana and C. atomus declined and the less eutrophic taxon C. choctawhatcheeana increased (Figure 2E). Although a clear recovery of Laajalahti could be observed after the cessation of waste water loading, there is still a marked difference between the diatom assemblages of the lower part of the core compared to the present diatom assemblages with no sign of a recovery back to more diverse benthic communities.
Lake Taihu
The first notable changes in the diatom assemblages of Lake Taihu can be seen between around 1943, when the dominant species changes from Aulacoseira ambigua to A. granulata (Figure 2F). Both species are favored by turbulent conditions as they are heavily silicified and thus have a high sinking rate (Bradbury, 1975) and thrive in meso- to eutrophic shallow lakes (e.g., Manoylov et al., 2009). Although Lake Taihu has been categorized as oligotrophic before 1960 (Qin et al., 2007), these two Aulacoseira taxa, and also the presence of Asterionella formosa, which is favored by elevated nutrient concentrations (e.g., Anderson et al., 1995), suggests that the lake has been mesotrophic at least since the 1800s. This finding is in line with, e.g., Dong et al. (2008), who inferred limnic TP values around 50 μg/l before 1900, using a diatom-based quantitative inference model. As precipitation increased slightly during this period the change between A. ambigua and A. granulata might have been caused by small increase in nutrient concentrations via increased in-wash from the catchment, or increased turbulence as A. granulata is heavier than A. ambigua. A second major shift occurred around 1980 as the proportion of A. granulata decreased and, e.g., Cyclotella atomus, C. meneghiniana, Stephanodiscus hantzschii, S. minutulus, and Cyclostephanos tholiformis increased. These taxa are known indicators of nutrient-rich waters (e.g., Bradshaw et al., 2002; Dong et al., 2006). This change is caused by the rapid increase in the population density and an increase of in-washed waste water (e.g., Qin et al., 2007). In the 1980s and 1990s the amount of domestic pollution doubled, whereas the input of industrial TP decreased to one third (Qin et al., 2007). This change reflects rapid economic growth and land use alteration. As there are no clear changes in temperature and precipitation at this time, the main cause behind this marked aquatic change in Lake Taihu can be addressed to anthropogenic activity. This is also supported by the measured TN and TP records, which increased clearly in the beginning of the 1990s remaining high until present (e.g., Mao et al., 2009; Deng et al., 2014). The water quality of Lake Taihu has deteriorated significantly from class I before 1970 to class V in the late 1990s (State Environmental Protection Administration, 2000; Dong et al., 2008).
Changes in Aquatic Ecosystems Surrounded by Different Land-use in Finland and China
Clear changes, especially in aquatic ecosystems under anthropogenic pressure were observed. The lakes surrounded by a forested catchment area have remained relatively pristine until the last few decades. Although nutrient concentrations have increased slowly, especially in Lake Lugu, the impact of climate warming and related variables (e.g., changes in stratification and ice cover) seem to be the driving mechanism behind changes in the diatom assemblages of both lakes (Figures 3A,B). This is not surprising, as both lakes are located relatively remotely suffering only from minor human disturbance (Figure 1). The relatively pristine condition of these lakes is also supported by the lowest beta-diversity values of all lake pairs. The lakes impacted by an agricultural catchment have experienced more changes due to the elevated impact of anthropogenic activities. Both lakes have undergone an artificial water-level decrease, which has altered the diatom composition toward benthic and small-sized planktonic taxa. Climate change has also impacted both lakes, however, a clearer response can be seen in the high latitude Lake Pyhäjärvi, whereas anthropogenic activities have overridden the climate signal in Lake Taibai (Figures 3C,D). This lake had also the highest beta-diversity value of all lakes suggesting a significant overall change in the diatom assemblages. Also the aquatic ecosystems surrounded by an urban catchment have undergone several changes related to anthropogenic activities. The changes differ both in time and magnitude as Laajalahti has been affected by human disturbance since the 1920s, whereas Lake Taihu has changed distinctively since the 1980s. As the catchment of Lake Taihu is heavily constructed comprising ca. 40 million inhabitants, the anthropogenic pressure on Lake Taihu is much stronger compared with Laajalahti. This can be seen as a drastic deterioration of the water quality and a drinking water problem (Qin et al., 2010). However, the beta-diversity values are very similar suggesting, that although the anthropogenic stress on Lake Taihu is clearly stronger than in Laajalahti the magnitude of changes in their diatom assemblages is comparable. This points toward an insensitivity of diatom assemblages (no further change in species composition and life forms) at very high nutrient concentrations, which has been discussed previously in, for example, Weckström et al. (2007).
The changes in aquatic ecosystems surrounded by the same catchment type are rather similar regardless of their geographical setting. This suggests that their response to external pressure is comparable. The changes between different catchment types, however, differ in magnitude and timing. Aquatic ecosystems situated in relatively pristine conditions change slowly due to the lack of anthropogenic pressure. The aquatic ecosystems surrounded by human activities, instead, change within years, especially if located in areas with intensive agricultural activities or densely populated urbanized areas.
Conclusions
Palaeolimnology has been used for decades to reconstruct past environmental conditions, and in the framework of WFD can be used to define “reference” conditions in the absence of long-term monitoring data. However, many of these studies have been spatially restricted. Here were demonstrate the usefulness of the palaeolimnological approach regardless of the different geographical and environmental settings of sites. We used relatively time- and cost-efficient fossil diatom analysis from six radiometrically dated sediment series derived from aquatic ecosystems representing catchment areas with varying land use (forests, urban areas, and agriculture) from Finland and China. By using simple multivariate statistics (DCCA, PCA) we were able to demonstrate the time, magnitude and direction of changes of the study sites, and relate them to different environmental variables. The observed changes in diatom assemblages differed both spatially and temporally and are mostly related to anthropogenic impact and climate forcing. The first distinct changes in the Finnish sites were observed around 1715 in the agricultural site due to an artificial anthropogenic water level decrease, around 1915 in the urban site due to the increasing population of the catchment area, and around 1989 in the forested site due to climate change and due to slightly increased anthropogenic pressure. In China, first clear changes in the diatom assemblages were observed since 1870 in the agricultural site and around 1943 in the urban catchment due to the increased land use activities and dramatic increase in population density especially since the 1980s. First subtle changes in the forested site can be seen around 1939 followed by more marked changes since 1991 due to increased air temperature and tourism.
Despite the long distance between the sites and their different land-use characteristics, the direction, magnitude and timing of changes during the last 150 years are well comparable between the high latitudes of Finland and the mid-low latitudes of China. This study reinforces the sensitivity of aquatic ecosystems to climate change and anthropogenic impact and underlines the usefulness of the palaeolimnological approach as a tool for determining reference conditions for these markedly changed systems.
We also show that the commonly suggested threshold for reference conditions at ca. 1850 for, especially, European lakes and the second major change in aquatic ecosystems at ca. 1950 after the increased use of artificial fertilizers do not necessarily apply to aquatic environments with differing geographical and environmental settings. Thus, instead of relying on such generalizations of the environmental history of aquatic ecosystems, it is of vital importance to assess individual sites using palaeolimnological methods before deciding on management and restoration goals.
Conflict of Interest Statement
The authors declare that the research was conducted in the absence of any commercial or financial relationships that could be construed as a potential conflict of interest.
Acknowledgments
Pyhäjärvi studies were supported by Pyhäjärvi Restoration Program and Academy of Finland funded projects CARE (203591) and Lakes in Trouble (256240). Funding for field and laboratory studies on Pyhäjärvi came from many sources over the years, in particular a series of grants from the Academy of Finland (including Nos. 09/109, 09/074, 1071004, 1071149, 1071255, 35619, 44130, 52271, 104483, 201414, 203591, 256240), EU's Financial Instrument for Fisheries Guidance (Pyhäjärvi Biomanipulation Project 2002-2006), European Regional Development Fund, Ministry of Agriculture and Forestry (biomanipulation funding since 2010) and Pyhäjärvi Protection Fund. This work was also supported by the Chinese Academy of Sciences (Grant GJHZ1214 and KZZD-EW-TZ-08) and the China Ministry of Science and Technology (Grants 2012CB956103 and 2013CB956501). The two reviewers are acknowledged for their constructive comments.
Supplementary Material
The Supplementary Material for this article can be found online at: http://journal.frontiersin.org/article/10.3389/fevo.2015.00126
References
Anderson, N. J., Blomqvist, P., and Renberg, I. (1997). An experimental and palaeoecological study of algal responses to lake acidification and liming in three central Swedish lakes. Eur. J. Phycol. 32, 35–48. doi: 10.1080/09541449710001719355
Anderson, N. J., Renberg, I., and Segerström, U. (1995). Diatom production responses to the development of early agriculture in a boreal forest lake-catchment (Kassjon, northern Sweden). J. Ecol. 83, 809–822. doi: 10.2307/2261418
Appleby, P. G., and Oldfield, F. (1978). The calculation of lead-210 dates assuming a constant rate of supply of unsupported 210Pb to the sediment. Catena 5, 1–8. doi: 10.1016/S0341-8162(78)80002-2
Battarbee, R., and Bennion, H. (2011). Palaeolimnology and its developing role in assessing the history and extent of human impact on lake ecosystems. J. Paleolimnol. 45, 399–404. doi: 10.1007/s10933-010-9423-7
Battarbee, R. W., Morley, D., Bennion, H., Simpson, G. L., Hughes, M., and Bauere, V. A. (2011). A palaeolimnological meta-database for assessing the ecological status of lakes. J. Paleolimnol. 45, 405–414. doi: 10.1007/s10933-010-9417-5
Bennett, K. (1996). Determination of the number of zones in a biostratigraphical sequence. New Phytol. 132, 155–170. doi: 10.1111/j.1469-8137.1996.tb04521.x
Bennion, H., and Battarbee, R. W. (2007). The European Union Water Framework Directive: opportunities for palaeolimnology. J. Paleolimnol. 38, 285–295. doi: 10.1007/s10933-007-9108-z
Bennion, H., Battarbee, R. W., Sayer, C., Simpson, G. L., and Davidson, T. A. (2011). Defining reference conditions and restoration targets for lake ecosystems using palaeolimnology: a synthesis. J. Paleolimnol. 45, 533–544. doi: 10.1007/s10933-010-9419-3
Bennion, H., Fluin, J., and Simpson, G. L. (2004). Assessing eutrophication and reference conditions for Scottish freshwater lochs using subfossil diatoms. J. Appl. Ecol. 41, 124–138. doi: 10.1111/j.1365-2664.2004.00874.x
Birks, H. J. B., and Gordon, A. D. (1985). Numerical Methods in Quaternary Pollen Analysis. London: Academic Press.
Bouleau, G., and Pont, G. (2015). Did you say reference conditions? Ecological and socio-economic perspectives on the European Water Framework Directive. Environ. Sci. Policy 47, 32–41. doi: 10.1016/j.envsci.2014.10.012
Bradbury, J. P. (1975). Diatom Stratigraphy and Human Settlement in Minnestoa. Boulder: Geological Society of America.
Bradshaw, E. G., Anderson, N. J., and Jensen, J. P. (2002). Phosphorus dynamis in Danish lakes and the implications for diatom ecology and palaeoecology. Freshwater Biol. 47, 1963–1975. doi: 10.1046/j.1365-2427.2002.00938.x
Bradshaw, E. G., Nielsen, A. B., and Anderson, N. J. (2006). Using diatoms to assess the impacts of prehistoric, pre-industrial and modern land-use on Danish lakes. Reg. Environm. Change 6, 17–24. doi: 10.1007/s10113-005-0007-4
Canter, H. N., and Lund, J. W. G. (1948). Studies on plankton parasites. New Phytol. 47, 238–261. doi: 10.1111/j.1469-8137.1948.tb05102.x
Cao, Y., Zhang, E., Langdon, P. G., Liu, E., and Shen, J. (2014). Chironomid-inferred environmental change over the past 1400 years in the shallow, eutrophic Taibai Lake (south-east China): separating impacts of climate and human activity. Holocene 24, 581–590. doi: 10.1177/0959683614522308
Charles, D., Smol, J. P., and Engstrom, D. R. (1994). “Paleolimnological approaches to biological monitoring,” in Biological Monitoring of Aquatic Systems, eds S. L. Loeb and A. Spacie (Boca Raton, FL: CRC Press), 233–293.
Chen, C. H, Zhao, L., Zhu, C., Wang, J., Jiang, J., and Yang, S. (2014). Response of diatom community in Lugu Lake (Yunnan–Guizhou Plateau, China) to climate change over the past century. J. Paleolimnol. 51, 357–373. doi: 10.1007/s10933-013-9760-4
Clarke, A. L., Weckström, K., Conley, D. J., Anderson, N. J., Adser, F., Andrén, E., et al. (2006). Long-term trends in eutrophication and nutrients in the coastal zone. Limnol. Oceanogr. 51, 385–397. doi: 10.4319/lo.2006.51.1_part_2.0385
Cremer, H., and Wagner, B. (2003). The diatom flora in the ultra-oligotrophic Lake El'gygytgyn, Chukotka. Polar Biol. 26, 105–114. doi: 10.1007/s00300-002-0445-0
Deevey, E. S. (1984). “Stress, strain and stability in lacustrine ecosystems,” in Lake Sediments and Environmental History, eds E. Y. Haworth and J. W. G. Lund (Leicester: Leicester University Press), 203–229.
Deng, J., Qin, B., Paerl, H. W., Zhang, Y., Wu, P., Ma, J., et al. (2014). Effects of nutrients, temperature and their interactions on Spring phytoplankton community succession in Lake Taihu, China. PLoS ONE 9:e113960. doi: 10.1371/journal.pone.0113960
Dixit, S. S., Smol, J. P., Kingston, J. C., and Charles, D. F. (1992). Diatoms: powerful indicators of environmental change. Environ. Sci. Technol. 26, 22–33. doi: 10.1021/es00025a002
Dong, X. H., Bennion, H., Battarbee, R., Yang, X., Yang, H., and Liu, E. (2008). Tracking eutrophication in Taihu Lake using the diatom record: potential and problems. J. Paleolimnol. 4, 413–429. doi: 10.1007/s10933-007-9170-6
Dong, X. H., Yang, X. D., and Liu, E. F. (2006). Diatom records and reconstruction of epilimnetic phosphorus concentration in Lake Taibai (Hubei Province) over past 400 years. J. Lake Sci. 18, 597–604. doi: 10.18307/2006.0607
Downing, J., Prairie, Y., Cole, J., Duarte, C., Tranvik, L., Striegl, R., et al. (2006). The global abundance and size distribution of lakes, ponds, and impoundments. Limnol. Oceanogr. 51, 2388–2397. doi: 10.4319/lo.2006.51.5.2388
EDDI (2001). The European Diatom Database. Available online at: http://craticula.ncl.ac.uk/Eddi/jsp/index.jsp
European Union (2000). Directive 2000/60/EC of the European Parliament and of the Council of 23 October 2000 establishing a framework for Community action in the field of water policy. OJ C 327, 1–72.
Guilizzoni, P., Marchetto, A., Lami, A., Gerli, S., and Musazzi, S. (2011). Use of sedimentary pigments to infer past phosphorus concentration in lakes. J. Paleolimnol. 45, 433–445. doi: 10.1007/s10933-010-9421-9
Henriksen, A., Mannio, J., Wilander, A., Moiseenko, T., Traaen, T., Skjelkvåle, B. L., et al. (1997). Regional Lake Surveys in the Barents Region of Finland, Norway, Sweden and Russian Kola, 1995, Report 45/97. Oslo: Norwegian Institute of Water Research.
Hettelingh, J.-P., Downing, R. J., and de Smet, P. A. M. (eds.). (1991). “Mapping critical loads concept for Europe,” in CCE Technical Report No. 1 (Bilthoven: National Institute of Public Health and Environmental Protection (RIVM)).
Jeppesen, E., Meerhoff, M., Davidson, T. A., Søndergaard, M., Lauridsen, T. L., Beklioglu, M., et al. (2014). Climate change impacts on lakes: an integrated ecological perspective based on a multi-faceted approach, with special focus on shallow lakes. J. Limnol. 73, 88–111. doi: 10.4081/jlimnol.2014.844
Jeppesen, E., Mehner, T., Winfield, I. J., Kangur, K., Sarvala, J., Gerdeaux, D., et al. (2012). Impacts of climate warming on lake fish assemblages: evidence from 24 European long-term data series. Hydrobiologia 694, 1–39. doi: 10.1007/s10750-012-1182-1
Juggins, S. (2007). C2 Version 1.5: Software for Ecological and Palaeoecological Data Analysis and Visualisation. Newcastle upon Tyne: University of Newcastle.
Kirkkala, T. (2014). Long-term nutrient load management and lake restoration: case of Säkylän Pyhäjärvi (SW Finland). Ann. Univ. Turkuensis A II 286, 55.
Krammer, K., and Lange-Bertalot, H. (1986). “Bacillariophyceae 1, Naviculaceae,” in Süsswasserflora von Mitteleuropa, Band 2, eds H. Ettl, J. Gerloff, H. Heynig, and D. Mollenhauer (Jena; Stuttgart; Lübeck; Ulm: Gustav Fisher), 867.
Krammer, K., and Lange-Bertalot, H. (1988). “Bacillariophyceae 2, Bacillariaceae, Epithemiaceae, Surirellaceae,” in Süsswasserflora von Mitteleuropa, Band 2, eds H. Ettl, J. Gerloff, H. Heynig, and D. Mollenhauer (Jena; Stuttgart; Lübeck; Ulm: Gustav Fisher), 596.
Krammer, K., and Lange-Bertalot, H. (1991a). “Bacillariophyceae 3, Centrales, Fragilariaceae, Eunotiaceae,” in Süsswasserflora von Mitteleuropa, Band 2, eds H. Ettl, J. Gerloff, H. Heynig, and D. Mollenhauer (Jena; Stuttgart; Lübeck; Ulm: Gustav Fisher), 576.
Krammer, K., and Lange-Bertalot, H. (1991b). “Bacillariophyceae 4, Achnanthaceae, Kritishe Ergänzungen zu Navicula (Lineolatae) und Gomphonema,” in Süsswasserflora von Mitteleuropa, Band 2, eds H. Ettl, G. Gärtner, J. Gerloff, H. Heynig, and D. Mollenhauer (Jena; Stuttgart; Lübeck; Ulm: Gustav Fisher), 536.
Laakkonen, S., and Lehtonen, P. (1999). A quantitative analysis of discharges into the Helsinki urban sea area in 1850–1995. Eur. Water Mgmt. 2, 30–39.
Li, Y., Gong, Z., and Shen, J. (2007). Freshwater diatoms in eight lakes in the Yunnan Plateau, China. J. Freshwater Ecol. 22, 169–171. doi: 10.1080/02705060.2007.9664161
Liu, E., Yang, X., Shen, J., Dong, X., Zhang, E., and Wang, S. (2007). Environmental response to climate and human impact during the last 400 years in Taibai Lake catchment, middle erach of Yangtze River, China. Sci. Total Environ. 385, 196–207. doi: 10.1016/j.scitotenv.2007.06.041
Lotter, A. F., and Juggins, S. (1991). “PLOPROF, TRAN and ZONE. Programs for plotting, editing and zoning of pollen and diatom data.” in INQUA Commission for the Study of the Holocene, Working Group on Data Handling Methods, Newsletter 6.
Mackereth, F. J. H. (1969). A short core sampler for subaqueous deposits. Limnol. Oceanogr. 14, 145–151. doi: 10.4319/lo.1969.14.1.0145
Manoylov, K. M., Ognjanova-Rumenova, N., and Stevenson, R. J. (2009). Morphotype variations in subfossil diatom species of Aulacoseira in 24 Michigan Lakes, USA. Acta Bot. Croat. 68, 401–419.
Mao, X., Xu, F., Xu, B., and Gao, Y. (2009). Changes of water quality and eutrophication in Taihu Lake. Wat. Res. Prot. 25, 48–51.
Millennium Ecosystem Assessment (2005). Ecosystems and Human Well-being. Current State and Trends. Washington, DC: Island Press.
MOLTEN (2004). Monitoring Long-term Trends in Eutrophication and Nutrients in the Coastal Zone. Availabe online at: http://Craticula.ncl.ac.uk/Molten/jsp/
Moser, K. A., MacDonald, G. M., and Smol, J. P. (1996). Applications of freshwater diatoms to geographical research. Progr. Phys. Geogr. 20, 21–52. doi: 10.1177/030913339602000102
Moss, B., Kosten, S., Meerhoff, M., Battarbee, R. W., Jeppesen, E., Mazzeo, N., et al. (2011). Allied attack: climate change and nutrient pollution. Inland Wat. 1, 101–105. doi: 10.5268/IW-1.2.359
OIVA database (2015). OIVA – The Environmental and Geographical Information Service, Finland's Environmental Administration. Available online at: http://wwwp2.ymparisto.fi/scripts/oiva.asp
Olsson, H., Blomqvist, P., and Olofsson, H. (1992). Phytoplankton and zooplankton community structure after nutrient additions to the oligotrophic Lake Hecklan, Sweden. Hydrobiologia 243/244, 147–155. doi: 10.1007/BF00007030
Pollard, P., and Huxham, M. (1998). The European Water Framework Directive: a new era in the management of aquatic ecosystem health? Aquat. Conserv. Mar: Freshwat. Ecosyst. 8, 773–792.
Putkinen, S., and Saarelainen, J. (1998). Kullenbergin näytteenottimen uusi kevennetty malli. Geologi 50, 22–23.
Qi, Y. Z. (1995). Flora Algarum Sinicarum Aquae Dulcis, Tomus IV, Bacillariophyta, Centricae. Beijing: Science Press.
Qin, B., Xu, P., Wu, Q., Luo, L., and Zhang, Y. (2007). Environmental issues of Lake Taihu, China. Hydrobiologia 581, 3–14. doi: 10.1007/s10750-006-0521-5
Qin, B., Zhu, G., Gao, G., Zhang, Y., Li, W., Paerl, H. W., et al. (2010). A drinking water crisis in Lake Taihu, China: linkage to climate variability and lake management. Environ. Manage. 45, 105–112. doi: 10.1007/s00267-009-9393-6
Räsänen, J., Kauppila, T., and Salonen, V.-P. (2006). Sediment-based investigation of naturally or historically eutrophic lakes – implications for lake management. J. Environ. Manage. 79, 253–265. doi: 10.1016/j.jenvman.2005.08.001
Rautio, A., and Korkka-Niemi, K. (2011). Characterization of Groundwater-Lake Water interactions at Lake Pyhäjärvi, SW Finland. Bor. Environ. Res. 16, 363–380.
Rautio, A., and Korkka-Niemi, K. (2015). Chemical and isotopic tracers indicating groundwater/surface-water interaction within a boreal lake catchment in Finland. Hydrogeol. J. 23, 687–705. doi: 10.1007/s10040-015-1234-5
Renberg, I. (1990). A 12,600 year perspective of the acidification of Lilla Öresjön, southwest Sweden. Philos. Trans. R. Soc. B 327, 357–361. doi: 10.1098/rstb.1990.0073
Renberg, I. (1991). The HON-Kajak sediment corer. J. Paleolimnol. 6, 167–170. doi: 10.1007/BF00153740
Renberg, I., and Hansson, H. (2008). The HTH sediment corer. J. Paleolimnol. 40, 655–659. doi: 10.1007/s10933-007-9188-9
Saros, J. E., and Anderson, N. J. (2015). The ecology of the planktonic diatom Cyclotella and its implications for global environmental change studies. Biol. Rev. 90, 522–541. doi: 10.1111/brv.12120
Saros, J. E., Michel, T. J., Interlandi, S. J., and Wolfe, A. P. (2005). Resource requirements of Asterionella formosa and Fragilaria crotonensis in oligotrophic alpine lakes: implications for recent phytoplankton community reorganizations. Can. J. Fish. Aquat. Sci. 62, 1681–1689. doi: 10.1139/f05-077
Sarvala, J., Helminen, H., and Auvinen, H. (1998). Portrait of a flourishing freshwater fishery: Pyhäjärvi, a lake in SW-Finland. Bor. Environ. Res. 3, 329–345.
Saulnier-Talbot, É. (2015). Overcoming the disconnect: are paleolimnologists doing enough to make their science accessible to aquatic managers and conservationists? Front. Ecol. Evol. 3:32. doi: 10.3389/fevo.2015.00032
Smol, J. P. (2008). Pollution of Lakes and Rivers: A Paleoenvironmental Perspective, 2nd Edn. Oxford: Blackwell.
Smol, J. P., Wolfe, A. P., Birks, H. J. B., Douglas, M. S. V., Jones, V. J., Korhola, A., et al. (2005). Climate-driven regime shifts in arctic lake ecosystems. Proc. Natl. Acad. Sci. U.S.A. 102, 4397–4402. doi: 10.1073/pnas.0500245102
Snoeijs, P. (1993). Intercalibration and Distribution of Diatom Species in the Baltic Sea, Vol. 1. The Baltic Marine Biologists Publication 16a. Uppsala: Opulus Press.
Snoeijs, P., and Balashova, N. (1998). Intercalibration and Distribution of Diatom Species in the Baltic Sea, Vol. 5. The Baltic Marine Biologists Publication 16e. Uppsala: Opulus Press.
Snoeijs, P., and Kasperovièiene, J. (1996). Intercalibration and Distribution of Diatom Species in the Baltic Sea, Vol. 4. The Baltic Marine Biologists Publication 16d. Uppsala: Opulus Press.
Snoeijs, P., and Potapova, M. (1995). Intercalibration and Distribution of Diatom Species in the Baltic Sea, Vol. 3. The Baltic Marine Biologists Publication 16c. Uppsala: Opulus Press.
Snoeijs, P., and Vilbaste, S. (1994). Intercalibration and Distribution of Diatom Species in the Baltic Sea, Vol. 2. The Baltic Marine Biologists Publication 16b. Uppsala: Opulus Press.
Stabell, B. (1985). The development and succession of taxa within the diatom genus Fragilaria Lyngbye as a response to basin isolation from the sea. Boreas 14, 273–286. doi: 10.1111/j.1502-3885.1985.tb00916.x
State Environmental Protection Administration (2000). Water Protection in the Three Rivers and Three Lakes of China. Beijing: Chinese Environmental Press.
ter Braak, C. J. F., and Šmilauer, P. (2007–2012). Canoco Reference Manual and User's Guide: Software for 623 Ordination (version 5.0). Microcomputer Power, Itaca.
ter Braak, C. J. F., and Verdonschot, P. F. M. (1995). Canonical correspondence analysis and related multivariate methods in aquatic ecology. Aquat. Sci. 57, 255–289. doi: 10.1007/BF00877430
Valkama, E, Uusitalo, R., Ylivainio, K., Virkajärvi, P., and Turtola, E. (2009). Phosphorus fertilization: a meta-analysis of 80 years of research in Finland. Agr. Ecosyst. Environ. 130, 75–85. doi: 10.1016/j.agee.2008.12.004
Ventelä, A.-M., Amsinck, S. L., Kauppila, T., Johansson, L. S., Jeppesen, E., Kirkkala, T., et al. (2015). Ecosystem changes in large and shallow Lake Säkylän Pyhäjärvi, Finland, during the past ~400 years - implications for management. Hydrobiologia. doi: 10.1007/s10750-015-2552-2. [Epub ahead of print].
Ventelä, A.-M., Kirkkala, T., Lendasse, A., Tarvainen, M., Helminen, H., and Sarvala, J. (2011). Climate related challenges in long-term management of Säkylän Pyhäjärvi (SW Finland). Hydrobiologia 660, 49–58. doi: 10.1007/s10750-010-0415-4
Ventelä, A.-M., Tarvainen, M., Helminen, H., and Sarvala, J. (2007). Long-term management of Pyhäjärvi (southwest Finland): eutrophication, restoration – recovery? Lake Reserv. Manage. 23, 428–438. doi: 10.1080/07438140709354028
Wang, Q., Yang, X., Anderson, N. J., Zhang, E., and Li, Y. (2014). Diatom response to climate forcing of a deep, alpine lake (Lugu Hu, Yunnan, SW China) during the Last Glacial Maximum and its implications for understanding regional monsoon variability. Quaternary Sci. Rev. 86, 1–12. doi: 10.1016/j.quascirev.2013.12.024
Weckström, J., Korhola, A., and Blom, T. (1997a). Diatoms as quantitative indicators of pH and water temperature in subarctic Fennoscandian lakes. Hydrobiologia 347, 171–184. doi: 10.1023/A:1003091923476
Weckström, J., Korhola, A., and Blom, T. (1997b). The relationship between diatoms and water temperature in thirty subarctic Fennoscandian lakes. Arctic Alpine Res. 29, 75–92. doi: 10.2307/1551838
Weckström, K. (2006). Assessing recent eutrophication in coastal waters of the Gulf of Finland (Baltic Sea) using subfossil diatoms. J. Paleolimnol. 35, 571–592. doi: 10.1007/s10933-005-5264-1
Weckström, K., Juggins, S., and Korhola, A. (2004). Defining background nutrient concentrations for coastal waters of the Gulf of Finland, Baltic Sea. Ambio 33, 324–327. doi: 10.1579/0044-7447-33.6.324
Weckström, K., Korhola, A., and Weckström, J. (2007). Impacts of eutrophication on diatom species richness in coastal waters of the Baltic Sea. Ambio 36, 155–160. doi: 10.1579/0044-7447(2007)36[155:IOEODL]2.0.CO;2
Whitmore, T. J., Brenner, M., Jiang, Z., Curtis, J. H., Moore, A. M., Engstrom, D. R., et al. (1997). Water quality and sediment geochemistry in lakes of Yunnan Province, southern China. Environ. Geol. 32, 45–55. doi: 10.1007/s002540050192
Witkowski, A. (1994). Recent and Fossil Diatom Flora of the Gulf of Gdansk, Southern Baltic Sea. Origin, Composition and Changes of Diatom Assemblages During the Holocene. Bibliotheca Diatomologica, 28. Berlin: J. Cramer.
Witkowski, A., Lange-Bertalot, H., and Metzeltin, D. (2000). “Diatom flora of marine coasts I,” in Iconographia Diatomologica, Annotated Diatom Micrographs 7, ed H. Lange-Bertalot (Ruggell: A R.G. Gantner Verlag, K.G), 925.
Wu, G., Zhang, Q., Zheng, X., Mu, L., and Dai, L. (2008). Water quality of Lugu Lake: changes, causes and measurements. Int. J. Sustain. Dev. World Ecol. 15, 10–17. doi: 10.1080/13504500809469763
Xiao, X., Yang, X., Shen, J., Wang, S., Xue, B., and Tong, X. (2013). Vegetation history and dynamics in the middle reach of the Yangtze River during the last 1500 years revealed by sedimentary records from Taibai Lake, China. Holocene 23, 57–67. doi: 10.1177/0959683612450195
Yang, G. S., Ma, R. H., Zhang, L., Jiang, J. H., Yao, S. C., Zhang, M., et al. (2010). Lake status, major problems and protection strategy in China. J. Lake Sci. 22, 799–810.
Yu, Y., Zhang, M., Qian, S., Li, D., and Kong, F. (2010). Current status and development of water quality of lakes in Yunnan. J. Lake Sci. 22, 820–828.
Keywords: palaeolimnology, european water framework directive, diatoms, lake management, land-use, eutrophication, climate effects
Citation: Weckström J, Liao M, Yu G, Amsinck S, Kauppila T, Qin B, Zhu G, Sarvala J, Weckström K, Tarvainen M, Kirkkala T, Nurminen L and Ventelä A-M (2015) Responses of Aquatic Ecosystems to Environmental Changes in Finland and China. Front. Ecol. Evol. 3:126. doi: 10.3389/fevo.2015.00126
Received: 03 June 2015; Accepted: 19 October 2015;
Published: 12 November 2015.
Edited by:
Isabelle Larocque-Tobler, The L.A.K.E.S. Institute, SwitzerlandReviewed by:
Teresa Vegas-Vilarrúbia, University of Barcelona, SpainLaurent Millet, Centre National de la Recherche Scientifique Chrono-Environnement, France
Copyright © 2015 Weckström, Liao, Yu, Amsinck, Kauppila, Qin, Zhu, Sarvala, Weckström, Tarvainen, Kirkkala, Nurminen and Ventelä. This is an open-access article distributed under the terms of the Creative Commons Attribution License (CC BY). The use, distribution or reproduction in other forums is permitted, provided the original author(s) or licensor are credited and that the original publication in this journal is cited, in accordance with accepted academic practice. No use, distribution or reproduction is permitted which does not comply with these terms.
*Correspondence: Jan Weckström, jan.weckstrom@helsinki.fi