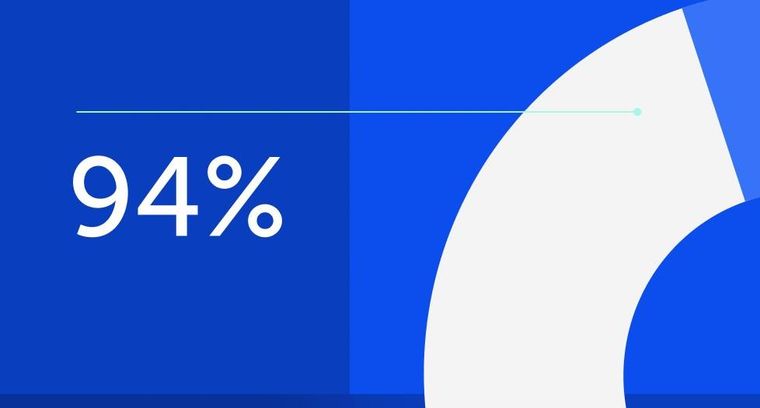
94% of researchers rate our articles as excellent or good
Learn more about the work of our research integrity team to safeguard the quality of each article we publish.
Find out more
PERSPECTIVE article
Front. Ecol. Evol., 08 September 2015
Sec. Urban Ecology
Volume 3 - 2015 | https://doi.org/10.3389/fevo.2015.00106
Green infrastructure consists of ecosystems that provide valuable services to urban areas. Constructed ecosystems, including green roofs, bioretention systems, constructed wetlands and bioreactors are artificial, custom-built components of green infrastructure that are becoming more common in cities. Small size, strong spatial boundaries, ecological novelty and the role of human design characterize all constructed ecosystems, influencing their functions and interactions with other urban ecosystems. Here I outline the relevance of ecology and evolution in understanding the functioning of constructed ecosystems. In turn, a research focus on the distinctive aspects of constructed ecosystems can contribute to fundamental science.
Green infrastructure originally referred to natural ecosystems in and around urban areas and the corridors that connect them (Weber and Wolf, 2000; Hostetler et al., 2011). Current definitions emphasize that green infrastructure is designed and managed in order to make a large contribution to urban ecosystem services (European Union, 2013). Usage of the term has expanded to include ecosystems constructed in the built environment that carry out evapotranspiration and other functions characteristic of natural ecosystems. Here, the built environment refers to buildings, roads and associated infrastructure such as parking lots, and is also referred to as “gray infrastructure” (Tanner et al., 2014). The built environment is usually characterized by hard, impermeable surfaces and these characteristics result in distinct changes to urban microclimates, hydrology, and soil properties relative to natural ecosystems. The green infrastructure components of the built environment include green roofs, living walls, bioretention systems such as bioswales or raingardens, water treatment wetlands and other artificial habitats (Table S1) (Gill et al., 2007). One of the “Grand Challenges” of urban ecology is to understand the built environment as a set of ecosystems that interact with each other and with more natural habitats in the urban matrix (Pataki, 2015). Especially in the urban core, constructed ecosystems form an important component of the ecology of cities.
Constructed ecosystems can be defined as engineered systems featuring interacting living and non-living components, designed to produce valuable services (Table S1) (Ranalli and Lundholm, 2008). Ecosystems such as green roofs and walls, raingardens and sewage treatment wetlands are obviously part of green infrastructure, as the ecosystem services produced contribute toward mitigating the negative environmental impact of cities. As components of city landscapes, energy, materials and organisms can flow between these systems and other urban ecosystems, warranting a landscape approach to constructed ecosystems (Braaker et al., 2014). Constructed ecosystems that occur primarily indoors, such as living walls, can also be considered a valuable part of green infrastructure as they provide ecosystem services and enhance human well-being (Table S1) (Pataki, 2015). Some constructed ecosystems, such as the various types of bioreactors and biofiltration systems increasingly used to treat wastewater, may require significant isolation from other ecosystems to carry out their functions and may have limited interaction between system components and organisms from outside. One of the key variables influencing the function of such ecosystems and their effect on urban ecology as a whole is thus the degree of connection with other ecosystems (Todd and Josephson, 1996; Lundholm, 2015a).
There is already a good deal known about the function of the natural components of green infrastructure, such as urban forest and wetland patches, but constructed ecosystems represent a relatively new addition to the field. I surveyed Google Scholar for various keywords related to urban ecology and green infrastructure. While references to the parent fields of ecology and evolution have stayed relatively constant over the last decade, references to constructed ecosystems have increased dramatically over the same period (Figure 1). The burgeoning interest in constructed ecosystems has been matched by an increase in their prevalence in urban landscapes (Soreanu et al., 2013; Liu et al., 2014; Williams et al., 2014; Wu et al., 2015), so a full understanding of urban ecology is incomplete without recognition of the structure and function of these components of green infrastructure. This paper provides an ecologist's perspective on the commonalities between seemingly disparate kinds of constructed ecosystem and how they differ from natural ecosystems. I outline the importance of ecological understanding in improving the functioning of constructed ecosystems. I also outline how the distinct features of constructed ecosystems can yield fundamental insight into ecology and evolution.
Figure 1. Google Scholar search hits for terms related to green infrastructure and constructed ecosystems for 5 year intervals.
As ecosystems, installations as different as green roofs or walls on building exteriors and membrane bioreactors deep inside water treatment facilities share key features. In each case, engineered components, such as the physical containers of bioreactors, are essential to the function of the system. These components directly impact ecosystem functioning, for example, engineered retention fabrics underlying green roofs contribute to their stormwater capture functions (Savi et al., 2013). They also support and influence the biological components of the systems. Human design is thus an essential factor underlying all constructed ecosystems. Constructed ecosystems feature relatively simple designs, compared with the obvious complexity of their natural counterparts. Indeed, some of these systems feature tight control over some aspects of the biological communities as well, as in treatment wetlands installed with single plant species, but there are always spontaneous dynamics that can affect community structure and ecological functioning as well, and the degree to which ongoing human intervention organizes these ecosystems can vary greatly, even within the same kind of ecosystem (Lundholm, 2015a). There is thus a “wild” element inherent to constructed ecosystems and complexity comes with this wildness despite human attempts at simplification and control.
In comparison to other highly human-dominated ecosystems, such as forest plantations or agricultural fields, constructed ecosystems tend to have extremely distinct spatial boundaries differentiating them from other ecosystems. This is inherent due to the physical containers for the individual ecosystems nested within the built environment (i.e., the edges of the building roof create a stark boundary for green roof systems) and the spatially heterogeneous configuration of the urban landscape itself (Alberti and Marzluff, 2004). Constructed ecosystems also tend to be relatively small and this leads to the relevance of treating them as ecological islands (McGuire et al., 2015). Being small may make these systems more sensitive to outside influences which could destabilize functioning (Ewel et al., 2013). While some degree of isolation from surrounding ecosystems is inherent in the spatial limits and small size of constructed ecosystems, we can recognize a continuum of isolation with outdoor ecosystems highly influenced by the flows of materials, energy and organisms from other ecosystems, to more self-contained, indoor ecosystems, such as bioreactors, which often require isolation to carry out their functions.
Like urban ecosystems in general, constructed ecosystems feature high levels of ecological novelty: combinations of species or environmental conditions that have not occurred in the evolutionary history of the organisms or populations involved (Carthey and Banks, 2014; Fridley and Sax, 2014; McGuire et al., 2015). In some cases, engineered features, small size, or isolation result in these novelties. If novelty results in conditions that exceed the tolerance of individual organisms, stress, reduced fitness, changes to community structure and consequences to ecosystem functioning can result (Speak et al., 2013; Li et al., 2015). However, it is also possible that novelty can favor certain species. Isolation may free a plant population from pests, leading to higher productivity (Hawkes, 2007) or select for species tolerant of pollution (McGuire et al., 2013). Land managers and agriculturalists have exploited ecological novelty to increase ecosystem functioning, for example, when nitrogen fixing crops are introduced to an area without native nitrogen fixers resulting in erosion control and increased productivity (Magnússon, 1997). Our understanding of ecological novelty is limited, and ecologists and designers of constructed ecosystems need to collaborate to determine whether we can take advantage of ecological novelty to improve ecosystem functioning.
A major cause of novelty in constructed ecosystems comes from their creation from scratch in disconnection from natural ecosystems, often resulting in a lack of biological legacy and ecological memory inherent to the dynamics of natural ecosystems (Schaefer, 2009). For terrestrial ecosystems, the soil represents an ecological reservoir containing seeds and an entire food web based on microbial activities, but natural soils rarely form the basis of plant-based constructed ecosystems like green roofs, walls and treatment wetlands. This lack of biological legacy or ecological memory may result in depauperate microbial communities (John et al., 2014) which could have profound effects on ecosystem functioning. The degree of isolation from other ecosystems may also influence the degree to which ecological memory of adjacent ecosystems can influence a constructed ecosystem. Design can also play a key role in whether biological legacy of natural ecosystems can contribute to constructed ecosystems. Green roofs using natural soils instead of the commonly applied artificial substrates represents a way of incorporating some ecological memory into a constructed ecosystem (Best et al., 2015). Bioaugmentation of bioreactor microbial consortiums is an analogous procedure in a different system (Todd and Josephson, 1996; Curtis and Sloan, 2004).
The growth of ecological engineering acknowledges the importance of merging ecology and design into green infrastructure (Mitsch and Jørgensen, 2003). Ecologists have become more involved with research in these areas (e.g., Graham and Smith, 2004), but there are compelling reasons for more involvement of ecologists and evolutionary biologists in these systems, especially in urban areas.
While I have argued that constructed ecosystems are distinct from natural and most agricultural ecosystems, a body of research suggests ecological insight derived from studies of other systems can be applied toward the improvement of constructed ecosystem functioning (Graham and Smith, 2004; McMahon et al., 2007; Cook-Patton, 2015). The composition of species taking part in constructed ecosystems plays a large role in their function (Iamchaturapatr et al., 2007; Liu et al., 2007; Lundholm and Williams, 2015), as it does in other ecosystems. Work by ecologists toward understanding the role of functional traits in ecosystem functioning could provide a general basis for selection of biological components to enhance constructed ecosystem functions (Lundholm et al., 2015; Storkey et al., 2015).
Ecologists have driven research into the value of biodiversity in the functioning of ecosystems for the last three decades. Including greater biodiversity in constructed ecosystems can improve their functionality (Akratos and Tsihrintzis, 2007; McMahon et al., 2007; Cook-Patton, 2015) but relatively few studies have documented these effects and the underlying mechanisms are relatively unknown. Multifunctionality, a key frontier of biodiversity-ecosystem functioning research (Hector and Bagchi, 2007), is especially important in understanding full cost-benefit accounting of constructed ecosystems (Carter and Keeler, 2008; Spatari et al., 2011), and is likely to be affected by the taxonomic or functional diversity of the component biota (Lundholm, 2015b). While the engineered components of these systems are essential to understanding their function, more collaborative involvement of ecologists is necessary to fully understand how they work and their contribution to green infrastructure. The degree of ongoing maintenance and design intervention can also affect ecosystem functions and research is needed to determine how much management vs. allowing spontaneous dynamics is optimal for any constructed ecosystem (Todd and Josephson, 1996; Mitsch and Jørgensen, 2003; Dunnett, 2015; Lundholm, 2015a).
Constructed ecosystems are becoming more common in urban areas. Green roofs, living walls, bioretention systems, vegetated pavements are increasingly components of city infrastructure targeting thermal moderation, infiltration, mitigation of runoff related problems, and softening the appearance of urban environments. Most of the research on these systems has been from the “inside-out,” in other words, focusing on the design engineering and function of the ecosystem. But these outdoor ecosystems interact with other components of the urban matrix, and are sufficiently prevalent in some cities that we can approach them from the “outside-in”: as habitats that take place within an urban landscape with interactions with and influences on surrounding urban ecosystems. One consequence of constructed green infrastructure built to provide thermal or hydrological functions is that they are used as habitat by species not originally included in their design, leading to their potential in biodiversity conservation initiatives (e.g., Williams et al., 2014).
Another consequence of the spread of constructed ecosystems is that they have become further embedded in the complex socio-ecological matrix of cities. Like all urban ecosystems, socio-economic forces also shape the design, placement and functioning of constructed ecosystems (Tanner et al., 2014). One of the key differences among the varied types of constructed ecosystems is the identity of their designers, with some [e.g., those contained in wastewater facilities (Table S1)] requiring high levels of technical expertise, precluding broad participation in their design. Other constructed ecosystems, such as green roofs and walls may facilitate more participatory design and interactions between experts and laypeople. Visibility and accessibility to urban citizens also varies greatly depending on the type of ecosystem and context of its placement within the built environment, possibly resulting in heterogeneous psychological and sociological impacts.
Constructed ecosystems can provide disservices as well. Green roofs may have negative impacts on species of conservation concern, for example if they act as ecological traps (MacIvor, 2015), attract nuisance wildlife (Fernandez-Canero and Gonzalez-Redondo, 2010), or are considered unattractive by local human populations (Loder, 2014). In some cases, the material and energy costs of constructed green infrastructure may outweigh their benefits, as in the case when green roofs provide too much heat sink, resulting in higher cooling costs in tropical environments (Jim, 2014), or when treatment wetlands result in significant release of greenhouse gases (Ström et al., 2007). Both “inside-out” and “outside-in” perspectives are important to understand the potential impact of constructed ecosystems on city ecology, and studies of the linkages between constructed ecosystem patches and other ecosystem in the urban matrix are essential (Braaker et al., 2014).
Urban home gardens have received considerable attention as ecosystems in the last decade (Cameron et al., 2012). In urban core environments, private horticulture is often restricted to containers placed on hard surfaces or suspended off the ground (Figure 2). The ecosystems created in this kind of microgreening represent constructed ecosystems as they are highly artificial environments, strongly spatially bounded, potentially very isolated from natural ecosystems and other green infrastructure, feature high levels of ecological novelty, and have less ecological memory than in-situ urban gardens based on local soils. Nevertheless, they provide ecosystem functions, especially visual relief and interest in otherwise hard-surfaced environments and should be studied to determine the overall contribution to urban ecosystem services. Container gardening is also important for food production in many parts of the world (Ghosh, 2004; Abegunde et al., 2009). Compared with many other types of constructed ecosystem, container gardens, like indoor potted plant ecosystems (e.g., Orwell et al., 2004) they are often designed and installed by ordinary citizens outside of the professional and academic fields of ecological engineering. The emphasis on green infrastructure to date has been on “big”: wide expanses of relatively natural forest, wetland or riverine habitats, or large organisms (trees in the urban forest) but the role of microgreening in food production (Hui, 2011), ameliorating microclimates (Hagishima et al., 2007), and providing visual relief (Kaplan, 2001; Groenewegen et al., 2006) may be important where it provides the only green infrastructure in the urban core and can be readily implemented by urban residents.
Figure 2. Examples of constructed ecosystems. (A) outdoor living wall; (B) indoor living wall; (C) intensive (deep growing medium) green roof; (D) extensive (shallow growing medium) green roof; (E) container gardens; (F) bioswale.
The restoration of damaged ecosystems has been called an “acid test” for ecology (Bradshaw, 1987), as sound predictive capability is a prerequisite to recreating structure and function. Others argued for a more general heuristic value of restoration ecology, citing that we can learn much from restoring ecosystems in a way that working non-manipulatively with relatively intact ecosystems cannot (Harper, 1987). This understanding has been extended to constructed ecosystems (Mitsch and Jørgensen, 2003; Graham and Smith, 2004). All ecosystems should be comprehensible using the same set of basic concepts, so learning about one kind of ecosystem should generate knowledge that can improve general understanding of how ecosystems work, with the caveat that many of the details are system-specific and dependent on local context and historical contingency.
The goal-driven nature of constructed ecosystems often trains the focus of ecologists to different questions than are usually asked of natural ecosystems. For example, biodiversity-ecosystem functioning studies ask what happens to functioning when species are lost from an ecosystem, whereas constructed ecosystems usually begin simple and we ask what happens when we engineer biotic complexity into the system (Lundholm, 2015b). The range of ecosystem functions produced by constructed ecosystems is considerably broad, thus many questions about the function of these systems cannot simply be answered by resorting to the literature on natural systems, because the same questions have not been asked (Quijas et al., 2010). As an example, most biodiversity-ecosystem functioning work on terrestrial systems emphasizes productivity and biomass whereas other physical properties of constructed ecosystems, such as green roof optical properties (Lundholm and Williams, 2015), may be important to their functioning. So constructed ecosystems can direct research into new areas. Likewise, constructed ecosystems may offer a further advantage compared with ecological restoration activities for generating ecological understanding in that there is often no biological legacy to begin with. We can thus get at the importance of ecological memory and legacy by comparing systems that differ in the extent to which they receive subsidies from natural populations, communities, and ecosystems. In general, focusing on the ways in which constructed ecosystems differ from others may be a productive approach: we can examine the ways in which isolation, novelty, and varying amounts of biological legacy influence the structure and function of constructed ecosystems. A further logistical advantage is that manipulations can involve the entire system itself, rather than relying on simplified microcosms of much larger natural ecosystems (Graham and Smith, 2004; Oberndorfer et al., 2007).
While much of this research is necessary to increase our general understanding of how constructed ecosystems function, understanding the effects of the distinct features of constructed ecosystems in a general sense may require comparisons with other ecosystems that share some similarities with constructed ecosystems. Natural or urban habitats with similar physical and chemical properties to constructed ecosystems have been used as templates for selection of biota for the artificial ecosystems (Lundholm and Richardson, 2010) and these may warrant further comparison. This has begun with urban infrastructure like green roofs where ecologists have sought to compare ecological processes in constructed versus other urban ecosystems (Ksiazek et al., 2012; Quispe and Fenoglio, 2015) and determine whether the habitat value for species of conservation concern is equivalent to that of ground-level habitats (Colla et al., 2009).
While the focus on ecosystem processes requires an ecosystem ecology approach to constructed ecosystems, several key features of these systems suggest that evolutionary studies are also highly relevant, as in other urban ecosystems (Tanner et al., 2014). There is reason to believe that selection pressures may be particularly high in constructed ecosystems, due to relatively homogeneous conditions and high levels of ecological novelty, and other features such as isolation and small population sizes can enhance drift effects, much like in natural islands (Ewel et al., 2013), leading to rapid evolution. The growing emphasis on evolutionary processes that shape ecosystem functioning (Lipowsky et al., 2011; Srivastava et al., 2012; Schöb et al., 2015) also warrants attention given the importance of ecosystem service provisioning inherent to constructed ecosystems. Evolution in these novel environments may have produced location-specific genotypes, for example, green roofs in Berlin have been present for over 100 years (Köhler and Poll, 2010), more than enough time for genetic divergence between plant populations. Again, the potential for such evolution, should it prove to be widespread, is interesting in its ability to shed light on general evolutionary processes (Cheptou et al., 2008), but it is also of relevance to the functioning of constructed ecosystems, for example, when genetic diversity within a population drives ecosystem-level processes (Cook-Patton et al., 2011).
Constructed ecosystems are key components of green infrastructure in cities. The science underlying the functioning of these ecosystems has progressed rapidly but there is still much to be gained by greater involvement of ecologists and evolutionary biologists. Ecology, evolution and evolutionary ecology are necessary for understanding constructed ecosystems themselves, and as elements interacting with other ecosystems in urban landscapes. As with the rest of urban ecology, it goes without saying that interdisciplinary understanding is required to push forward our understanding of the value of constructed ecosystems, and biological scientists will continue to work with physical and social scientists to generate a more comprehensive understanding of green infrastructure. We can hope that recognizing these artificial creations as ecosystems will also lead to a better understanding of basic evolutionary and ecological principles.
This work was funded by National Science and Engineering Research Council (Canada) Discovery Grant 311788-2010.
The author declares that the research was conducted in the absence of any commercial or financial relationships that could be construed as a potential conflict of interest.
The Supplementary Material for this article can be found online at: http://journal.frontiersin.org/article/10.3389/fevo.2015.00106
Abegunde, A. A., Omisore, E. O., Oluodo, O. F., and Olaleye, D. (2009). Commercial horticultural practice in Nigeria: its socio-spatial effects in Lagos city. Afr. J. Agricult. Res. 4, 1064–1072. Available online at: http://www.academicjournals.org/journal/AJAR/article-abstract/BB7CB2D35762
Akratos, C. S., and Tsihrintzis, V. A. (2007). Effect of temperature, HRT, vegetation and porous media on removal efficiency of pilot-scale horizontal subsurface flow constructed wetlands. Ecol. Eng. 29, 173–191. doi: 10.1016/j.ecoleng.2006.06.013
Alberti, M., and Marzluff, J. M. (2004). Ecological resilience in urban ecosystems: linking urban patterns to human and ecological functions. Urban Ecosyst. 7, 241–265. doi: 10.1023/B:UECO.0000044038.90173.c6
Best, B. B., Swadek, R. K., and Burgess, T. L. (2015). “Soil-based green roofs,” in Green Roof Ecosystems, ed. R. Sutton (New York, NY: Springer International Publishing), 139–174.
Braaker, S., Ghazoul, J., Obrist, M. K., and Moretti, M. (2014). Habitat connectivity shapes urban arthropod communities: the key role of green roofs. Ecology 95, 1010–1021. doi: 10.1890/13-0705.1
Bradshaw, A. D. (1987). “The reclamation of derelict land and the ecology of ecosystems,” in Restoration Ecology: A Synthetic Approach to Ecological Research, eds W. R. Jordan, M. E. Gilpin, and D. Aber (Cambridge, UK: Cambridge University Press), 53–74.
Cameron, R. W., Blanuša, T., Taylor, J. E., Salisbury, A., Halstead, A. J., Henricot, B., et al. (2012). The domestic garden–Its contribution to urban green infrastructure. Urban For. Urban Gree. 11, 129–137. doi: 10.1016/j.ufug.2012.01.002
Carter, T., and Keeler, A. (2008). Life-cycle cost–benefit analysis of extensive vegetated roof systems. J. Environ. Manag. 87, 350–363. doi: 10.1016/j.jenvman.2007.01.024
Carthey, A. J., and Banks, P. B. (2014). Naïveté in novel ecological interactions: lessons from theory and experimental evidence. Biol. Rev. 89, 932–949. doi: 10.1111/brv.12087
Cheptou, P. O., Carrue, O., Rouifed, S., and Cantarel, A. (2008). Rapid evolution of seed dispersal in an urban environment in the weed Crepis sancta. Proc. Natl. Acad. Sci. U.S.A. 105, 3796–3799. doi: 10.1073/pnas.0708446105
Colla, S. R., Willis, E., and Packer, L. (2009). Can green roofs provide habitat for urban bees (Hymenoptera: Apidae)? Cities Envir. 2, 1–12. Available online at: http://digitalcommons.lmu.edu/cgi/viewcontent.cgi?article=1017&context=cate
Cook-Patton, S. C., McArt, S. H., Parachnowitsch, A. L., Thaler, J. S., and Agrawal, A. A. (2011). A direct comparison of the consequences of plant genotypic and species diversity on communities and ecosystem function. Ecology 92, 915–923. doi: 10.1890/10-0999.1
Cook-Patton, S. C. (2015). “Plant biodiversity on green roofs,” in Green Roof Ecosystems, ed R. Sutton (New York, NY: Springer International Publishing), 193–209.
Curtis, T. P., and Sloan, W. T. (2004). Prokaryotic diversity and its limits: microbial community structure in nature and implications for microbial ecology. Curr. Opin. Microbiol. 7, 221–226. doi: 10.1016/j.mib.2004.04.010
Dunnett, N. (2015). “Ruderal green roofs,” in Green Roof Ecosystems, ed R. Sutton (New York, NY: Springer International Publishing), 233–255.
European Union. (2013). Building a Green Infrastructure for Europe. Brussels: Publications Office of the European Union.
Ewel, J. J., Mascaro, J., Kueffer, C., Lugo, A. E., Lach, L., and Gardener, M. R. (2013). “Islands: where novelty is the norm,” in Novel Ecosystems: Intervening in the New Ecological World Order, eds R. J. Hobbs, E. S. Higgs, and C. Hall (Chichester, UK: John Wiley & Sons), 29–44.
Fernandez-Canero, R., and Gonzalez-Redondo, P. (2010). Green roofs as a habitat for birds: a review. J. Anim. Vet. Adv. 9, 2041–2052. doi: 10.3923/javaa.2010.2041.2052
Fridley, J. D., and Sax, D. F. (2014). The imbalance of nature: revisiting a Darwinian framework for invasion biology. Global Ecol. Biogeogr. 23, 1157–1166. doi: 10.1111/geb.12221
Ghosh, S. (2004). Food production in cities. Acta Horticult. 643, 233–239. doi: 10.17660/actahortic.2004.643.30
Gill, S. E., Handley, J. F., Ennos, A. R., and Pauleit, S. (2007). Adapting cities for climate change: the role of the green infrastructure. Built Environ. 33, 115–133. doi: 10.2148/benv.33.1.115
Graham, D. W., and Smith, V. H. (2004). Designed ecosystem services: application of ecological principles in wastewater treatment engineering. Front. Ecol. Environ. 2, 199–206. doi: 10.1890/1540-9295(2004)002[0199:DESAOE]2.0.CO;2
Groenewegen, P. P., van den Berg, A. E., de Vries, S., and Verheij, R. A. (2006). Vitamin G: effects of green space on health, well-being, and social safety. BMC Public Health 6:149. doi: 10.1186/1471-2458-6-149
Hagishima, A., Narita, K. I., and Tanimoto, J. (2007). Field experiment on transpiration from isolated urban plants. Hydrol. Process. 21, 1217–1222. doi: 10.1002/hyp.6681
Harper, J. L. (1987). “The heuristic value of ecological restoration,” in Restoration Ecology: A Synthetic Approach to Ecological Research, eds W. R. Jordan, M. E. Gilpin, and D. Aber (Cambridge, UK: Cambridge University Press), 35–46.
Hawkes, C. V. (2007). Are invaders moving targets? The generality and persistence of advantages in size, reproduction, and enemy release in invasive plant species with time since introduction. Am. Nat. 170, 832–843. doi: 10.1086/522842
Hector, A., and Bagchi, R. (2007). Biodiversity and ecosystem multifunctionality. Nature 448, 188–190. doi: 10.1038/nature05947
Hostetler, M., Allen, W., and Meurk, C. (2011). Conserving urban biodiversity? Creating green infrastructure is only the first step. Land. Urban Plann. 100, 369–371. doi: 10.1016/j.landurbplan.2011.01.011
Hui, S. C. (2011). “Green roof urban farming for buildings in high-density urban cities,” in Hainan China World Green Roof Conference 2011 (Hong Kong), 1–9.
Iamchaturapatr, J., Yi, S. W., and Rhee, J. S. (2007). Nutrient removals by 21 aquatic plants for vertical free surface-flow (VFS) constructed wetland. Ecol. Eng. 29, 287–293. doi: 10.1016/j.ecoleng.2006.09.010
Jim, C. Y. (2014). Heat-sink effect and indoor warming imposed by tropical extensive green roof. Ecol. Eng. 62, 1–12. doi: 10.1016/j.ecoleng.2013.10.022
John, J., Lundholm, J., and Kernaghan, G. (2014). Colonization of green roof plants by mycorrhizal and root endophytic fungi. Ecol. Eng. 71, 651–659. doi: 10.1016/j.ecoleng.2014.08.012
Kaplan, R. (2001). The nature of the view from home: psychological benefits. Environ. Behav. 33, 507–542. doi: 10.1177/00139160121973115
Köhler, M., and Poll, P. H. (2010). Long-term performance of selected old Berlin greenroofs in comparison to younger extensive greenroofs in Berlin. Ecol. Eng. 36, 722–729. doi: 10.1016/j.ecoleng.2009.12.019
Ksiazek, K., Fant, J., and Skogen, K. (2012). An assessment of pollen limitation on Chicago green roofs. Landsc. Urban Plan. 107, 401–408. doi: 10.1016/j.landurbplan.2012.07.008
Li, L., He, Q., Ma, Y., Wang, X., and Peng, X. (2015). Dynamics of microbial community in a mesophilic anaerobic digester treating food waste: relationship between community structure and process stability. Bioresour. Technol. 189, 113–120. doi: 10.1016/j.biortech.2015.04.015
Lipowsky, A., Schmid, B., and Roscher, C. (2011). Selection for monoculture and mixture genotypes in a biodiversity experiment. Basic Appl. Ecol. 12, 360–371. doi: 10.1016/j.baae.2011.03.005
Liu, J., Sample, D. J., Bell, C., and Guan, Y. (2014). Review and research needs of bioretention used for the treatment of urban stormwater. Water 6, 1069–1099. doi: 10.3390/w6041069
Liu, Y.J., Mu, Y.J., Zhu, Y.G., Ding, H., and Crystal Arens, N. (2007). Which ornamental plant species effectively remove benzene from indoor air? Atmos. Environ. 41, 650–654. doi: 10.1016/j.atmosenv.2006.08.001
Loder, A. (2014). ‘There’s a meadow outside my workplace': a phenomenological exploration of aesthetics and green roofs in Chicago and Toronto. Land. Urban Plann. 126, 94–106. doi: 10.1016/j.landurbplan.2014.01.008
Lundholm, J. T. (2015a). Spontaneous dynamics and wild design in green roofs. Israel J. Ecol. Evol. doi: 10.1080/15659801.2015.1025511. [Epub ahead of print].
Lundholm, J. T. (2015b). Green roof plant species diversity improves ecosystem multifunctionality. J. Appl. Ecol. 52, 726–734. doi: 10.1111/1365-2664.12425
Lundholm, J. T., and Richardson, P. J. (2010). Habitat analogues for reconciliation ecology in urban and industrial environments. J. Appl. Ecol. 47, 966–975. doi: 10.1111/j.1365-2664.2010.01857.x
Lundholm, J., Tran, S., and Gebert, L. (2015). Plant functional traits predict green roof ecosystem services. Environ. Sci. Technol. 49, 2366–2374. doi: 10.1021/es505426z
Lundholm, J. T., and Williams, N. S. (2015). “Effects of vegetation on green roof ecosystem services,” in Green Roof Ecosystems, ed R. Sutton (New York, NY: Springer International Publishing), 211–232.
MacIvor, J. S. (2015). Building height matters: nesting activity of bees and wasps on vegetated roofs. Israel J. Ecol. Evol. doi: 10.1080/15659801.2015.1052635. [Epub ahead of print].
Magnússon, S.H. (1997). “Restoration of eroded areas in Iceland,” in Restoration Ecology and Sustainable Development, eds K. M. Urbanska, N. R. Webb, and P. J. Edwards (Cambridge, UK: Cambridge University Press), 188–211.
McGuire, K. L., Payne, S. G., Palmer, M. I., Gillikin, C. M., Keefe, D., Kim, S. J., et al. (2013). Digging the New York City skyline: soil fungal communities in green roofs and city parks. PLoS ONE 8:e58020. doi: 10.1371/journal.pone.0058020
McGuire, K. L., Payne, S. G., Orazi, G., and Palmer, M. I. (2015). “Bacteria and fungi in green roof ecosystems,” in Green Roof Ecosystems, ed R. Sutton (New York, NY: Springer International Publishing), 175–191.
McMahon, K. D., Martin, H. G., and Hugenholtz, P. (2007). Integrating ecology into biotechnology. Curr. Opin. Biotechnol. 18, 287–292. doi: 10.1016/j.copbio.2007.04.007
Mitsch, W. J., and Jørgensen, S. E. (2003). Ecological engineering: a field whose time has come. Ecol. Eng. 20, 363–377. doi: 10.1016/j.ecoleng.2003.05.001
Oberndorfer, E., Lundholm, J., Bass, B., Coffman, R. R., Doshi, H., Dunnet, N., et al. (2007). Green roofs as urban ecosystems: ecological structures, functions, and services. Bioscience 57, 823–833 doi: 10.1641/B571005
Orwell, R., Wood, R., Tarran, J., Torpy, F., and Burchett, M. (2004). Removal of benzene by the indoor plant/substrate microcosm and implications for air quality. WaterAir Soil Pollut. 157, 193–207. doi: 10.1023/B:WATE.0000038896.55713.5b
Pataki, D. E. (2015). Grand challenges in urban ecology. Front. Ecol. Evol. 3:57. doi: 10.3389/fevo.2015.00057
Quijas, S., Schmid, B., and Balvanera, P. (2010). Plant diversity enhances pro- vision of ecosystem services: a new synthesis. Basic Appl. Ecol. 11, 582–593. doi: 10.1016/j.baae.2010.06.009
Quispe, I., and Fenoglio, M. S. (2015). Host–parasitoid interactions on urban roofs: an experimental evaluation to determine plant patch colonisation and resource exploitation. Insect Conserv. Div. doi: 10.1111/icad.12127. [Epub ahead of print].
Ranalli, M., and Lundholm, J. (2008). Biodiversity and ecosystem function in constructed ecosystems. CAB Rev. Perspect. Agricult. Vet. Sci. Nutr. Nat. Resour. 3, 1–16. doi: 10.1079/PAVSNNR20083034
Savi, T., Andri, S., and Nardini, A. (2013). Impact of different green roof layering on plant water status and drought survival. Ecol. Eng. 57, 188–196. doi: 10.1016/j.ecoleng.2013.04.048
Schaefer, V. (2009). Alien invasions, ecological restoration in cities and the loss of ecological memory. Restorat. Ecol. 17, 171–176. doi: 10.1111/j.1526-100X.2008.00513.x
Schöb, C., Kerle, S., Karley, A. J., Morcillo, L., Pakeman, R. J., Newton, A. C., et al. (2015). Intraspecific genetic diversity and composition modify species-level diversity– productivity relationships. New Phytol. 205, 720–730. doi: 10.1111/nph.13043
Soreanu, G., Dixon, M., and Darlington, A. (2013). Botanical biofiltration of indoor gaseous pollutants - a mini-review. Chem. Eng. J. 229, 585–594. doi: 10.1016/j.cej.2013.06.074
Spatari, S., Yu, Z., and Montalto, F. A. (2011). Life cycle implications of urban green infrastructure. Environ. Pollut. 159, 2174–2179. doi: 10.1016/j.envpol.2011.01.015
Speak, A. F., Rothwell, J. J., Lindley, S. J., and Smith, C. L. (2013). Reduction of the urban cooling effects of an intensive green roof due to vegetation damage. Urban Climate 3, 40–55. doi: 10.1016/j.uclim.2013.01.001
Srivastava, D. S., Cadotte, M. W., MacDonald, A. A. M., Marushia, R. G., and Mirotchnick, N. (2012). Phylogenetic diversity and the functioning of ecosystems. Ecol. Lett. 15, 637–648. doi: 10.1111/j.1461-0248.2012.01795.x
Storkey, J., Döring, T., Baddeley, J., Collins, R., Roderick, S., Jones, H., et al. (2015). Engineering a plant community to deliver multiple ecosystem services. Ecol. Appl. 25, 1034–1043. doi: 10.1890/14-1605.1
Ström, L., Lamppa, A., and Christensen, T. R. (2007). Greenhouse gas emissions from a constructed wetland in southern Sweden. Wetlands Ecol. Manag. 15, 43–50. doi: 10.1007/s11273-006-9010-x
Tanner, C. J., Adler, F. R., Grimm, N. B., Groffman, P. M., Levin, S. A., Munshi-South, J., et al. (2014). Urban ecology: advancing science and society. Front. Ecol. Environ. 12, 574–581. doi: 10.1890/140019
Todd, J., and Josephson, B. (1996). The design of living technologies for waste treatment. Ecol. Eng. 6, 109–136. doi: 10.1016/0925-8574(95)00054-2
Weber, T., and Wolf, J. (2000). Maryland's green infrastructure—using landscape assessment tools to identify a regional conservation strategy. Environ. Monit. Assess. 63, 265–277. doi: 10.1023/A:1006416523955
Williams, N. S., Lundholm, J., and Scott MacIvor, J. (2014). Do green roofs help urban biodiversity conservation? J. Appl. Ecol. 51, 1643–1649. doi: 10.1111/1365-2664.12333
Keywords: built environment, green roof, ecological engineering, treatment wetland, ecological novelty
Citation: Lundholm JT (2015) The ecology and evolution of constructed ecosystems as green infrastructure. Front. Ecol. Evol. 3:106. doi: 10.3389/fevo.2015.00106
Received: 30 June 2015; Accepted: 25 August 2015;
Published: 08 September 2015.
Edited by:
Joseph P. McFadden, University of California, Santa Barbara, USAReviewed by:
Jari Niemelä, University of Helsinki, FinlandCopyright © 2015 Lundholm. This is an open-access article distributed under the terms of the Creative Commons Attribution License (CC BY). The use, distribution or reproduction in other forums is permitted, provided the original author(s) or licensor are credited and that the original publication in this journal is cited, in accordance with accepted academic practice. No use, distribution or reproduction is permitted which does not comply with these terms.
*Correspondence: Jeremy T. Lundholm, Department of Biology, Saint Mary's University, 923 Robie Street, Halifax, NS B3H 3C3, Canada,amx1bmRob2xtQHNtdS5jYQ==
Disclaimer: All claims expressed in this article are solely those of the authors and do not necessarily represent those of their affiliated organizations, or those of the publisher, the editors and the reviewers. Any product that may be evaluated in this article or claim that may be made by its manufacturer is not guaranteed or endorsed by the publisher.
Research integrity at Frontiers
Learn more about the work of our research integrity team to safeguard the quality of each article we publish.