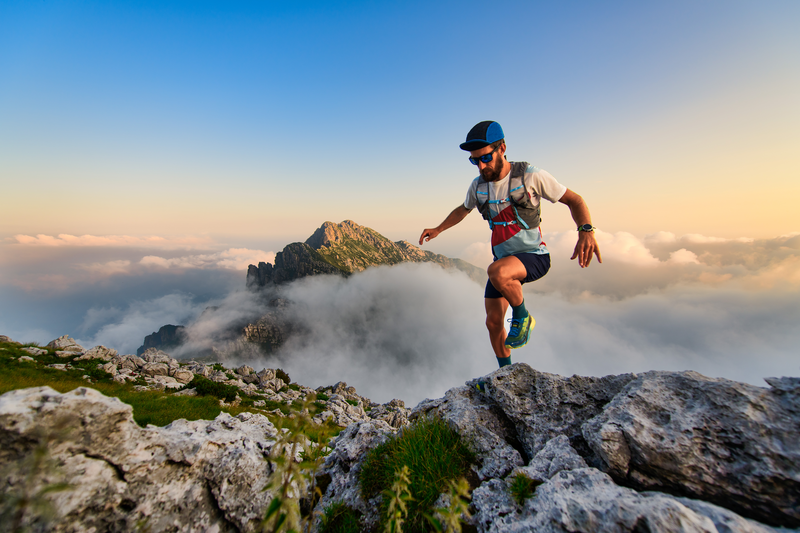
94% of researchers rate our articles as excellent or good
Learn more about the work of our research integrity team to safeguard the quality of each article we publish.
Find out more
ORIGINAL RESEARCH article
Front. Ecol. Evol. , 10 July 2015
Sec. Chemical Ecology
Volume 3 - 2015 | https://doi.org/10.3389/fevo.2015.00075
This article is part of the Research Topic Sexually Dimorphic Chemosensory Circuits and Behaviour in Insects View all 6 articles
Social living is beneficial because it allows conspecifics to interact in ways that increase their chances of survival and reproduction. A key mechanism underlying these benefits is the ability to recognize conspecifics; thus, allowing the production of coordinated social interactions. Identification of such individuals is often through chemical communication: the individuals' pheromonal profile indicates their sex, species, and even past experiences. However, we know little about how the chemosensory system of conspecifics detects and how the nervous system processes this information. One of the best documented pheromonal detection mechanisms is that of cis-Vaccenyl Acetate (cVA) made by male Drosophila melanogaster and transferred to females during mating. Sensing of cVA by males inhibits courtship behavior toward already mated females. Sensing of cVA on other males also inhibits courtship and increases aggression. In this hybrid review/research article, we discuss the pheromonal system of Drosophila putting an emphasis on the molecular and cellular mechanisms involved in cVA sensing by the olfactory system, perception by the nervous system and ultimately the regulation of social interactions. The behavioral effect of cVA is context- as well as experience-dependent leading us to conclude that cVA plays a modulatory role in regulating social interactions rather than being a recognition pheromone. We also provide new behavioral data on the function of the Odorant Binding Protein Lush, which binds cVA in olfactory sensilla and help sensing this chemical. Our data indicate that lush may be involved in the sensing of additional pheromones to cVA and suggest the existence of a lush-independent cVA detection system. Interpretation of our data in the light of our current knowledge about pheromonal recognition in Drosophila indicates that this system is incompletely understood.
Recognition of the identity and status of conspecifics permits coordinated behaviors, such as males displaying courtship toward females and not toward males. Several aspects of conspecific recognition in animals are mediated through pheromones: chemical signals produced by one individual that change the behavior of others. The molecular and cellular basis of pheromone-mediated recognition has been extensively dissected in the fruit fly Drosophila melanogaster (Billeter and Levine, 2013; Laturney and Billeter, 2014). In this species, pheromones vary in quantity and quality between individuals of different sexes and status. This variation is caused by genetic diversity (Ferveur et al., 1997; Marcillac et al., 2005a; Chertemps et al., 2006, 2007; Fernández et al., 2010), aging (Kuo et al., 2012) and exposure to environmental factors, such as diet (Fedina et al., 2012) and social experience (Butterworth, 1969; Kent et al., 2008; Krupp et al., 2008). The repertoire of pheromones displayed by an individual fruit fly appears to act as a biographical indicator that can be sensed by other flies, thereby informing their social interactions.
Flies produce an excess of 30 different hydrocarbons displayed on the surface of their cuticle hence called cuticular Hydrocarbons (CHs) (Yew et al., 2008; Everaerts et al., 2010; Levine et al., 2010) (Figure 1A). These CHs vary qualitatively and quantitatively between males and females as well as between Drosophila species (Antony and Jallon, 1982; Jallon and David, 1987). Cells called oenocytes located directly under the cuticle of the abdomen synthesize these CHs in adults (Billeter et al., 2009) (Figure 1A). Elimination of the oenocytes results in a dramatic reduction in CHs and a breakdown in recognition (Billeter et al., 2009). Wild-type D. melanogaster males display courtship and attempt to mate with both males and females that lack oenocytes (Oe−) (Billeter et al., 2009); and wild-type males exposed to Oe− males will court those males rather than display aggression toward them (Wang et al., 2011). Taken together, these data indicate that the Oenocytes produce a signal that conveys sexual identity. Furthermore, males from sibling Drosophila species will attempt to court and mate with D. melanogaster Oe− females indicating that Oenocytes also convey species identity (Savarit et al., 1999; Billeter et al., 2009).
Figure 1. Pheromonal system of Drosophila melanogaster. (A) A male engaging in courtship with a female taps her abdomen using his first pair of legs. The female abdomen contains cells called Oenocytes (green dots). (B) The olfactory system is located in the third antennal segment, and (C) is made of three distinct sensillum-types that differ in morphology. (D) The trichoid sensilla are a site of pheromone detection. The pheromone cVA (green dot) enters the sensillum and is bound to the Odorant Binding protein Lush. Lush is secreted by support cells (Gray cells) in the lymph of the sensillum. Lush allows cVA to bind to the Or67d receptor (pink dots) which forms a dimer with the Orco co-recptor (blue dot). Or67d and Orco are both expressed in the dendrite of the olfactory neuron (blue cell) that is housed in the sensillum. (E) The male leg possesses sensilla (black hair) that express gustatory receptors, such as Gr32a (blue dots). During tapping, these sensilla are in contact with the surface of the female abdomen, which is coated with cuticular hydrocarbons (blue and pink chemical structures).
Just as removing the Oenocytes from the fly showed us the role of these cells in sexual and species identification, placing specific CHs onto flies can reveal the role of each individual chemical. The recognition of Oe− flies by social partners can be manipulated by perfuming them with individual CHs. Attraction of D. melanogaster males to Oe− males is blocked by perfuming with the male CH 7-Tricosene (7-T), and restored male aggression (Miyamoto and Amrein, 2008; Billeter et al., 2009; Wang et al., 2011; Thistle et al., 2012; Fan et al., 2013). The attraction of males from other Drosophila species to Oe− females is delayed by perfuming them with the D. melanogaster female-specific CH 7,11-Heptacosadiene (7,11-HD). The results of these tests not only revealed the role of CHs in courtship behavior, they also indicated a more general rule for chemical communication in these species. The logic of sexual recognition in Drosophila appears to be based on a general attractiveness onto which single sex- and species-specific CHs are superimposed by the Oenocytes to block interactions that reduce evolutionary fitness, such as male–male courtship and interspecies courtship.
Although we are starting to understand the chemical signals that indicate an individual's identity, our understanding of how conspecifics sense and perceive these signals is limited. Flies engage in touching via their legs during social interactions, which has been associated with information transfer within groups of flies (Schneider et al., 2012; Ramdya et al., 2015). Part of the information appears to be mechanosensory and related to the sense of touch itself (Schneider et al., 2012; Ramdya et al., 2015). However, touching also has a chemosensory component. For instance, during courtship males tap females with their first pair of legs (Figure 1A). The fly legs and body are covered with hairs that contain taste receptors (Vosshall and Stocker, 2007) (Figure 1E). The taste receptor Gr32a is located on the first pair of legs (Figure 1E). D. melanogaster males lacking the gene encoding the Gr32a receptor court females from other species (Fan et al., 2013), court males from their own species (Miyamoto and Amrein, 2008) and have reduced aggression toward conspecific males (Wang et al., 2011; Andrews et al., 2014). Gr32a− mutant males are insensitive to 7-T indicating that Gr32a is a receptor for that pheromone (Wang et al., 2011). Although it probably has a mechanosensory component, one function of tapping during courtship appears to be connected to the chemosensory sensing of CH of conspecific and is in part connected to sex and species recognition (Kohatsu et al., 2011; Fan et al., 2013). The female-specific 7,11-HD pheromone, which is important for species-recognition, seems to be detected by gustatory neurons on the legs. Gustatory neurons expressing the ion-channels pickpocket-23 and −25 (ppk-23 and −25) respond physiologically to this pheromone (Starostina et al., 2012; Thistle et al., 2012; Toda et al., 2012). However, the role of ppk is not yet known: it may act as CH receptors or as effectors downstream of receptors for 7,11-HD and other CHs. Regardless of this complexity, it is clear that the family of Gustatory receptors (Ebbs and Amrein, 2007) as well as a novel family of Ionotropic chemosensory receptors (Koh et al., 2014) are implicated in CH detection in the legs. The observation that receptors for CHs are part of the gustatory and not the olfactory system indicate that CHs are detected upon contact between flies. The use of contact pheromones for recognition makes sense because it allows close association between an identity signal and the actual individual. A pheromone with high volatility would render association with a specific individual difficult, especially under the crowded condition in which flies often find themselves (Spieth, 1974). An important caveat in deducing the volatility of a chemical compound acting as pheromone is that its molecular weight alone is not a good predictor of volatility. There is evidence that CH have some degree of volatility despite their heavier molecular weight compared to most odorants opening the possibility that they may be also be detected by the olfactory system (Farine et al., 2012).
The D. melanogaster pheromone whose sensing and perception has been studied in most details is cis-Vaccenyl Acetate (cVA). cVA is produced outside of the oenocytes in a second pheromone-producing organ called the ejaculatory bulb, which is part of the male reproductive tract (Butterworth, 1969; Brieger and Butterworth, 1970). cVA is a unique pheromone in that it has a wide repertoire of behavioral functions. cVA is transferred to females together with the ejaculate during mating (Brieger and Butterworth, 1970). The presence of cVA on a mated female diminishes her attractiveness to males (Jallon, 1984; Kurtovic et al., 2007; Billeter et al., 2009), perhaps a form of chemical mate guarding by the male in an attempt to reduce female promiscuity. However, cVA does not simply function as a male repellant. The inhibitory effect of cVA on male courtship is enhanced by experience; naive males court virgins more than mated females, who have acquired cVA, but males that have the experience of rejection by mated females learn to further suppress courtship specifically toward mated but not virgin females (Siegel and Hall, 1979). This selective suppression is linked to an association by males between the presence of cVA on a female and her rejection behavior (Ejima et al., 2007), which enhances sensitivity to cVA and thus further reduces courtship (Keleman et al., 2012). The response to cVA is thus modified by the experience of prior exposure to that chemical.
The role of cVA extends to the regulation of male–male aggression. cVA increases aggression during sudden encounters between naive males (Wang and Anderson, 2010; Liu et al., 2011), which indicates that it is an aggression stimulating pheromone. However, long-term exposure to cVA reduces male–male aggressiveness (Liu et al., 2011), showing that response to this chemical is modified by experience. cVA thus functions in an experience-dependent manner in both courtship in aggression, whereby the length of exposure to cVA influences the level of these social behaviors.
There is a sex-specific processing component to the response to cVA (Ruta et al., 2010; Kohl et al., 2013). While cVA has a suppressive effect on male courtship, it acts as an aphrodisiac to females, who mate quicker with males when they sense this pheromone (Kurtovic et al., 2007; Ronderos and Smith, 2010). That cVA enhances male appeal to females but reduces female appeal to males indicates that it has opposite effects on male and female sexual attraction. This suggests that the sexes perceive this pheromone differently. Moreover, females are attracted to lay eggs nearby a source of cVA as the microdistribution of eggs within a food patch correlates with the microdistribution of cVA (Wertheim et al., 2006). Since this behavior can only be produced by females this is one more evidence for the sex-specific processing of cVA. We will see below that the processing of cVA is affected by developmental events that set up different neuronal connections and physiology in males and females (Ruta et al., 2010; Kohl et al., 2013).
Finally, cVA also has a non-sex specific function; in the presence of food, cVA promotes aggregation of both males and females (Bartelt et al., 1985; Wertheim et al., 2002; Xu et al., 2005; Lebreton et al., 2014). Again, prior experience of exposure to cVA has an effect in the context of aggregation. Females who have been chronically exposed to cVA are no longer attracted to aggregate around food laced with cVA (Lebreton et al., 2014). This phenomenon is of interest in the context of social niche construction: the ability to regulate one's social environment. It has been proposed that as cVA concentration increases so too does male–male aggression and that would result in increased dispersion suggesting a model for regulating group size via cVA (Wang and Anderson, 2010). Although the accuracy of this model awaits testing, the discovery of the experience-dependent effect of cVA suggests a modification. Because of the habituation to cVA it is possible that only new comer males would display aggression in a large group size, which might promote dispersal of resident males. As the number of flies who aggregate on a food patch and mate their increases, so does cVA concentration. Males also modulate the amount of cVA they produce or display depending on their social context, offering yet another way to modulate cVA concentration (Kent et al., 2008). Over time males become less aggressive, and mated females might become less attracted to males, both because they became less sensitive to cVA. Other factors are surely also at play such as the availability of food (Simon et al., 2011) and the genotype of males (Saltz and Foley, 2011), but cVA is likely part of a key mechanism in social niche construction.
We conclude that the perception of cVA has both an innate and experience-dependent component affecting social behaviors in Drosophila making it difficult to assign a specific function to this chemical.
How can cVA play a simultaneous role in male courtship suppression, female sexual receptivity and egg laying, male aggression and fly aggregation? The answer comes from the observation that cVA has little effect by itself. The first report of this phenomenon came from the work of Bartelt et al. (1985), who found that cVA had to be combined with food in order to attract flies. The aggregative effect of cVA comes from association with yeast-derived odorants (Xu et al., 2005; Schlief and Wilson, 2007; Lebreton et al., 2012, 2014). As yeast-derived odorants such as vinegar are attractive to flies in absence of cVA (Becher et al., 2012) but cVA is not attractive by itself (Bartelt et al., 1985), the role of cVA may be to increase the attractiveness of food rather than render it attractive.
cVA also functions in combination with the CH system. The female pheromone 7,11-HD can decrease the inhibitory effect of cVA on male courtship showing that males evaluate both chemicals when deciding how much to court a female (Billeter et al., 2009; Kohatsu et al., 2011). For male–male aggression, Wang et al. (2011) found that the 7-T pheromone dominantly controls behavioral responses to cVA. 7-T is essential for the aggression-promoting influence of cVA, as a males that cannot sense cVA still displays aggression but a male that cannot sense 7-T does not (Wang et al., 2011). The context-dependent roles of cVA thus depends on a combinatorial effect with other chemicals. In all these contexts cVA increases or decreases an attraction or repulsion that is already there. We conclude that cVA is better described as a gain regulator of the response to other pheromones than a classical pheromone.
The third segment of the antenna harbors hair-like structures called sensilla (Figure 1B) (Shanbhag et al., 1999), which house the dendrites of one to four Olfactory Receptor Neurons (ORN) (Couto et al., 2005; Fishilevich and Vosshall, 2005). A class of olfactory sensilla called trichoid differ anatomically from the two other main types of olfactory sensilla, basiconic, and coelonic (Figure 1C), and are thought to be the general site of volatile pheromones detection (van der Goes van Naters and Carlson, 2007; Vosshall and Stocker, 2007). The only identified pheromone detected by the olfactory system in Drosophila is cVA and is indeed detected by a subclass of trichoid sensilla called T1, which only contains one olfactory neuron expressing the odorant receptor Or67d (Couto et al., 2005; Fishilevich and Vosshall, 2005) (Figure 1D). In null mutants for the Or67d receptor gene, the electrophysiological response of T1 sensilla to cVA is absent indicating that Or67d is a receptor for that pheromone (Ha and Smith, 2006; Ejima et al., 2007; Kurtovic et al., 2007). The electrophysiological activity of the Or67d receptor relies on a pairing with the Orco co-receptor expressed in all classical olfactory neurons demonstrated by the observation that mutation in Orco abrogates the electrophysiological response of T1 sensilla to cVA (Figure 1D) (Ha and Smith, 2006; Benton et al., 2007; Jin et al., 2008). cVA is thus sensed by the olfactory system and by a specific type of trichoid sensillum.
How can males and females respond differently to cVA in the context of sex but not in the context of aggregation? Electrophysiological recordings from the T1 sensilla of males and females show the same electrophysiological response suggesting that males and females possess and use the same olfactory channel to detect this chemical (Kurtovic et al., 2007; van der Goes van Naters and Carlson, 2007). Differences in sensing are thus unlikely to be the basis for the sexually dimorphic response to that pheromone. Recordings from interneurons directly downstream of the Or67d Odorant receptor neurons reveals the same electrophysiological pattern of activation between males and females, indicating no sexual difference in neuronal coding of cVA signal immediately downstream of the first order olfactory neuron (Datta et al., 2008). However, these second order interneurons have different pre-synaptic projections that connect to different third-order neurons in males and females (Datta et al., 2008; Ruta et al., 2010; Kohl et al., 2013). These third order neurons have different developmental origins in males and females and respond differently to cVA (Kohl et al., 2013). The circuitry downstream of cVA sensing is therefore different between males and females, which likely is at the basis of the differences in behavioral responses to this chemical.
Investigation into the neuronal circuitry supporting olfactory information processing has shed light on how cVA can produce differences in behavioral responses between males and females. We mentioned earlier that cVA must be sensed in conjunction with 7-T in order to influence aggression. Mutants for the Gr32a receptor do not show aggression toward male flies, even though they are capable of sensing cVA (Wang et al., 2011). cVA is not sufficient to trigger aggression and this is supported by the observation that male mutants for the Or67d receptor still display aggression toward males (Wang et al., 2011). cVA instead is important for regulating the level of aggression, not the recognition of appropriate opponents. The means through which it does this is beginning to be revealed. Acute exposure to cVA increases aggression via its sensing by the Or67d receptor (Wang and Anderson, 2010; Liu et al., 2011), but long-term or chronic exposure to cVA reduces aggression through activation of a second cVA receptor called Or65a (Liu et al., 2011) expressed in other trichoid sensilla. The way Or65a modulate the response to cVA is elegantly shown by Lebreton et al. (2014). Long-term exposure to cVA activates the Or65a receptor neurons, which inhibits the interneurons that receive input from Or67d via inter-glomerular inhibition (Lebreton et al., 2014). The long-term response to cVA is thus the result of an inhibition of the output neurons of the Or67d receptor by the Or65a olfactory neuron. This system allows gain control over the response to cVA and fine regulation of aggression levels toward individual recognized as males by their expression of 7-T. cVA is thus not a recognition pheromone but a pheromone that modulates the intensity of specific social interactions including aggregation, egg-laying, courtship, and aggression. A key challenge in understanding how cVA is processed will be to understand where the cVA signal(s) is/are integrated in the brain with information coming from other sensory inputs such as food odorants and pheromones as well as touch, vision and internal states and how this integration modulates social behaviors.
Despite the wealth of information on the sensing and behavioral function of cVA, it is clear that we lack an understanding of how the sensing of cVA is integrated with that of other pheromones. This ultimately indicates that the logic of pheromonal communication in a species as well studied as D. melanogaster is still unclear. For instance, we indicated above that Oe− male and female flies remain attractive to wild-type males despite lacking CHs (Billeter et al., 2009). This suggests the presence of an unidentified pheromone attractive to males that is expressed in both sexes and not produced in the oenocytes. Interestingly, mutants for the Or47b receptor, another Or expressed in trichoid sensilla, are not attracted to Oe− flies (Couto et al., 2005; Fishilevich and Vosshall, 2005; Wang et al., 2011). A newly identified pheromone called methyl laurate made outside the Oenocytes by both males and females is detected by the Or47b and Or88a odorant receptors (Dweck et al., 2015). This new pheromone is detected by a trichoid sensillum like cVA. As Methyl laurate is activatory for male courtship and cVA is mostly inhibitory, these two pheromones might thus have different valence and help modulate male sexual behaviors.
The lush mutant was identified based on a behavioral defect in chemosensory attraction to alcohols (Kim et al., 1998) and was later shown to extend to social interactions. Normally, D. melanogaster aggregate around a common food source which is enhanced by the emission of cVA by flies already present on the substrate (Wertheim et al., 2006). However, a hypomorphic mutation in lush prevents Drosophila aggregation to a source of cVA demonstrating a role in social behavior (Xu et al., 2005). Mutations in lush results in a dramatically reduced electrophysiological response of the T1 sensilla and Or67d olfactory neuron to cVA (Xu et al., 2005; Ha and Smith, 2006; Gomez-Diaz et al., 2013). Sequence analysis of lush reveals that it is an Odorant Binding Protein, a type of protein expressed in chemosensory sensilla thats binds small odorants. Lush is expressed in the lymph of the sensilla, where it binds cVA and might shuttle this pheromone to the Or67d receptor (Figure 1D). The necessity of Lush for cVA sensing has led to the proposal that cVA and Lush together form the ligand for Or67d (Laughlin et al., 2008; Ronderos and Smith, 2010). In the context of courtship, a dominant-active mutant of lush results in an increased in Or67d neurons electrical spiking in absence of cVA, mimicking to some extend the exposure to cVA (Ronderos and Smith, 2010). Males expressing this mutation dramatically reduce their courtship toward virgin females, indicating that activated Lush can mimic the sensing of cVA on females and inhibit courtship in absence of that pheromone (Ronderos and Smith, 2010). However, this model fails to explain why the Or67d receptor in Lush mutants respond to cVA when presented at close range with a 70-fold concentration of cVA (Gomez-Diaz et al., 2013). In absence of Lush, an air stream passing through a source of cVA seven to 70 times more concentrated than that made by a single male (assuming ~500 ng cVA per male, Lebreton et al., 2014) fails to activate the Or67d receptor (Benton et al., 2007). This model implies a very specific interaction between cVA and Lush, but this fails to explain why Lush is present in all trichoid sensilla, including those that do not respond to cVA (Shanbhag et al., 2001).
The behavioral function of Lush in detecting pheromones has only been studied in the context of a response to cVA (Xu et al., 2005; Ronderos and Smith, 2010), leaving it unclear whether it mediates the detection of other pheromones. Lush is not only expressed in trichoid sensilla sensitive to cVA but in all trichoid sensilla (Shanbhag et al., 2001), indicating that this molecule is involved in the processing of additional odorants, or might have an unappreciated role in all trichoid sensilla. Lush is able to bind in vitro to the Bombyx mori pheromone Bombykol (Katti et al., 2012). As Bombykol is a long-chain hydrocarbon molecule similar in structure to D. melanogaster CHs, this raise the possibility that Lush participates in the detection of these pheromones. The lack of reported courtship defects in lush1 mutants males leaves this possibility untested. Here we investigated the role of Lush in sexual recognition and pheromone processing in response to both CH and cVA to investigate its contribution to the sensing of identified D. melanogaster pheromones.
All fly strains were reared on medium containing agar (10 g/L), glucose (167 mM), sucrose (44 mM), yeast (35 g/L), cornmeal (15 g/L), wheat germ (10 g/L), soya flour (10 g/L), molasses (30 g/L), propionic acid, and Tegosept in a 12:12 h light/dark cycle (LD 12:12) at 25°C. Virgin adults were collected shortly after eclosion using CO2 anesthesia and were kept in same-sex groups of 20 in food vials, unless stated otherwise, and aged for 6–7 days. The Canton-S strain was used as the wild-type D. melanogaster strain. lush1 (Kim et al., 1998) and orco2 (Larsson et al., 2004) mutant flies were placed into the Canton-S genetic background. Oenocyte-less (Oe−) adults were obtained from the progeny of the cross of “+: PromE(800)-Gal4, tubP-Gal80ts;+” to “+: UAS-StingerII, UAS-hid/CyO;+.” Control adults were obtained from the progeny of the cross of “+: PromE(800)-Gal4, tubP-Gal80ts;+” to “+: UAS-StingerII;+” (Billeter et al., 2009). Progeny from this cross were kept at 18°C until eclosion. Adult progeny were collected at room temperature and kept at 25°C for at least 24 h. Adults were subjected to three heat-treatments at 30°C (on days 2–4) during the day and returned to 25°C overnight. Adults were left to recover from these temperature treatments for 24 h before behavioral experiments.
For CH analysis, flies were anesthetized on ice and placed into individual glass microvials (Varian Inc., Palo Alto, CA) containing 50 μl of hexane spiked with 10 ng/ml each of octadecane (C18) and 10 ng/ml of hexacosane (C26) as internal standards. CHs were analyzed using a Varian CP3800 gas chromatograph with a flame ionization detector as described in Ejima et al. (2007). Varian Star Integrator software (Varian Inc., Palo alto, Ca) was used to quantify compounds based on peak areas.
Application of synthetic compounds was performed as described in Billeter et al. (2009). Control flies were processed as perfumed flies but sham treated by tumbling them in a perfuming vial without Hydrocarbon compounds added. High purity cVA (~99%) was obtained from Pherobank (Pherobank BV, The Netherlands) and applied at a final dose of 2000 μg/fly.
To determine the mating latency of males with females, one virgin male and female were aspirated into a 35 × 10 mm petri dish layered with food media. Flies where transferred to assay chambers using a mouth pipette, with the tip changed between flies to avoid CH contamination. Experiments began between Zeitgeber time (ZT) 7 and 8 and were housed in a temperature controlled chamber set at 25°C in a 12:12 light:dark cycle. Cameras (Hitachi CCD camera with the Northern eclipse software (v. 7.0) or Canon S10 camera using the ZoomBrowser EX software (Canon) took pictures of the dishes at 2-min intervals from the beginning of the experiment to determine the onset of copulation (Krupp et al., 2008, 2013; Billeter et al., 2012). The mating latency was calculated as the time elapsed between the introduction of the male and female in the dish and the beginning of copulation. A successful copulation was scored when a pair was seen in copulo in more than five successive frames (D. melanogaster mates for an average of 16 min). Red light was utilized to visualize the chambers during the dark phase.
Courtship assays of male–male D. melanogaster were performed in a cylindrical Plexiglas chamber (10 mm diameter by 5 mm depth). Pairs of flies were continuously video-recorded for 30 min from the moment of introduction. One experienced observer scored videos blind to the genotype or perfuming of the flies. A Courtship Index (CI) was determined as the fraction of a 10-min observation period spent by the male exhibiting courtship steps such as following, tapping, wing extension, licking, and attempting copulation. CI started from the first bout of courtship, defined as the first wing extension, and not from the moment of introduction. Flies that did not initiate courtship within 20 min were excluded because their CI could not be accurately determined. The courtship latency is determined as the time between introduction of the male and female to the first sign of courtship. Tester males were collected shortly after eclosion and aged individually for 6–7 day in small vials with diet to avoid reduction in courtship due to male–male courtship habituation.
Statistical analyses were performed with the GraphPad Prism software for Mac (version 5.0, GraphPad software inc.). Mating latency data were log-transformed, and courtship data arcsine-transformed to approximate a normal distribution unless the raw values defined a normal distribution. Normality was tested before and after transformation using Kolmogorov–Smirnov test. P-values were determined by ANOVA followed by the post-hoc Tukey–Kramer test when comparing multiple groups.
To determine the function of lush in male reproductive success, we housed a lush1 mutant or Canton-S wild-type male with a virgin control female and observed their latency to mate. Mating latency measures how long it takes for a male and a female to begin copulation. As such it measures both the ability of the male to successfully court and the female's receptivity to his courtship. The mating latency of lush1 males with control females is dramatically increased compared to Canton-S males (Figures 2A,B), suggesting that lush males are not attractive to females who otherwise mate normally with wild-type males. As lush is expressed in the olfactory system, it is likely that lush1 males fail to sense a female stimulatory pheromone and thus are slow at initiating or maintaining courtship resulting in delayed mating acceptance by females. Alternative hypotheses will be explored in the discussion.
Figure 2. Lush detects a female stimulatory compound. (A) Mean mating latency in pairs consisting of 1 virgin male and female of the indicated genotype. The number of replicates is indicate above the bar graphs. Error bars indicate Standard Error of the Mean (SEM). Bar graphs labeled with same letters are not significantly different from each other as determined by a One-Way ANOVA [ANOVA F(3, 59) = 74.4; P < 0.0001] followed by Tukey post-hoc test. (B) Cumulative percentage of pairs of virgin male and female of the indicated genotypes. Data is same as in (A) but plotted as cumulative mating to illustrate when most copulations take place.
To test the possibility that the female stimulatory pheromone that lush1 mutant mails fail to sense is a CH, we exposed lush1 males to Oe− females. If that pheromone was a CH we would expect lush1 males to have the same delayed mating latency as with Control females (Figures 2A,B). However, exposure of lush1 males to Oe− females resulted in a dramatic decrease in mating latency from 2 h with a control female to 10 min with an Oe− female (Figures 2A,B). The interaction between lush1 males and Oe− females suggests that Oe− females provide an attractive signal that is not a CH and that does not required lush to be sensed. These results therefore suggest the existence of two attractive female pheromones, one that may be a CH and one that is independent from that system.
We manipulated two factors in these experiments; the presence of functional lush in males and the presence of CH in females (Figure 2). Although both factors affect mating latency, statistical analysis indicates a significant interaction between the function of lush in males and the presence of CH pheromones in females [Two-Way ANOVA: lush in males × female CH: F(1, 59) = 18.97; P < 0.0001; lush in males: F(1, 59) = 136.02; P < 0.0001; female CH: F(1, 59) = 63.06; P < 0.0001]. This significant statistical interaction indicates that lush and the sensing by males of female CH are connected but that this connection is different depending on the presence or absence of both factors. Beside suggesting the existence of two independent female attractive signals, these experiments imply the existence of an inhibitory female CH which does not require lush for its sensing. This inhibitory CH pheromone would explain why lush1 males have such a long mating latency with control females that is dramatically reduced with Oe− females. These data suggest that Lush may help sensing distinct classes of pheromones in addition to cVA.
To directly test the function of lush in allowing the sensing of cVA, we tested the mating latency of wild-type and lush1 males with virgin females with a normal CH profile perfumed or not with 2 μg of cVA. This quantity is four times that found on recently mated females (Lebreton et al., 2014) and 30-fold less than the dose of cVA that demonstrably activate Or67d receptor in absence of Lush (Gomez-Diaz et al., 2013). This dose has been previously shown to reduce male mating latency (Zawistowski and Richmond, 1986; Billeter et al., 2009). We tested two factors; the genotype of the males (wild-type or lush1) and the presence or absence of cVA on virgin females (Figure 3). Wild-type males had a slower mating latency with virgin females perfumed with cVA than with virgin females not perfumed with cVA, consistent with an anti-aphrodisiac role of cVA (Figure 3A). lush1 males were equally slow to mate with cVA or non-cVA perfumed females, confirming that lush is required for cVA sensing and that the amount of cVA used to perfume flies is unlikely to artificially activate Or67d in absence of lush (Figure 3A). Statistical analysis shows a significant interaction between genotype of the males and the presence of cVA on females [Two-Way ANOVA male genotype × cVA on females F(2, 61) = 20.21; P < 0.0001; lush1 in males F(1, 61) = 87.67; P < 0.0001; cVA on females F(1, 61) = 28.41; P < 0.0001]. The significant statistical interaction between lush1 mutant males and CVA perfuming of females stems from the fact that lush1 males are slower than wild-type males at mating with both control females that are or not perfumed with cVA (Figure 3A). This again suggests that lush mediates not only the sensing of CVA but also that of an attractive signal that stimulates mating.
Figure 3. Lush detects cVA and an unknown inhibitory signal. (A) Mean mating latency in pairs consisting of 1 virgin male of the indicated genotype with one control female perfumed with 2000 ng of cVA or not perfumed. The number of replicates is indicated above the bar graphs. Error bars indicate Standard Error of the Mean (SEM). Bar graphs labeled with same letters are not significantly different from each other as determined by a One-Way ANOVA [ANOVA F(3, 61) = 46.88; P < 0.0001] followed by Tukey post-hoc test. (B) Mean mating latency in pairs consisting of 1 virgin male of the indicated genotype with one Oenocyte-less (Oe−) female perfumed or not with 2000 ng of cVA. The number of replicates is indicated above the bar graphs. Error bars indicate Standard Error of the Mean (SEM). Bar graphs labeled with same letters are not significantly different from each other as determined by a One-Way ANOVA [ANOVA F(3, 60) = 135.7; P < 0.0001] followed by Tukey post-hoc test.
We next tested the mating latency of wild-type males and lush1 males with virgin Oe− females perfumed or not with cVA (Figure 3B). As we previously documented, perfuming Oe− females with cVA dramatically reduced their attractiveness for a wild-type Canton-S male compared to perfuming a control female (Figure 3B) (Billeter et al., 2009). This suggests that female CHs normally mitigate the anti-aphrodisiac effect of that pheromone (Figure 3B). As the lush1 mutation blocks cVA-sensing (Xu et al., 2005), these mutant males should find both Oe− females that are or are not perfumed with cVA equally attractive and thus mate with them much faster than wild-type males. Strikingly, perfuming Oe− females with cVA also dramatically increased the mating latency of lush1 males. This result indicates that lush1 males are still able to detect cVA (Figure 3B). The perception of cVA is thus modified by the presence or absence of female CH indicating the presence of a lush-independent cVA sensing system. Statistical analysis backs up this conclusion as indicated by a lack of significant interaction between genotype of the male and the presence of cVA on Oe− females, but significant effect of male genotype and cVA on females [Two-Way ANOVA: Interaction male genotype × cVA on females: F(1, 60) = 3.37; P = 0.07; male genotype: F(1, 60) = 24.14; P < 0.0001; cVA on females: F(1, 60) = 347.77; P < 0.0001].
Our data on lush1 male mating latency with females indicate that lush is partly required for the response to the anti-aphrodisiac cVA but also to attractant pheromones: one that is made by the Oenocytes and thus likely to be a CH and one that belongs to an unidentified pheromonal system. To investigate the relationship between lush and the sensing of courtship stimulating compounds, we made use of the fact that Oe− males trigger strong courtship by wild-type Canton-S males (Billeter et al., 2009). Oe− males are devoid of CH but still express cVA at wild-type levels (Billeter et al., 2009) showing that cVA is not sufficient to suppress male courtship (Figure 4A).
Figure 4. Lush is not required for the sensing of identified stimulatory male or females CHs. (A) Male–male courtship index in pairs consisting of 2 virgin males of the indicated genotypes. (B) Latency to initiate courtship in males of the indicated genotypes with Oe− males. The number of replicates is indicated above the bar graphs. Error bars indicate Standard Error of the Mean (SEM).
We first tested the courtship behavior of wild-type, lush1 males or orco− males who lack a functional classical odorant receptor repertoire (Larsson et al., 2004; Benton et al., 2006), toward control and Oe− males. Surprisingly, we observed no difference in CI between male genotypes: none of these males courted control males and all of them courted Oe− males vigorously [Two-Way ANOVA: Tester male genotype: F(2, 134) = 0.09; P = 0.9; Target male CH: F(1, 134) = 144.47; P < 0.0001] (Figure 4A). This indicates that lush and Orco are not necessary to sense inhibitory pheromones made by the Oenocytes because they court control males at wild-type levels. Although they courted Oe− males at similar intensities than Orco− and wild-type males (Figure 4A), the time to first bout of courtship (the courtship latency) of lush1 males toward Oe− males was delayed compared to that of wild-type and orco− males (Figure 4B). The delayed courtship latency of lush1 mutants indicates that lush has a role in the sensing or perception of an attractive pheromone that is not made in the Oenocytes that stimulates courtship initiation.
Our experiments provide behavioral evidence that the Odorant Binding Protein lush is involved in pheromonal detection beyond that of cVA. Further, it shows that lush is not always necessary for the behavioral effect of cVA suggesting the existence of a lush-independent cVA sensing system. These data begin to explain the expression pattern of lush in trichoid sensilla that are not sensitive to cVA and thus suggest that lush participates in sensing more pheromones than just cVA. Additionally, our experiments suggest the existence of a complex pheromonal recognition system in D. melanogaster and of unidentified pheromones.
Virgin females lack cVA yet lush1 males have abnormally long mating latency with those females (Figures 2A,B). In absence of courtship data, delayed mating latency could be alternatively interpreted as the female not being willing to mate despite the best effort of lush1 males or that lush1 males do not exhibit strong courtship toward those females delaying mating acceptance. Direct observation of the courtship behavior of lush1 males toward Oe− males shows that lush1 males can produce level of courtship similar to wild-type males, but that they are delayed in initiating courtship (Figures 4A,B). We thus favor the hypothesis that lush1 males have delayed mating latency with females because they are slow at initiating courtship. This would indicate that lush is required to sense a stimulatory factor from wild-type virgin females. Because lush is expressed in the pheromone-sensing part of the olfactory system (Kim and Smith, 2001; Shanbhag et al., 2001; Benton et al., 2007), this factor is likely to be a volatile and a pheromone. If there was only one female stimulatory pheromone and it was a CH, lush1 males should be equally slow at mating with control females than Oe−. They are however much faster indicating the presence of a second non-CH stimulatory pheromone that does not require lush to be sensed. The proposal that females possess two attractive pheromonal systems fails to explain the dramatic increase in mating latency of lush1 with Control but not with Oe− females. This behavioral difference reveals the existence of an inhibitory pheromone made by the oenocytes. This conclusion is in line with our previous report that wild-type males mate quicker with Oe− females than control males indicating that the female oenocytes produce a pheromone with an inhibitory effect on males mating latency (Billeter et al., 2009). The extremely delayed mating latency of lush1 males with control females would thus be caused by two factors: an inability to sense a non-oenocyte-derived stimulatory pheromones, amplified by the ability to sense an inhibitory oenocyte-derived pheromone. We propose a model in which a balance between one repressive and two stimulatory female pheromones allows to fine tune male–female recognition by making attractiveness a graded response (Figure 5A).
Figure 5. Model of female pheromones sensing by males during courtship. (A) A balance between one repressive and two stimulatory female pheromones fine tunes male–female recognition and makes attractiveness a graded response. lush mutant males fail to perceive one stimulatory and one inhibitory cuticular hydrocarbon pheromone made by the Oenocyte (Oe), but still sense the second stimulatory pheromone not originating from the oenocytes. (B) lush mutant males may suppress courtship toward females perfumed with cVA because they can still sense cVA via a second sensory channel. The strong inhibitory effect of cVA-perfuming on Oenocyte-less (Oe−) females would be linked to a failure by males to sense the stimulatory pheromone coming from the CH system.
This model however does not take into account the possibility that males regulate their behavior based on sensing their own cVA levels. Sensing by the male of his own cVA may normally interact with female pheromones determining his own level of sexual arousal. While it is an untested hypothesis, testing it would be difficult for two reason. The first is that sensing of cVA by males normally reduces their courtship and aggression levels (Kurtovic et al., 2007; Wang and Anderson, 2010; Liu et al., 2011). A mutation affecting a male's ability to sense his own cVA could thus be expected to result in an increased courtship toward both males and females, but that is not the case in our experiments (Figures 2, 4). Second, it is unlikely that males can continuously sense their own cVA because it only seems to be released in the environment during social interactions indicating that males can control the release of this compound (Everaerts et al., 2010).
The role of lush in cVA sensing is well documented. However, the suppression of lush1 male mating latency with cVA-perfumed Oe− females shows that these males can still sense cVA. This observation leads us to suggest the existence of a lush-independent cVA sensing system (Figure 5B). As lush is expressed in all trichoid sensilla (Shanbhag et al., 2001), it is unlikely that this secondary cVA system is the Or65a receptor, which has been shown to respond to chronic exposure to cVA and dampen response by the Or67d receptor (Liu et al., 2011; Lebreton et al., 2014). Despite a lack of electrophysiological data, it is possible that lush would impact the function of Or65a, given that it is expressed in the trichoid sensilla that house this receptor (Shanbhag et al., 2001; Couto et al., 2005). So what could this secondary system be? Thistle et al. showed that cVA can activate ppk23-positive taste neurons on the male foreleg (Thistle et al., 2012). lush mutant males may suppress courtship toward Oe− females perfumed with cVA because they can still sense cVA through their taste system. The reason why lush1 males are strongly inhibited by cVA perfumed control females would be linked to a failure to sense the stimulatory pheromone coming from the CH system (Figure 5B).
An alternative explanation for our findings is that the dose of cVA we used can activate Or67d in absence of Lush. It has been shown that a 30-fold dose applied on a piece of paper and brought a close proximity to the T1 sensilla can activate electrophysiological response of the Or67d receptor in absence of Lush (Gomez-Diaz et al., 2013). While we perfumed flies with a much lower dose (four-fold higher than natural levels) we really do not know how much cVA is normally available to males during courtship given that most cVA in mated females might be present inside the female and not outside (Lebreton et al., 2014). We therefore do not know in how much excess our cVA perfuming, which is applied on the surface of the female, is compared to natural levels. This illustrates how little we really know about the location and availability of cVA in general.
The interaction of lush mutant males with female pheromones reveals an unexpected complexity in pheromone processing in Drosophila. Compounds like cVA are detected by two separate receptors in the olfactory system and may also be sensed by the taste system (Ha and Smith, 2006; Kurtovic et al., 2007; van der Goes van Naters and Carlson, 2007; Liu et al., 2011; Thistle et al., 2012; Lebreton et al., 2014). Detection of the same pheromone by both the olfactory and taste systems may increase the range of functions of a single compound. For instance, at longer range the smell of cVA combined with the smell of food triggers attraction to food substrate already occupied by flies (Bartelt et al., 1985; Wertheim et al., 2002; Lebreton et al., 2012). In the meantime, the taste system would not be able to detect low concentrations of cVA at such a long distance. At close contact, male or female CH and cVA might be sensed by both the gustatory and olfactory systems (Figure 1A). Pheromonal detection by the olfactory systems might indicate the environmental concentration of cVA allowing to determine the number of individual in a group and the taste system being more directed would identify specific social partners. Integration of these two senses would result in modulation of the intensity of social interactions with a specific individual, while taking into account the social environment. This is perhaps not so far fetched as pheromones like 7-T and cVA have a degree of volatility and can even be deposited on the substrate acting as good environmental indicator of group composition and size (Farine et al., 2012). There are also evidences that females pheromones are perceived both by the olfactory and taste system (Inoshita et al., 2011).
Another principle of pheromonal recognition is that the stimulatory pheromones of females are matched by inhibitory pheromones (Figure 5A). This system would allow for a graded response by males to female pheromones. This would explain why the main stimulatory CH of females 7,11-HD is expressed at different levels in different strains of D. melanogaster and elicits different level of courtship response by males from different strains (Marcillac et al., 2005b; Grillet et al., 2012; Pischedda et al., 2014). This difference in level does not make sense in terms of the function of 7,11-HD as a species-specific pheromone inhibiting courtship from males from different species because amounts of 7,11-HD as low as 70 ng completely block mating with males of sibling species (Marcillac and Ferveur, 2004; Billeter et al., 2009). Yet Strains of D. melanogaster have concentration of 7,11-HD far exceeding this dose [e.g., Canton-S: ca. 370 ng 7,11-HD (Billeter et al., 2009)]. Females of many strains produce amounts of dienes that largely exceed the threshold required for mate preference. A view in which pheromones counterbalance each other would help resolve this issue. Greater amount of 7,11-HD might for instance reduce the inhibitory effect of cVA (Billeter et al., 2009) in populations where males synthesize large amounts of cVA. These differences might also come from individual variation in the sensitivity of the male chemosensory system to each of these pheromones (Pischedda et al., 2014).
The pheromonal system of Drosophila melanogaster is an excellent system in which to study the basic mechanisms underlying the regulation of social interactions. As discussed in the introduction of this article, investigation of this system has allowed identification of several chemicals acting as pheromones, the cells and enzymatic pathways that produce these pheromones, the sensory neurons that detect them, and has even offered a glimpse at the neuronal circuitry that act downstream of the sensing of these chemicals. It is becoming clear that social interactions are not normally regulated by single pheromones, but by the integration of several signals. The challenge now is to understand where and how the sensing of different pheromones, environmental chemicals and other sensory cues such as vision (Agrawal et al., 2014) are integrated in the brain and how this integration regulates social interactions. This challenge is complicated by the fact that not all Drosophila pheromones have been identified as indicated by behavioral data and the existence of several orphan chemosensory receptors that seem to be sensitive to unidentified pheromone (Lu et al., 2012; Thistle et al., 2012; Toda et al., 2012; Koh et al., 2014). New behavioral assays and new analytical chemical methods are revealing the existence of additional pheromone classes, such as larval (Farine et al., 2014; Mast et al., 2014) and male (Yew et al., 2009) pheromones, and very recently an attractive pheromone found in both males and females and several Drosophila species (Dweck et al., 2015) that is likely to be the long-anticipated non-sex and non-species-specific attractive compound made by Drosophila species (Savarit et al., 1999; Billeter et al., 2009) making this field exciting, fast evolving and far from resolved.
The authors declare that the research was conducted in the absence of any commercial or financial relationships that could be construed as a potential conflict of interest.
We thank Bloomington Drosophila Stock Center for fly stocks. We thank Meghan Laturney and two referees for feedback on the manuscript. This work was supported in parts by grants from the Canadian Institute for Health Research, the National Science and Engineering Research Canada, Canadian Institute For Advanced Research and the Canada Research Chair program to JL and the Swiss National Fund and Dutch organization for scientific research (NWO) to JB.
Agrawal, S., Safarik, S., and Dickinson, M. H. (2014). The relative roles of vision and chemosensation in mate recognition of Drosophila. J. Exp. Biol. 217, 2797–2805. doi: 10.1242/jeb.105817
Andrews, J. C., Fernández, M. P., Yu, Q., Leary, G. P., Leung, A. K. W., Kavanaugh, M. P., et al. (2014). Octopamine neuromodulation regulates Gr32a-linked aggression and courtship pathways in Drosophila males. PLoS Genet. 10:e1004356. doi: 10.1371/journal.pgen.1004356
Antony, C., and Jallon, J.-M. (1982). The chemical basis for sex recognition in Drosophila melanogaster. J. Insect Physiol. 28, 873–880. doi: 10.1016/0022-1910(82)90101-9
Bartelt, R. J., Schaner, A. M., and Jackson, L. L. (1985). Cis-Vaccenyl Acetate as an aggregation pheromone in Drosophila melanogaster. J. Chem. Ecol. 11, 1747–1756. doi: 10.1007/BF01012124
Becher, P. G., Flick, G., Rozpêdowska, E., Schmidt, A., Hagman, A., Lebreton, S., et al. (2012). Yeast, not fruit volatiles mediate Drosophila melanogaster attraction, oviposition and development. Funct. Ecol. 26, 822–828. doi: 10.1111/j.1365-2435.2012.02006.x
Benton, R., Benton, R., Sachse, S., Sachse, S., Michnick, S. W., Michnick, S. W., et al. (2006). Atypical membrane topology and heteromeric function of Drosophila odorant receptors in vivo. PLoS Biol. 4:e20. doi: 10.1371/journal.pbio.0040020
Benton, R., Vannice, K. S., and Vosshall, L. B. (2007). An essential role for a CD36-related receptor in pheromone detection in Drosophila. Nature 450, 289–293. doi: 10.1038/nature06328
Billeter, J.-C., Atallah, J., Krupp, J. J., Millar, J. G., and Levine, J. D. (2009). Specialized cells tag sexual and species identity in Drosophila melanogaster. Nature 461, 987–991. doi: 10.1038/nature08495
Billeter, J.-C., Jagadeesh, S., Stepek, N., Azanchi, R., and Levine, J. D. (2012). Drosophila melanogaster females change mating behaviour and offspring production based on social context. Proc. Biol. Sci. 279, 2417–2425. doi: 10.1098/rspb.2011.2676
Billeter, J.-C., and Levine, J. D. (2013). Who is he and what is he to you? Recognition in Drosophila melanogaster. Curr. Opin. Neurobiol. 23, 17–23. doi: 10.1016/j.conb.2012.08.009
Brieger, G., and Butterworth, F. M. (1970). Drosophila melanogaster: identity of male lipid in reproductive system. Science 167, 1262–1262. doi: 10.1126/science.167.3922.1262
Butterworth, F. M. (1969). Lipids of Drosophila: a newly detected lipid in the male. Science 163, 1356–1357. doi: 10.1126/science.163.3873.1356
Chertemps, T., Duportets, L., Labeur, C., Ueda, R., Takahashi, K., Saigo, K., et al. (2007). A female-biased expressed elongase involved in long-chain hydrocarbon biosynthesis and courtship behavior in Drosophila melanogaster. Proc. Natl. Acad. Sci. U.S.A. 104, 4273–4278. doi: 10.1073/pnas.0608142104
Chertemps, T., Duportets, L., Labeur, C., Ueyama, M., and Wicker-Thomas, C. (2006). A female-specific desaturase gene responsible for diene hydrocarbon biosynthesis and courtship behaviour in Drosophila melanogaster. Insect Mol. Biol. 15, 465–473. doi: 10.1111/j.1365-2583.2006.00658.x
Couto, A., Alenius, M., and Dickson, B. J. (2005). Molecular, anatomical, and functional organization of the Drosophila olfactory system. Curr. Biol. 15, 1535–1547. doi: 10.1016/j.cub.2005.07.034
Datta, S. R., Vasconcelos, M. L., Ruta, V., Luo, S., Wong, A., Demir, E., et al. (2008). The Drosophila pheromone cVA activates a sexually dimorphic neural circuit. Nature 452, 473–477. doi: 10.1038/nature06808
Dweck, H. K. M., Ebrahim, S. A. M., Thoma, M., Mohamed, A. A. M., Keesey, I. W., Trona, F., et al. (2015). Pheromones mediating copulation and attraction in Drosophila. Proc. Natl. Acad. Sci. U.S.A. 112, E2829–E2835. doi: 10.1073/pnas.1504527112
Ebbs, M. L., and Amrein, H. (2007). Taste and pheromone perception in the fruit fly Drosophila melanogaster. Pflugers Arch. 454, 735–747. doi: 10.1007/s00424-007-0246-y
Ejima, A., Smith, B. P. C., Lucas, C., van der Goes van Naters, W., Miller, C. J., Carlson, J. R., et al. (2007). Generalization of courtship learning in Drosophila is mediated by cis-vaccenyl acetate. Curr. Biol. 17, 599–605. doi: 10.1016/j.cub.2007.01.053
Everaerts, C., Farine, J.-P., Cobb, M., and Ferveur, J.-F. (2010). Drosophila cuticular hydrocarbons revisited: mating status alters cuticular profiles. PLoS ONE 5:e9607. doi: 10.1371/journal.pone.0009607
Fan, P., Manoli, D. S., Ahmed, O. M., Chen, Y., Agarwal, N., Kwong, S., et al. (2013). Genetic and neural mechanisms that inhibit Drosophila from mating with other species. Cell 154, 89–102. doi: 10.1016/j.cell.2013.06.008
Farine, J.-P., Cortot, J., and Ferveur, J.-F. (2014). Drosophila adult and larval pheromones modulate larval food choice. Proc. Biol. Sci. 281, 20140043. doi: 10.1098/rspb.2014.0043
Farine, J.-P., Ferveur, J.-F., and Everaerts, C. (2012). Volatile Drosophila cuticular pheromones are affected by social but not sexual experience. PLoS ONE 7:e40396. doi: 10.1371/journal.pone.0040396
Fedina, T. Y., Kuo, T.-H., Dreisewerd, K., Dierick, H. A., Yew, J. Y., and Pletcher, S. D. (2012). Dietary effects on cuticular hydrocarbons and sexual attractiveness in Drosophila. PLoS ONE 7:e49799. doi: 10.1371/journal.pone.0049799
Fernández, M. P., Chan, Y.-B., Yew, J. Y., Billeter, J.-C., Dreisewerd, K., and Kravitz, E. A. (2010). Pheromonal and behavioral cues trigger male-to-female aggression in Drosophila. PLoS Biol. 8:e1000541. doi: 10.1371/journal.pbio.1000541
Ferveur, J. F., Savarit, F., O'Kane, C. J., Sureau, G., Greenspan, R. J., and Jallon, J. M. (1997). Genetic feminization of pheromones and its behavioral consequences in Drosophila males. Science 276, 1555–1558. doi: 10.1126/science.276.5318.1555
Fishilevich, E., and Vosshall, L. B. (2005). Genetic and functional subdivision of the Drosophila antennal lobe. Cur. Biol. 15, 1548–1553. doi: 10.1016/j.cub.2005.07.066
Gomez-Diaz, C., Reina, J. H., Cambillau, C., and Benton, R. (2013). Ligands for pheromone-sensing neurons are not conformationally activated odorant binding proteins. PLoS Biol. 11:e1001546. doi: 10.1371/journal.pbio.1001546
Grillet, M., Everaerts, C., Houot, B., Ritchie, M. G., Cobb, M., and Ferveur, J.-F. (2012). Incipient speciation in Drosophila melanogaster involves chemical signals. Sci. Rep. 2:224. doi: 10.1038/srep00224
Ha, T. S., and Smith, D. P. (2006). A pheromone receptor mediates 11-cis-vaccenyl acetate-induced responses in Drosophila. J. Neurosci. 26, 8727–8733. doi: 10.1523/JNEUROSCI.0876-06.2006
Inoshita, T., Martin, J.-R., Marion-Poll, F., and Ferveur, J.-F. (2011). Peripheral, central and behavioral responses to the cuticular pheromone bouquet in Drosophila melanogaster males. PLoS ONE 6:e19770. doi: 10.1371/journal.pone.0019770
Jallon, J.-M. (1984). A few chemical words exchanged by Drosophila during courtship and mating. Behav. Genet. 14, 441–478. doi: 10.1007/BF01065444
Jallon, J.-M., and David, J. R. (1987). Variation in cuticular hydrocarbons among the eight species of the Drosophila melanogaster subgroup. Evolution 41, 294–302. doi: 10.2307/2409139
Jin, X., Ha, T. S., and Smith, D. P. (2008). SNMP is a signaling component required for pheromone sensitivity in Drosophila. Proc. Natl. Acad. Sci. U.S.A. 105, 10996–11001. doi: 10.1073/pnas.0803309105
Katti, S., Lokhande, N., González, D., Cassill, A., and Renthal, R. (2012). Quantitative analysis of pheromone-binding protein specificity. Insect Mol. Biol. 22, 31–40. doi: 10.1111/j.1365-2583.2012.01167.x
Keleman, K., Vrontou, E., Krüttner, S., Yu, J. Y., Kurtovic-Kozaric, A., and Dickson, B. J. (2012). Dopamine neurons modulate pheromone responses in Drosophila courtship learning. Nature 489, 145–149. doi: 10.1038/nature11345
Kent, C., Azanchi, R., Smith, B., Formosa, A., and Levine, J. D. (2008). Social context influences chemical communication in D. melanogaster males. Curr. Biol. 18, 1384–1389. doi: 10.1016/j.cub.2008.07.088
Kim, M. S., Repp, A., and Smith, D. P. (1998). LUSH odorant-binding protein mediates chemosensory responses to alcohols in Drosophila melanogaster. Genetics 150, 711–721.
Kim, M. S., and Smith, D. P. (2001). The invertebrate odorant-binding protein LUSH is required for normal olfactory behavior in Drosophila. Chem. Senses 26, 195–199. doi: 10.1093/chemse/26.2.195
Koh, T.-W., He, Z., Gorur-Shandilya, S., Menuz, K., Larter, N. K., Stewart, S., et al. (2014). The Drosophila IR20a clade of ionotropic receptors are candidate taste and pheromone receptors. Neuron 83, 850–865. doi: 10.1016/j.neuron.2014.07.012
Kohatsu, S., Koganezawa, M., and Yamamoto, D. (2011). Female contact activates male-specific interneurons that trigger stereotypic courtship behavior in drosophila. Neuron 69, 498–508. doi: 10.1016/j.neuron.2010.12.017
Kohl, J., Ostrovsky, A. D., Frechter, S., and Jefferis, G. S. X. E. (2013). A bidirectional circuit switch reroutes pheromone signals in male and female brains. Cell 155, 1610–1623. doi: 10.1016/j.cell.2013.11.025
Krupp, J. J., Billeter, J.-C., Wong, A., Choi, C., Nitabach, M. N., and Levine, J. D. (2013). Pigment-dispersing factor modulates pheromone production in clock cells that influence mating in Drosophila. Neuron 79, 54–68. doi: 10.1016/j.neuron.2013.05.019
Krupp, J. J., Kent, C., Billeter, J.-C., Azanchi, R., So, A. K.-C., Schonfeld, J. A., et al. (2008). Social experience modifies pheromone expression and mating behavior in male Drosophila melanogaster. Curr. Biol. 18, 1373–1383. doi: 10.1016/j.cub.2008.07.089
Kuo, T.-H., Yew, J. Y., Fedina, T. Y., Dreisewerd, K., Dierick, H. A., and Pletcher, S. D. (2012). Aging modulates cuticular hydrocarbons and sexual attractiveness in Drosophila melanogaster. J. Exp. Biol. 215, 814–821. doi: 10.1242/jeb.064980
Kurtovic, A., Widmer, A., and Dickson, B. J. (2007). A single class of olfactory neurons mediates behavioural responses to a Drosophila sex pheromone. Nature 446, 542–546. doi: 10.1038/nature05672
Larsson, M. C., Domingos, A. I., Jones, W. D., Chiappe, M. E., Amrein, H., and Vosshall, L. B. (2004). Or83b encodes a broadly expressed odorant receptor essential for Drosophila olfaction. Neuron 43, 703–714. doi: 10.1016/j.neuron.2004.08.019
Laturney, M., and Billeter, J.-C. (2014). Neurogenetics of female reproductive behaviors in Drosophila melanogaster. Adv. Genet. 85, 1–108. doi: 10.1016/B978-0-12-800271-1.00001-9
Laughlin, J. D., Ha, T. S., Jones, D. N. M., and Smith, D. P. (2008). Activation of pheromone-sensitive neurons is mediated by conformational activation of pheromone-binding protein. Cell 133, 1255–1265. doi: 10.1016/j.cell.2008.04.046
Lebreton, S., Becher, P. G., Hansson, B. S., and Witzgall, P. (2012). Attraction of Drosophila melanogaster males to food-related and fly odours. J. Insect Physiol. 58, 125–129. doi: 10.1016/j.jinsphys.2011.10.009
Lebreton, S., Grabe, V., Omondi, A. B., Ignell, R., Becher, P. G., Hansson, B. S., et al. (2014). Love makes smell blind: mating suppresses pheromone attraction in Drosophila females via Or65a olfactory neurons. Sci. Rep. 4:7119. doi: 10.1038/srep07119
Levine, J., Billeter, J.-C., Krull, U., and Sodhi, R. (2010). The cuticular surface of D. melanogaster: ToF-SIMS on the fly. Surf. Interface Anal. 43, 317–321. doi: 10.1002/sia.3455
Liu, W., Liang, X., Gong, J., Yang, Z., Zhang, Y.-H., Zhang, J.-X., et al. (2011). Social regulation of aggression by pheromonal activation of Or65a olfactory neurons in Drosophila. Nat. Neurosci. 14, 896–902. doi: 10.1038/nn.2836
Lu, B., LaMora, A., Sun, Y., Welsh, M. J., and Ben-Shahar, Y. (2012). ppk23-Dependent chemosensory functions contribute to courtship behavior in Drosophila melanogaster. PLoS Genet. 8:e1002587. doi: 10.1371/journal.pgen.1002587
Marcillac, F., Bousquet, F., Alabouvette, J., Savarit, F., and Ferveur, J.-F. (2005a). A mutation with major effects on Drosophila melanogaster sex pheromones. Genetics 171, 1617–1628. doi: 10.1534/genetics.104.033159
Marcillac, F., and Ferveur, J.-F. (2004). A set of female pheromones affects reproduction before, during and after mating in Drosophila. J. Exp. Biol. 207, 3927–3933. doi: 10.1242/jeb.01236
Marcillac, F., Houot, B., and Ferveur, J.-F. (2005b). Revisited roles of Drosophila female pheromones. Chem. Senses 30(Suppl. 1), i273–i274. doi: 10.1093/chemse/bjh220
Mast, J. D., De Moraes, C. M., Alborn, H. T., Lavis, L. D., and Stern, D. L. (2014). Evolved differences in larval social behavior mediated by novel pheromones. eLife 3:e04205. doi: 10.7554/eLife.04205
Miyamoto, T., and Amrein, H. (2008). Suppression of male courtship by a Drosophila pheromone receptor. Nat. Neurosci. 11, 874–876. doi: 10.1038/nn.2161
Pischedda, A., Shahandeh, M. P., Cochrane, W. G., Cochrane, V. A., and Turner, T. L. (2014). Natural variation in the strength and direction of male mating preferences for female pheromones in Drosophila melanogaster. PLoS ONE 9:e87509. doi: 10.1371/journal.pone.0087509
Ramdya, P., Lichocki, P., Cruchet, S., Frisch, L., Tse, W., Floreano, D., et al. (2015). Mechanosensory interactions drive collective behaviour in Drosophila. Nature 519, 233–236. doi: 10.1038/nature14024
Ronderos, D. S., and Smith, D. P. (2010). Activation of the T1 neuronal circuit is necessary and sufficient to induce sexually dimorphic mating behavior in Drosophila melanogaster. J. Neurosci. 30, 2595–2599. doi: 10.1523/JNEUROSCI.4819-09.2010
Ruta, V., Datta, S. R., Vasconcelos, M. L., Freeland, J., Looger, L. L., and Axel, R. (2010). A dimorphic pheromone circuit in Drosophila from sensory input to descending output. Nature 468, 686–690. doi: 10.1038/nature09554
Saltz, J. B., and Foley, B. R. (2011). Natural genetic variation in social niche construction: social effects of aggression drive disruptive sexual selection in Drosophila melanogaster. Am. Nat. 177, 645–654. doi: 10.1086/659631
Savarit, F., Sureau, G., Cobb, M., and Ferveur, J. F. (1999). Genetic elimination of known pheromones reveals the fundamental chemical bases of mating and isolation in Drosophila. Proc. Natl. Acad. Sci. U.S.A. 96, 9015–9020. doi: 10.1073/pnas.96.16.9015
Schlief, M. L., and Wilson, R. I. (2007). Olfactory processing and behavior downstream from highly selective receptor neurons. Nat. Neurosci. 10, 623–630. doi: 10.1038/nn1881
Schneider, J., Dickinson, M. H., and Levine, J. D. (2012). Social structures depend on innate determinants and chemosensory processing in Drosophila. Proc. Natl. Acad. Sci. U.S.A. 109(Suppl. 2), 17174–17179. doi: 10.1073/pnas.1121252109
Shanbhag, S., Müller, B., and Steinbrecht, R. (1999). Atlas of olfactory organs of Drosophila melanogaster 1. Types, external organization, innervation and distribution of olfactory sensilla. Int. J. Insect Morph. Embryol. 28, 377–397. doi: 10.1016/S0020-7322(99)00039-2
Shanbhag, S. R., Hekmat-Scafe, D., Kim, M. S., Park, S. K., Carlson, J. R., Pikielny, C., et al. (2001). Expression mosaic of odorant-binding proteins in Drosophila olfactory organs. Microsc. Res. Tech. 55, 297–306. doi: 10.1002/jemt.1179
Siegel, R. W., and Hall, J. C. (1979). Conditioned responses in courtship behavior of normal and mutant Drosophila. Proc. Natl. Acad. Sci. U.S.A. 76, 3430–3434. doi: 10.1073/pnas.76.7.3430
Simon, J. C., Dickson, W. B., and Dickinson, M. H. (2011). Prior mating experience modulates the dispersal of Drosophila in males more than in females. Behav. Genet. 41, 754–767. doi: 10.1007/s10519-011-9470-5
Spieth, H. T. (1974). Courtship behavior in Drosophila. Annu. Rev. Entomol. 19, 385–405. doi: 10.1146/annurev.en.19.010174.002125
Starostina, E., Liu, T., Vijayan, V., Zheng, Z., Siwicki, K. K., and Pikielny, C. W. (2012). A Drosophila DEG/ENaC subunit functions specifically in gustatory neurons required for male courtship behavior. J. Neurosci. 32, 4665–4674. doi: 10.1523/JNEUROSCI.6178-11.2012
Thistle, R., Cameron, P., Ghorayshi, A., Dennison, L., and Scott, K. (2012). Contact chemoreceptors mediate male-male repulsion and male-female attraction during Drosophila courtship. Cell 149, 1140–1151. doi: 10.1016/j.cell.2012.03.045
Toda, H., Zhao, X., and Dickson, B. J. (2012). The Drosophila female aphrodisiac pheromone activates ppk23+ sensory neurons to elicit male courtship behavior. Cell Rep. 1, 1–9. doi: 10.1016/j.celrep.2012.05.007
van der Goes van Naters, W., and Carlson, J. R. (2007). Receptors and neurons for fly odors in Drosophila. Curr. Biol. 17, 606–612. doi: 10.1016/j.cub.2007.02.043
Vosshall, L. B., and Stocker, R. F. (2007). Molecular architecture of smell and taste in Drosophila. Annu. Rev. Neurosci. 30, 505–533. doi: 10.1146/annurev.neuro.30.051606.094306
Wang, L., and Anderson, D. J. (2010). Identification of an aggression-promoting pheromone and its receptor neurons in Drosophila. Nature 463, 227–231. doi: 10.1038/nature08678
Wang, L., Han, X., Mehren, J., Hiroi, M., Billeter, J.-C., Miyamoto, T., et al. (2011). Hierarchical chemosensory regulation of male-male social interactions in Drosophila. Nat. Neurosci. 14, 757–762. doi: 10.1038/nn.2800
Wertheim, B., Allemand, R., Vet, L. E. M., and Dicke, M. (2006). Effects of aggregation pheromone on individual behaviour and food web interactions: a field study on Drosophila. Ecol. Entomol. 31, 216–226. doi: 10.1111/j.1365-2311.2006.00757.x
Wertheim, B., Dicke, M., and Vet, L. E. M. (2002). Behavioural plasticity in support of a benefit for aggregation pheromone use in Drosophila melanogaster. Entomol. Exp. Appl. 103, 61–71. doi: 10.1046/j.1570-7458.2002.00954.x
Xu, P., Atkinson, R., Jones, D. N. M., and Smith, D. P. (2005). Drosophila OBP LUSH is required for activity of pheromone-sensitive neurons. Neuron 45, 193–200. doi: 10.1016/j.neuron.2004.12.031
Yew, J. Y., Cody, R. B., and Kravitz, E. A. (2008). Cuticular hydrocarbon analysis of an awake behaving fly using direct analysis in real-time time-of-flight mass spectrometry. Proc. Natl. Acad. Sci. U.S.A. 105, 7135–7140. doi: 10.1073/pnas.0802692105
Yew, J. Y., Dreisewerd, K., Luftmann, H., Müthing, J., Pohlentz, G., and Kravitz, E. A. (2009). A new male sex pheromone and novel cuticular cues for chemical communication in Drosophila. Curr. Biol. 19, 1245–1254. doi: 10.1016/j.cub.2009.06.037
Keywords: pheromones, cuticular hydrocarbons, olfactory and gustatory system, sexual behavior, social behavior, aggregation, odorant binding proteins, Drosophila melanogaster
Citation: Billeter J-C and Levine JD (2015) The role of cVA and the Odorant binding protein Lush in social and sexual behavior in Drosophila melanogaster. Front. Ecol. Evol. 3:75. doi: 10.3389/fevo.2015.00075
Received: 14 April 2015; Accepted: 23 June 2015;
Published: 10 July 2015.
Edited by:
Sebastien Lebreton, Swedish University of Agricultural Sciences, SwedenReviewed by:
Gregory S. X. E. Jefferis, MRC Laboratory of Molecular Biology, UKCopyright © 2015 Billeter and Levine. This is an open-access article distributed under the terms of the Creative Commons Attribution License (CC BY). The use, distribution or reproduction in other forums is permitted, provided the original author(s) or licensor are credited and that the original publication in this journal is cited, in accordance with accepted academic practice. No use, distribution or reproduction is permitted which does not comply with these terms.
*Correspondence: Jean-Christophe Billeter, Groningen Institute for Evolutionary Life Science, University of Groningen, PO Box 11103, Nijenborgh 7, Groningen, 9700 CC, Netherlands,ai5jLmJpbGxldGVyQHJ1Zy5ubA==
Disclaimer: All claims expressed in this article are solely those of the authors and do not necessarily represent those of their affiliated organizations, or those of the publisher, the editors and the reviewers. Any product that may be evaluated in this article or claim that may be made by its manufacturer is not guaranteed or endorsed by the publisher.
Research integrity at Frontiers
Learn more about the work of our research integrity team to safeguard the quality of each article we publish.