- 1The L.A.K.E.S Institute, Lyss, Switzerland
- 2Centre for Ecological Research and Forestry Applications (CREAF-CSIC), Cerdanyola del Vallès, Spain
Lake Muzzano (45°59′50″N 8°55′41″E, 337 m a.s.l.) is a hyper-eutrophied lake located in the Tessin region of Switzerland. Almost every year, algal blooms (Microcystis) cover the lake with a thickness of 1–2 cm. These blooms associated with periods of anoxia in summer have led to fish kills in 1967 and 1994. In the hope of avoiding these blooms, a bypass bringing water away from the lake has been established in 1999. This solution was not adequate as blooms kept reoccurring. Sediment removal was then proposed by the Tessin Canton as a possible remediation technique and The L.A.K.E.S Institute had a mandate in 2010 to study the lake (present and past state) to determine the reasons creating anoxia and algal blooms. The present state of the lake shows that anoxia is still occurring when the algal bloom covers the lake's surface. Subfossil diatom and chironomid analyses show that the baseline conditions were those found before 1922 AD when the lake was oligotrophic and supported a diversified community of chironomids suggesting good oxygenation. After 1922 AD, circulation to the lake was cut out and nutrients accumulated in the lake leading to anoxia and the establishment of Microcystis. Heavy metal analysis in the sediment shows that the concentration is above the national recommendation and thus sediment should not be removed or should be stored with hazardous material. Based on the present status of the lake and paleolimnological results, two solutions are proposed: to further decrease the nutrients coming in the lake (possibly using filtrating plants) followed by flushing to increase lake water circulation. Physical capping of the sediment to avoid exchange of heavy metals and phosphorus release at the water/sediment interface could also be envisaged once the two prime solutions are in place.
Introduction
In the UK, Scandinavia and Canada, using paleolimnology to determine the baseline limnological conditions to restore a lake has precedents (see papers in this issue). In Switzerland, this is not the case. Restoration solutions are generally based on short term data, with restoration goals which are generally not quantified. Furthermore, most restoration solutions primarily focus on phosphorus control and assume other possible factors are just concomitant. This study is the first one in Switzerland to use paleolimnology to (a) define the baseline conditions for lake restoration and (b) study the past ecosystems to identify the “real” problems leading to eutrophication.
Our study site is Lake Muzzano located in the Italian part (Tessin) of Switzerland. The lake is hyper-eutrophied and suffers from recurrent Microcystis algal blooms (Bottinelli, 1999; Bottinelli et al., 2000; Isenburg et al., 2000; Pedrotta, 2009; Studio Blu Progetti Sagl, 2011–2012). Furthermore, anoxia has been measured during summer (Isenburg et al., 2000; Hale et al., 2009; Pedrotta, 2009; Studio Blu Progetti Sagl, 2011–2012). The anoxia-inducing algal blooms have led to fish kills in 1967 and 1994 (Aquarius, 1999). To avoid fish kills, a bypass deriving the major inflow has been installed in 1999. Large pipelines bring the majority of water underground through a small river leading to Lake Lugano. However, water still flows in Lake Muzzano when the system overflows. In theory, overflow should occur only during periods of heavy rain. More than 10 years after the bypass was installed, algal blooms are still occurring. Assuming that the quantity of nutrients coming to the lake was adequately reduced by the bypass, it was suggested that the problem in Lake Muzzano was internal phosphorus loading via sediment and thus sediment removal was proposed. Lake Muzzano is owned by a private non-profit organization called Pro Natura which has the mandate of preserving the lake which houses a unique species of water chestnut (Trapa natans var. muzzanensis). Before removing the sediment, as suggested, Pro Natura wanted to be sure that sediment removal would not affect this unique water chestnut community. The L.A.K.E.S Institute was then mandated to study Lake Muzzano. The goals of this research are thus to:
(1). Establish the nutrient budget. None of the previous studies had established a comprehensive study of inflows and outflow. Pedrotta (2009) had looked at the inflows and outflow for a few months in 2006 and 2007 but no data were available on a whole year. Isenburg (1998) and Hale et al. (2009) only looked at the nutrients in the lake without comparing with inflows and outflow. Furthermore, the quantity of Phosphorus possibly released from the sediment was never quantified.
(2). Determine the baseline conditions as a target for remediation. Biological and sedimentological analyses will be used to determine the pre-disturbance limnological conditions and study the historical changes of Lake Muzzano through time. This reconstruction should be made from a period of low human impact to reconstruct the natural variability of this ecosystem.
(3). Evaluate sediment removal as a possible restoration solution. The L.A.K.E.S Institute wonders what is contained in the sediment (e.g., heavy metals).
Materials and Methods
The Study Site
Lake Muzzano (45°59′50″N 8°55′41″E, 337 m a.s.l.) is located in the Tessin Canton of Switzerland. The lake's catchment has three main localities contributing to its input of water: Muzzano [population: 837 inhabitants in 2010 (www.muzzano.ch)], Sorengo (1700 inhabitants in 2010 (www.sorengo.ch) and Collina d'Oro (population: 4493 inhabitants in 2011 (www.collinadoro.com). Berganzona, a part of Lugano, also contributes to the input of water to the lake (population: 5375 inhabitants in 2013, http://www.lugano.ch/lugano-politica/quartieri/breganzona.html).
The lake is shallow (3.2 m maximum depth) with an area of 0.22 km2. The lake has three major and four minor (sporadic) inflows (Figure 1). The outflow goes to the major Lake Lugano. Lake Muzzano is turbid with a Secchi depth of 0.6 m measured in March 2012 (Figure 1). Between June and October, Microcystis blooms are 1–2 cm thick (Figure 1).
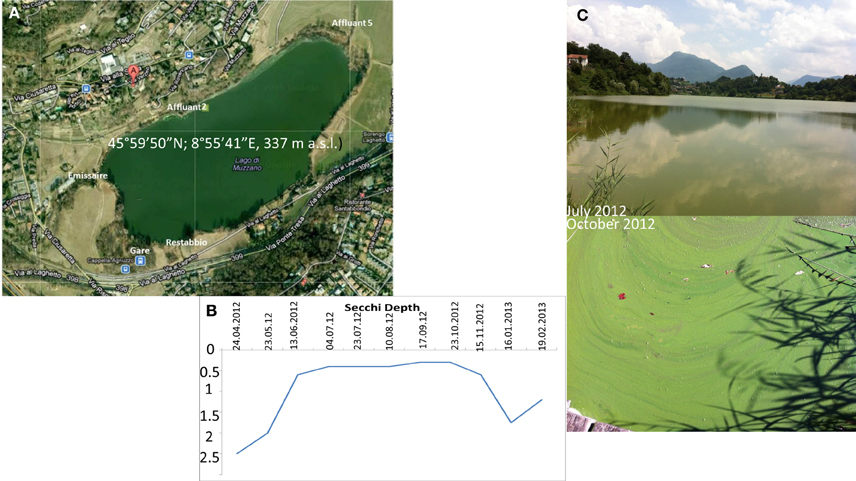
Figure 1. (A) Lake Muzzano and its inflows (Affluant 5 being the larger one, Affluant 2, Restabbio, and Gare being non-permanent ones) and its outflow (Emissaire). (B) Secchi depth (m) and (C) Pictures of the lake in July and October 2002 showing Microcystis algal blooms.
Lake Muzzano became stratified in the summer of 2012 (Figure 2A). In August 10th, 2012, the lake was strongly stratified with temperatures almost 5°C colder below 2.5 m than at the surface. A weak stratification was observed in September and disappeared by October. This stratification has been also recorded in previous years (Isenburg, 1998; Hale et al., 2009; Studio Blu Progetti Sagl, 2011–2012). In such a shallow lake (maximum 3 m depth), polymixis is generally expected. In Lake Muzzano, the stratification is probably due to the presence of algal blooms in the surface of the water column accumulating heat at the surface. When wind occurs, this thermal stratification is destroyed and mixing (uniform temperatures in the water column) occurs. In 2012, the lake was anoxic during summer (Figure 2A). Anoxia was also recorded in previous years (Isenburg, 1998; Isenburg et al., 2000; Hale et al., 2009; Studio Blu Progetti Sagl, 2011–2012).
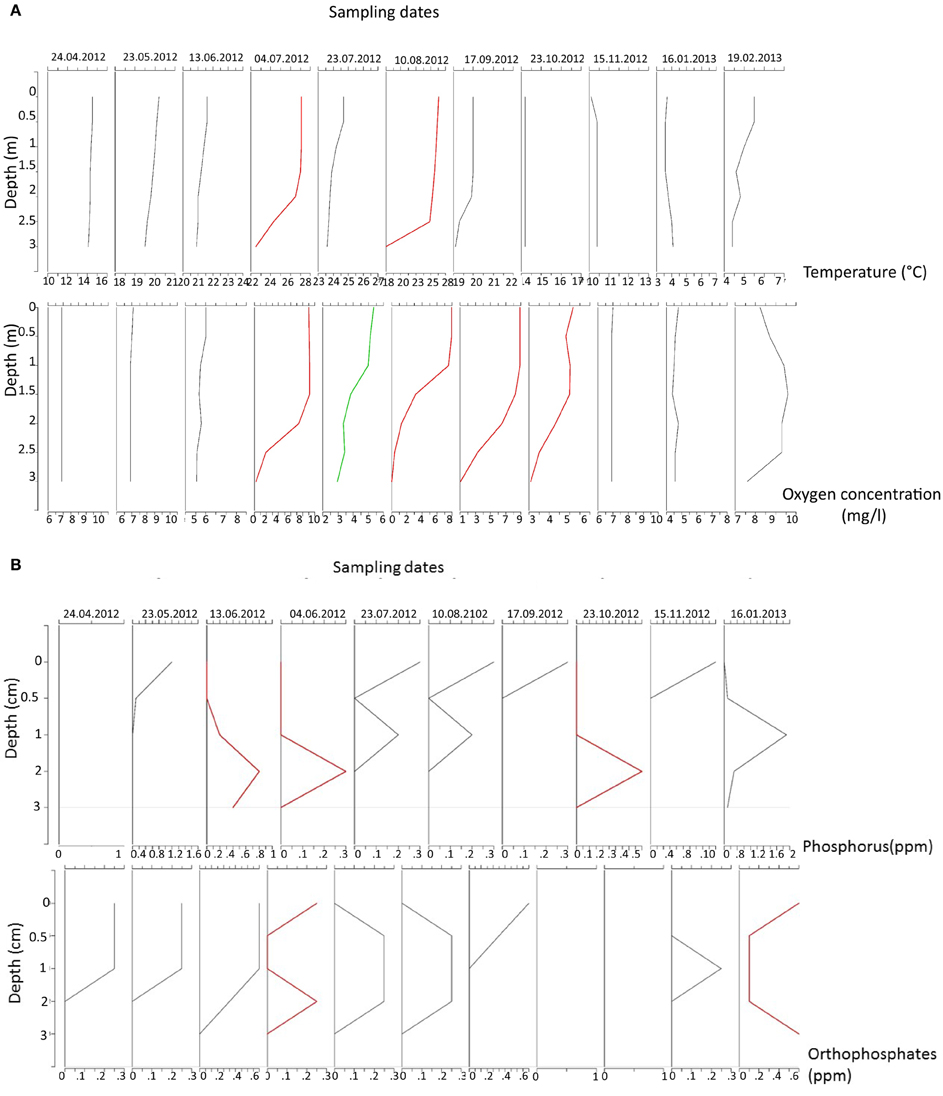
Figure 2. (A) Temperature and oxygen concentration measured during the sampling period. Red lines indicate dates of established thermocline and anoxia. (B) Phosphorus and Orthophosphates measured during the sampling period. Red lines indicate dates when the concentration in nutrients was higher at the bottom compared to the surface.
Nutrient Concentration in the Lake and Nutrient Budget in Inflows and Outflow
Water samples (1 l) were taken monthly in the inflows, the outflow and in the lake (surface, 0.5, 1, 2, and 3 m). Nitrates (NO3), Phosphorus (TP), Phosphates (Orthophosphates), and Ammonium (NH4) were measured in these water samples using kit tests (concentration in ppm). In June 2012, the Canton of Tessin decided to provide more accurate measurements of these parameters and the monthly water samples from the inflows and outflow were sent to the Laboratorio dell'Ufficio del monitoraggio ambientale in Bellinzona for analysis. The results (in μg/L or mg/L) were used to determine how much of nutrients are still coming in Lake Muzzano and how much comes out through the outflow. The concentrations were also compared to concentrations described as “good,” “acceptable,” or “mediocre” by the canton (BAFU, 2010).
The debit of each inflow needed to be calculated to know the concentration of nutrients coming into the lake (mg/s) and coming out of the lake. The balance between inflows and outflow provides an understanding of the circulation and the retention time of nutrients. The longer the retention time, more nutrients are available for algal growth. The width and depth of the inflows and outflows have been measured. The flow was measured using a pulse counter measuring counts/minutes. These counts/minutes were transformed into speed following the graph included in the users' manual. The debit (Q) was calculated following this formula:
Where Q: debit in m3/s
V: speed of current
Sm: area of the inflow
To calculate the Phosphorus loading per year, the average concentration * the debit for each inflow should be summed (Li et al., 2003) following the formula below:
where Ci is the concentration at time i, Qi is the flow at time i and K is the time interval (252 days).
Sediment Analyses
Four sediment cores were extracted in March 2012 at the deepest (3.2 m) part of the lake using a UWITEC corer. All cores were transported complete to the laboratory. The longest core (44 cm) was used for most analyses. One half of the core was used for dating and loss-on-ignition measurements, the other half was used for diatom, chironomid and heavy metal analyses.
The half core for dating was cut every 0.5 cm until 15 cm and every cm until 44 cm. All the samples from the surface to 15 cm were freeze-dried and weighted. They were sent to Flett Research (Winnipeg, Canada) for radioisotope (210Pb, 137Cs, 226Ra) measurements. An age-depth model was developed using the Bayesian calibration program OxCal 4.2 (Bronk Ramsey, 2013) and constrained to the know peaks of 137Cesium in 1986 (Chernobyl) and 1963 (Von Gunten et al., 2008).
Loss-on-Ignition (LOI) followed the method exposed in Heiri et al. (2001). First, the water content of all samples is calculated by weighting the wet sediment and subtracting the dry sediment (48 h at 105°C). The percentage of organic matter was calculated after heating the sediment to 550°C for 4 h.
Chironomids were analyzed in all samples at 0.5 and 1 cm for a total of 62 samples. The samples were placed in KOH 10% overnight and filtered in a 90 μm mesh. The sample left in the mesh was poured in a Bogorov counting tray and observed under a magnifier at 10X. Each head capsule was individually picked and mounted in a drop of Hydromatric on a microscope slide and covered with a cover slip. This procedure is very time consuming. To pick one sample can take up to half a day of preparation. The chironomids were identified under a light microscope at 40X-100X. The identification followed Larocque and Rolland (2006) and Brooks et al. (2007). Anoxic taxa were described following Quinlan et al. (1998).
The same samples (62) were analyzed for diatoms following standard procedures (Battarbee et al., 2000). The identification followed Krammer and Lange-Bertalot (1986–1991). The final taxonomy was harmonized to fit the taxonomy and taxonomic resolution of the SWISS-TP dataset in order to apply a TP transfer function using ERNIE software from the EDDI diatom database (http://craticula.ncl.ac.uk/Eddi/jsp/index.jsp). pH was described using the known ecology of diatom species described in the reference manual cited above.
The diatom and chironomid stratigraphies were subdivided into assemblage zones with the technique of optimal splitting by information content (Birks and Gordon, 1985) and the number of statistically significant zones was identified using the Psimpoll 4.27 software (Bennett, 2009).
Surface samples (slices 0–1, 1–3, 3–5, and 5–7 cm) were sent to Columbia Analytical Services, Inc. (www.caslab.com) to measure iron-bounded Phosphorus following the method detailed by Psenner et al. (1998). With anoxia, SO4 increases in the water-sediment interface which oxidizes iron and Phosphorus is released to the water column. The concentration of Iron-bounded Phosphorus is thus important to quantify and determine how much can be released and if this amount significantly exceed the concentration coming into the lake from the inflows.
To calculate the amount of Phosphorus load, the formula from Nürnberg et al. (1994) was be used:
RR = areal release rate (mg/m2/ d)
AF = anoxic factor (d/yr)
In this formula, the period of anoxia (ti in days) is multiplied by the corresponding hypolimnetic area (ai in m2), and all the products were added up. The grand total id divided by the lake surface area (/lo, mZ) to yield AF.
Sediment at every cm of the core was analyzed in an ICP spectrophotometer from the Institute of Geography, University of Bern. First, each sample was dried in an oven at 100°C overnight to remove the water. The sample was then crushed to obtain fine-grained sediment. The sediment was diluted using a microwave and the following procedure: HF and NaNO3 were added to each sample, the samples were placed in the microwave for 40 min then filtered. The filtered liquid was placed in the ICP-MS spectrophotometer. The concentration of eight different metals [Lead (Pb), Zinc (Zn), Copper (Cu), Cadmium (Cd), Chromium (Cr), Titanium (Ti), and Nickel (Ni) and Arsenic (As)] was measured in each sample. Those are the most common metals found in the Tessin area. They are also the metals usually measured in environmental assessments (BAFU, 2010). The concentration obtained is then multiplied by the dilution (50) and divided by the weight of the sediment (0.25–0.35 g) to obtained μg/kg. Those concentrations were then compared to the contaminated values defined by the Canton (BAFU, 2010).
Results
Nutrients in the Lake
Total Phosphorus was higher at 3 m-depth only at one date in June 2012. Higher concentrations at 2 m were observed on the 4th of July and in October (Figure 2B). Only in February 2013 was Phosphorus higher at 3 m than at other levels. Similar results were obtained for Ammonium and Nitrates (Supplementary Image 1).
Nutrient Inflows vs. Outflow
Concentrations of each nutrient at each sampling date are provided as Supplementary Image 3. A summarized debit is provided in Table 1. Except for Total Phosphorus, the flows of nutrients are higher in the inflows. Most importantly, the Orthophosphates (PO4P) coming in is higher than coming out.
Phosphorus Loading
Following the formula exposed in the method section, the amount of Orthophosphate (PO4) coming to the lake is 152 kg/year and Total Phosphorus is 257 kg/year. The amount coming out of the lake is 22.27 Kg/year of PO4 and 225 kg/year TP.
Possible Release of Phosphorus Form the Sediment
The concentrations of the various forms of phosphorus in the sediment are presented in Table 2. From these concentrations, it is possible to calculate the potential amount of phosphorus release from the sediment following the formula presented in the method section. The specific data are presented as Supplementary Image 2. The total phosphorus present in the sediment is 152 kg/year and the phosphorus which can be released from the sediment is 19 kg/year. This possibly released phosphorus represents 12% of the total Phosphorus measured in the sediment.
Dating of Sediment
The activity in 210Pb varied in the first 2 cm suggesting mixing in the very fluid sediment (Figure 3A). The activity slowly decreased afterwards which indicates a good stratigraphy and preservation of slices without too much mixing, which is surprising in such fluid sediment until 5 cm. Using an age-depth model (based on the dates of 210Pb and 226Radon Figure 3B) and constrained to the 137Cesium peaks of 1963 and 1986, the sediment rate was in average ca. 0.3 cm/year. It was higher (ca. 0.6 cm/year for the first 5 cm), decreased to ca. 0.23 cm/year until 31 cm, increased to ca, 0.5 cm until 40 cm and decreased to ca. 0.25 for the last 3 cm. The 43-cm core, after 210Pb dating represented the last ca. 176 years with an error of about 10 years at the deepest part of the core and about 2 years (date of the model—date of the 137Cesium peak) at the beginning of the core.
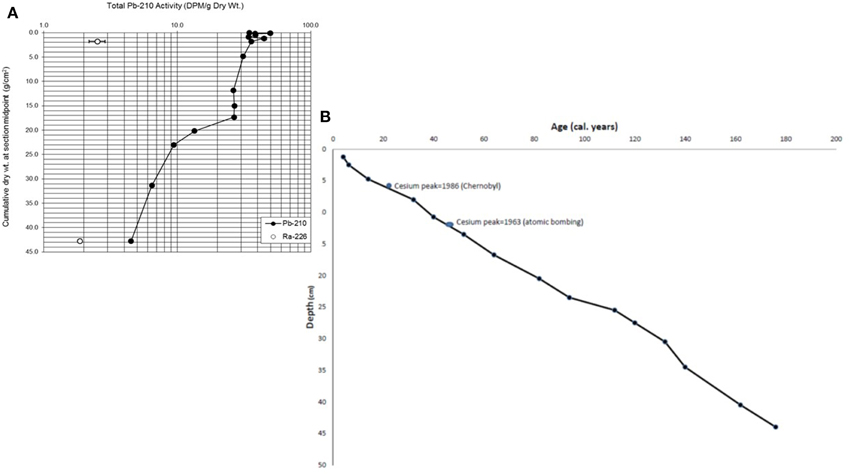
Figure 3. (A) Lead concentration. Graph provided by Flett Research. (B) Age-depth model developed using the Bayesian calibration program OxCal 4.2 (Bronk Ramsey, 2013) and constrained to the know peaks of 137Cesium in 1986 (Chernobyl) and 1963 (Von Gunten et al., 2008).
Loss-on-ignition
The percentage of water decreases down the core, but is always relatively high (>40%) (Figure 4A). In the upper 25 cm, the water content is almost always higher than 88%. The organic carbon varies as well through time with percentages between 8 and 32%. The sediment was typically black with organic matter (Figure 4B). After the sediment was heated at 550°C, the sediment remaining had an orange color, suggesting high concentration of iron (Figure 4C).
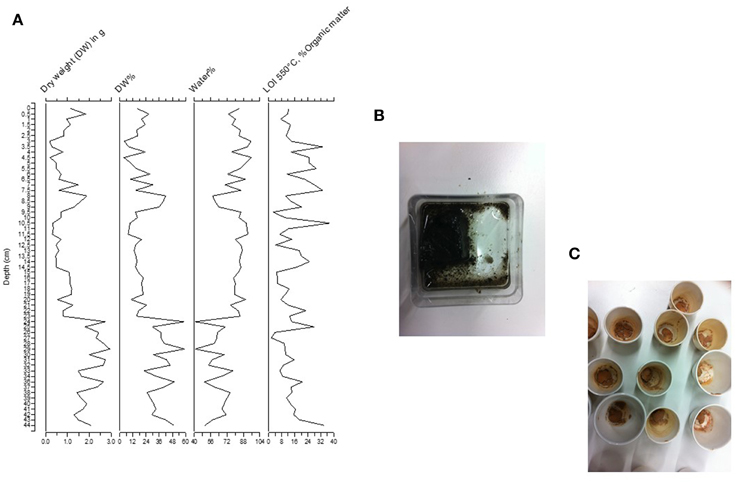
Figure 4. (A) Weight of sediment, water percentage and loss-on-ignition (LOI) percentages. (B) Color of wet sediment and (C) color of sediment after heating at 550°C. The red color suggests high iron concentration.
Chironomids
Sixty-two samples were analyzed for chironomids, but many had few head capsules and were merged together. The total of samples presented here is 36 since ca. 1853 AD (Figure 5). Thirty-five taxa were found in the samples. Only those present in at least two levels are presented in the figure.
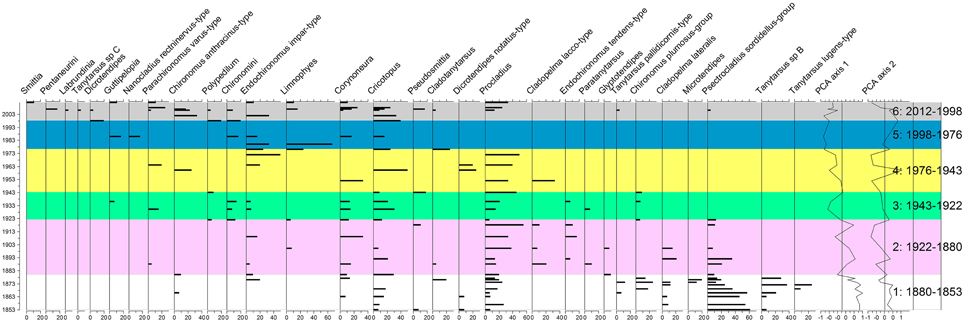
Figure 5. Chironomid diagram. The taxa are in percentages. The zones were created using the technique of optimal splitting by information content (Birks and Gordon, 1985) and the number of statistically significant zones was identified using the Psimpoll 4.27 software (Bennett, 2009).
The chironomid assemblages were significantly different during five episodes between ca. 1880 AD and 2012 (Figure 5). Those periods were statistically identified by the technique of optimal splitting by information content (Birks and Gordon, 1985) and the number of statistically significant zones was identified using the Psimpoll 4.27 software (Bennett, 2009).
Between 1880 and 1853 AD (zone 1), the dominating taxa (different Tanytarsus, Psectrocladius sordidellus-group and Cladopelma) are all taxa preferring mesotrophic and acidophilic states. Many of these taxa decrease or even disappear in zone 2 (1880–1922 AD). Zone 2 seems a transitional zone with increases in Procladius (meso to eutrophic taxon, often the last to survive anoxia), Cricotopus (eutrophic), Corynoneura (associated with macrophyte and eutrophic), and Glyptotendipes (likes detritus-rich sediment, meso to eutrophic conditions), Endochironomus tendens-type (acidophilic, meso) and Endochironomus impar-type (eutrophic). In zone 3 (1922–1943 AD), all acidophilic (e.g., Psectrocladius sordidellus-group, Tanytarsus) disappear. Procladius, Cricotopus, Endochironomus impar-type all increase. These are all eutrophic taxa. In zone 4 (1943–1976 AD) Procladius dominates with other eutrophic taxa (Cricotopus, Corynoneura) with some anoxic taxa (Chironomus). In zone 5 (1976–1998 AD), many taxa disappear. This is the section where many samples needed to be pulled together as very few head capsules were found. Endochironomus impar-type, a eutrophic taxa, dominates. Procladius completely disappeared. This is a taxon which can survive under anoxic conditions for a few weeks. In zone 6 (1998–2012), Procladius reappears toward the end as well as Chironomus anthracinus-group, a taxon surviving anoxia for a few weeks. New taxa (Limnophyes, Smittia) appear during this zone. Limnophyes and Smittia are often considered as semi-terrestrial taxa, suggesting an increase in running water coming to the lake.
The taxon Chironomus has been shown to be sensitive to heavy metals (Vermeullen et al., 2000) and can be used as an indicator of contamination due to deformation of its mentum caused by exposition. One deformed C. anthracinus-type has been found in level 2–3 cm (ca. 2005–2007 AD).
Diatoms
Seven zones were statistically identified in the diatom stratigraphy (Figure 6). Zone 1 (1853–1877 AD) was dominated by Aulacoseira muzzanensis, Cyclotella pseudocomensis, Aulacoseira ambigua, and Achnanthidium minutissimum. The reconstructed TP changed from 30 to <80 μg/l suggesting a change from meso-eutrophic to mesotrophic conditions. The species present suggest low pH conditions. In zone 2 (1877–1922 AD) the same species as zone 1 were present but A. ambigua dominated while A. minutissimum decreased. These changes were associated with an increase in the reconstructed TP from 70 to 120 μg/l thus to hyper-eutrophic conditions. Based on the species, the pH conditions were still acidic. In zone 3 (1922–1943 AD) the TP first increased to 180 μg/l and decreased to 120 μg/l. The communities changed to a dominance of Cyclotella meneghiniana, Stephanodiscus parvus, Stephanodiscus hantzschii, and Aulacoseira granulata. The species present suggest higher pH than in previous zones. This high pH remained through the present. Zone 4 (1942–1964 AD) was marked by a decrease of reconstructed TP to 100 μg/l (hyper-eutrophic) but the species dominating changed to Fragilaria crotonensis and Asterionella formosa. The taxa present in zone 3 are still there but at lower percentages. In zone 5 (1964–1980 AD), the reconstructed TP oscillated between hyper-eutrophic (120 μg/l) and eutrophic (>80 μg/l) and was marked by a re-increase of A. ambigua and A. muzzanensis. In zone 6 (1980–1998 AD) one sample (1993) had no diatoms. In zone 7 (1998–2012 AD) the inferred TP oscillated around the average (vertical line in Figure 6) but still represented hyper/eutrophic conditions.
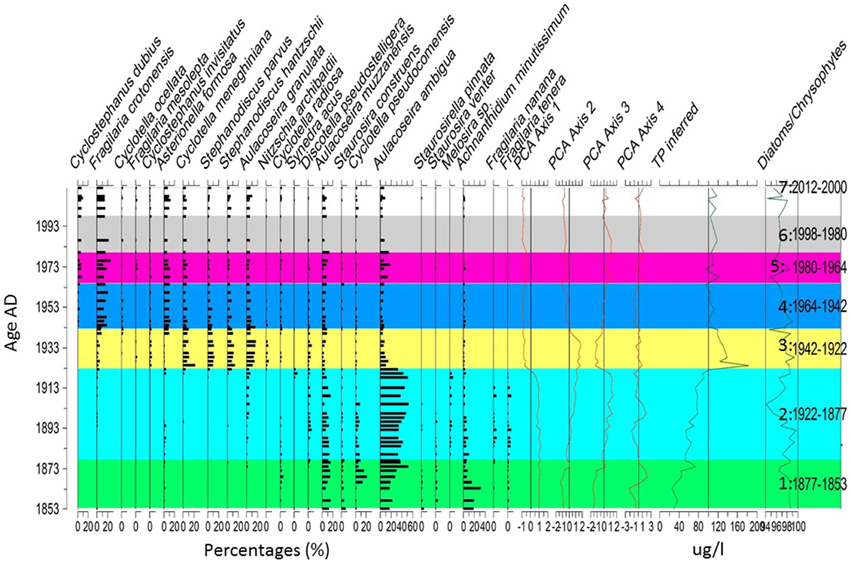
Figure 6. Diatom diagram. The taxa are in percentages. The zones were created using the technique of optimal splitting by information content (Birks and Gordon, 1985) and the number of statistically significant zones was identified using the Psimpoll 4.27 software (Bennett, 2009).
Heavy Metals
Generally, the present concentrations (μg/kg) of Cr, Ni, Cu, Zn, Pb are above the “heavily polluted level” (red lines, Giesy and Hoke, 1990) while Cadmium (Cd) was always at an accepted level (Figure 7). The concentrations exceed the level of “heavily polluted” since 1895 for Cr, 1950 for Ni, 1860 for Cu and Zn, 1932 for As, 1972 for Ti, and 1875 for Pb.
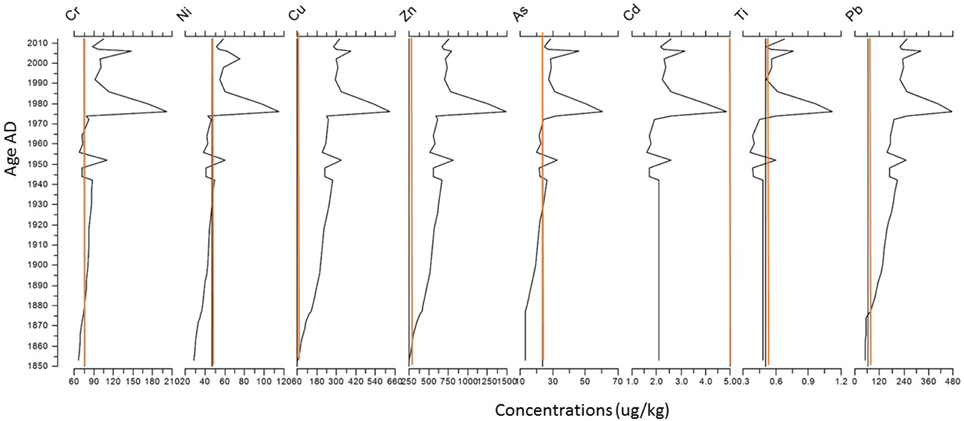
Figure 7. Heavy metal concentrations. The red lines indicate levels quantified as polluted following Giesy and Hoke (1990).
Discussion
The discussion is made around the three objectives of this research: (1) Establishing the nutrient budget, (2) Determining the baseline conditions as a remediation objective and (3) Evaluating sediment removal as a possible restoration solution.
Establish the Budget of Nutrients
The flow of nutrients was almost always higher in the inflows compared to the outflow except in one date, in November, when Microcystis algae left the lake and accumulated in the outflow. For algal bloom development, the most important variables are orthophosphates and nitrates which can be used directly by the algae. The flows of these two nutrients were higher in the inflows compared to the outflow suggesting that a large amount is used by the algae or sediment before they come out of the lake. The external Orthophosphate (PO4) load was evaluated at 152 kg/year and the external load of Total Phosphorus was evaluated at 257 kg/year. In 1999, the bypass was supposed to reduce this charge to below 30 kg/year, as suggested by Vollenweider (1967). Our results suggest that this was not achieved. Pedrotta (2009) evaluated this load at 35 kg/year. However, her evaluation was based only on data from end of summer to October which, as shown by our data, does not include any spring inputs to the lake, which are higher in total nutrients. Furthermore, our estimates are also based on a few heavy rain events, which were never considered in other studies. Our results show that to properly evaluate the load of nutrients, data on a complete year of sampling should be taken into account. The load was measured over 9 months with the precise method from the laboratory. The inflows was much higher in April than at any other months (except during heavy rains) thus our estimate of the external load might even be slightly under-estimated.
The outflow of nutrients was generally higher during periods when the thermocline was broken, suggesting an increase in circulation. Based on these results, two major problems are thus identified:
(a). The amount (concentration and flow) of nutrients coming to the lake is high (257 kg/year) and
(b). These nutrients remain for too long in the lake. They are then used by the algae to develop, especially Microcystis. A stagnant environment favors the establishment of Microcystis which floats.
Other parameters influencing the development of Microcystis are low N:P ratios, high pH and temperature. These parameters are in Lake Muzzano, all present because they are also dependant on the nutrient budget (higher Phosphorus coming in the lake than Nitrates) and lack of circulation (temperature remains higher at the surface as well as pH).
Phosphorus Release from Sediment
During the sampling in 2012, Phosphorus could have been released from the sediment during two dates with anoxic conditions. The concentrations of Phosphorus in the lake water at the bottom were higher than at the surface during these dates. However, it seems that this Phosphorus release was less important in 2012 than in previous years when Orthophosphate concentrations were constantly higher at 3 m than at the surface (Isenburg, 1998; Hale et al., 2009).
The sediment analysis of the upper 7 cm, the most fluid part of the core, suggests that the whole amount of Mobile Phosphorus (the Phosphorus which can be released from the sediment and used for algal development) is 19.6 kg/year which is much less than the amounts coming in the lake (257 kg TP/year; 152 PO4 kg/year). Thus, only 7.6% of the Phosphorus could be released from the sediment and used by the algae. In other words, the amount of phosphorus coming from external sources is much larger than the amount possibly released from the sediment. These suggests that, for remediation, the input of nutrients should be treated before investing in “non-permanent” in-lake solutions, such as sediment removal, to avoid resuspension of phosphorus from the sediment.
Determining the Baseline Conditions as a Remediation Objective
Chironomids surviving periods of anoxia are Chironomini, C. anthracinus-type, Chironomus plumosus-type, Procladius, and Corynoneura (Quinlan et al., 1998). The chironomid analysis showed that anoxia started after ca. 1922 AD when taxa which cannot support low levels of oxygen disappeared and Chironomini, Chironomus plumosus-type, Corynoneura, and Procladius dominated the assemblages. The diatoms showed increases of TP to a level of hyper-eutrophy (>120 μg/l). The stronger anoxic period was between 1976 and 1998 with the disappearance of the most anoxia-tolerant taxon (Procladius) and many levels during this period had no chironomids. Following this period (1998–2012 AD), Procladius started to re-appear, as well as C. anthracinus-type, two taxa surviving periods of anoxia. The return of these taxa suggests a slight amelioration of the anoxic condition in the recent years.
The diatom stratigraphy showed seven zones with major changes identified by the zonation program. The most significant changes are concomitant with changes in the chironomid stratigraphy which occurred during five periods (1880–1853; 1853–1877, 1877–1922, 1922–1943, 1943–1976, 1976–1998, and 1998–2010 AD). Considering the errors in dating (±5 years) and the absence of chironomids in many samples, these changes suggest that chironomids and diatoms were influenced by factors influencing the assemblages similarly or concomitantly such as nutrients and oxygen levels. The TP inferences suggest that Lake Muzzano was mesotrophic- slightly eutrophic until 1922 AD with a substantial increase after 1922 AD when the lake became hyper-eutrophic. This hyper-eutrophic state seems to have been maintained until recently. Slight decreases in TP were observed in 2005–2006 AD but re-increased slightly to the present state.
Both indicators showed very concomitant changes. Given the extent of current human activity in the catchment, a reasonable target to which restore the lake is the conditions between 1853 and 1922, where there was human activity although not yet to a point that detrimentally affected the lake. Those are the conditions to which the ecosystem should be brought back to. The chironomids indicate that a good circulation of the lake was present as no anoxia (no taxon suggesting low oxygen levels) was recorded. The diatoms indicate a meso/eutrophic level (<80 μg/l TP) and both indicators suggest acidic conditions based on their taxonomic assemblages. The baseline conditions for nutrients are thus 50 μg/l TP (the average until 1922) with low pH.
The chironomids also show that a major problem since 1922 AD is the change in lake's circulation. At the beginning of the twentieth century, the catchment of Lake Muzzano was substantially changed with draining of the land to create fields and the diversion of many inflows to create only one major inflow and one major outflow. The changes in inflows and outflows created a decrease in the circulation of the lake and started to induce periods of anoxia. This decrease of circulation, and increase in the amount of nutrients to the lake induced a change of state from meso/eutrophic to hyper-eutrophic, which substantially contributed to the decrease of oxygen available, leading to further periods of anoxia.
With circulation, Microcystis cannot remain in suspension and reproduce. Thus, increasing the circulation when Microcystis appears in the environment would prevent its establishment. Microcystis appears generally in spring (May in Lake Muzzano), thus increasing the circulation at that time would help reducing their bloom. Another problem of stagnant environment is the creation of a thermocline blocking the exchange between the air and the deepest part of the lake. In this case, the hypolimnion becomes anoxic. Lake Muzzano is shallow (3-m deep) and is a special case. The thermocline and lack of oxygen is only created when there is no wind or precipitation and when the Microcystis are dominating the ecosystem. Our results suggest that this state of anoxia and strong thermocline would not happen in the absence of Microcystis. Thus, preventing the Microcystis to establish, in spring, before they start to overgrow, would help in reducing the problem of anoxia.
Based on our paleolimnological results, two major changes should be made to the ecosystem of Lake Muzzano.
(a). Reduce further the inflows of nutrients.
(b). Re-establish a circulation which will discourage the development of Microcystis and a thermocline blocking oxygen and heat exchanges.
Proposed Reduction of Phosphorus
The proposed reduction of Phosphorus is quantified on the baseline conditions defined by the diatoms. The present phosphorus in the lake was measured in average at 145.3 μg/l in 2011 (from present data, Cantonal Laboratory of Ticino) and inferred by diatoms at 117 μg/l. The difference between measured and inferred values is within the error of the model. Although values are not always exactly the same as the instrumental data, diatom reconstruction in various countries show that the pattern of reconstruction is consistent with instrumental data (e.g., Bigler and Hall, 2003). The baseline conditions (50 μg/l) were inferred by diatoms before 1922 AD. To obtain this concentration in the lake, the amount coming from the inflows should be reduced to 69 kg P/year.
Many solutions to reduce the amount of phosphorus exist. Some are permanent, some need to be re-applied regularly (see editorial). In the case of Lake Muzzano, since the Canton cannot invest to redo the bypass, one solution would be to add filtering plants at the exit of the main inflow. Floating platforms with Phragmites and/or Typha could be installed. Those filtration systems have shown to decrease the nutrient levels by 30–60% (OIKOS 2000). The goal of this paper is not to discuss the proposed solutions in-depth, and so limited details are given here. The reader should just be aware of the solution proposed to the Tessin Canton and Pro Natura.
Re-establish Circulation
As for phosphorus control, restoring circulation has many solutions, permanent or sporadic (see editorial in this special issue). Increasing the circulation of the lake by dilution/flushing (i.e., increasing the amount of clean water coming to the lake) is a solution which could be envisaged in Lake Muzzano. However, this solution should not be made independently of solution (a) otherwise the high nutrient concentrations will be “flushed” to Lake Lugano.
Increasing circulation would be optimal for Microcystis control as they cannot float and establish themselves in turbulent water (Zehnder and Gorham, 1960) and circulation would increase oxygen distribution through the water column preventing anoxia.
Evaluating Sediment Removal as a Possible Restoration Solution
Since the 1980's, the sediments of Lake Muzzano are highly polluted. Restoration techniques envisaged must take into account these high concentrations. Ar (Arsenic), Nickel (Ni), and Titanium (Ti) are elements naturally present in the soils and rocks, and although their concentrations can have increased with mining, the levels measured in the sediment suggest more a source from soils/rocks weathering (Pfeifer et al., 2002). Arsenic, Nickel and Titanium concentrations have been shown to increase, similarly with other heavy metals, suggesting increase weathering and transport to the lake through the inflows.
The peaks of increased concentrations in 2006–2007, 1980, and 1954 correspond to increases in organic matter percentages which also suggest an increase of material transported to the lake by increases in inflows. These periods possibly correspond to major constructions time in the area of Lake Muzzano which possibly increased the transport of organic matter and heavy metals to the lake.
In 2005–2007, the increase in heavy metal concentrations had led to a strong decrease in the diatoms/chrysophytes ratio and one deformed Chironomus was found. Chironomus is the most robust of the chironomids. If only one head capsule is deformed, it already suggests high impact on the taxon (Brooks et al., 2005). It can survive anoxia and high concentrations of heavy metals (Brooks et al., 2005). It is possible that the creation of protective areas in Lake Muzzano created weathering of the soils and increased leaching of material contained in rocks and soils to the lake. But, the effect was limited to 1–2 years. In 2011, it seems that the building of parking places between the railway and the lake might have possibly increased the amount of heavy metals entering the lake, also by weathering. This is, at the moment, the only explanation The L.A.K.E.S Institute found for the recent increase in all heavy metals. As Lake Muzzano is a protected area, it is (hopefully) unlikely that chemical products were again dumped in the lake. These results suggest that if major construction needs to be done around the lake, the weathering of heavy metals from rock and soils should be considered.
The contamination could be also treated, or at least contained, for the health of the Lake Muzzano ecosystem. There is a possibility for heavy metals to be released during period of anoxia. Although macrophytes can be used to filter heavy metals (phytoremediation), there is surely a level when they start to suffer from the high concentrations (Feiler et al., 2007). Ebadati et al. (2005) found high concentration of zinc, lead, cupper and cadmium in Phragmites australis, Typha angustifolia, and Potamogeton crispus without any problem on their growth. These plants are commonly used for filtering of heavy metals. For Lake Muzzano, the return of Nympheacea would be favorable. Their disappearance is certainly linked to the increase in phytoplankton and thus turbid water. Nympheacea have been shown to be good filters of heavy metals (Larocque-Tobler, 2013a).
For soils, contaminated sites should legally be decontaminated. There is no such law for lake sediment. The only decision to make is if the effort of decontamination will have an impact on toxicity of the present organisms. Komerek and Zeman (2004) have shown that heavy metals (Cd, Zn, Cu, Hg) are strongly bonded to the sediment and can be released only under acidic conditions in the sediment. Flyhammar and Hakansson (1999) have shown that heavy metals are released only under acidic and anoxic conditions. The presence of iron in the sediment helps the heavy metals to remain bounded. Eggleton and Thomas (2004) suggest that disturbance of the sediment (re-suspension) by benthos or by strong wind could potentially help releasing the heavy metals. For the case of Lake Muzzano, the higher pH and the presence of iron in the sediment (shown in our sediment (color red) and Isenburg et al., 2000) help for the heavy metals to remain in the sediment. With proposed solutions, if anoxia is greatly decreased, the risk of release of heavy metals will be further decreased. Sediment trap data and the Beryllium suggest that there was very little re-suspension of the sediment in 2012 (data not shown here). However, a study of the heavy metals in the water would be important to do, as heavy metals in the water could directly influence the organisms.
Options exist to limit the resuspension of heavy metals which can occur during anoxic periods. The first one is, of course, to avoid anoxic periods and this would be obtained with the solutions proposed for nutrients and circulation. Wildi et al. (2004) have looked at possible restoration techniques and suggested avoiding sediment removal as it often re-suspend the heavy metals back into the system and also contribute to the pollution of groundwater, which is often used for drinking water. In our opinion, the best solution to contain the heavy metals in the sediment to avoid re-contamination would be to use a capping agent, possibly clay material (Helioz-Rybicka and Kyziol, 1992). Discussing this solution (and others) is not part of this paper, but can be found in Larocque-Tobler (2013b). However, capping alone cannot be envisaged as a solution without having made the main solutions which are the reduction of nutrients and the increase of circulation. Capping alone is a non-permanent solution.
Other Problems
Lake Muzzano is a shallow lake (3 m depth). Shallow lakes are generally more resilient to the decrease of external nutrient inputs (Havens and Schelske, 2001) and their recovery time is about 10–15 years. This restoration time can be decreased when other processes (e.g., biomanipulation) are done at the same time as nutrient decrease (Jeppesen et al., 2007; Louette et al., 2009). The problems of restoration in shallow lakes is further discussed in this special issue.
Temperature affects the development of Microcystis (Liu et al., 2011). Summer temperature has increased in Lake Muzzano in the past 10 years (Supplementary Image 4). It is thus hypothesized that under climate change, cyanobacterial blooms might become more common in the future in Lake Muzzano. It is thus time to envisage long-term solutions to minimize the effect of warming. This topic is further discussed in other papers of this special issue.
Conclusions
This study showed that Lake Muzzano has become slightly clearer since the bypass was installed in 1999, at least for the months of May–April 2012. The chironomids suggest a slight amelioration of anoxia after 2000 but the diatoms suggest almost no amelioration in the TP level. However, the situation with Microcystis blooms and anoxia when Microcystis is established were recurrent. The blooms were due to two situations: high nutrient input and lack of circulation. Both situations occurred since ca. 1922 AD as reconstructed by diatoms and chironomids. The input of nutrients to the lake exceeded the amount possibly produced by the sediment and the amount coming out of the lake. The phosphorus analysis from the sediment showed that if it was released under anoxic conditions, the amount would still be substantially lower than the input from inflows. The concentration of heavy metals in the sediment has slightly decreased since the 1970's but are still in concentrations suggesting heavily polluted sediment. Sediment removal is thus prohibited. To bring back the ecosystem to its baseline conditions (low pH, low TP levels (50 μg/l) and oxygen above 4 mg/l, further decrease in nutrients input and increase circulation are proposed. This could be done by filtering plants and flushing/dilution. To avoid re-suspension of heavy metals from the sediment, capping is a solution which can be envisaged.
Conflict of Interest Statement
The authors declare that the research was conducted in the absence of any commercial or financial relationships that could be construed as a potential conflict of interest.
Acknowledgments
Thank you Alberto E. Conelli and Giuliano Greco from OIKOS 2000 for choosing The L.A.K.E.S Institute for this mandate and their help during sampling. Thanks also go to Martina Spinelli from Pro Natura and Massimiliano Foglia, Dipartimento del territorio, Ufficio della natura e del paesaggio for his help and discussions.
Supplementary Material
The Supplementary Material for this article can be found online at: http://journal.frontiersin.org/article/10.3389/fevo.2015.00070
References
Aquarius. (1999). Lago di Muzzano- Analyse des Causes des Mortalités de Poissons, Neuchâtel Mai 1999. Bellinzona: Rapport Aquarius.
BAFU. (2010). Recommandations Pour L'évaluation de la Qualité Hygiénique des Eaux de Baignade de Lacs et de Rivières. Bern: Office Fédéral de L'environnement, des Forêts et du Paysage (OFEFP).
Battarbee, R. W., Jones, V. J., Flower, R. J., Cameron, N. G., Bennion, H., Carvalho, L., et al. (2000). “Diatoms,” in Tracking Environmental Change Using Lake Sediments. Vol. 3. Terrestrial, Algal, and Siliceous Indicators, eds J. P. Smol, H. J. B. Birks, and W. M. Last (Dordrecht: Kluwer Academic Publishers), 155–202.
Bennett, K. D. (2009). Documentation for Psimpoll 4.27 and Pscomb 1.03. C Programs for Plotting and Analyzing Pollen Data. The 14Chrono Centre, Archaeology and Palaeoecology, Queen's University of Belfast, Belfast, UK. Available online at: http://www.chrono.574qub.ac.uk/psimpoll/psimpoll.html
Bigler, C., and Hall, R. I. (2003). Diatoms as quantitative indicators of July temperature: a validation attempt at century-scale with meteorological data from northern Sweden. Palaeogeogr. Palaeoclimatol. Palaeoecol. 189, 147–160. doi: 10.1016/S0031-0182(02)00638-7
Birks, H. J. B., and Gordon, A. D. (1985). “The analysis of pollen stratigraphical data: zonation,” in Numerical Methods in Quaternary Pollen Analysis, eds H. J. B. Birks and A. D. Gordon (London: Academic Press), 47–90.
Bottinelli, M. (1999). Fioriture di cianobatteri della specie Microcystis wesenbergii nel lago di Muzzano. Tesi Sperimentale di Laurea in Scienze Naturali, Universita degli Studi di Pavia, 172.
Bottinelli, M., Tonolla, M., Forlani, G., Crivelli, C., Sanangelantoni, A. M., and e Peduzzi, R. (2000). Fioriture di cianobatteri della specie Microcystis wesenbergii nel lago di Muzzano (Svizzera). Boll. Della Soc. Tic. Di Sci. Nat. 88, 53–61.
Bronk Ramsey, C. (2013). OxCal Version 4.2. University of Oxford Radiocarbon Accelerator Unit. Computer Program. Available online at: http://c14.arch.ox.ac.uk/embed.php?File=oxcal.html
Brooks, S. J., Udachin, V., and Williamson, B. J. (2005). Impact of copper smelting on lakes in the southern Ural Mountains, Russia, inferred from chironomids. J. Paleolimnol. 33, 229–241. doi: 10.1007/s10933-004-3936-x
Brooks, S. J. B., Heiri, O., and Langdon, P. J. (2007). Using and Identifying Chironomid Larvae in Palaeoecology, QRA Technical Guide No 10. London: Quaternary Research Association.
Ebadati, F., Esmaeeli, A., and Bakhtiari, R. (2005). Heavy metals in macrophytes and sediments of Minkaleh wetland. J. Environ. Stud. 31, 57–53.
Eggleton, J., and Thomas, K. V. (2004). A review of factors affecting the release and bioavailability of contaminants during sediment disturbance events. Environ. Int. 30, 973–980. doi: 10.1016/j.envint.2004.03.001
Feiler, U., Spira, D., and Heininger, P. (2007). “Investigation of spiked artificial and natural sediments with the myriophyllum sediment contact test,” in 17th Annual Meeting of SETAC (Porto).
Flyhammar, P., and Hakansson, K. (1999). The Release of Heavy Metals in Stabilized MSW by Oxidation. Final Report, AFR-REPORT 268, AFN, Naturvårdsverket Swedish Environmental Protection Agency 106 48 Stockholm, Sweden.
Giesy, J. P., and Hoke, R. A. (1990). “Freshwater sediment quality criteria: toxicity bioassessment,” in Sediments: Chemistry and Toxicity of In-place Pollutants, eds J. P. Giesy and H. Muntau (Chelsea, MI: Lewis Publishers), 265–348.
Hale, B. W., Hartman, K. J., and Schoenenberger, N. (2009). Condizioni e tendenze della qualità dell'acqua nel Laghetto di Muzzano, Svizzera, nel periodo maggio–ottobre 2007. Boll. Soc. Tic. Sci. Nat. 97, 67–74.
Havens, K. E., and Schelske, C. L. (2001). The importance of considering biological processes when setting total maximum daily loads (TMDL) for phosphorus in shallow lakes and reservoirs. Environ. Pollut. 113, 1–9. doi: 10.1016/S0269-7491(00)00235-9
Helioz-Rybicka, E., and Kyziol, J. (1992). Clays and clay minerals as the natural harries for heavy metals in pollution mechanisms—illustrated by Polish rivers and soils. Mitt. Osterr. Geol. Ges. 83, 163–176.
Heiri, O., Lotter, A. F., and Lemcke, G. (2001). Loss on ignition as a method for estimating organic and carbonate content in sediments: reproducibility and comparability of results. J. Paleolimnol. 25, 101–110. doi: 10.1023/A:1008119611481
Isenburg, C. (1998). Aspects Limnologiques et Microbiens du lac de Muzzano (TI). Genève: Université de Genève.
Isenburg, C., Loizeau, J.-L., Tonolla, M., and Peduzzi, R. (2000). Aspetti limnologici e microbiologici del laghetto di Muzzano (TI). Boll. Soc. Tic. Sci. Nat. 88, 41–51.
Jeppesen, R., Meerhoff, M., Jacobsen, B. A., Hansen, R. S., Sondegaard, M., Jensen, J. P., et al. (2007). Restoration of shallow lakes by nutrient control and biomanipulation—the successful strategy varies with lake size and climate. Hydrobiologia 581, 269–285. doi: 10.1007/s10750-006-0507-3
Komerek, A., and Zeman, V. (2004). Dynamics of Cu, Zn, Cd, and Hg release from sediments at surface conditions. Bull. Geosci. 79, 99–106.
Krammer, K., and Lange-Bertalot, H. (1986–1991). “Bacillariophyceae,” in Süsswasserfora von Mitteleuropa, Vol. 2 (1–4), eds H. Ettl, J. Gerloff, H. Heynig, and D. Mollenhauer (Stuttgart; New York, NY: Gustav Fischer Verlag), 876.
Larocque, I., and Rolland, N. (2006). Le Guide Visuel des Chironomides Subfossiles, du Québec à l'île d'Ellesmere. Quebec City, QC: INRS rapport de Recherche R-900.
Li, H.-E., Hun-Wei, J. H.-E., and Ming, C. A. (2003). Nutrient load estimation methods for rivers. Int. J. Sediment Res. 18, 346–351.
Liu, X., Lu, X., and Chen, W. (2011). The effects of temperature and nutrient ratios on Microcystis blooms in Lake Taihu, China: an 11-year investigation. Harmful Algae 10, 337–343. doi: 10.1016/j.hal.2010.12.002
Louette, G., Declerck, S., Vanderkechove, J., and De Meester, L. (2009). Evaluation of restoration measures in a Shallow Lake through a comparison of present day zooplankton communities with historical sample. Restor. Ecol. 17, 629–640. doi: 10.1111/j.1526-100X.2008.00409.x
Nürnberg, D., Wollenburg, I., Dethleff, D., Eicken, H., Kassens, H., Letzig, T., et al. (1994). Sediments in Arctic sea ice: implications for entrainment, transport and release. Mar. Geol. 119, 185–214. doi: 10.1016/0025-3227(94)90181-3
Pedrotta, T. (2009). Analisi Qualitativa Degli Immissari e dell'emissario del Laghetto di Muzzano. Tesi di Laurea in AGRN.
Pfeifer, H. R., Beatrizotti, G., Berthoud, J., Derossa, M., Girardet, A., Jäggli, M., et al. (2002). Natural arsenic contamination of surface and ground waters in southern Switzerland (Ticino). Bull. Appl. Geol. 7, 81–103.
Psenner, R., Pucsko, R., and Sage, M. (1998). Fractionation of Phosphorus in suspended matter and sediment. Arch. Hydrobiol. Suppl. 30, 98–103.
Quinlan, R., Hall, R. I., and Smol, J. (1998). Quantitative inferences of past hypolimnetic anoxia in south-central Ontario lakes using fossil midges (Diptera: Chironomidae). Can. J. Fish Aquatic Sci. 55, 587–596. doi: 10.1139/f97-279
Studio Blu Progetti Sagl. (2011–2012). Monitoraggio della Qualita delle Acque del Laghetto di Muzzano. Bellinzona: Repubblica e Cantone Ticino – Dipartimento del territorio – Divisione dell'ambiente.
Vermeullen, A. C., Liberloo, G., Dumont, P., Ollevier, F., and Goddereis, B. (2000). Exposure of Chironomus riparius larvae (diptera) to lead, mercury and β-sitosterol: effects on mouthpart. Chemosphere 41, 1581–1591. doi: 10.1016/S0045-6535(00)00033-3
Vollenweider, R. A. (1967). Lago di Muzzano: Valutazione del Carico in Sostanze Eutrofizzanti- Istituto Italiano di Idrobiologia. Verbania-Pallanza.
Von Gunten, L., Grosjean, M., Beer, J., Grob, P., Morales, A., and Urrutia, R. (2008). Age modeling of young non-varved lake sediments: methods and limits. Examples from two lakes in Central Chile. J. Paleolimnol. 47, 583–600. doi: 10.1007/s10933-012-9582-9
Wildi, W., Dominik, J., Loizeau, J.-L., Thomas, R.-L., and Favarger, P. Y. (2004). River, reservoir and lake sediment contamination by heavy metals downstream from urban areas of Switzerland. Lakes Reservoirs Res. Manage. 9, 75–87. doi: 10.1111/j.1440-1770.2004.00236.x
Keywords: diatoms, chironomids, heavy metals, sediment removal, anoxia, algal blooms, capping, filtering plants
Citation: Larocque-Tobler I and Pla-Rabès S (2015) Using paleolimnology to find restoration solutions: the case of Lake Muzzano, Switzerland. Front. Ecol. Evol. 3:70. doi: 10.3389/fevo.2015.00070
Received: 06 March 2015; Accepted: 15 June 2015;
Published: 01 July 2015.
Edited by:
Franco Biondi, University of Nevada, USAReviewed by:
Francoise Chalie, Centre Européen de Recherches et d'Enseignement des Géosciences de l'Environnement, FranceNeal Michelutti, Queen's University, Canada
Copyright © 2015 Larocque-Tobler and Pla-Rabès. This is an open-access article distributed under the terms of the Creative Commons Attribution License (CC BY). The use, distribution or reproduction in other forums is permitted, provided the original author(s) or licensor are credited and that the original publication in this journal is cited, in accordance with accepted academic practice. No use, distribution or reproduction is permitted which does not comply with these terms.
*Correspondence: Isabelle Larocque-Tobler, The L.A.K.E.S Institute, Bielstrasse 53 B, 3250 Lyss, Switzerland, isabelle.larocque@thelakesinstitute.com