- 1Unit of Chemical Ecology, Department of Plant Protection Biology, Swedish University of Agricultural Sciences, Alnarp, Sweden
- 2Biological Control Laboratory, Colombian Corporation for Agricultural Research, Mosquera, Colombia
The insect olfactory system discriminates odor signals of different biological relevance, which drive innate behavior. Identification of stimuli that trigger upwind flight attraction toward host plants is a current challenge, and is essential in developing new, sustainable plant protection methods, and for furthering our understanding of plant-insect interactions. Using behavioral, analytical and electrophysiological studies, we here show that both females and males of the Egyptian cotton leafworm, Spodoptera littoralis (Lepidoptera, Noctuidae), use blends of volatile compounds to locate their host plant, cotton, Gossypium hirsutum (Malvales, Malvaceae). Female S. littoralis were engaged in upwind orientation flight in a wind tunnel when headspace collected from cotton plants was delivered through a piezoelectric sprayer. Although males took off toward cotton headspace significantly fewer males than females flew upwind toward the sprayed headspace. Subsequent assays with antennally active synthetic compounds revealed that a blend of nonanal, (Z)-3 hexenyl acetate, (E)-β-ocimene, and (R)-(+)-limonene was as attractive as cotton headspace to females and more attractive to males. Two compounds, 4,8-dimethyl-1,3(E),7-nonatriene (DMNT) and (R)-(−)-linalool, both known plant defense compounds may have reduced the flight attraction of both females and males; more moths were attracted to blends without these two compounds, however, other compounds such as benzaldehyde may also be behavioral antagonists. Our findings provide a platform for further investigations on host plant signals mediating innate behavior, and for the development of novel insect plant protection strategies against S. littoralis.
Introduction
Volatile chemical compounds are important in mediating foraging, host, and mate finding behavior in insects (Bruce et al., 2005; Bengtsson et al., 2006; De Bruyne and Baker, 2008). Plant headspace consists of complex blends of dozens of compounds and it is blends, rather than single compounds that convey the specificity of signals and are essential in eliciting odor-driven behavior (De Bruyne and Baker, 2008).
Male and female moths show sexually dimorphic behavior in response to plant volatiles but it remains unclear whether they respond to different stimuli. Females rely on host plant volatiles to locate appropriate egg laying sites since plant volatiles convey information about host specificity and physiological, and phenological state of the plant (Fenemore, 1988; Renwick, 1989; Sun et al., 2003; Reddy and Guerrero, 2004; Mowrey and Portman, 2012). Although males use female-produced pheromones to locate receptive females they may use host plant volatiles to find areas where there is a higher probability of finding receptive females (Reddy and Guerrero, 2004; Trona et al., 2010, 2013; Varela et al., 2011; Party et al., 2013).
The cotton leafworm, Spodoptera littoralis (Boisduval, 1833) (Lepidoptera, Noctuidae), has become a model species for research on odor-mediated behavior and neurophysiology since its chemical ecology and physiology has been thoroughly studied (Anderson et al., 1993, 1995; Anton and Hansson, 1995; Anderson and Alborn, 1999; Jonsson and Anderson, 1999; Binyameen et al., 2012; Saveer et al., 2012). We recently showed that volatile compounds attract gravid S. littoralis females and unmated males to cotton plants (Saveer et al., 2012; Kromann et al., 2015). However, the composition of the host plant volatile blend that elicits attraction remains unknown and is the scope of this study.
Identifying host plant volatile components that mediate innate behavior in insects is not only essential to understand neurophysiological, ecological and evolutionary aspects (Hansson and Stensmyr, 2011), but also holds potential in the development of novel crop protection strategies (Cork et al., 2005; Witzgall et al., 2010). As a first step in this, we obtained robust flight attraction of female and male S. littoralis to natural cotton headspace at a known concentration using a piezoelectric sprayer. We then identified 11 antennally active compounds through GC-EAD analysis of the collected cotton headspace and out of those we managed to developed a blend composed of a subset of only four compounds that attracted significantly more moths than cotton headspace.
Materials and Methods
Insects
Spodoptera littoralis was obtained from the Dept. of Entomology, Alexandria University, Egypt. Insects were gathered in 2008 and the culture was renewed with fresh insects from Egypt at least once a year through the study period. Insects were raised on a semisynthetic agar-based diet (modified from Hinks and Byers, 1976) under a 16L:8D photoperiod, at 24°C and 50–60% relative humidity (RH). Males and females were separated as pupae into 30 × 30 × 30 cm plexiglass cages. Three-day-old old moths were used in all bioassays. Females were mated 24–27 h before testing. Adult age was monitored by shifting pupae to a new box daily.
Plant Material
Cotton seedlings (Gossypium hirsutum L., cv. Delta Pineland 90) were grown individually at 25°C and 70% RH, under daylight and an artificial light source (400 W). Cotton plants used in headspace collection had 8–10 fully developed true leaves.
Headspace Collection
Plant odor was collected using a dynamic headspace collection set-up as described by Saveer et al. (2012). A cotton plant was placed inside a 5-l glass cylinder. The bottom of the stem was held tightly in an orifice (ca. 1 cm diameter), shaped by two adjacent glass panes. Charcoal-filtered continuous air stream (350 ml/min) was pushed through the glass cylinder by an inlet pump. At the top of the cylinder, air was drawn through two outlets, situated opposite to each other, equipped with two air filters (air flow 150 ml/min, each). The excess air left the jar via the opening around the stem, avoiding the entry of unfiltered air. Volatiles were collected during 24 h (12L:12D) at 22°C from individual plants.
Air filters were made of glass tubes (4 × 40 mm), packed with 50 mg Super Q adsorbent (80/100 mesh, Altech, Deerfield, IL, USA) between glass wool plugs. The filters were rinsed with 2 ml re-distilled ethanol and n-hexane (LabScan, Malmö, Sweden) before use. The compounds collected in each column were eluted with 500 μl re-distilled n-hexane. Headspace was collected during a total of 1848 h from 40 plants. These odor collections were pooled for wind tunnel experiments, and sub-samples were condensed under a stream of nitrogen to contain 10 min equivalents/μl, transferred to glass capillaries, sealed, and kept at −20°C for use in chemical analysis.
Chemical Analysis
Plant headspace collections were analysed on a combined gas chromatography and mass spectrometry (GC–MS; 6890 GC and 5975 MS; Agilent Technologies, Santa Clara, CA, USA), operated in the electron impact ionization mode at 70 eV. The GC was equipped with fused silica capillary columns (30 m × 0.25 mm, d.f. 0.25 mm), DB-wax (J&W Scientific, Folsom, CA, USA) or HP-5MS (Agilent). Helium was used as the mobile phase at an average linear flow rate of 35 cm/s. Two microliters of each sample were injected in splitless mode during 30 s, with an injector temperature of 225°C. The GC oven temperature for both columns was programmed from 30°C (3 min hold) at 8°C/min to 225°C (5 min hold). Separation and identification of linalool and limonene enantiomers was performed on a fused silica capillary column (30 m × 0.25 mm) coated with HP-chiral 20B (d.f. 0.25 mm; Agilent). Two microliters were injected manually, splitless, during 6 s at 225°C, to enable sharp injections and narrow, separated peaks of the enantiomers. The GC was programmed from 80°C (2 min hold) at 10°C/min to 110°C, and was held isothermal for 3 min, for separation of linalool enantiomers and was programmed from 30°C (3 min hold) at 8°C/min to 225°C (10 min hold) for separation of limonene enantiomers. Compounds were identified according to retention times (Kovat's indices) and mass spectra, in comparison with a NIST library (Agilent), our own library (Alnarp 11) and authentic standards. For quantification, 100 ng of heptyl acetate (99.8% chemical purity; Aldrich) was added as an internal standard.
Electrophysiology
In order to screen the antennal active components from the natural headspace of cotton plants, we studied the antennal responses of mated female S. littoralis (n = 8−10) to pooled headspace extracts using gas chromatograph-coupled electroantennographic detection (GC-EAD) using an EAD setup (IDAC-2; Syntech; Kirchzarten, Germany) coupled to an Agilent 6890 GC. For GC-EAD recordings the above mentioned GC programs were used. At the GC effluent, 4 psi of nitrogen were added and the effluent was split 1:1 in a Gerstel 3D/2 low dead volume four-way cross (Gerstel; Mülheim, Germany) between the flame ionization detector and the EAD. The GC effluent capillary which led to the EAD passed through a Gerstel ODP-3 transfer line that tracked oven temperature and was connected to a glass tube (30 × 8 mm), where it was mixed with charcoal filtered air (18–20°C, 50 cm/s).
Antennae were cut at the base and one or two segments were inserted into a glass capillary filled with Beadle-Ephrussi Ringer that served as a recording electrode. The recording electrode was connected to a high impedance DC amplifier interphase box (IDAC-2; Syntech). Reference electrodes were prepared by cutting the glass capillary at an angle of 45° at the tip and filled with ringer solution. After cutting a distal segment, antennae were rested above the ringer solution without inserting it into a reference electrode to avoid loss of segments being exposed to the odor. Chromatograms and EAD runs were superimposed and averaged. Antennal active compounds were verified using synthetic standards on both male and female antennae.
Synthetic Mixtures and Chemicals
Synthetic cotton blends of the GC-EAD active compounds (Figure 1), in the proportions and concentrations found in cotton headspace were prepared in redistilled ethanol (Labscan) since it was determined to be a proper solvent for studies in lepidopterans (El-Sayed et al., 1999). The individual chemical compounds used in the blends were β-myrcene (97% chemical purity; Fluka), (R)-(+)-limonene (95% chemical purity; Aldrich), (E)-β-ocimene (91% chemical purity; Fluka), 4,8-dimethyl-1,3(E),7-nonatriene (DMNT) (95% chemical purity; provided by Wittko Francke), (Z)-3 hexenyl acetate (99% chemical purity; Aldrich), (R)-(−)-linalool (95% chemical purity; Firmenich), nonanal (90% chemical purity; Fluka), decanal (95% chemical purity; Sigma), benzaldehyde (99.5% chemical purity; Aldrich), β-caryophyllene (98% chemical purity; Aldrich), and α-humulene (98% chemical purity; Aldrich).
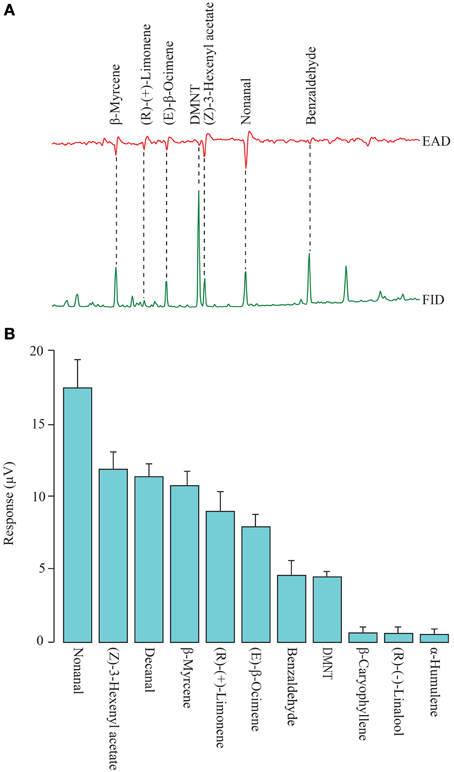
Figure 1. (A) Representative trace of the antennal response of mated Spodoptera littoralis females to natural cotton headspace extracts that were tested in the flight tunnel. FID, flame ionization detector; EAD, electroantennographic detection. (B) Antennal response for GC-EAD recordings (N = 8–10) in female moths. Bars represent 1 standard error.
Wind Tunnel Bioassays
Wind Tunnel
Flight attraction experiments of female and male S. littoralis to cotton headspace and synthetic blends were performed in a plexiglass wind tunnel (180 × 90 × 60 cm), illuminated from above and side at 6 lux, with a wind speed of 30 cm/s, temperature of 24 ± 2°C and 60 ± 10% RH. Incoming and outgoing air was filtered with active charcoal (Witzgall et al., 2001).
Males and females were kept in separate rooms in order to avoid pre-exposing males to pheromone before experiments. About 1 h before experiments moths were transferred to individual 2.5 × 12.5 cm glass tubes closed with gauze, which were kept in the wind tunnel room before testing, to allow them to acclimatize to the environment. Experiments were carried out between 1 and 4 h after the onset of the scotophase.
Unmated male and mated female moths were placed individually in glass tubes on a platform at the downwind end of the tunnel and observed for 5 min. 40 individual insects were used for every treatment. The following steps of the behavioral sequence were recorded: activation (walking in the tube and wing-fanning), takeoff, upwind flight (over 90 cm from takeoff), source approach (30 cm from source), and landing at the source.
Odor Delivery
Cotton headspace collections and synthetic odor blends were delivered from the center of the upwind end of the wind tunnel via a piezo-electric spraying mechanism (El-Sayed et al., 1999; Becher et al., 2010). Samples were loaded into a 1-ml glass syringe operated by a microinjection pump (CMA Microdialysis AB, Solna, Sweden) that delivered test solutions at a constant rate of 10 μl/min through Teflon tubing into a glass capillary with a narrow, elongated tip. The capillary was attached to a piezo-ceramic disk, which vibrated at ~100 kHz, producing an aerosol that was carried downwind. A glass cylinder (95 mm diameter × 100 mm height), covered by a fine metallic mesh (pore size 2 mm) was placed in front of the capillary to avoid it being damaged by insect contact. Synthetic blends were formulated to mimic natural headspace concentrations and release equivalents from plants for spraying in experiments.
Statistical Analysis
Generalized linear models (GLM) with a Bernoulli binomial distribution were used to analyze behavioral data. Takeoff, upwind flight and sex were used as the target effects. Post-hoc Wald pairwise comparison tests were used to identify differences between treatments. Significance was determined at α = 95%, however, some treatments were considered to be significantly different at α = 94% and are indicated in the results. All statistical analysis were carried out using SPSS v.20 and R (IBM Corp, 2013; R Core Team, 2013).
Results
Attraction to Sprayed Cotton Headspace
Previously, we have shown robust upwind flight attraction of mated female S. littoralis to cotton plants in a wind tunnel study, where 75% of the females initiated flight, 40% flew upwind over 150 cm and 22% contacted the plant (Saveer et al., 2012). In comparison we here show that fewer mated females responded to re-vaporized, sprayed headspace extracts from the cotton plants: 35% of the test females took off and 10% flew upwind. Only 18% of unmated males responded to the sprayed extract by taking off and none flew upwind. Females were more attracted to sprayed cotton headspace than males, but the difference was not significant (z = 1.811, p = 0.0701; Figure 2). Females and males did not respond to sprayed ethanol alone (0% takeoff for both males and females) or an absolute blank (0% takeoff for females and 2% for males).
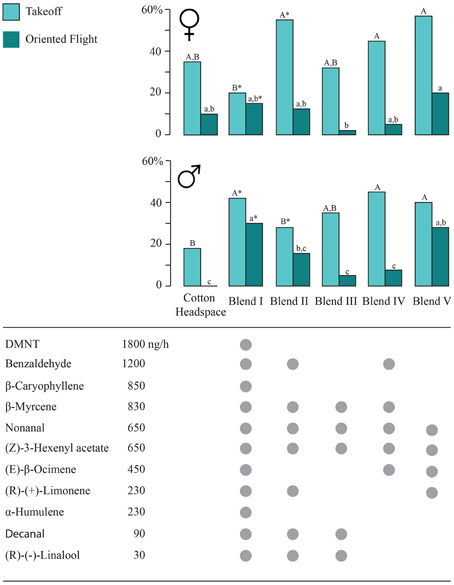
Figure 2. Flight response of Spodoptera littoralis females and males to sprayed cotton headspace, and synthetic blends using a piezoelectric sprayer in a flight tunnel assay. Headspace was released at 1800 ng/h of the main compound DMNT. Gray circles indicate which compounds are included in each blend. Different letters indicate statistical differences between the blends, and asterisks indicate treatments which differ between males and females. A generalized linear model (GLM) with a Bernoulli binomial distribution and post-hoc Wald pairwise comparison tests were used to identify differences between treatments (N = 50).
Antennal Active Compounds and Synthetic Blends
GC-EAD recordings showed that seven compounds, β-myrcene, (R)-(+)-limonene, (E)-β-ocimene, 4,8-dimethyl-1,3(E),7-nonatriene (DMNT), (Z)-3-hexenyl acetate, nonanal, and benzaldehyde, evoked a consistent antennal response in all recordings (n = 8−10, Figure 1). Four additional compounds, (R)-(−)-linalool, decanal, β-caryophyllene and α-humulene, occasionally elicited a response. The response to these compounds was verified through EAG with synthetic standards. The most abundant antennally active compounds in cotton headspace were DMNT and benzaldehyde (Figure 1).
Attraction to Sprayed Synthetic Blends
A series of wind tunnel experiments was conducted to determine the behaviorally salient odorants (Figure 2) that were identified through GC-EAD analysis from the natural headspace extracts (Figure 1). An 11-component synthetic blend (Blend I), containing all antennally active compounds, released at the same ratios and rates, attracted more males than females (Figure 2). Significantly more males took off (42%) and flew upwind (30%); in comparison, 20% of the females took flight, and 15% of them flew upwind (z = 3.82, p = 0.019; z = 1.89, p = 0.053 for takeoff and upwind flight, respectively). This is in contrast with the behavioral response to headspace, which attracted more females than males (Figure 2).
All synthetic blends tested (Blend I–V) were as attractive, or more attractive than cotton headspace in both sexes. The four-component blend (Blend V; containing nonanal, (Z)-3 hexenyl acetate, (E)-β-ocimene, and (R)-(−)-linalool) was the most attractive blend for females, eliciting the highest percentage of takeoff and upwind flight. Blend V attracted significantly more males than cotton headspace and did not differ significantly from Blend I. Blend V was significantly more attractive than the blends III and IV in both males and females (Figure 2).
Discussion
The cotton leafworm, S. littoralis, relies on olfactory signals to locate food, hosts and mating sites. Our previous studies have shown that the olfactory response is plastic, as males and females respond to odor signals in accordance to their internal physiological state (Saveer et al., 2012; Kromann et al., 2015). However, it was unknown which chemicals mediate the flight attraction response. Here we show that a 4-component blend of plant volatiles mediates attraction of S. littoralis males and females to their host plant cotton, G. hirsutum (Figure 2).
Cotton headspace induced a strong attraction response in females but failed to attract males (Figure 2). Using the wind tunnel protocol of Saveer et al. (2012) and Kromann et al. (2015), fewer moths responded to sprayed cotton headspace than to live cotton plants. Neither headspace nor Blend I, containing all antennally active compounds found in headspace, elicited landings at the source, whereas ~20% landed on cotton plants (Saveer et al., 2012; Kromann et al., 2015).
The discrepancy between the attraction to cotton plants and to cotton headspace could be due to the sprayer device, which was used to release headspace. An alternative explanation is differences between the chemical composition of plant headspace and our headspace collections.
The piezo sprayer delivers vaporized solutions at a known purity and rate, and makes it possible to compare the behavioral response to plant headspace and synthetic mimics. In comparison, passive dispensers, such as filter paper and rubber septa, release chemicals at unknown, exponentially decreasing rates and distort blend proportions, depending to compound vapor pressure (El-Sayed et al., 1999; Tasin et al., 2006a,b). However, the sprayer does not provide a visual stimulus, which may affect the landing and flight response, since insects integrate olfactory and visual stimuli during upwind flight (Reisenman et al., 2000; Balkenius and Dacke, 2010).
Subsets of the compounds found in cotton headspace are sufficient to elicit upwind flight, and a 4-component blend is more attractive than the complete 11-component blend (Figure 2). This leads us to believe that our headspace collections contained attraction antagonists, which may be released by plants that may have been damaged or stressed during volatile collections (Tasin et al., 2005; Zakir et al., 2012).
We hypothesize that the presence of known plant defense compounds, such as (R)-(−)-linalool and DMNT, in our cotton headspace may reduce attraction of males and females to cotton headspace and synthetic blends. However, we cannot exclude the possibility that other compounds are involved in the observed reduction in attraction of Blends III and IV. (R)-(−)-linalool and DMNT are produced in response S. littoralis feeding on cotton and are well-known defense compounds (Loughrin et al., 1994; Turlings et al., 1995; Anderson and Alborn, 1999; Zakir et al., 2012; Zakir Ali, 2012; Allmann et al., 2013). That these compounds are detected by olfactory sensory neurons in S. littoralis (Binyameen et al., 2012; Saveer et al., 2012), further corroborates their biological relevance.
Linalool has been shown to both reduce firing of pheromone sensitive neurons in S. littoralis (Party et al., 2009) and to interfere with orientation on a locomotion compensator (Party et al., 2013). Xiao et al. (2012) found that rice lines producing (S)-(+)-linalool attract both herbivores and predators and parasitoids. In Manduca sexta (Linnaeus), the enantiomers of linalool have a different behavioral effect: females preferentially oviposit on plants emitting (S)-(+)-linalool, while they prefer control plants over plants emitting (R)-(−)-linalool (Reisenman et al., 2010). These results concur with our wind tunnel study and corroborate the idea that (R)-(−)-linalool is in part responsible for reduced attraction to Blends I, II, and particularly to Blend III.
DMNT has often been associated with the attraction of predators and parasitoids, since it is commonly found among herbivore-induced plant volatile bouquets. The effect of DMNT as a single compound has only been shown in the predatory mite Phytoseiulus persimilis (Athias-Henriot) (Dicke et al., 1990; De Boer et al., 2004), while blends containing DMNT have been studied in many herbivores, predators or parasitoids (Hoballah et al., 2002; Kappers et al., 2005; Kos et al., 2013; Mccormick et al., 2014). Larvae of Spodoptera frugiperda (Smith) and Lobesia botrana (Dennis and Schiffermüller) are attracted to a blend of host volatiles and DMNT (Carroll et al., 2008; Becher and Guerin, 2009). DMNT does not reduce attraction of grape berry moth Paralobesia viteana (Clemens) and the European grapevine moth L. botrana in wind tunnel assays (Tasin et al., 2006a, 2007; Cha et al., 2008, 2011). Taken together, these studies show that the effect of DMNT is context-dependent and further studies are needed to determine its role in S. littoralis host attraction.
Our data support the long-standing assertion that host plant finding by gravid females of herbivorous insects is mediated by plant volatiles (Bruce et al., 2005), and lends support to the idea that males also use plant volatiles to orient toward host plants in order to find females more efficiently (Dickens et al., 1993; Coracini et al., 2004; Von Arx et al., 2012; Trona et al., 2013). Along with the literature on induced defense compounds, our results suggest that (R)-(−)-linalool and DMNT have an antagonistic effect on S. littoralis host plant attraction. Further studies on potential antagonists are necessary since this study unable to rule out the antagonistic effect of other compounds such as benzaldehyde and β-myrcene. Identification of a simple, four-component blend that elicits attraction in both males and females of S. littoralis will facilitate further studies on the physiology, ecology, and behavior of this species.
Conflict of Interest Statement
The authors declare that the research was conducted in the absence of any commercial or financial relationships that could be construed as a potential conflict of interest.
Acknowledgments
Special thanks to Medhat Sadek and Mohamed Khallaf from Assiut University for their help in providing insects from the field. This study was supported by the Linnaeus environment “Insect Chemical Ecology, Ethology and Evolution” IC-E3 (Formas, SLU), The Colombian Corporation for Agricultural Research (Corpoica) and the Colombian Administrative Department of Science, Technology, and Innovation (Colciencias).
References
Allmann, S., Spaethe, A., Bisch-Knaden, S., Kallenbach, M., Reinecke, A., Sachse, S., et al. (2013). Feeding-induced rearrangement of green leaf volatiles reduces moth oviposition. Elife 2:e00421. doi: 10.7554/eLife.00421
Anderson, P., and Alborn, H. (1999). Effects on oviposition behaviour and larval development of Spodoptera littoralis by herbivore-induced changes in cotton plants. Entomol. Exp. Appl. 92, 45–51. doi: 10.1046/j.1570-7458.1999.00523.x
Anderson, P., Hansson, B. S., and Lofqvist, J. (1995). Plant odor specific receptor neurons on the antennae of female and male Spodoptera littoralis. Physiol. Entomol. 20, 189–198. doi: 10.1111/j.1365-3032.1995.tb00001.x
Anderson, P., Hilker, M., Hansson, B. S., Bombosch, S., Klein, B., and Schildknecht, H. (1993). Oviposition deterring components in larval frass of Spodoptera littoralis (Boisd) (Lepidoptera, Noctuidae): a behavioral and electrophysiological evaluation. J. Insect Physiol. 39, 129–137. doi: 10.1016/0022-1910(93)90104-y
Anton, S., and Hansson, B. S. (1995). Sex pheromone and plant-associated odor processing in antennal lobe interneurons of male Spodoptera littoralis (Lepidoptera, Noctuidae). J. Comp. Physiol. A 176, 773–789.
Balkenius, A., and Dacke, M. (2010). Flight behaviour of the hawkmoth Manduca sexta towards unimodal and multimodal targets. J. Exp. Biol. 213, 3741–3747. doi: 10.1242/jeb.043760
Becher, P. G., Bengtsson, M., Hansson, B. S., and Witzgall, P. (2010). Flying the fly: long-range flight behavior of Drosophila melanogaster to attractive odors. J. Chem. Ecol. 36, 599–607. doi: 10.1007/s10886-010-9794-2
Becher, P. G., and Guerin, P. M. (2009). Oriented responses of grapevine moth larvae Lobesia botrana to volatiles from host plants and an artificial diet on a locomotion compensator. J. Insect Physiol. 55, 384–393. doi: 10.1016/j.jinsphys.2009.01.006
Bengtsson, M., Jaastad, G., Knudsen, G., Kobro, S., Backman, A. C., Pettersson, E., et al. (2006). Plant volatiles mediate attraction to host and non-host plant in apple fruit moth, Argyresthia conjugella. Entomol. Exp. Appl. 118, 77–85. doi: 10.1111/j.1570-7458.2006.00359.x
Binyameen, M., Anderson, P., Ignell, R., Seada, M. A., Hansson, B. S., and Schlyter, F. (2012). Spatial organization of antennal olfactory sensory neurons in the female Spodoptera littoralis moth: differences in sensitivity and temporal characteristics. Chem. Senses 37, 613–629. doi: 10.1093/chemse/bjs043
Boisduval, J. A. (1833). Faune Entomologique de Madagascar, Bourbon et Maurice: Lépidoptères. Paris: Librairie Encyclopédique de Roret.
Bruce, T. J. A., Wadhams, L. J., and Woodcock, C. M. (2005). Insect host location: a volatile situation. Trends Plant Sci. 10, 269–274. doi: 10.1016/j.tplants.2005.04.003
Carroll, M. J., Schmelz, E. A., and Teal, P. E. A. (2008). The attraction of Spodoptera frugiperda neonates to cowpea seedlings is mediated by volatiles induced by conspecific herbivory and the elicitor inceptin. J. Chem. Ecol. 34, 291–300. doi: 10.1007/s10886-007-9414-y
Cha, D. H., Linn, C. E. Jr. Teal, P. E., Zhang, A., Roelofs, W. L., and Loeb, G. M. (2011). Eavesdropping on plant volatiles by a specialist moth: Significance of ratio and concentration. PLoS ONE 6:e17033. doi: 10.1371/journal.pone.0017033
Cha, D. H., Nojima, S., Hesler, S. P., Zhang, A., Linn, C. E.Jr. Roelofs, W. L., et al. (2008). Identification and field evaluation of grape shoot volatiles attractive to female grape berry moth (Paralobesia viteana). J. Chem. Ecol. 34, 1180–1189. doi: 10.1007/s10886-008-9517-0
Coracini, M., Bengtsson, M., Liblikas, I., and Witzgall, P. (2004). Attraction of codling moth males to apple volatiles. Entomol. Exp. Appl. 110, 1–10. doi: 10.1111/j.0013-8703.2004.00124.x
Cork, A., Alam, S. N., Rouf, F. M. A., and Talekar, N. S. (2005). Development of mass trapping technique for control of brinjal shoot and fruit borer, Leucinodes orbonalis (Lepidoptera: Pyralidae). Bull. Entomol. Res. 95, 589–596. doi: 10.1079/ber2005389
De Boer, J. G., Posthumus, M. A., and Dicke, M. (2004). Identification of volatiles that are used in discrimination between plants infested with prey or nonprey herbivores by a predatory mite. J. Chem. Ecology 30, 2215–2230. doi: 10.1023/B:JOEC.0000048784.79031.5e
De Bruyne, M., and Baker, T. C. (2008). Odor detection in insects: Volatile codes. J. Chem. Ecology 34, 882–897. doi: 10.1007/s10886-008-9485-4
Dicke, M., Sabelis, M. W., Takabayashi, J., Bruin, J., and Posthumus, M. A. (1990). Plant strategies of manipulating predator-prey interactions through allelochemicals - Prospects for application in pest-control. J. Chem. Ecol. 16, 3091–3118. doi: 10.1007/bf00979614
Dickens, J. C., Smith, J. W., and Light, D. M. (1993). Green leaf volatiles enhance sex attractant pheromone of the tobacco budworm, Heliothis virescens (Lep.: Noctuidae). Chemoecology 4, 175–177. doi: 10.1007/bf01256553
El-Sayed, A., Godde, J., and Arn, H. (1999). Sprayer for quantitative application of odor stimuli. Environ. Entomol. 28, 947–953.
Fenemore, P. G. (1988). Host-plant location and selection by adult potato moth, Phthorimaea operculella (Lepidoptera: Gelechiidae): a review. J. Insect Physiol. 34, 175–177.
Hansson, B. S., and Stensmyr, M. C. (2011). Evolution of insect olfaction. Neuron 72, 698–711. doi: 10.1016/j.neuron.2011.11.003
Hinks, C., and Byers, J. (1976). Biosystematics of the genus Euxoa (Lepidoptera: Noctuidae): V. Rearing procedures, and life cycles of 36 species. Can. Entomol. 108, 1345–1357.
Hoballah, M. E. F., Tamo, C., and Turlings, T. C. J. (2002). Differential attractiveness of induced odors emitted by eight maize varieties for the parasitoid Cotesia marginiventris: is quality or quantity important? J.Chem. Ecol. 28, 951–968. doi: 10.1023/a:1015253600083
Jonsson, M., and Anderson, P. (1999). Electrophysiological response to herbivore-induced host plant volatiles in the moth Spodoptera littoralis. Physiol. Entomol. 24, 377–385. doi: 10.1046/j.1365-3032.1999.00154.x
Kappers, I. F., Aharoni, A., Van Herpen, T., Luckerhoff, L. L. P., Dicke, M., and Bouwmeester, H. J. (2005). Genetic engineering of terpenoid metabolism attracts, bodyguards to Arabidopsis. Science 309, 2070–2072. doi: 10.1126/science.1116232
Kos, M., Houshyani, B., Overeem, A. J., Bouwmeester, H. J., Weldegergis, B. T., Van Loon, J. J. A., et al. (2013). Genetic engineering of plant volatile terpenoids: effects on a herbivore, a predator and a parasitoid. Pest Manag. Sci. 69, 302–311. doi: 10.1002/ps.3391
Kromann, S. H., Saveer, A. M., Binyameen, M., Bengtsson, M., Birgersson, G., Hansson, B. S., et al. (2015). Concurrent modulation of neuronal and behavioural olfactory responses to sex and host plant cues in a male moth. Proc, R. Soc. Lond. Ser. B Biol. Sci. 282, 20141884–20141884. doi: 10.1098/rspb.2014.1884
Loughrin, J. H., Manukian, A., Heath, R. R., Turlings, T., and Tumlinson, J. H. (1994). Diurnal cycle of emission of induced volatile terpenoids by herbivore-injured cotton plant. Proc. Natl. Acad. Sci. U.S.A. 91, 11836–11840.
Mccormick, A. C., Irmisch, S., Reinecke, A., Boeckler, G. A., Veit, D., Reichelt, M., et al. (2014). Herbivore-induced volatile emission in black poplar: regulation and role in attracting herbivore enemies. Plant Cell Environ. 37, 1909–1923. doi: 10.1111/pce.12287
Mowrey, W. R., and Portman, D. S. (2012). Sex differences in behavioral decision-making and the modulation of shared neural circuits. Biol. Sex Differ. 3:8. doi: 10.1186/2042-6410-3-8
Party, V., Hanot, C., Büsser, D. S., Rochat, D., and Renou, M. (2013). Changes in odor background affect the locomotory response to pheromone in moths. PLoS ONE 8:e52897. doi: 10.1371/journal.pone.0052897
Party, V., Hanot, C., Said, I., Rochat, D., and Renou, M. (2009). Plant terpenes affect intensity and temporal parameters of pheromone detection in a moth. Chem. Senses 34, 763–774. doi: 10.1093/chemse/bjp,060
R Core Team. (2013). R: A Language and Environment for Statistical Computing. R foundation for statistical computing, Vienna. Available online at: http://www.R-project.org/
Reddy, G. V. P., and Guerrero, A. (2004). Interactions of insect pheromones and plant semiochemicals. Trends Plant Sci. 9, 253–261. doi: 10.1016/j.tplants.2004.03.009
Reisenman, C. E., Figueiras, A. N. L., Giurfa, M., and Lazzari, C. R. (2000). Interaction of visual and olfactory cues in the aggregation behaviour of the haematophagous bug Triatoma infestans. J. Comp. Physiol. A 186, 961–968. doi: 10.1007/s003590000149
Reisenman, C. E., Riffell, J. A., Bernays, E. A., and Hildebrand, J. G. (2010). Antagonistic effects of floral scent in an insect-plant interaction. Proc. R. Soc. B Biol. Sci. 277, 2371–2379. doi: 10.1098/rspb.2010.0163
Renwick, J. A. A. (1989). Chemical ecology of oviposition in phytophagous insects. Experientia 45, 223–228. doi: 10.1007/bf01951807
Saveer, A. M., Kromann, S. H., Birgersson, G., Bengtsson, M., Lindblom, T., Balkenius, A., et al. (2012). Floral to green: mating switches moth olfactory coding and preference. Proce. R. Soc. 279, 2314–2322. doi: 10.1098/rspb.2011.2710
Sun, F., Du, J.-W., and Chen, T.-H. (2003). The behavioral responses of Spodoptera litura (F.) males to the female sex pheromone in wind tunnel and field trapping tests. Acta Entomologica Sinica 46, 126–130.
Tasin, M., Anfora, G., Ioriatti, C., Carlin, S., De Cristofaro, A., Schmidt, S., et al. (2005). Antennal and behavioral responses of grapevine moth Lobesia botrana females to volatiles from grapevine. J. Chem. Ecol. 31, 77–87. doi: 10.1007/s10886-005-0975-3
Tasin, M., Bäckman, A.-C., Bengtsson, M., Ioriatti, C., and Witzgall, P. (2006a). Essential host plant cues in the grapevine moth. Naturwissenschaften 93, 141–144. doi: 10.1007/s00114-005-0077-7
Tasin, M., Backman, A. C., Bengtsson, M., Varela, N., Ioriatti, C., and Witzgall, P. (2006b). Wind tunnel attraction of grapevine moth females, Lobesia botrana, to natural and artificial grape odour. Chemoecology 16, 87–92. doi: 10.1007/s00049-005-0332-6
Tasin, M., Bäckman, A.-C., Coracini, M., Casado, D., Ioriatti, C., and Witzgall, P. (2007). Synergism and redundancy in a plant volatile blend attracting grapevine moth females. Phytochemistry 68, 203–209. doi: 10.1016/j.phytochem.2006.10.015
Trona, F., Anfora, G., Balkenius, A., Bengtsson, M., Tasin, M., Knight, A., et al. (2013). Neural coding merges sex and habitat chemosensory signals in an insect herbivore. Proc. R. Soc. B 280, 1–8. doi: 10.1098/rspb.2013.0267
Trona, F., Anfora, G., Bengtsson, M., Witzgall, P., and Ignell, R. (2010). Coding and interaction of sex pheromone and plant volatile signals in the antennal lobe of the codling moth Cydia pomonella. J. Exp. Biol. 213, 4291–4303. doi: 10.1242/jeb.047365
Turlings, T. C. J., Loughrin, J. H., Mccall, P. J., Rose, U. S. R., Lewis, W. J., and Tumlinson, J. H. (1995). How caterpillar-damaged plants protect themselves by attracting parasitic wasps. Proc. Natl. Acad. Sci. U.S.A. 92, 4169–4174. doi: 10.1073/pnas.92.10.4169
Varela, N., Avilla, J., Anton, S., and Gemeno, C. (2011). Synergism of pheromone and host-plant volatile blends in the attraction of Grapholita molesta males. Entomol. Exp. Appl. 141, 114–122. doi: 10.1111/j.1570-7458.2011.01171.x
Von Arx, M., Schmidt-Busser, D., and Guerin, P. M. (2012). Plant volatiles enhance behavioral responses of grapevine moth males, Lobesia botrana to sex pheromone. J. Chem. Ecol. 38, 222–225. doi: 10.1007/s10886-012-0068-z
Witzgall, P., Bengtsson, M., Rauscher, S., Liblikas, I., Backman, A. C., Coracini, M., et al. (2001). Identification of further sex pheromone synergists in the codling moth, Cydia pomonella. Entomol. Exp. Appl. 101, 131–141. doi: 10.1046/j.1570-7458.2001.00898.x
Witzgall, P., Kirsch, P., and Cork, A. (2010). Sex pheromones and their impact on pest management. J. Chem. Ecol. 36, 21. doi: 10.1007/s10886-009-9737-y
Xiao, Y., Wang, Q., Erb, M., Turlings, T. C. J., Ge, L., Hu, L., et al. (2012). Specific herbivore-induced volatiles defend plants and determine insect community composition in the field. Ecol. Lett. 15, 1130–1139. doi: 10.1111/j.1461-0248.2012.01835.x
Keywords: Spodoptera littoralis, Egyptian cotton leafworm, kairomone, host plant volatiles, headspace, upwind flight, wind tunnel, electrophysiology
Citation: Borrero-Echeverry F, Becher PG, Birgersson G, Bengtsson M, Witzgall P and Saveer AM (2015) Flight attraction of Spodoptera littoralis (Lepidoptera, Noctuidae) to cotton headspace and synthetic volatile blends. Front. Ecol. Evol. 3:56. doi: 10.3389/fevo.2015.00056
Received: 31 December 2014; Accepted: 17 May 2015;
Published: 03 June 2015.
Edited by:
Astrid T. Groot, University of Amsterdam, NetherlandsCopyright © 2015 Borrero-Echeverry, Becher, Birgersson, Bengtsson, Witzgall and Saveer. This is an open-access article distributed under the terms of the Creative Commons Attribution License (CC BY). The use, distribution or reproduction in other forums is permitted, provided the original author(s) or licensor are credited and that the original publication in this journal is cited, in accordance with accepted academic practice. No use, distribution or reproduction is permitted which does not comply with these terms.
*Correspondence: Felipe Borrero-Echeverry, Unit of Chemical Ecology, Department of Plant Protection Biology, Swedish University of Agricultural Sciences, PO Box 102, Alnarp 230 53, Sweden,ZmVsaXBlLmJvcnJlcm9Ac2x1LnNl
†Present Address: Ahmed M. Saveer, Department of Biological Sciences, Vanderbilt University, Nashville, USA