- 1Animal Behavior Graduate Group, Department of Neurobiology Physiology and Behavior, University of California, Davis, Davis, CA, USA
- 2Department Biology II, Ludwig-Maximilians University of Munich, Munich, Germany
- 3Department of Biology, University of Oulu, Oulu, Finland
- 4Department of Biology, University of Eastern Finland, Joensuu, Finland
Despite the ever increasing interest in animal personalities, i.e., among-individual variation in behavior, there are still several gaps in our understanding of how experiences during ontogeny influence the expression of behavior in adulthood. Immune challenges during ontogeny have been proposed to drive feedback loops between investment in immune function and personality type. In this study we investigate the effects of an early immune challenge, in the form of an introduced bacterial pathogen, on the development of personality in field crickets. Our results indicate that early pathogen exposure does not influence life history characteristics, immune response, or mean level of boldness behavior. Instead, early immune challenge affects the presence of personality later in the adult stage. Specifically, immune challenged individuals lack repeatability in some aspects of boldness behavior, indicating that among-individual variation is not present, while non-immune challenged individuals remain repeatable in their boldness behavior. This study joins a slowly growing body of literature indicating that experiences during ontogeny can have large influences on the among-individual differences in behaviors, thus affecting the presence of personality as adults.
Introduction
A wealth of literature shows that animal personalities occur commonly across various taxa (Sih et al., 2004; Dingemanse and Réale, 2005; Bell et al., 2009). These personalities consist of among-individual level behavioral differences that are maintained over time and/or contexts, and result in some individuals being consistently more bold, active, or aggressive, compared to other conspecifics within a population (Bell and Stamps, 2004; Dingemanse et al., 2004; Dochtermann and Jenkins, 2007; Kortet and Hedrick, 2007; Pruitt et al., 2008, 2011). Personalities have been shown to both influence and be influenced by a range of ecological processes (Smith and Blumstein, 2008; Cote et al., 2011; Fogarty et al., 2011; Sih et al., 2012; Wolf and Weissing, 2012). Despite the continuously increasing interest in animal personality, there still exist several major gaps in our understanding of the environmental processes which affect the expressed variation in behavior. One of the suggested ecological forces that may generate and maintain behavioral variation is the experienced pathogenic environment (Kortet et al., 2010). Specifically, exposure to pathogens may drive feedback loops that create or diminish individual differences in behavior (i.e., increased immune function resulting from pathogen exposure facilitates increased boldness/aggression/exploration by reducing risk of parasitism/disease). One major challenge in the field is to understand the role of early life experiences, specifically that of parasitic infections, in explaining the development of personality over ontogeny, especially in relation to the simultaneous development of the immune system. In this study we investigate the effects of juvenile exposure to an opportunistic pathogenic bacteria on expression of mean level of adult boldness behavior, variation in individual boldness scores, and immune response using the cricket, Gryllus integer.
The role of early life experience in the development of animal personalities has been given relatively little attention outside of a few model systems (Higley et al., 1991; Caspi et al., 2005; Groothuis et al., 2008). Furthermore, the majority of current research on personality focuses predominantly on adult individuals, and does not consider the role of ontogeny in determining personality. Overlooking the importance of juvenile life stages may lead to misinterpretations as to how stable personality is over time. Several conceptual papers address the importance of early life experience, and discuss the associated implications (Stamps and Groothuis, 2010a,b; Groothuis and Trillmich, 2011). Generally, variation in experiences during ontogeny could cause variation in the expression of developmental plasticity, and in turn alter the consistency of behavior across both time and context, as well as correlations between multiple personality traits (Stamps and Groothuis, 2010b). This could be a byproduct of the costs of plasticity varying over ontogeny, such that the costs of plasticity during development are lower than the costs of plasticity during adulthood (Hoverman and Relyea, 2007). Indeed, several studies have shown that adult personality traits are sensitive to environmental conditions during ontogeny (Butler et al., 2012; DiRienzo et al., 2012; Bengston et al., 2014). For example, crickets reared in the presence of conspecific acoustic signals are less aggressive than those reared in the absence of conspecific acoustic signals (DiRienzo et al., 2012). Given these potentially significant contributions of early life experiences to adult behavioral phenotype, it is important to increase our knowledge about the role of experiential factors during ontogeny in determining adult personality.
We do not currently understand how individuals solve predicted tradeoffs between personality and investment in immune function or parasite tolerance (Kortet et al., 2010). Immune function in itself is costly, both to develop and use, and might force organisms to make tradeoffs with other energetically costly traits, including behaviors (e.g., activity, aggression) (Kortet et al., 2010). The freshwater snail, Lymnea stagnalis, exhibits a negative correlation between predator-avoidance behavior and immune function, such that individuals who devote more time to avoidance behaviors suffer from decreased immune function (Rigby and Jokela, 2000; Barber and Dingemanse, 2010). Alternatively, immune function-personality interactions may drive positive feedback loops between the two. Intrinsically efficient immunity or high investment in immune function due to stochastic events early in life may allow for increased expression of costly traits such as boldness, activity, and aggression which attract and/or increase exposure to parasites, but further help the individuals to invest more in immunity through the ability to acquire more resources (Kortet et al., 2010). Additionally, such positive feedback loops may promote consistent among-individual differences in behavior, thus driving the development of personality (Kortet et al., 2010; Luttbeg and Sih, 2010). Understanding how personality is related to immune function is important because behaviors can affect fitness by altering the rate at which an individual encounters pathogens in its environment (Kortet et al., 2010). For example, Wilson et al. (1993) found that in the wild pumpkinseed sunfish, Lepomis gibbosus, individuals who were caught in traps, and thus deemed more exploratory, carried higher levels of certain pathogens (“blackspot” infection) relative to the population as a whole. Interestingly, the same exploratory fish also harbored lower levels of other pathogens (“white grub” infection), suggesting that behaviors do not always associate with parasite loads as expected, or alternatively are driven by other state-dependent factors not identified in these experiments.
Early life experience and investment in immune system can undoubtedly interact with one another to affect later adult personality, although how they interact is currently poorly understood (Kortet et al., 2010). The development of the immune system could be directly related to expression of personality later in life. For example, in insects immune challenges at an early age have been shown to produce long-lasting immune system up-regulation or immune priming that can persist for a large proportion of an organism's lifespan (Moret and Siva-Jothy, 2003; Sadd and Schmid-Hempel, 2006). Such an investment could directly affect the level of adult behavioral expression. Indeed, in both rodents and mallard ducks, individuals that experienced an immune challenge as juveniles were found to have more exploratory and active personality types as adults relative to those not given an immune challenge (Rico et al., 2010; Butler et al., 2012). It is hypothesized that such an exposure might be a signal of a pathogen-dense environment, and the associated increases in activity levels might promote the search for a less pathogen-dense environment (Kortet et al., 2010). Still, evidence regarding how exposure to pathogens early in life affects the development of behavioral expression is limited, and it is unknown if juvenile pathogen exposure has a consistent positive effect on later personality type.
The goal of our experiments was to probe the interplay of individuals' early life experiences in the form of a bacterial infection and study how the pathogenic exposure affects investment in immune function and subsequent expression of boldness (i.e., the willingness to expose oneself in a novel, potentially risky environment). We focused on the following three questions: (1) How does exposure to pathogens as juveniles affect among-individual level variation in behavior as adults (i.e., personality)? (2) Does exposure to pathogens as juveniles affect the relationship between immune function and personality as adults? (3) How is adult mean level of behavior affected by pathogen exposure in the juvenile stage? We studied these questions by exposing juvenile field crickets to either a pathogenic bacteria or control solution, and then measured boldness behavior repeatedly as adults. After the behavioral measurements, we assessed two measures of immune function: Phenoloxidase activity, which when activated produces secondary components that aid in pathogen defense (González-Santoyo and Córdoba-Aguilar, 2012), and encapsulation response, which is an immune response to multicellular foreign bodies (Paskewitz and Riehle, 1994; Gillespie et al., 1997). We predicted that (1) juveniles exposed to pathogens would display greater among-individual variation in behavior relative to those not exposed to pathogens as a result of positive feedback loops between immune function and boldness behavior. We predicted that (2) groups exposed to pathogens would invest more in immune function as a result of the exposure (Moret and Siva-Jothy, 2003; Sadd and Schmid-Hempel, 2006), and thus demonstrate greater immune responses as adults. We also predicted that (3) pathogen-exposed juveniles would demonstrate greater boldness in a novel environment relative to those not exposed to pathogens. Finally, we predicted that (4) exposed juveniles would demonstrate a positive correlation between immune function and boldness as adults.
Materials and Methods
Study Animals
This study was conducted from November, 2011 through March, 2012 at the University of Oulu, Finland. We used field cricket (Gryllus integer) individuals from a laboratory population (approximately 8–9th generation) that was founded by individuals from a wild population (Davis, California, USA). The populations periodically received additional wild-caught crickets in order to avoid potential inbreeding and increase genetic diversity. Crickets were maintained at the Experimental Unit of the University of Oulu. At the start of the experiment, we sorted 315 nymphs (~1 week old) from the laboratory population into individual plastic containers (length 128 × width 98 × height 73 mm). They were held at a 12:12 h light:dark cycle at 27°C ± 1°C, and provided ad libitum food (reindeer pellets, Rehuraisio OY, poron herkku) and water. Individuals were provided a unique identification number at the outset. The identification numbers were given in sequential order as the crickets were sorted into their individual containers, and the numbers were written on the individual container the cricket was associated with. Nymphal body mass ranged from 0.0004 to 0.0010 g (n = 10). Due to the small size, we did not weigh each nymph beyond the first 10 in order to avoid possible damage from handling.
Treatment Groups
A total of three treatment groups were created: (1) juvenile bacterial injection, (2) juvenile control injection, (3) no injection control. Crickets from treatments 1 and 2 were injected with a Hamilton microsyringe between the 3rd and 4th segment of the abdomen. Injections took place on three separate days (November 28th, 30th, and December 4th, 2012) when individuals were on average at the 3rd instar (range 2nd–4th instar). Group one received a 5 μl injection of a 10−4 dilution of a 24-h culture of the opportunistic bacterial pathogen Serratia marcescens. Pilot data revealed that injections at stronger concentrations (10−3) were lethal (see also Kortet et al., 2012). Group two received a 5 μl injection of sterile nutrient broth. Group three received no injection. A new bacteria culture was created for each day in which crickets were injected. Bacterial growth was evident each day given the change in color in the growth medium. Individual body mass in all treatment groups was taken at this same time point. All crickets were checked for maturation three times a week on Monday, Wednesday, and Friday. Adult body weight and sex was recorded 5 days after maturation. Of the approximately 315 nymph that were initially sorted (105 per treatment), a total of 224 individuals reached maturity (juvenile bacterial injection n = 73, juvenile control injection n = 73, control n = 78). Sixty four individuals died after the treatment application, but before reaching maturity (juvenile bacterial injection n = 24, juvenile control injection n = 22, control n = 18). The remaining individuals either did not mature, or matured after the 5 month period in which this project was conducted.
Boldness Trials
Individual boldness was quantified using a novel-environment test, which is an established method for assessing boldness in field crickets (Hedrick, 2000; Kortet and Hedrick, 2007; Hedrick and Kortet, 2012; Dirienzo et al., 2013), and has previously been shown to be repeatable (Kendal's W = 0.337) (Niemelä et al., 2012). Seven days after adult maturation, individual crickets were placed inside a semi-opaque vial within an unfamiliar arena (19 × 19 × 11 cm). The vial was placed in a vertical position to prevent the cricket from coming out. After a 2 min acclimation period the vial was gently placed in the horizontal position allowing the cricket to exit. We recorded the latency for the cricket to become active after being placed in the horizontal position and the latency for the cricket to fully emerge from the vial. Low values of these measures indicate high levels of boldness (i.e., willingness to expose oneself to risk in a novel environment). All trials were conducted in dark conditions with only dim red light. Cricket vision is poor in red light (Briscoe and Chittka, 2001); thus this minimized external and observer influences while mimicking nocturnal conditions. The boldness trial was repeated on the following day using the same procedures. The vial was cleaned with 70% isopropyl alcohol and the sand in the arena was changed after each trial. All boldness trials were conducted between 9:30 and 15:00. Trials were limited to 20 min for logistical reasons.
Encapsulation Response
Encapsulation response was measured by inserting a small nylon monofilament implant into the abdomen. This method elicits a non-specific immune response that results in the encapsulation of the foreign body (e.g., fungi, nematodes, parasitoids) (Rantala and Kortet, 2003; Koskimäki et al., 2004), and is widely used to estimate the strength of insect immune response (Rantala and Kortet, 2003; Simmons et al., 2005; Kortet et al., 2007, 2012). 24 h after the final boldness trial, we placed a 2 mm-long implant between the 2nd and 3rd segments of the abdomen. The implant was made from 0.16 mm fishing line (Stroft GTM, Reinfeld, Germany), which was knotted at one end and roughened with P400 sand paper to increase encapsulation area. Crickets were immobilized with CO2 before implantation. The cricket immune system was allowed to encapsulate the implant for 24 h before removal. All implants were cleaned with 70% ethanol before inserting into the cricket. For the removal of the implants, crickets were immobilized again with CO2. After the removal, the implants were frozen at −20°C for later analysis. Analysis consisted of photographing an individual implant from three angles using a light microscope and attached camera. Using the program Image-J (http://rsbweb.nih.gov/ij/), we measured the gray value of the reflected light from the area of the implant that showed melanization. As the strength of encapsulation we used the average of the three gray values subtracted from the gray value of a clear control implant. Thus, larger values indicate a stronger encapsulation/immune response as less light is reflected from the melanized implant (Rantala and Kortet, 2003).
Phenoloxidase Activity
After the implants were removed, we recovered 5μl of hemolymph by removing one of the hind legs and collecting the hemolymph with a micropipette. After removal of the leg the crickets were quickly sacrificed by decapitation while they were still under the influence of CO2. The hemolymph was then mixed with 40 μl of a phosphate buffered saline (PBS) solution (pH 7.4, Sigma-Aldrich), and frozen at −20°C until analyzed in May 2013 at the University of Eastern Finland. Total phenoloxidase activity was determined photometrically as the linear rate of increase (compared to the PBS control) in optical density during 30 min at 490 nm, after addition of 180 μl L-Dopa (Sigma-Aldrich, China) in concentration of 2.366 g l−1 in 1/15 M KH2PO4 (9.073 g l−1): 1/15 M Na2HPO4 × 2 H2O (11.87 g l−1) 83:17 buffer (pH 6.2). Samples (20 μl) were analyzed in duplicate. Equal reaction time among samples was ensured by the automated L-Dopa injection in the used plate reader (FLUOstar Omega, BMG Labtech, Germany).
Statistical Analysis
The statistical software package R version 3.1.1 (R Core Team, 2015) was used for all the analyses. The presence of personality (among-individual repeatability) in each of the treatment groups was measured by calculating the repeatability between the first and second measure of the log-transformed latency to become active and binomial measure of if the individual emerged from the vial or not. Repeatability scores of each treatment group were calculated separately using the rptR package, which allows for the calculation of repeatability values (intraclass correlation coefficient) for both Gaussian and binomial data (Nakagawa and Schielzeth, 2010). This package calculates repeatability values for binomial data as the ratio between among-individual variance and among-individual variance plus the residual variance, i.e., total phenotypic variance (fixed to (pi∧2)/3 for binomial data). Given that the residual variance in our model is fixed, any changes in repeatability are attributed to differences in among-individual variance (Nakagawa and Schielzeth, 2010).
We assessed the relationship between life history characteristics and covariates using general linear models. The three life history characteristics assessed were adult body weight, the number of days to reach maturity, and growth rate. Growth rate was measured simply as the adult body mass divided by the number of days until maturation given the nymphal mass (0.0004–0.001 g) at the start of the treatment was negligible relative to the range adult mass (0.405–0.858 g). Factors included treatment group and sex. Four models were fitted for each response variable: treatment group as a main effect, sex as a main effect, both treatment and sex as a main effect, and an interaction between treatment and sex. All models, as well as a null model, were fitted using the BBMLE package (Bolker and R Development Core Team, 2014). After fitting they were compared using Akaike information criteria (AIC) (Burnham and Anderson, 2002). If the ΔAICc between two models is greater than two, the model with the lower AIC is considered to fit statistically better (Richards, 2005). Akaike weights, ωi, were also calculated. These weights estimate the probability of a model being the best fit for the data relative to the other models in the set.
In order to assess if boldness was affected by bacterial treatment as well as by the covariates, we used a combination of generalized linear mixed models. Latency to become active measurements were log transformed to achieve normality (Shapiro-Wilk W = 0.8026, p < 0.001). We created a series of generalized linear mixed models with normal error distributions. Individual identification number was included as a random effect, and sex, treatment group, development rate, adult weight, and juvenile weight as fixed effects. All models included individual ID as a random effect, and then either one of the factors/covariates alone as a fixed effect. Model with biologically relevant interactions between fixed effects were also created (e.g., juvenile weight * sex), as well as models containing the same pair of fixed effects without the interaction (e.g., juvenile weight + sex). Potentially collinear covariates (e.g., juvenile weight, adult weight, development rate) were never included in the same model. See Table 1 for a full list of model structures. The cricket latency to emerge values were highly truncated against the 20 min limit of our trial, as a large number of the crickets did not emerge from the vial (percent emerged: juvenile bacterial injection trial one = 60%, trial 2 = 49%, juvenile control injection trial one = 60%, trial 2 = 55%, control trial one = 60%, trial 2 = 56%). Thus, we converted the measure to a binomial measure and used a generalized linear mixed model with binomial error distribution and a logit link function to assess the effect of treatment and other factors on the probability of the cricket exiting the vial (Hammond-Tooke et al., 2012). The model covariate structures were the same as previously used. All models, as well as a null model, were fitted using the lme4 package (Bates et al., 2014). Model R2 values were calculated following the methods described in Nakagawa and Schielzeth (2013). The methods allows for the calculation of the marginal R2 value of mixed models, which is the proportion of variance described solely by the fixed effects within the model, as well as the conditional R2, which is the proportion of variance described by both the fixed and random effects within model (Nakagawa and Schielzeth, 2013).
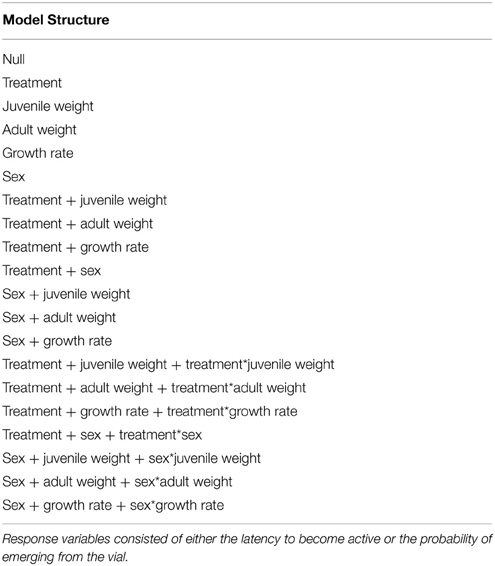
Table 1. List of model structures used to analyze relationship between boldness, treatment, and life history characteristics.
To understand if immune response is related to personality type we used separate general linear models, because we did not have the measures of immunity for all the individuals (hemolymph samples obtained: juvenile bacterial injection n = 50, juvenile control injection n = 53, control n = 50; implants recovered: juvenile bacterial injection n = 40, juvenile control injection n = 48, control n = 44). The models consisted of phenoloxidase activity or encapsulation response as response variable. Explanatory factors included sex, treatment group, development rate, adult weight, and juvenile weight as fixed effects. Models were created with these covariates as fixed effects only or with interactions between them. Additionally, we created models that included the average of the untransformed boldness scores across the two treatments (average latency to become active and latency to emerge) as variables. We also created models that included the absolute value of the difference in untransformed boldness scores between the two trials as covariates. The averages and absolute value of the boldness scores will allow us to assess how mean level of personality and change in boldness across the two trials are related to immune function, respectively. All models, as well as a null model, were fitted using the lme4 package.
Results
Repeatability of Boldness
Latency to become active within the vial was repeatable in all three treatment groups (Table 2). Treatment had a large effect on the repeatability of emergence from the vial (Table 2). 45% of juveniles who received a bacterial injection changed their behavior across both trials (e.g., emerge on the first trial, stayed in the second, or vice-versa), compared to 40% of control injections and 29% of non-injected controls. Both control groups were repeatable in terms of their tendency to exit vs. remain in the vial, while juveniles who received a bacterial injection were not repeatable in this behavior. Thus, the among-individual variation decreased as a result of juvenile bacterial injection.

Table 2. Repeatability values for the (log) latency to become active within the vial and the likelihood of fully emerging from the vial.
Life History
Bacterial infection did not affect life history characteristics. The best fit models for growth rate, days until maturation, and adult body weight included only the main effect of sex (Δ AIC to next model >3 in all comparisons). Thus, only the top models are presented for the three life history characteristics measured (Tables 3). The general trend indicates that males had larger adult body mass than females (female = 0.579 g, SE = 0.007, male = 0.624 g, SE = 0.008), faster growth rate (female = 0.010 g day−1, SE = 0.000, male = 0.012 g/day, SE = 0.000), and took less time to reach maturity (female = 59.257 days, SE = 1.320, male = 55.35 days, SE = 1.075). It is important to note the small R2 values suggest that these best fit models still only account for a small proportion of the variance.
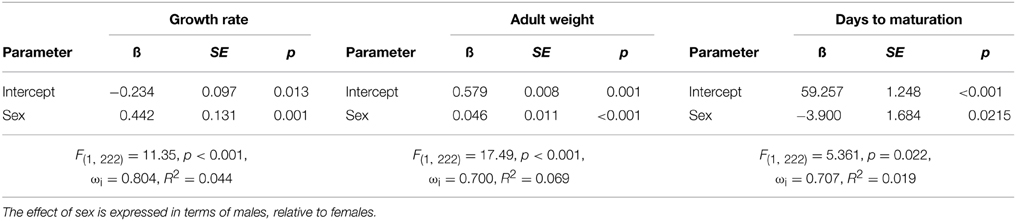
Table 3. Top general linear models predicting growth rate, weight at maturation (adult weight), and the number of days until maturation.
Treatment, Sex- and Size-Dependency of Boldness
The latency to become active within the vial was best explained by two models. The top model contained sex and the weight at the time of injection (Tables 4, 6), and carried a large proportion of the weight (ωi = 0.541). Parameter estimates indicate that males (1.685 min, SE = 0.222) had shorter latencies to become active than females (2.501 min, SE = 0.338). The next best fit model had a delta Δ AIC of 1.2 from the top model can carried a moderate proportion of the weight (ωi = 0.296), indicating it was also a good fit for the data. The model contained an interaction between sex and juvenile weight, but only the parameter estimate for sex was reliably below zero (Table 6), again indicating that male crickets had shorter latencies to become active relative to females. The remaining models all had a Δ AIC greater than two and carried a decreasing proportion of the weight, and thus are not presented further.
The probability to emerge from the vial was best explained by two models. The top model contained only a main effect of weight at the time of injection (Tables 5, 6), and carried a large proportion of the weight (ωi = 0.524). The negative main effect of weight at the time of injection suggests that crickets who were large at the time of injection were less likely to leave the vial during the 20 min trial. To illustrate this effect of body size, one can take the exponent of the parameter estimate multiplied by a juvenile weight to provide the odds an individual will emerge from the vial. In this case, a juvenile who weighed 0.3 g at the time of injection will have approximately 22% chance that they will emerge, while a juvenile who weighed 0.1 g at the time of injection will have approximately a 60% chance of emerging. The next best fit model carried a moderate proportion of the weight (ωi = 0.220), and contained main effects of sex and juvenile weight. Only the parameter estimate for juvenile weight was reliable below zero, and had nearly the same magnitude as the parameter estimate in the top model. The remaining models all had a Δ AIC greater than two from the top model and carried a decreasing proportion of the weight, and thus are not presented further.
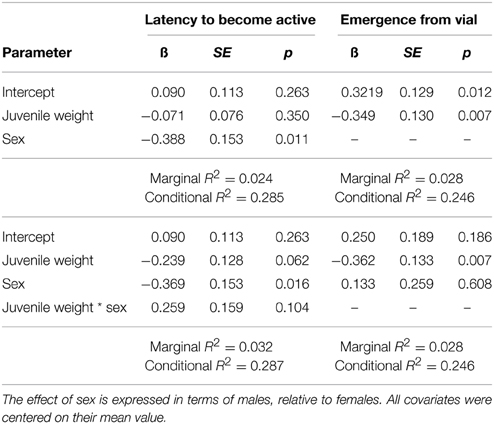
Table 6. Top generalized linear mixed models predicting the (log) latency to become active within the vial and the likelihood of fully emerging from the vial.
Immune Function-Personality Interactions
Model comparison indicated that adult phenoloxidase activity was not influenced by treatment (linear rate of increase in optical density relative to PBS control: control = 0.122, SE = 0.053, juvenile control injection = 0.116, SE = 0.044, juvenile bacterial injection = 0.145, SE = 0.079), but was influenced by the average latency to become active of the individual (Table 7). The top model carried 41% of the weight, and had ΔAIC greater than two from the null model (ΔAIC = 3.1). The parameter estimate indicates a negative relationship between the average level of boldness and phenoloxidase activity such that individuals who become active quickly had higher phenoloxidase activity relative to those who take longer to become active. Encapsulation response did not vary by treatment (difference in gray value from control implant: control = 70.826, SE = 3.874, juvenile control injection = 73.670, SE = 3.461, juvenile bacterial injection = 70.826, SE = 3.021), and instead was best explained by a single model containing an interaction between weight at injection and sex. This model carried 67% of the weight and was greater than 2 AIC points from the nearest model. The model contained a significant sex effect indicating that females had lower encapsulation rates relative to males (Table 7).
Discussion
Our results indicate, against our predictions, that early exposure to bacterial pathogens does not directly influence life history characteristics or the mean-level expression of boldness. Instead, exposure to pathogens affected the expression of behavioral variance at the individual level: the repeatability of boldness behavior, in terms of willingness to emerge from the vial as adults, was affected by exposure to pathogen in juvenile stage. Control treatments demonstrated repeatability of boldness as adults, while those who received bacterial injection as juveniles demonstrated a lack of repeatability in their tendency to emerge from the vial. This was counter to our prediction that bacterial infection would increase among-individual level variation in behavior. Together, these results suggest that early life experience may not influence the mean expression of behaviors, as we hypothesized, but instead might decrease the repeatability of behaviors. Finally, and counter to our predictions, treatment had no influence on immune function itself or the boldness-immune function relationship. However, we observed a positive relationship between boldness and phenoloxidase activity in hemolymph that was independent of infection treatment. Yet, we found no such relationship between encapsulation response and boldness.
Experience during development has been shown to influence a range of personality traits in a variety of taxa (DiRienzo et al., 2012; Bengston et al., 2014; Härkönen et al., 2014). Yet, in this study, we found that pathogen exposure during early juvenile stages did not directly influence the level of boldness in the population, but instead impacted the repeatability in one aspect of boldness behavior (emergence from the vial), but not the other aspect of boldness behavior (latency to become active). This result is similar to a recent study in the Eurasian minnows, Phoxinus phoxinus, that were experimentally infected with the brain-encysted trematode parasite, Diplostomum phoxi (Kekäläinen et al., 2014). Experimentally infected minnows were not repeatable in terms of their exploration behavior, in contrast to the significantly repeatable control groups. Yet, infection had the opposite effect on other behaviors, as infected individuals were repeatable in their activity levels, while control individuals were not repeatable in their activity levels (Kekäläinen et al., 2014). Granted, the brain infecting parasite used in the Kekäläinen et al. (2014) study is dramatically different from the bacterial pathogen used in this study. Yet, the similar trend of a lack of repeatability in response to pathogens is interesting, but the adaptive value, if any, of why individuals will or will not develop consistent variation in behavior in some conditions is unknown. One possible explanation might be that the early pathogen exposure predicts some level of future environmental variability where greater behavioral plasticity is favored. As seen in this study, the decrease in among-individual variance when exposed to pathogens suggest that extreme personality types might be less favored in a pathogen rich environment. Alternatively, increased within-individual variation in behaviors might be a behaviorally mediated risk-spreading strategy against potential future pathogen rich environment instead of increased immunity. Interestingly, the repeatability of the latency to become active was not affected by bacterial treatment. This measure might become uncoupled from the probability of emergence as activity within a safe environment is unlikely to increase encounter rates with pathogens outside the refuge. However, this is highly speculative and a large amount of additional research is needed to understand the fitness consequences associated with highly variable behavior in pathogen rich environments.
Regardless of the adaptive nature, if there is one, this result provides a new insight regarding the sensitivity of personality with respect to environmental conditions during ontogeny. Here, a single event several weeks before adulthood resulted in a difference in the behavioral repeatability, but did not affect the population mean level boldness behavior. This study, as well as other studies investigating the role of experience in personality development, highlight the importance of accounting for experience during ontogeny when investigating personality. For example, if individuals confront repeatable variation in their environmental conditions, which is inevitable in natural conditions, it may generate among-individual variation in behaviors. This in turn would drive environmentally induced personality (Stamps and Groothuis, 2010a). Furthermore, seasonal and yearly variation in environmental conditions will subsequently affect not only the mean personality type in population, but also the level of behavioral consistency. Thus, the impacts of personality on ecological and evolutionary processes will be affected by any associated developmental effects on personality (Wolf and Weissing, 2012).
Contrary to predictions, we did not discover a relationship between personality and immune function within treatments. Yet, we did discover a negative relationship between the latency to become active and phenoloxidase activity independent of treatment. Overall, individuals who became active more quickly also had higher phenoloxidase activity. This result is in line with previous findings indicating that lytic activity, another aspect of invertebrate immune function, demonstrates a negative relationship with freezing time (Kortet et al., 2007). Still this same study did not show a relationship between lytic activity and the latency to become active (as measured in this study). Also, it is important to note that the R2 of the best fit model (R2 = 0.033) was small, suggesting that boldness itself explains a rather small proportion of the variation in phenoloxidase activity. Current theories suggest that the immune function and personality may become correlated through positive feedback loops where early investment in immune function facilities increases in costly personality traits such as boldness, aggression, and activity (Kortet et al., 2010). While intuitive, empirical studies demonstrating such positive feedback loops between immune function and personality are rare (but see Butler et al., 2012). Alternative theories posit that individuals must make tradeoffs between costly traits, and are backed up by several studies demonstrating a negative relationship between personality traits and immune function (Wilson et al., 1993; Rigby and Jokela, 2000; Barber and Dingemanse, 2010).
The differences in the repeatabilities between the treatment groups suggest that our immune challenge did affect aspects of personality development, likely by increasing adult within-individual variance, but not immune function. The lack of effect of bacterial treatment on immune function could be for several reasons. In this experiment, all individuals were given ad libitum food and water. The lack of resource limitation may have prevented individuals from having to make tradeoffs between personality and immune traits (Van Noordwijk and De Jong, 1986; Stearns, 1989). Additionally, the bacterial treatment group received only a single injection. It is possible that it requires more frequent pathogen exposure, or a greater magnitude of exposure, to trigger changes in immune investment compared to changes in behavioral variation. Finally, the pathogen exposure may have affected the mean trait value of other aspects of personality that are more energetically costly, such as aggressive behavior or activity levels which were not measured in this study. Nonetheless, our results provide evidence that immune function and personality are related, yet, the relationship between the two is not affected by the developmental experiences used in this study.
In conclusion, we demonstrate that a single early exposure to a bacterial pathogen is sufficient to alter the expression of boldness personality as adults. Our results underline the sensitivity of early developmental stages for ecological conditions in the context of personality development. Moreover, treatment did not trigger alternative mean level immune investment or an immune-personality tradeoff, potentially due to housing conditions during the experiment (e.g., ad lib. food), or simply because immune function does not covary with this particular aspect of personality. Thus, we suggest additional research is needed to better understand the immune-personality relationship. Future studies will employ more ecologically relevant conditions (e.g., food limitation) and behaviors (e.g., activity) in combination with varied frequencies of pathogen exposure. Nonetheless, our results provide new insight into the important role of early ecological experience in the ontogeny of personality, and highlight both the practical and theoretical necessity of understanding this complex relationship.
Conflict of Interest Statement
The authors declare that the research was conducted in the absence of any commercial or financial relationships that could be construed as a potential conflict of interest.
Acknowledgments
We thank the University of Oulu and UEF for hosting ND while conducting this research. We also thank the National Science Foundation Nordic Research Opportunity, the Emil Aaltonen foundation, Academy of Finland (project 127398) and Tekes: The Finnish Funding Agency for Innovation for their support in this project. ND was supported by a NSF Graduate Research Fellowship. RK and PN was supported by the Academy of Finland. We also thank Jouni Laakso and Lauri Mikonranta for providing the bacterial culture of Serratia marcescens used in this experiment.
References
Barber, I., and Dingemanse, N. J. (2010). Parasitism and the evolutionary ecology of animal personality. Philos. Trans. R. Soc. B. Biol. Sci. 365, 4077–4088. doi: 10.1098/rstb.2010.0182
PubMed Abstract | Full Text | CrossRef Full Text | Google Scholar
Bates, D., Maechler, M., Bolker, B., and Walker, S. (2014). lme4: Linear Mixed-Effects Models Using Eigen and S4. R package version 1.1-7.
Bell, A. M., Hankison, S. J., and Laskowski, K. L. (2009). The repeatability of behaviour: a meta-analysis. Anim. Behav. 77, 771–783. doi: 10.1016/j.anbehav.2008.12.022
PubMed Abstract | Full Text | CrossRef Full Text | Google Scholar
Bell, A. M., and Stamps, J. A. (2004). Development of behavioural differences between individuals and populations of sticklebacks, Gasterosteus aculeatus. Anim. Behav. 68, 1339–1348. doi: 10.1016/j.anbehav.2004.05.007
Bengston, S. E., Pruitt, J. N., and Riechert, S. E. (2014). Differences in environmental enrichment generate contrasting behavioural syndromes in a basal spider lineage. Anim. Behav. 93, 105–110. doi: 10.1016/j.anbehav.2014.04.022
Bolker, B. R Development Core Team. (2014). bbmle: Tools for general maximum likelihood estimation. R package version 1.0.17. Available online at: http://CRAN.R-project.org/package=bbmle
Briscoe, A. D., and Chittka, L. (2001). The evolutoin of color vision in insects. Annu. Rev. Entomol. 46, 471–510. doi: 10.1146/annurev.ento.46.1.471
PubMed Abstract | Full Text | CrossRef Full Text | Google Scholar
Burnham, K., and Anderson, D. (2002). Model Selection and Multimodel Inference: A Practical Information-Theoretic Approach. New York, NY: Springer.
Butler, M. W., Toomey, M. B., McGraw, K. J., and Rowe, M. (2012). Ontogenetic immune challenges shape adult personality in mallard ducks. Proc. Biol. Sci. 279, 326–333. doi: 10.1098/rspb.2011.0842
PubMed Abstract | Full Text | CrossRef Full Text | Google Scholar
Caspi, A., Roberts, B. W., and Shiner, R. L. (2005). Personality development: stability and change. Annu. Rev. Psychol. 56, 453–484. doi: 10.1146/annurev.psych.55.090902.141913
PubMed Abstract | Full Text | CrossRef Full Text | Google Scholar
Cote, J., Fogarty, S., Brodin, T., Weinersmith, K., and Sih, A. (2011). Personality-dependent dispersal in the invasive mosquitofish: group composition matters. Proc. Biol. Sci. 278, 1670–1678. doi: 10.1098/rspb.2010.1892
PubMed Abstract | Full Text | CrossRef Full Text | Google Scholar
Dingemanse, N. J., Both, C., Drent, P. J., and Tinbergen, J. M. (2004). Fitness consequences of avian personalities in a fluctuating environment. Proc. Biol. Sci. 271, 847–852. doi: 10.1098/rspb.2004.2680
PubMed Abstract | Full Text | CrossRef Full Text | Google Scholar
Dingemanse, N. J., and Réale, D. (2005). Natural selection and animal personality. Behaviour 142, 1159–1184. doi: 10.1163/156853905774539445
DiRienzo, N., Pruitt, J. N., and Hedrick, A. V. (2012). Juvenile exposure to acoustic sexual signals from conspecifics alters growth trajectory and an adult personality trait. Anim. Behav. 84, 861–868. doi: 10.1016/j.anbehav.2012.07.007
Dirienzo, N., Pruitt, J. N., and Hedrick, A. V. (2013). The combined behavioural tendencies of predator and prey mediate the outcome of their interaction. Anim. Behav. 86, 317–322. doi: 10.1016/j.anbehav.2013.05.020
Dochtermann, N. A., and Jenkins, S. H. (2007). Behavioural syndromes in Merriam's kangaroo rats (Dipodomys merriami): a test of competing hypotheses. Proc. Biol. Sci. 274, 2343–2349. doi: 10.1098/rspb.2007.0622
PubMed Abstract | Full Text | CrossRef Full Text | Google Scholar
Fogarty, S., Cote, J., and Sih, A. (2011). Social personality polymorphism and the spread of invasive species: a model. Am. Nat. 177, 273–287. doi: 10.1086/658174
PubMed Abstract | Full Text | CrossRef Full Text | Google Scholar
Gillespie And, J. P., Kanost, M. R., and Trenczek, T. (1997). Biological mediators of insect immunity. Annu. Rev. Entomol. 42, 611–643. doi: 10.1146/annurev.ento.42.1.611
PubMed Abstract | Full Text | CrossRef Full Text | Google Scholar
González-Santoyo, I., and Córdoba-Aguilar, A. (2012). Phenoloxidase: a key component of the insect immune system. Entomol. Exp. Appl. 142, 1–16. doi: 10.1111/j.1570-7458.2011.01187.x
Groothuis, T. G., Carere, C., Lipar, J., Drent, P. J., and Schwabl, H. (2008). Selection on personality in a songbird affects maternal hormone levels tuned to its effect on timing of reproduction. Biol. Lett. 4, 465–467. doi: 10.1098/rsbl.2008.0258
PubMed Abstract | Full Text | CrossRef Full Text | Google Scholar
Groothuis, T. G., and Trillmich, F. (2011). Unfolding personalities: the importance of studying ontogeny. Dev. Psychobiol. 53, 641–655. doi: 10.1002/dev.20574
PubMed Abstract | Full Text | CrossRef Full Text | Google Scholar
Hammond-Tooke, C. A., Nakagawa, S., and Poulin, R. (2012). Parasitism and behavioural syndromes in the fish Gobiomorphus cotidianus. Behaviour 149, 601–622. doi: 10.1163/156853912X648903
Härkönen, L., Hyvärinen, P., Paappanen, J., Vainikka, A., and Tierney, K. (2014). Explorative behavior increases vulnerability to angling in hatchery-reared brown trout (Salmo trutta). Can. J. Fish. Aquat. Sci. 71, 1900–1909. doi: 10.1139/cjfas-2014-0221
Hedrick, A., and Kortet, R. (2012). Sex differences in the repeatability of boldness over metamorphosis. Behav. Ecol. Sociobiol. 66, 407–412. doi: 10.1007/s00265-011-1286-z
Hedrick, A. V. (2000). Crickets with extravagant mating songs compensate for predation risk with extra caution. Proc. Biol. Sci. 267, 671–675. doi: 10.1098/rspb.2000.1054
PubMed Abstract | Full Text | CrossRef Full Text | Google Scholar
Higley, J., Hasert, M., Suomi, S., and Linnoila, M. (1991). Nonhuman primate model of alcohol abuse: effects of early experience, personality, and stress on alcohol consumption. Proc. Natl. Acad. Sci. U.S.A. 88, 7261–7265. doi: 10.1073/pnas.88.16.7261
PubMed Abstract | Full Text | CrossRef Full Text | Google Scholar
Hoverman, J. T., and Relyea, R. A. (2007). How flexible is phenotypic plasticity? Developmental windows for trait induction and reversal. Ecology 88, 693–705. doi: 10.1890/05-1697
PubMed Abstract | Full Text | CrossRef Full Text | Google Scholar
Kekäläinen, J., Lai, Y.-T., Vainikka, A., Sirkka, I., and Kortet, R. (2014). Do brain parasites alter host personality?—Experimental study in minnows. Behav. Ecol. Sociobiol. 68, 197–204. doi: 10.1007/s00265-013-1634-2
Kortet, R., and Hedrick, A. (2007). A behavioural syndrome in the field cricket Gryllus integer: intrasexual aggression is correlated with activity in a novel environment. Biol. J. Linn. Soc. 91, 475–482. doi: 10.1111/j.1095-8312.2007.00812.x
Kortet, R., Hedrick, A. V., and Vainikka, A. (2010). Parasitism, predation and the evolution of animal personalities. Ecol. Lett. 13, 1449–1458. doi: 10.1111/j.1461-0248.2010.01536.x
PubMed Abstract | Full Text | CrossRef Full Text | Google Scholar
Kortet, R., Niemelä, P. T., Vainikka, A., and Laakso, J. (2012). Females prefer bold males; an analysis of boldness, mate choice, and bacterial resistance in the field cricket Gryllus integer. Ecol. Parasitol. Imunol. 1, 1–6. doi: 10.4303/epi/235580
Kortet, R., Rantala, M. J., and Hedrick, A. (2007). Boldness in anti-predator behaviour and immune defence in field crickets. Evol. Ecol. Res 9, 185–197. Available online at: http://citeseerx.ist.psu.edu/viewdoc/summary?doi=10.1.1.391.6714
Koskimäki, J., Rantala, M. J., Taskinen, J., Tynkkynen, K., and Suhonen, J. (2004). Immunocompetence and resource holding potential in the damselfly, Calopteryx virgo L. Behav. Ecol. 15, 169–173. doi: 10.1093/beheco/arg088
Luttbeg, B., and Sih, A. (2010). Risk, resources and state-dependent adaptive behavioural syndromes. Philos. Trans. R. Soc. B. 365, 3977–3990. doi: 10.1098/rstb.2010.0207
PubMed Abstract | Full Text | CrossRef Full Text | Google Scholar
Moret, Y., and Siva-Jothy, M. T. (2003). Adaptive innate immunity? Responsive-mode prophylaxis in the mealworm beetle, Tenebrio molitor. Proc. Biol. Sci. 270, 2475–2480. doi: 10.1098/rspb.2003.2511
PubMed Abstract | Full Text | CrossRef Full Text | Google Scholar
Nakagawa, S., and Schielzeth, H. (2010). Repeatability for Gaussian and non-Gaussian data: a practical guide for biologists. Biol. Rev. 85, 935–956. doi: 10.1111/j.1469-185X.2010.00141.x
PubMed Abstract | Full Text | CrossRef Full Text | Google Scholar
Nakagawa, S., and Schielzeth, H. (2013). A general and simple method for obtaining R2 from generalized linear mixed-effects models. Methods Ecol. Evol. 4, 133–142. doi: 10.1111/j.2041-210x.2012.00261.x
Niemelä, P. T., Vainikka, A., Hedrick, A. V., and Kortet, R. (2012). Integrating behaviour with life history: boldness of the field cricket, Gryllus integer, during ontogeny. Funct. Ecol. 26, 450–456. doi: 10.1111/j.1365-2435.2011.01939.x
Paskewitz, S., and Riehle, M. A. (1994). Response of Plasmodium refractory and susceptible strains of Anopheles gambiae to inoculated Sephadex beads. Dev. Comp. Immunol. 18, 369–375. doi: 10.1016/0145-305X(94)90002-7
PubMed Abstract | Full Text | CrossRef Full Text | Google Scholar
Pruitt, J., Dirienzo, N., Kralj-Fišer, S., Johnson, J., and Sih, A. (2011). Individual- and condition-dependent effects on habitat choice and choosiness. Behav. Ecol. Sociobiol. 65, 1987–1995. doi: 10.1007/s00265-011-1208-0
Pruitt, J. N., Riechert, S. E., and Jones, T. C. (2008). Behavioural syndromes and their fitness consequences in a socially polymorphic spider, Anelosimus studiosus. Anim. Behav. 76, 871–879. doi: 10.1016/j.anbehav.2008.05.009
Rantala, M. J., and Kortet, R. (2003). Courtship song and immune function in the field cricket Gryllus bimaculatus. Biol. J. Linn. Soc. 79, 503–510. doi: 10.1046/j.1095-8312.2003.00202.x
R Core Team. (2015). R: A Language and Environment for Statistical Computing. Vienna: R Foundation for Statistical Computing.
Richards, S. A. (2005). Testing ecological theory using the information- theoretic approach: examples and cautionary results. Ecology 86, 2805–2814. doi: 10.1890/05-0074
Rico, J. L. R., Ferraz, D. B., Ramalho-Pinto, F. J., and Morato, S. (2010). Neonatal exposure to LPS leads to heightened exploratory activity in adolescent rats. Behav. Brain. Res. 215, 102–109. doi: 10.1016/j.bbr.2010.07.001
PubMed Abstract | Full Text | CrossRef Full Text | Google Scholar
Rigby, M. C., and Jokela, J. (2000). Predator avoidance and immune defence: costs and trade-offs in snails. Proc. Biol. Sci. 267, 171–176. doi: 10.1098/rspb.2000.0983
PubMed Abstract | Full Text | CrossRef Full Text | Google Scholar
Sadd, B. M., and Schmid-Hempel, P. (2006). Insect immunity shows specificity in protection upon secondary pathogen exposure. Curr. Biol. 16, 1206–1210. doi: 10.1016/j.cub.2006.04.047
PubMed Abstract | Full Text | CrossRef Full Text | Google Scholar
Sih, A., Bell, A., and Johnson, J. C. (2004). Behavioral syndromes: an ecological and evolutionary overview. Trends Ecol. Evol. 19, 372–378. doi: 10.1016/j.tree.2004.04.009
PubMed Abstract | Full Text | CrossRef Full Text | Google Scholar
Sih, A., Cote, J., Evans, M., Fogarty, S., and Pruitt, J. (2012). Ecological implications of behavioural syndromes. Ecol. Lett. 15, 278–289. doi: 10.1111/j.1461-0248.2011.01731.x
PubMed Abstract | Full Text | CrossRef Full Text | Google Scholar
Simmons, L. W., Zuk, M., and Rotenberry, J. T. (2005). Immune function reflected in calling song characteristics in a natural population of the cricket Teleogryllus commodus. Anim. Behav. 69, 1235–1241. doi: 10.1016/j.anbehav.2004.09.011
Smith, B. R., and Blumstein, D. T. (2008). Fitness consequences of personality: a meta-analysis. Behav. Ecol. 19, 448–455. doi: 10.1093/beheco/arm144
PubMed Abstract | Full Text | CrossRef Full Text | Google Scholar
Stamps, J., and Groothuis, T. G. (2010a). The development of animal personality: relevance, concepts and perspectives. Biol. Rev. 85, 301–325. doi: 10.1111/j.1469-185X.2009.00103.x
PubMed Abstract | Full Text | CrossRef Full Text | Google Scholar
Stamps, J. A., and Groothuis, T. G. (2010b). Developmental perspectives on personality: implications for ecological and evolutionary studies of individual differences. Philos. Trans. R. Soc. Lond. B Biol. Sci. 365, 4029–4041. doi: 10.1098/rstb.2010.0218
PubMed Abstract | Full Text | CrossRef Full Text | Google Scholar
Stearns, S. C. (1989). Trade-offs in life-history evolution. Funct. Ecol. 3, 259–268. doi: 10.2307/2389364
Van Noordwijk, A. J., and De Jong, G. (1986). Acquisition and allocation of resources: their influence on variation in life history tactics. Am. Nat. 128, 137–142. doi: 10.1086/284547
Wilson, D. S., Coleman, K., Clark, A. B., and Biederman, L. (1993). Shy-bold continuum in pumpkinseed sunfish (Lepomis gibbosus): an ecological study of a psychological trait. J. Comp. Psychol. 107, 250. doi: 10.1037/0735-7036.107.3.250
Wolf, M., and Weissing, F. J. (2012). Animal personalities: consequences for ecology and evolution. Trends Ecol. Evol. 27, 452–461. doi: 10.1016/j.tree.2012.05.001
PubMed Abstract | Full Text | CrossRef Full Text | Google Scholar
Keywords: animal personality, boldness, Gryllus, field cricket, immune function
Citation: DiRienzo N, Niemelä PT, Skog A, Vainikka A and Kortet R (2015) Juvenile pathogen exposure affects the presence of personality in adult field crickets. Front. Ecol. Evol. 3:36. doi: 10.3389/fevo.2015.00036
Received: 18 December 2014; Accepted: 20 March 2015;
Published: 08 April 2015.
Edited by:
Peter Schausberger, University of Natural Resources and Life Sciences, AustriaReviewed by:
Michelle L. Hall, University of Melbourne, AustraliaSarah E. Bengston, University of Arizona, USA
Copyright © 2015 DiRienzo, Niemelä, Skog, Vainikka and Kortet. This is an open-access article distributed under the terms of the Creative Commons Attribution License (CC BY). The use, distribution or reproduction in other forums is permitted, provided the original author(s) or licensor are credited and that the original publication in this journal is cited, in accordance with accepted academic practice. No use, distribution or reproduction is permitted which does not comply with these terms.
*Correspondence: Nicholas DiRienzo, Department of Neurobiology, Physiology and Behavior, University of California, Davis, One Shields Ave., Davis, CA 95618, USAbmRpcmllbnpvQGdtYWlsLmNvbQ==