- 1Department of Biodiversity Research, Global Change Research Centre, Czech Academy of Sciences, Brno, Czech Republic
- 2Faculty of Science, Institute for Environmental Studies, Charles University, Prague, Czech Republic
- 3Department of Agriculture, Yamagata University, Tsuruoka, Japan
- 4Biological and Environmental Sciences, The University of Tennessee at Chattanooga, Chattanooga, TN, USA
- 5School of Biological Sciences, University of East Anglia, Norwich, UK
Some experiments indicate that ladybirds can significantly suppress aphid abundance. For example, exclusion of predators by caging aphid-infested plants repeatedly results in higher aphid populations and faster aphid population growth rates. However, aphidophagous ladybirds have never proved effective in controlling aphid populations in the field, which is consistent with the theoretical prediction that long-lived predators cannot be effective in controlling a short-lived prey (the generation time ratio hypothesis, GTR). To resolve this paradox, field experiments, involving two species of ladybirds, Coccinella septempunctata bruckii and Harmonia axyridis were used to determine their efficiency in suppressing populations of the aphid, Aphis gossypii, on small shrubs of Hibiscus syriacus under natural conditions. Instead of by caging, the effect of each ladybird species on aphid population dynamics was determined by removing all the eggs of C. septempunctata from 8 shrubs, those of H. axyridis from a further 8 shrubs, all those of both species from an additional 12 shrubs and leaving the eggs on 6 control shrubs. These predators did not have a negative effect on the peak numbers of the aphids. Thus, one should be cautious when interpreting the results of cage experiments, used to assess the efficiency of predators in reducing the abundance of their prey.
Introduction
The outstanding success of the ladybird beetle, Rodolia cardinalis Mulsant in controlling the cottony-cushion scale, Icerya purchasi Maskell, an important pest of citrus in California late in the nineteenth century, resulted in the widespread use of ladybirds as biocontrol agents (Dixon and Kindlmann, 1998). Predaceous coccinellids are more often used as a symbol of biological control than any other taxon (Hodek, 1973; Klausnitzer and Klausnitzer, 1979; New, 1991; Majerus, 1994; Hodek and Honek, 1996; Jervis and Kidd, 1996; Obrycki and Kring, 1998). However, despite the initial optimism (Hagen and van den Bosch, 1968; Hodek, 1970, 1973), ladybirds have never proved effective in controlling aphids (Hagen, 1974; Gordon, 1985; Obrycki and Kring, 1998; Iperti, 1999; Dixon, 2000). It is therefore surprising that many quantitative assessments indicate that coccinellids can substantially suppress aphid density below the predator-free value (e.g., Chambers et al., 1983; Michels et al., 2001; Symondson et al., 2002; Basky, 2003; Cardinale et al., 2003; Snyder et al., 2008, 2004; Klausnitzer and Klausnitzer, 1979; Thies et al., 2011). This apparent contradiction needs to be resolved.
The explanation is evident in the case of assessments that rely on a correlation between prey and predator densities (e.g., Hattingh and Samways, 1994; Elliott and Kieckhefer, 2000; Basky, 2003; Freier et al., 2007), as such correlations are not causal: high abundance of predators at locations where prey is abundant does not necessarily mean that these predators regulate prey abundance (Hughes, 1973). In some studies correlations between the predator-to-prey ratio and predator density are analyzed (Zhao et al., 2013a,b), but not the direct effect of natural enemies on prey. However, correlations do not prove a causal effect.
Less easy to explain are the results obtained using exclusion techniques, such as cages, which are frequently used for evaluating the effect of natural enemies (Luck et al., 1988). The growth rates and peak densities of aphid populations within cages that exclude natural enemies are usually larger than those in uncaged populations (e.g., Chambers et al., 1983; Elliott and Kieckhefer, 2000; Michels et al., 2001; Basky, 2003; Cardinale et al., 2003; Schmidt et al., 2003; Thies et al., 2011) or cages with ladybirds or other predators (Messina and Sorenson, 2001; Snyder et al., 2004, 2008). However, cages change the microenvironment (Hand and Keaster, 1967), especially temperature, which is thought to be important in determining the outcome of predator-prey interactions (Frazer and Gilbert, 1976; Frazer et al., 1981). Even more importantly, cages prevent aphids from emigrating, which is their usual response to high density (Dixon, 1998, 2005). Some exclusion cages, like those used by Schmidt et al. (2003) and Thies et al. (2011) do not even exclude predators and therefore the results obtained using such cages are seriously flawed (Ameixa and Kindlmann, 2011). Interestingly, when only polythene enclosures, 60 cm high, buried to a depth of 30 cm, and not cages, were used (Holland et al., 1996), which do not affect the microenvironment of the manipulated plots, allow aphids to emigrate, but exclude ground predators, there was no difference in the number of grain aphids in control plots and those where the number of ground predators were reduced. Thus, the results from exclusion or inclusion experiments, using cages, must be interpreted with caution (Luck et al., 1988) and those obtained by Schmidt et al. (2003) and Thies et al. (2011) cannot be considered as proof of predator efficiency because the cages they used are not impermeable to predators.
Hand removal as a means of assessing the effectiveness of natural enemies is rarely used because it is labor intensive (Luck et al., 1988). However, this method has much less of a secondary effect on the prey population than caging. In this study the eggs of two species of ladybirds, Coccinella septempunctata bruckii Mulsant (Coleoptera: Coccinellidae) and Harmonia axyridis Pallas (Coleoptera: Coccinellidae) were removed in order to determine their effectiveness in suppressing populations of the cotton aphid, Aphis gossypii Glover (Homoptera: Aphididae), on small shrubs of Hibiscus syriacus L. under natural conditions. These predators did not have a negative effect on the peak numbers of their prey.
Theory—the GTR Hypothesis
From an evolutionary perspective, both predator and prey strive to maximize their reproductive potentials. However, whilst the existence of prey is not dependent on predators the latter are dependent on prey. Therefore, it is advantageous for predators to conserve their prey. Thus, for predators the optimal strategy involves counteracting pressures to maximize their own reproduction and survival with conserving enough prey to sustain a sufficient food supply for its offspring. This is very nicely exemplified by long-lived insect predators feeding on short-lived prey (Dixon and Kindlmann, 1998; Kindlmann and Dixon, 1999, 2001). As most of these predators suffer enormous egg and larval mortality due to cannibalism and intraguild predation, selection acts mainly on optimizing their oviposition strategies in terms of maximizing the likelihood that their offspring will survive until reproductive age. The oviposition strategy of a predator with a long larval developmental time will depend on a longer projection of the future prey abundance in a patch, bearing in mind there are likely to be more bottlenecks or a higher probability of a bottleneck than for predators with short developmental times, and consequently they must be more conservative in terms of conserving their prey (the GTR hypothesis, Dixon and Kindlmann, 1998; Kindlmann and Dixon, 1999, 2001).
Aphidophagous predators are a good example. Their prey live in colonies, which are characterized by an initial rapid increase followed by an equally rapid decline in abundance resulting in extinction of the colony. The decline is not caused by predators or parasites, even if they contribute to it. Instead, aphids cause the decline by strongly reacting to their own density and switching to producing migrants, which look for another more suitable host. Thus, when the aphid density is high, most of the newborn leave the colony immediately after reaching adulthood. The dynamics of different colonies is not synchronized in time, as they feed on different host plants with different phenologies. At a large spatial scale, at any instant, populations of aphids exist as patches of prey, associated with patches of good host plant quality. That is, aphid predators exploit patches of prey that vary greatly in quality both spatially and temporally and have evolved a strategy for optimally exploiting aphids (Dixon, 2000, 2005).
Adults of aphid predators are winged, can easily move between patches and therefore are able to find patches containing an abundance of prey. Thus, in terms of the predator's fitness the availability of energy is not a limiting factor. Its immature stages are confined to one patch and if this contains few aphids they starve and eat each other. Mortality of immature stages due to starvation, cannibalism or intraguild predation is enormous: 98–99% and is mainly a consequence of low numbers of prey at any time during larval development. Thus, egg and larval cannibalism is adaptive in these predators, as eating conspecific competitors will increase the likelihood of survival of their larvae (Dixon, 2000, 2005).
Because of the immense egg and larval mortality, selection acts mainly on optimum oviposition strategies, which are those that ensure the maximum likelihood that their offspring will survive rather than maximization of the amount of food eaten by the predator per unit time. The optimum oviposition strategy of the adult is therefore determined mainly by expectations of future bottlenecks in prey abundance, as these will affect survival of its offspring, more than the number of prey present in a patch, because adults are not limited by the amount of food as they can find another colony (Kindlmann and Dixon, 1999, 2001).
A good long-term forecast of the quality of a prey colony becomes especially important for ovipositing predators if the ratio of generation time of the predator to that of the prey (generation time ratio, GTR) is large. This is because the oviposition strategy of a predator with a long larval developmental time will depend on a longer projection of the future prey abundance in a patch, which is dependent on more bottlenecks or a higher probability of a bottleneck than that of a predator with a short developmental time, and consequently must be more conservative in terms of conserving their prey. As a result, such predators tend to be less effective in controlling their prey.
Aphid predators are a good example of this hypothesis, as their developmental time often spans several aphid generations, during which the aphid numbers vary dramatically. Laying eggs in the presence of conspecific larvae is strongly selected against in these predators, because it results in the eggs being eaten by older conspecific larvae. In addition, laying eggs late in the development of an ephemeral patch of prey is maladaptive, as there is insufficient time for all the larvae to complete their development. Thus, eggs laid by predators late in the existence of a patch of prey are at a disadvantage, as they are highly likely to be eaten by larvae of predators that hatch from the first eggs to be laid.
Empirical data indicate that several different species of aphid predators have evolved mechanisms that enable them to oviposit preferentially in patches of prey that are in an early stage of development and avoid those that are already being attacked by larvae. Females of these species strongly react to the smell of larval tracks of their own species or of other aphid predators by immediately ceasing oviposition and leaving the aphid colony. This response strongly reduces the number of eggs laid per patch and consequently their effectiveness in regulating the abundance of aphids.
Methods
The study site was located on the Yamagata University farm (Tsuruoka, Yamagata pref., 38° 43′N, 139° 49′E) and consisted of about 100 shrubs of H. syriacus L., which is the primary host of the cotton aphid, A. gossypii Glover. At this site, this aphid is mainly attacked by two species of ladybird: C. septempunctata bruckii Mulsant and H. axyridis Pallas (Yasuda and Shinya, 1997).
We selected 34 similar-sized shrubs for the manipulative experiment. After identification to species but before hatching, we removed all the eggs and adults of C. septempunctata bruckii over a period of 2 years from 8 shrubs, those of H. axyridis from another 8 shrubs, those of both species from an additional 12 shrubs, and no eggs from the remaining 6 control shrubs. Sticky bands were placed at the bottom of each shrub in order to prevent larvae from other shrubs colonizing the experimental shrubs.
It is difficult to identify coccinellids at the egg stage because they are often similar in size, color and number in a batch. Therefore, we removed a few eggs from each egg mass and placed them in Petri dishes kept at 25°C and a 14L:10D photoperiod in the laboratory, and identified to species when the larvae hatched. Eggs reared in the laboratory hatched earlier than those left on the shrubs, which enabled the removal of ladybird eggs from the experimental shrubs before hatching.
The shrubs were sampled 14 times during 2000 (May 24–June 25) and 43 times during 2001 (May 2–June 13). The numbers of aphids per 15 randomly selected leaves and of all the species of predator found on the shrubs were counted.
We used Two-Way ANOVA for testing the effect of year and treatment on the peak numbers of aphids.
Results
On average, 12.9 egg batches of H. axyridis and 13.9 of C. septempunctata bruckii were laid on each shrub in 2000 and 2001. Almost no parasitized aphids were observed. The resulting peak numbers of aphids are shown in Figure 1 and their averages are presented in Table 1. The results indicate that the presence of predators had no significant effect on the peak numbers of aphids (Two-Way ANOVA gives F = 95.8, P = 0.002 for the effect of year and F = 4.95, P = 0.11 for the effect of predator removal), despite the large numbers of ladybird larvae that hatched on shrubs, from which predators were not removed (Table 1).
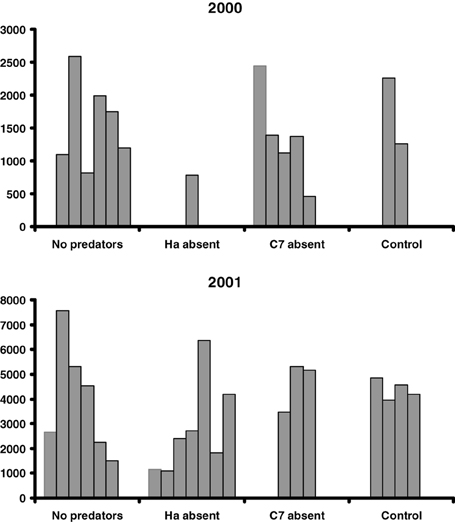
Figure 1. Peak numbers of aphids per 15 leaves on shrubs from which eggs of all predators were removed (No predators), only C. septempunctata bruckii eggs and adults were removed (C7 absent), only H. axyridis eggs and adults were removed (Ha absent) and no eggs or adults were removed (Control).

Table 1. Average peak numbers (± SD) of aphids per 15 leaves and mean numbers (± SD) of larvae of H. axyridis (Ha) and C. septempunctata bruckii (C7) per shrub in the different treatments in 2000 and 2001.
In addition to C. septempunctata bruckii and H. axyridis, 3 other species of ladybird: Propylea japonica, Scymnus posticalis and S. hoffmanni, which are smaller than C. septempunctata bruckii and H. axyridis, and unidentified species of syrphids and spiders, were found on the shrubs. The numbers of these predators were low in the 2 years, except for S. posticalis in 2000.
Discussion
In this study, the most abundant species of ladybirds, C. septempunctata bruckii and H. axyridis, did not have a negative effect on the peak numbers of aphids. Interestingly, the mean peak numbers of aphids on the control shrubs, where both species of predator were present, were consistently greater, although not significantly so, than those on the shrubs from which one or both species of predator were removed (Table 1).
H. axyridis is a top predator in this system (Dixon, 2000; Sato et al., 2008). Thus, intraguild predation could have weakened the negative influence of C. septempunctata bruckii on aphid abundance, and resulted in larger aphid populations. That this may be the case is shown in Figure 1 and Table 1: removal of H. axyridis apparently resulted in a reduced (smaller peaks) aphid abundance. However, even in this case, the negative influence of C. septempunctata bruckii on aphid peak abundance was weak and not statistically significant.
It is also unlikely that the other species of predators present affected the outcome, as they were all too rare and too small to have any effect on aphid dynamics. Similarly, parasites were also very rare in this system. All this supports the conclusion that the efficiency of natural enemies, when measured using cage experiments, is likely to be overestimated, as the cages prevent the prey from emigrating, which is a common response to high densities in many herbivores, including aphids.
The low effectiveness of ladybirds was not because they were rare in this system. There were on average 225 ladybird larvae in 2000 and 234 ladybird larvae in 2001 on each shrub (Table 1), each able to consume between 10 and 100 aphids per day (Hukusima and Kamei, 1970), which is sufficient to potentially consume all the aphids on a shrub within a few days.
The low efficiency of insect predators, especially aphidophagous ladybirds that feed on highly aggregated and ephemeral patches of prey such as aphid colonies, is supported by recent theoretical predictions. Kindlmann and Dixon (1999, 2001) proposed that the ratio of the generation time of insect predators to that of their prey (generation time ratio, GTR) is a good predictor of the effectiveness of a natural enemy in suppressing its prey. According to their hypothesis, when GTR is large, the natural enemy is unable to significantly reduce the abundance of its prey. This hypothesis is supported by the results of an empirical study of Mills (2006). In the system studied here the developmental times of the aphids and ladybirds are about 7 and 30–35 days, respectively. That is, in this system the GTR is about 4–5, which indicates that these predators should have little effect on aphid abundance (Kindlmann and Dixon, 1999, 2001).
The mechanism underlying GTR has been well studied both in the laboratory and the field. A major factor in this is density dependent cannibalism, which serves to regulate predator abundance (Dixon, 2000; Dixon and Kindlmann, 2012). The results reported here support the prediction of the GTR hypothesis. However, the generality of the prediction needs to be tested by hand removal of natural enemies from other predator-prey systems. If these experiments confirm that the low efficiency of predators in suppressing prey populations in predator-prey systems with a GTR greater than one is the norm and not restricted to ladybird-aphid systems, then the general view, largely based on the results of cage experiments, that insect predators regulate the abundance of their prey, will have to be reconsidered. Although tedious, hand removal of predators from a system in which the prey are free to leave is a more reliable means of assessing the effectiveness of predators in reducing the abundance of their prey.
Few shrubs were used in this experiment. This is understandable, bearing in mind the labor involved in counting all the aphids, eggs, larvae etc. on the shrubs. Therefore, the statistical tests are not very strong. However, our aim was to show that in the absence of predators the peak numbers of aphids were not larger, as would be the case if the GTR hypothesis was invalid. It is commonly assumed that predators must have a significant effect on the abundance of their prey. The GTR hypothesis claims the contrary with predators having a negligible effect on the abundance of their prey in systems with a large GTR. Therefore, the results presented in this paper are in accordance with the predictions of the GTR hypothesis. Those believing in a strong effect of predators on their prey in systems with a large GTR need to present a stronger case based on more reliable results than those based on the exclusion cage experiments of Schmidt et al. (2003) and Thies et al. (2011). In addition, they need to explain why the GTR hypothesis is wrong.
Conflict of Interest Statement
The authors declare that the research was conducted in the absence of any commercial or financial relationships that could be construed as a potential conflict of interest.
Acknowledgments
AFGD acknowledges support for this research from grant number LO1415 of the MSMT within the National Sustainability Program I (NPU I), PK from grant No. 14-36098G of the GA CR.
References
Ameixa, O., and Kindlmann, P. (2011). Some exclusion cages do not exclude predators. Eur. J. Environ. Sci. 1, 67–69.
Basky, Z. (2003). “Predators and parasitoids on different cereal aphid species under caged and no caged conditions in Hungary,” in Proceedings of the 8th International Symposium on Ecology of Aphidophaga: Biology, Ecology and Behaviour of Aphidophagous Insects, eds A. O. Soares, M. A. Ventura, V. Garcia, and J. L. Hemptinne (Ponta Delgada Azores: Arquipélago - Life and Marine Science, Supplement 5), 95–102.
Cardinale, B. J., Harvey, C. T., Gross, K., and Ives, A. R. (2003). Biodiversity and biocontrol: emergent impacts of a multi-enemy assemblage on pest suppression and crop yield in an agroecosystems. Ecol. Lett. 6, 857–865. doi: 10.1046/j.1461-0248.2003.00508.x
Chambers, R. J., Sunderland, K. D., Wyatt, I. J., and Vickerman, G. P. (1983). The effects of predator exclusion and caging on cereal aphids in winter wheat. J. Appl. Ecol. 20, 209–224. doi: 10.2307/2403387
Dixon, A. F. G. (2000). Insect Predator-Prey Dynamics: Ladybird Beetles and Biological Control. Cambridge: Cambridge University Press.
Dixon, A. F. G. (2005). Insect Herbivore-Host Dynamics: Shrub-Dwelling Aphids. Cambridge: Cambridge University Press.
Dixon, A. F. G., and Kindlmann, P. (1998). “Generation time ratio and the effectiveness of ladybirds as classical biological control agents,” in Pest Management—Future Challenges: Proceedings 6th Australasian Applied Entomology and Research Conference, Vol. 1, eds M. P. Zalucki, R. A. I. Drew, and G. C. White (Brisbane, QLD: The University of Queensland Press), 314–320.
Dixon, A. F. G., and Kindlmann, P. (2012). Cannibalism, optimal egg size and vulnerable developmental stages in insect predators. Eur. J. Environ. Sci. 2, 84–88.
Elliott, N. C., and Kieckhefer, R. W. (2000). Response by coccinellids to spatial variation in cereal aphid density. Pop. Ecol. 42, 81–90. doi: 10.1007/s101440050012
Frazer, B. D., and Gilbert, N. (1976). Coccinellids and aphids: a quantitative study of the impact of adult ladybirds (Coleoptera: Coccinellidae) preying on field populations of pea aphids (Homoptera: Aphididae). J. Ent. Soc. B. C. 73, 33–56.
Frazer, B. D., Gilbert, N., Ives, P. M., and Raworth, D. A. (1981). Predator reproduction and the overall predator-prey relationship. Can. Entomol. 113, 1015–1024. doi: 10.4039/Ent1131015-11
Freier, B., Triltsch, H., Mowes, M., and Moll, E. (2007). The potential of predators in natural control of aphids in wheat: results of a ten-year field study in two German landscapes. BioControl 52, 775–788. doi: 10.1007/s10526-007-9081-5
Gordon, R. D. (1985). The Coccinellidae (Coleoptera) of America north of Mexico. J. N.Y. Entomol. Soc. 93, 1–912.
Hagen, K. S. (1974). The significance of predaceous Coccinellidae in biological and integrated control of insects. Mem. Hors. Ser. 7, 25–44.
Hagen, K. S., and van den Bosch, R. (1968). Impact of pathogens, parasites, and predators on aphids. Ann. Rev. Entomol. 13, 325–384. doi: 10.1146/annurev.en.13.010168.001545
PubMed Abstract | Full Text | CrossRef Full Text | Google Scholar
Hand, L. F., and Keaster, A. J. (1967). The environment of an insect field cage. J. Econ. Entomol. 60, 910–915. doi: 10.1093/jee/60.4.910
Hattingh, V., and Samways, M. J. (1994). Physiological and behavioral characteristics of Chilocorus spp. (Coleoptera: Coccinellidae) in the laboratory relative to effectiveness in the field as biocontrol agents. J. Econ. Entomol. 87, 31–38. doi: 10.1093/jee/87.1.31
Hodek, I. (1970). Coccinellids and the modern pest management. BioScience 20, 543–552. doi: 10.2307/1295013
Holland, J. M., Thomas, S. R., and Hewitt, A. (1996). Some effects of polyphagous predators on an outbreak of cereal aphid (Sitobion avenae F.) and orange wheat blossom midge (Sitodoplosis mosellana Gehin). Agr. Ecosyst. Environ. 59, 181–190. doi: 10.1016/0167-8809(96)01053-5
Hughes, R. D. (1973). Quantitative evaluation of natural enemy effectiveness. J. Appl. Ecol. 10, 321–351. doi: 10.2307/2404731
PubMed Abstract | Full Text | CrossRef Full Text | Google Scholar
Hukusima, S., and Kamei, M. (1970). Effects of various species of aphids as food on development, fecundity and longevity of Harmonia axyridis Pallas (Coleoptera: Coccinellidae). Res. Bull. Fac. Agr. Gifu Univ. 29, 53–66.
Iperti, G. (1999). Biodiversity of predaceous Coccinellidae in relation to bioindication and economic importance. Agr. Ecosyst. Env. 74, 323–342. doi: 10.1016/S0167-8809(99)00041-9
Jervis, M., and Kidd, N. (1996). Insect Natural Enemies: Practical Approaches to Their Study and Evaluation. NewYork, NY: Chapman and Hall.
Kindlmann, P., and Dixon, A. F. G. (1999). Generation time ratios—determinants of prey abundance in insect predator—prey interactions. Biol. Control 16, 133–138. doi: 10.1006/bcon.1999.0754
Kindlmann, P., and Dixon, A. F. G. (2001). When and why top-down regulation fails in arthropod predator-prey systems. Basic Appl. Ecol. 2, 333–340. doi: 10.1078/1439-1791-00071
Luck, R. F., Shepard, B. M., and Kenmore, P. E. (1988). Experimental methods for evaluating arthropod natural enemies. Ann. Rev. Entomol. 33, 367–391. doi: 10.1146/annurev.en.33.010188.002055
Messina, F. J., and Sorenson, S. M. (2001). Effectiveness of lacewing larvae in reducing Russian wheat aphid populations on susceptible and resistant wheat. Biol. Control 21, 19–26 doi: 10.1006/bcon.2000.0914
Michels, G. J., Elliott, N. C., Romero, R. A., Owings, D. A., and Bible, J. B. (2001). Impact of indigenous coccinellids on Russian wheat aphids and greenbugs (Homoptera: Aphididae) infesting winter wheat in the Texas Panhandle. Southwestern Entomol. 26, 97–114.
Mills, N. J. (2006). Accounting for differential success in the biological control of homopteran and lepidopteran pests. N. Z. J. Ecol. 30, 61–72.
Obrycki, J. J., and Kring, T. J. (1998). Predaceous Coccinellidae in biological control. Ann. Rev. Entomol. 43, 295–321.
Sato, S., Jimbo, R., Yasuda, H., and Dixon, A. F. G. (2008). Cost of being an intraguild predator in predatory ladybirds. Appl. Entomol. Zool. 43, 143–147. doi: 10.1303/aez.2008.143
Schmidt, M. H., Lauer, A., Purtauf, T., Thies, C., Schaefer, M., and Tscharntke, T. (2003). Relative importance of predators and parasitoids for cereal aphid control. Proc. R. Soc. Lond. B 270, 1905–1909. doi: 10.1098/rspb.2003.2469
PubMed Abstract | Full Text | CrossRef Full Text | Google Scholar
Snyder, G. B., Finke, D. L., and Snyder, W. E. (2008). Predator biodiversity strengthens aphid suppression across single- and multiple-species prey communities. Biol. Control 44, 52–60. doi: 10.1016/j.biocontrol.2007.09.006
Snyder, W. E., Ballard, S. N., Yang, S., Clevenger, G. M., Miller, T. D., Ahn, J. J., et al. (2004). Complementary biocontrol of aphids by the ladybird beetle Harmonia axyridis and the parasitoid Aphelinus asychis on greenhouse roses. Biol. Control 30, 229–235. doi: 10.1016/j.biocontrol.2004.01.012
Symondson, W. O. C., Sunderland, K. D., and Greenstone, M. H. (2002). Can generalist predators be effective biocontrol agents? Ann. Rev. Entomol. 47, 561–594. doi: 10.1146/annurev.ento.47.091201.145240
PubMed Abstract | Full Text | CrossRef Full Text | Google Scholar
Thies, C., Haenke, S., Scherber, C., Bengtsson, J., Bommarco, R., Clement, L. W., et al. (2011). The relationship between agricultural intensification and biological control: experimental tests across Europe. Ecol. Appl. 21, 2187–2196. doi: 10.1890/10-0929.1
PubMed Abstract | Full Text | CrossRef Full Text | Google Scholar
Yasuda, H., and Shinya, K. (1997). Cannibalism and interspecific predation in two predatory ladybirds in relation to prey abundance in the field. Entomophaga 42, 53–163.
Zhao, Z.-H., Hui, C., He, D.-H., and Ge, F. (2013b). Effects of position within wheat field and adjacent habitats on the density and diversity of cereal aphids and their natural enemies. BioControl 58, 765–776. doi: 10.1007/s10526-013-9536-9
Keywords: biological control, generation time ratio, population dynamics, predator-prey systems, ladybirds, aphids
Citation: Kindlmann P, Yasuda H, Kajita Y, Sato S and Dixon AFG (2015) Predator efficiency reconsidered for a ladybird-aphid system. Front. Ecol. Evol. 3:27. doi: 10.3389/fevo.2015.00027
Received: 10 January 2015; Accepted: 02 March 2015;
Published: 18 March 2015.
Edited by:
Mauricio Lima, Pontificia Universidad Catolica de Chile, ChileReviewed by:
Cang Hui, Stellenbosch University, South AfricaSergio Andrés Estay, Universidad Austral de Chile, Chile
Copyright © 2015 Kindlmann, Yasuda, Kajita, Sato and Dixon. This is an open-access article distributed under the terms of the Creative Commons Attribution License (CC BY). The use, distribution or reproduction in other forums is permitted, provided the original author(s) or licensor are credited and that the original publication in this journal is cited, in accordance with accepted academic practice. No use, distribution or reproduction is permitted which does not comply with these terms.
*Correspondence: Pavel Kindlmann, Global Change Research Centre, Czech Academy of Sciences, Bělidla 4a, 602 00 Brno, Czech Republic pavel.kindlmann@centrum.cz