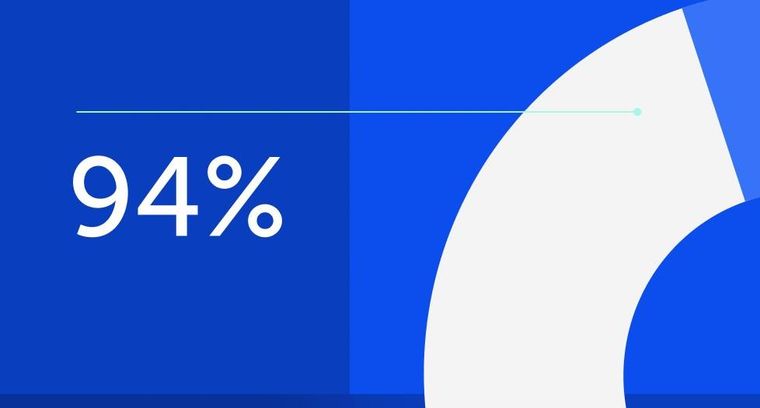
94% of researchers rate our articles as excellent or good
Learn more about the work of our research integrity team to safeguard the quality of each article we publish.
Find out more
ORIGINAL RESEARCH article
Front. Earth Sci., 08 April 2025
Sec. Solid Earth Geophysics
Volume 13 - 2025 | https://doi.org/10.3389/feart.2025.1558854
This article is part of the Research TopicAdvanced Materials and Technologies for Sustainable Development of Underground ResourcesView all 44 articles
Soil-rock mixtures are extensively used in geotechnical engineering applications, such as embankment construction, dam engineering, and slope reinforcement, where their compressive deformation characteristics play a crucial role in influencing the stability and settlement behavior of these structures. This study investigates how variations in rock content (W), effective stress (σv) and fine-grained soil properties (quartz sand and silty red clay) affect the one-dimensional compression behavior of soil-rock mixtures. Key compression parameters, including the compression index Cc and the secondary compression index Ca, were obtained and analyzed through one-dimensional consolidation tests to assess the deformation characteristics of these mixtures. Results show that under the same effective stress (σv), both the Cc and Ca exhibit different trends with W, depending on the properties of the fine-grained soil. Soil-rock mixtures with silty red clay demonstrate more pronounced secondary consolidation effects at low rock content, whereas mixtures with quartz sand display weaker secondary consolidation overall. The significantly lower Ca/Cc values in the quartz sand mixtures suggest that secondary settlement is much smaller in these mixtures compared to those containing silty red clay.
Soil-rock mixtures can be broadly classified into natural and artificial types based on their formation processes. Natural soil-rock mixtures are highly heterogeneous systems formed since the Quaternary period through geological processes such as gravitational, fluvial and glacial deposition, as well as residual weathering and tectonic activity. These mixtures typically comprise multiple materials with relatively continuous gradation, resulting in complex compositions and a diverse range of particle sizes. In contrast, artificial soil-rock mixtures usually consist of fewer materials types, exhibiting a specific range of particle sizes that create a discontinuous hierarchical structure. Despite their simpler composition, artificial mixtures play a crucial role in modern geotechnical engineering, where they are widely used as fill materials for embankments, dams, tunnels, and slope reinforcement (Wei et al., 2024; Liu et al., 2021). The compression behavior of soil-rock mixtures significantly influences the stability and long-term performance of these structures. While established theories effectively address the compression behavior of homogeneous soils, such as clays and sands (Mesri and Vardhanabhuti, 2009; Suneel et al., 2018; Feng et al., 2017; Xu et al., 2023) the mechanical behavior of soil-rock mixtures is more complex due to differences in particle size, material composition, and mechanical properties between the soil and rock components. The internal heterogeneity of these mixtures, along with the distinct behaviors of soil and rock, poses challenges in accurately predicting their mechanical properties. A comprehensive study of the compression deformation behavior of soil-rock mixtures is essential for advancing engineering design and ensuring the safety of such structures (Simpson and Evans, 2016; Wei et al., 2024).
The theory of one-dimensional consolidation is a cornerstone in understanding soil compression behavior and has broad applications in analyzing soil compressibility, predicting settlement, and addressing various engineering challenges. Terzaghi (1943), in his seminal work Theoretical Soil Mechanics, established the foundational principles of one-dimensional consolidation theory, which continue to underpin modern soil mechanics. This theory employs the e (void ratio)- σv (effective stress) curve or e-lg (σv) curve to characterize soil compression behavior, distinguishing between primary and secondary consolidation phases. It also facilitates the derivation of key parameters such as the compression index (Cc) and the secondary compression index (Ca). Extensive research has focused on the compression behavior of homogeneous soils, such as clays and sands. Skempton T (1957) expanded the practical applications of this theory by investigating consolidation coefficients and pore water pressure, providing valuable methods to predict settlement in clays. Chuhan et al. (2003) studied sand compression under high-stress conditions, uncovering key mechanisms such as porosity reduction and mechanical compaction. Mesri and Vardhanbhuti. (2009) analyzed over 100 sand samples, focusing on particle rearrangement, friction, and particle strength during primary and secondary compression phases. Similarly, Suneel et al. (2018) identified a strong correlation between the initial void ratio and secondary compression index in Korean natural soft soils. Further studies on clays have enhanced our understanding of creep behavior and the structural factors influencing consolidation. Zhu et al. (2016) introduced the material constant “q” to quantify the effects of soil structure on creep, while Ye and Ye. (2016) developed an eight-parameter constitutive model to simulate the behavior of over-consolidated clays. Nie et al. (2023) through scanning electron microscope and multivariate analysis, examined the compressibility, shear strength, and microstructural evolution of compacted loess. In contrast, soil-rock mixtures have received comparatively less attention, despite their complex mechanical behavior resulting from the interaction between soil and rock components and their structural heterogeneity. Santagata et al. (2008) studied the compressibility of high-organic soils (40%–60% organic matter), showing that organic content significantly influences soil compressibility, and underscoring the differences between inorganic clays and fibrous peat. Wang and Korkiala-Tanttu (2020) focused on the effects of cement and lime stabilization on soft clays, particularly their impact on creep coefficients, compression indices, and overall compressibility. Wu et al. (2019) conducted incremental loading tests on sand-soil mixtures, identifying secondary compression index and proposing the secondary compression behavior (long-term deformation) mechanism. Moreover, Chai and Duy. (2013) explored the consolidation and undrained shear strength of clay soils reinforced with geosynthetics, providing valuable insights into how reinforcement materials can enhance both consolidation and shear strength. Xu et al. (2020) investigated the compressibility of calcareous sand-clay mixtures, demonstrating that clay content significantly affects compressibility, creep behavior, and particle breakage. In addition, some researchers have employed particle modeling to study the micromechanics of one-dimensional consolidation in soils (Qu et al., 2023a; Qu et al., 2023b). Recent studies have also explored the role of mineral composition and microstructural characteristics in determining the compressibility of cemented soil-rock mixtures (Wu et al., 2025; Wu et al., 2024; Wu et al., 2022).
Although extensive research has been conducted on the compressibility, consolidation behavior, and secondary consolidation characteristics of homogeneous soils, the one-dimensional compression behavior of soil-rock mixtures remains relatively underexplored. Most existing studies on soil-rock mixtures focus on specific rock content or particular types of mixtures, lacking a comprehensive understanding of their behavior under varying conditions. This gap is significant because the compression deformation behavior of soil-rock mixtures is influenced by multiple factors, with rock content and fine-grained soil properties being key variables. The presence of rock fragments alters the structural characteristics and stress transmission paths within the mixture, while the physical properties of fine-grained soils—such as particle shape, grain size distribution, and plasticity index—affect the overall compressibility of the soil-rock mixture (Gong and Liu, 2015; Qian et al., 2024; Wu et al., 2020). Furthermore, Liu et al. (2024) examined the influence of coarse aggregate distribution uniformity on the compaction characteristics of soil-rock mixtures under cyclic loading, demonstrating that its effect varies with different rock content levels, with the most significant impact observed at moderate rock content.
This study aims to address this gap by systematically investigating how varying rock content and fine-grained soil properties influence the compression behavior of soil-rock mixtures. To achieve this, a series of one-dimensional consolidation tests using soil-rock mixtures prepared with two representative fine-grained soils—silty red clay and quartz sand. Multi-stage loading consolidation tests were performed to measure key compression parameters, including void ratio, compression index (Cc), and secondary compression index (Ca). Through analysis of the experimental data, this study reveals the combined effects of rock content and fine-grained soil properties on the compressibility of soil-rock mixtures, providing valuable insights for engineering applications.
The soil-rock mixtures utilized in this study were artificially prepared, with coarse and fine soils collected from the Nanning region in Guangxi, China. Figure 1 illustrates the coarse and fine soils used in the tests, with the coarse-grained soil primarily composed of gravel (Figure 1A). To examine the influence of various fine-grained soil properties on the one-dimensional compression behavior of soil-rock mixtures, two types of fine-grained soils were selected: quartz sand (Figure 1B) and silty red clay (Figure 1C). It is important to clarify that coarse-grained soil and fine-grained soil in this context refer to different particle size fractions within the mixtures, rather than traditional soil classification standards in soil mechanics. X-ray diffraction tests indicated that quartz constitutes over 99% of quartz sand, while the silty red clay comprises approximately 11.5% quartz, 10% hematite, and 78.5% clay minerals, of which kaolinite accounts for 76% and montmorillonite for 24%. In addition, SEM analyses show that quartz sand particles are well-defined at low magnifications but exhibit numerous cracks and defects at higher magnifications, which likely contribute to particle breakage under stress. In contrast, the silty red clay exhibits pronounced flocculation, with fine particles forming dense clusters. Moreover, the progression of red soil formation in the Nanning region influences its mechanical properties: as redox weathering proceeds, aluminum and iron ions are redistributed, leading to an increase in the crystalline and amorphous phases within the clay. These changes enhance inter-particle cohesion and the internal strength of clay aggregates, thereby reducing expansive and shrinkage behavior and ultimately affecting the overall compressibility of the soil–rock mixture. The basic physical properties of the experimental materials were measured using standard methods, including the pycnometer method, volumetric method, oven-drying method, vibrating compaction method, and combined liquid limit-plastic limit test. Table 1 summarizes the basic material properties—specific gravity, density, natural moisture content, optimum moisture content, and liquid limit—of the soil-rock mixture components. These values were obtained using standard test methods, such as the specific gravity bottle method, volumetric method, oven-drying method, surface vibratory compaction method, and the liquid-plastic limit test, all conducted in accordance with GB/T50123-2019 and JTG3430-2020.
Figure 1. Experimental material used in this study. (A) gravel. (B) quartz sand. (C) silty red clay .
A fully automated large-scale one-dimensional consolidation apparatus was utilized to perform one-dimensional compression tests on the soil-rock mixtures. The apparatus, illustrated in Figure 2, includes a vertical stress loading system, a sample consolidation chamber, a pore water pressure control system, and a data acquisition system. This configuration enables a range of one-dimensional consolidation tests, such as incremental loading, strain-controlled loading, and continuous stress loading. The specifications of the apparatus are as follows: the consolidation chamber accommodates samples with a diameter of 252 mm and a height of 70 mm, and it is designed to withstand a maximum pressure of 2.0 MPa. The vertical stress loading system has a maximum load capacity of 200 kN and can achieve a maximum axial compression displacement of 50 mm. Load and displacement measurement accuracy is within ±0.5% of the full scale, while pore water pressure and backpressure measurement accuracy is within ±1% of the full scale.
This study primarily investigates the effects of rock content and fine-grained soil properties on the compression characteristics of soil-rock mixtures through a series of one-dimensional compression tests. To systematically analyze the influence of rock content (W), six levels were established: 0%, 20%, 40%, 60%, 80%, and 100%, where 0% represents pure fine-grained soil and 100% represents pure coarse soils. While rock content in practical engineering applications typically ranges from 30% to 70%, a broader gradient was chosen to comprehensively capture variations in mechanical behavior across the full spectrum, a methodology commonly employed in experimental studies to investigate nonlinear trends and transition thresholds (Simpson and Evans, 2016). To assess the impacts of fine-grained soil properties, two types of fine-grained soils were selected: quartz sand and silty red clay. Take the fine-grained soil of quartz sand, for example, the grading curves for soil-rock mixture specimens with different W are shown in Figure 3. The testing procedure followed the standard for soil test methods (GB/T50123-2019) and the specifications for highway subgrade soil tests (JTG3430-2020). The detailed process was as follows: First, the soil-rock mixture samples were prepared by thoroughly mixing the fine and coarse components to ensure a homogeneous distribution. To maintain consistent moisture content and prevent external fluctuations, the prepared mixtures were promptly sealed in plastic film prior to testing. The sealed samples were then placed in a consolidation chamber and saturated for 24 h using vacuum pressure with distilled water. After saturation, the samples underwent pre-consolidation at 50 kPa for 24 h to ensure uniform compaction. Following pre-consolidation, multi-stage consolidation tests were conducted under effective stress levels of 200 kPa, 400 kPa, 600 kPa, 800 kPa, and 1,600 kPa. Although most foundation and subgrade loading conditions typically fall within 1,000 kPa, the upper limit of 1,600 kPa was selected to simulate more extreme conditions, such as those encountered in high-load structures or rock-based foundations, as also reported by Wu et al. (2019) and Nie et al. (2023). Each loading increment was maintained until the settlement rate of the sample reached 0.01 mm/h before advancing to the next stress level. In summary, this experimental program involved six levels of rock content, two types of fine-grained soils, and five levels of effective stress, resulting in a total of 155 tests.
Figure 3. Grading curves of soil-rock mixture samples with varying rock content W (fine-grained soil: quartz sand).
The compression coefficient, α, for a specific pressure range can be calculated using Equation 1:
where
where
Figure 6 depict the void ratio-effective stress (e-σv) relationship curves for soil-rock mixtures with varying rock contents (W), using silty red clay and quartz sand as the fine-grained soils, respectively. These figures illustrate that, during one-dimensional consolidation, e shows a nonlinear decreasing trend as effective stress (σv) increases. At lower levels of effective stress, the decrease in e is gradual, while at higher stress levels, the reduction becomes more linear. Vaughan et al. (1988) attribute this behavior to the yielding of the soil structure, where the applied effective stress exceeds the strength of the soil particles skeleton, causing the structural bonds to fail and resulting in significant compaction. For a given fine-grained soil, the void ratio is clearly influenced by the rock content (W) at any σv. In mixtures with silty red clay, the e initially decreases with increasing W, reaching a minimum when W = 20%, as shown in Figure 6A. In contrast, for mixtures with quartz sand, the e also decreases initially with increasing W, but the minimum e occurs when W = 60%, as shown in Figure 6B. This indicates that the minimum e varies depending on the nature of the fine-grained soil. The differences in the minimum e for different fine-grained soils are likely due to the distinct interactions between the rocks and the fine-grained soil matrix. For silty red clay, its smaller particle size and higher plasticity may allow it to fill the voids between rocks more effectively at lower W levels. In contrast, quartz sand, with its coarser grains and different packing characteristics, requires a higher W to achieve optimal packing and minimal void ratio. This phenomenon aligns with the theory of particle rearrangement and void structure changes proposed by Xiao et al. (2021), which suggests that the interaction between coarse and fine particles significantly influences the compressibility of soil-rock mixtures.
Figure 6. The relationship between e and σv for the samples with different W. (A) Fine-grained soil is silty red clay. (B) Fine-grained soil is quartz sand.
Based on the e-σv relationship curves in Figure 6, the compression index Cc under incremental loading was determined. Figure 7 presents the Cc-σv curves for soil-rock mixtures samples with varying rock contents (W). For the silty red clay samples (Figure 7A), the compression index Cc decreases gradually with increasing effective stress, stabilizing when the stress exceeds 600 kPa. This indicates that at lower stress levels, the compressibility of the soil-rock mixture is relatively high, but as the effective stress increases, the compressibility diminishes and eventually stabilizes. This behavior is consistent with the findings from compression tests on cohesive soils reported by Nash and Sills (1992) and Gasparre and Coop (2008), which show that cohesive soils exhibit reduced compressibility as their structure consolidates and compaction becomes more uniform under increasing stress. In contrast, the quartz sand samples (Figure 7B) exhibit an increasing compression index Cc as effective stress rises. This can be attributed to the more pronounced particle rearrangement and abrasion in sandy soils under high-stress conditions. As stress increases, sand particles undergo significant rearrangement and breakage, leading to greater compressibility. This observation aligns with the work of Mesri and Vardhanabhuti (2009), Mesri (1977) and Xiao et al. (2021), who found that particle crushing and abrasion under high stress can substantially affect soil compressibility. In sandy soils, especially those with hard and crushable particles, fragmentation and abrasion become more pronounced under high stress, reducing particle size (Wu and Wang, 2023). As stress increases, particle breakage produces smaller fragments that fill the voids between larger particles, thereby further increasing the compression index Cc.
Figure 7. The relationship between Cc and σv for the samples with different W. (A) Fine-grained soil is silty red clay. (B) Fine-grained soil is quartz sand.
Based on the data from Figures 7, 8 illustrates the relationship between the compression index (Cc) and rock content (W) for soil-rock mixtures with silty red clay and quartz sand as the fine-grained components, respectively. The trends in Cc vary depending on the type of fine-grained soil. For mixtures with silty red clay (Figure 8A), Cc follows an increasing-then-decreasing trend as W increases. At W = 0 (pure fine-grained soil), the Cc value is relatively low. However, as W rises to between 20% and 60%, Cc increases significantly, indicating greater compressibility. This may be due to an optimal balance between the rock fragments and fine-grained soil within this range, where the rock particles provide structural support, enhancing the compressibility of the mixtures. Once W exceeds 60%, Cc begins to decrease, suggesting that when rock content becomes too high, the coarse-grained skeleton dominates the mixture, reducing overall compressibility. In contrast, mixtures with quartz sand as the fine-grained component (Figure 8B) show much less sensitivity to changes in W. The compression index Cc exhibits minimal variation as rock content increases, implying that the interaction between quartz sand and rock fragments is relatively weak. The addition of rock fragments has little effect on the mixture’s compressibility. This is likely due to the large particle size and low compressibility of quartz sand, meaning that the presence of rock fragments does not significantly alter the overall compressibility of the soil-rock mixture. The trends observed in Figure 8 are consistent with the findings of Sowers et al. (1951), who noted that the compressibility of soil-rock mixtures is influenced not only by rock content but also by the type of fine-grained soil. Factors such as water content and inter-particle friction play a significant role in determining the compressibility of the mixture. According to common classifications, soils with Cc>0.167 are considered highly compressible, those with 0.033<Cc<0.167 are moderately compressible, and soils with Cc<0.033 are classified as low-compressibility soils (Gu et al., 2019). Based on this classification, the mixtures with quartz sand are identified as low-to-moderately compressible, while the mixtures with silty red clay fall into the moderately-to-highly compressible range. Overall, the properties of the fine-grained soil play a key role in determining the compressive behavior of the soil-rock mixture (Gong et al., 2024; Nie et al., 2024). The silty red clay, with its higher plasticity and cohesiveness, interacts more dynamically with the coarse particles, leading to greater variability in compressibility. On the other hand, quartz sand, due to its rigidity and low compressibility, results in a more stable compressive behavior, largely independent of rock content.
Figure 8. The relationship between Cc and rock content W under different σ. (A) Fine-grained soil is silty red clay. (B) Fine-grained soil is quartz sand.
Figure 9 illustrates the relationship between the secondary compression index (Ca) and effective stress (σv) for soil-rock mixtures with varying rock content (W), where the fine-grained soils are silty red clay and quartz sand, respectively. In both cases, Ca decreases with increasing effective stress, stabilizing after 400 kPa. This suggests that secondary consolidation effects are more pronounced under lower stress conditions. As stress increases, particle rearrangement stabilizes, resulting in a reduction in secondary consolidation. However, when σv exceeds 800 kPa, a slight increase in Ca is observed, indicating that further particle rearrangement or breakage occurs under higher stress, enhancing the secondary consolidation effect. A comparison between Figures 9A, B reveals that Ca values for mixtures with silty red clay as the fine-grained soil are generally higher than those with quartz sand, particularly at lower stress levels. This indicates that secondary consolidation is more significant in mixtures with silty red clay, likely due to more complex interactions between the fine particles. In contrast, for mixtures with quartz sand, the Ca is less pronounced at low stress levels due to the rigidity and low plasticity of quartz sand, which restricts particle movement. However, under higher stress conditions, particularly above 800 kPa, particle breakage and rearrangement in the quartz sand mixtures become more prominent, resulting in increased secondary consolidation. Furthermore, for mixtures with silty red clay, the Ca values decrease as the rock content W increases, and the Ca-σv curve flattens, suggesting that an increase in rock content reduces secondary consolidation effects. This could be due to the dominance of coarse particles in the soil structure as rock content rises. However, for mixtures with quartz sand, no such trend is observed, indicating that the abrasion and breakage of sand particles, in combination with the rock fragments, contribute to a more complex and significant interaction. These observations are consistent with previous studies. Suneel et al. (2018) and Santagata et al. (2008) conducted compression tests on soft clays from different regions and found that Ca values decrease and stabilize after consolidation pressures exceed 200 kPa. Similarly, Mesri and Vardhaabhuti (2009), Mesri (1977) and Bjerrum (1973) noted that for sandy soils, particle abrasion and breakage under high stress significantly increase secondary consolidation effects.
Figure 9. The relationship between Ca and σv for soil-rock mixtures with different rock content. (A) Fine-grained soil is silty red clay. (B) Fine-grained soil is quartz sand.
Based on the data from Figures 9, 10 illustrates the relationship between the secondary compression index (Ca) and rock content (W) for soil-rock mixtures with silty red clay and quartz sand as fine-grained soils, respectively. For the silty red clay samples (Figure 10A), Ca shows higher values at lower W, indicating that secondary consolidation effects are more pronounced when fine-grained soil dominates the mixture. As W increases, Ca gradually decreases, suggesting that as the proportion of coarse-grained material rises, the structure of the soil-rock mixture becomes more influenced by the rock particles. This reduces the impact of the fine-grained soil on compression behavior and results in a lower secondary consolidation effect. These findings are consistent with previous research on fine-grained soil behavior in soil-rock mixtures. Mesri and Vardhaabhuti (2009), Mesri (1977) and Bjerrum (1973) noted that the secondary compression index tends to stabilize at high rock content because the load-bearing skeleton is primarily composed of coarse-grained particles, with secondary consolidation mainly occurring through the rearrangement of fine particles. For the quartz sand samples (Figure 10B), Ca is generally lower than in the silty red clay mixtures, with more subtle changes in Ca as W increases. After W exceeds 20%, there is a slight rise in Ca, suggesting that rock fragments contribute to particle rearrangement and breakage, though less significantly than in clay-based mixtures. While the compressibility of sand-dominated mixtures remains relatively stable, the gradual wear and breakage of particles at higher rock content can still lead to some increase in secondary consolidation. This observation aligns with Wu et al. (2019), who found that in sand-dominated mixtures, secondary consolidation is primarily influenced by the arrangement and contact of coarse particles, though particle abrasion at higher stress levels can enhance secondary compression effects. In conclusion, as shown in Figures 10A, B, soil-rock mixtures with silty red clay exhibit more pronounced secondary consolidation, especially at lower rock content, due to the cohesive nature of the clay and its propensity for particle rearrangement over time. In contrast, mixtures with quartz sand show weaker secondary consolidation overall, as the rigidity of sand particles limits rearrangement. In quartz sand mixtures, secondary consolidation is primarily influenced by particle wear and breakage at higher rock content, which contributes to a slight increase in Ca under elevated stress levels. Based on relative comparisons and the trends observed in previous studies (Suneel et al., 2018; Mesri and Castro, 1987; Bjerrum, 1973), silty red clay samples exhibit moderate to high secondary consolidation, while quartz sand mixtures fall into the low secondary consolidation category.
Figure 10. The relationship between Ca and rock content W under different σv. (A) Fine-grained soil is silty red clay. (B) Fine-grained soil is quartz sand.
Figure 11 illustrates the relationship between the ratio of the secondary compression index Ca to the primary compression index Cc and rock content W for soil-rock mixtures with silty red clay and quartz sand as the fine-grained components, respectively. Mesri and Vardhaabhuti (2009), Mesri (1977) observed through extensive consolidation tests that the Ca/Cc ratio remains nearly constant for a given soil type, which can be used to define long-term compression (secondary consolidation) behavior. Thus, understanding how the Ca/Cc ratio varies with W is essential for interpreting secondary consolidation effects in soil-rock mixtures. Figure 11A shows the variation of Ca/Cc with W for mixtures with silty red clay. A linear fit of the data yielded an R2 value greater than 0.8, indicating a strong correlation. The figure demonstrates that as W increases, the Ca/Cc ratio gradually decreases. This suggests that at lower rock content, secondary consolidation effects are more pronounced in silty red clay mixtures, while primary consolidation becomes more dominant as rock content increases. However, when W > 40, the ratio stabilizes between 0.037 and 0.041. This implies that at higher rock content, the increased proportion of rock fragments reduces the influence of fine-grained soil on secondary consolidation, resulting in compression behavior that is increasingly controlled by primary consolidation. Figure 11B depicts the variation of the Ca/Cc for mixtures with quartz sand as the fine-grained component. Compared to silty red clay, the Ca/Cc values for quartz sand mixtures are generally lower, ranging between 0.008 and 0.017, with only slight changes as W increases. This indicates that rock content has limited effect on the secondary consolidation behavior of quartz sand mixtures, where secondary settlement is relatively low and primary consolidation dominates the compression process. The linear fit also shows an R2 value greater than 0.8, confirming a strong correlation. By comparing Figures 11A, B, it is evident that mixtures with silty red clay exhibit more pronounced secondary consolidation effects at low rock content, while mixtures with quartz sand show weaker secondary consolidation overall. The significantly lower Ca/Cc values in the quartz sand mixtures indicate that secondary settlement in these mixtures is much smaller than in those with silty red clay. This difference can be attributed to the physical properties of the fine-grained soils: silty red clay has stronger inter-particle friction and cohesion, leading to greater deformation during secondary consolidation, whereas quartz sand exhibits a more stable particle arrangement, resulting in less deformation during the secondary consolidation phase. These findings are consistent with previous studies and further validate the significant influence of fine-grained soil properties on the compressibility of soil-rock mixtures (Suneel et al., 2018; Mesri and Castro, 1987; Wang et al., 2017).
Figure 11. The relationship between compression index Cc and secondary compression index Ca (The inset graph shows the ratio of Ca/Cc as a function of W). (A) Fine-grained soil is silty red clay. (B) Fine-grained soil is quartz sand.
This study examines the effects of rock content (W), fine-grained soil properties and effective stress (σv) on the compression deformation behavior of soil-rock mixtures through one-dimensional consolidation tests. The main conclusions are as follows:
(1) Significant differences in the compression index Cc were observed across soil-rock mixtures with varying fine-grained soils. For silty red clay, Cc decreases with increasing effective stress and stabilizes at 600 kPa. Additionally, Cc follows a “first increase then decrease” trend with W, peaking at W = 60%. In contrast, for quartz sand, Cc increases steadily with effective stress but is less sensitive to changes in W.
(2) The nature of the fine-grained soil plays a significant role in influencing the secondary compression index (Ca). Compared to quartz sand, silty red clay exhibits a higher Ca, indicating a more pronounced secondary consolidation effect. For silty red clay, Ca decreases as W increases, while for quartz sand, the effect of W on Ca is minimal, with relatively narrow variation.
(3) Soil-rock mixtures with silty red clay exhibit more pronounced secondary consolidation effects at low rock content, while mixtures with quartz sand show weaker secondary consolidation overall. The significantly lower Ca/Cc values in the quartz sand mixtures indicate that secondary settlement in these mixtures is much smaller than in those with silty red clay.
(4) From an engineering application perspective, the selection of fine-grained soil type and rock content significantly influences the compressibility and secondary consolidation characteristics of soil-rock mixtures. Silty red clay mixtures demonstrate higher compressibility and secondary consolidation, requiring more careful settlement predictions, whereas quartz sand mixtures exhibit lower compressibility and better long-term stability. Regarding rock content, for silty red clay mixtures, an optimal range of 20%–40% provides a stable balance between compressibility and secondary consolidation. In quartz sand mixtures, the minimum void ratio occurs at 60%–80% rock content, contributing to improved mechanical stability.
While these conclusions offer valuable insights into the static consolidation behavior of soil-rock mixtures, it is important to acknowledge certain limitations in our study. In particular, the effects of moisture variability and dynamic loading conditions were not addressed, which could further influence compressibility and settlement behavior. Future research should explore these aspects to provide a more comprehensive understanding of soil-rock mixture performance under field conditions.
The original contributions presented in the study are included in the article/supplementary material, further inquiries can be directed to the corresponding author.
JG: Data curation, Methodology, Supervision, Formal analysis, Resources, Writing–original draft, Writing–review and editing. JW: Conceptualization, Data curation, Writing–review and editing. SZ: Data curation, Formal analysis, Writing–review and editing. YX: Methodology, Funding acquisition, Software, Writing–review and editing. LD: Data curation, Software, Writing–review and editing. ZL: Conceptualization, Funding acquisition, Writing–review and editing. XP: Data curation, Supervision, Writing–original draft. MJ: Data curation, Formal Analysis, Methodology, Writing–review and editing.
The author(s) declare that financial support was received for the research and/or publication of this article. This research was financially supported by the National Natural Science Foundation of China (No. 52368045), the Guangxi Science and Technology Base and Talent Project (No. 2021AC19244) and the Innovation Project of Guangxi Graduate Education (YCSW2023088). The authors express their appreciation for the financial assistance.
Author JG was employed by Hualan Design and Consulting Group.
The remaining authors declare that the research was conducted in the absence of any commercial or financial relationships that could be construed as a potential conflict of interest.
The author(s) declare that no Generative AI was used in the creation of this manuscript.
All claims expressed in this article are solely those of the authors and do not necessarily represent those of their affiliated organizations, or those of the publisher, the editors and the reviewers. Any product that may be evaluated in this article, or claim that may be made by its manufacturer, is not guaranteed or endorsed by the publisher.
Bjerrum, L. (1973). Geotechnical problems involved in foundations of structures in the North Sea. Géotechnique 23, 319–358. doi:10.1680/geot.1973.23.3.319
Chai, J., and Duy, Q. N. (2013). Geocomposite induced consolidation of clayey soils under stepwise loads. Geotext. and Geomembranes 37, 99–108. doi:10.1016/j.geotexmem.2013.02.006
Chuhan, F. A., Kjeldstad, A., Knut, H., and Høeg, K. (2003). Experimental compression of loose sands: relevance to porosity reduction during burial in sedimentary basins. Can. Geotech. J. 40 (5), 995–1011. doi:10.1139/T03-050
Feng, W. Q., Lalit, B., Yin, Z. Y., and Yin, J. H. (2017). Long-term Non-linear creep and swelling behavior of Hong Kong marine deposits in oedometer condition. Comput. Geotechnics 84, 1–15. doi:10.1016/j.compgeo.2016.11.009
Gasparre, A., and Coop, M. R. (2008). Quantification of the effects of structure on the compression of a stiff clay. Can. Geotech. J. 45, 1324–1334. doi:10.1139/T08-052
Gong, J., and Liu, J. (2015). Analysis on the mechanical behaviors of soil-rock mixtures using discrete element method. Procedia Eng. 102, 1783–1792. doi:10.1016/j.proeng.2015.01.315
Gong, J., Pang, X., and Ou, J. X. (2024). Effects of particle shape, physical properties and particle size distribution on the small-strain stiffness of granular materials: a DEM study. Comput. Geotech. 165, 105901–105903. doi:10.1016/j.compgeo.2023.105903
Gu, X., Qian, H., Liu, H., and Wang, S. (2019). Foundation and groundwork. 4nd ed. Beijing, China: China Architecture and Building Press.
Liu, S., Liao, J., and C, H. W. Z. Z. (2024). Study on the influence of coarse aggregate distribution uniformity on the compaction characteristics of gap-graded gravels. Comput. Geotech. 170, 1. doi:10.1016/j.compgeo.2024.106293
Liu, S., Xu, S., Wu, P., Wan, L., and Li, J. (2021). Compaction density evaluation of Soil-Rock mixtures by the additive mass method. Constr. Build. Mater. 306, 124882. doi:10.1016/j.conbuildmat.2021.124882
Mesri, G., and Castro, A. (1987). Cα/Cc concept and K 0 during secondary compression. J. geotechnical Eng. 113 (3), 230–247. doi:10.1061/(ASCE)0733-9410(1987)113:3(230)
Mesri, G., and Godlewski, P. M. (1977). Time and stress-compressibility interrelationship. J.of Geotech.Engrg 103, 417–430. doi:10.1061/ajgeb6.0000421
Mesri, G., and Vardhanabhuti, B. (2009). Compression of granular materials. Rev. Can. De. Géotechnique 46, 369–392. doi:10.1139/T08-123
Nash, D. F. T., Sills, G., and Davison, L. R. (1992). One-dimensional consolidation testing of soft clay from Bothkennar. Géotechnique 42, 241–256. doi:10.1680/geot.1992.42.2.241
Nie, J. Y., Cui, Y., Wang, G., Wang, R., Zhang, N., Zhang, L., et al. (2024). A comprehensive numerical investigation of multi-scale particle shape effects on small-strain stiffness of sands. Géotechnique 75, 323–336. doi:10.1680/jgeot.23.00118
Nie, Y., Ni, W., Lu, X., Tuo, W., and Yuan, K. (2023). Macroscopic mechanical behavior and microstructural evolution of compacted loess in the Chinese Loess Plateau. Soil Tillage Res. 232, 105767. doi:10.1016/j.still.2023.105767
Qian, J., Zhang, C., Zhou, W., Tang, Y., Li, J., and Li, C. (2024). A thermodynamically consistent constitutive model for soil-rock mixtures: a focus on initial fine content and particle crushing. Comput. Geotech. 169, 106233. doi:10.1016/j.compgeo.2024.106233
Qu, T., Guan, S., Feng, Y. T., Ma, G., Zhou, W., and Zhao, J. (2023a). Deep active learning for constitutive modelling of granular materials: from representative volume elements to implicit finite element modelling. Int. J. Plast. 164, 103576. doi:10.1016/j.ijplas.2023.103576
Qu, T., Zhao, J., Guan, S., and Feng, Y. T. (2023b). Data-driven multiscale modelling of granular materials via knowledge transfer and sharing. Int. J. Plast. 171, 103786. doi:10.1016/j.ijplas.2023.103786
Santagata, M., Bobet, A., Johnston, C. T., and Hwang, J. (2008). One-dimensional compression behavior of a soil with high organic matter content. J. Geotech. Geoenviron. Eng. 134, 1–13. doi:10.1061/(asce)1090-0241(2008)134:1(1)
Simpson, D. C., and Evans, T. M. (2016). Behavioral thresholds in mixtures of sand and kaolinite clay. J. Geotech. Geoenviron. Eng. 142. doi:10.1061/(ASCE)GT.1943-5606.0001391
Skempton, T. A. W., and Bjerrum, L. (1957). A contribution to the settlement analysis of foundations on clay. Geotechnique 4, 168–178. doi:10.1680/geot.1957.7.4.168
Sowers, H., Georgeb, L., and Sowers, G. F. (1951). Introductory soil mechanics and foundations. Introd. soil Mech. Found. 72, 405. doi:10.1097/00010694-195111000-00014
Suneel, M., Konni, G. R., Chul, I. J., and Dung, N. T. (2018). Secondary compression index equation for soft clays. Geotech. Geol. Eng. 36 (2), 1387–1392. doi:10.1007/s10706-017-0358-x
Vaughan, P. R., Maccarini, M., and Mokhtar, S. M. (1988). Indexing the engineering properties of residual soil. Q. J. Eng. Geol. 21, 69–84. doi:10.1144/gsl.qjeg.1988.021.01.05
Wang, D., and Korkiala-Tanttu, L. (2020). 1-D compressibility behaviour of cement-lime stabilized soft clays. Eur. J. Environ. Civ. Eng. 24, 1013–1031. doi:10.1080/19648189.2018.1440633
Wang, Z., Wong, R. C. K., Qiao, L., and Qiu, W. (2017). Reconsidering secondary compressibility of soil. Int. J. Civ. Eng. 15, 411–418. doi:10.1007/s40999-016-0054-x
Wei, Y., Yang, Y., Wang, J., Zhou, T., Liu, H., Li, J., et al. (2024). Performance of cast-in-place piles in dynamically compacted soil-rock mixtures. Case Stud. Constr. Mater. 20, e03267. doi:10.1016/j.cscm.2024.e03267
Wei, Y., Yang, Y., Zhao, M., Zhou, T., Liu, H., Li, J., et al. (2024). Effectiveness of high-energy dynamic compaction on Layered soil-rock mixture geomaterials based on field test. Bull. Eng. Geol. Environ. 83, 413. doi:10.1007/s10064-024-03912-6
Wu, J., Jing, H., Gao, Y., Meng, Q., Yin, Q., and Du, Y. (2022). Effects of carbon nanotube dosage and aggregate size distribution on mechanical property and microstructure of cemented rockfill. Cem. Concr. Compos. 127, 104408. doi:10.1016/j.cemconcomp.2022.104408
Wu, J., Jing, H., Yin, Q., Yu, L., Meng, B., and Li, S. (2020). Strength prediction model considering material, ultrasonic and stress of cemented waste rock backfill for recycling gangue. J. Clean. Prod. 276, 123189. doi:10.1016/j.jclepro.2020.123189
Wu, J., Wong, H. S., Zhang, H., Yin, Q., Jing, H., and Ma, D. (2024). Improvement of cemented rockfill by premixing low-alkalinity activator and fly ash for recycling gangue and partially replacing cement. Cem. Concr. Compos. 145, 105345. doi:10.1016/j.cemconcomp.2023.105345
Wu, J., Yang, S., Williamson, M., Wong, H. S., Bhudia, T., Pu, H., et al. (2025). Microscopic mechanism of cellulose nanofibers modified cemented gangue backfill materials. Adv. Compos. Hybrid Mater. 8 (2), 177. doi:10.1007/s42114-025-01270-9
Wu, M., and Wang, J. (2023). Exploring particle breakage in sand under triaxial shearing using combined X-ray tomography and particle tracking method. Géotechnique 74, 1684–1699. doi:10.1680/jgeot.22.00351
Wu, Z., Deng, Y., Cui, Y., Chen, Y., Wang, Q., and Feng, Q. (2019). Investigations on secondary compression behaviours of artificial soft sand-clay mixtures. Soils Found. 59, 326–336. doi:10.1016/j.sandf.2018.11.008
Xiao, Y., Zhao, C., Sun, Y., Wang, S., Wu, H., Chen, H., et al. (2021). Compression behavior of MICP-treated sand with various gradations. Acta Geotechnica Int. J. Geoengin. 16, 1391–1400. doi:10.1007/s11440-020-01116-2
Xu, D., Huang, M., and Zhou, Y. (2020). One-dimensional compression behavior of calcareous sand and marine clay mixtures. Int. J. Geomech. 20, 4020137. doi:10.1061/(ASCE)GM.1943-5622.0001763
Xu, P., Qian, H., Chen, J., Wang, L., Abliz, X., He, X., et al. (2023). New insights into microstructure evolution mechanism of compacted loess and its engineering implications. Bull. Eng. Geol. Environ. 82 (1), 36. doi:10.1007/s10064-022-03058-3
Ye, G. L., and Ye, B. (2016). Investigation of the overconsolidation and structural behavior of Shanghai clays by element testing and constitutive modeling. Undergr. Space 1, 62–77. doi:10.1016/j.undsp.2016.08.001
Keywords: soil-rock mixtures, rock content, one-dimensional compression, compression index, secondary compression index
Citation: Gong J, Wang J, Zhang S, Xing Y, Deng L, Long Z, Pang X and Jiang M (2025) Impact of rock content and fine-grained soil properties on the compressive deformation behavior of soil-rock mixtures. Front. Earth Sci. 13:1558854. doi: 10.3389/feart.2025.1558854
Received: 11 January 2025; Accepted: 20 March 2025;
Published: 08 April 2025.
Edited by:
Jiangyu Wu, China University of Mining and Technology, ChinaReviewed by:
Lei Jin, Jiangsu Open University, ChinaCopyright © 2025 Gong, Wang, Zhang, Xing, Deng, Long, Pang and Jiang. This is an open-access article distributed under the terms of the Creative Commons Attribution License (CC BY). The use, distribution or reproduction in other forums is permitted, provided the original author(s) and the copyright owner(s) are credited and that the original publication in this journal is cited, in accordance with accepted academic practice. No use, distribution or reproduction is permitted which does not comply with these terms.
*Correspondence: Mingjie Jiang, MjAxODAxMjFAZ3h1LmVkdS5jbg==
Disclaimer: All claims expressed in this article are solely those of the authors and do not necessarily represent those of their affiliated organizations, or those of the publisher, the editors and the reviewers. Any product that may be evaluated in this article or claim that may be made by its manufacturer is not guaranteed or endorsed by the publisher.
Research integrity at Frontiers
Learn more about the work of our research integrity team to safeguard the quality of each article we publish.