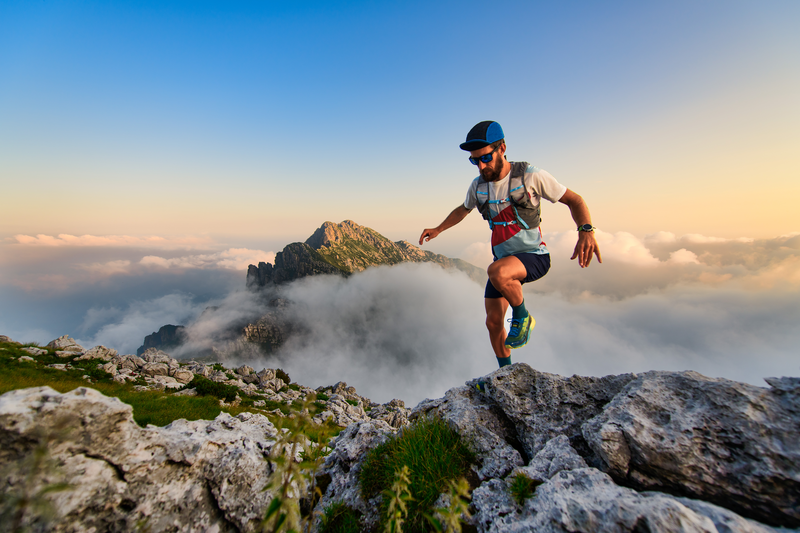
94% of researchers rate our articles as excellent or good
Learn more about the work of our research integrity team to safeguard the quality of each article we publish.
Find out more
ORIGINAL RESEARCH article
Front. Earth Sci.
Sec. Geohazards and Georisks
Volume 13 - 2025 | doi: 10.3389/feart.2025.1556061
This article is part of the Research Topic Evolution Mechanism and Prevention Technology of Karst Geological Engineering Disasters View all 3 articles
The final, formatted version of the article will be published soon.
You have multiple emails registered with Frontiers:
Please enter your email address:
If you already have an account, please login
You don't have a Frontiers account ? You can register here
Grouting technology is a crucial method for mitigating karst water inrush disasters. However, traditional grouting materials face significant limitations when addressing catastrophic karst water inrush under conditions of high-water pressure, large water inflow, and complex hydraulic environments. To overcome these challenges, this study developed a high-performance modified claycement grouting material. A series of indoor experiments were conducted to investigate the effects of the water-to-solid ratio, clay content, and modifier dosage on the material's grouting performance. The optimal mix ratio was identified as water: cement: clay = 2:1:1, with an admixture dosage of 0.75%, by comparing the engineering performance of the new material against pure cement and traditional cement-clay grouting materials. The applicability and effectiveness of the developed grouting material were further validated in a practical engineering application within a water conservancy hub project in Guiping City, Guangxi Province. Results indicate that the new material not only effectively mitigates the risk of catastrophic karst water inrush but also offers advantages such as low cost, ecological benefits, and environmental sustainability. This study provides reliable technical support for emergency grouting applications and demonstrates potential for wider use.
Keywords: Grouting, Karst water gushing, Grouting material, admixture, Rheological properties
Received: 06 Jan 2025; Accepted: 24 Feb 2025.
Copyright: © 2025 Liang, Song, Fan and Wang. This is an open-access article distributed under the terms of the Creative Commons Attribution License (CC BY). The use, distribution or reproduction in other forums is permitted, provided the original author(s) or licensor are credited and that the original publication in this journal is cited, in accordance with accepted academic practice. No use, distribution or reproduction is permitted which does not comply with these terms.
* Correspondence:
Zilong Song, Hunan Institute of Water Resources and Hydropower Research, Changsha, China
Disclaimer: All claims expressed in this article are solely those of the authors and do not necessarily represent those of their affiliated organizations, or those of the publisher, the editors and the reviewers. Any product that may be evaluated in this article or claim that may be made by its manufacturer is not guaranteed or endorsed by the publisher.
Research integrity at Frontiers
Learn more about the work of our research integrity team to safeguard the quality of each article we publish.