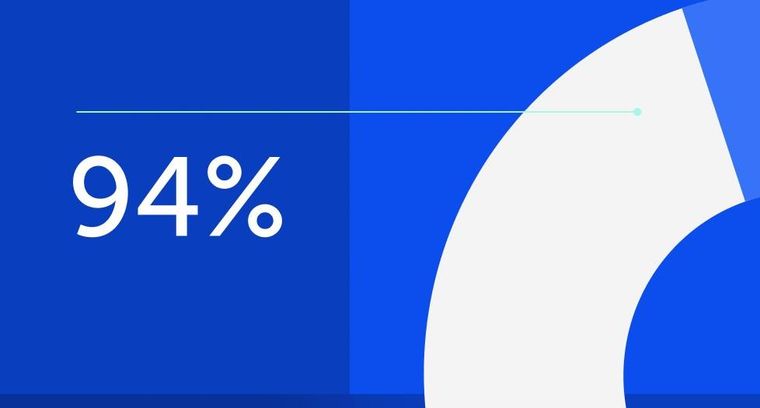
94% of researchers rate our articles as excellent or good
Learn more about the work of our research integrity team to safeguard the quality of each article we publish.
Find out more
ORIGINAL RESEARCH article
Front. Earth Sci., 11 March 2025
Sec. Geohazards and Georisks
Volume 13 - 2025 | https://doi.org/10.3389/feart.2025.1542855
The environmental geological disaster of open-pit mine poses a great threat to the safety of surrounding residents, the stability of urban social economy and the sustainable development of ecological environment. By combining disaster system theory with complex network theory, this study built a network evolution model including 45 distinct disaster nodes and 71 connecting edges through the analysis of disaster-pregnant environment, disaster-causing factors and disaster-bearing body of open-pit mine environmental geological and identified key nodes and connecting edges in the network according to the structural characteristics of the complex network. The results indicate that the environmental geological disasters of open-pit mine are composed of source disaster events, chain-generated disaster events, and final disaster events. By controlling the key nodes of chain disasters such as road disruption, inconvenient living conditions and water pollution, or by cutting off road disruption → traffic obstruction, inconvenient living conditions → disturb social stability, vegetation destruction → soil erosion, soil erosion → degradation of soil function, drainage pipe rupture → poor drainage and other key links of the chain-generated, can effectively control or cut off the network transmission path of the environmental geological disaster chain of open-pit mine. The research results can provide scientific basis for environmental geological disaster control and chain-breaking in open-pit mine, and help to ensure the safety of open-pit mining areas and promote the sustainable development of mining cities.
Mining environmental geological disaster refer to the disasters caused by mining, such as collapse, landslides, mudslides, ground subsidence, destruction of aquifers, and destruction of topographic and geomorphological landscapes, which affect the production and life of the residents, and these environmental geological disasters have become a major bottleneck hindering the sustainable development of the city. Unlike general mines, open-pit mines have distinctive features, namely, a larger exposed surface area and more complex geological conditions, which have resulted in more serious geological and environmental problems. For example, the West and East open-pit mines in Fushun, China, have resulted in a 74.73-square-kilometre subsidence area due to long-term high-intensity coal mining, accounting for 53.4 percent of the area of Fushun city, posing a serious threat to the safety of surrounding residents, the stability of urban social economy and the sustainable development of ecological environment. In view of the complexity of environmental geological disaster in open-pit mine, the evolution process of disaster involves multi-factor coupling and multi-link transmission, which is a typical complex system, which is difficult to be effectively solved by the traditional single hazard analysis method. Therefore, it is necessary to analyze the environmental geological disaster of open-pit mine from the perspective of complex system to minimize its risk, which is not only a major problem in the comprehensive mine management, but also an urgent problem for the sustainable development of resource-based cities.
In recent years, the study of disaster complex system has gradually shifted from single disaster to deep analysis of complex disaster. Compound disaster research can be divided into two kinds of analysis methods: disaster superposition analysis and disaster coupling analysis. The main difference between the two is whether the interaction between disasters is taken into account. Disaster superposition analysis generally first analyzes a single disaster independently, and then adopts certain methods to comprehensively analyze multiple disasters (Bernal et al., 2017). However, disasters in reality usually interact with each other, so it is necessary to use the disaster coupling analysis method to further explore the mutual relationship between disasters. Gill and Malamud (2014) put forward four types of interaction between disasters by analyzing multi-hazard combination cases, including trigger relationship, effect of increasing risk probability, effect of reducing risk probability, and accidental overlap in time and space. This kind of research mainly focuses on the interrelationship between the two disasters. Shi (1996) proposed and improved the “disaster system theory” based on the complexity of disaster systems. Based on the spatio-temporal relationship between disasters, he proposed to discuss complex disasters from the perspective of disaster chain. He pointed out that under certain disaster-prone environmental conditions, there is a mutual transformation mechanism between the disaster-causing factors and the vulnerability of the disaster-bearing body in the regional disaster system. Gill’s model failed to fully explain the evolution process of disasters because of the interaction and dynamic evolutionary relationship between disasters. Therefore, based on the disaster system theory, the study of the evolutionary relationship of complex disasters from the perspective of disaster chain is helpful to reveal the disaster evolution law and the risk characteristics of multi-disaster systems. Guo and Qin (1987) proposed the concept of disaster chain for the first time, believing that disaster chain is a phenomenon of a series of disasters occurring in succession. Taking the Kobe earthquake in Japan in 1995 as an example, Menoni (2001) studied the interaction and coupling effects between disaster events, replaced the prevailing concept of simple coupling disaster loss with the concept of disaster loss chain, and proposed the concept of disaster loss and failure chain. Wen (2000) defines generalized disaster chain as a phenomenon in which one generalized disaster initiates another or more generalized disasters. Mizrahi (2020) analyzed the dynamic laws of cascade disaster evolution, propagation and control by constructing a continuous time model of information cascade and domino effect. Wang and Hou (2023) used DE-Light GBM and SMOTE-Tomek model to convert the risk value of the disaster chain into the sum of the risk value of rainstorm and secondary geological disaster, so as to assess the risk of the storm-geological disaster chain. In addition, many scholars have studied the chain effect of disasters through the theories of Natech problem (O'Reilly et al., 2023; Ricci et al., 2023; Di Maio et al., 2023; Hao et al., 2023), cascade disaster (Sutrisno et al., 2022; Triyanti et al., 2023), cascade event (Ntambara and Umuhoza, 2022; Poulomi et al., 2022), cascade effect (Tang et al., 2023) or domino effect (Li X. et al., 2023) and other risk fields with multiple disasters.
Most of the research methods of disaster chain are qualitative analysis and description of the formation and evolution process mechanism and characteristics of disaster chain from different angles. It mainly includes the probability analysis method based on data, the research method based on remote sensing and the research method based on complex network. Han et al. (2019) used Bayesian networks to assess the risk of earthquake disaster chain based on probability analysis method. Xu et al. (2011) used remote sensing images and field survey data to study the disaster chain of earthquake landslide, debris flow, drastic river bed evolution and ecological destruction caused by Wenchuan earthquake. Chen et al. (2021) combined disaster chain and complex network theory to build a weighted complex network model and analyze urban traffic system from macro and micro perspectives. In the above studies, the probability analysis method mainly focuses on the relationship between disaster-causing factors, and cannot accurately describe the relationship between disasters in the disaster chain. The remote sensing measurement method mainly focuses on geological disasters, and the other disasters are few. As a tool used to study the geometric properties, formation mechanism and evolution law of networks, complex network method can be organically combined with all kinds of network events in reality, and directly used to explore the dynamic process of disaster chain accumulation and amplification. Therefore, some scholars use complex network methods to study the evolution process of disasters with the help of network characteristic indicators, such as basic characteristics, static structure or dynamic characteristics of the system. At present, it has been used in many aspects such as urban rail transit (Li et al., 2021), fire (Song et al., 2020), agriculture (Luo et al., 2020) and mountain railway slip-flow disaster (Yang et al., 2020). The research covers the evolution and transmission of disasters, risk assessment and prevention and control measures. Based on the improved SIR Model, Chen and Yin (2023) studied the transmission mechanism of the supply chain of emergency supplies under the background of the novel coronavirus epidemic, and established an enterprise risk early warning system accordingly. Hu et al. (2022) analyzed the cascade effect caused by typhoons by using complex network method, and identified the types of disaster events in urban areas and their vulnerability paths.
Research on mine disaster risk mainly focuses on the internal geological disaster risk caused by mining. For example, Li et al. (2024) studied the instability mechanism of weak sandwich open-pit slope through physical and numerical simulation. Fang et al. (2024) analyzed the classification and characteristics of slopes caused by excavation, laying a foundation for the safe management of mining activities. Some scholars also studied the chain reaction caused by single disaster species in mining area (Qi et al., 2022; Yan et al., 2022), for example, Niu et al. (2023) studied the prevention and control methods of underground debris flow by applying disaster chain theory. In the form of disaster chain, the study on the evolution process of disaster from internal disaster in mining area to disaster in urban system under the action of mining is limited to Shao et al. (2023), who built disaster chain evolution model of underground tungsten mine under the influence of rainstorm and flood, taking rainstorm and flood as disaster source and destruction of urban system as final result. However, for the open-pit mine with more complex environmental geological conditions, its harm to the mining area and the city is a long-term accumulation and cyclic evolution process in time and space, which is rarely mentioned in the current research. The environmental geological disaster of open-pit mine are complex and dynamic, and the disaster-bearing body is not only in the mine, but also becomes a new disaster-causing factors with the evolution of the disaster, spawning a new disaster-pregnant environment, and finally acting on the surrounding area of the mine and the urban system. Therefore, in order to study the evolutionary relationship between environmental geological disasters in open-pit mines, the risk of environmental geological disasters in open-pit mines can be analyzed from the perspective of disaster chain with the help of network characteristic indexes based on disaster system theory and complex network theory, and the law of disaster evolution can be revealed.
From the above studies, the research on environmental geological disasters in mines has made some progress, but due to the complex slope conditions of open-pit mines, large-scale ecological damage and other characteristics, the research on environmental geological disasters in open-pit mines is still insufficient, especially for the analysis of the evolution of environmental geological disaster in open pit mine is still underdeveloped. Therefore, different from previous studies, the article starts from the perspective of disaster chain, applies the disaster system theory and complex network method, analyses the disaster mechanism of the environmental geological disaster chain of open-pit mines, constructs the network evolution model of the disaster chain, comprehensively analyses the importance of the nodes of the disaster network and the vulnerability of the edges, identifies the key factors of the disaster and the key links in the disaster chain of the disaster, and puts forward the risk management and control measures of the key factors and the key links. This study aims to provide scientific foundations for the prevention and control of secondary or derivative environmental geological disasters in open-pit mines, support decision-making for chain-breaking and disaster reduction, and enhance pre-disaster preparation and in-disaster management to minimize the losses caused by disaster chain reactions.
The theory of disaster system proposes that the main structure of disaster system consists of disaster-pregnant environment, disaster-causing factor and disaster-bearing body (Shi, 1996). Disaster chain refers to the chain reaction of a series of disaster events triggered by the initial disaster-causing factors in the disaster-pregnant environment in a disaster system. Specifically, the initial disaster-causing factor, under the gestation of the disaster-pregnant environment, first causes a primary disaster, and then the primary disaster becomes a new disaster-causing factor, which further triggers secondary disasters. This process is repeated constantly, leading to the progressive evolution of disaster events in time and space, and finally forming a disaster chain (Gao et al., 2024). Accordingly, the environmental geological disaster chain of open-pit mine area is defined as: Within the scope of damage caused by open-pit mining activities, the balance of geological environment in open-pit mining areas is disrupted due to external dynamic factors such as crustal movement and precipitation or human factors such as mining, which leads to environmental geological disasters. After the occurrence of environmental geological disasters, the environmental geological disasters act on the disaster-bearing body inside the mine, causing pollution and damage to the mine, and the disaster-bearing body inside the damaged mining area breeds a new disaster-pregnant environment. It becomes a new disaster-causing factors, thus forming a chain reaction of a series of disaster events, leading to a series of secondary disaster events occurring successively, until it affects the residents around the mine and the urban economy, ecological environment and other disaster-bearing body.
From the definition of the environmental geological disaster chain in open-pit mines, it can be known that in the disaster chain, disaster-causing factors act on disaster-bearing body, and disaster-bearing body can also transform into disaster-causing factors. Therefore, the disaster-forming mechanism of the disaster chain can be analyzed from the process of disaster propagation. As depicted in Figure 1, within a disaster-pregnant environment, two primary disaster-causing factors: unstable slopes and slag accumulation affect disaster-bearing body, including houses, lifelines, the geological environment, and surface ecology in the vicinity of the mining area. After being impacted by the disaster, the above disaster-bearing body become new disaster-causing factors and impacting other disaster-bearing body in the surrounding area of the open-pit mine and even in the city, ultimately affecting the city’s ecological environment and socio-economic system. The specific manifestations of each part are detailed below.
Disaster-pregnant environment is the background condition for the occurrence of disasters. The disaster-prone environment of open pit environmental geological disasters refers to the disturbance of the original geological structure and ecological balance in the process of open-pit mine, which makes the geological, hydrological and ecological constraints that maintain regional stability ineffective, thus forming the potential background conditions for disaster breeding. The formation of this environment is mainly influenced by the regional environmental stability and the spatio-temporal distribution of natural disasters (Shi, 1996). Consequently, the disaster-pregnant environment of the environmental geological disaster chain in open-pit mines can be categorized into two main types (Zhao and Tian, 2021). Firstly, the disaster-pregnant environment influenced by regional stability mainly refers to the ecological and geological environment such as ground features and geological structures that inherently exist in the open-pit mine and its affected area, such as the changes in the slope gradient of open-pit mine slopes, surface ecology, and geological structures caused by human mining engineering activities. Secondly, the disaster-pregnant environment affected by the temporal and spatial distribution laws of natural disasters encompasses the environmental changes that are passively induced in the open-pit mine due to natural events like climate variations and crustal activities. For instance, rainfall and earthquakes can cause changes in the slope gradients of mine slopes, surface ecology, and geological structures.
Disaster-causing factors refer to the direct factors that lead to the release of disaster energy or the occurrence of disaster events in the context of the formation of disaster-pregnant environment. Disaster-causing factors of open-pit mine environmental geological disasters refer to the direct factors that cause sudden release of disaster energy and lead to the occurrence of open-pit mine environmental geological disasters when the environmental balance is broken and the stable state reaches the limit due to open-pit mining activities. Different disaster causing factors are produced in different environmental systems (Hao et al., 2022; He et al., 2024). In the context of environmental geological disasters in open-pit mines, mining activities are the primary disaster-causing factors, while natural disasters such as rainfall and earthquakes serve as auxiliary factors. This paper focuses on investigating the disaster-causing factors that stem from the environmental stability of the hazard-prone environment. Additionally, under certain conditions, the disaster-bearing body can become a disaster-causing factor in subsequent events within the disaster chain. For example, a landslide that topples high-voltage lines can transform the damaged power facilities into a new disaster-causing factor, leading to power outages. Considering the specific characteristics of environmental geological disasters in open-pit mines, factors such as geological faults, folds, potential sliding masses, slope angle humidity, and the accumulation of mine slag from mining activities collectively constitute the disaster-causing factors. These can be categorized into two types: mine slag accumulation disasters and unstable slope disasters.
Slag accumulation is the phenomenon of waste rock accumulation caused by stripping of topsoil and rock in open-pit mine. Due to the large scale of mining and the large amount of stripping material, if not properly treated, it will form a slag accumulation disaster, affecting mine production and urban environment. At the same time, the open-pit slope is exposed to the natural environment for a long time, and the rock mass is susceptible to weathering and rainfall, and the strength is reduced, and with the increase of mining depth, the high and steep slope increases, resulting in the slope stability is reduced. The unstable slope disaster of open-pit mine refers to the deformation, sliding and collapse of the rock mass of the slope in the process of open pit mining due to the influence of geological conditions, hydrological conditions, mining activities and other factors, which causes serious harm to mine production, personnel safety and equipment.
Disaster-bearing body refers to the carrier of damage caused by accidents, including personnel, property, natural environment, etc. (Wang et al., 2024; Wang et al., 2023). The disaster-bearing body of environmental geological disaster of open-pit mine refers to the residents around the mine area, the urban social and economic system and the urban ecological system that may be damaged when the disaster occurs, and the specific manifestations are casualties, property losses, social instability and ecological damage. In this study, the disaster-bearing body of environmental geological disasters in open-pit mines are located in the surrounding areas and urban systems of mining areas. The initial disaster causing factor is mining behavior, which first affects the natural stress field of rock mass and the groundwater system. With the continuous expansion of disaster chain intensity, the impact scope involves various aspects such as atmosphere, soil, water and biology. Therefore, the disaster-bearing body influenced by the environmental geological disaster chain of open-pit mines encompass all the constituent elements of the human living environment and human beings themselves, including the objects acted upon by three types of disaster-causing factors: the residents around the mining area, the ecological environment system, and the socio-economic system.
The residents around the mining area are mainly manifested as follows: on the one hand, geological disasters such as open-pit mine landslide, ground subsidence, and ground cracks lead to serious damage to buildings, underground pipelines, and roads, posing a threat to the safety of surrounding residents. On the other hand, frequent geological disasters lead to fear among residents, which is not conducive to social stability. In addition, when the disaster reaches a certain extent, residents are forced to move and the community structure is changed. Long-term instability may cause the cohesion of community residents to decline, the social relationship network to be impacted, and the psychological insecurity of residents (Fang et al., 2023).
The ecological environment system is mainly manifested as follows: Open-pit mines, spent ore dump, and areas affected by landslides and subsidence occupy large areas of land, leading to a reduction in the land’s greening rate. Agricultural cultivated land in the subsidence areas is severely flooded and largely abandoned. The accumulation of mine slag and the leaching of pollutants have resulted in significant heavy metal contamination of the surrounding soil, further exacerbating soil pollution. Rivers flowing through the mining area are heavily polluted, leading to a gradual depletion of water resources and a decline in the groundwater level (Feng et al., 2024).
The socio-economic system is mainly manifested as follows: The damage to infrastructure, residential houses and commercial buildings demands a considerable amount of funds for repair or reconstruction, bringing direct economic losses to residents and enterprises. The ongoing monitoring, early warning, and disaster prevention and mitigation efforts in the mining area require significant capital investment. The extensive damage to infrastructure and the ecological environment has led to a notable outflow of the working-age population, contributing to a demographic shift characterized by an aging urban population. Additionally, these factors have hindered the influx of talent and external enterprises, thereby restraining the city’s economic development (Zhou et al., 2022).
In conclusion, the specific disaster-pregnant environment and the characteristics of disaster-bearing body around open-pit mines result in a distinct pattern of environmental geological disaster chains, influenced by various disaster-causing factors, as illustrated in Figure 2.
The environmental geological disaster events in open-pit mining areas have a complex propagation mechanism (Su et al., 2022; Xu et al., 2022). When a disaster occurs, it is often accompanied by the creation of secondary disasters, which may lead to the formation of disaster chains. In the system, the same disaster nodes may appear in different disaster chains, which can be represented by the nodes and the connections between them. Due to the existence of the same disaster nodes in different disaster chains, these nodes make connections between different disaster chains, forming a complex network of disaster chains.
In the research of environmental geological disaster chains in open-pit mines, disaster events are defined as network nodes, and the propagation relationships among disasters are regarded as the edges connecting these nodes, so as to abstract the topology of the network and form a complex network. This paper employs complex network theory to analyze the evolution of the environmental geological disaster chain network in open-pit mines, with the specific analysis process shown in Figure 3. Firstly, based on the disaster-causing mechanism of environmental geological disasters in open-pit mines, disaster events are selected through literature research and field investigations, and a complex network evolution model based on the interrelatedness of these events is constructed. Then, the importance of nodes and the vulnerability of edges in the model are analyzed. The importance of nodes is described by node degree, betweenness centrality, and clustering coefficient, while the vulnerability of connecting edges is evaluated by edge betweenness, connectivity, and average path length. Finally, through a comprehensive analysis of the environmental geological disaster network in open-pit mines, critical nodes and connecting edges are identified, and corresponding prevention, emergency response, and chain-breaking mitigation measures are proposed.
Figure 3. Analysis process of environmental geological disaster chain network evolution in open-pit mine.
Nodes play a crucial role as control factors in network systems. The higher the importance of a node, the more significant the corresponding disaster event becomes within the network (Jiang and Yue, 2021). For a more precise assessment of the significance of nodes, this study, in conjunction with the evolutionary traits of the environmental geological disaster chain network in open-pit mines, assumes that the edge probability between nodes in a complex unweighted network is 1. Based on the applicable range of node characteristic parameters, three key indicators were selected to quantify the characteristics of nodes: the in-degree and out-degree, which reflect the node’s connectivity within the local network; the betweenness centrality, which indicates the node’s role as a key intermediary in the complex network; and the clustering coefficient, which represents the aggregation characteristics of the node. These three indicators not only account for local connectivity but also consider global intermediary roles and local clustering features. Finally, based on the calculation results of these three indicators, the top three nodes with the highest frequency of appearance in the top rankings for each indicator were selected as key nodes.
Node degree. Since the environmental geological disaster chain network of open-pit mines is a directed graph, the degree of disaster event nodes can be categorized into out-degree and in-degree. The out-degree represents the degree of influence of the event on other secondary disasters. A higher out-degree signifies more severe consequences caused by the event. The in-degree reflects the degree to which the event node is affected. The greater the in-degree, the more paths that lead to the event, and the greater the difficulty of control.
Betweenness centrality. In the network of environmental geological disaster chains in open-pit mines, the betweenness centrality of a node refers to the proportion of shortest paths that pass through the node relative to the total number of shortest paths. The shortest path represents the fastest way for a disaster to progress from node s to node t. Betweenness centrality reflects the extent to which a disaster event, acting as an intermediary, influences other events in the disaster evolution network. It serves as a measure of the risk diffusion effect of disaster events within a complex disaster network. Its expression is as follows:
where, Cb(v) represents the betweenness centrality of the node.
Clustering coefficient. In the network of environmental geological disaster chains in open-pit mines, the clustering coefficient of a node is used to measure the degree of clustering between nodes in the disaster complex network. A higher clustering coefficient indicates a stronger connection between the event node and other disaster events, reflecting a higher degree of aggregation of the disaster events. The node clustering coefficient is determined by calculating the ratio of the number of actual edges between neighboring nodes to the maximum possible number of edges that could exist between them. Its expression is as follows:
where, Cv represents the node clustering coefficient. T(v) is the number of actual existing edges among the neighboring nodes of node v; kv is the degree of node v; kv (kv −1) represents the maximum possible number of edges among all the neighbors of node v. Cv ∈[0,1], where Cv = 0 indicates that node vi is in an isolated state without any group connection, and Cv = 1 indicates that all the other nodes connected to node vi are also interconnected.
The network clustering coefficient reflects the aggregation of nodes in the network, and the larger the value, the better the overall clustering of the network. Its expression is as follows:
The greater the vulnerability of network edges, the higher their importance (Alizadeh et al., 2023). Based on the edge parameter characteristics analysis in the evolutionary process of complex networks provided by scholars such as Li et al. (2021), Yang et al. (2020), and Luo et al. (2020), this study selects edge betweenness, average path length, and network connectivity to construct the vulnerability of connecting edges.
Edge betweenness (B). Edge betweenness refers to the number of shortest paths that pass through a particular edge in a network. In the network of environmental geological disaster chains in open-pit mines, the higher the edge betweenness of a connecting edge, the more disaster evolution paths it influences, thereby having a greater impact on the disaster evolution process. Its expression is as follows:
where, Bi is the betweenness of edge i, and njk(i) is the number of times the shortest path between nodes j and k passes through edge i.
Connectivity (H). Network connectivity refers to the proportion of connected nodes in the network relative to all nodes, reflecting the connectivity and stability of the network. In the network of environmental geological disaster chains in open-pit mines, if a certain connection edge is removed from the complex disaster network and the connectivity decreases, resulting in the interruption of specific hazardous events that would have occurred in the original disaster chain network, it suggests that this edge will influence the development of subsequent disaster nodes, demonstrating that this connection edge is in a relatively vulnerable link during the disaster evolution process. Its expression is as follows:
where, H is the connectivity, n is the total number of nodes in the network, and ni is the number of nodes that can be reached starting from node i.
Average path length (L). The average path length of a network is the average length of the shortest paths between all pairs of nodes. In the network of environmental geological disaster chains in open-pit mines, this metric reflects the evolution rate of disaster events in the network. When the removal of a connecting edge increases the network’s average path length, it suggests that the removal can delay the disaster evolution process, indicating that the connecting edge plays a critical role in the network. Therefore, by comparing the average path length of the network after the removal of different connecting edges, the impact of these edges on the propagation of disaster evolution can be analyzed. Its expression is as follows:
where, L is the average path length, n is the total number of network nodes, and dij is the shortest distance between nodes i and j.
Vulnerability of connecting edges (V). This study constructs a vulnerability of connecting edges to characterize their significance in the disaster evolution process. Analyzing the vulnerability of the complex network topology based on the variations of network connection edges enables the assessment of the risk value of connection edges in disaster events. This research constructs the vulnerability index of connection edges in disaster complex networks based on three indicators: the average path length of the network, the betweenness of edges, and the connectivity of the network. Consequently, the vulnerability Vi of connecting edge i is calculated using Equation (7). Bi denotes the betweenness of connecting edge i, and Li and Hi represent the average path length and the connectivity of the network after the removal of connecting edge i. Its expression is as follows:
where, k is the total number of edges in the disaster chain, Bi is the betweenness of edge i, Li and Hi are the average path length and connectivity of the network after removing edge i, respectively.
Relative vulnerability of edges (VR). Since the vulnerability assessment results of the edges are numerically concentrated, in order to present the results more intuitively, this study adopts a grading method similar to that used in climate vulnerability assessment (Elangbam and Manglem, 2024). The natural breaks method is employed to classify the edge vulnerability assessment results. The ratio of the edge vulnerability to the difference between the maximum and minimum vulnerability is utilized for comparison to obtain the relative vulnerability. This relative vulnerability is then used to grade the vulnerability assessment results of the edges. Its expression is as follows:
The vulnerability is divided into four levels: low vulnerability, medium vulnerability, high vulnerability, and extremely high vulnerability, representing the urgency of chain-breaking and disaster reduction from weak to strong. The relative vulnerability classification table is shown in Table 1. For the connecting edge of the open-pit environmental geological disaster chain network, when the relative vulnerability is 0–0.25, it is low vulnerability. The chain breaking treatment of this edge is not very urgent, and can be considered as part of long-term planning. When the relative vulnerability is 0.25–0.75, attention should be paid to the edge, and it is recommended to consider its chain breaking treatment measures in the medium-term planning. When the relative vulnerability is 0.5–0.75, it indicates that the edge is more important, and it is recommended to give priority to its chain breaking treatment measures. When the relative vulnerability value is 0.75–1, it indicates that these edges are very fragile, and their chain breaking processing has a very important impact on the entire disaster evolution network structure, and is a key link in disaster management. It is recommended to take immediate measures to cut off the connection with the next disaster event.
In the environmental geological disaster chain system of open-pit mines, the interaction of various disaster-causing factors maintains the system in a state of continuous dynamic change, complicating the identification of disaster events. Additionally, the relevant management institutions of open-pit mines lack complete statistical data on disaster situations (Loupasakis, 2020). Therefore, based on the disaster-forming mechanism of the environmental geological disaster chain in open-pit mines, methods such as literature analysis, field investigations, and expert consultations are employed to select environmental geological disaster events in open-pit mines. Firstly, academic online platforms such as Web of Science and CNKI, as well as online news reports, disaster situation reports on government websites, and emergency management case database were used to collect disaster occurrence process, accident experience and lessons related to topics such as “open-pit mine risks”, “open-pit mine disasters”, and “mine geological environment”. The environmental geological disaster events in the open-pit mine area were preliminarily identified. Secondly, field investigations were conducted at several open-pit mines, including Fushun West Open-pit Mine, Fushun East Open-pit Mine, Fuxin Haizhou Mine, and Anshan Dagushan Iron Mine, the environmental geological disaster events are supplemented. Finally, by integrating the environmental geological disaster events of open-pit mines obtained from literature, cases, and investigations, and through various means such as distributing questionnaires, sending emails, and conducting interviews via teleconferences to numerous experts and workers in the fields of mine safety and disaster risk management from institutions like the government’s geological disaster prevention department and the mining bureau, the following 45 representative and highly probable environmental geological disaster events in open-pit mines regarding the disaster chain formation mechanism were ultimately obtained. As shown in Table 2.
Table 2. Disaster event evolution of environmental geological disaster chain network in open-pit mine.
The evolution system of environmental geological disasters in open pit mine is a complex system, including primary disasters, secondary disasters induced by them, and derivative disasters. After identifying the environmental geological disaster event, it is necessary to analyze its causality. Through questionnaire survey of experts engaged in the field of mine safety and disaster risk management, this paper finds out whether there is a mutual influence relationship between disaster events and analyzes the network relationship between disaster events. In addition, the disaster-forming mechanism and pattern of environmental geological disaster chains in open-pit mines indicate that although open-pit mines are influenced by various factors such as the original topographic conditions, hydrogeology, ore characteristics, and mining methods, the extent of damage caused by disasters varies (Wang et al., 2019). However, the primary disaster factors mainly stem from slag accumulation disaster (Wang et al., 2021) and unstable slope disaster (Li W. et al., 2023; Salvoni and Dight, 2016) resulting from mining activities. Among the numerous disaster chains triggered by these two disaster-causing factors, there exist identical disaster events, causing the interconnection among disaster chains and forming a network structure. Based on the disaster-forming mechanism and patterns of environmental geological disasters in open-pit mines, this study applies complex network methods. By combining the disaster events selected in Table 2 with the survey results on the triggering relationships between these events, a network evolution model of the environmental geological disaster chain in open-pit mines, as shown in Figure 4. The model encompasses two sub-disaster systems: the slag heap disaster and the unstable slope disaster. These two sub-systems mutually evolve under the influence of the disaster-pregnant environment, multiplying the scale and destructive power of disasters. According to the evolution sequence of disasters in the disaster chain, disaster evolution events can be classified into three categories: source disaster events, chain-generated disaster events, and final disaster events. Source disaster events refer to the initial events that trigger the outbreak of environmental geological disasters in open-pit mines in the disaster-pregnant environment, namely, the initial disaster-causing factors. When the initial disaster-causing factors act on the disaster-bearing body, new disaster events will emerge. At this point, these disaster events create new disaster-pregnant environments and transform into new disaster-causing factors, continuing to act on new disaster-bearing body. The series of disaster events resulting from this repeated evolution process are referred to as chain-generated disaster events. After the evolution of chain-generated disaster events, the disaster finally acts on the urban socio-economic system and ecological environment system, resulting in the final system damage event, which is called the final disaster events. The constructed disaster chain evolution model comprises 45 nodes and 71 connecting edges. The 45 nodes represent 45 disaster events, and the 71 directional connecting edges represent the development relationships between disaster events, with the direction arrows indicating the direction of disaster evolution.
Utilizing the complex network node importance analysis method outlined in Section 2.2.2 and Formulas 1–3, the node and edge information of the constructed open-pit mining environmental geological disaster chain network model were input into the Python NetworkX library. By defining the connections between nodes and edges, the corresponding network graph was constructed. The out-degree, in-degree, betweenness centrality, and clustering coefficient of each disaster event in the open-pit mine environmental geological disaster chain network were computed using Python programming. The results are shown in Figure 5.
Figure 5. Node importance parameter of environmental geological disaster chain network in Open-pit mine. (A) Node degree. (B) Betweenness Centrality. (C) Clustering coefficient.
The in-degree and out-degree are metrics that characterize the input and output relationships of disaster events within a network. The in-degree of an event signifies the number of inducing events leading to it, while the out-degree signifies the number of events that it triggers. As depicted in Figure 5A, with the exception of the source disaster events, nodes such as V7 (building damage), V3 (water pollution), and V24 (fire and explosion) exhibit relatively high out-degrees. This indicates that these events have a broad influence and can lead to severe consequences in the evolution of the disaster chain. Conversely, terminal disaster events like V25 (inconvenient living conditions) and V8 (road disruption) display relatively high in-degrees, suggesting that these events are precipitated by multiple factors and are consequently more challenging to manage.
To analyze the “bridging” role of specific events in the propagation path, the betweenness centrality of each event in the evolutionary network graph was calculated. As shown in Figure 5B, environmental geological disaster network in the open-pit mine, nodes V8 (road disruption), V25 (inconvenient living conditions), V28 (land destruction), and V13 (vegetation destruction) exhibit relatively high betweenness centrality values. This indicates that these nodes exert significant influence within the network, are closely connected to other subsequent nodes, and play critical roles in leading to disasters around the open-pit mine.
The clustering coefficient results reflect the extent of correlation among various disasters in the disaster chain. As depicted in Figure 5C, within the evolution network of environmental geological disasters in open-pit mines, there are 11 disaster events with a clustering coefficient greater than 0, These include V15 (ground fissure), V35 (mechanical damage), V16 (subgrade settlement), V14 (building subsidence), V37 (road subsidence), V8 (road disruption), V1 (slag accumulation disaster), V3 (water pollution), V5 (soil pollution), V36 (factory shutdowns or operations with hazards), and V2 (unstable slope disaster). They constitute 24% of the total disaster events. This implies that these risk events are closely associated with the adjacent events in the disaster network and present a certain degree of collectivization (Zhou et al., 2023). Nevertheless, the extent of collectivization is not high. The overall clustering coefficient of the disaster chain network is C = 0.046, with most nodes having a coefficient of 0, indicating that the majority of disaster events are not closely linked. There is a significant number of “single node - single node” types of disaster evolution events, which are relatively manageable. By severing the propagation pathways between nodes, the final disaster can be effectively controlled. Therefore, the evolution relationships of disaster events with a clustering coefficient greater than 0 are more complex and warrant attention in disaster control strategies.
The top three nodes with the highest occurrence frequencies, as previously mentioned, are identified as key nodes within the network: V8 (road disruption), V25(Inconvenient living conditions), and V3(water pollution). Targeted disaster prevention measures are accordingly implemented for these critical nodes.
(1) For the disaster node of V8 (road disruption), the primary inducing factors are unstable slope disasters and subgrade settlement. It is mainly due to the damage of surrounding roads caused by unstable slopes such as landslides in open-pit mines, resulting in road disruption and subsequent traffic obstruction. To prevent such road disruption disaster events, physical measures can be implemented, including backfilling and footing of open-pit mine slopes, slope reinforcement, crack filling, anti-slide piles, grouting reinforcement, and slope cutting with load reduction. Moreover, technical disaster reduction measures such as the establishment of early warning and forecasting mechanisms, real-time monitoring systems for open-pit mines. These systems may incorporate high-precision remote sensing technology, manual inspection systems, and expert assessment measures. The disaster event resulting from the evolution of the road disruption node is traffic obstruction. Consequently, emergency measures for the V8 node involve traffic control, maintenance of normal traffic order, and timely emergency rescue operations, including on-site investigation, operation, safety monitoring, warning, and the transfer of affected personnel on the affected road surface. For instance, in 2013, two surface cracks emerged on the southern slope of Qiantai Mountain in the south of the Fushun West Open-pit Mine, with a total length exceeding 2.7 km and the overall volume of the deformed mass exceeding 100 million cubic meters. This resulted in the cracking of roads in the nearby Dongli Community and blocked traffic. The local government promptly activated an emergency response plan, organized the evacuation of residents from the affected area, and prevented casualties.
(2) For the disaster node of V25 (inconvenient living conditions), the primary inducing factors include building damage, traffic obstruction, water outage, electric power accident, power outages, and gas outage. These are largely attributed to the impact of unstable slope disasters around open-pit mines, where large-scale slope deformations, underground seepage, and mine-induced earthquakes lead to disasters such as surface subsidence and ground fissures in the surrounding areas. These phenomena lead to tensile cracks in water, electricity, and gas pipelines, and water intrusion easily causes structural damage. Hence, during the design and construction of pipelines, strict quality control should be imposed on pipeline equipment and materials, for instance, by setting up flexible joints to address the damage to pipe gallery structures caused by ground fissure activities. For underground pipelines, waterproofing efforts should be meticulous, and two approaches can be adopted: waterproofing of deformation joints and self-waterproofing of concrete structures. Meanwhile, surrounding houses are affected by disasters, resulting in phenomena such as wall cracking, wall bulging, inclination and cracking of wall columns, and foundation detachment. Reinforcement should be carried out for the foundations, walls, beams, and columns of buildings around open-pit mines, and pre-disaster early warning should be enhanced. The disaster events evolving from inconvenient living conditions can disturb social stability. Therefore, the emergency measures for the V25 node are, first, to promptly carry out emergency repairs of infrastructure such as water, electricity, and gas in the disaster-stricken area. Secondly, for severely affected areas, it is necessary to evacuate the masses to corresponding emergency shelters. Finally, the maintenance of public security in the disaster-stricken area should be strengthened. For example, in 2016, a rainfall-induced landslide occurred on the northern slope of the Fushun West Open-pit Mine, causing severe damage to electrical infrastructure and disrupting multiple power transmission lines. The Fushun Power Supply Bureau immediately activated its emergency response plan, mobilized emergency power generators and repair crews, and carried out urgent repairs on the damaged electrical facilities to restore power supply.
(3) For the disaster node of V3 (water pollution), the primary inducing factor is slag accumulation disaster. To address this, the surface layer of the slag can be filled and compacted with loess. This approach prevents the slag from coming into contact with air and rainwater, thereby reducing the risk of leaching and other chemical reactions that could exacerbate water pollution. Furthermore, ecological restoration can be initiated by introducing green plants to the treated surface, which not only facilitates the ecological governance of solid waste but also gradually harmonizes the treated area with the surrounding ecological environment, restoring its ecological functions. The disaster events resulting from the evolution of water pollution include increased cancer risk, animal deaths, and soil pollution. Sewage is subjected to filtration, purification, and disinfection processes before being used for plant irrigation, while drinking water undergoes strict disinfection protocols before consumption. For instance, on 20 July 2021, Zhengzhou experienced an extreme heavy rainfall event. Local communities and rescue teams distributed alum and bleaching powder for water purification and disinfection to ensure the safety of drinking water.
The measures outlined above are technically mature and have been proven successful in previous cases, such as the comprehensive management project at the Fushun West Open-pit Mine. Since 2019, the West Open-pit Mine has implemented various engineering measures, including backfilling and footing of open-pit mine slopes, slope cutting with load reduction, along with technical measures such as slope monitoring radar and the installation of GPS rock movement monitoring points. It has also implemented multiple ecological management measures, including mine pit water prevention and ecological restoration. However, these measures require significant upfront investment. For example, the total investment for the measures carried out at the Fushun West Open-pit Mine over the past 5 years has reached approximately 2.7 billion yuan. The economic benefits, social stability, and environmental benefits derived from these investments are long-term and substantial. For instance, after grouting reinforcement on the north slope of the West Open-pit Mine, the physical and mechanical strength of the rock and soil mass on the north slope has been enhanced. After filling and compacting 7,000 m of surface cracks on the south slope, no new cracks or damage have emerged, and the deformed area of the slope has regained stability. Through vegetation planting in the western internal spoil bank, the area of ecological restoration has reached 6.7 ha. These achievements have prevented the occurrence of disasters, reduced the direct economic losses of surrounding residents, mitigated the impact of disasters on residents’ lives, and played a positive role in maintaining the stability of the urban social economy. Moreover, the improvement in the ecological environment of the mining area has enhanced the local ecological quality and promoted the sustainable development of the urban environment.
Based on the complex network edge vulnerability analysis method and Formulas 4–8 presented in section 2.2.2, Python programming was utilized to calculate the edge betweenness, connectivity, average path length, vulnerability of connecting edges, and relative vulnerability of connecting edges in the environmental geological disaster chain network of open-pit mines. The calculated values are summarized in Table 3. To enhance the intuitiveness of these results, line graphs depicting edge betweenness, connectivity, average path length, and vulnerability of connecting edges were created and are displayed in Figure 6. Additionally, the relative vulnerabilities of connecting edges within the environmental geological disaster chain network of open-pit mines are presented, with edge vulnerabilities categorized into four grades. This classification facilitates an intuitive assessment of the influence of each edge on the overall network, as illustrated in Figure 7.
Table 3. Vulnerability calculation at the connection edge of evolution network of environmental geological disaster chain in open-pit mine.
Figure 6. Vulnerability parameters at the connecting edge of environmental geological disaster chain network in Open-pit mine. (A) Edge betweenness. (B) Connectivity. (C) Average path length. (D) Vulnerability of connecting edges.
Figure 7. Vulnerability scale at the connection edge of evolution network of environmental geological disaster chain in Open-pit mine.
Based on the previous analysis of the vulnerability of connecting edges in the network, it is observed that higher vulnerability correlates with increased fragility. It is known from Equation 7 that the vulnerability of a certain edge in the network is related to the edge betweenness, connectivity and average path length of that edge. Analysis of Table 3 and Figure 6 reveals no simple direct positive or negative correlation between individual indicator values and edge vulnerability. For example, the vulnerability of each edge does not exhibit a consistently increasing or decreasing trend in relation to edge betweenness, connectivity, or average path length. Therefore, a comprehensive consideration of these indicators is necessary for a scientific and holistic analysis of edge vulnerability. Five parameters-edge betweenness, connectivity, average path length, vulnerability of connecting edges, and relative vulnerability of connecting edges-were selected for the analysis, leading to the following conclusions.
As shown in Figure 6A, in the environmental geological disaster chain network of the open-pit mine, the edges V8→V30 and V25→V45 have relatively high edge betweenness values, which are 19 and 17 respectively. This suggests that the shortest paths in the network pass through these two edges more frequently, namely, road disruption → traffic obstruction and inconvenient living conditions → disturb social stability, have a significant influence on the disaster evolution paths within the network. Therefore, priority should be given to implementing chain-breaking disaster reduction measures on these two edges. On the other hand, the betweenness centrality of the edge from V2→V15 is only 1, suggesting that the influence of the edge representing unstable slope → ground fissure is limited. Thus, implementing chain-breaking disaster reduction measures on this edge would have a minimal effect.
As shown in Figure 6B, the edge with the lowest connectivity in the open-pit mining environmental geological disaster chain network is V8 → V30. This indicates that the removal of the edge representing road disruption → traffic obstruction leads to the interruption of specific hazardous events that would otherwise occur in the original disaster chain network, suggesting that this edge influences the development of subsequent disaster nodes. Additionally, there are 7 edges in the network with a connectivity of 1, suggesting that these edges provide the disaster network with greater resilience and elasticity. Consequently, performing disconnection and disaster reduction from these 7 edges would have little effect. In contrast, the edge V8→V30 forms a relatively isolated chain-like structure with high vulnerability compared to the other 71 edges with higher connectivity. Therefore, disconnection and disaster reduction can be conducted from this edge.
As shown in Figure 6C, after removing the edge V5 → V28, the network’s average path length increases to 2.191. This indicates that bypassing the edge soil pollution → land degradation in the disaster propagation process from the source event to the final event increases the disaster propagation path length. It suggests that cutting this edge can effectively reduce the propagation speed of the disaster from the source event to the resulting disaster event, thus delaying the evolution of the disaster and providing additional time for rescue and mitigation efforts. In contrast, removing the edge V26 → V27 results in the smallest average path length of 1.962, indicating that the removal of the edge soil erosion → degradation of soil function has the least impact on the speed of disaster propagation in the network. Breaking this edge does not effectively control the environmental geological disasters in the open-pit mine.
As shown in Figures 6D, 7, the vulnerabilities of edges V8→V30, V25→V45, V13→V26, V26→V27, and V9→V31 have attained the extremely high vulnerability level. Specifically, the vulnerability of edge V8→V30 is 41.614, while the vulnerabilities of the other four edges are all above 30, namely, 36.972, 34.322, 34.132, and 32.181, respectively. This indicates that these five edges pose an extremely high risk within the network. Cutting these edges to break the chains and sever their connection to subsequent disaster events has a significant impact on the evolution of the network structure of environmental geological disasters in open-pit mines and is a critical aspect of disaster risk control. In the evolution process of the environmental geological disaster chain network in open-pit mines, there are five edges with extremely high vulnerability, for which immediate chain-breaking disaster reduction measures are required. There are six edges with high vulnerability that should receive close attention and be monitored and prevented. There are 21 edges with medium vulnerability, which can be addressed with additional measures during comprehensive management. There are 39 edges with low vulnerability, where chain-breaking disaster reduction is not urgent. Overall, the majority of the edges are categorized as low vulnerability. Therefore, the focus of disconnection and disaster mitigation efforts should be on the vulnerable links constituted by the extremely high vulnerability, high vulnerability, and medium vulnerability edges.
As observed in Figure 7, two complete vulnerable links are formed by edges categorized as having extremely high vulnerability, high vulnerability, and medium vulnerability, respectively, connecting the source disaster event to the ultimate disaster event. The detailed results are depicted in Figure 8. These two complete vulnerable links represent the two primary paths of disaster transmission. The first path is a closed-loop transmission system triggered by slag accumulation disasters. In this system, slag accumulation can lead to water pollution, slag spontaneous combustion, and Soil pollution. Slag contains flammable substances like coal gangue, which is prone to spontaneous combustion, causing fires and explosions that further pollute the environment. Additionally, heavy metals and inorganic salts in the slag infiltrate the soil, causing soil pollution, damaging the vegetation and land in the mining area and the city, influencing the growth of crops, and incurring property losses. The Vegetation destruction exacerbates soil erosion, leading to degradation of soil function and the formation of a fragile disaster transmission chain. This represents a critical focus for chain-breaking disaster mitigation efforts and holds a key position in the complex network of environmental geological disasters in open-pit mines. The second path involves geological disasters that are caused by unstable slopes. The exposure of slope rock masses and increased excavation depth can lead to geological disasters such as landslides and ground fissures, which subsequently damage the drainage system in the mining area and cause water accumulation on roads. After prolonged and repeated influences, the road foundation is undermined, triggering a series of events including road disruption, road subsidence, and Traffic obstruction, which cause inconvenience to residents’ lives and ultimately disrupt social stability. Therefore, the nodes and connecting edges in these two paths should be the primary focus of disaster mitigation efforts.
Figure 8. Vulnerable links in the evolution network of environmental geological disaster in open-pit mine.
In the context of the evolution network of environmental geological disaster in open-pit mines, timely implementation of disaster mitigation measures targeting vulnerable links can significantly reduce the overall network risk. Thus, by analyzing the edge betweenness, connectivity, average path length, and edge connection vulnerability as discussed in the previous section, it was concluded that the edges V8→V30, V25→V45, V13→V26, V26→V27, and V9→V31 significantly influence the network. In this research, targeted suggestions for network disconnection and disaster reduction are put forward for these five key connection edges.
(1) Enhance road disaster resistance and resilience. Concerning the disaster evolution process of V8 (road disruption) → V30 (traffic obstruction). On the one hand, proactive measures such as backfilling, compacting, and grouting are recommended to address ground fissures and subsidence disasters around open-pit mines. These interventions aim to stabilize the roadbed and elevate the water-stabilized layer of subsided roads, thereby improving the overall structural integrity of the roadways. On the other hand, in the event of a disaster, it is imperative to initiate swift restoration and rescue operations in the affected road areas. Additionally, evacuation of vehicles in congested sections and the implementation of traffic control measures are essential to ensure safety and maintain traffic flow when necessary.
(2) Perfect the social early warning, emergency response and rescue mechanisms. Regarding the disaster evolution process of V25 (inconvenient living conditions) → V45 (disturb social stability). Firstly, it is crucial to enhance the awareness of disaster prevention and mitigation among the entire population. This can be achieved by conducting disaster education and regular geological disaster drills. Secondly, ensuring the availability of emergency supplies and services is essential. In the event of disasters, timely organization of rescue efforts, evacuation and relocation of threatened personnel, provision of emergency shelters for the affected, and psychological counseling are vital. Finally, improving the disaster relief fund system is crucial. This includes providing government assistance, social donations, and a variety of policy-based and commercial insurance systems for the affected people and areas.
(3) Increase the vegetation coverage within and around open-pit mines. Regarding the disaster evolution process of V13 (vegetation destruction) → V26 (soil erosion). Initially, the selection of suitable tree species should be based on a comprehensive assessment of the geological environment, soil conditions, climate temperature, and other relevant factors specific to the open-pit mine. Subsequently, considering the topographic and geological characteristics of the mine, rapid afforestation can be achieved through the use of spray seeding. Particularly in areas such as slopes and other inclined soil regions, prompt vegetation restoration is crucial, with a focus on activities such as water retention and maintenance to ensure the long-term success of the vegetation establishment.
(4) Improve soil quality and restore soil functions. Regarding the disaster evolution process of V26 (soil erosion) → V27 (degradation of soil function). Soil erosion and the decline in soil functions, resulting from the accumulation of mine waste around open-pit mines, are significant causes of surface ecological disruption. Therefore, measures such as soil covering, sealing, tamping, and greenery planting can be conducted on waste piles to improve soil quality and achieve ecological governance of solid waste. Additionally, sound ecological planning should be implemented to gradually harmonize the treated area with the adjacent ecological environment and restore its ecological functions.
(5) Enhance the disaster resilience of urban pipeline infrastructure. Regarding the disaster evolution process of V9 (drainage pipe rupture) → V31 (gas outage). Firstly, for the open-pit mine slopes prone to geological disasters, physical measures such as backfilling and footing, anti-slide piles, and slope reduction and load reduction for unstable slopes are implemented. Additionally, technical disaster reduction measures such as the establishment of early warning and forecasting mechanisms and real-time monitoring systems are adopted. Secondly, strict quality control is exercised over the pipeline equipment and materials during the design and construction process to ensure durability and reliability. Finally, in the event of disasters, immediate actions are taken to repair damaged drainage public facilities, control the collapse area, seal dangerous places, demarcate a warning area, implement traffic control and other necessary measures, and evacuate, disperse, and properly resettle threatened personnel.
The chain-breaking disaster reduction measures outlined above are technically feasible but face some implementation challenges. For instance, in enhancing the disaster resilience of roads and pipeline infrastructure, the Fushun West Open-pit Mine has employed measures such as backfilling, slope reduction, and grouting to improve the disaster resistance of roads and other infrastructure. However, these measures pose different technical difficulties and key points under various geological conditions, requiring tailored adjustments and optimization according to local conditions. Regarding ecological restoration and soil quality enhancement, Fushun West Open-pit Mine has improved the regional ecological environment quality through vegetation planting and soil remediation. Nevertheless, ecological restoration demands long-term maintenance and management. Despite the challenges, the benefits brought about by these measures are remarkable. For instance, through backfilling and soil covering as well as ecological restoration, the ecological restoration area of West Open-pit Mine has reached 432.75 ha, facilitating the sustainable development of the ecosystem in Fushun City. Additionally, by enhancing the disaster resistance of infrastructure such as roads, the influence of disasters on the surrounding residents and the urban economic and social system has been mitigated, promoting the transformation of mining cities. For example, the Fushun Coal Mine Museum has now become a tourist hotspot, driving the development of the local tourism industry.
Based on the theory of disaster systems, this study constructs a network evolution model for environmental geological disaster chains in open-pit mines and analyzes it using complex network theory. The following conclusions are drawn.
(1) A network evolution model for environmental geological disaster chains in open-pit mines was constructed. Based on the theory of disaster systems, a complex network evolution model consisting of 45 events and 71 edges was constructed to simulate the evolution process of environmental geological disaster chains in open-pit mines. This model offers a novel perspective for understanding and predicting the propagation paths of environmental geological disaster chains.
(2) Identify the key nodes and vulnerable connection edges of the environmental geological disaster chain network in open-pit mines. Using complex network analysis methods and network characteristic indicators, the key nodes (road disruption, inconvenient living conditions and water pollution) and key edges (road disruption → traffic obstruction, inconvenient living conditions → disturb social stability, vegetation destruction → soil erosion, soil erosion → degradation of soil function, drainage pipe rupture → poor drainage) in the disaster chain were identified. The identification of these key factors provides clear targets for disaster prevention and control, facilitating the targeted formulation of disaster management strategies.
(3) Propose risk control measures and chain-breaking disaster reduction strategies for environmental geological disasters in open-pit mines. Targeted risk control and chain-breaking disaster reduction measures have been proposed for key nodes and vulnerable connection edges, including enhancing the disaster resilience of roads, improving the social early warning system, and increasing vegetation coverage, etc. These measures offer significant practical value and provide a scientific basis for the prevention and control of environmental geological disasters in open-pit mines.
This research integrates the theory of disaster systems with the theory of complex networks to explore the evolutionary process of disaster chains in open-pit mines via network structure. It establishes systematic connections between the source disaster events in the environmental geological disasters of open-pit mines and the ultimate disaster events impacting surrounding communities, urban socio-economic systems, and urban ecosystems. By analyzing the interactions and cumulative effects among the disasters in open-pit mines, it offers a novel perspective for the application of the disaster system theory under complex environmental geological conditions. Through identifying the key links in the disaster chain, the study proposes mitigation measures to break the chain, which can help mining companies take more targeted disaster prevention actions and ensure the safety of surrounding residents. Additionally, it aids in understanding the relationship between environmental geological disasters in open-pit mines and urban ecology and socio-economic systems during the transformation process of resource-based cities, providing valuable insights for the formulation and implementation of mining policies in practice.
The evolution mechanism of environmental geological disasters in open-pit mines are complex and variable, involving multiple factors and interactions. Future research should be more targeted and comprehensive. For example, it could focus on specific types of mines (such as coal mines and metal mines) to investigate their unique evolution mechanisms of environmental geological disasters, thereby compensating for the deficiency of this study which merely covers common disaster events. Furthermore, the environmental geological disaster chain network established in this paper is an unweighted directed network, merely reflecting the unidirectional causal relationship of disaster events, without taking into account the differences in the degree of influence between events. Future studies could introduce a weighted directed network, for example, by determining the weights of transmission effects between nodes, to better reflect the intensity of interactions between different disasters. This would allow for more accurate predictions of the disaster chain evolution process, providing more targeted theoretical support for the formulation of disaster prevention and control strategies.
The original contributions presented in the study are included in the article/supplementary material, further inquiries can be directed to the corresponding author.
DF: Conceptualization, Project administration, Writing–review and editing. JZ: Methodology, Writing–original draft. JW: Writing–review and editing.
The author(s) declare that financial support was received for the research, authorship, and/or publication of this article. This research was supported by the key project of the Ministry of Science and Technology of China (2017YFC1503102).
Author JW was employed by Henan railway construction investment in southeastern Henan Construction Investment Co., Ltd.
The remaining authors declare that the research was conducted in the absence of any commercial or financial relationships that could be construed as a potential conflict of interest.
The authors declare that no Generative AI was used in the creation of this manuscript.
All claims expressed in this article are solely those of the authors and do not necessarily represent those of their affiliated organizations, or those of the publisher, the editors and the reviewers. Any product that may be evaluated in this article, or claim that may be made by its manufacturer, is not guaranteed or endorsed by the publisher.
Alizadeh, S., Pósfai, M., and Ghasemi, A. (2023). Input node placement restricting the longest control chain in controllability of complex networks. Sci. Rep-Uk 13, 3752. doi:10.1038/s41598-023-30810-w
Bernal, G., Salgado-Gálvez, M., Zuloaga Romero, D., Tristancho, J., González, D., and Cardona, O. (2017). Integration of probabilistic and multi-hazard risk assessment within urban development planning and emergency preparedness and response: application to manizales, Colombia. Int. J. Disast. Risk Sci. 8, 270–283. doi:10.1007/s13753-017-0135-8
Chen, H., Zhang, L., and Ran, L. (2021). Vulnerability modeling and assessment in urban transit systems considering disaster chains: a weighted complex network approach. Int. J. Disast. Risk Re 54, 102033. doi:10.1016/j.ijdrr.2020.102033
Chen, J., and Yin, T. (2023). Transmission mechanism of Post-COVID-19 emergency supply chain based on complex network: an improved SIR model. Sustainability 15, 3059. doi:10.3390/su15043059
Di Maio, F., Marchetti, S., and Zio, E. (2023). Robust multi-objective optimization of safety barriers performance parameters for NaTech scenarios risk assessment and management. Reliab. Eng. Syst. Safe. 235, 109245. doi:10.1016/j.ress.2023.109245
Elangbam, G., and Manglem, A. (2024). Disrict level climate vulnerability assessment of Manipur. Decc. Geogr. 62, 236–247.
Fang, K., Jia, S., Tang, H., Zhou, R., Kong, Z., Fu, Y., et al. (2024). Arching effect in slopes under excavation: classification and features. Eng. Geol. 337, 107563. doi:10.1016/j.enggeo.2024.107563
Fang, K., Miao, M., Tang, H., Jia, S., Dong, A., An, P., et al. (2023). Insights into the deformation and failure characteristic of a slope due to excavation through multi-field monitoring: a model test. Acta Geotech. 18, 1001–1024. doi:10.1007/s11440-022-01627-0
Feng, D., Bai, G., and Wang, L. (2024). Influence of large open-pit mines on the construction and optimization of urban ecological networks: a case study of Fushun City, China. PloS One 19, e0303016. doi:10.1371/journal.pone.0303016
Gao, F., Gao, X., Yang, C., and Li, J. (2024). Research on the evolution network model of the landslide disaster chain: a case study of the Baige landslide. Appl. Sci. 14, 499. doi:10.3390/app14020499
Gill, J., and Malamud, B. (2014). Reviewing and visualizing the interactions of natural hazards. Rev. Geophys. 52, 680–722. doi:10.1002/2013RG000445
Han, L., Zhang, J., Zhang, Y., Ma, Q., Alu, S., and Lang, Q. (2019). Hazard assessment of earthquake disaster chains based on a bayesian network model and ArcGIS. ISPRS Int. J. Geo-Inf. 8, 210. doi:10.3390/ijgi8050210
Hao, J., Liu, L., Long, Z., Chu, Y., Zhang, D., Chen, X., et al. (2023). Scenario deduction of Natech accident based on dynamic Bayesian network: a case study of landslide accident in a liquor storage tank area in Guizhou Province, China. J. Loss Preven. Proc. 83, 105067. doi:10.1016/j.jlp.2023.105067
Hao, T., Zheng, X., Wang, H., Xu, K., and Yu, Y. (2022). Development of a method for weight determination of disaster-causing factors and quantitative risk assessment for tailings dams based on causal coupling relationships. Stoch. Env. Res. Risk A 37, 749–775. doi:10.1007/s00477-022-02316-w
He, B., Li, C., Yao, Q., Wang, H., Luo, L., and Ma, M. (2024). The disaster-causing factors of the flash floods for the July 20th extreme rainstorm in Henan, China. Front. Earth Sci. 12, 1438421. doi:10.3389/feart.2024.1438421
Hu, Z., Wu, G., Wu, H., and Zhang, L. (2022). Cross-sectoral preparedness and mitigation for networked typhoon disasters with cascading effects. Urban Clim. 42, 101140. doi:10.1016/j.uclim.2022.101140
Jiang, Y., and Yue, L. (2021). Identification of key nodes in urban subway disaster network based on EDK algorithm. IOP Conf. Ser. Mat. Sci. Eng. 769, 032025. doi:10.1088/1755-1315/769/3/032025
Li, G., Hu, Z., Wang, D., Wang, L., Wang, Y., Zhao, L., et al. (2024). Instability mechanisms of slope in open-pit coal mines: from physical and numerical modeling. Int. J. Min. Sci. Techno. 34, 1509–1528. doi:10.1016/j.ijmst.2024.10.003
Li, H. R., Wang, Z. H., Yang, Q. F., Zhang, Y. J., and Ouyang, Z. L. (2021). Evolutionary model and risk analysis of metro disaster chain undercomplex network. China. Saf. Sci. J. 31, 141–147. doi:10.16265/j.cnki.issn1003-3033.2021.11.020
Li, W., Lu, X., Wu, R., and Wang, H. (2023a). Evolution analysis of research on disaster-causing mechanism and prevention technology of mine goaf disaster. Environ. Sci. Pollut. R. 30, 93388–93406. doi:10.1007/s11356-023-29170-9
Li, X., Chen, G., Amyotte, P., Alauddin, M., and Khan, F. (2023b). Modeling and analysis of domino effect in petrochemical storage tank farms under the synergistic effect of explosion and fire. Process Saf. Environ. 176, 706–715. doi:10.1016/j.psep.2023.06.054
Loupasakis, C. (2020). Contradictive mining–induced geocatastrophic events at open pit coal mines: the case of Amintaio coal mine, West Macedonia, Greece. Arab. J. Geosci. 13, 582. doi:10.1007/s12517-020-05633-y
Luo, J. H., Lin, X. S., Mou, F. Y., Li, H. W., and Zhang, L. (2020). Static risk analysis on rainstorm-agricultural disaster chain inmountainous areas based on complex network. China. Saf. Sci. J. 30, 163–170. doi:10.16265/j.cnki.issn1003-3033.2020.03.025
Menoni, S. (2001). Chains of damages and failures in a metropolitan environment: some observations on the Kobe earthquake in 1995. J. Hazard. Mat. 86, 101–119. doi:10.1016/S0304-3894(01)00257-6
Mizrahi, S. (2020). Cascading disasters, information cascades and continuous time models of domino effects. Int. J. Disast. Risk Re. 49, 101672. doi:10.1016/j.ijdrr.2020.101672
Niu, X., Hou, K., and Sun, H. (2023). Study on the prevention and control of downhole debris flows based on disaster chain theory. Water 15, 2367. doi:10.3390/w15132367
Ntambara, B., and Umuhoza, R. (2022). Optimization of Rwanda power system protection in power blackouts and cascading events. SN Appl. Sci. 4, 296. doi:10.1007/s42452-022-05188-5
O'reilly, G. J., Shahnazaryan, D., Dubini, P., Brunesi, E., Rosti, A., Dacarro, F., et al. (2023). Risk-aware navigation in industrial plants at risk of NaTech accidents. Int. J. Disast. Risk Re 88, 103620. doi:10.1016/j.ijdrr.2023.103620
Poulomi, G., Ning, L., and Dadiyorto, W. (2022). Editorial: multivariate extremes and compound, interconnected and cascading events: understanding the past and projections into the future. Front. Water 4, 1052694. doi:10.3389/frwa.2022.1052694
Qi, Q., Yan, B., Liu, W., Zhang, B., and Liu, J. (2022). Study on the mechanism and chain generation relationship of geological disaster secondary coal mine accidents. Sustainability 14, 15425. doi:10.3390/su142215425
Ricci, F., Casson, M. V., and Cozzani, V. (2023). Natech accidents triggered by cold waves. Process Saf. Environ. 173, 106–119. doi:10.1016/j.psep.2023.03.022
Salvoni, M., and Dight, P. M. (2016). Rock damage assessment in a large unstable slope from microseismic monitoring - MMG Century mine (Queensland, Australia) case study. Eng. Geol. 210, 45–56. doi:10.1016/j.enggeo.2016.06.002
Shao, Y., Chen, S., Wang, Q., Liu, P., Yu, S., and Li, J. (2023). Disaster chain evolution and risk analysis of enderground tungsten mine induced by rainstorm and flood. Nonferrous Met. Sci. Eng. 7, 1–10. doi:10.13264/j.cnki.ysjskx.2024.05.015
Shi, P. J. (1996). Theory and practice of disaster study. J. Natl. Disast. 05, 8–19. doi:10.13577/j.jnd.1996.0402
Song, Y. H., Zhang, X. Y., and Lv, W. (2020). Construction of disaster chain network model and risk analysis onwildland-urban interface fire. J. Saf. Sci. Technol. 16, 122–128. doi:10.11731/j.issn.1673-193x.2020.05.019
Su, Y., Rong, G., Ma, Y., Chi, J., Liu, X., Zhang, J., et al. (2022). Hazard assessment of earthquake disaster chains based on deep learning—a case study of Mao County, Sichuan Province. Front. Earth Sci. 9, 683903. doi:10.3389/feart.2021.683903
Sutrisno, D., Suwarno, Y., Widodo, A., Cipta, A., Setyanta, B., Darmawan, M., et al. (2022). Cascading disasters triggered by earthquake hazards: an infrastructure resilience approach. IOP Conf. Ser. Mat. Sci. Eng. 1109, 012003. doi:10.1088/1755-1315/1109/1/012003
Tang, P., Zhong, W., Wen, J., Shao, S., Zhou, D., and Huang, S. (2023). Developing and understanding cascading effects scenario of typhoons in coastal mega-cities from system perspectives for disaster risk reduction: a case study of shenzhen, China. Int. J. Disast. Risk Re 92, 103691. doi:10.1016/j.ijdrr.2023.103691
Triyanti, A., Surtiari, G. a. K., Lassa, J., Rafliana, I., Hanifa, N. R., Muhidin, M. I., et al. (2023). Governing systemic and cascading disaster risk in Indonesia: where do we stand and future outlook. Disaster Prev. Manag. 32, 27–48. doi:10.1108/DPM-07-2022-0156
Wang, F., Yang, Y., and Wu, L. (2023). Research on the functional relationship among historical disasters, disaster bearing bodies and disaster reduction capacity in China. J. Emerg. Manag. Disaster Commun. 04 (04), 143–152. doi:10.1142/S2689980923500094
Wang, J., Zhang, Q., Yin, W., Qi, S., Gao, D., and Ma, D. (2021). On-site measurement on compaction characteristics of coal gangue and surface subsidence disaster in deep Backfilling Mining. Front. Earth Sci. 9, 724476. doi:10.3389/feart.2021.724476
Wang, L., Xu, Y., Chen, Q., Wu, J., Luo, J., Li, X., et al. (2024). Research on remote-sensing identification method of typical disaster-bearing body based on deep learning and spatial constraint strategy. Remote Sens-Basel 16, 1161. doi:10.3390/rs16071161
Wang, Q., and Hou, J. (2023). Hazard assessment of rainstorm-geohazard disaster chain based on multiple scenarios. Nat. Hazards 118, 589–610. doi:10.1007/s11069-023-06020-y
Wang, S., Zhou, W., Cai, Q., Shi, X., Lu, X., and Luan, B. (2019). The coal mining model under slippery slope in Yiminhe Open Pit coal Mines. Geotech. Geolo. Eng. 37, 3727–3737. doi:10.1007/s10706-019-00864-7
Wen, C. J. (2000). Generalized disaster, disaster chain and their prevention and control. J. Catastr. 15, 14–19. doi:10.3969/j.issn.1000-811X.2000.04.003
Xu, M., Wang, Z., Qi, L., Liu, L., and Zhang, K. (2011). Disaster chains initiated by the Wenchuan earthquake. Environ. Earth Sci. 65, 975–985. doi:10.1007/s12665-011-0905-3
Xu, Z. D., Xiaojiang, L., Wei, X., Bin, S., Xuanya, L., and Dajun, X. (2022). Analysis on the disaster chain evolution from gas leak to explosion in urban utility tunnels. Eng. Fail. Anal. 140, 106609. doi:10.1016/j.engfailanal.2022.106609
Yan, B., Liu, J., Qi, Q., Liu, W., and Li, X. (2022). Study on chain relationship and risk assessment model of coal mine geological disasters. Arab. J. Geosci. 15, 900. doi:10.1007/s12517-022-10184-5
Yang, C. R., L, Y. F., Zou, X., Tan, Y., and Fan, H. H. (2020). Risk assessment of the entire process of railway collapse-landslide-mudslide disaster chain in difficult mountainous areas based onComplex network. Railw. Stand. Des. 64, 47–51. doi:10.13238/j.issn.1004-2954.201908180003
Zhao, Y., and Tian, S. (2021). Identification of hidden disaster causing factors in coal mine based on Naive Bayes algorithm. J. Intell. Fuzzy Syst. 41, 2823–2831. doi:10.3233/JIFS-202726
Zhou, B., Yan, X., Lv, Y., and Xuan, Q. (2023). Adversarial attacks on clustering coefficient in complex networks. IEEE T. Circuits-II 71, 2199–2203. doi:10.1109/TCSII.2023.3337896
Keywords: open-pit mine, environmental geological disaster, disaster chain, complex network, disaster-forming mechanism, risk analysis
Citation: Feng D, Zhang J and Wang J (2025) Evolutionary model and risk analysis of environmental geological disaster chain in open-pit mine under complex network. Front. Earth Sci. 13:1542855. doi: 10.3389/feart.2025.1542855
Received: 11 December 2024; Accepted: 17 February 2025;
Published: 11 March 2025.
Edited by:
Weile Li, Chengdu University of Technology, ChinaReviewed by:
Han Du, Tsinghua University, ChinaCopyright © 2025 Feng, Zhang and Wang. This is an open-access article distributed under the terms of the Creative Commons Attribution License (CC BY). The use, distribution or reproduction in other forums is permitted, provided the original author(s) and the copyright owner(s) are credited and that the original publication in this journal is cited, in accordance with accepted academic practice. No use, distribution or reproduction is permitted which does not comply with these terms.
*Correspondence: Jing Zhang, emhhbmdqaW5nbG50dUAxNjMuY29t
Disclaimer: All claims expressed in this article are solely those of the authors and do not necessarily represent those of their affiliated organizations, or those of the publisher, the editors and the reviewers. Any product that may be evaluated in this article or claim that may be made by its manufacturer is not guaranteed or endorsed by the publisher.
Research integrity at Frontiers
Learn more about the work of our research integrity team to safeguard the quality of each article we publish.