- 1Geological Studies Unit, Indian Statistical Institute, Kolkata, India
- 2Department of Earth Sciences, Pondicherry University, Pondicherry, India
In recent years, deposits of deep channelized fluvial systems have been documented in some pre-Silurian successions, casting doubts on the exclusive sheet-braided models of pre-vegetational fluvial sedimentation. In this study, we explore the Neoproterozoic Ramgiri Formation to (i) understand the surface processes in a pre-vegetation fluvial system, (ii) examine the existence of sheet-braided or channelized architecture in this succession, and (iii) identify possible process controls that might have resulted in either a sheet braided or channelized architecture in these deposits. This study examines a ∼50 km-long transect of the outcrop belt, subdividing the succession into ten facies and three facies associations, supported by sedimentological logs and paleocurrent data. Facies association I consists of sheet-like units of weakly channelized, stratified pebble-coble conglomerate alternating with trough cross-stratified pebbly sandstone. Facies association II consists of comparatively finer, pebbly, coarse-grained, sheet sandstones with small trough cross-strata, capped by thin mudstones. Both associations show an abundance of dm-thick, mass flow or hyperconcentrated flow deposits. The regional paleocurrent patterns of these two associations are dominantly NE-trending, transverse to the basin axis, representing proximal (FA I) and distal (FA II) megafan deposits. Facies association III, in contrast, contains >3 m deep channel scours, thick simple and compound bedforms, and bars. The overall paleoflow of FA III is toward the SE, parallel to the basin axis, and represents deposition from a lower-gradient, perennial, sandy braided river. We infer that the flows were sheet-like on the megafan because of its steeper gradient, resulting in flow attenuation and widening on a non-cohesive substrate, whereas the lower-gradient, higher-discharge axial drainage of FA III gave rise to deeper channel bedforms similar to the Phanerozoic fluvial deposit. The geophysical and tectonic data indicate that the Godavari basin developed as a half-graben with an active normal fault on its NE margin and a roll-over anticline on the SW margin. The Ramgiri megafans developed on the roll-over margin, and the adjacent low-gradient axial basin plain was occupied by deeper channels, resulting in the simultaneous development of contrasting fluvial architectures.
1 Introduction
Vegetation appears to exert a major influence on the fluvial processes by moderating the flood hydrograph, enhancing substrate cohesiveness, controlling sediment availability, and contributing to soil development (Davies and Gibling, 2010; Davies et al., 2011 and references therein). Precambrian fluvial processes, therefore, are inferred to have been different due to the absence of land vegetation (Schumm, 1968). Theoretical postulates and many case studies document the shallow and wide nature of the pre-vegetation rivers, which produced sheet-like sandstone bodies. These formations are inferred to have resulted from the unconfined, shallow flow of the channels that rapidly widened by eroding the non-cohesive substrate (Fuller, 1985; Sonderholm and Trisgaard, 1998; Long, 2004; Eriksson et al., 2005; for a review, refer to Davies and Gibling, 2010). The term “sheet-braided” has been coined to describe these typical fluvial deposits (Cotter, 1978; Eriksson et al., 2006). The overall depositional architecture and inferred channel dynamics have influenced many researchers to compare the inferred pre-vegetation surface processes with the dryland rivers, which are marked by the limited vegetation cover and the ephemeral, flashy nature of flood discharge (Sneh, 1983; Abdullatif, 1989; Tooth, 2000).
It is argued that pre-vegetational earth surface processes were distinct from the fluvial systems that developed after the Silurian, following the advent of land vegetation (Schumm, 1968; Cotter, 1978; Davies and Gibling, 2010). The absence of land vegetation probably resulted in reduced chemical weathering, which, in turn, led to limited production of mud and soils (Retallack, 2001). In addition, in the absence of moderating effects of vegetation, surface flows were flashy, marked by stronger and more frequent discharge fluctuations, irrespective of climatic conditions. In the absence of muddy, cohesive floodplain deposit, the channels were prone to rapid avulsion, rapid lateral expansion, and the transport of high sediment loads (Davies et al., 2011; Santos et al., 2014; Eriksson et al., 2006). It has been documented in several cases that such alluvial plain deposits were subjected to increased eolian reworking and deposition (Chakraborty and Chaudhuri, 1993; Trewin, 1993; Trisgaard and Oxnevad, 1998). Thus, many Precambrian and early Paleozoic fluvial deposits are characterized by stacked sheet sandstone bodies, typically consisting of decimeter-thick, trough cross-bedded units that lack internal complexity and show little evidence of deep channelization (Cotter, 1978; Davies and Gibling, 2010; Davies et al., 2011; Santos et al., 2014; Ielpi and Rainbird, 2016). However, the recent findings of strongly sinuous channels on the surface of Mars challenge the idea that vegetation is the sole factor influencing channel planform patterns, and this has sparked renewed interest in possible analogs on the earth, particularly in Precambrian fluvial successions (Beyer et al., 2012; Santos et al., 2014; Matsubara et al., 2015; Ielpi and Rainbird, 2016; Ielpi et al., 2017). Several studies have already reported meandering river deposits from the Precambrian strata (Sweet, 1988; Santos and Owen, 2016). It appears that bank stabilization is an important factor for developing river meanders, but the same can be provided by the abundance of muddy sediments in the channels or by early cementation of the channel-enclosing sediments (Marconato et al., 2014; Matsubara et al., 2015; Braudrick et al., 2009). Recently, studies of large-scale outcrops of Precambrian fluvial successions involving satellite images and oblique aerial photos, integrated using photogrammetric techniques, revealed the presence of very wide and deep channels within several Proterozoic fluvial sandstone bodies (Ielpi and Rainbird, 2016; Ielpi et al., 2017; Ghinassi and Ielpi, 2017). The evidence of deep channelization and the presence of large downstream and laterally accreting bars point to the morphodynamic similarity of many Precambrian rivers with post-land-vegetation rivers. However, it is important to recognize that notwithstanding the record of deep channeled sandstone, more than half of these fluvial deposits (e.g., Burnside River Formation, Canada) are typically cross-stratified ‘sheet-braided’ sandstone units, which are traditionally believed to be the hallmark of pre-vegetation alluvial facies (Ielpi and Rainbird, 2016). Thus, the search for a distinctive style of the pre-vegetation fluvial system remains relevant, and this underscores the need for more case studies for the reconciliation of the effects of the absence of vegetation on the surface processes and their record in the pre-Silurian fluvial deposits. In this perspective, this study explores the well-exposed fluvial strata of the Neoproterozoic Ramgiri Formation of the Sullavai Group in the southwestern Proterozoic belt of the Pranhita–Godavari Valley in Telangana, India (Figure 1).
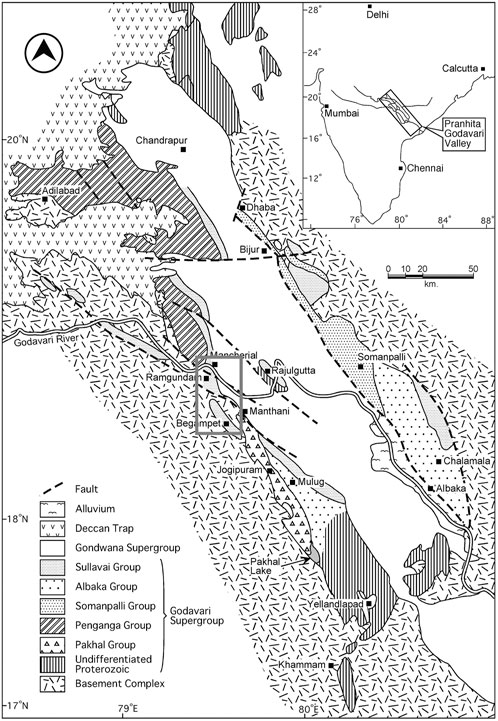
Figure 1. Geological map of the Pranhita–Godavari Valley showing the disposition of different stratigraphic units. The gray-colored box shows the study area. The inset map shows the position of the Pranhita–Godavari Valley in India.
The primary objective of this work is to explore the facies and architecture of the Ramgiri rocks, spread over ∼50 km of the strike length of the exposure belt. We analyze the regional distribution of facies and the paleocurrent pattern of the Ramgiri fluvial deposits to explore the relationship between the Ramgiri drainage network and the paleogeomorphology of the Godavari Valley half-graben basin. Additionally, we present a brief comparison of the architectural characteristics of the Ramgiri Formation with those of the overlying Neoproterozoic fluvial deposits of the Mancheral Quartzite (Chakraborty and Chaudhuri, 1993; Chakraborty, 1994; Chakraborty, 1999), which are exposed in the adjacent areas.
2 Geologic setting
Undeformed or mildly deformed, unmetamorphosed, and unfossiliferous Proterozoic deposits occur in several Precambrian sedimentary basins of India and are collectively referred to as Purana Basins in Indian stratigraphy (Ramkrishna and Vaidyanathan, 2010; Chaudhuri, 2003; Conrad et al., 2011; Meert and Pandit, 2015; Valdiya, 2016; Saha et al., 2016). One such succession, the Godavari Supergroup, spans from the Mesoproterozoic to Neoproterozoic and is exposed as two parallel NW–SE trending belts along the Pranhita–Godavari Valley (P–G Valley). These belts are separated by a central zone of Permo-Triassic rocks belonging to the Gondwana Supergroup (Chaudhuri and Chanda, 1991; Chaudhuri et al., 1999; Chakraborty, 1994; Saha and Chaudhuri, 2003; Joy et al., 2015; Figure 1). The present study is focused on the southwestern outcrop belt of the P–G Valley, where the Proterozoic succession has been subdivided into several groups, with the Sullavai Group being the youngest (Table 1). The Ramgiri Formation forms the basal unit of this group and erosively overlies the sediments of the Penganga Group (around the Mancheral town; 18o52′17″N, 79o 26′ 40″E) or the Pakhal Group (in the Ramagundam area; 18°48′ N, 79°27′ E). In other sections, it directly overlies the Archean basement gneisses (Chakraborty, 1994). The Mancheral Quartzite erosively overlies the Ramgiri Formation around Mancheral and is, in turn, overlain by the Venkatpur Sandstone (Chakraborty, 1994; Joy et al., 2015; Table 1). More recently, some researchers have classified the laterally extensive arkosic sandstone and conglomerate units—previously defined as the Ramgiri Formation—as Nalla Gutta Sandstone, grouping them with the underlying Proterozoic Penganga Group (Chaudhuri et al., 2012). The stratigraphic relationship between the red, arkosic pebbly sandstone in the Ramagundam area and other units of the Penganga Group remains difficult to evaluate due to faulted juxtaposition of these units, whereas the succession around Mancheral is more consistent with its inclusion within the Sullavai Group (Chakraborty, 1994; Chaudhuri, 2003; Joy et al., 2015).
Based on gravity anomaly studies, the P–G Valley basin has long been identified as a half-graben rift basin (Qureshy et al., 1968; Mishra et al., 1989). This rift basin is believed to have formed along an Archean structural grain (the boundary between the Bhandara and Eastern Dharwar cratonic nuclei) during the Early Proterozoic and has been rejuvenated at different times to accommodate several phases of Proterozoic and Mesozoic sedimentation (Naqvi and Rogers, 1987; Chaudhuri et al., 2012; Conrad et al., 2011). Gravity and magnetic studies suggest the presence of a rift structure with a major fault along the northeastern margin of the basin, which extends over 100 km to the north, below the Deccan Trap, and the maximum sediment thickness reaches approximately 6 km close to the northeastern faulted margin (Figure 1). The southwestern limb of the basin appears to be a passive, unfaulted margin characterized by a rollover anticline (cf. Qureshy et al., 1968; Chaudhuri et al., 2002; cf., Withjack et al., 2002).
The Ramgiri Formation consists of a succession of purple to red arkosic pebbly sandstone and conglomerate, with minor amounts of mudstone, and its thickness varies from 250 to more than 500 m. It is very well-exposed in the Nalla Gutta area (Figure 2) near Ramagundam town and in the Ramgiri Fort hill area (18°34′ N, 79° 33′E). A previously available K–Ar date from the Sullavai Group indicated a depositional age of approximately 871 ± 14 Ma (Chaudhuri and Howard, 1985). More recently, Amarasinghe et al. (2014) and Joy et al. (2015), based on the U–Pb dating of the detrital zircons, provided an estimate of the maximum depositional age for the Mancheral Quartzite and Venkatpur Sandstone, inferred to be younger than 970 Ma and 709 Ma, respectively. This suggests that the basal Sullavai Ramgiri Formation, which underlies the Mancheral Quartzite, has a maximum depositional age of <1000 Ma.
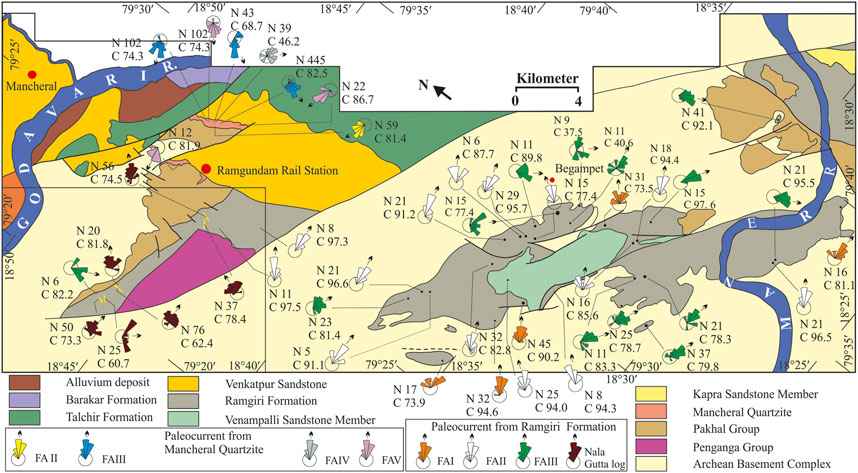
Figure 2. Geological map of the study area showing the paleocurrent data measured from different facies associations of the Ramgiri Formation and Mancheral Quartzite. The rectangular box marked in the lower left corner shows the position of the inset map in Figure 3.
3 Methodology
Previously, Chakraborty (1994) conducted a detailed mapping of approximately 800 square kilometers of the Neoproterozoic Sullavai Group in the southwestern belt of the P–G Valley, covering ∼100 km of strike length between Mancheral in the north and Laknavaram (18° 09ʹ 14.88ʺN, 80° 06ʹ 13.34ʺE) in the south. The current study focuses on the approximately 50 km strike-parallel exposure belt (Figures 2, 3), where the Ramgiri Formation and Mancheral quartzite are well-exposed. Approximately 900 paleocurrent measurements (Figure 2) were taken from the Ramgiri Formation in the study area, and four detailed sedimentological logs (Figure 3) were prepared, covering a cumulative thickness of 967 m, to examine the pattern of vertical succession of facies within the Ramgiri Formation. In each of the logs with conglomerate beds, the long axes of the clasts were measured. The 10 largest clasts were measured from each bed, and its mean was used as the maximum particle size (MPS). In the case of more massive conglomerate beds, the individual bed thicknesses were measured. The succession has been divided into 10 facies, mainly considering lithology and sedimentary structures. These facies, based on their preferred occurrences, paleocurrent patterns, and lithosome geometry, have been grouped into three facies associations. The classification of facies and facies association based on the objective, field-observable criteria, and their process interpretation follows the standard technique of facies analysis, as outlined in many standard texts (cf. Reading, 1996; Walker and Posamentier, 2006; James and Dalrymple, 2010).
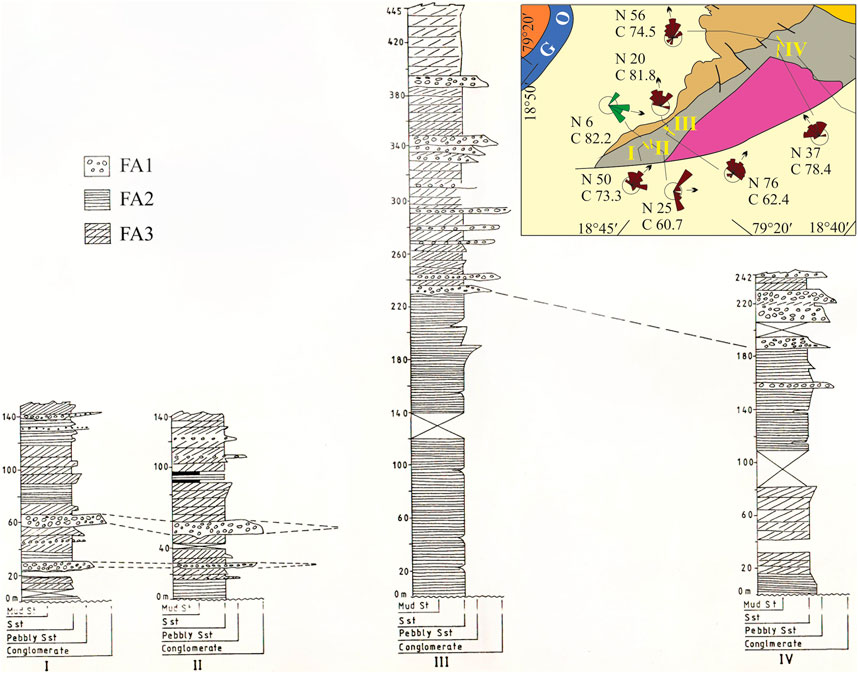
Figure 3. Synoptic sedimentary logs and their correlation in the Nala Gutta area of Ramgiri Formation; four log locations (I–IV) are marked by yellow lines in the inset map.
4 Sedimentology of the Ramgiri Formation
The essential features of the ten facies and three facies associations have been described in Table 2, 3, respectively. The facies associations and their interpretations are further discussed in the following paragraphs:
4.1 Conglomerate–sandstone facies association (FA I)
Facies association 1 (FA I) (Figures 4A, B) comprises the coarsest material of the Ramgiri Formation and mainly consists of F1, F4, F3, and F2 (in decreasing order of abundance) and a few beds of F5 and F8 (Table 2). Clast-supported sandy conglomerates (F1) and trough cross-bedded pebbly sandstones (F4) are the dominant constituents of the association. Interbedding of various types of conglomerates and sandstones builds up 1.6 m–5 m-thick succession, showing both coarsening-upward (CU) (Figure 5B) and fining-upward (FU) trends (Figures 4, 5A). The F1 conglomerates (Table 2) mostly have erosive, slightly undulating lower contacts and are internally horizontal/low-angle-stratified and less commonly cross-stratified (Figures 4, 5A). Although the FA I sandstone–conglomerate beds are sheet-like (W:T>>25), careful observation reveals the presence of irregular scours that are 25–62 cm deep (Figure 4). F2 conglomerate beds (Table 2) are generally massive and have flat, non-erosive bases (Figure 4B). However, some of these massive beds show poorly developed parallel stratification and bed-parallel clast orientation. Their contacts with the overlying sandstones are generally sharp, although gradational contacts are not uncommon. Individual CU/FU units of FA I are tabular bodies and can be traced laterally for several tens to nearly two hundred meters. The stacked FA I conglomerate–sandstone units are 6 m–30 m thick, and they commonly show a coarsening-upward grain-size trend (Figure 5B). Such a lithosome can be traced for approximately a km toward the southeast where it gradually pinches out within FA II or FA III units (Figure 3). A plot of bed thickness versus the maximum particle size of F2 beds (measured from both FA I and FA II units) shows a linear correlation (Figure 5C). Variations in the cross-bed azimuths measured from superposed beds in a vertical succession (log) are high (Figure 5B), and the mean direction of the paleoflow measured from different sections in the Nalla Gutta area shows a radial spread between NNE and ESE (Figure 2). Mean flow directions between exposures in the Begumpet area show less variability, and their mean directions cluster around the NE (Figures 2, 5B). The exposure of FA I and FA II units in Figure 5D is only approximately 100 m wide, and the calculated width-to-thickness ratio (W: T) of some of the channelized sandstone bodies >100. If we could have examined these sandstone bodies in wider exposures, the aspect ratio would have been even greater.
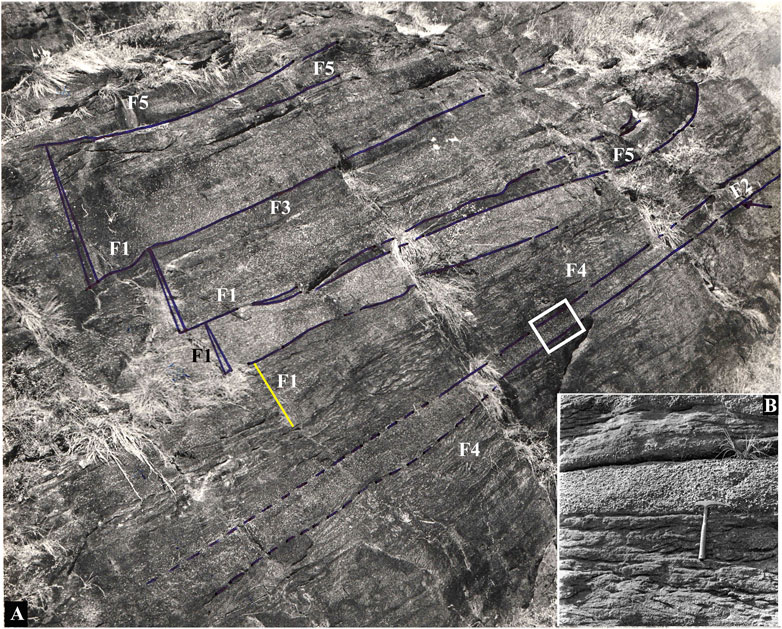
Figure 4. (A) Field photograph of facies association 1 in the Nalla Gutta area. Notably, fining-up F1 conglomerates (marked) mostly have erosive, undulating, lower contacts (inked lines). The cross-bedded sandstone beds (F4) in the lower part of the exposure and finer sediment-filled channels (F5) are marked. Tabular and massive conglomerate beds of F2 (marked) are toward the lower right of the exposure. (B) Enlarged view of the F2 massive conglomerate (box in A) interlayered with trough cross-bedded F4.
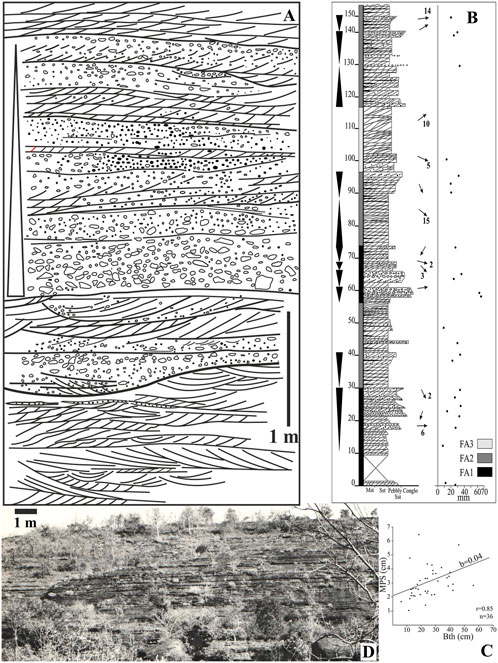
Figure 5. (A) Line drawing of an outcrop showing the grossly fining-upward trend in the conglomerate–sandstone facies association (FA I) in the Nalla Gutta area. Notably, F2 pebble-to-cobble conglomerate beds are massive and have flat, non-erosive bases. (B) Sedimentary logs measured from the Ramgiri Formation (location I marked in Figure 3). Note the coarsening- and fining-upward conglomerate–pebbly sandstone successions. The vector mean of the paleocurrents measured from each level is marked by arrows; dots on the right mark the MPS of the conglomerate beds. (C) Correlation diagram of MPS/BTh data from F2 beds of the Ramgiri Formation; b, regression coefficient (line gradient); n, no. of datasets; r, correlation coefficient. (D) Sheet-braided architecture of the FA I and FA II in the Ramgiri fluvial succession; exposed view is approximately 30 m high.
Interpretation: the clast-supported stratified conglomerate with an erosive base and trough cross-stratified pebbly sandstone is a common feature in modern gravelly alluvium (Hein and Walker, 1977; Bluck, 1974; Bridge, 2003) and ancient braidplain deposits, including many of the pre-vegetational fluvial deposits (Rust, 1984; Simpson and Eriksson, 1989; McCormick and Grotzinger, 1993; Long, 2004; Sohn et al., 1999). Some of the observed beds show a linear correlation of particle size and bed thickness (Figure 5C), probably implying a single plug-flow type emplacement instead of a stacked surge-type succession of mass flow deposits (c.f., Nemec and Steel, 1984; Major, 1997). Some of the features of the F2 conglomerate, such as poorly developed horizontal stratification, bed-parallel orientation of clasts, and the presence of thin sandy lenses within massive conglomerate beds, are more akin to hyperconcentrated flow deposits (Smith, 1986; Todd, 1989; Benvenuti, 2003) common in volcanic terrain or other alluvial settings, where sediment availability is high, leading at times to increased concentration of entrained detritus.
However, there are certain differences between FA I succession and common fluvial facies. The first noticeable difference is in its paleocurrent pattern. In all the six sedimentological logs measured in the Nalla Gutta area (Figures 2, 3), the vertically stacked successions of FA I show vector means varying from ESE to NNE. Although variation is expected between successive channel bodies, the regional paleoslope is not expected to change in a radial fashion from one sandbody to the overlying one. We infer this paleocurrent pattern to represent the radial morphology of the paleogeomorphic units, similar to the alluvial fans or fluvial megafans (Bull, 1977; Blair and McPherson, 2009; Assine, 2005; Chakraborty and Ghosh, 2010; Owen et al., 2017). As the active sedimentation lobe changed with time, the paleocurrent pattern of successively stacked units changed, replicating a radial pattern. Second, there is a paucity of conglomerates originating from mass flow/hyperconcentrated flow. The F2 beds comprise only ∼15% of the total FA I succession. In addition, the overall grain size of the deposits is significantly finer (clast size up to ∼100 mm), indicating a difference with proximal bouldery mass flow-dominated alluvial fan successions. The described features are more consistent with sediment-charged, shallow, gravelly stream flow deposits and stream-driven density flow deposits (Todd, 1989; Went, 2005; Went, 2020). The frequent presence of channel-fill units of F5 denotes the late-stage channelized run-off in a subaerial environment commonly associated with fluvial deposits rather than with mass flow-dominated gravelly deposits of high-gradient alluvial fans (c.f., Blair and McPherson, 1994). These observations form the basis of our interpretation of FA I succession as proximal megafan or fluvial fan deposits (cf. Nichols and Fisher, 2007; Ielpi and Ghinassi, 2016). In these fluvial megafans, traction current was the main depositing agent, and the locus of active deposition shifted within the fan surface rather arbitrarily with time (Assine, 2005; Karssenberg and Bridge, 2008; Chakraborty and Ghosh, 2010). Thinner F2 layers represent occasional hyperconcentration of debris in the stream flow. Tens of meter-scale coarsening- and fining-upward trend in the conglomerate-dominated units reflect the progradation or abandonment of the megafan lobes, as is frequently observed in the proximal part of the fluvial megafan deposits (Chakraborty and Ghosh, 2010; Weissmann et al., 2013).
4.2 Sheet-like pebbly sandstone–thin mudstone facies association (FA II)
Facies association 2 (FA II) (Figures 5B, 6–8) is comparatively finer-grained and dominantly consists of small trough cross-bedded pebbly, coarse sandstone (F3) with a minor amount of massive to faintly stratified pebble or granule conglomerate (F2; Figure 8). Thin mudstone layers (F8), ripple laminated sandstone (F9, Figures 6, 7A), and shallow channel fills (F5, Figure 7B) comprise a minor part of the association. This facies association is common over most of the study area and is characterized by the remarkable lateral continuity of the beds, their sheet-like geometry, the monotonous, repetitive nature of the sequence (Figures 5C, 6), and the regionally consistent northeast to easterly paleoflow direction (Figure 2). The repeated transitions from small cross-bedded pebbly sandstone (F3) to thin mudstone layers (F8) are always sharp (Figure 6). Although individual F3 beds show an FU trend at places (Figure 7A), a tens-of-meter-scale CU grain-size trend is more common in the FA II successions (Figure 5B). Locally, rocks of this facies association show a low-angle intraformational unconformity within the FA III sedimentation units (Chakraborty, 1994). In the Nala Gutta area, it laterally intertongues with FA I deposits.
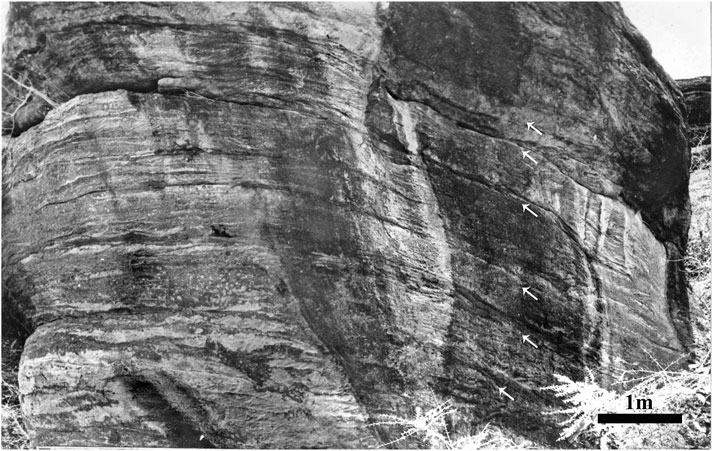
Figure 6. Field photograph of FA II showing sheet-like sandstone of small-trough cross-beds (F3) topped by thin mudstones layers (arrowed).
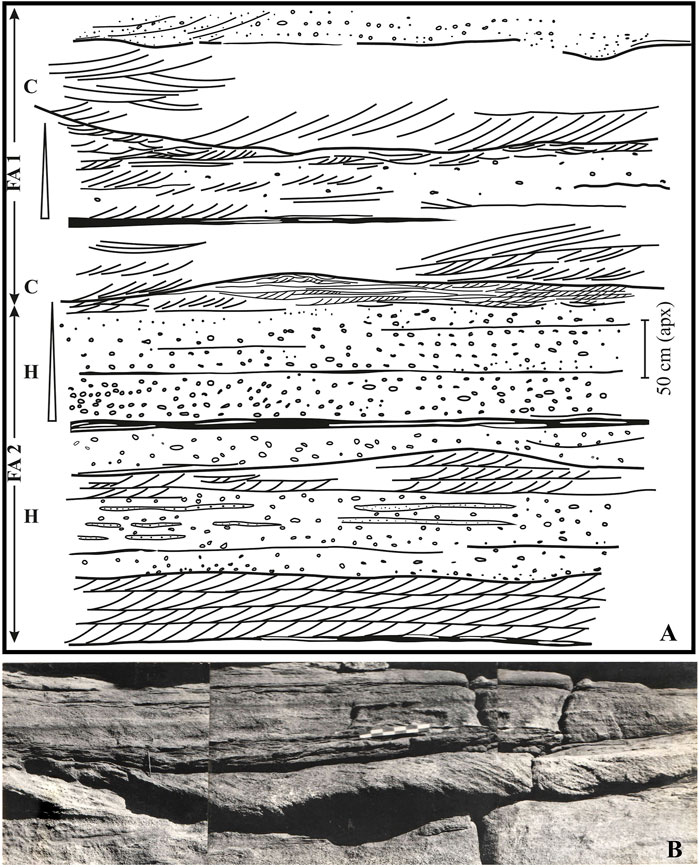
Figure 7. (A) Field sketch of a succession of FA I and FA II in the Nalla Gutta area. Flat-base massive sandy conglomerate with thin interlayered sandstone beds inferred as hyperconcentrated flow deposits. Gradation of hyperconcentrated flow deposits (H) to small cross-beds or ripple laminations; thin mudstone layers separating the beds; FA I beds show larger bedforms and ∼50 cm deep channel fills. (B) Small asymmetrically filled channel with overlying fine muddy siltstone.
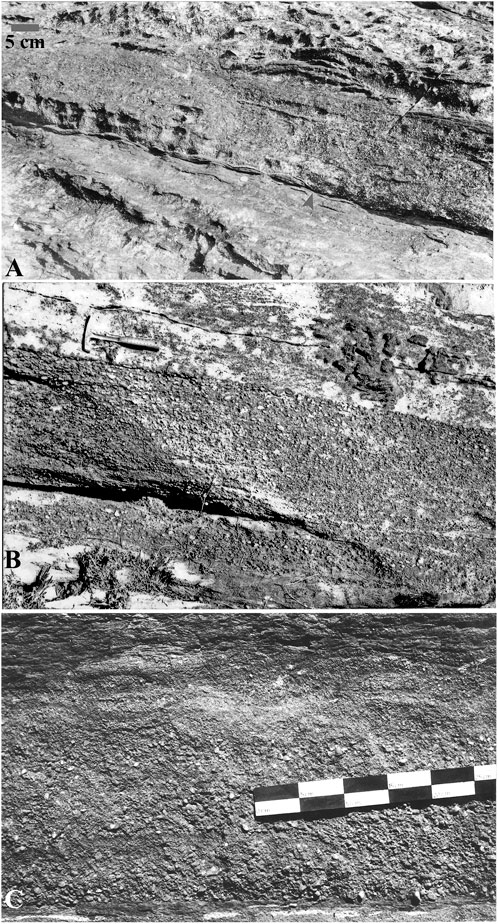
Figure 8. (A) Massive- to parallel-stratified pebbly sandstones preserve ripple forms in the underlying muddy siltstone, indicating non-erosive emplacement of the pebbly sandstone. The pebbly sandstone is inferred as hyperconcentrated flow deposit. (B) Approximately 65 cm thick, massive pebbly conglomerate with faint sand-rich horizontal stratification; clasts are oriented parallel to bed boundaries; cross-bedded sandstone (above) with a sharp contact. (C) Massive fining-upward small pebble conglomerate grading into mudstone.
Lithologically, FA II differs from FA I rocks by having a finer clast size, more frequent thin mudstone beds (F8), and thinner bed packages. Some of the interlayered F2 beds, as mentioned earlier, show a linear correlation of MPS: BTh plots (Figure 5C). The association forms 25–110 cm-thick tabular units that can be traced for greater than several hundred meters in the outcrops (Figure 5C). Trough cross-beds are mostly 3–15 cm thick, and isolated larger planar cross-beds, which occur infrequently, range in thickness from 25 to 42 cm. Shallow, wide channel-fills, up to 25 cm thick, with a width: depth ratio >25:1, occur in certain areas (Figure 7B). The fine pebble to granule conglomerate beds (F2), mostly ranging in thickness from 10 to 30 cm, have a sharp yet non-erosional basal contact and, in some places, preserve delicate ripple forms in the underlying muddy siltstone beds (Figure 8A). The F2 beds are internally massive and either ungraded or normally graded, transitioning upward into ripple laminated sandstone (F9, Figure 7A) and muddy siltstone (Figure 8C). They may show faint horizontal stratification (Figures 7A, 8A).
The paleocurrent direction of this facies association is consistently clustered around a NE to E mean direction and systematically varies both laterally and vertically (Figures 2, 5B). The isolated, larger planar cross-beds show an oblique paleotransport direction, contributing to the high dispersion of paleocurrent azimuths in some exposures.
Interpretation: a unimodal paleocurrent pattern, the dominance of small trough cross-beds, and the presence of small channel-form deposits indicate the deposition of this facies association from shallow, wide, low-sinuosity channels (Cotter, 1978; Dott et al., 1986; Fedo and Cooper, 1990; McCormick and Grotzinger, 1993; Long, 2004; Long, 2006; Ielpi and Rainbird, 2016). Aggradation was dominantly through migration and climbing of small, sinuous, crested dunes in shallow, wide, poorly channelized flow (Mckee et al., 1967; Williams, 1971; Ielpi and Rainbird, 2016). Obliquely migrating thicker planar cross-beds denote the development of rare unit bars in comparatively deeper parts of the otherwise sheet-like channel flows (cf., Cant and Walker, 1978; Fedo and Cooper, 1990). Impersistent, thin, muddy, fine sandstone/mudstone is inferred to represent waning flood deposits, and their sharp transition provides evidence for flashy discharge, followed by the rapid waning of the flow in a vegetation-free earth surface (Picard and High, 1973). Although the flow was inferred to be flashy, plane parallel strata were absent because of the coarser grain size of FA II. The dominant architecture comprises stacked sheet-like bodies separated by thin mudstone drapes (Figure 6) and the local presence of small channel fills (Figure 7B). Low-angle intraformational discordance in this facies association is indicative of ongoing syn-sedimentation tectonic deformation.
Massive to faintly horizontally stratified, sheet-like granule conglomerate beds lacking any bedforms and showing clast orientation parallel to the bedding plane, are characterized by flat, non-erosive bases deposited from hyperconcentrated flow with rheological characteristics intermediate between a Newtonian fluid and a Bingham plastic (Fisher, 1971; Pierson and Scott, 1985; Costa, 1988; Todd, 1989; Sohn et al., 1999; Sohn and Son, 2004). They resemble similar conglomerates of FA I but generally have a finer clast size and thinner strata. More clast-packed massive beds probably denote non-cohesive debris flow, and fining-upward massive beds resemble typical turbidite-like deposits (Major, 1997; Moscariello et al., 2002; Dasgupta, 2003; Cartigny et al., 2013).
Similar alluvial settings are common in present-day extreme climatic regimes (Williams, 1971; Karcz, 1972; Boothroyd and Ashley, 1975; Sneh, 1983) and have also been reported from many Precambrian fluvial deposits (Dott et al., 1986; Fedo and Cooper, 1990; McCormick and Grotzinger, 1993; Long, 2004; Eriksson et al., 2006; Ielpi and Rainbird, 2016). However, in a vegetation-free Precambrian landscape, flashy discharge and rapid waning could develop irrespective of climatic extremities. Unprotected by binding vegetation, loose debris were abundant, transforming the flow into a hyperconcentrated flow or a non-cohesive debris flow, leading to the development of interlayered F2 beds.
In the Nalla Gutta section, both the lateral transitions of FA I into FA II in the downcurrent direction and the vertical alternations between these two facies associations, along with broad similarity in the facies composition and inferred processes, finer grain size, and lesser bed thickness, indicate that the FA II succession represents the distal part of the FA I sediments. We infer that FA II sediments accumulated in the distal part of the megafans. The few-meter-scale coarsening upward trend probably denotes the progradation of the megafan and the eventually transition from FA II to FA I succession. A locally radial paleocurrent pattern and its consistent vector mean orientation at a high angle to the trend of the outcrop belt support similar transverse megafan origin for FA II.
In summary, the sheet-like lithosome, smaller bedform size, interlayered thin mudstone beds, and broadly transverse to basin axis paleoflow direction of FA II are consistent with the distal megafan setting. Limited evidence of channelization, finer grain-size, and discontinuous mudstone beds are expected to be more common in the gentler gradient toe regions of the megafans (Nichols and Fisher, 2007; Arzani, 2012; Sneider et al., 2021). However, due to coarser grain size, there is limited evidence of plane parallel stratification in the distal toe region of the Ramgiri megafan. The abundance of easily entrainable loose fragments on the vegetation-free megafan surface produced repeated thin mass flow or hyperconcentrated flow deposits. All these features of FA II can be attributed to a vegetation-free, easily erodible sandy substratum and the relatively high gradient of the stream channels. Theoretical considerations indicate that pre-Devonian streams may have had a width: depth ratio in excess of 500 (Fuller, 1985). Increased gradient tends to decrease the depth of the flood water (Frostick et al., 1988; Blair and McPherson, 1994). It is postulated that both these factors, the erodible substratum and higher gradient, acted in conjunction to produce the observed facies pattern and the typical sheet-braided architecture of the rocks of this association.
4.3 Medium/large-scale cross-bedded sandstone association (FA III)
Facies association 3 (FA III; Figures 9–12) predominantly consists of medium- to large-scale planar and trough cross-strata (F6 and F4), with subordinate plane-parallel strata (F7), ripple-laminated strata (F9), channel-fills (F5), and layers of mudstone and sandstone: mudstone heteroliths (F8, Figure 12). The planar cross-sets are 30–172 cm thick, and the trough cross-beds are 20–50 cm thick, forming cosets that range from 0.9 m to >5 m in thickness. Individual strata are 1.4 m–5.6 m thick and, in some places, are associated with preserved channel margins showing relief from 0.9 m to >3 m (Figure 10). Reactivation surfaces and smaller down-dipping intrasets (compound cross-strata) are common (Figures 9B, 11). Parallel-stratified and ripple-laminated medium- to fine-grained sandstone capping the cosets of large cross-strata are usually several decimeters thick (Figure 9A). Fine sandstone, siltstone, and mudstone units can be up to 70 cm thick in some instances; in one exposure southeast of the Begumpet red mudstone, the thickness reaches 4 m. This association occurs in all the studied sectors of the Ramgiri Formation.
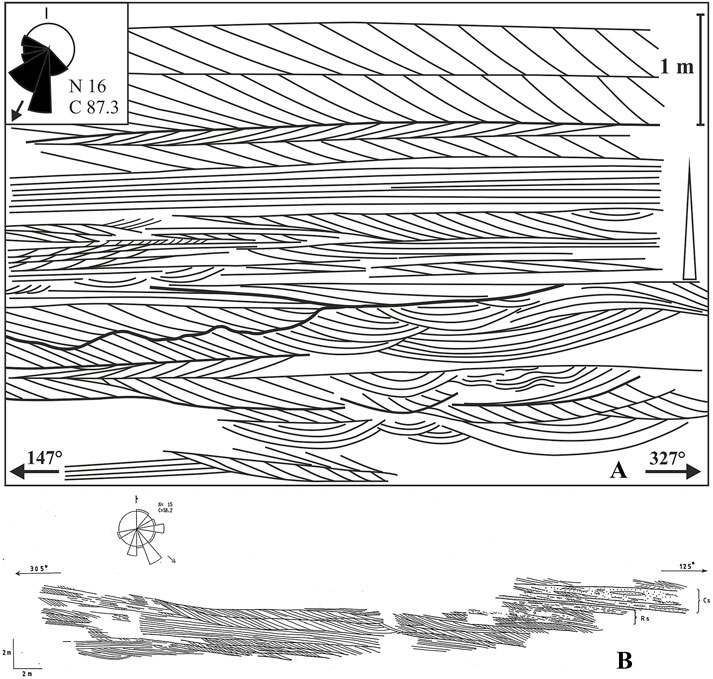
Figure 9. Field sketches showing the features of the FA III deposits. (A) Coset of large trough cross-beds overlain by a shallow channel, smaller bedforms, and plane-parallel stratification; top of the exposure marked by large planar cross-strata. SW-ward flow. (B) Coarsening-upward braided bar sequence within the Ramgiri Formation. The section can be divided into upstream (NW) and downstream (SE) ends and a central part. Complex interlayering cross-beds and parallel to low-angle lamination grade into rippled, fine-grained sandstone (Rs); the entire sequence is overlain by coarse, pebbly sandstone (Cs).
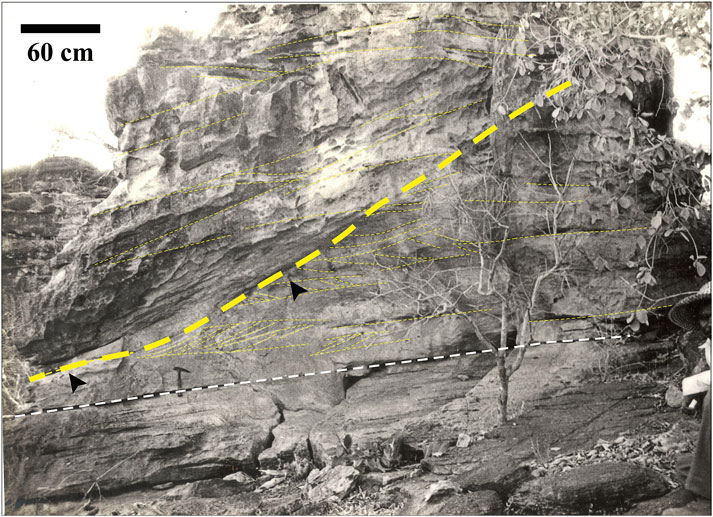
Figure 10. > 3 m deep channel scour in FA III (marked by dotted line). Lower portion of the exposure shows the coset of troughs and plane parallel stratification. Geological hammer for scale.
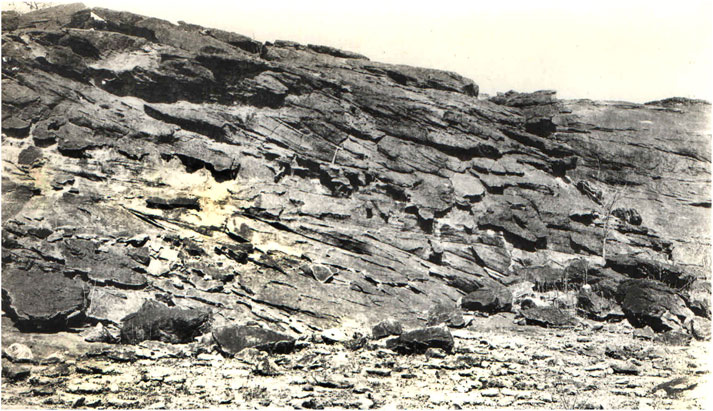
Figure 11. Coset of downcurrent-accreting planar cross set in a braided bar succession of FA III, Edlapur area. Two sets of downcurrent-accreting compound cross-strata are separated by a unit of parallel stratification. Geological hammer for scale.
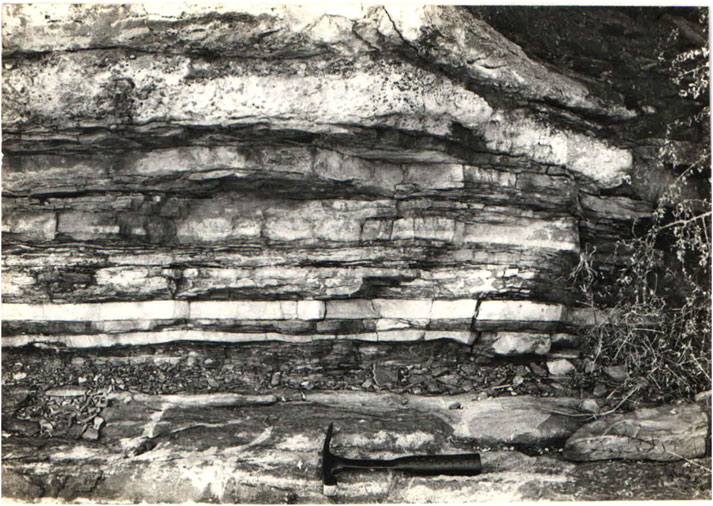
Figure 12. Exposure showing comparatively thicker mudstone and an overlying sandstone–mudstone heterolithic unit. Note the thickening and sanding upward succession in the heterolithic unit; F8, FA III succession, Nala Gutta area.
In some exposures, the sandstone succession is dominated by cosets of large planar sets separated by 5–20 cm-thick red mudstone beds. At other exposures, haphazard interbedding of cosets of planar cross-strata, medium-to-large trough cross-strata, planar laminated strata, and 40–60 cm deep channel scours characterize the FA III succession (Figure 9A). Lateral tracing of the succession in suitable exposure reveals a more complex interlayering of different facies (Figure 9B). The basal part of the exposure depicted in Figure 9B shows cosets of large trough sets, which, in the downstream direction, are overlain by downcurrent-accreting cosets of planar cross-strata (DA element). The planar cross-strata thicken as they migrate in the downcurrent direction, and the coset is truncated by a few small trough sets at the top, which show flow in a direction at a high angle to the underlying planar sets. Above this, the northwestern part of the exposure consists of a coset of smaller-sized cross-strata that pinch out downstream within a unit of parting lineated plane parallel or low-angle strata set (Figure 9B). The top central part of the exposure consists of two cosets of larger planar cross-sets. The planar sets migrate over gently (7°–10°) southeastward-inclined surfaces, and each of the individual cross-strata tends to initially thicken downstream and then pinch out, producing a wedge/lens-shaped set. Many of the planar sets in the downcurrent end develop into trough-shaped strata. The upper part of the succession is exposed in the southeastern end and comprises two units. The lower unit (70 cm, marked Rs in Figure 9B) consists of fine-grained clayey sandstone with ripple lamination and is overlain by a ∼100 cm-thick unit of coarse-grained, granule-rich sandstone with small cross-strata. The mean paleocurrent direction is toward the SE (Figure 9B). The smaller trough cross-strata and ripple foresets show variable paleoflow varying between SW and ENE (Figure 9B).
Interpretation: repeated occurrences of larger channel-form erosional scour of different dimensions, the dominance of planar cross-strata and other unidirectional bedforms, and paucity of mudstone are consistent with the deposition of this facies association from a braided river system (Smith, 1971; Miall, 1996). The succession shown in Figure 9B resembles the internal architecture of a braid bar (c.f., Bristow et al., 1987; Best et al., 2003).The northwestern (upstream) part of panel 9B shows shallow water features of a bar head, which, in the central part of the panel, changes into thicker planar sets accreting over low-angle, downstream-inclined surfaces, resembling bar-front dune accretion into a deeper pool (Chakraborty, 1999; Best et al., 2003). The top of the bar succession comprising finer-grained rippled sand present in the downstream part of the panel diagram (Rs) represents deposition from bar-tail slough channels (Bluck, 1976; Bristow et al., 1987). The coarse granule-rich sandstone at the top denotes the winnowing of the bar tip by a shallow sheet flow during the low water stage (Bluck, 1976; Haszeldine, 1983).
5 Discussion
5.1 Sheet-braided vs. channelized pre-vegetation fluvial deposits
Numerous reports from pre-Silurian fluvial deposits indicate that sheet-braided channel systems dominate these successions (Cotter, 1978; Long, 1978; Fuller, 1985; Trewin, 1993; Sonderholm and Trisgaard, 1998; Went, 2005; Davies et al., 2011). However, recent studies of the Precambrian fluvial deposits using very large-scale outcrops in different basins reveal the presence of deep channels and macroforms, illustrating certain morphometric similarities of these river systems with the post-vegetation perennial fluvial systems (Ielpi and Rainbird, 2016; Ielpi et al., 2017; Ghinassi and Ielpi, 2017). However, despite the presence of deep channelized deposits, nearly 50% of the Precambrian fluvial deposits in these reports still comprise ‘sheet-braided’ deposits (Ielpi and Rainbird, 2016). Interestingly, an examination of the Ramgiri Formation reveals the presence of both sheet sandstones in FA I and FA II (Figures 5, 6) and deeper channelized flow, marked by the development of ∼5 m-thick compound bars in FA III (Figure 9B).
A careful examination of well-exposed sheet sandstone bodies of FA I reveals the presence of subtle concave-up to irregular scours at places (Figures 4, 7). Channelized scours are also common in FA II, but they are rather small and usually filled by a single bedform or muddy deposits (Figure 7B), indicating their origin from late-stage runoff rather than from flood-stage erosional scours by rivers. Both FA I and FA II contain channelized scour-fills with a width: depth ratio of >25; therefore, they are not representative of the channels that deposited the deeper channelized sandstone bodies. The preserved channelized erosion surfaces in FA I have a width: depth ratio varying between 25 and >100 in the limited outcrop width of ∼100 m. Larger outcrops will probably yield values much higher than this. Although it is difficult to obtain precise morphometric measurements in the available outcrops, the sheet geometry of the sandstone bodies is beyond doubt.
In contrast to the features of the FA I and FA II megafan deposits, the FA III units of the Ramgiri Formation are characterized by i) the presence of larger bedforms, including the development of compound downstream-accreting bars that attain a thickness of ∼5 m in some areas; ii) a paleocurrent pattern parallel to the basin axis; iii) a lack of conglomerates, particularly the mass flow deposits common in megafan facies; and iv) occurrences of comparatively thicker mudstone or mudstone–sandstone heterolith facies (F8). The facies and architecture of this facies association strongly resemble the fluvial deposits of the modern-day braided channels (Smith, 1971; Cant and Walker, 1978; Bluck, 1979; Miall, 1994; Santos and Stevaux, 2000; Lunt and Bridge, 2004; Sambrook Smith et al., 2009). We visualize that the axial system was fed by the numerous smaller transverse drainages of the megafans, and the basin axial plain had a gentler gradient than the transverse megafan surfaces. The higher discharge and lower gradient of these rivers favored the development of a greater depth of the bank full channels, which resulted in the formation of larger bedforms and bars (Leopold and Wolman, 1957; Frostick et al., 1988; Blair and McPherson, 1994). The development of the macroforms of different dimensions has been recorded in many of the pre-vegetation alluvial deposits (Chakraborty, 1999; Long, 2004; Long, 2006; Long, 2011; Ghinassi and Ielpi, 2017), which probably have similar low gradients and larger discharge. A perennial flow or, rather, the absence of rapid discharge fluctuation probably favored the growth and preservation of compound bedforms, which formed in the deeper water of these axial streams. The variation in the geomorphology, channel gradient, and discharge pattern probably exerted control on the type of the channels and the shape of the sediment body formed by them.
5.2 Discharge and flow character
One of the most striking characteristics of FA II sheet sandstones is that these units can be traced laterally for several hundred meters in suitable exposures. Individual beds, which are 25–100 cm thick and composed of small pebble/granule-rich coarse sandstone, generally have a flat base. Internally, they are comprised of a monotonous sequence of small trough cross-strata and are invariably capped by thin mudstone layers (Figure 6). The sharp transition of the pebbly, very coarse sandstone to mudstone units denotes abrupt changes in the flow velocity, as in the case of flashy discharge with a peaked hydrograph of many ephemeral streams (Sneh, 1983; Tooth, 2000), as has also been inferred for many of the pre-vegetation rivers (Trewin, 1993; Love and Williams, 2000; Davies and Gibling, 2010). We did not observe any extensive desiccated mudflat or well-developed paleosol or eolian sabkha deposits interlayered with coarse, pebbly sandstone in the Ramgiri Formation, implying that the climate was not arid and the alluvial system did not suffer from prolonged dry periods of the riverbed following the deposition of a single unit of FA I or FA II. The stacked succession comprising sheet sandstone topped by thin mudstone, therefore, possibly represents the rapidly waning repeated flood pulses. Such features of rapid flow stage fluctuation should be reconcilable with the surface processes on the vegetation-free landscape of the pre-Silurian time (Davies and Gibling, 2010), where discharge modulation by vegetation was absent. However, the present dataset from the Ramgiri Formation is not sufficient to interpret the climatic setting and meteorological cycles of precipitation.
5.3 Mass flow and/or hyperconcentrated flow deposits in fluvial succession
Another important feature of FA I and FA II is the common occurrence of small pebble to granule-rich mass flow or hyperconcentrated flow deposits (F2; Figures 4, 5A, 7A, 8, 13). Although very common in the exposures of FA I and FA II, the mass flow deposits comprise only ∼8% of the measured successions. The bed thickness of most of these F2 units is less than 40 cm, and the mean of the 10 largest clasts mostly varies between 20–50 mm (Figure 5C). The smaller clast size, reduced thickness, and yet regular interlayering with cross-stratified sandstone indicate that these deposits do not represent the mass flow generated by large-scale slope failures in the upstream hinterland region of high-gradient alluvial fans (cf. Blair and McPherson, 2009). These mass flow beds are inferred to have developed through the rapid entrainment of abundant loose debris available on a vegetation-free surface during the Precambrian. Intense physical weathering, reduced chemical breakdown, and mud production due to the absence of vegetation (Algeo and Scheckler, 1998; Davies and Gibling, 2010) ensured the availability of a large volume of loose debris on the exposed Ramgiri alluvial plain. The rapid entrainment of loose debris, unbound by soils, muddy sediments, or vegetation, was likely much more common during the Precambrian than in the post-Silurian earth surface (Sonderholm and Trisgaard, 1998; Eriksson et al., 2006; Davies and Gibling, 2010). Figure 13 shows the lateral transformation of cross-bedded pebbly sandstone to the hyperconcentrated flow and vice versa over a short distance within FA II. The flow transformation of the dilute stream flow through the incorporation or entrainment (balking) of loose debris from the substratum during stream floods has been widely reported from volcanic or steeper alluvial surfaces (Costa, 1988; Todd, 1989; Smith and Lowe, 1991; Sohn et al., 1999; Benvenuti, 2003). It is inferred that similar processes were operative in the vegetation-free alluvial plain of Ramgiri. We emphasize that the repeated occurrence of mass flow or hyperconcentrated flow deposits in the Ramgiri Formation is probably one of the typical features of vegetation-free surface processes on the Precambrian earth, and the existing deposits should be explored for similar occurrences of alluvial plain debris flow or hyperconcentrated flow deposits.
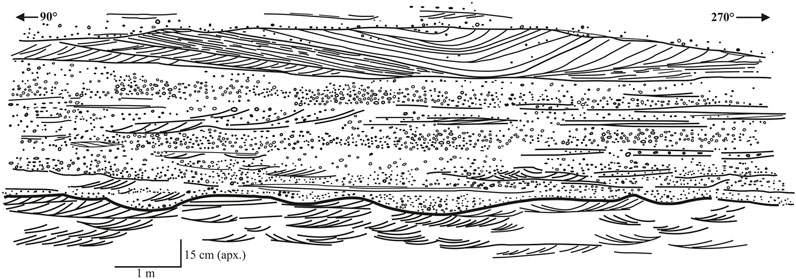
Figure 13. Field sketch illustrating the features of F2 conglomerate bed. Detailed field sketch of a sheet-like unit of crudely parallel-stratified pebbly conglomerate with sandy lenses, showing lateral gradation from massive conglomerate to thin parallel- and cross-stratified sandstone and vice versa within the exposure. The transition from massive conglomerate to parallel and cross-strata indicates flow transformation from hyperconcentrated to more dilute fluid gravity flow. Slightly undulating erosive base of the conglomerate unit and overlying channel-fill deposit; presence of mudstone unit (marked by straight lines) within the complex fill of the channelized scour.
5.4 Megafan interpretation
FA I and FA II of the Ramgiri Formation have been interpreted as proximal and distal parts of large, low-gradient megafan deposits. Megafans or distributive fluvial systems (DFSs) are known to form huge, low-gradient, sedimentary bodies at the basin margin across different climatic and tectonic settings worldwide (Gohain and Prakash, 1990; Leier et al., 2005; Weissmann et al., 2010; Hartley et al., 2010). These large, fan-shaped sediment bodies have a gentler surface slope than high-gradient alluvial fans (Blair and McPherson, 1994), but they are generally steeper than the alluvial plain streams (Chakraborty and Ghosh, 2010). However, the identification and distinction of megafans from riverine deposits or alluvial fans in the ancient rock record is often problematic and is based on the application of a limited subset of criteria originally identified from megafan deposits that include a radial drainage pattern, proximal to distal variation in grain size, changing channel body dimension and channel planform type, increase in the relative proportion of floodplain to channel deposit, and changing soil type (Weissmann et al., 2013; Hartley et al., 2013; Owen et al., 2015; Owen et al., 2017). However, in the case of pre-vegetational fluvial setting, their sheet-like sandstone units, paucity of fine-grained floodplain deposits, and absence/paucity of paleosols or biogenic activity make this interpretation of megafan deposits even more difficult (Ielpi and Ghinassi, 2016). The distinction between high-gradient alluvial fan deposits (sensu Blair and McPherson, 1994) and large-scale fluvial megafan deposits should be discernible even in the Precambrian. Instead of the dominance of mass flow or supercritical sheet-flood couplet deposits (sand–gravel couplet facies c.f., Blair, 1999), the megafan deposits should be largely dominated by channel-fill deposits. Overall grain sizes of the megafans are finer than those of the high-gradient alluvial fans, and the rate of downstream changes in the grain size of alluvial fans is more rapid than similar changes in the megafans (Hubert and Fillipov, 1989; Blair and McPherson, 1998; Nichols and Fisher, 2007; Chakraborty and Ghosh, 2010; Owen et al., 2015). The radial pattern of the paleocurrent noted in vertical profiles (in the Nalla Gutta sector; Figures 2, 3, 5B) possibly indicates a radial drainage pattern on the paleogeomorphological features that deposited FA I and FA II. Although these units contain thinner and finer beds of mass flow or hyperconcentrated flow deposits, volumetrically, F2 pebbly conglomerates are significantly less than the proportion of mass flow beds in the high-gradient alluvial fan deposits. The distal part of the megafan, as in the case of FA II, is dominated by finer grain size, thinner pebbly sandstone/conglomerate beds, and thinner bedforms, which are believed to be a response to decreased gradient and sheet-flood processes (cf. Roaring River dam breakout alluvial fan, Blair, 1987; Gao et al., 2019). Many of the modern megafans are also dominated by sheet-flood generated plane parallel lamination (Chakraborty and Ghosh, 2010, their Figures 8, 9A). The FA I units merge downstream with the FA II succession, corroborating the proximal–distal relationship of these two facies associations (Figure 3). Both the FA I and FA II succession show overall paleocurrent transverse to the basin axis, denoting their similarity to most of the modern megafans drainages (Weissmann et al., 2013). As discussed above, we interpret that the sheet geometry of the FA I and FA II, in addition to the erodible substrate of the pre-vegetation earth surface, was also influenced by the comparatively higher gradient of the megafans. This becomes more evident because of the distinctly different architecture of the axial streams (FA III).
The geophysical and tectonic setting of the Godavari graben indicates that the basin was an asymmetric rift basin that developed along the suture between the Dharwar and Bastar Archean cratonic nuclei (Qureshy et al., 1968; Mishra et al., 1989; Chaudhuri et al., 2012). The northeastern margin was marked by a major active fault system, whereas the southwestern basin margin was marked by a passive roll-over anticline (c.f., Withjack et al., 2002). The juxtaposition of the geophysical maps with the outcrop belt suggests the development of the Ramgiri Formation on the passive margin of the Godavari half-graben. Many of the roll-over anticlines of the half-graben basins are marked by the development of large megafans (Frostick et al., 1988; Leeder and Gawthorpe, 1987). We, therefore, postulate that the Ramgiri megafan developed on the roll-over anticline of the Proterozoic Godavari graben, and the axial stream system of the FA III developed in the adjacent axial part of the basin (Figure 14). Nambi Breccia (conglomerate) occurs in the eastern limb of the Proterozoic succession Godavari graben, and it is considered to be equivalent to the basal Sullavai succession in the western limb (Chaudhuri, 2003), probably representing a gravelly alluvial fan–fan delta system. This fan-delta is related to the major active fault present in the eastern margin of the Godavari graben (cf., Qureshy et al., 1968; Mishra et al., 1989; Chaudhuri et al., 2015). As shown in our paleogeographic reconstruction (Figure 14), on the roll-over anticline on the western limb, granitic basement-derived huge megafans of Ramgiri arkosic sandstone were deposited, whereas the synthetic fault system within the basin uplifted the local basement of the Pakhal Group of sediments that were drained by a comparatively smaller fluvial system of Mancheral Quartzite.
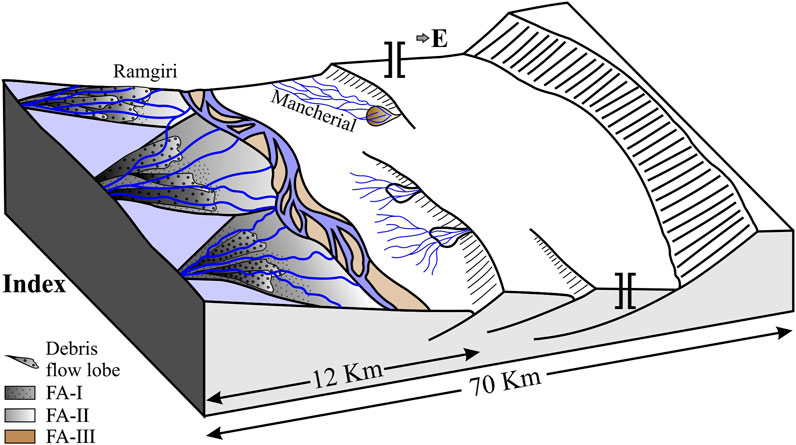
Figure 14. Cartoon diagram illustrating the paleogeographic scenario of the Ramgiri Formation and the Mancheral quartzite in the SW Precambrian outcrop belt of the Godavari graben. Active fault margin in the east and the roll-over anticline on the western margin with Ramgiri megafans and the axial braided river system. Synthetic faults in the basin that produced highlands, which are drained by the Mancheral fluvial system. The scale of the half-graben basin and the width of the Sullavai outcrop belt are marked separately.
6 Comparison with Mancheral Quartzite
Mancheral Quartzite, a succession of conglomerate to coarse-grained quartzose sandstone, erosively overlies the Ramgiri Formation and occurs close to the Ramgiri Formation (Table 1; Figures 1, 2). The Mancheral Quartzite, having a younger age and being a well-known fluvial deposit (Chakraborty, 1999, Supplementary Datasheet A1), shows certain important differences with the Ramgiri facies as follows: i) the paleocurrent pattern of the Mancheral quartzite is opposite to that of the Ramgiri Formation and is consistently toward the south to southwest (Figure 2); ii) the sandstone is predominantly quartzose to quartz arenite in composition; iii) several first-order channelized erosion surfaces (fifth-order surfaces of Miall, 1996), each several meters deep, have been recognized in the Mancheral Quartzite (Chakraborty and Chaudhuri, 1993, their Figures 2, 3); iv) the interlayering of the eolian sediments is common and is consistent with the reworking of the alluvial plain in the vegetation-free setting (Chakraborty and Chaudhuri, 1993); v) ferruginous soil profile of ∼1.2–3 m thickness is well-developed in some places in the alluvial plain (Supplementary Datasheet A1). The features clearly indicate the following: A. the Mancheral Quartzite and Ramgiri Formation indicate their contrasting provenance. Mancheral Quartzite was fed by Mesoproterozoic quartzite units of underlying Pakhal and Penganga Groups, whereas the Ramgiri Formation sampled the Archean gneiss of East Dharwar province, flanking the southwestern basin margin (Chakraborty, 1994). B. The presence of extensive vertisol-like cracks in the soil profiles and the development of eolian and sabkha sequences probably indicate a semi-arid climate with high seasonal rainfall during the deposition of Mancheral Quartzite. A lack of similar features might indicate a more humid environment for the Ramgiri Formation (Chakraborty, 1994). C. Several features common in the present-day alluvial rivers (channelization, macroforms, and development of the soil profile) are also observed in the Mancheral fluvial system. In contrast, the architecture of the FA I and FA II in the Ramgiri Formation are more similar to the sheet-braided model for pre-vegetation rivers, whereas the axial stream deposits of FA III record the development of features similar to that of the Mancheral fluvial system. The channelization and their preservation in the Mancheral quartzite were possibly favored by the development of early iron-oxide cementation (Supplementary Figure S1E) that provided the necessary cohesiveness of the sediments. Based on this comparison, it is reasonable to assume that the Ramgiri Formation, devoid of paleosols, eolian, or sabkha deposits, was probably deposited in a more humid climate (Chakraborty, 1994). The lack of cohesive substrate and the higher gradient of the Ramgiri megafans contributed to the development of the extensive sheet-braided character of FA I and FA II.
The study of the Ramgiri Formation reveals the presence of both the typical ‘sheet-braided’ sandstones and the development of >3 m deep channelized sandstone with the growth of channel-filling compound bars. Existence of both the above-mentioned architectural types has been observed in many of the Precambrian fluvial deposits (Long, 2006; Long, 2011; Ielpi and Rainbird, 2016; Ielpi et al., 2017), underscoring the assertion that all the pre-vegetational channel systems are not necessarily exclusively ‘sheet-braided’ type. Our exploration of the Ramgiri Formation indicates that in addition to the factors contributed by a pre-vegetational setting such as non-cohesive sediment, lack of vegetation and mud, and precipitation-induced flashy discharge on an un-vegetated surface, the higher gradient of the transverse megafan surfaces was probably one of the main reasons for developing sheet-braided architecture in FA I and FA II. On the other hand, the axial streams of the Ramgiri Formation (FA III), which must have had a lower gradient and larger catchment, inducing less peaked hydrograph, were characterized by deeper flow and the development of larger bedforms. The preservation of several-meter-deep channel scours in the overlying Mancheral Quartzite might have been facilitated by the development of paleosols and a thick fluvio-eolian complex in a wide floodplain. It is, therefore, reasonable to assume that the Ramgiri Formation, devoid of soil profiles, eolian, and/or sabkha units, was possibly subjected to a more humid climate close to the basin margin highlands.
7 Conclusion
The examination of the Neoproterozoic Ramgiri Formation reveals the following:
1. Commonly occurring thin beds of small pebble- and granule-rich mass flow or hyperconcentrated flow deposits are common in the Ramgiri megafan deposits, reflecting the rapid entrainment of abundant surface debris by the flashy discharge, and it is considered to reflect the typical character of non-vegetated Precambrian earth surfaces.
2. The sheet sandstones of megafan deposits (FA II) comprise a monotonous succession of small-trough cross-strata capped by thin units of mudstone. The sharp contact between the mudstone and underlying pebbly sandstone indicates a rapidly waning flashy discharge, a feature typical of a vegetation-free landscape.
3. Both axial and transverse drainages are well-developed in the Ramgiri Formation. The transverse megafan drainages are typified by thin sheet-like shallow braided stream deposits. The axial drainage, on the other hand, is characterized by deeper channelization and the development of compound bars and larger sandy bedforms.
4. Deposits of both channelized flow and sheet flow in the different facies associations of the Ramgiri Formation provide a realistic facies character and channel behavior of the pre-vegetational alluvial system and counteract the bias in favor of exclusively “sheet-braided” depositional models for Precambrian alluvium.
5. A comparison with slightly younger Precambrian fluvial deposits of the Mancheral Quartzite, which occur in the same basin, indicates that the climatic factors favoring the eolian reworking of the exposed sandy alluvial plain, soil profile development, and early iron cementation probably contributed to the development and preservation of channelization in the Mancheral fluvial deposits.
6. We suggest that the lack of cohesiveness (due to vegetation, soil development, and early diagenetic cements), high gradient, and flashy discharge were the major factor influencing the sheet-braided architecture of the megafan deposits in the Ramgiri Formation, whereas a perennial drainage, lower gradient, and higher proportion of mud in the axial drainage gave rise to features similar to those of Phanerozoic rivers.
Data availability statement
The original contributions presented in the study are included in the article/Supplementary Material; further inquiries can be directed to the corresponding author.
Author contributions
TC: conceptualization, formal analysis, funding acquisition, investigation, methodology, project administration, supervision, visualization, writing–original draft, and writing–review and editing. ST: data curation, formal analysis, funding acquisition, project administration, software, validation, visualization, and writing–review and editing.
Funding
The author(s) declare that financial support was received for the research, authorship, and/or publication of this article. This research was funded by the Indian Statistical Institute Kolkata (Grants awarded to TC).
Acknowledgments
The authors thank the Indian Statistical Institute for providing funding and infrastructure. They are grateful to Late Ashru K, who introduced the problem to TC and encouraged the authors to take up the research on the Precambrian Fluvial system. They greatly appreciate the help of Shankar Narayan Das and Tapas Kar of ISI during fieldwork and in the laboratory.
Conflict of interest
The authors declare that the research was conducted in the absence of any commercial or financial relationships that could be construed as a potential conflict of interest.
Generative AI statement
The authors declare that no generative AI was used in the creation of this manuscript.
Publisher’s note
All claims expressed in this article are solely those of the authors and do not necessarily represent those of their affiliated organizations, or those of the publisher, the editors and the reviewers. Any product that may be evaluated in this article, or claim that may be made by its manufacturer, is not guaranteed or endorsed by the publisher.
Supplementary material
The Supplementary Material for this article can be found online at: https://www.frontiersin.org/articles/10.3389/feart.2025.1542510/full#supplementary-material
References
Abdullatif, O. M. (1989). Channel-fill and sheet-flood facies sequences in the ephemeral terminal River Gash, Kassala, Sudan. Sediment. Geol. 63, 171–184. doi:10.1016/0037-0738(89)90077-8
Algeo, T. J., and Scheckler, S. E. (1998). Terrestrial, marine teleconnections in the Devonian: links between the evolution of land plants, weathering processes, and marine anoxic events. Phil. Trans. R. Soc. Lond. B 353, 113–130. doi:10.1098/rstb.1998.0195
Amarasinghe, U., Chaudhuri, A., Collins, A. S., Deb, G., and Patranabis-Deb, S. (2014). Evolving provenance in the proterozoic pranhita-godavari basin, India. Geosci. Front. 6, 453–463. doi:10.1016/j.gsf.2014.03.009
Arzani, N. (2012). Catchment lithology as a major control on alluvial megafan development, Kohrud Mountain range, central Iran. Earth Surf. Process. Landforms 37, 726–740. doi:10.1002/esp.3194
Assine, M. L. (2005). River avulsions on the Taquari megafan, Pantanal wetland, Brazil. Geomorphology 70, 357–371. doi:10.1016/j.geomorph.2005.02.013
Benvenuti, M. (2003). Facies analysis and tectonic significance of lacustrine fan-deltaic successions in the Pliocene–Pleistocene Mugello Basin, Central Italy. Sediment. Geol. 157, 197–234. doi:10.1016/s0037-0738(02)00234-8
Best, J. L., Ashworth, P. J., Bristow, C. S., and Roden, J. (2003). Three-dimensional sedimentary architecture of a large, mid-channel sand braid bar, Jamuna River, Bangladesh. J. Sediment. Res. 73, 516–530. doi:10.1306/010603730516
Beyer, R. A., Stack, K. M., Griffes, J. L., Milliken, R. E., Herkenhoff, K. E., Byrne, S., et al. (2012). An atlas of Mars sedimentary rocks as seen by HIRISE. SEPM Sp. Pub 102, 49–95. doi:10.2110/pec.12.102.0049
Blair, T. C. (1987). Sedimentary processes, vertical stratification sequences, and geomorphology of the Roaring River alluvial fan, Rocky Mountain National Park, Colorado. J. Sediment. Petrology 57, 1–8.
Blair, T. C. (1999). Cause of dominance by sheetflood vs. debris-flow processes on two adjoining alluvial fans, Death Valley, California. Sedimentology 46, 1015–1028. doi:10.1046/j.1365-3091.1999.00261.x
Blair, T. C., and McPherson, J. G. (1998). Recent debris-flow processes and resultant form and facies of the Dolomite alluvial fan, Owens Valley, California. J. Sediment. Res. 68, 800–818. doi:10.2110/jsr.68.800
Blair, T. C., and McPherson, J. G. (2009). “Processes and forms of alluvial Fans,” in Geomorphology of desert environments. Editors A. G. Parsons, and A. D. Abrahams (Springer Science). doi:10.1007/978-1-4020-5719-9_14
Blair, T. C., and McPherson, J. G. (1994). Alluvial fans and their natural distinction from rivers based on morphology, hydraulic processes, sedimentary processes and Facies. J. Sediment. Res. A64, 451–490.
Bluck, B. J. (1974). Structure and directional properties of some valley sandur deposits in southern Iceland. Sedimentology 21, 533–554. doi:10.1111/j.1365-3091.1974.tb01789.x
Bluck, B. J. (1976). Sedimentation in some Scottish river of low sinuosity, 69. Transactions of the Royal Society of Edinburgh, 425–456.
Bluck, B. J. (1979). Structure of coarse-grained braided stream alluvium, 70. Transactions of the Royal Society of Edinburgh, 181–221. doi:10.1017/S0080456800012795
Boothroyd, J. C., and Ashley, G. H. (1975). “Process, bar morphology, and sedimentary structures on braided outwash fans, northeastern Gulf of Alaska,”in. Glaciofluvial and glaciolacustrine sedimentation. Editors A. V. Jopling, and B. C. McDonald (Tulsa: Spec. Publc. Soc. Econ. Paleont. Miner.), 23, 193–222.
Braudrick, C. A., Dietrich, W. E., Leverich, G. T., and Sklar, L. S. (2009). Experimental evidence for the conditions necessary to sustain meandering in coarse-bedded rivers. Proc. Natl. Acad. Sci. 106, 16936–16941. doi:10.1073/pnas.0909417106
Bridge, J. S., and Best, J. (1997). Preservation of planar laminae due to migration of low-reliefbed waves over aggrading upper-stage plane beds: comparison of experimental data with theory. Sedimentology 44, 253–262. doi:10.1111/j.1365-3091.1997.tb01523.x
Bristow, C. S., Ethridge, F. G., and Flores, R. M. (1987). “Brahmaputra River: channel migration and deposition,” in Recent developments in fluvial sedimentology, SEPM special Publication39. Editor M. D. Harvey, 63–74.
Bull, W. B. (1977). The Alluvial fan environment. Prog. Phys. Geogr. 1, 222–270. doi:10.1177/030913337700100202
Cant, D. J., and Walker, R. G. (1978). Fluvial processes and facies sequences in the sandy braided South Saskatchewan River, Canada. Sedimentology 25, 625–648. doi:10.1111/j.1365-3091.1978.tb00323.x
Cartigny, M. J. B., Eggenhuisen, J. T., Hansen, E. W. M., Postma, G., et al. (2013). Concentration-dependent flow stratification in experimental high-density turbidity currents and their relevance to turbidite facies models. J. Sediment. Res. 83, 1046–1064. doi:10.2110/jsr.2013.71
Chakraborty, T. (1991). Sedimentology of a proterozoic erg: the Venkatpur sandstone, Pranhita-Godavari Valley, south India. Sedimentology 38, 301–322. doi:10.1111/j.1365-3091.1991.tb01262.x
Chakraborty, T. (1994). Stratigraphy of the late proterozoic Sullavai group, Pranhita-Godavari Valley, Andhra Pradesh. Indian J. Geol. 66, 124–147.
Chakraborty, T. (1999). Reconstruction of fluvial bars from proterozoic mancheral quartzite, Pranhita-Godavari Valley, India. in Fluvial sedimentology VI. Editors N. D. Smith, and J. Rogers Oxford International Association of Sedimentologists Spec. Publ. 28, 451–466.
Chakraborty, T., and Chaudhuri, A. K. (1993). Fluvial-aeolian interactions in a Proterozoic alluvial plain: example from the Mancheral Quartzite, Sullavai Group, Pranhita-Godavari Valley, India. in Dynamics and environmental Context of the aeolian sedimentary systems. Editor K. Pye Special Publication Geological Society of London, 72, 127–141.
Chakraborty, T., and Ghosh, P. (2010). The geomorphology and sedimentology of the Tista megafan, Darjeeling Himalaya: implications for megafan building processes. Geomorphology 115, 252–266. doi:10.1016/j.geomorph.2009.06.035
Chaudhuri, A. K. (2003). Stratigraphy and palaeogeography of the Godavari Supergroup in the south-central Pranhita-Godavari Valley, south India. J. Asian Earth Sci. 21 (6), 595–611. doi:10.1016/S1367-9120(02)00047-0
Chaudhuri, A. K., and Chanda, S. K. (1991). “The proterozoic basin of Pranhita-Godavari Valley: an overview,” in Sedimentary basins of India: TectonicContext: nainital, ganodaya prakashan. Editors S. K. Tandon, C. C. Pant, and S. B. Casshyap, 13–30.
Chaudhuri, A. K., Deb, G. K., and Patranabis Deb, S. (2015). Conflicts in stratigraphic classification of the Puranas of the Pranhita–Godavari Valley: review, recommendations and status of the “Penganga” sequence. in Precambrian basins of India: stratigraphic and tectonic context. Editor R. Mazumder, and P. G. Eriksson Geological Society, London, Memoirs, 43, 165–183.
Chaudhuri, A. K., Deb, G. K., Patranabis Deb, S., and Sarkar, S. (2012). Paleogeographic and tectonic evolution of the Pranhita–Godavari Valley, central India: a stratigraphic perspective. Am. J. Sci. 312, 766–815. doi:10.2475/07.2012.03
Chaudhuri, A. K., and Howard, J. D. (1985). Ramgundam Sandstone: a middle Proterozoic shoal-bar sequence. J. Sedim. Petrol. 55, 392–397.
Chaudhuri, A. K., Mukhopadhyay, J., Patranabis Deb, S., and Chanda, S. K. (1999). The neoproterozoic cratonic successions of peninsular India. Gondwana Res. 2 (2), 213–225. doi:10.1016/s1342-937x(05)70146-5
Chaudhuri, A. K., Saha, D., Deb, G. K., Patranabis Deb, S., Mukherjee, M. K., and Ghosh, G. (2002). The Purana basins of southern cratonic province of India - a case for mesoproterozoic fossil rifts. Gondwana Res. 5, 23–33. doi:10.1016/s1342-937x(05)70884-4
Conrad, J. E., Hein, J. R., Chaudhuri, A. K., Patranabis-Deb, S., Mukhopadhyay, J., Deb, G. K., et al. (2011). Constraints on the development of Proterozoic basins in central India from40Ar/39Ar analysis of authigenic glauconitic minerals. Geol. Soc. Am. Bull. 123, 158–167. doi:10.1130/b30083.1
Costa, J. E. (1988). “Rheologic, geomorphic, and sedimentologic differentiation of water floods, hyperconcentrated flows and debris flows,” in Flood geomorphology. Editors V. R. Baker, R. C. Kochel, and R. C. Patton (Chichester: J. Wiley and Sons), 113–122.
Cotter, E. (1978). Evolution of the fluvial style with special reference to the central Appalachian Paleozoic. Fluv. Sedimentol. 5, 361–384.
Dasgupta, P. (2003). Sediment gravity flow—the conceptual problems. Earth-Science Rev. 62, 265–281. doi:10.1016/s0012-8252(02)00160-5
Davies, N. S., and Gibling, M. R. (2010). Cambrian to Devonian evolution of alluvial systems: the sedimentological impact of the earliest land plants. Earth Sci. Rev. 98, 171–200. doi:10.1016/j.earscirev.2009.11.002
Davies, N. S., Gibling, M. R., and Rygel, M. C. (2011). Alluvial facies evolution during the Palaeozoic greening of the continents: case studies, conceptual models and modern analogues. Sedimentology 58, 220–258. doi:10.1111/j.1365-3091.2010.01215.x
Dott, R. H., Byers, C. W., Fielder, G. W., Stenzel, S. R., and Winfree, K. E. (1986). Aeolian to marine transition in Cambro—Ordovician cratonic sheet sandstones of the northern Mississippi valley, U.S.A. Sedimentology 33, 345–367. doi:10.1111/j.1365-3091.1986.tb00541.x
Eriksson, P. G., Bumby, A. J., Brumer, J. J., and van der Neut, M. (2006). Precambrian fluvial deposits: Enigmatic palaeohydrological data from the c. 2–1.9 Ga Waterberg Group, South Africa. Sediment. Geol. 190, 25–46. doi:10.1016/j.sedgeo.2006.05.003
Eriksson, P. G., Catuneanu, O., Sarkar, S., and Trisgaard, H. (2005). Patterns of sedimentation in the Precambrian. Sediment. Geol. 176, 17–42. doi:10.1016/j.sedgeo.2005.01.003
Fedo, C. M., and Cooper, J. D. (1990). Braided fluvial to marine transition: the basal Lower Cambrian Wood Canyon Formation, southern Marble Mountains, Mojave Desert, California. J. Sediment. Petrology 20, 220–234.
Fisher, R. V. (1971). Features of coarse grained high-concentration fluids and their deposits. J. Sedim. Petrol. 41, 916–927.
Frostick, L., Reid, I., Jarvis, J., and Eardley, H. (1988). Triassic sediments of the Inner Moray Firth, Scotland: early rift deposits. J. Geol. Soc. 145, 235–248. doi:10.1144/gsjgs.145.2.0235
Fuller, A. O. (1985). A contribution to the conceptual modeling of pre-Devonian fluvial systems, Presidential address, Geocongress 1984. Trans. Geol. Soc. Afr. 88, 189–194.
Gao, C., Ji, Y., Wu, C., Jin, J., Ren, Y., Yang, Z., et al. (2019). Facies and depositional model of alluvial fan dominated by episodic flood events in arid conditions: An example from the Quaternary Poplar Fan, north-western China. Sedimentology 67 (4), 1750–1796. doi:10.1111/sed.12684
Ghinassi, M., and Ielpi, A. (2017). Precambrian snapshots: Morphodynamics of Torridonian fluvial braid bars revealed by three-dimensional photogrammetry and outcrop sedimentology. Sedimentology 65, 492–516. doi:10.1111/sed.12389
Gohain, K., and Prakash, B. (1990). “Morphology of the Kosi Megafan,” in Alluvial fans: a field approach. Editors A. Rachoki, and M. Church (Chichester, UK: John Willey and Sons Ltd), 151–178.
Hartley, A. J., Weissmann, G. S., Bhattacharayya, P., Nichols, G. J., Scuderi, L. A., Davidson, S. K., et al. (2013). “Soil development on modern distributive fluvial systems: preliminary observation ns with implications for interpretation of paleosols in the rock record,”in. New frontiers in paleopedology and terrestrial paleoclimatology. Editor S. Driese (SEPM (Society for Sedimentary Geology) Special Publication), 104, 149–158. doi:10.2110/sepmsp.104.10
Hartley, A. J., Weissmann, G. S., Nichols, G. J., and Warwick, G. L. (2010). Large distributive fluvial systems: characteristics, distribution and controls on development. J. Sediment. Res. 80, 167–183. doi:10.2110/jsr.2010.016
Haszeldine, R. S. (1983). Fluvial bars reconstructed from a deep, straight channel, Upper Carboniferous Coalfield of Northeast England. J. Sediment. Petrology 53, 1233–1247.
Hein, F. J., and Walker, R. G. (1977). Bar evolution and development of stratification in the gravelly braided Kicking Horse River, British Columbia. Can. J. Earth Sci. 14, 562–570. doi:10.1139/e77-058
Hubert, J. F., and Fillipov, A. J. (1989). Debris flow deposits in alluvial fans on the westflank of White Mountains, Owens Valley, California. Sediment. Geol. 61, 177–205.
Ielpi, A., and Ghinassi, M. (2016). A sedimentary model for Early Palaeozoic fluvial fans, Alderney Sandstone Formation (Channel Islands, UK). Sed. Geol. 342, 31–46. doi:10.1016/j.sedgeo.2016.06.010
Ielpi, A., and Rainbird, R. H. (2016). Reappraisal of Precambrian sheet-braided rivers: Evidence for 1·9 Ga deep-channelled drainage. Sedimentology 63, 1550–1581. doi:10.1111/sed.12273
Ielpi, A., Robert, H., Rainbird, R. H., Ventra, D., and Ghinassi, M. (2017). Morphometric convergence between Proterozoic and post-vegetation rivers. Nat. Commun. 8, 15250. doi:10.1038/ncomms15250
James, N. P., and Dalrymple, R. W.(2010). Facies models 4. Newfoundland, Canada: Geological Association of Canada. 1–586.
Joy, S., Jelsma, H., Tappe, S., and Armstrong, R. (2015). SHRIMP U–Pb zircon provenance of the Sullavai Groupof Pranhita–Godavari Basin and Bairenkonda Quartziteof Cuddapah Basin, with implications for the SouthernIndian Proterozoic tectonic architecture. J. Asian Earth Sci. 111, 827–839. doi:10.1016/j.jseaes.2015.07.023
Karcz, I. (1972). Sedimentary structures formed by flash floods in southern Israel. Sediment. Geol. 7, 161–182. doi:10.1016/0037-0738(72)90001-2
Karssenberg, D., and Bridge, J. S. (2008). A three-dimensional numerical model of sediment transport,erosion and deposition within a network of channel belts,floodplain and hill slope: extrinsic and intrinsic controls onfloodplain dynamics and alluvial architecture. Sedimentology 55, 1717–1745. doi:10.1111/j.1365-3091.2008.00965.x
Leeder, M. R., and Gawthorpe, R. L. (1987). “Sedimentary models for extensional tilt-block/halfgraben basins,”in Continental extensional tectonics. Editors M. P. Coward, J. F. Dewey, and P. L. Hancock (Geological Society of London, Special Publication), 28, 139–152. doi:10.1144/gsl.sp.1987.028.01.11
Leier, A. L., Decelles, P. G., and Pelletier, J. D. (2005). Mountains, monsoons, and megafans. Geology 33, 289–292. doi:10.1130/g21228.1
Leopold, L. B., and Wolman, M. G. (1957). River channel patterns: braided, meandering and straight. Washington: United Stated Govt Printing Office.
Long, D. G. F. (1978). Proterozoic stream deposits: some problems of recognition and interpretation of ancient fluvial systems. in Fluvial sedimentology. Editor A. D. Miall Calgary: Mem. Can. Soc. Petrol. Geol, 5, 313–341.
Long, D. G. F. (2004). “Precambrian Rivers,” in The precambrian earth; tempos and events. Editors P. Eriksson, W. Altermann, D. Nelson, W. Mueller, and O. Catuneanu Elsevier, 660–663.
Long, D. G. F. (2006). Architecture of pre-vegetation sandy-braided perennial and ephemeral river deposits in the Paleoproterozoic Athabasca Group, northern Saskatchewan, Canada as indicators of Precambrian fluvial style. Sed. Geol. 190, 71–95. doi:10.1016/j.sedgeo.2006.05.006
Long, D. G. F. (2011). “Architecture and depositional style offluvial systems before land plants: a comparison of Precambrian, early Paleozoic modern river deposits,”in From River to RockRecord: the preservation of fluvial sediments and their subsequent interpretation. Editors S. Davidson, S. Leleu, and C. P. North (SEPM Spec. Publ.), 97, 37–61.
Love, S. E., and Williams, B. P. (2000). Sedimentology, cyclicity and floodplain architecture in the Lower Old Red Sandstone of SW Wales. Geol. Soc. Lond. Spec. Publ. 180 (1), 371–388. doi:10.1144/gsl.sp.2000.180.01.19
Lunt, I. A., and Bridge, J. S., (2004). Evolution and deposits of a gravelly braid bar,Sagavanirktok River, Alaska. Sedimentology 51, 415–432. doi:10.1111/j.1365-3091.2004.00628.x
Major, J. J. (1997). Depositional Processes in Large-Scale Debris-Flow Experiments. J. Geol. 105, 345–366. doi:10.1086/515930
Marconato, A., Almeida, R. P., Turra, B. B., and Fragoso-Cesar, A. R. S. (2014). Pre-vegetation fluvialfloodplains and channel-belts in the Late Neoproterozoic-Cambrian Santa Barbara group (Southern Brazil). Sed. Geol. 300, 49–61.
Matsubara, Y., Howard, A. D., Burr, D. M., Williams, R. M. E., Dietrich, W. E., and Moore, J. M. (2015). River meandering on Earth and Mars: A comparative study of Aeolis Dorsa meanders, Mars and possible terrestrial analogs of the Usuktuk River, AK, and the Quinn River, NV. Geomorphology 240, 102–120. doi:10.1016/j.geomorph.2014.08.031
McCormick, D. S., and Grotzinger, J. P. (1993). Distinction of marine from alluvial facies in the Paleoproterozoic (1.9 Ga) Burnside Formation, Kilohogok Basin, N.W.T. Canada. J. Sediment. Petrol. 63, 398–419.
McKee, E. D., Crosby, E. J., and Berryhill, H. L. (1967). Flood deposits, Bijou Creek, Colorado, JUNE =)(. J. Sedim. Petrol. 37, 829–851. doi:10.1306/74d717b2-2b21-11d7-8648000102c1865d
Meert, J. G., and Pandit, M. K. (2015). Chapter 3- The Archaean and Proterozoic history of Peninsular India: tectonic framework for Precambrian sedimentary basins in India. in Precambrian Basins of India: Stratigraphic and Tectonic Context. London, Memoirs: Geological Society, 43, 29–54. doi:10.1144/m43.3
Miall, A. D. (1994). Reconstructing fluvial macroform architecture from two-dimensional outcrops: examples from the Castlegate Sandstone, Book Cliffs, Utah. J. Sediment. Res. B64, 146–158.
Miall, A. D. (1996). The geology of fluvial deposits: Sedimentary facies. in Basin analysis and petroleum geology. Heidelberg: Springer-Verlag Inc., 582.
Mishra, D. C., Gupta, S. B., and Venkatarayudu, M. (1989). Godavari rift and its extension towards the east coast of India. Earth Planet. Sci. Lett. 94, 344–352. doi:10.1016/0012-821x(89)90151-9
Moscariello, A., Marchi, L., Maraga, F., and Mortara, G. (2002). Alluvial fans in the ItalianAlps: sedimentary facies and processes. in Flood and megaflood processes and deposits: recentand ancient examples. International Association of Sedimentologist Special Publication, 32, 141–166. doi:10.1002/9781444304299.ch9
Nemec, W., and Steel, R. J. (1984). Alluvial and coastal conglomerates: Their significant features and some comments on gravelly mass-flow deposits. in Sedimentology of gravels and conglomerates. Editors E. H. Koster, and R. J. Steel 10, 1–31.
Nichols, J. G., and Fisher, J. A. (2007). Processes, facies and architecture of fluvial distributary system deposits. Sediment. Geol. 195, 75–90. doi:10.1016/j.sedgeo.2006.07.004
Owen, A., Nichols, G. J., Hartley, A. J., and Weissmann, G. S. (2017). Vertical trends within the prograding Salt Wash distributive fluvial system, SW United States. Basin Res. 29, 64–80. doi:10.1111/bre.12165
Owen, A., Nichols, G. J., Hartley, A. J., Weissmann, G. S., and Scuderi, L. A. (2015). Quantification of a distributive fluvial system. The salt Wash DFS of the Morrison Formation, SWUSA. J. Sediment. Res. 85, 544–561. doi:10.2110/jsr.2015.35
Owen, G. (1996). Experimental soft-sediment deformation: structures formed by the liquefaction of unconsolidated sands and some ancient examples. Sedimentology 43, 279–293. doi:10.1046/j.1365-3091.1996.d01-5.x
Picard, M. D., and High, L. R. (1973). Sedimentary structures of ephemeral streams. Amsterdam: Elsevier, 223.
Pierson, T. C., and Scott, K. M. (1985). Downstream dilution of a lahar: transition from debris flow to hyperconcentrated streamflow. Water Resour. Res., 21(10), 1511–1524. doi:10.1029/WR021i010p01511
Qureshy, M. N., Krishna Brahman, N., Garde, S. C., and Mathur, B. K. (1968). Gravity anomalies and the Godavari Rift, India. Bull. Geol. Soc. Am. 79, 1221–1229.
Ramakrishnan, M., and Vaidyanathan, R. (2010). Geology of India. Bangalore: Geological Society of India, 1–998.
Reading, H. G. (1996). Depositional sedimentary environment: processes, facies and stratigraphy. 3rd Edition. Oxford Blackwell Science, 687.
Rust, B. R. (1984). Proximal braidplain deposits in the Middle Devonian Malbaie Formation of Eastern Gaspe, Quebec, Canada. Sedimentology 31, 675–695. doi:10.1111/j.1365-3091.1984.tb01230.x
Saha, D., and Chaudhuri, A. K. (2003). Deformation of the Proterozoic successions in the Pranhita–Godavari basin, south India—a regional perspective. J. Asian Earth Sci. 21, 557–565. doi:10.1016/s1367-9120(02)00023-8
Saha, D., Patranabis-Deb, S., and Collins, A. S. (2016). Chapter one-Proterozoic stratigraphy of Southern Indian Cratons and Global context. in Stratigraphy and Time Scales, 1–59. doi:10.1016/bs.sats.2016.10.003
Sambrook Smith, G. H., Ashworth, P. J., Best, J. L., Lunt, I. A., Orfeo, O., and Parsons, D. R. (2009). The sedimentology and alluvial architecture of a large braid bar, Río Paraná, Argentina. J. Sediment. Res. 79, 629–642. doi:10.2110/jsr.2009.066
Santos, M. G. M., Almeida, R. P., Godinho, L. P. S., Marconato, A., and Mountney, N. P. (2014). Distinct styles of fluvial deposition in a Cambrian rift basin. Sedimentology 61, 881–914. doi:10.1111/sed.12074
Santos, M. G. M., and Owen, G. (2016). Heterolithic meandering-channel deposits from the Neoproterozoicof NW Scotland: Implications for palaeogeographic reconstructions of Precambrian sedimentary environments. Precambrian Res. 272, 226–243. doi:10.1016/j.precamres.2015.11.003
Santos, M. L., and Stevaux, J. C. (2000). Facies and architectural analysis of channel sandy macroforms in the upper Parana River. Quat. International 72, 87–94. doi:10.1016/s1040-6182(00)00024-0
Schumm, S. A. (1968). Speculations Concerning Paleohydrologic Controls of Terrestrial Sedimentation. Geol. Soc. Am. Bull. 79, 1573–1588. doi:10.1130/0016-7606(1968)79[1573:scpcot]2.0.co;2
Simpson, E. L., and Eriksson, K. A. (1989). Sedimentology of the Unicoi Formation in southern and central Virginia — evidence for late Proterozoic to early Cambrian rift-to-passive margin transition. Geol. Soc. Am. Bull. 101, 42–54. doi:10.1130/0016-7606(1989)101<0042:sotufi>2.3.co;2
Smith, G. A. (1986). Coarse-grained nonmarine volcaniclastic sediment: Terminology and depositional process. Bull. Geol. Soc. 97, 1–10. doi:10.1130/0016-7606(1986)97<1:cnvsta>2.0.co;2
Smith, G. A., and Lowe, D. R. (1991). “Lahars: Volcano-Hydrologic Events and Deposition in the Debris Flow—Hyperconcentrated Flow Continuum,”in Sedimentation in volcanic setting. Editors R. V. Fisher, and G. A. Smith (SEPM Special Publication), 45, 59–70. doi:10.2110/pec.91.45.0059
Smith, N. D. (1971). Transverse Bars and Braiding in the Lower Platte River, Nebraska. GSA Bull. 82 (12), 3407–3420. doi:10.1130/0016-7606(1971)82[3407:tbabit]2.0.co;2
Snieder, S., Griffiths, C. M., Owen, A., Hartley, A. J., and Howell, J. A. (2021). Stratigraphic forward modelling of distributive fluvial systems based on the Huesca System, Ebro Basin, northern Spain. Basin Res. 33 (6), 3137–3158. doi:10.1111/bre.12597
Sohn, Y. K., Rhee, C. W., and Kim, B. C. (1999). Debris Flow and Hyperconcentrated Flood-Flow Deposits in an Alluvial Fan, Northwestern Part of the Cretaceous Yongdong Basin, Central Korea. Journalof Geol. 107, 111–132. doi:10.1086/314334
Sohn, Y. K., and Son, M. (2004). Synrift stratigraphic geometry in a transfer zone coarse-grained delta complex, Miocene Pohang Basin, SE Korea. Sedimentology 51, 1387–1408. doi:10.1111/j.1365-3091.2004.00679.x
Sonderholm, M., and Trisgaard, H. (1998). Proterozoic fluvial styles: response to changing accommodation space (Riveradal Sandstone, eastern North Greenland). Sedimentary.
Sweet, I. P. (1988). Early Proterozoic stream deposits: braided or meandering – evidence from central Australia. Sediment. Geol. 58, 277–293. doi:10.1016/0037-0738(88)90073-5
Todd, S. P. (1989). Stream-driven, high-density gravelly traction carpets: possible deposits in the Trabeg Conglomerate Formation, SW Ireland and some theoretical considerations of their origin. Sedimentology 36, 513–530. doi:10.1111/j.1365-3091.1989.tb02083.x
Tooth, S. (2000). Downstream changes in dryland river channels: the Northern Plains of arid central Australia. Geomorphology 34, 33–54. doi:10.1016/s0169-555x(99)00130-0
Trewin, N. H. (1993). Controls on fluvial deposition in mixed fluvial and aeolian facies within the Tumblagooda Sandstone (Late Silurian) of Western Australia. Sediment. Geol. 85, 387–400. doi:10.1016/0037-0738(93)90094-l
Trisgaard, H., and Oxnevad, I. E. I. (1998). Preservation of pre-vegetational mixed fluvio–aeolian deposits in a humid climatic setting: an example from the Middle Proterozoic Eriksfjord Formation, Southwest Greenland. Sediment. Geol. 120, 295–317. doi:10.1016/s0037-0738(98)00037-2
Weissmann, G. S., Hartley, A. J., Nichols, G. J., Scuderi, L. A., Olson, M., Buehler, H., et al. (2010). Fluvial form in modern continental sedimentary basins: Distributive fluvial systems. Geology 38, 39–42. doi:10.1130/g30242.1
Weissmann, G. S., Hartley, A. J., Scuderi, L. A., Nichols, G. J., Davidson, S. K., Owen, A., et al. (2013). in Prograding distributive fluvial systems — geomorphic models and ancient examples. Editors S. G. Driese, and L. C. Nordt, 131–147. doi:10.1007/978-3-319-25029-8
Went, D. J. (2005). Pre-vegetation alluvial fan facies andprocesses: an example from the Cambro-Ordovician Rozel Conglomerate Formation, Jersey, Channel Islands. Sedimentology 52, 693–713. doi:10.1111/j.1365-3091.2005.00716.x
Went, D. J. (2020). Fluvial shoal water deltas: pre-vegetation sedimentation through the fluvial–marine transition, Lower Cambrian, English Channel region. Sedimentology 67, 330–363. doi:10.1111/sed.12645
Williams, G. E. (1971). Flood deposits of the sand-bed ephemeral streams of Central Australia. Sedimentology 17, 1–40. doi:10.1111/j.1365-3091.1971.tb01128.x
Keywords: pre-vegetation fluvial deposit, sheet-braided facies, alluvial plain mass-flow deposits, Precambrian channel-bars, Precambrian megafan, Ramgiri Formation, Pranhita–Godavari valley
Citation: Chakraborty T and Taral S (2025) Neoproterozoic fluvial succession of the Ramgiri Formation, Pranhita–Godavari Valley, India: implications for sheet-braided versus deep channelized models of pre-vegetation fluvial systems. Front. Earth Sci. 13:1542510. doi: 10.3389/feart.2025.1542510
Received: 09 December 2024; Accepted: 28 January 2025;
Published: 19 March 2025.
Edited by:
François Fournier, Aix-Marseille Université, FranceReviewed by:
Murat Gül, Mugla University, TürkiyeSantosh Kumar Rai, Wadia Institute of Himalayan Geology, India
Copyright © 2025 Chakraborty and Taral. This is an open-access article distributed under the terms of the Creative Commons Attribution License (CC BY). The use, distribution or reproduction in other forums is permitted, provided the original author(s) and the copyright owner(s) are credited and that the original publication in this journal is cited, in accordance with accepted academic practice. No use, distribution or reproduction is permitted which does not comply with these terms.
*Correspondence: Tapan Chakraborty, dGFwYW4uZ3N1QGdtYWlsLmNvbQ==