- 1School of Infrastructure Engineering, Nanchang University, Nanchang, China
- 2School of Architecture and Environmental Engineering, Nanchang Institute of Science and Technology, Nanchang, China
The technique of storing tailings with a waste rock embankment network integrates the high waste dumping site and high tailings pond engineering into one, effectively reducing the construction footprint. However, the significant particle size difference between waste rock and tailings often leads to seepage damage. Therefore, understanding the development mechanism of seepage deformation in tailings stored with waste rock embankment networks is crucial. This study examines the influence of different slurry concentrations (5% and 10%) and waste rock media lengths (30 cm, 60 cm, and 90 cm) on the infiltration and deformation of tailings within a waste rock medium. A specially designed infiltration test device was employed to simulate the process, and a three-dimensional visual dynamic microscopic simulation of tailings transport in waste rock was performed using a computational fluid dynamics-discrete element method (CFD-DEM) coupling. The study investigated the contact loss mechanism of tailings in the waste rock medium and the particle transport behavior through a combination of macroscopic and microscopic analyses. Results indicate that at 600 s, with a 60 cm media length, the filtration rates for 5% and 10% slurry concentrations were 15.2% and 6.6%, respectively. For a 10% concentration slurry at 600 s, the filtration rates for 30 cm, 60 cm, and 90 cm media lengths were 4.2%, 6.6%, and 15.9%, respectively. The experimental and numerical simulation results demonstrate that higher tailings concentrations lead to a higher likelihood of clogging in the waste rock medium, reducing the cumulative filtrate mass. The filtration rate initially rises sharply, peaks, and then decreases rapidly before stabilizing. Moreover, shorter waste rock media lengths result in earlier filtration onset, lower peak filtration rates, and lower stable filtration rates in the later stage. These findings provide valuable references for the future engineering design of tailings ponds utilizing waste rock embankments.
1 Introduction
Waste rock and tailings are the main solid wastes produced in the process of mine production, and their separate stockpiling has caused a series of problems, such as waste of land resources, environmental pollution, and safety (Shengo, 2021). In response to this situation, a new method of joint tailings discharge, using waste rock and waste rock grid stacking tailings, can not only accelerate the dissipation of superstatic pore water pressure in the discharge process but also improve the anti-slip stability of tailings storage (Zhou et al., 2017).
At present, numerous studies have been conducted on the issue of permeability stability. Song et al. (2016) analyzed the change in the infiltration coefficient during the infiltration of muddy water through an indoor simulation test and concluded that the infiltration of muddy water led to the elevation of the infiltration line, which lowered the flooding and seismic capacity of the dam body. Cui (2015) carried out tests on sand columns of muddy water seepage and silt-plugging mechanisms. The relationship between the permeability coefficient of the tailings dam and the porosity of the tailings sand and other parameters with the permeability time was investigated. A mathematical model of muddy water seepage from a tailings dam was established. Zhu et al. (2020) used the discrete unit method and the coupled method of computational fluid dynamics to establish a numerical model of infiltration deformation of poorly graded coarse-grained soil mixed with coarse and fine particle groups and investigated the transport law of fine particles in the process of infiltration deformation from the fine level. Zhu et al. (2016) carried out a contact scour damage test of clay and gravel using a horizontal infiltration tester and studied the contact scour phenomenon of gravel soil and clay soil as well as the influencing factors. Zhang et al. (2020) established a numerical model coupled with discrete elements and hydrodynamics in consideration of the effects of gradation, density, pore size, etc., and compared the results to determine that the critical hydraulic gradient of horizontally oriented seepage in chalk is higher than that of vertically oriented seepage. For the accelerated drainage performance of a waste rock dyke network, Jaouhar et al. (2011) numerically analyzed the pore water pressure dissipation process of tailings stockpiled in a waste rock dyke network by using the two-dimensional finite element method and showed that the spacing of the waste rock dyke network and the magnitude of the horizontally oriented permeability coefficient of the tailings are the main factors influencing the rapidity of tailings consolidation rate.
In the whole process of tailings slurry penetrating the waste rock media layer, the microscopic manifestation of the development process of penetration and deformation of waste rock and tailings is the transport law of suspended particles in the media pores. Ahfir et al. (2009) investigated the transport and deposition of suspended particles in saturated particulate porous media and found that the flow rate, the particle size, the internal structure of the porous media, and the length of the porous media are the important factors for the transport and deposition of suspended particles in the saturated porous media. It was also found that the flow velocity, particle size, internal structure of the porous medium, and length of the porous medium are important factors for the transport and deposition of suspended particles in saturated porous media. Dai et al. (2016) investigated the effects of particle size, concentration, and pore structure on the transport properties of suspended particles by using seepage tests in porous media under constant head. Chen and Bai (2012) and Chen et al. (2012) conducted indoor experiments on four different concentrations of suspended particles at three different seepage velocities to study the influence of suspended particle concentration on their migration and sedimentation characteristics. They found that gravity and seepage velocity are important factors affecting particle transport in saturated porous media. Liu et al. (2015) studied the effect of the particle deposition process on the porosity and permeability decay of porous media through theoretical analysis and sandbox experiments and established a mathematical computation model to quantitatively describe the change rule of permeability in the process of particle deposition. Zhang et al. (2016) carried out indoor soil column infiltration experiments of suspended silica powder particles using sodium fluorescein as a tracer to study the effect of seepage velocity on pore structure. Zamani and Maini (2009) concluded that particle deposition changes the pore morphology, thus altering the porosity and local pressure gradient of the porous medium, leading to a decrease in permeability. Liu et al. (2014) studied the effects of suspended particle size and porous media size on the migration and deposition characteristics of particles through sand migration-deposition simulation experiments and classified the migration and deposition types of suspended particles in porous media as “cake filtration,” “migration-deposition,” “porous media,” “porous media,” “porous media,” “migration-deposition type,” and “free migration type.” Jiang et al. (2018) used the discrete element software PFC3D to simulate the permeability evolution of coarse-grained soil and determined that the particle migration caused changes in the soil particle structure to form permeability deformation, which had different degrees of influence on parameters such as the porosity and permeability coefficient of coarse-grained soil.
Scholars have mainly studied infiltration deformation, which is the transport and deposition of suspended particles in porous media. The transport and migration of suspended particles in porous media are mainly related to factors such as particle size, concentration, medium pore structure, medium length, and seepage velocity. However, the permeability deformation of tailings stored in waste rock embankments is still in its infancy. This article draws on the research methods of permeability deformation and the results of the transport law of suspended particles in porous media to explore the influence of different concentrations and waste rock medium lengths on the permeability deformation of tailings stored in waste rock embankments through indoor experiments. The experimental conclusions are verified through computational fluid dynamics - discrete element method (CFD-DEM) coupling.
2 Test method
2.1 Test set-up
A custom-designed horizontal permeability test system for tailings infiltration in a waste rock medium was developed (Figure 1). The system comprises a slurry feeding device, flow measurement apparatus, infiltration device, and mass collection system. The slurry feeding unit consists of a mixer and a flexible pump to control the slurry concentration and flow rate. The infiltration test device includes a 10-cm-diameter plexiglass column with variable lengths (30 cm, 60 cm, and 90 cm) and a tailing box (15 cm × 15 cm × 20 cm) at the outlet. A 3.5 cm height difference between the tailing box base and the lowest point of the waste rock medium section ensures gravity-driven infiltration.
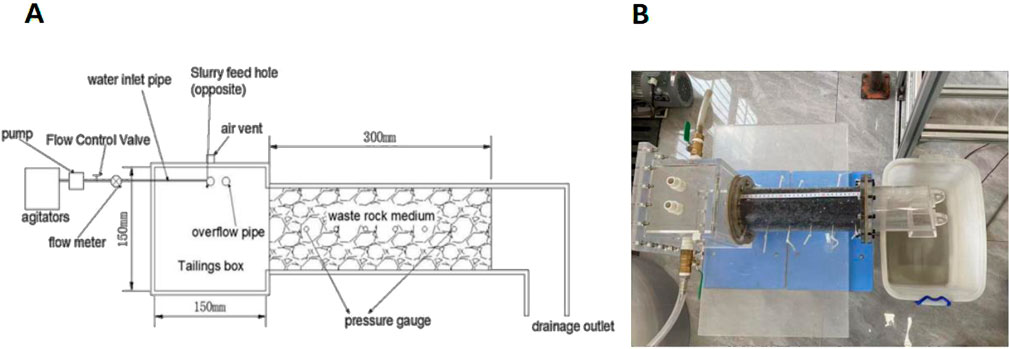
Figure 1. Installation diagram. (A) Waste rock medium tailings permeability test system, and (B) top view of the installation.
During the infiltration test, the infiltration rate of the slurry is zero when the slurry water level is lower than the lowest point of the waste rock layer cross-section, and the infiltration flow starts to appear only after the liquid level rises to the waste rock layer cross-section. Screens were placed at the entrance and exit of the test section to ensure uniform slurry flow in and out while preventing waste rock filler particles from flowing out; the quality collection system consisted of a filtrate collection box and an electronic analytical balance to monitor the change in filtrate quality per unit of time in real time.
2.2 Test materials
The tailings used in this experiment were sampled from the site, cleaned with clean water, and dried in an oven. The specific gravity was measured to be 2.72. The particle size distribution curve of the tailings was analyzed using a wet laser particle size analyzer, as shown in Figure 2A. The average median particle size of the tailings was d60 = 32.757 µm, d30 = 19.098 µm, and d10 = 9.529 µm. The particle content greater than or equal to 0.075 mm does not exceed 50%, and the unevenness coefficient Cu = d60/d10 = 1.72 < 5. The curvature coefficient Cc of the tailings tested in this study is 1.16. According to the soil classification standard, the tailings are well graded. Figure 2B shows the electron microscopy image of the tailings. From this image, it can be seen that most of the tailings particles appear as polyhedra or elongated flakes, which are prone to clogging during the infiltration process of waste rock media.
Considering the influence of particle size and shape, waste rock with a particle size range of 2–4 mm is used as the filling material for the waste rock medium. During each filling, the water surface is 1–2 cm higher than the top surface of the sample to ensure that the filled waste rock is saturated. For the loading of waste rock, the compaction instrument is controlled every 10 cm (3 times) to control the difference in porosity of different groups of experimental waste rock and reduce experimental errors. The permeability coefficient of the waste rock media layer was determined by a normal head test. The parameters of the waste rock were obtained as follows: the particle size of the waste rock ranges from 2 mm to 4 mm, the average porosity is 45%, and the average permeability coefficient K is 2.5 × 10−2 m/s.
2.3 Data processing
The experiment used slurry with concentrations of 5% and 10%, and a water test was used as the control group. The length of the waste rock medium layer was tested under three working conditions: 30 cm, 60 cm, and 90 cm. The collection box is placed on a high-precision electronic scale, which can be connected to a computer via a serial port to achieve real-time recording of the filtrate quality. The recording interval is set to 1 s. At time t, the filtration rate vm,t of the slurry at time t can be obtained by subtracting the mass of the filtrate obtained at the next time interval from the mass of the filtrate obtained at that time. Its magnitude reflects the speed of dehydration during the experiment. The specific calculation is shown in the following formula:
mt represents the mass of the filtrate obtained at time t; mt+1 represents the mass of the filtrate obtained at time t + 1;
3 Analysis of test results
3.1 Influence of different slurry concentrations on the permeation process
Three different mass concentrations of tailings slurry, 5%, 10%, and clear water, were tested at three waste rock media lengths of 30 cm, 60 cm, and 90 cm, and the corresponding time versus cumulative filtrate mass curves are shown in Figure 3A.
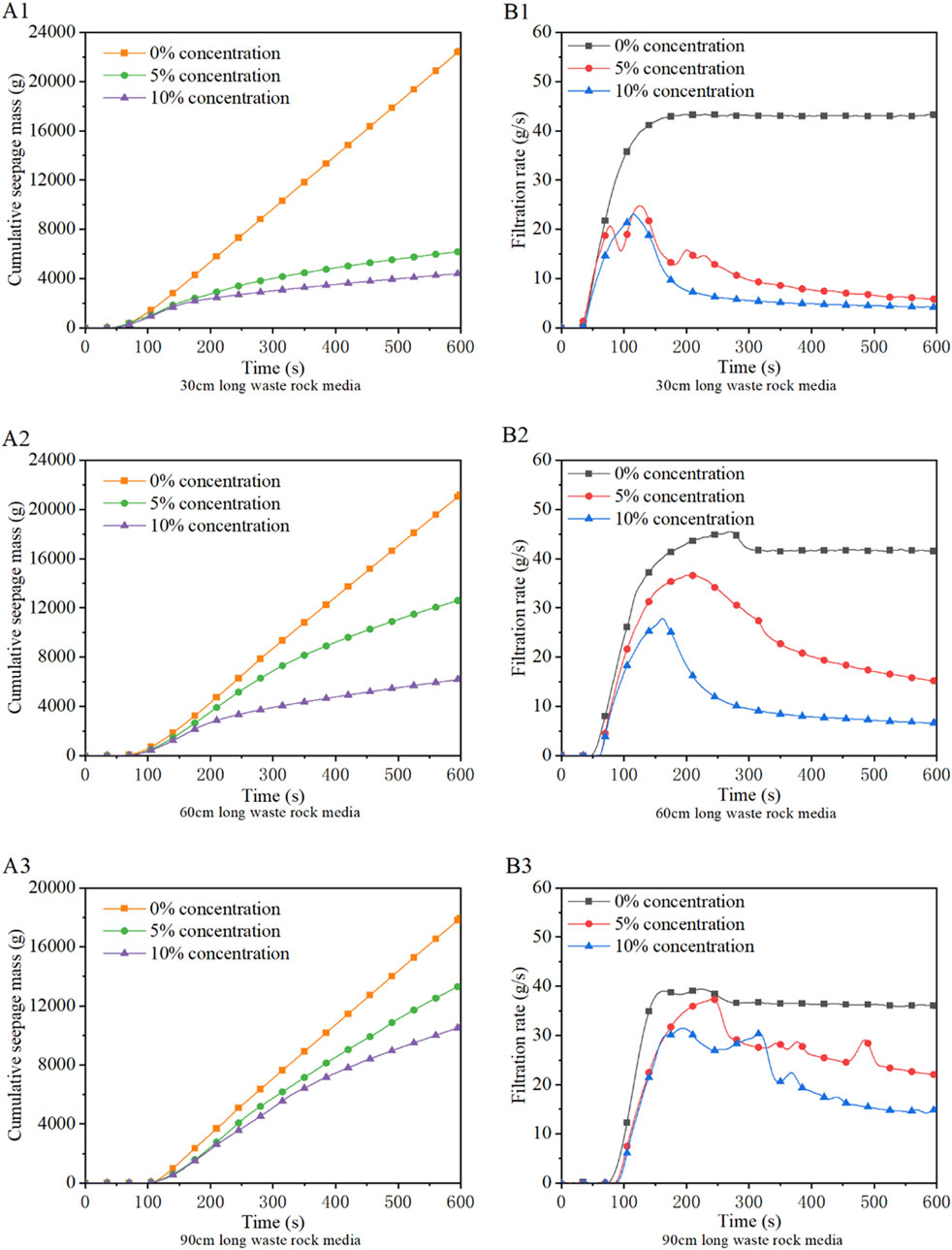
Figure 3. Graph of the effect of different concentrations of tailings slurry. (A) Law of change of cumulative filtrate mass with time and (B) law of change of filtration rate with time.
From Figure 3A, it can be seen that the effect of slurry concentration on seepage volume has a good regularity.
Figure 3B shows the filtration rate curve obtained from the cumulative filtrate mass curve. The filtration rate of the slurry grows sharply and soon reaches the peak. With the passage of time, the rate gradually slows and finally converges to a stable value. The clear water filtration rate is more ideal: it can be approximated as a double folding line, that is, a sharp increase to the peak rate, which stays basically unchanged. The greater the slurry concentration, the earlier the peak filtration rate occurs, and the lower the peak point, and the lower the rate in the stable filtration stage.
There are two main reasons for the above phenomena: on the one hand, under the action of gravity, van der Waals forces, etc., the coarse particles in the tailings slurry will be trapped in the pore channels of the waste rock medium, gradually forming local blockages. The increase in the concentration is manifested as an increase in the number of tailings particles in the suspension, which increases the probability of tailings entering the waste rock. Compared with clean water, the faster and more severe the blockage occurs inside the waste rock. On the other hand, under a hydraulic gradient, the permeable surface of the waste rock medium layer is subjected to a large hydrodynamic effect, and the fine particles of the waste rock will undergo deformation and reorganization, forming a seepage channel. The formation of blockage is not conducive to the filtration of the tailings slurry. The formation of internal seepage channels in the waste rock promotes the filtration of the slurry. Throughout the entire filtration stage, the two eliminate each other. In the initial stage of seepage, the seepage channel is rapidly formed under the action of water flow, and at this time, the seepage channel plays a dominant role. Therefore, the filtration rate increases rapidly in the initial stage. After a period of time, the effect of the two is comparable, and the growth of the filtration rate gradually slows down and reaches its peak. After the basic formation of the seepage channel in the later stage, the number and cross-section of channels no longer increase, but the retention of tailings particles increases. The layer pores become increasingly clogged. The filtration rate shows a rapid and gradually decreasing trend. As the pore blockage of waste rock media gradually intensifies, the filtration rate will gradually decrease. However, after a period of decline, the tailings particles in the pores will move a certain distance and form a stable filtration medium layer together with the waste rock. The filtration rate gradually decreases and tends to stabilize, and the drainage and filtration properties of the waste rock layer tend to stabilize.
3.2 Effect of different lengths of waste rock on the infiltration process
The test curve in Figure 4A shows that in the initial stage of slurry penetration, the longer the waste rock media length, the smaller the cumulative filtrate mass. After a period of time, it will appear that the cumulative filtrate mass of different waste rock lengths is equal at a certain moment. Then, it will appear that the longer the media length, the greater the cumulative filtrate mass, and the difference becomes more obvious. At 600 s, the cumulative filtrate mass of the waste rock media layer with a length of 90 cm is 2.39 times that of the length of 30 cm.
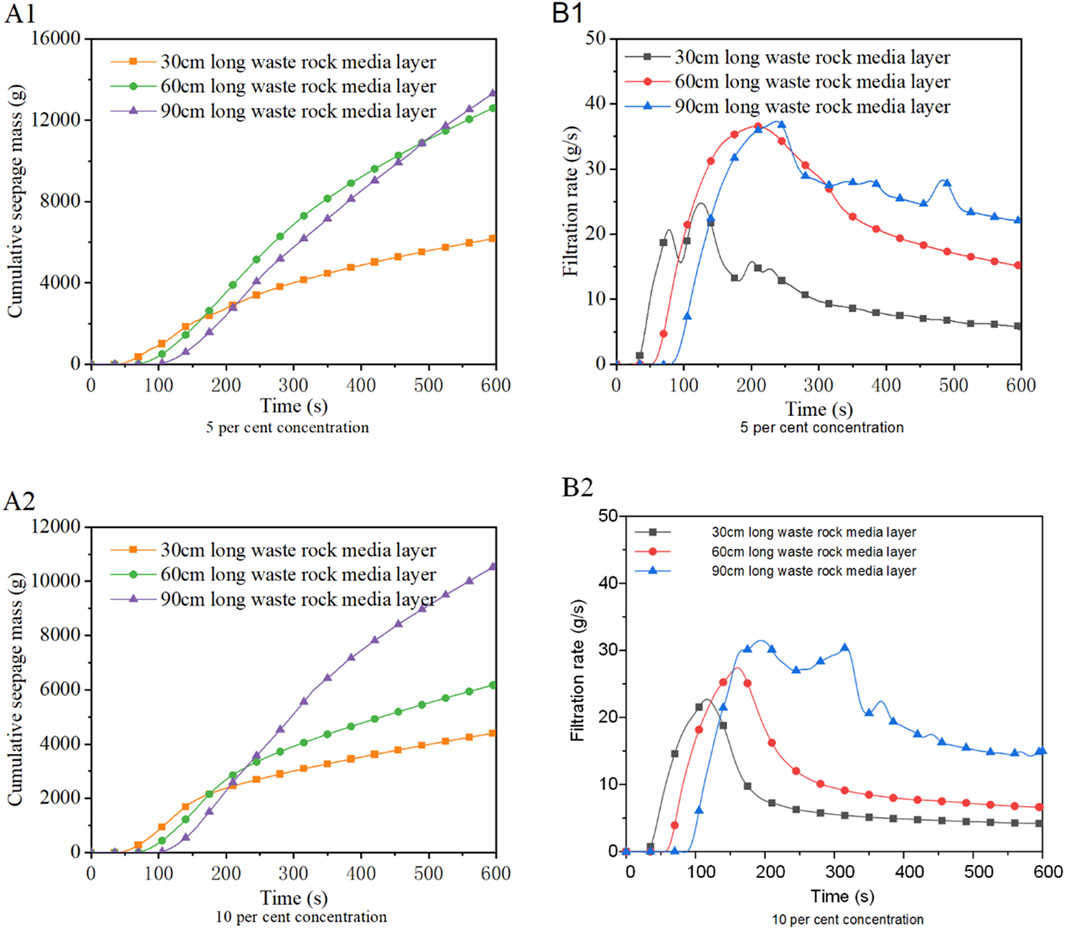
Figure 4. Graph of the effect of length of different waste rock media. (A) Filtrate mass vs. time and (B) filtration rate vs. time.
From the time-varying curves of filtration rates for different medium lengths in Figure 4B, it can be seen that the shorter the length of the waste rock medium, the smaller the corresponding peak value of the filtration rate, and the earlier the peak point appears. This phenomenon is mainly due to the shorter length of the medium and the nonwoven fabric set at the outlet: the higher the initial slurry seepage flow rate, the smaller the seepage length. Therefore, the more tailings particles are retained per unit seepage length and the higher the probability of pore blockage in the waste rock medium. In addition, due to the large hydraulic gradient, the tailings particles are subjected to greater hydrodynamic forces, so more particles will not remain near the contact surface between the slurry and the waste rock medium layer but will enter the pores inside the waste rock medium section, making it more prone to clogging. Taking into account the above two reasons, the shorter the length of the medium, the earlier it reaches the peak filtration rate; the corresponding peak rate is also smaller.
The longer the length of the waste rock media, the slower the decline after the filtration rate reaches the peak and the higher the value of the stable filtration rate in the later stage. The longer the length of the medium, the greater the permeability path of the tailings slurry, the smaller the hydraulic gradient, and the weaker the permeability force acting on the tailings particles, as shown in Figure 5. The tailings particles are subjected to screening, adsorption, and other effects, and the particles are retained in the pores (Xu et al., 2006). Some particles are retained at the contact surface between the slurry and the waste rock medium layer due to insufficient water use and are deposited under the action of gravity, resulting in fewer tailings particles entering the waste rock pores. The tailings particles are less likely to enter the internal pores of the waste rock, making it less likely for blockage to occur. The overall permeability degradation rate is also slower, and the filtration performance of the stable filtration medium layer is less degraded, while the permeability performance remains good. Therefore, the filtration rate during the stable stage is higher (Mohammed and Moghal, 2016).
Fine tailings particles will undergo flocculation in water environments. The resulting flocculation structure is called floc particles (Wu, 2017). The interparticle interaction of floc particle groups is weak, and the structure is very loose. The coarse particles in the tailings attract the previously deposited tailings particles, gradually forming a honeycomb structure with a high porosity.
When larger particles pass through narrow pores, they block the pores and increase the local pore water pressure (Yin et al., 2012). Especially when the length of the waste rock medium is longer, there is less blockage in the latter half of the pore channel. The increase in water pressure causes the collapse of the honeycomb or flocculation structure, resulting in a sudden increase in the filtration rate. However, soon new honeycomb or flocculation structures will form and gradually clog pore channels. The filtration rate will rapidly decrease, then return to a slow decrease, and gradually stabilize.
4 Numerical simulation
The numerical simulation method based on coupled fluid and particle flow (CFD-DEM) can be used as an effective complement to the physical model tests in this paper to analyze microscopic variables that cannot be detected in the model tests (Nan et al., 2023), such as particle loss, flow field pressure and distribution, and tailings mass distribution within the waste rock. Based on the numerical simulation results, the microscopic phenomena and variables that cannot be recorded in the test can be better observed. The contact loss phenomenon of tailings under different tailings mass concentrations and lengths of the waste rock media layer can be observed to achieve the three-dimensional visual and dynamic simulation, grasp the transport law of tailings particles, and understand the mechanism of deterioration of the permeability performance of the media in the waste rock media more deeply (Onyelowe et al., 2024).
4.1 Numerical modeling of contact loss from waste rock tailings and parameter setting
In order to simulate the seepage process of tailings in the waste rock pores, the discrete element method was used to construct the pore structure composed of 2–4 mm waste rock particles, as in Figure 6A, to achieve the simulation of the distribution of pore structure in the porous medium. The porosity was calculated to be approximately 45%. The density and viscosity of the fine-grained tailings slurry in the fluid domain were determined experimentally, and the relevant parameters were calibrated by angle-of-rest experiments and discrete element tests. The nickel fine-grained tailings were selected for several experiments, and the angle of repose was determined to be in the range of 47.1°–50.2°. In addition, a range of 27.1°–31.2° for the angle of repose of waste rock was obtained from several angle-of-repose tests. The results were based on specific gravity measurements of tailings samples (2.72 g/cm3), specific gravity measurements of waste rock samples (2.81 g/cm3), and particle size distributions (waste rock: 2–4 mm, fine-grained tailings: 1 to 100 µm). By using round particles to represent the tailings particles and considering the computational cost, according to Wang and Leung (2008), the particle size was appropriately enlarged during the discrete elemental simulation of tailings, corresponding to approximately 500,000 tailings particles, which accommodates the computational accuracy and computational efficiency. According to the tailings particle gradation curve d60, tailings particles consisting of three particle sizes (100 µm, 150 µm, and 200 µm) and ratios (60%, 30%, and 10%) were selected to simulate the actual tailings gradation, as shown in Figure 6B.
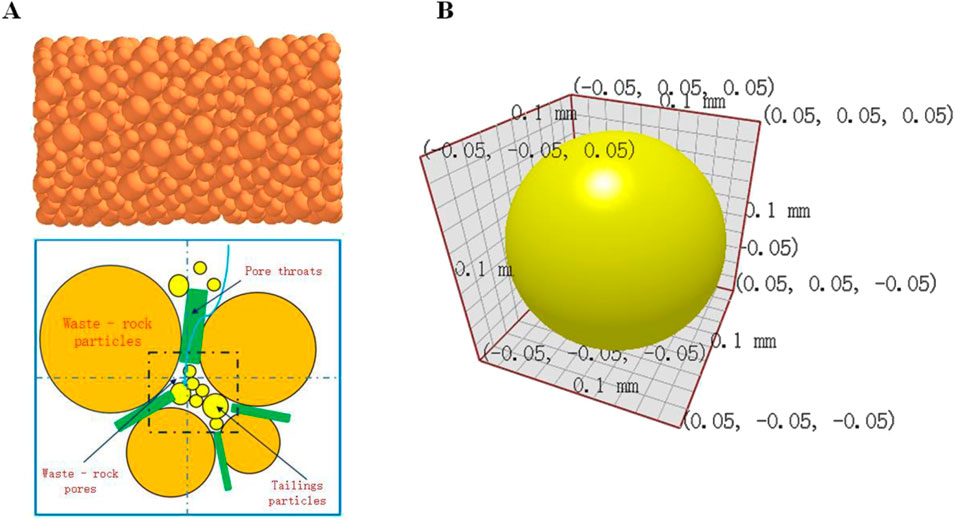
Figure 6. Numerical simulation parameter map. (A) Schematic diagram of the waste rock medium layer and waste rock pores and (B) DEM tailings particle shape size.
Other parameters were adjusted by calibrating discrete element numerical simulations. The experimental results were matched with the numerical simulation results by adjusting the coefficient of recovery between particles and the coefficients of dynamic and static friction, as shown in Figure 7. The values of the parameters shown in Table 1 were determined by repeated angle-of-repose tests and numerical simulations.
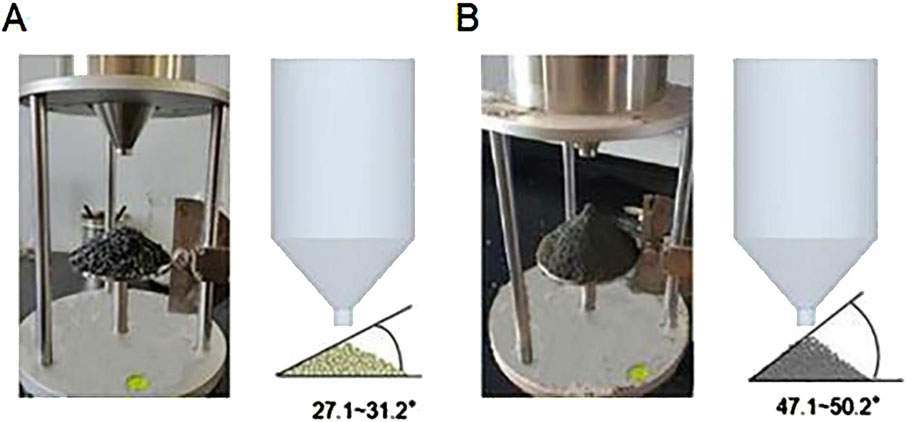
Figure 7. Calibration of angle-of-repose parameters. (A) Waste rock particle angle-of-repose test and simulation and (B) tailings particle angle-of-repose test and simulation.
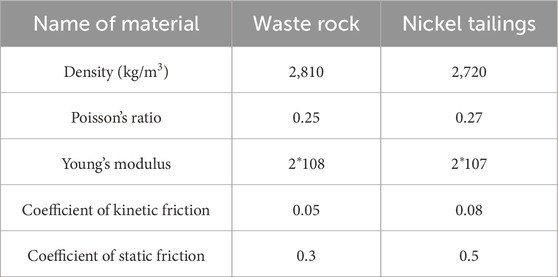
Table 1. Parameters of waste rock tailings determined by a combination of angle-of-repose tests and numerical modeling.
On the one hand, the more simulated particles, the slower the calculation, so the simulation is set to 10,000 time steps. After testing, the time step can be a better simulation of the effect of tailings on the permeability of the waste rock media deterioration process. On the other hand, a device that is too large will cause too many particles and lead to the simulation of the slow speed of the operation. To improve the speed of the operation, the model will be scaled up.
On the basis of the determined parameter values, the coupled hydrodynamic-discrete element solution was achieved by setting fixed boundary conditions. The specific material property parameters are shown in Table 2. In the simulation, for the step size setting, FLUENT’s step size is generally an integer multiple of the step size in the DEM. The simulation CFD time step is 5 × 10−4 s, and the DEM time step is 1 × 10−6 s. In CFD-DEM, it is necessary to calculate the volume fraction of particles intersecting with the grid based on the computational stability of the solver, the accuracy of the trailing force calculation, and other issues. The grid size is more than three times the size of the smallest particles.
In order to control the results of the physical model test, the numerical simulation adopts the following four working conditions, as shown in Table 2. The particle flow rate of 1 × 10−4 kg/s in the DEM software corresponds to a 10% mass concentration, and the particle flow rate of 5 × 10−5 kg/s corresponds to a 5% mass concentration.
4.2 Effect of slurry concentration on particle transport and media permeability
Figures 8A, B show the development of siltation inside the waste rock with different concentrations. In the case of the same calculation time, due to the large difference between the diameter of the waste rock particles and the diameter of the tailings particles, the tailings particles are able to enter into the waste rock very well, so the siltation development stage of the waste rock inside the slurry flushed by the concentration of 1 × 10−4 kg/s particles is faster than that of the 5 × 10−5 kg/s concentration. The siltation under the same simulation length is also more serious, and the permeability of the waste rock medium deteriorates faster. The distribution of tailings at different concentrations is compared with the simulated distribution of tailings. With the increased concentration, the tailings are more widely distributed in the length and height directions, and the blockage of the internal pore channels of the waste rock is more serious.
Figure 9A shows the mass distribution of tailings accumulation in the length direction inside the waste rock under different concentrations. The mass distribution of tailings in the waste rock is analyzed in Figure 9A. Both concentrations increase near the end of the waste rock tailings residue. The distribution of the length direction decreases gradually as the slurry from the seepage moves into the seepage process. Figure 9B shows that the tailings particles are constantly captured by the waste rock pore space so as to incur agglomeration. However, some flocs have too little attraction to each other, and the flocs will break up in the seepage process. Through the simulation, it was found that a large number of tailings particles were intercepted near the nonwoven fabric and inside the waste rock, which verified the effectiveness of the combination of waste rock and nonwoven fabric on the interception of tailings particles, and thus provided a basis for the storage of tailings in the waste rock dyke network. According to Figures 9A, 10A, the higher the concentration of tailings particles on the nonwoven fabric, the more intense is the blockage. The mass of tailings particles deposited near the nonwoven fabric at a concentration of 1 × 10−4 kg/s is 2.26 times that of the concentration of 5 × 10−5 kg/s, and more particles are blocked on the top of the nonwoven fabric, which leads to the more serious decrease in the nonwoven fabric’s water permeability performance. Meanwhile, the seepage of the internal pore channels of small-concentration waste rock is better, and the particles of the latter part with a higher concentration are lost rather than those with a low concentration. The loss rate is lower than the lower concentration, and the overall particle loss rate is approximately twice that of C = 0.05 g/s.
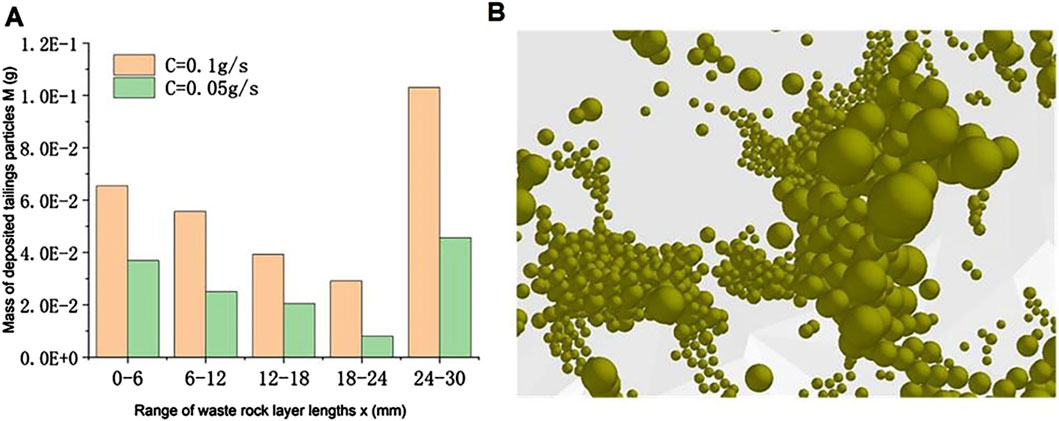
Figure 9. Tailings accumulation mass distribution and particle agglomeration state. (A) Tailings accumulation mass distribution in the direction of length of waste rock with different concentrations and (B) tailings particle agglomeration state.
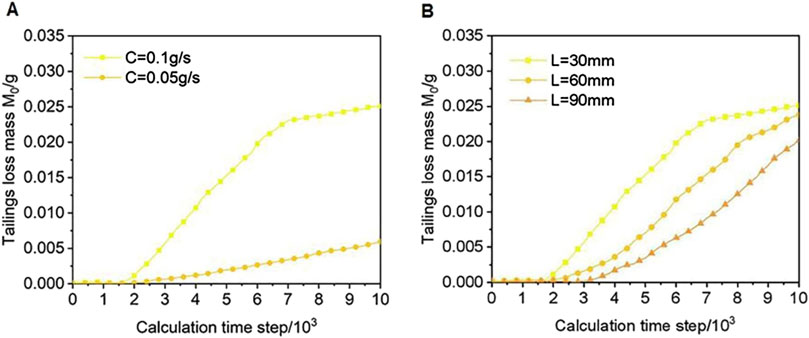
Figure 10. Tailings particle loss. (A) Loss of tailings particles at different concentrations and (B) tailings particle loss for different waste rock lengths.
4.3 Effect of waste rock layer length on particle transport and media permeability
Comparison of Figures 8A, 11A shows the penetration of waste rock tailings under different waste rock media lengths. The whole section of the waste rock media layer with the short media length was silted up, while the 90 mm long waste rock layer was silted up only at the left end. The 30 mm, 60 mm, and 90 mm waste rock lengths corresponded to the mass of tailings that did not enter into the waste rock layer, which were 1.74 × 10−4 g, 2.07 × 10−4 g, and 2.17 × 10−4 g, indicating that the longer the length of waste rock, the more difficult it is for the tailings to enter the waste rock. The total tailings content in the statistical waste rock was 2.925 × 10−4 g, 2.812 × 10−4 g, and 2.724 × 10−4 g, respectively. The difference in the amount of tailings deposited inside the waste rock was not large, but the increase in the length of the waste rock media improved the ability of the internal pore channels to store tailings, so for the same quality of waste rock, the particles-into-siltation performance declined less.
Figure 10B shows the tailings particle loss and loss rate. In the waste rock length of 30 mm, the beginning stage of tailings loss is more serious. After a period of time, the rate decreases significantly, indicating that tailings particles in the interior of the waste rock, especially in the waste rock and nonwoven fabrics, form more serious siltation. While the length of the waste rock particles loss curve is still rising, its siltation is not yet very serious. From Figures 12A, B, it is seen that the longer the length of the waste rock medium, the tailings are more widely distributed in the longitudinal direction. The larger tailings particles have been captured by the waste rock body before reaching the seepage outlet, so there are fewer tailings particles deposited in the vicinity of the waste rock and the nonwoven fabric, and the overall permeability performance is maintained at a better level. The pore channel’s ability to perform tailings transport is better preserved, and the loss of tailings particles of the longer lengths of the waste rock is seen to be still growing at the end of the simulation. This model maintains an increase, while the shorter models show a decreasing trend. Compared with the test tailings distribution, the simulation results are similar to the test accumulation: tailings particles accumulate more in the bottom of the waste rock and less in the upper part. The longer the length of the waste rock, the more likely are the tailings particles to be dispersed uniformly in the longitudinal direction at the bottom of the waste rock. There are still many pore channels in the upper part for the filtrate as well as the tailings particles to be filtered out, so after a period of time, the filtration rate of the longer media is faster.
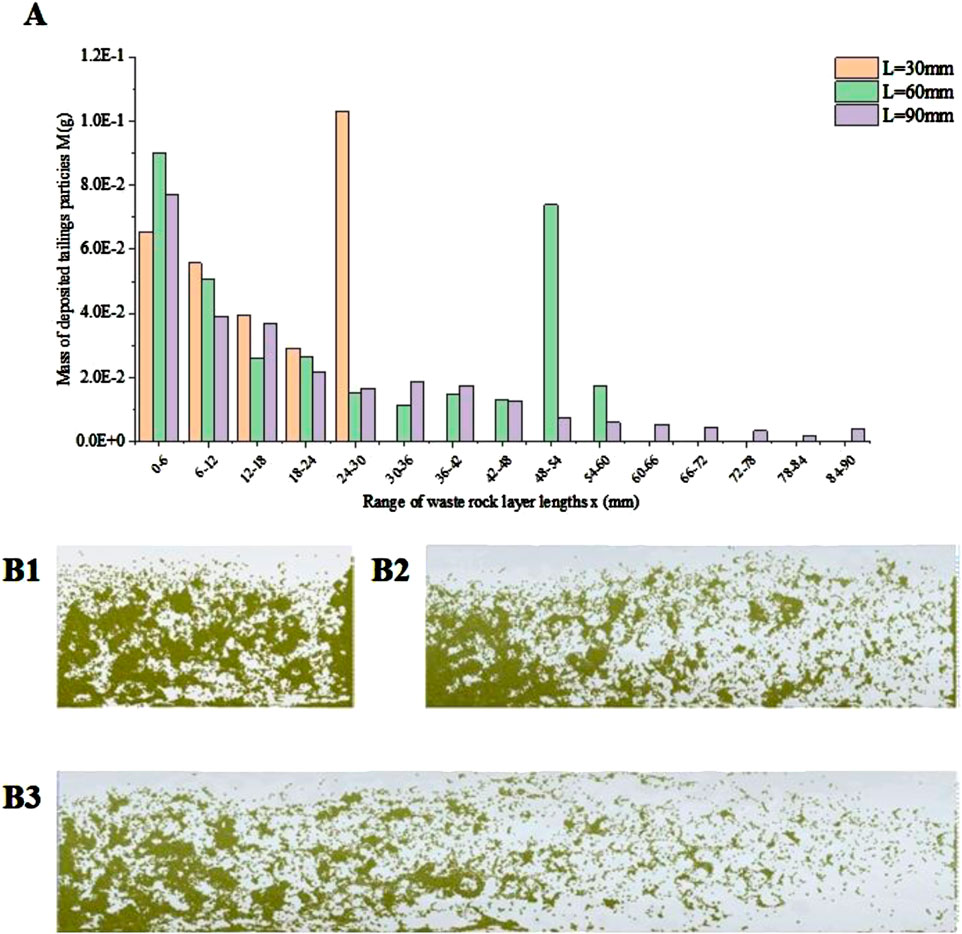
Figure 12. Distribution of tailings within different waste rock lengths. (A) Distribution of tailings accumulation mass and (B) distribution of tailing.
5 Limitations
This article conducted indoor model experiments and numerical simulations on the influencing factors of horizontal infiltration tailings contact loss in the waste rock medium layer, which can provide reference and guidance for the waste rock embankment storage processes. However, the real waste rock embankment tailings dam is a complex structure, and the indoor model experiments have certain differences from the actual infiltration process of waste rock embankment tailings. Therefore, some issues need to be improved:
(1) The physical model testing equipment requires the development of a three-dimensional testing device that can dynamically observe and collect data samples in real time. It can automatically collect indicators such as seepage velocity, pressure changes, flow rate, and tailings concentration changes and has good working stability and data accuracy under general testing conditions. At a later stage, with the help of CT scanning, it is hoped to obtain accurate information on the distribution of tailings in the waste rock, as well as to analyze the porosity of the waste rock.
(2) Due to the small diameter of tailings particles, the number of simulations can reach hundreds of thousands, and the simulation time cannot be too long. The model was scaled during the simulation. With the advancement of technology and the improvement of calculation methods, longer simulation times can be planned in the future to achieve full-scale simulations. In addition, the actual tailings particles are flaky, and the waste rock morphology is also more complex, but in order to improve the computational efficiency of the simulation, a spherical shape is used to model the waste rock particles.
6 Conclusion
In this paper, the migration and deposition characteristics of tailings particles in the waste rock media layer are investigated through experiments. The results of several sets of tests under different slurry concentrations and different waste rock media lengths are analyzed and discussed, and the development mechanism of infiltration and deformation is analyzed in terms of cumulative filtrate mass, filtration rate, siltation, etc. Finally, numerical simulation using the coupled method of fluid and particulate flow (CFD-DEM) is carried out to verify the obtained conclusions as follows:
(1) When the length of waste rock media is the same, with the increase in the mass concentration, the less cumulative filtrate mass, the earlier and lower the peak filtration rate appears, and the lower the filtration rate in the stable filtration stage. At the early stage of seepage, under the hydraulic gradient, the fine particles of waste rock are deformed and reorganized to rapidly form a seepage channel, and the filtration rate increases very quickly. After the basic formation of the seepage channel, siltation continues to occur, the filtration speed decreases from fast to slow, and the tailings particles and the waste rock together form a stable filtration media layer.
(2) When the slurry concentration is the same, the shorter the length of the waste rock medium, the earlier the filtration rate starts and reaches the peak rate, and the corresponding peak filtration rate is lower. In the initial stage of seepage, the shorter the length of the waste rock, the greater the hydraulic gradient, the faster the initial seepage rate, and the more tailings particles are retained per unit seepage length. The greater the hydraulic force received by the tailings, the easier it is for them to invade the interior of the waste rock and cause blockage. The longer the length of the medium, the smaller the hydraulic gradient, and the less hydrodynamic force the tailings particles are subjected to. Therefore, more particles remain near the contact surface between the slurry and the waste rock medium layer, and they are deposited in the tailings tank under gravity instead of entering the pores inside the waste rock medium section, making it less prone to clogging. The stable filtration performance of the filter medium layer deteriorates less, and the permeability coefficient remains better, resulting in a higher filtration rate after stabilization.
(3) In the filtration rate decline phase, there will be a sudden increase in the rate of the local water pressure increases, leading to the formation of tailings particles flocculation structures or honeycomb structures collapse, causing a filtration rate surge.
(4) The coupled CFD-DEM numerical simulation method can be used to simulate the development process of infiltration deformation of waste rock tailings under different mass concentrations and waste rock lengths and to analyze the connection between macroscopic and microscopic variables and phenomena in the development process of contact loss from the microscopic point of view. The results of the 3D dynamic simulation analysis both supplemented the model test and verified the reliability of the test results.
(5) The contact loss process of waste rock tailings stockpiled in the waste rock dyke network is systematically investigated through indoor physical modeling and numerical simulation analysis. Different particle size characteristics of waste rock and well-graded nickel tailings are used to study the development mechanism of contact loss of waste rock and tailings and the deterioration of permeability of the medium to provide a certain scientific basis and theoretical foundation for the design and construction of waste rock dyke network stockpile tailings storage projects.
Data availability statement
The original contributions presented in the study are included in the article material, further inquiries can be directed to the corresponding author.
Author contributions
MS: conceptualization, writing – review and editing, and writing – original draft. MO: writing – original draft and writing – review and editing. JX: formal analysis, validation, and writing – original draft. SW: data curation, software, and writing – original draft. ZL: resources, visualization, and writing – original draft. XL: methodology, project administration, and writing – review and editing.
Funding
The author(s) declare that financial support was received for the research and/or publication of this article. This study was supported by the National Natural Science Foundation of China (52069013 and 52169027), which is gratefully acknowledged.
Conflict of interest
The authors declare that the research was conducted in the absence of any commercial or financial relationships that could be construed as a potential conflict of interest.
Publisher’s note
All claims expressed in this article are solely those of the authors and do not necessarily represent those of their affiliated organizations, or those of the publisher, the editors and the reviewers. Any product that may be evaluated in this article, or claim that may be made by its manufacturer, is not guaranteed or endorsed by the publisher.
References
Ahfir, N. D., Benamar, A., Alem, A., and Wang, H. Q. (2009). Influence of internal structure and medium length on transport and deposition of suspended particles: a laboratory study. Transp. Porous Med. 76 (2), 289–307. doi:10.1007/s11242-008-9247-3
Chen, X., and Bai, B. (2012). Effect of gravity on transport of particles in saturated porous media. Chin. J. Geotech. Eng. 34 (09), 1661–1667.
Chen, X., Bai, B., Yan, Y., and Jia, D. (2012). Influence of concentration of suspended particles on transport and deposition characteristics in saturated porous media. Rock Soil Mech. 33 (08), 2343–2348. doi:10.16285/j.rsm.2012.08.010
Cui, Q. (2015). Research on the muddy water siltation mechanism and ItsEffect on tailings dam. North China University of Technology. master's thesis.
Dai, C., Qi, Y., Lei, H., Lli, Q., and Li, C. (2016). Deposition effect of suspended microparticle on the local permeability in porous media. Hydrogeol. Eng. Geol. 43 (06), 1–6. doi:10.16030/j.cnki.issn.1000-3665.2016.06.01
Jaouhar, E. M., Aubertin, M., and James, M. (2011). Effect of mine waste rock inclusions on the consolidation of tailings.
Jiang, Z., Yuan, T., Liu, D., and Li, X. (2018). Mesoscopic numerical tests on seepage failure characteristics of coarse grained soils. Chin. J. Geotech. Eng. 40 (04), 752–758+706. doi:10.11779/CJGE201804020
Liu, Q., Cui, X., and Zhang, C. (2015). Research advances in the characterization of transportation and deposition of suspenden particles inporous media. Chin. J. Rock Mech. Eng. 34 (12), 2410–2427. doi:10.13722/j.cnki.jrme.2014.1427
Liu, Q., Cui, X., Zhang, C., and Zhan, T. (2014). Effects of particle size on characteristics of transportation and deposition of suspended particles in porous media. Chin. J. Geotech. Eng. 36 (10), 1777–1783. doi:10.11779/CJGE201410003
Mohammed, S. A. S., and Moghal, A. A. B. (2016). Efficacy of nano calcium silicate (NCS) treatment on tropical soils in encapsulating heavy metal ions: leaching studies validation. Innov. Infrastruct. Solutions 1 (1), 21. doi:10.1007/s41062-016-0024-9
Nan, X., Liu, X., Wu, B., Zhang, H., Song, K., and Wang, X. (2023). Coupled CFD-DEM simulation and experimental study of particle distribution and accumulation during tailings seepage process. J. Clean. Prod. 427, 139229. doi:10.1016/j.jclepro.2023.139229
Onyelowe, K. C., Moghal, A. A. B., Ahmad, F., Rehman, A. U., and Hanandeh, S. (2024). Numerical model of debris flow susceptibility using slope stability failure machine learning prediction with metaheuristic techniques trained with different algorithms. Sci. Rep. 14 (1), 19562. doi:10.1038/s41598-024-70634-w
Shengo, L. M. (2021). Review of practices in the managements of mineral wastes: the case of waste rocks and mine tailings. Water, Air, and Soil Pollut. 232 (7), 273–331. doi:10.1007/s11270-021-05198-w
Song, Z., Wang, J., and Zhao, F. (2016). Influence on stability of tailings dam under muddy water infiltration. Met. Mine 05, 183–186. doi:10.3969/j.issn.1001-1250.2016.05.038
Wang, Y.-H., and Leung, S.-C. (2008). A particulate-scale investigation of cemented sand behavior. Can. Geotech. J. 45 (1), 29–44. doi:10.1139/T07-070
Wu, S. (2017). Effect of particle size on physico-MechanicalProperties of tailings and dam stability study. Chongqing University. dissertation.
Xu, S., Gao, B., and Saiers, J. E. (2006). Straining of colloidal particles in saturated porous media. Water Resour. Res. 42 (12). doi:10.1029/2006WR004948
Yin, G., Zhang, Q., Wei, Z., Wang, W., and Geng, W. (2012). Experlmentalstudy of migration characteristics of pore water and its effect on meso-structure of tailings. Chin. J. Rock Mech. Eng. 31 (01), 71–79. doi:10.3969/j.issn.1000-6915.2012.01.009
Zamani, A., and Maini, B. (2009). Flow of dispersed particles through porous media — deep bed filtration. J. Petrol Sci. Eng. 69 (1-2), 71–88. doi:10.1016/j.petrol.2009.06.016
Zhang, C., Qing, Y., Zhong, Q., and Wang, B. (2020). Permeability characteristics of silty sand under vertical and horizontal seepages. Chin. J. Geotech. Eng. 42 (S2), 163–167. doi:10.11779/CJGE2020S2029
Zhang, P., Bai, B., and Jiang, S. (2016). Coupled effects of hydrodynamic forces and pore structure on suspended particle transport and deposition in a saturated porous medium. Rock Soil Mech. 37 (05), 1307–1316. doi:10.16285/j.rsm.2016.05.012
Zhou, H., Dong, W., and Cui, X. (2017). A new method for Co-disposal of tailings and waste rock. Nonferrous Met. Min. Process. Sect. 03, 60–65. doi:10.3969/j.issn.1671-9492.2017.03.013
Zhu, B., Cao, X., Li, W., Deng, G., Zhang, Y., and Zhang, Y. (2020). Numerical simulation of seepage deformation process in poorly-graded coarse soil. CSTPCD 18 (03), 178–185. doi:10.13244/j.cnki.jiwhr.2020.03.003
Keywords: waste rock dyke network, infiltration deformation, waste rock media, CFD-DEM coupling, contact loss
Citation: Sheng M, Ouyang M, Xu J, Wang S, Lei Z and Liu X (2025) The influence of tailings concentration and waste rock medium length on horizontal seepage deformation. Front. Earth Sci. 13:1477752. doi: 10.3389/feart.2025.1477752
Received: 08 August 2024; Accepted: 18 February 2025;
Published: 07 April 2025.
Edited by:
Changshuo Wang, Ningbo University, ChinaReviewed by:
Arif Ali Baig Moghal, National Institute of Technology Warangal, IndiaXia Bian, Hohai University, China
Copyright © 2025 Sheng, Ouyang, Xu, Wang, Lei and Liu. This is an open-access article distributed under the terms of the Creative Commons Attribution License (CC BY). The use, distribution or reproduction in other forums is permitted, provided the original author(s) and the copyright owner(s) are credited and that the original publication in this journal is cited, in accordance with accepted academic practice. No use, distribution or reproduction is permitted which does not comply with these terms.
*Correspondence: Xinxin Liu, aHVhc2h1aWxpdXhpbkAxMjYuY29t