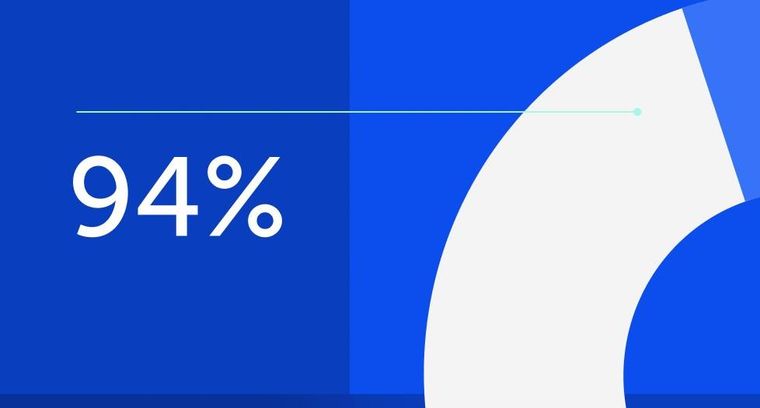
94% of researchers rate our articles as excellent or good
Learn more about the work of our research integrity team to safeguard the quality of each article we publish.
Find out more
REVIEW article
Front. Earth Sci., 17 March 2025
Sec. Quaternary Science, Geomorphology and Paleoenvironment
Volume 13 - 2025 | https://doi.org/10.3389/feart.2025.1477290
Since the early Neolithic (∼10,000 years ago), the Iranian Plateau has witnessed the development of sedentary human settlement facilitated by periods of favorable climatic conditions prompting gradual or sweeping changes. Climate factors significantly drove the hydroclimatic conditions in western and southeastern Iran, which varied in response to the Mid-Latitude Westerlies (MLW) and Indian Summer Monsoon (ISM). In addition, the input of dust and its eastward transport from the Arabian Peninsula and North Africa coincided with the North Atlantic cooling events. Peak wet conditions during the early Holocene in southeastern (c. 11.4–9.6 ka BP) and western Iran (c. 10.2–8.6 ka BP) indicate different timings in regional precipitation. The northward displacement of the Intertropical Convergence Zone at the beginning of the early Holocene caused the ISM to expand over southeastern Iran. At the same time, it strengthened the sub-tropical high-pressure and northward expansion over western Iran, resulting in dry conditions. Between 7.8 and 6.3 ka BP, gradual weakening and southward movement of the ISM and the decrease in intensity of the subtropical high-pressure systems over the Zagros region resulted in southeastern Iran becoming mild and the western region humid. Between 6.3 and 5.0 ka BP, a decrease in solar insolation ushered dusty and arid conditions on the Iranian plateau. Notably, human activities in the region started experiencing significant changes around the mid-Holocene. A concurrence exists during the wet (c. 5.0–4.5 ka BP) and dry (c. 4.2–3.2 ka BP) periods, coinciding with the rise and decline of multiple Bronze Age settlements. These settlements flourished in exchange and trade, pyro-technologies, and agro-pastoral production, demonstrating an increasing complexity in social organization and vulnerability to climate change. After transitioning into the Iron Age, southeastern Iran experienced relatively wet conditions c. 2.9 to 2.3 ka BP and 1.6 to 1.3 ka BP coincided with major territorial expansions and advancements under the Achaemenid and Sassanian dynasties. Merging the historical and archaeological data with palaeoenvironmental conditions indicates a concurrence of unfolding climatic and cultural changes, suggesting cascading effects that led to growth or settlement decline and abandonment.
The hydroclimate on the Iranian Plateau exhibits intricate dynamics resulting from the interplay between several atmospheric systems, namely, the Mid-Latitude Westerlies (MLW), the southwest Indian Summer Monsoon (ISM), and the northeast Siberian Anticyclone, showcasing the complexity of the climate systems. (Figure 1A). These complex interactions have significantly influenced the climate and hydrological patterns across the Iranian Plateau since the end of the Last Glacial Maximum (LGM) c. 20 ka BP (Sharifi et al., 2015; Vaezi et al., 2019). Moreover, changes in fluvial discharge and fluctuations in lake levels played a major role in shaping the bio-climatic zones on the Iranian Plateau (Djamali et al., 2010a). These changes are argued to have influenced the emergence and subsequent development of urban societies stretching from Western Asia to the Indus Valley (Staubwasser and Weiss, 2006; Sharifi et al., 2015; Petrie, 2011; Matthews, 2013; Weiss, 2016; Petrie and Weeks, 2018; Sinha et al., 2019; Marchetti et al., 2024). However, this relationship remains complex and the subject of ongoing debate, underscoring the need for further research (Middleton, 2018; Middleton, 2024).
Figure 1. Major climate systems in West Asia (Sharifi et al., 2015; Vaezi et al., 2019) and the location of Bronze Age settlements in SE Iran. (A) The red and blue lines in panel A depict the estimated present-day position of the Intertropical Convergence Zone (ITCZ) and its location during the Early Holocene, respectively. The abbreviations ISM, MLW, and SH correspond to the Indian Summer Monsoon, Mid-Latitude Westerlies, and Siberian High Anticyclone, respectively. Black stars indicate the locations of palaeoenvironmental studies, primarily along the Zagros Mountains and southeastern Iran. (B) Map of the Iranian Plateau showing the locations of archaeological sites: 1- Godin Tepe, 2- Tepe Giyan, 3- Baba Jan, 4- Kalleh Nisar, 5- Bani Surmah, 6- Mir Kheir, 7- Kunji Cave, 8- Deh Luran, 9- Susiana, 10- Tepe Kopandeh, 11- Deh Dumen, 12- Tal-i Malyan, 13- Tol-e Nurabad, 14- Tal-i Bakun/Tal-i Mushki, 15- Hormangan, 16- Tom-e Gavan, 17- Shahr-i Sokhta, 18- Shahdad/Tepe Dehno/Mokhtarabad, 19- Tal-i Iblis, 20- Tal-i Atashi, 21- Jiroft/Konar Sandal/Keshit, 22- Tepe Gav Koshi, 23- Hajiabad Varamin, 24- Tepe Gaz Tavilla, 25- Tepe Yahya, 26- Shamsabad. Principal regions mentioned in the text are marked with black circles. Modern provinces are labeled with grey letters and indicated with five-sided polygons.
The climates of western and southeastern Iran, the two focus regions in this review, exhibit significant differences due to their distinct geographical and topographical features. Western Iran, characterized by the mountainous Zagros range, experiences a Mediterranean climate with hot, dry summers and cool, wet winters, receiving 400–800 mm of annual precipitation mainly from October to April. This climate supports diverse ecosystems, including forests and grasslands. In contrast, southeastern Iran is defined by arid desert landscapes, primarily the Lut Desert. It features a desert-type climate marked by extreme summer heat exceeding 50 °C with minimal rainfall (50–250 mm annually), resulting in limited biodiversity dominated by drought-resistant vegetation. Overall, western Iran has higher humidity and more consistent rainfall, while southeastern Iran is mainly dry and subject to erratic precipitation patterns.
The palaeoenvironmental reconstruction of Iran, particularly the Zagros region, has played a pivotal role in providing valuable insights into the ongoing discussion surrounding the influence of climate change on the Neolithic transition in West Asia. The unique features and significant role of the Zagros region underscore its importance in the influence of climate change on human history (Petrie and Weeks, 2018; Fazeli Nashli and Matthews, 2013; Matthews and Fazeli Nashli, 2022). It helps understand the societal changes during the early Holocene when humans transitioned from mobile hunter-gatherer lifestyles to largely sedentary farming and herding communities.
The Chalcolithic societies of the seventh and sixth millennia BP, through their consolidation of the lifeways of their Neolithic forebears, established denser, sometimes hierarchical settlement systems. These were built on integrated agro-pastoral production and the development and mastery of specialized crafts producing ceramics and metals (Matthews and Fazeli Nashli, 2022). These significant developments laid the foundations for the emergence of the Early Bronze Age (EBA) settlement on the Iranian Plateau towards the end of the sixth millennium BP. The EBA settlements, with their crucial role in exchange and trade, agriculture, and cultural advancements, were instrumental in shaping the so-called ‘Middle Asian Interaction Sphere’ (MAIS; Possehl, 2007) that linked societies from Mesopotamia to the Indus Valley and from the shores of the Persian Gulf to Central Asia in the fifth millennium BP (Madjidzadeh and Pittman, 2008; Mashkour et al., 2013; Mutin and Minc, 2019; Beni et al., 2021; Matthews and Fazeli Nashli, 2022). Around 4 ka BP, the MAIS began to disintegrate, ushering in a period characterized by de-urbanization and, in some places, the near-total disappearance of sedentary sites during the fourth millennium BP (Petrie and Weeks, 2018). The subsequent Iron Age witnessed the (re-) emergence of towns and cities. These urban centers integrated under the imperial Persian dynasties and were the catalysts of significant historical and cultural events in this region (Staubwasser and Weiss, 2006; Mancini-Lander, 2009; Sharifi et al., 2015; Cronin, 2021).
Throughout these developments involving shifts in agro-pastoral strategies, alterations in social and political structures, advancements in water management techniques, alterations in land use, and partial or largescale population migrations, the Iranian Plateau experienced ongoing climatic changes and phytogeographic shifts which greatly influenced the potential for human settlement in this region (Mashkour et al., 2013; Sharifi et al., 2015; Gurjazkaite et al., 2018). The transformation in the area dramatically impacted the environment and social structures. This concept has been highlighted in several pre-modern societies, such as the Mayans, Norse Greenlanders, and Easter Islanders, affected by environmental and climate change (Diamond, 2005). Weiss and Bradley (2001) focused on droughts triggering societal disruptions in Mesopotamia and the Near East. Such disruptions are often labeled as instances of societal ‘collapse,’ defined as a substantial, relatively rapid, and lasting decline in an established level of socio-political complexity, indicated by reduced population, social stratification, economic specialization, centralized control, and trade/exchange networks (Tainter, 1988; Diamond, 2005). Current discussions of ‘collapse’ recognize the challenges in defining the term and highlight the significant elements of continuity that characterize societies once described as having collapsed. These debates emphasize the need for multifactorial and multi-dimensional explanations influenced by environmental, cultural, and political dynamics and historical contingencies (e.g., Middleton, 2018; Middleton, 2024). The idea that adverse climate events universally triggered a cultural collapse in premodernity does not match the available archaeological evidence from Western Asia, or indeed across the globe, and represents an oversimplification of collective events that were always multi-faceted and nuanced (Butzer and Endfield, 2012; Haldon et al., 2018). Nevertheless, the literature review indicates that despite claims that greater social complexity supported an increasing decoupling of demographic and climatic trends since the mid-Holocene (Palmisano et al., 2021), the variable environmental settings of ancient agro-pastoral communities across Western Asia regularly exposed them to climate change. This seemingly affected social resilience and influenced urban and demographic cycles during the later periods (Fleitmann et al., 2022; Marchetti et al., 2024).
In Western Asia, researchers have examined abrupt events to explore the causality between climate and historical/social changes (e.g., Staubwasser et al., 2003; Thompson, 2006; Sharifi et al., 2015; Marsh et al., 2018; Jones et al., 2019; Sinha et al., 2019; Lawrence et al., 2022). Until recently, studies in Iran focused primarily on western Iran, especially the Zagros region (Djamali et al., 2008; Kehl, 2009; Nicoll and Küçükuysal, 2013), resulting in a lack of comprehensive geographic coverage. Additionally, excavations of prehistoric and early archaeological records face persistent challenges due to the varying survival rates of artifacts, including provenance and inferences drawn from various categories of archaeological evidence (Petrie and Weeks, 2018). Consequently, when examining different sites within a broader geographical scope, diverse growth patterns, decline, settlement, or abandonment can confound, tracing the subtle societal changes that have occurred over time (Petrie and Weeks, 2018; Eskandari, 2021a). This situation is further complicated by the proportionally low and uneven coverage of excavated archeological sites within Iran, making it challenging to build a broad, integrated, and reliable picture of settlement patterns. In particular, it is difficult at some sites to distinguish the effects of human activities and climate on palaeovegetation histories (Djamali et al., 2010b; Roberts et al., 2011; Petrie and Weeks, 2018). Nevertheless, some high-resolution multi-proxy palaeoclimate studies conducted in the Zagros region and southeastern Iran are particularly significant due to the presence of early human settlements that originated in these regions (Djamali et al., 2009a; Djamali et al., 2009b; Jones et al., 2015; Sharifi et al., 2015; Gurjazkaite et al., 2018; Petrie and Weeks, 2018; Safaierad et al., 2020; Vaezi et al., 2019; Vaezi et al., 2022; Matthews and Fazeli Nashli, 2022; see Table 1; Figures 2, 3). These studies are valuable for reconstructing palaeoclimate conditions and their potential to align these climate records with archaeological findings, providing valuable insights into the historical and cultural contexts of early human settlements along the Iranian borderlands. Reviewing these palaeoclimate studies in western and southeastern Iran could help synthesize spatiotemporal changes over larger geographic areas, providing a foundation for understanding the emergence of urban settlements and the development of exchange, trade, and cultural networks during the Bronze and Iron Ages.
Figure 2. Summary of palaeoenvironmental changes for the last 20 ka in western and southeastern Iran (see Table 1; Figure 1 for site locations and supporting references). (A) Comparison of palaeoclimate conditions inferred based on different proxies from the Gol-e Zard Cave (Carolin et al., 2019), Lake Parishan (Jones et al., 2015), Sibaki Cave (Soleimani et al., 2023), Lake Zeribar (Stevens et al., 2001), Lake Mirabad (Griffiths et al., 2001), and Lake Neor (Sharifi et al., 2015) in western Iran impacted by MLW and Mediterranean palaeoclimate records from Lake Tecer (Kuzucuoğlu et al., 2011), stalagmites in Soreq Cave (Bar-Matthews et al., 2003; Bar-Matthews et al., 1999), and Dead Sea (Migowski et al., 2006). (B) Palaeoclimate data from the Jazmurian Playa (Vaezi et al., 2019), Konar Sandal peat bog (Safaierad et al., 2020), and Daryache peat bog (Vaezi et al., 2022) in southeastern Iran are affected by both ISM and MLW. Summary of regional archaeological chronologies of the Iranian Plateau (Jones et al., 2019). The blue and red bars represent considerable wet and dry periods in western and southeastern Iran, which are correlated with global climatic events: LGM, Last Glacial Maximum; HS1, Heinrich Stadial 1; YD, Younger Dryas; B/A, Bølling–Allerød interstadial; PBO, Pre-boreal oscillation; and the 8.2, 4.2, 3.2 events. These periods are also aligned with the cultural history. Due to academic conventions, the diagonal lines indicate variability in the start and end dates of named periods across different regions. Additionally, significant differences are noted in the onset times of certain periods, particularly the Neolithic, across various areas of Iran.
Figure 3. Comparison of dry and wet periods and agricultural practices in western and southeastern Iran along with historical and archaeological accounts of climate change and cultural transitions in West Asia since 5 ka BP. (A) Major ruling dynasties and cultural periods in Iran since the Early Bronze Age. The humid periods coincided with the most extensive territories (Vaezi et al., 2022) under the Achaemenid rule at its peak c. 2430 BP (Mcdonough, 2011; Colburn, 2013) and Sasanians c. 620 AD (Turchin et al., 2006), respectively. (B) The blue and red bars represent the wet and dry periods in western and southeastern Iran. (C) Drought and historical records of famine from Iran, Mesopotamia (MSP), and Eastern Mediterranean (EM; Sharifi et al., 2015). For data on panel C, see Table 2. (D) Abundance of Cerealia-type pollen as an index of intensive agricultural practices from the Daryache peat sequence in southeastern Iran (Vaezi et al., 2022), Lake Parishan in southwestern Iran (Jones et al., 2015), and Lake Almalou (Djamali et al., 2009b) in northwestern Iran. The upper graph shows cultivated trees, including Juglans, Olea, Vitis, Platanus, and Populus from Lake Almalou (Djamali et al., 2009b). The green bars represent peaks in agricultural activities.
By gathering palaeoclimate, palaeoenvironment, and palaeovegetation data focusing on western and southeastern Iran, we aim to construct a regional framework for climate change. This region witnessed the rise and fall of multiple early complex societies (Petrie and Weeks, 2018; Matthews and Fazeli Nashli, 2022; Figure 1). To understand these dynamics, we aim to build a regional framework incorporating data on climate change, settlement scale and density, social complexity, economy, and subsistence, allowing us to explore these interactions. The review focuses on the climatic conditions that prevailed/varied during the post-LGM and Holocene, setting the stage for human migration, cultural development, and the occurrence of early sedentary communities that flourished around the mid-to-late Holocene. The dynamics of this rich cultural heritage have been linked to climate change through a mosaic of interdisciplinary evidence gathered from the multi-proxy records of climate variability and climate-related historical events in archaeology, geochemical proxies (e.g., biomarkers, stable isotopes, elemental ratios), pollen records, sedimentology, and palaeoclimate modeling efforts (e.g., Griffiths et al., 2001; Stevens et al., 2006; Jones and Roberts, 2008; Djamali et al., 2009a; Djamali et al., 2009b; Djamali et al., 2010a; Djamali et al., 2010b; Jones et al., 2013; Jones et al., 2015; Sharifi et al., 2015; Gurjazkaite et al., 2018; Carolin et al., 2019; Safaierad et al., 2020; Safaierad et al., 2023; Vaezi et al., 2019; Vaezi et al., 2022). Certain periods and locations have been investigated extensively using multiple proxies, allowing for a more comprehensive understanding and enabling researchers to draw richer insights and detailed inferences. The proxies have been interpreted to provide valuable insights into the palaeoenvironmental changes (see Figures 2, 3), e.g., 1) indication of low lake level or lake level decline = dry conditions; 2) high lake level or lake level increase = wet conditions; 3) frequent floods = wet conditions; 4) negative δ18O in speleothems and ostracod shells = wet conditions; 5) positive δ13C in speleothem = dry conditions; 6) high percentage of oak-derived pollen = wet conditions; 7) high percentage of Artemisia pollen = dry conditions; and 8) aeolian layers in lacustrine and marine sedimentary profiles = dry conditions (Sharifi et al., 2015). However, the varying thresholds and sensitivities to moisture and temperature in different climate proxies and locations pose challenges to predicting regional palaeoclimate interpretations or generalizations.
Additionally, we examined the impacts of external climate shifts on subsistence, settlement patterns, and political structures associated with the mid-to-late Holocene, enhancing the empirical discourse. In doing this, the discussion delves into the intricate tapestry of climatic fluctuations, incorporating data from various sources to provide a nuanced understanding of historical climate dynamics and their impact on human settlements spanning millennia. We unravel the interplay between climate variations and cultural transitions toward more advanced phases by synthesizing evidence from existing literature on climate change and human settlements in the region and the prevalence of famines and droughts linked to climatic shifts in archeological and palaeoclimate studies (Figure 3; Table 2). By expanding the scope of regional data beyond the western and southeastern Iranian plateau, we aim to gain deeper insights into whether droughts, famines, and wet/dry conditions were widespread across the entire Middle East or primarily driven by localized factors.
Table 2. Records of drought and famine in Iran, Mesopotamia, and the eastern Mediterranean region inferred from archaeological and palaeoenvironmental studies since the fifth millennium BP.
The time frame in this interval extends from the latter part of the LGM, c. 20 ka BP, until 14.6 ka BP. Pollen records from Lake Zeribar (Van Zeist and Wright Jr, 1963; Van Zeist and Bottema, 1977), Lake Urmia in NW Iran (Bottema, 1986; Djamali et al., 2008), and Lake Van, Turkey (Wick et al., 2003) show similar assemblages, indicating the prevalence of the Iranian Turanian mountain steppe vegetation along the Zagros Mountains. A new multi-proxy palaeoenvironmental record from the Hashilan wetland in the central Zagros Mountains, western Iran (Safaierad et al., 2023), is consistent with previous evidence of pollen dominance of an Artemisia-Amaranthaceae dry steppe vegetation in the Zagros region (Van Zeist and Bottema, 1977; Djamali et al., 2008; Djamali et al., 2010a; Djamali et al., 2010b). These records indicate a cold and dry climate during the glacial period in the Zagros region, consistent with recent findings from southeastern Iran, which show severe droughts during the LGM and Heinrich Stadial 1 (Safaierad et al., 2023). The positive δ18O record from Lake Zeribar (Stevens et al., 2001) and stalagmites in Soreq Cave (Bar-Matthews et al., 2003; Bar-Matthews et al., 1999) between 19.0 and 16.6 ka BP (Figure 2) indicate dry and cool conditions. Likewise, stalagmites retrieved from Sibaki Cave in the southern Zagros Mountains of the Fars province have documented distinct growth hiatuses from 17.9 to 17.2 ka BP and from 15.3 to 14.7 ka BP (Soleimani et al., 2023), aligning with dry conditions (high δ18O and δ13C values) in Soreq Cave. The alignment of dry conditions on the Iranian Plateau with other regional archives indicates that arid conditions were widespread across the Middle East during the terminal Pleistocene.
The dust input from c. 18.0 to 16.5 and 15.1 to 14.6 ka BP in a peat bog in Konar Sandal, southeastern Iran, corresponds to the cold Heinrich events (Safaierad et al., 2020). Likewise, sharp increases in dust have been reported in other regional archives, e.g., the western Arabian Sea (Pourmand et al., 2007) and the western Mediterranean Sea (Rodrigo-Gámiz et al., 2011). The enhanced dust input at the onset of Heinrich Stadial 1a (Figure 2) aligns with the documented freshwater discharge from European ice sheets into the North Atlantic between 18.2 and 16.7 ka BP (Bond and Lotti, 1995; Toucanne et al., 2015; Safaierad et al., 2020). The decrease in aeolian activity in southeastern Iran after 16.5 ka BP (Safaierad et al., 2020) is consistent with the termination of freshwater discharge from European ice sheets (Bond and Lotti, 1995). Safaierad et al. (2020) proposed that the movement of sea ice to the northern regions and displacement of the Northern Hemisphere westerlies initiated this change, implying causality.
The dusty and dry conditions in southeastern Iran ended abruptly with the onset of the Bølling–Allerød c. 14.6 ka BP inferred based on the low dust input from 14.6 to 12.7 ka BP in Konar Sandal (Safaierad et al., 2020). During this period, the lack of evaporite minerals and the appearance of illite, along with very low aeolian inputs, suggest a more significant input of freshwater into the Jazmurian playa and wet conditions (Vaezi et al., 2019). The wet conditions in southeastern Iran coincided with increased summer monsoon intensity further east in the Arabian Sea (Overpeck et al., 1996). Comparing the Bølling-Allerød period to the late Pleistocene, the δ18O and δ13C values of the stalagmite in Sibaki cave were relatively lower, while the growth rates were higher (Soleimani et al., 2023). These findings suggest higher temperatures and/or shorter drip intervals, indicating wetter conditions in the region (Soleimani et al., 2023). Similar wet conditions have been recorded in the Socotra Island speleothem (Shakun et al., 2007) based on the negative δ18O values. The δ18O record in Lake Zeribar (Stevens et al., 2001) and Soreq Cave (Bar-Matthews et al., 1999; Bar-Matthews et al., 2003) gradually decreased from 16.6 ka BP onwards, reaching their lowest values at the onset of Bølling-Allerød and holding steady until 13.8 ka BP. Thus, wet conditions seem widely prevalent on the Iranian Plateau during the first half of Bølling-Allerød (Figure 2).
During the period (c. 13.5–11.7 ka BP), the Sibaki Cave experienced dry conditions and lower temperatures, supported by higher δ18O and δ13C values in the stalagmite (Soleimani et al., 2023). Growth hiatuses in the stalagmite further confirm the prevalence of dry conditions in southwest Iran during this period (Soleimani et al., 2023). More positive δ18O records from Lake Zeribar (Stevens et al., 2001) and Soreq Cave (Bar-Matthews et al., 1999; Bar-Matthews et al., 2003) imply that dry conditions prevailed in these areas, too. Likewise, the Lake Neor peat sequence in northwestern Iran was impacted by the MLW and indicated dusty and dry conditions over the region between 12.3 and 11.2 ka BP (Sharifi et al., 2015). The highest sedimentation rate correlates with the deposition of coarse sand and high magnetic susceptibility between 12.7 and 11.8 ka BP, indicating a high-energy environment and extensive erosion (Vaezi et al., 2019). Severe arid and dusty conditions during the Younger Dryas were present in southeastern Iran, as indicated by the high Zr/Al, Si/Al, and Ti/Al ratios (Vaezi et al., 2019; Safaierad et al., 2020). The weak ISM and low precipitation during this period were inferred based on the well-dated δ18O record in marine sediments from the western Arabian Sea (Sirocko et al., 1993). Similar arid conditions prevailed in other ISM-dominated regions, such as the Arabian Sea, Bay of Bengal (Chauhan, 2003), and the Indo-Gangetic plains (Sharma et al., 2004).
In Iran, the arid conditions highlighted by previous workers (Mayewski et al., 1997; Sharifi et al., 2015) coincided with the cold Younger Dryas in northwest Europe (Dansgaard et al., 1989). The North Atlantic cold events caused by the release of freshwater and the breakup of icebergs from the North American ice sheets (Hoff et al., 2016; Ng et al., 2018) impacted the input of dust over the Iranian Plateau (Sharifi et al., 2015; Safaierad et al., 2020). It is suggested that the enhanced interhemispheric temperature contrast during these cold events (Clark et al., 2012; Singarayer et al., 2017) forced the Atlantic meridional overturning (Denton et al., 2005) and ushered a weak ISM (Wang et al., 2001; Stager et al., 2011). This eventually resulted in dry conditions over the Iranian Plateau and reduced surface air temperature over the ice-covered ocean (Kutzbach et al., 2014).
Dusty and dry conditions during the Younger Dryas and the 8.2 ka event occurred due to the release of freshwater and icebergs from the North American ice sheets (Hoff et al., 2016; Ng et al., 2018) into the North Atlantic (Safaierad et al., 2020). This sudden change resulted in severe dusty and dry conditions over the Iranian Plateau (Sharifi et al., 2015; Vaezi et al., 2019; Safaierad et al., 2020). While freshwater and iceberg discharge from the European ice sheets led to a concomitant northward displacement of the Northern Hemisphere westerlies (McGee et al., 2010), it resulted in a gradual increase in aeolian activity over the southeastern Iranian Plateau at the onset of Heinrich Stadial 1 (Safaierad et al., 2020). The increase in dust from North Africa and the Arabian Peninsula during the North Atlantic cooling events since the LGM (McGee et al., 2013) occurred due to the southward shift in the MLW (McGee et al., 2010). The flow of strong Northern Hemisphere westerlies over the world’s largest dust bowls (i.e., North Africa and the Arabian Peninsula) during the North Atlantic cooling events, e.g., Heinrich Stadial 1, the Younger Dryas and the 8.2 ka event, resulted in huge dust plumes circling over the Iranian Plateau (Sharifi et al., 2015; Safaierad et al., 2020). It is proposed that the intensity of the northeastern Siberian Anticyclone directly impacted the increase in aeolian activity across northwestern Iran, especially during the Younger Dryas (Sharifi et al., 2015).
The archaeological record of Iran for the terminal Pleistocene is sparse and uneven and, in most instances, cannot support a close assessment of cultural adaptations to changing climate. The small numbers of known sites and patchy site distributions reflect several confounding factors – bias in survey coverage, limited fieldwork, poor preservation due to the age and ephemerality of most sites complicated by poor chronological control due to limited absolute dating - that mask cultural adaptation during this period (Shoaee et al., 2021).
In general, modern human (Homo sapiens) populations are argued to have arrived in Iran at c. 40–45 ka BP (Matthews and Fazeli Nashli, 2022), or possibly slightly earlier (Vallini et al., 2024), replacing existing Neanderthal (H. neanderthalensis) populations. This resulted in the characteristic cultural materials of the Iranian Upper Palaeolithic, typically referred to as Baradostian (in the central Zagros and northern Plateau) and Rostamian (in the southern Zagros). Upper Palaeolithic caves, rock shelters, and open-air sites are recorded in the central and southern Zagros, on the western edge of the Iranian Plateau, and the northern fringe of the central desert (Shoaee et al., 2021), and more recently in SE Iran (Anjomrooz et al., 2022). Upper Palaeolithic populations were mobile foragers who moved seasonally to access locally available plant and animal resources. Archaeological sites display preferences for hunting different wild animal species such as onager, sheep, goat, gazelle, boar, or deer (Matthews and Fazeli Nashli, 2022).
There appears to have been a multi-millennial gap, or at least a substantial reduction, in the human settlement of Iran at the end of the Upper Palaeolithic period, coinciding directly with the worsening climatic conditions of the LGM (Matthews and Fazeli Nashli, 2022; Shoaee et al., 2021: Table 1, Figure 11). However, human habitation can be documented in various parts of Iran from c. 20–10 ka BP, termed the Epipalaeolithic period, at sites characterized by variations on a distinctive ‘Zarzian’ lithic tradition (Olszewski, 2012; Tsuneki, 2013; Asouti et al., 2020; Shoaee et al., 2021: Figure 9). Epipalaeolithic groups continued a mobile hunting and gathering mode of existence that in the central Zagros is argued to represent moderately long seasonal occupations of caves and rock-shelters, alongside the use of task-oriented open-air camps (Asouti et al., 2020), although regional patterns of site use appear to have varied somewhat (Tsuneki, 2013; Rosenberg, 2003). Zooarchaeological and archaeobotanical studies in Palegawra (Iraq) indicate the exploitation of local grassland, woodland, and wetland habitats (Asouti et al., 2020) for a range of wild plants (grasses, legumes, nuts, and tubers) and wild animals (cattle, red deer, onager, fallow deer, boar, caprine, and gazelle). Contemporary Zarzian groups in the Iranian Zagros exploited an increasingly wide range of local wild animals, including cattle, sheep/goat, equids, gazelle, deer, pig, as well as land snails, birds, and fish (Shoaee et al., 2021). Late Epipalaeolithic sites in the southern Caspian region (e.g., the cave sites of Komishan, Kamarband, Hotu, and Ali Tepe), often termed ‘Mesolithic’, also showed evidence for exploitation of marine resources such as seal, which varied through time in ways that likely reflect the changing local environment and Caspian Sea levels (Matthews and Fazeli Nashli, 2022; Vahdati Nasab et al., 2019: Table 1).
During the early Holocene in southeastern Iran, wet conditions extended from c. 11.4–9.6 ka BP in the Jazmurian playa (Vaezi et al., 2019). The sharp decline in aeolian inputs (indicated by the low Zr/Al and Ti/Al ratios) combined with the absence of evaporites and increasing illite content support the shift in environmental conditions (Vaezi et al., 2019). The presence of illite suggests freshwater input, most likely due to an increase in precipitation. Similarly, in Konar Sandal, the low lithogenic abundance (Al, Ti, Si, and Zr) during the early Holocene and a rise in organic carbon suggest increased humidity in southeastern Iran (Safaierad et al., 2020). Consistent with this, evidence from other palaeoclimate archives, such as the Qunf cave in Oman (Fleitmann et al., 2003), marine sediments in the Arabian Sea (Gupta et al., 2005), and lacustrine sediments in northwestern India (Dixit et al., 2014) support changes during the early Holocene.
The Sibaki Cave records in SW Iran indicate a shift towards a more humid climate observed from 11.2 ka BP onwards that peaked at c. 10.7 ka BP (Soleimani et al., 2023). However, the peak wet conditions in southwest Iran appeared a few centuries before they manifested in west and northwest Iran but occurred a few centuries later than in southeast Iran. During the early Holocene, peak wet conditions dominated western and northwestern Iran c. 10.2 to 8.6 ka BP, while southeastern Iran experienced increased regional precipitation c. 11.4 to 9.6 ka BP. For example, in Lake Neor, the sharp decrease in aeolian activity and wet conditions started c. 10.2 ka BP (Sharifi et al., 2015). The delay in precipitation increased during the early Holocene and was documented in the δ18O records from Lakes Zeribar and Mirabad (Stevens et al., 2001; Stevens et al., 2006), indicating opposite climatic responses in western Iran. Nevertheless, this trend is consistent with changes in the Northern Hemisphere solar insolation (Sharifi et al., 2018; Safaierad et al., 2023). In contrast, the Hashilan wetland in western Iran experienced prolonged dry summers and frequent spring/summer dust storms during the early Holocene (Safaierad et al., 2023). These conditions led to seasonal wetland desiccation and delayed oak expansion in Zagros (Djamali et al., 2010a; Safaierad et al., 2023). The significant dominance of aeolian activity observed in western Iran during the onset of the early Holocene, as documented by Safaierad et al. (2023) and Sharifi et al. (2015), Sharifi et al. (2018), contrasts with high-resolution studies in southeast Iran (Vaezi et al., 2019; Safaierad et al., 2020), which do not report aeolian inputs.
With the onset of the early Holocene, high solar insolation led to the northward displacement of the ITCZ and its strengthening, resulting in an overall northward expansion of the ISM, as reported in many studies (Overpeck et al., 1996; Gupta et al., 2003; Fleitmann et al., 2007) and enhanced ISM precipitation in southeastern Iran (Vaezi et al., 2019; Safaierad et al., 2020). On the other hand, this shift in circulation patterns strengthened and extended the dominance of the sub-tropical high-pressure over western Iran (Djamali et al., 2010b; Hamzeh et al., 2016; Safaierad et al., 2023). Consequently, there were northward movements of the MLW storm tracks (Sharifi et al., 2018), resulting in enhanced aeolian inputs reported from the Hashilan Wetland (Safaierad et al., 2023). The atmospheric pressure in the Zagros region could cause a decrease in spring rainfall and an extended dry season (Djamali et al., 2010a).
Except for some short periods, c. 9.2 and 8.2 ka BP, the ISM remained strong over southeastern Iran until 7.8 ka BP when it began to shift southward gradually (Vaezi et al., 2019; Safaierad et al., 2020). The 8.2 ka BP cold and dry event, also known as the Bond Event 5, coincided with the injection of icebergs into the North Atlantic (Safaierad et al., 2020), which caused a southward shift of the ITCZ (Dixit et al., 2014), and abrupt weakening of ISM in the northern hemisphere (Gupta et al., 2003). This change was reflected in the extreme peaks of dust in southeastern Iran (Vaezi et al., 2019; Safaierad et al., 2020). This region influenced by ISM, is highly susceptible to shifts in the ITCZ. Consistent with these events, sudden dry periods during this time have been reported in speleothems from northern Oman and Yemen (Fleitmann et al., 2003; Fleitmann et al., 2007) and southeastern China (Dykoski et al., 2005) indicating the regional nature of these climatic shifts. This cold, dry event is also captured in the Mediterranean palaeoclimate records, e.g., a peak in dust inputs in Lake Neor (Sharifi et al., 2015) in western Iran and a sharp drop in the Dead Sea level (Migowski et al., 2006). The sudden weakening of the ISM circulation c. 8.2 ka BP led to a southward movement of dry MLW (Sharifi et al., 2018; Safaierad et al., 2023). This, caused extensive dust storms during winter in the entire region (both western and southeastern Iran; Sharifi et al., 2015; Sharifi et al., 2018; Safaierad et al., 2020) in the Middle East (Migowski et al., 2006). The arid condition confirms the influence of the North Atlantic fluctuations on decreasing ISM intensity in the Northern Hemisphere (Gupta et al., 2003).
The early Holocene marks an uptick in evidence of human activities in the region, although the archaeological record is still sparse. Although reliable dates are scarce, the Neolithic stages in Iran can be divided into aceramic (c. 11.5 ka BP) and ceramic (after 9.0 ka BP) phases that emerged in the Zagros mountains (Peasnall, 2002; Weeks, 2013; Petrie and Weeks, 2018; Matthews and Fazeli Nashli, 2022; Azadi et al., 2023). Evidence for Neolithic occupation c. 9.0 ka BP in southeast Iran is extremely scarce, comprising only the site at Tepe Gav Koshi in the Esfandageh region west of Jiroft. This was occupied initially from 9.0–8.7 ka BP and re-occupied towards the end of the ninth millennium BP, broadly coinciding with the 8.2 ka event. According to Fazeli Nashli and Matthews (2013), the Tepe Gav Koshi Neolithic site had some distinct characteristics, such as the use of obsidian and ceramic, the presence of mud-brick structures typical for Neolithic sites further to the west and north, and plant remains that exhibit consumption of pistachio nuts. There was little evidence of agriculture. Notably, the animal remains excavated are predominantly wild species (sheep and goats) hunted by the community. Overall, little connection between climate and cultural changes can be drawn from limited evidence beyond the basic observation that climate aridification did not drive the abandonment of the region.
Archaeological evidence in Western Asia indicates a significant impact of the 8.2 ka cold and dry event on human communities coinciding with abandonment and migration (Flohr et al., 2016; Safaierad et al., 2023). Archaeological records for this period in southern Iran are limited and characterized by highly uneven spatial coverage, making it challenging to connect changes in settlement patterns influenced by the 8.2 ka event. In the Kohgiluyeh region of the south-to-southwest Zagros mountains, traditional settled life began around the eighth millennium BP. Azadi et al. (2023) documented this emergence, revealing a gradual process of Neolithization in the area. The fertile lands were pivotal for the sedentary communities, and their subsistence strategies focused on a combination of cultivation, animal domestication, and herding. In addition, there appears to be a continuity of Neolithic settlement in the SW Iranian lowlands, as represented by material from Deh Luran and Susiana (Delougaz et al., 1996; Alizadeh, 2003; Alizadeh, 2008: 4–6, Table 1). In SW Iranian highlands, Neolithic communities dependent upon domesticated animals and plants and supplemented by hunting are known in small numbers from shortly before the ninth millennium BP, with continuity in settlement until around the eighth millennium BP (Alizadeh, 2021; Azadi et al., 2023). Here, the 8.2 ka BP event has been linked with changing subsistence practices, in particular, an increased reliance on hunting wild animals alongside the exploitation of domesticates at several sites (c. 8.4–8.0 ka BP). This is demonstrated by zooarchaeological remains from Tal-e Mushki and Hormangan and the lithic toolkit excavated at the latter site (Mashkour et al., 2006; Nishiaki, 2010; Abe and Khanipour, 2019).
After 7.8 ka BP, a change occurred in the vegetation cover from steppe to forest-steppe, coinciding with the continuous increase of oak forests (Van Zeist and Bottema, 1977; Djamali et al., 2008; Djamali et al., 2010a; Safaierad et al., 2023) around Lake Urmia (Bottema, 1986), Zaribar and Mirabad (Van Zeist and Bottema, 1977) in Iran and Lake Van in Turkey (Wick et al., 2003). These woodlands gradually expanded from 7.8 to 6.0 ka BP coeval to a decrease in aeolian input as the summer season progressively shortened (Djamali et al., 2010b; Safaierad et al., 2023). The long, hot, and dry summers due to the dominance of sub-tropical high-pressure systems in the Zagros region (Djamali et al., 2010a) is the most likely cause of this delay in the expansion of oaks during the Early Holocene (Djamali et al., 2010b; Safaierad et al., 2023). In addition, the expansion of oaks can be due to the decrease in the length of summer and an increase in spring rains in connection with the reduction in the intensity and duration of the sub-tropical high-pressure system over the Zagros region after 7.8 ka BP (Djamali et al., 2010a; Safaierad et al., 2023).
Gradually, with the progressive displacement of the ITCZ (Fleitmann et al., 2007; Gupta et al., 2003) followed by the sub-tropical high-pressure systems (Djamali et al., 2010b), it is expected that the MLW had more influence on southeastern Iran during winter. The observed delay in the decrease in humidity towards the end of the early Holocene in southeast Iran compared to other ISM-dominated areas has been reported (e.g., Hamzeh et al., 2016; Vaezi et al., 2019). This is because southeastern Iran continued to receive rain during both rainy seasons, supported by the different moisture sources, namely, the MLW and ISM. This interpretation aligns with the observed decrease in dust in southeastern Iran, particularly from 7.8 to 6.3 ka BP (Safaierad et al., 2020). However, geochemical proxies suggest that although Mediterranean precipitation had increased, the reduction in ISM rainfall was more significant, resulting in less rainfall than the wet conditions during the early Holocene.
Eighth millennium BP - This long period spanning c. 7.8–6.3 ka BP coincides with southern Iran’s late Neolithic and Chalcolithic periods. Around the eighth millennium BP, in SE Iran, the ceramic Neolithic occupation is more evident through surveys (Prickett, 1986a) and excavations such as Tepe Yahya, Tepe Gaz Tavila (Beale, 1986; Prickett, 1986b), and Tal-i Iblis (Caldwell, 1967). The Darestan region, east of Bam, hosted an extremely dense cluster of Neolithic settlements, with 64 sites identified—39 featuring Neolithic pottery and 25 displaying an aceramic Neolithic pattern similar to Tell-e Atashi (Mutin, 2022). This period marked the use of domesticated plants and animals, with settlements occupying alluvial terrace fans, a common practice for early agricultural communities (Petrie and Thomas, 2012). In the Shah Maran-Daulatabad basin, a cluster of Neolithic sites surrounded Tepe Gaz Tavila, forming agricultural villages unique to Kerman during this time (Prickett, 1986b). Conversely, no Neolithic settlements were recorded in the Halil Rud and areas south of Jiroft or the Bard Sir Valley. In the highlands of SW Iran, Neolithic settlement began expanding significantly during the eighth millennium BP. The Kur River Basin saw fewer than a dozen settlements at the start of the millennium to grow over 100 by the Shamsabad phase (c. 7.6–7.0 ka BP; Sumner, 1990). These settlements relied on small-scale irrigation from springs and dry farming (Sumner, 1994).
Seventh millennium BP - As the seventh millennium BP unfolded, the Neolithic settlement density increased further in SE Iran, particularly in the Shah Maran-Daulatabad basin, with significant growth during the Yahya VI-VB and VA periods (Prickett, 1986b). This growth coincided with agricultural practices shifting as settlements transitioned from alluvial fan margins to low-terraced fields irrigated by captured runoff. However, the Soghun Valley, with its more abundant rainfall, lacked evidence of such irrigation systems (Prickett, 1986b). In the Halil Rud region and areas south of Jiroft, Early Chalcolithic settlement began emerging with Yahya VB-VC pottery types from the late eighth to mid-seventh millennium BP (Pfälzner et al., 2019). Settlement density increased during the Middle Chalcolithic period (Yahya VA pottery) in the latter half of the millennium. In the Bard Sir Valley, a handful of Chalcolithic sites also appeared (Prickett, 1986b). In the highlands of SW Iran, the Chalcolithic period saw a rise in settlement in the Kur River Basin, particularly during the Bakun period (c. 7.0–6.4 ka BP; Taylor, 2015). Most settlements were small farming villages (>2 ha), though larger sites (6–8 ha) indicated the development of a settlement hierarchy (Taylor, 2015). Agricultural intensification, including irrigation and shorter fallow periods drove this growth (Sumner, 1990; Sumner, 1994).
Late seventh to Early sixth millennium BP - In SE Iran, Chalcolithic settlement patterns became dense in Darestan and nearby regions, with nearly 90 sites dating from c. seventh to sixth millennium BP (Mutin and Garazhian, 2019; Mutin, 2022). Further north, evidence of Chalcolithic occupation emerged in Shahdad region in the Lut Desert at sites such as Tepe Dehno and Tepe East Dehno (Eskandari, 2018). In the Kur River Basin of SW Iran, a decline in settlement during the Late Bakun period (c. 6.4–6.1 ka BP) suggested negative impacts of agricultural intensification, such as soil salinization (Sumner, 1990). This transition coincided with increasing emphasis on pastoralism, particularly sheep and goat herding, leading to greater mobility and the rise of nomadic pastoralism during the sixth millennium BP (Sumner, 1990; Sumner, 1994; Alden, 2013).
Sixth millennium onwards - By the sixth millennium BP, climate change, including the onset of arid conditions c. 6.3 ka BP began to impact settlement patterns and agropastoral practices in Iran. These climatic shifts contextualize the broader changes observed in Chalcolithic settlement during this period. Notably, no evidence of human occupation has been identified before the end of the sixth millennium BP in the Sistan region.
Between 6.3 and 5.0 ka BP, Iran experienced relatively drier and dustier conditions, which is consistent with a decrease in solar insolation (Gupta et al., 2003; Fleitmann et al., 2007) that affected most of the Northern Hemisphere (Sharifi et al., 2015). Lake Neor experienced periods of enhanced dust coinciding with the African Humid Period ending between 6.0 and 5.0 ka BP (Gasse and Van Campo, 1994; Sharifi et al., 2015). Around 6.3 ka BP, a return to arid conditions was reported in speleothem records from northern Oman (Fleitmann et al., 2003). Due to the concurrent decrease in solar insolation in the Northern Hemisphere, the ITCZ and ISM moved further south c. 6.3 ka BP (Fleitmann et al., 2003). Since then, the summer monsoon rains in southeastern Iran have decreased significantly. It is likely that areas like southeastern Iran, located along the northern border of ISM, experienced relatively less arid conditions compared to the southern regions due to a shift in rainfall from ISM to a MLW-dominated regime (Hamzeh et al., 2016; Vaezi et al., 2019).
The archaeological period from the mid-sixth to fifth millennium BP incorporates late Chalcolithic and Early Bronze Age developments in southern Iran. In the highlands of SW Iran, this is characterized by a steady decline of known sedentary sites, continuing from the Late Bakun period into the Lapui period (c. 6.1–5.6 ka BP) and the Early to Middle Banesh periods (c. 5.6–4.9 ka BP). By the Middle Banesh period, the site of Tal-e Malyan became the largest recorded and possibly the first ‘urban’ settlement in the Kur River Basin, covering an area of 50 ha (Sumner, 1990; Alden, 2013). In contrast, the total settled area in the Kur River Basin decreased to its smallest extent since the Neolithic period. Rather than regarding the settlement data as indicative of concurrent population decline, this period is hypothesized as one in which there was a large-scale transition from settled farming to nomadic pastoralism (Sumner, 1990; Sumner, 1994; Alden, 2013).
Settlement in southeastern Iran can be documented for the entire period from the early sixth to fifth millennium BP, with discernible fluctuations in sites and settled areas. Excavations and surveys in the Shah-Maran-Daulatabad region (Prickett, 1986a; Prickett, 1986b; Beale, 1986) showed a decline during the sixth millennium BP (Iblis IV/V period), followed by near abandonment at the end of the sixth millennium BP (Yahya IVC period). Prickett (1986a: 774) described this period as “characterized by the fragmentation and collapse of the long-developing prehistoric settlement pattern” in Shah Maran-Daulatabad, which was not resettled until the third millennium BP with the advent of qanat irrigation (Prickett, 1986a: 782). In the Soghun Valley, Tepe Yahya was abandoned for most of the sixth millennium BP, but a small number of other sites were documented in this region (Prickett, 1986a: 776). Tepe Yahya was re-occupied during the late sixth millennium BP (Period IVC), aligning with the Proto-Elamite culture across the Iranian Plateau. Further east in the Jiroft region, settlement of the sixth millennium BP was documented at Mahtoutabad (Periods I-III; Desset et al., 2013; Vidale and Desset, 2013), the Halil Rud, and areas south of Jiroft (Pfälzner and Soleimani, 2017; Pfälzner et al., 2019). These studies suggest a decline in sedentary settlement in the early sixth millennium BP, followed by a substantial increase in the mid-sixth millennium BP (Late Chalcolithic II), before another decline into the Late Chalcolithic period III (c. 5.4–5.2 ka BP) and the Early Bronze Age I or Proto-Elamite period (c. 5.2–4.9 ka BP). Further north in the Bard Sir Plain, there is a substantial increase in sites during the mid-sixth millennium BP (Iblis IV/V periods) before they decreased during the late sixth millennium BP (Prickett, 1986a: 784, Table. 7.1; Prickett, 1986b: 237). A reduction of settlement leading into the Proto-Elamite period (i.e., the Early Bronze Age) thus occurred in Bard Sir, Jiroft, Soghun, and Shah-Maran-Daulatabad regions of southeastern Iran, resembling the pattern observed in the Kur River Basin during the same period, as well as in other areas of Iran (Alden, 2013) indicating reduced sedentary settlement that coincided with the first significant period of (inter)cultural contacts across the Iranian Plateau.
The overall palaeoclimate reconstruction indicates wet conditions over the Iranian Plateau between 5.0 and 4.5 ka BP (Figure 3). The Early Bronze Age coincided with episodes of low dust and humid conditions in Lake Neor (Sharifi et al., 2015). Wet conditions were also recorded in Lake Zeribar, NW Iran, c. 5.1 to 4.9 ka BP (Stevens et al., 2008; Wasylikowa and Witkowski, 2008) and in Lake Maharlou, southwest Iran (Djamali et al., 2009a). Similarly, a wet period occurred in Lake Tecer, Turkey (Kuzucuoğlu et al., 2011), Lisan, and the Dead Sea lakes in the Jordan Rift Valley (Migowski et al., 2006), and in the eastern Mediterranean region (Robinson et al., 2006; Bar-Matthews and Ayalon, 2011). The existence of wet conditions in southeastern Iran between 5.0 and 4.5 ka BP is supported by geochemical evidence from the Jazmurian playa, showing a decrease in aeolian input and an abrupt increase in rainfall between 5.0 and 4.7 ka BP, likely due to the southerly movement of the westerlies (Vaezi et al., 2019). The southward movement resulted in intensified MLW-driven winter rainfall over the Iranian Plateau (Dehai and Jing, 2012), contributing to a more humid climate (Arsalani et al., 2018). This southward shift in westerlies sustained the wet period.
Urban communities first appeared in Sumer, in southern Mesopotamia, c. 5.5 ka BP, and flourished from c. 5.0 ka BP onwards when favorable climatic conditions and rain dominated the Middle East (Weiss et al., 1993; Staubwasser and Weiss, 2006). Similar arguments have been made for Iran in the Early Bronze Age. The wet conditions between 5.0 and 4.5 ka BP over the Iranian Plateau (Figure 2) coincided with the flourishing of Bronze Age settlements in southeastern Iran, as seen most clearly in Jiroft, Jazzmurian basin, the western Dasht-e Lut, and Sistan (Madjidzadeh and Pittman, 2008; Lawler, 2011). Bronze Age settlement declines in the Shah Maran-Daulatabad basin, and there are substantial periods of abandonment at Tepe Yahya and Tal-i Iblis after the fifth millennium BP (Prickett, 1986a; Potts, 2001; Mutin and Lamberg-Karlovsky, 2013). In contrast, the Jiroft region settlement expanded as indicated by the expansion in area of the Early Bronze Age sites of Konar Sandal South (Madjidzadeh and Pittman, 2008; Mashkour et al., 2013) and Hajjiabad Varamin (Eskandari, 2021a). Occupation of these sites, characterized by extensive evidence for specialized craft production in stone and metal, continued into the second half of the fifth millennium BP. A survey in the regions south of Jiroft (Pfälzner and Soleimani, 2017; Pfälzner et al., 2019) also documents a substantial increase in settlement from the sixth to fifth millennium BP.
In the western Dasht-e Lut, large-scale urban occupation developed from the late sixth millennium BP and peaked in the mid-late fifth millennium BP at sites such as Shahdad, which covered nearly 100 ha (Eskandari, 2021b). This urban site, located on the Takab alluvial fan, was characterized by a dispersed, non-nucleated settlement pattern (Eskandari, 2021b: 40), and relied on water from springs and streams. Contemporary large, isolated, Early Bronze Age urban sites were recorded further south at Mokhtarabad (c. 70 ha) and Keshit (c. 400 ha; Eskandari et al., 2014). These sites are characterized by substantial evidence of specialized craftwork in stone, metal, and ceramics of different provenance. Evidently, a network of exchange, and communication flourished along the western edges of the Dasht-e Lut (Eskandari, 2021a; Eskandari, 2021b).
In Sistan, Shahr-i Sokhta is settled for the first time towards the end of the sixth millennium BP (Period I), reaching its greatest extent (c. 150 ha) in the early-mid fifth millennium BP (Periods II-III), showing continuous occupation into the later fifth millennium BP (Period IV). Other Early Bronze Age settlements are also recorded in various parts of the Iranian Sistan region and along the Helmand region up to Mundigak in Afghanistan–comprising what has been referred to as the ‘Helmand Civilization.’
Favorable climatic conditions supported agricultural practices in these dry landscapes and brought prosperity to the Early Bronze Age societies of southeastern Iran. Although evidence is not available from all the sites, it is evident that Bronze Age settlements in the region depended on an integrated oasis agricultural system comprising irrigated gardens of domesticated date palms that provided both fruit and suitable shaded micro-climates for growing other crops (Tengberg, 2012). At Shahr-i Sokhta and Konar Sandal South, this subsistence adaptation incorporated the cultivation of dates, wheat, barley, pistachio, and grapes, alongside managing domestic goats, sheep, and cattle. At both sites, this diet was supplemented by the hunting of wild animals, and at Shahr-i Sokhta, by the exploitation of Lake Hamoun and its shorelines for the hunting of marsh birds and fishing (Mashkour et al., 2013; Gala and Tagliacozzo, 2014).
In the highlands of Fars, the decline in sedentary settlement accompanying the Middle Banesh period, with its Proto-Elamite material elements, continued up to the early fifth millennium BP (c. 4.9–4.6 ka BP). A very large wall, encircling up to c. 200 ha, was built around the key site of Tal-e Malyan, although it is unlikely that the entire area inside the wall was settled. However, this is the only site known in the Kur River Basin from this period. Thus, early urbanism in Fars is characterized by a “radically reduced” sedentary population followed by a nearly four-century-long near-abandonment of the region by agriculturalists from the mid-fifth millennium BP. Sumner (1989) ascribed this to extensive salinization of agricultural soils.
In Luristan, central Zagros, the early fifth millennium BP is associated with a virtual absence of sedentary settlement. The archaeological record for this period consists entirely of burials from sites such as Mir Khair, Kalleh Nisar, and Kunji Cave (Emberling et al., 2002). The long-lived tradition of isolated cemeteries continued through the fifth millennium BP in Luristan, as seen at the EBA II-III sites such as Bani Surmah and Kalleh Nisar, and also further south-east at Deh Dumen (Kohgiluyeh and Boyer-Ahmad Province). Although Hole (2007) has documented Early Bronze Age villages in the Khorramabad Valley in the mid-fifth millennium BP, he notes that “for long stretches of time, Luristan may have lacked permanent settlements.” The evidence from Bronze Age Luristan has suggested a dominant pastoral nomadic lifestyle during this period built on seasonal transhumance. Sedentary settlement emerged to the north and east, in Kermanshah and Hamadan Provinces, as at Tepe Giyan and Godin Tepe. The latter shows discontinuous occupation phases from the early fifth millennium BP (period IV, when the site was characterized by material of Early Trans-Caucasian type) and from the mid-late fifth millennium BP to the later fourth millennium BP (periods III:6-III:2) (Gopnik and Rothman, 2011).
The Early Bronze Age settlements observed in southeastern and southwestern Iran and the central Zagros region differ from each other and from that of the central Iranian Plateau. In the central plateau, the fifth millennium BP is associated with the disappearance of all sedentary settlement in areas such as Varamin, Qazvin, and Kashan (Schmidt et al., 2011; Matthews and Fazeli Nashli, 2022: 263; Kirsten et al., 2023; Pollock et al., 2023). A somewhat different pattern characterizes the Qom region to the south, where early fifth millennium BP settlement is documented at Qoli Darvish (Matthews and Fazeli Nashli, 2022: 263). The Early Bronze Age footprint is also seen in the Varzaneh region east of Esfahan, on the southern Central Plateau, documented in surveys that recorded c. 50 Early (and possibly Middle) Bronze Age sites at Tepe Kopandeh and elsewhere (Pollard et al., 2013; Rafiei-Alavi et al., 2023).
The wet period was followed by a shift to dry conditions between 4.5 and 4.0 ka BP (Table 2; Figure 3). The multi-proxy sediment records from the peat sequence in the Lake Neor catchment suggested dry conditions with high aeolian activity between 4.3 and 4.1 ka BP (Sharifi et al., 2015). The decline in lake levels and dry conditions have also been recorded during this period in Lisan and Dead Sea Lakes (Migowski et al., 2006), Lake Tecer (Kuzucuoğlu et al., 2011), and the Eastern Mediterranean region (Robinson et al., 2006; Bar-Matthews and Ayalon, 2011) indicating a regional prevalence and occurrence.
Abrupt climate events, such as sudden droughts occurring c. 4.5 to 4.0 ka BP are linked to a decline in population and social complexity, which may have contributed to the collapse of ancient civilizations in the Middle East (Degroot et al., 2021; Lawrence et al., 2022; see Table 2 for cultural shifts related to inferred paleoclimate conditions). Adapting to the changing environment likely became increasingly challenging for the agro-pastoral communities during this period. The vulnerability of the early communities to natural catastrophes, e.g., famines, droughts, and floods driven by climate change, is being increasingly recognized as a possible cause for these changes observed in cultural centers extending from Mesopotamia to China (Weiss et al., 1993; Staubwasser and Weiss, 2006; Zhang et al., 2008; Dixit et al., 2014; Sharifi et al., 2015; Sinha et al., 2019; Degroot et al., 2021; Laskar and Bohra, 2021). For example, investigations suggested that the fall of the Akkadian Empire c. 4.2 ka BP coincided with high aeolian activity (Weiss et al., 1993; Cullen et al., 2000; Lawrence et al., 2022). Severe droughts occurred in central Iran (Schmidt et al., 2011; Carolin et al., 2019) and Palestine (Issar and Zohar, 2007), coinciding with the decline in upper Egypt and Mediterranean regions. The decline could have happened due to climate variability sweeping through west Asia and further into the Indus Valley (Mashkour et al., 2013; Sharifi et al., 2015; Sarkar et al., 2016; Fallah et al., 2017; Carolin et al., 2019; Petrie and Weeks, 2018). This change is speculated to have affected agriculture, exchange and trade, and daily livelihoods, with even greater significance in southeastern Iran, where its location at the desert fringe makes it particularly vulnerable to climate fluctuations.
In recent years, researchers have aimed to pinpoint the timing of the abrupt increase in dry and dusty conditions in the Middle East, which coincided with significant societal changes c. 4.2 ka BP. Stalagmite records from Gol-e-Zard Cave in North Central Iran identified two distinct dry or dusty periods, occurring abruptly: c. 4.51 to 4.40 ka BP and 4.26 to 3.97 ka BP (Carolin et al., 2019). Notably, urban settlements in northern Mesopotamia were abandoned c. 4.19 ka BP, coinciding with the fall of the Akkadian Empire, and there were no signs of repopulation until c. 3.9 ka BP (Carolin et al., 2019). The study established a strong correlation between a significant dust event c. 4.26 ka BP and the collapse of these settlements, suggesting that these regions were vulnerable to aridity, as both phenomena lasted approximately 290–300 years. The 4.2 ka BP event resulted in a significant decline in rainfall and a decrease in discharge from the Indus River, located in a region susceptible to changes in the ISM rainfall (Giesche et al., 2019). This change aligned with the decline of the urban phase of the Harappan civilization (Kotlia et al., 2018; Giesche et al., 2019). Likewise, a speleothem from the Dharamjali Cave in the Himalayas indicates 230 years of intensified drought c. 4.2 to 3.97 ka BP, with notable dry episodes occurring c. 4.19, 4.11, and 4.02 ka BP (Giesche et al., 2023).
Shahdad was largely abandoned at the end of the fifth millennium BP, an event that has been tentatively linked with a significant episode of flooding at the site related to the 4.2 ka BP event and associated climatic variability (Eskandari, 2021b). However, graves excavated at the site suggest continued human presence into the early centuries of the fourth millennium BP (Eskandari, 2021b). While the 4.2 event is characterized as an arid phase (Staubwasser and Weiss, 2006), the prevalence of floods raises questions. It is plausible that deforestation linked to aridification contributed to more pronounced flooding during peak rainfall. Shahr-i Sokhta appears to have been destroyed and abandoned mainly towards the end of the fifth millennium BP, although there is still some debate about the chronology (Helwing et al., 2019; Biscione and Sajjadi, 2022).
The downturn in climate towards the end of the Early Bronze Age has been regarded by many as critical to the decline/collapse in settlement, especially large urban sites, that characterized eastern Iran during the fourth millennium BP (Madjidzadeh and Pittman, 2008; Magee, 2013; Pittman, 2013; Gala and Tagliacozzo, 2014; Gurjazkaite et al., 2018; Biscione and Vahdati, 2020). Although the timing of these developments is not strictly synchronous, and regional and site-level variations can be seen, this situation is broadly described by Biscione and Vahdati (2020), who note “the abandonment by 1800/1700 BC of almost every proto-urban center east of the Tehran-Bandar Abbas line”. This picture contrasts significantly with other areas of Iran, including the southwestern regions of Fars and Khuzestan, the central Zagros region (Luristan, Kermanshah), and the Central Iranian Plateau. The resurgence of sedentary sites in the Kaftari period (c. 4.2–3.6 ka BP) marks the region’s densest pre/proto-historic settlements phase. Kaftari settlements relied on organized riverine irrigation systems and produced an agricultural surplus to engage with local mobile pastoralists. Analyses of charcoal deposits from the Banesh and Kaftari periods at Tal-e Malyan (Miller, 1985) indicated long-term vegetation changes across the fifth millennium BP, including deforestation of the Kur River Basin and the need to expand fuel procurement from the surrounding mountains.
This is recorded as a wet period in Lake Zeribar (Stevens et al., 2008; Wasylikowa and Witkowski, 2008). Similarly, the Jeita Cave, Lebanon, suggested a wet condition (Verheyden et al., 2008), while Lake Tecer in central Turkey recorded humid conditions c. 3.9 ka BP and from 3.6 to 3.4 ka BP (Kuzucuoǧlu et al., 2011). Wet conditions in northern Syria between 4.0 and 3.7 ka BP (Fiorentino et al., 2008) and humid conditions in the eastern Mediterranean region between 3.9 and 3.7 ka BP (Roberts et al., 2008) were also reported. Likewise, geochemical records from Mediterranean lakes showed several moist episodes dominating the region between 3.9 and 3.4 ka BP (Roberts et al., 2008).
A new multi-proxy palaeoenvironmental study near the archaeological complex at Konar Sandal, southeast Iran, showed that c. 3.9 ka BP, low Ti/Al and Si/Al, and high K/Al, along with high δ13C in organic matter and low C/N, suggest a wet period (Vaezi et al., 2022). Between 3.9 and 3.3 ka BP, Jiroft experienced wet/semi-wet conditions based on the average values of various elemental ratios (Si/Al, Ti/Al). The prevalence of wet conditions, extending from 3.8 to 3.5 ka BP, is further affirmed based on the dynamics of aquatic vegetation cover and abundance (Gurjazkaite et al., 2018). The semi-wet conditions in southeastern Iran are punctuated by two dry periods (Vaezi et al., 2022). They occurred c. 3.8 ka BP and c. 3.6–3.5 ka BP, respectively. The dry period is in agreement with a sharp decline in the Dead Sea level (Migowski et al., 2006; Neumann et al., 2007), and a short drought c. 3.6 ka BP in northern Syria (Fiorentino et al., 2008). The abundance of Cerealia-type pollen from Lake Almalou in northwestern Iran (Djamali et al., 2009b), Lake Parishan in southwestern Iran (Jones et al., 2015), and the Daryeche peat sequence in Konar Sandal (Gurjazkaite et al., 2018) are important indicators of agricultural activities during this period (Figure 2). Despite the overall minimal signs of agricultural activity from 4.0 to 3.2 ka BP, there are two possible periods of extensive agricultural activity c. 3.9 and 3.7 ka BP (Figure 3), as indicated in the pollen assemblage from Konar Sandal (Gurjazkaite et al., 2018). Consistent with this trend, the Cerealia-type pollen record from Lake Parishan in southwestern Iran (Jones et al., 2015) demonstrates an increase from 3.5 ka BP, reaching its peak c. 3.3 ka BP, and subsequently declining (Figure 3).
Many sites with ceramic assemblages closely related to the Oxus Civilization of southern Central Asia are now known in the Iranian and Afghan Sistan provinces (Biscione and Vahdati, 2020). They documented the continued human presence in the region into the first few centuries of the fourth millennium BC after the major urban sites and their satellites had been abandoned. Notably, both individual settlements and the overall settlement system in southeastern Iran appear to have witnessed a steep decline from the Middle Bronze Age (fourth millennium BP) onward. Although Tepe Yahya shows occupation during this period (IVA, c. 4.0–3.6 ka BP), potential contemporary settlements in Konar Sandal South (Thornton, 2012) and some nearby sites (N. Eskandari, personal communication) were abandoned during this time until c. 3.2 ka BP (Prickett, 1986a; Pfälzner et al., 2019; Shahsavari et al., 2024). After the occupation of Tepe Yahya (period IVA), which ended c. 3.6 ka BP, there is no evidence of sedentary settlement in the region for several centuries (Prickett, 1986a; Pfälzner et al., 2019; Shahsavari et al., 2024). A similar situation occurs in the Lut desert, Sistan, and Bard Sir Valley. Further afield, a dramatic reduction in known settlement at this time is also characteristic of Pakistani Baluchistan, extending even to the Indus Valley and to southern Central Asia. Biscione and Sajjadi (2022) refer to this period as the ‘crisis of Bronze Age urbanism.’ However, a contrasting situation arises in highland southwestern Iran, whereby the Kaftari period represents a peak in sedentary occupation in the Kur River Basin. Subsequently, in the later fourth millennium BP (Qaleh/Shogha-Teimuran periods, c. 3.6–2.9 ka BP), there was a significant decline in population at the Kur River Basin, reaching a low point in the early centuries of the third millennium BP (De Miroschedji, 2003). Additional contrasting patterns occur in Susiana and central western Iran around the fourth millennium BP. Susiana witnesses a dramatic growth in population and Elamite regional power (c. 4.0 ka BP), peaking during the Middle Elamite period (c. 3.5–3.1 ka BP) before a steep decline occurs in the subsequent Neo-Elamite period (De Miroschedji, 2003). At this time, Luristan continues to be mainly represented archaeologically by graveyards. In contrast, occupation further north in Kermanshah has identified a distinct upturn in settled occupation from the early fourth millennium BP and into the Late Bronze Age (Matthews and Fazeli Nashli, 2022).
On the central Iranian Plateau, settlements do not re-appear in the archaeological record until the mid-fourth millennium BP, contrasting with the concurrent decline observed in southern Iran. This pattern has been linked with climate change, characterized as the ‘Central Iranian Drought’ (Kirsten et al., 2023, p. 2). In contrast, the Qom region exhibits a different pattern, where the settlement appears to have increased through the fourth millennium BP across the Bronze Age to Iron Age transition (Matthews and Fazeli Nashli, 2022: 263).
Dry conditions have been reported during this period in Lake Neor, NW Iran, with enhanced deposition of dust (Sharifi et al., 2015). Dry episodes occurred at about the same time in Lake Zeribar (Stevens et al., 2008; Wasylikowa and Witkowski, 2008), western Iran, and in Lake Maharlou, southwest Iran (Schulman et al., 2001). The change is inferred based on evaporite minerals and low fluvial input (Brisset et al., 2018). Similarly, Lake Tecer experienced dry conditions and a sharp decline in lake levels during this period (Kuzucuoğlu et al., 2011). Droughts were also reported in Lake Van c. 3.3 ka BP (Lemcke and Sturm, 1997) and the Dead Sea between 3.3 and 3.0 ka BP (Migowski et al., 2006). The dusty and dry conditions are consistent with increased Ca and K levels in the GISP2 ice core in Greenland. Mayewski et al. (1997) used K trends to indicate that the Siberian High dominated during this period.
From c. 3.3 to 2.9 ka BP, the Jiroft region was exposed to dry and windy conditions based on the high Ti/Al and Si/Al ratios and very low δ13C in organic matter along with the presence of long-chain alkanes derived from terrestrial plant waxes (Vaezi et al., 2022). The prevalence of dry conditions in the region is further supported by the abundance of Artemisia and Calligonum pollen (Gurjazkaite et al., 2018). The dry conditions coincide with extended regional droughts and a pronounced decrease in cereal cultivation in the Jiroft region from 3.4 to 3.2 ka BP (Figure 3; Vaezi et al., 2022). Subsequently, the abundance of Cerealia-type pollen in the Daryache peat sequence gradually rises until it peaks at c. 2.8 ka BP.
The driest condition occurred c. 3.2 ka BP on the Iranian Plateau and coincided with dramatic disruptions in Late Bronze Age settlements in ancient Mesopotamia and the eastern Mediterranean region typically labeled as a ‘collapse’ (Weiss, 1982b; Table 1; Haggis, 1993; Kaniewski et al., 2010; Paulette, 2012; Kaniewski et al., 2010; Weiss, 2016; Manning et al., 2020; Baten et al., 2023). Similarly, hot and dry conditions c. 3.2 ka BP have been reported in archeological excavations from Ugarit, Syria (Alpert and Neumann, 1989). This period coincided with the decline in fluvial discharge in the Tigris and Euphrates Rivers, resulting in crop failure and the prevalence of widespread famines in Mesopotamia, ushering a steady decline (Kay and Johnson, 1981; Neumann and Parpola, 1987; Alpert and Neumann, 1989; Schulman et al., 2001; Kaniewski et al., 2010; Weiss, 2016; Manning et al., 2020; Baten et al., 2023).
Importantly, this period represents one of the few phases of near synchronicity in emerging settlements across southern Iran, in which nearly all regions display minimal evidence of sedentary occupation. In southeastern Iran, occupation was not attested to Sistan, the western Lut, the Bard Sir Valley, or the Bam region before c. 2.8 ka BP (Petrie and Weeks, 2018). However, recently published work demonstrates that, just across the border in Afghan Sistan, settlements existed in the Sar-o-Tar region c. 3.0 ka BP, supported by large-scale irrigation (Allen and Trousdale, 2019). Settlement decline, as seen in Iranian Sistan, was also thought to characterize the Jiroft region; however, recent research has documented early Iron Age sites (c. 3.3–3.0 ka BP) at Tom-e Gavan and Konar Sandal North (Mashkour et al., 2013; Shahsavari et al., 2024), partially filling the long settlement gap and aligning with the gradual rise in Cerealia-type pollen recorded in the Daryache peat sequence. The dramatic reduction in settlement in the Kur River Basin through the Qaleh/Shogha-Teimouran periods was noted above, with rare occupation possibly attested further to the west at Tol-e Nurabad in the Mamasani Valley (Petrie et al., 2009). In Khuzestan, the period extending from the late fourth to the early third millennium BP witnessed a dramatic decline in settlements before a modest rise occurred in the Neo-Elamite II period (mid-third millennium BP; De Miroschedji, 2003). In Central Zagros, the Late Bronze Age sites such as Baba Jan and other sites are deserted or shrink dramatically in size at the transition to the Early Iron Age. This pattern is seen in Luristan and Kermanshah and implies a change in subsistence practices with a greater emphasis on herding over agriculture. This adaptation is thought to have been initiated by changing climate or ecology (Neumann and Parpola, 1987; Overlaet, 2013).
The low C/N ratios and abundance of aquatic lipids and Sparganium-type pollen between 2.9 and 2.3 ka BP imply that waterlogging could have been sufficient to support dominant submerged/floating macrophyte populations in Konar Sandal (Gurjazkaite et al., 2018; Vaezi et al., 2022). This multi-proxy study in southeastern Iran suggested a different climatic condition that is mainly wet, compared to previous palaeoenvironmental studies to its west on the Iranian Plateau (Figure 2). For example, dry conditions have been reported from Lakes Neor and Zeribar, northwest Iran (Stevens et al., 2008; Wasylikowa and Witkowski, 2008; Sharifi et al., 2015), and Lake Maharlou, southwest Iran (Issar and Zohar, 2007). However, consistent with the wet conditions in the Jiroft region, Lake Tecer experienced humid conditions between 2.8 and 2.0 ka BP (Kuzucuoğlu et al., 2011). Notably, there is no sign of agricultural activity in Lake Almalou, NW Iran (Djamali et al., 2009b), in contrast to the extensive agricultural practices in southeast and southwest Iran. Perhaps the dry conditions in western Iran (Figure 3) prevented agriculture, while favorable climatic conditions supported agriculture to flourish in southern Iran.
The period extending from 2.9 to 2.3 ka BP corresponds with the termination of the Elamite kingdom, followed by the rise of the Medes and the Achaemenid Empires in Iran. The rise of these dynasties coincided with the wettest period and significant agricultural activities in the Jiroft region since the decline of the Bronze Age (Gurjazkaite et al., 2018; Vaezi et al., 2022). Vaezi et al. (2022) speculate that favorable climatic conditions may have played a role in supporting socio-economic stability, supporting resource provisioning, and the territorial expansion of the Achaemenid dynasty (Figure 1). Consistent with this, an increase in known settlements has been documented in Iranian Sistan since the Achaemenid period. Similarly, an increase in settlement in Jiroft began before the Achaemenid period but was particularly clear from that period onwards (Maresca, 2018). In southwest Iran, the Achaemenid period ushered a period of substantial and consistent settlement and growth spanning more than a millennium in both highland (Fars) and lowland (Khuzestan) regions (Sumner, 1986; De Miroschedji, 2003). Nonetheless, the simultaneous occurrence of widespread agriculture, favorable climatic conditions, and the rise of imperial powers (Medes, Achaemenids, and Seleucids) suggests a plausible climate-culture association and interdependence that should not be disregarded.
Dry conditions reappeared in Jiroft c. 2.3 to 2.2 ka BP based on increased aeolian input coeval with the decline in total aquatic lipids and Sparganium-type pollen. Notably, Cerealia-type pollen is absent in the peat sequence in Konar Sandal for c. 200 years (Vaezi et al., 2022). Around the same period, the highest Cerealia-type pollen counts occur in Lake Almalou in northwest Iran (Djamali et al., 2009b), corresponding to the rise of the Seleucid Empire between 2.3 and 2.2 ka BP (Figure 3). Likewise, a significant decrease in aeolian activity in Lake Neor (Sharifi et al., 2015), Lake Tecer (Kuzucuoğlu et al., 2011), and the Dead Sea lakes (Migowski et al., 2006) were consistent with a wet period. Thus, more investigations are needed to understand the reason for the sudden changes in land use and flip-flops in precipitation between the northwest and southeast regions in Iran.
A major increase in aeolian activity and dry conditions are predicted between 2.0 and 1.6 ka BP based on the high Ti/Al and the lowest δ13C values in the Daryache peat sequence (Vaezi et al., 2022). Despite the prevailing dry conditions, Jiroft experienced a significant increase in agricultural activity between 2.05 and 1.87 ka BP, which corresponds to the rule of the Parthian dynasty (Vaezi et al., 2022). Although it may have had less agricultural activity than the Medes and Achaemenid Empires, it still witnessed agricultural productivity during dry periods not seen since the late Bronze Age. The impressive agricultural productivity observed during the Parthian dynasty could be attributed to cultural adaptation to the arid environment and significant advancements in farming practices, such as the innovative maze of qanats/canals that was developed (Petrie and Weeks, 2018).
The Jiroft region experienced wet conditions again between 1.6 and 1.3 ka BP (Vaezi et al., 2022), aligning with the expansion of the later period of the Sassanian Empire (Daryaee, 2023). The favorable climatic condition was inferred based on the sharp decrease in aeolian inputs (Ti/Al) and high values of K/Ti coupled with low C/N and high δ13C in organic matter. Very low aeolian activity in Lake Neor (Sharifi et al., 2015), wet conditions in Lake Tecer (Kuzucuoğlu et al., 2011), and a sharp increase in Dead Sea lake levels (Migowski et al., 2006) are coeval to the ongoing changes in southeast Iran (Figure 3). A considerable increase in the cultivation of various trees around the Lake Almalou catchment, coinciding with the Sassanian Empire, has been reported (Djamali et al., 2009b). Thereafter, between 1.3 and 0.8 ka BP, Jiroft had mild conditions. However, the presence of Cerealia-type pollen is low (Gurjazkaite et al., 2018). Agricultural activities on the Iranian Plateau were marginal during this period, perhaps due to political instability and weak succession (Djamali et al., 2010b; Daryaee, 2012). Notably, several short droughts with high aeolian activity over the Iranian Plateau prevailed since 1.3 ka BP; these events were associated with war, political instability, and crises over access to resources (Sharifi et al., 2015). The decline of the Sasanian (1.3 ka BP) and Safavid Empires (0.95 ka BP) (Djamali et al., 2010a; Daryaee, 2012) also coincided with periods of high levels of atmospheric dust deposition as in Lake Neor (Sharifi et al., 2015). Similarly, the dry conditions recorded in central Anatolia from 1.8 to 1.9 AD (c. 0.2 ka BP to present) coincided with high aeolian input in Lake Neor (Sharifi et al., 2015). The unfavorable conditions may have exacerbated socio-economic instability, potentially playing a role in weakening the Sasanian Empire and facilitating its defeat by the Islamic armies.
The high-resolution palaeoclimate records spanning the LGM and post-LGM events provide a valuable and comprehensive overview of environmental changes sweeping the Iranian Plateau. Records spanning the last 20 ka provide distinct insights into the region’s temporal variability of climate and hydrological patterns. In addition, these studies advance our knowledge about palaeoclimate changes and their potential impacts on past human settlements and cultural transformations. This multifaceted approach sheds light on the complex interactions between environmental conditions and human societies, contributing to a holistic comprehension of the historical trajectory since the Neolithic period.
The strong correlation between the dust input over the Iranian Plateau with North Atlantic cooling events, e.g., Heinrich Stadial one and the Younger Dryas, supports the idea that atmospheric teleconnections most likely existed between these regions. Peak wet conditions during the early Holocene in southeastern Iran extended from 11.4 to 9.6 ka BP, whereas in western Iran, they occurred from 10.2 to 8.6 ka BP. During the early Holocene, high solar insolation played a significant role in causing changes in atmospheric circulation. Firstly, it led to the northward displacement of the ITCZ and the subsequent strengthening and northward expansion of the ISM. This caused increased rainfall and wet conditions in certain regions. On the other hand, the high solar insolation strengthened the descending air in sub-tropical high-pressure systems, leading to their northward expansion over the western Iranian Plateau. This caused dry conditions in the area, counteracting the wet conditions caused by the northward expansion of ISM.
Neolithic settlements emerged in Iran around the mid-11th millennium BP. They were founded on a mixed economy exploiting domesticated plants and animals, which allowed both seasonal and permanent sedentary settlements. First established in the Zagros mountains, Neolithic settlements were notably scarce in southeast Iran until later millennia. In eastern Iran, Neolithic settlements were strategically established around alluvial fans, allowing access to fertile soils and groundwater alongside irrigation by surface runoff capture.
Dusty, arid conditions covered the Iranian plateau c. 8.2 ka BP and subsequent cold and dry events coincided with the injection of icebergs into the North Atlantic Ocean. Between 7.8 and 6.3 ka BP, western Iran experienced a period of high humidity coinciding with the gradual expansion of oak forests. This is due to the decreased intensity and duration of the sub-tropical high-pressure systems over the Zagros region. During this period, southeastern Iran experienced a mild climate and increased precipitation. However, this region received less rainfall compared to the early Holocene. Between 6.3 and 5.0 ka BP, a decrease in solar insolation ushered in an arid period. The reduction in insolation impacted the amount of summer monsoon rains in southeast Iran, and the region shifted from the ISM to a MLW-dominated system. However, it is essential to note that a stable climate did not characterize the late Holocene period. Instead, the region experienced fluctuations in its climatic conditions, which were attributed to the irregularity in the atmospheric circulation of the MLW during the mid-Holocene period.
Notably, there was a significant change in environmental conditions over the Iranian Plateau c. 5.0 to 4.5 ka BP. During this period, aeolian activity decreased, followed by a corresponding increase in rainfall, indicating a shift towards wetter conditions. The beginning of this wet phase coincided with Early Bronze Age settlements flourishing in southeastern Iran, suggesting improved environmental conditions may have been beneficial. This period coincided with the consolidation of an integrated oasis-based agricultural system, a fundamental adaptation for all future human settlements in eastern Iran. Concurrent developments in technologies and exchange systems marked this period as the first instance of intense intercultural contact, representing a transformative period that experienced substantial growth in socioeconomic complexity. Subsequently, the region encountered arid conditions between 4.5 and 4.0 ka BP, which was evident from increased aeolian input and dry conditions, particularly c. 4.2 ka BP in Western Asia. This downturn in climate towards the end of the Early Bronze Age is coeval to the observed decline of large urban sites that characterized eastern Iran from the early fourth millennium BP. This dramatic change in environmental conditions is associated with the widespread decline of Early Bronze Age settlement across many (but not all) regions from Mesopotamia to China. The environmental changes likely impacted agricultural productivity and are correlated with the abandonment or relocation of settlements on the Iranian Plateau. Except for a wet period in the western region and a semi-wet phase in southeastern Iran from c. 4.0 to 3.4 ka BP, overall arid conditions prevailed in both regions from c. 4.5 to 2.9 ka BP. The driest conditions centered c. 3.2 ka BP coincided with the regional Late Bronze Age cultural decline across many areas of Iran, Western Asia, and the Mediterranean.
Consistent with various studies, we believe favorable climatic conditions contributed to increased socioeconomic stability, which seems more than coincidental and likely played a role in the success of the Persian Empires. The wet conditions from c. 2.9 to 2.3 and 1.6 to 1.3 ka BP, aligned with the expansive reigns of the Achaemenids and Sassanians. Despite arid conditions, the impressive agricultural productivity during the Parthian rule is attributed to cultural adaptation to the dry conditions and advancements in farming practices. Droughts during specific historical periods likely affected agricultural productivity, access to water resources, and overall food security, which could have destabilized these empires. Nevertheless, the interplay between environmental changes and the rise of organized human settlements is a complex and multidimensional phenomenon that calls for a comprehensive and more nuanced understanding of the historical, archaeological, and climatological evidence.
AV: Conceptualization, Data curation, Formal Analysis, Investigation, Writing–original draft, Writing–review and editing. LW: Conceptualization, Formal Analysis, Writing–original draft, Writing–review and editing. JR: Conceptualization, Data curation, Formal Analysis, Funding acquisition, Investigation, Project administration, Writing–review and editing, Writing–original draft.
The author(s) declare that financial support was received for the research, authorship, and/or publication of this article. This research was funded by Vetenskapsrådet grant E0402601 to JR
We appreciate the constructive and insightful suggestions from the reviewers, which have contributed to improving the manuscript. Theb.ai and DeepSeek-V3 were used to enhance the English language of this manuscript.
The authors declare that the research was conducted in the absence of any commercial or financial relationships that could be construed as a potential conflict of interest.
The author(s) declared that they were an editorial board member of Frontiers, at the time of submission. This had no impact on the peer review process and the final decision.
All claims expressed in this article are solely those of the authors and do not necessarily represent those of their affiliated organizations, or those of the publisher, the editors and the reviewers. Any product that may be evaluated in this article, or claim that may be made by its manufacturer, is not guaranteed or endorsed by the publisher.
Abe, M., and Khanipour, M. (2019). “The 8.2 ka event and re-microlithization during the late mlefaatian in the Zagros mountains: analysis of the flaked stone artifacts excavated from hormangan in North-eastern Fars, south-West Iran,” in Decades in deserts: essays on near eastern archaeology in honour of sumio fujii. Japan: Rokuichi Syobou, 305–317.
Alden, J. (2013). “The Kur River Basin in the Proto-Elamite era—surface survey, settlement patterns, and the appearance of full-time transhumant pastoral nomadism,” in Ancient Iran and its neighbours: local developments and long-range interactions in the fourth millennium BC. Editor C. Petrie (Oxford, England: Oxbow Books), 207–232.
Alizadeh, A. (2003). Excavations at the prehistoric mound of chogha bonut, khuzestan, Iran: seasons 1976/77, 1977/78, and 1996, 120. Chicago: Univ. Chicago Oriental Institute publications.
Alizadeh, A. (2008). Chogha Mish II. The Development of a prehistoric regional center in lowland Susiana, Southwest Iran: final report on the last six seasons of excavations 1972-1978, Orient. Inst. Publ. 35(1), pp. 143–146. Available online at: http://www.jstor.org/stable/41496973
Alizadeh, A. (2021). Review and synthesis of the neolithic cultural development in Fars, southern Iran. J. Neolithic Archaeol. 23, 1–27. doi:10.1016/j.dendro.2018.03.002
Allen, M., and Trousdale, W. B. (2019). Early Iron age culture of sistan, Afghanistan. Afghan. Afghan. 2 (1), 29–69. doi:10.3366/afg.2019.0025
Alpert, P., and Neumann, J. (1989). An ancient “Correlation” between streamflow and distant rainfall in the Near East. J. Near East. Stud. 48 (4), 313–314. doi:10.1086/373411
Anjomrooz, S., Vahdati Nasab, H., and Eskandari, N. (2022). New evidence of Pleistocene hominin occupations in Kerman Province, southern Iran. Antiquity 96 (390), 1592–1598. doi:10.15184/aqy.2022.120
Arsalani, M., Pourtahmasi, K., Azizi, G., Bräuning, A., and Mohammadi, H. (2018). Tree-ring based december–february precipitation reconstruction in the southern Zagros mountains, Iran. Dendrochronologia 49, 45–56. doi:10.1016/j.dendro.2018.03.002
Asouti, E., Baird, D., Kabukcu, C., Swinson, K., Martin, L., García-Suárez, A., et al. (2020). The Zagros epipalaeolithic revisited: new excavations and 14C dates from Palegawra cave in Iraqi kurdistan. Plos One 15 (9), e0239564. doi:10.1371/journal.pone.0239564
Azadi, A., Moscone, D., Eger, J., and Ricci, A. (2023). The first occupation of the mountains: Neolithic human-environmental interactions in the Kohgiluyeh region (southern Zagros, Iran). Quat. Int. 700-701, 27–38. doi:10.1016/j.quaint.2023.04.007
Bar-Matthews, M., and Ayalon, A. (2011). Mid-Holocene climate variations revealed by high-resolution speleothem records from Soreq Cave, Israel and their correlation with cultural changes. Holocene 21 (1), 163–171. doi:10.1177/0959683610384165
Bar-Matthews, M., Ayalon, A., Gilmour, M., Matthews, A., and Hawkesworth, C. J. (2003). Sea–land oxygen isotopic relationships from planktonic foraminifera and speleothems in the Eastern Mediterranean region and their implication for paleorainfall during interglacial intervals. Geochimica Cosmochimica Acta 67 (17), 3181–3199. doi:10.1016/s0016-7037(02)01031-1
Bar-Matthews, M., Ayalon, A., Kaufman, A., and Wasserburg, G. J. (1999). The Eastern Mediterranean paleoclimate as a reflection of regional events: Soreq cave, Israel. Earth Planet. Sci. Lett. 166 (1-2), 85–95. doi:10.1016/s0012-821x(98)00275-1
Baten, J., Benati, G., and Sołtysiak, A. (2023). Violence trends in the ancient Middle East between 12,000 and 400 BCE. Nature human behaviour7 (12), 2064–2073.
Beale, T. W. (1986). Excavations at Tepe Yahya, Iran 1967-1976: the early periods, American school of prehistoric research bulletin, 38. Cambridge: Peabody Museum of Archaeology and Ethnology.
Bell, B. (1971). The Dark Ages in ancient history. I. The first dark age in Egypt. Am. J. Archaeol. 75 (1), 1–26. doi:10.2307/503678
Beni, A. N., Marriner, N., Sharifi, A., Azizpour, J., Kabiri, K., Djamali, M., et al. (2021). Climate change: a driver of future conflicts in the Persian Gulf region? Heliyon 7 (2), e06288. doi:10.1016/j.heliyon.2021.e06288
Biscione, R., and Sajjadi, S. M. S. (2022). The research of the consiglio nazionale delle ricerche (national research Council) in eastern Iran: shahr-i Sokhta and sistan in late 3rd – early 2nd millennia and the diffusion of the greater khorasan civilization (Bactria-Margiana archaeological complex). Excav. Res. A. T. Shahr -i Sokhta 2.
Biscione, R., and Vahdati, A. A. (2020). “The BMAC presence in eastern Iran: state of affairs in December 2018–towards the Greater Khorasan civilization?,” in World Oxus Civiliz. (Routledge), 527–550. doi:10.4324/9781315193359-23
Bond, G. C., and Lotti, R. (1995). Iceberg discharges into the North Atlantic on millennial time scales during the last glaciation. Science 267 (5200), 1005–1010. doi:10.1126/science.267.5200.1005
Bottema, S. (1986). A late Quaternary pollen diagram from Lake Urmia (northwestern Iran). Rev. Palaeobot. Palynology 47 (3-4), 241–261. doi:10.1016/0034-6667(86)90039-4
Butzer, K. W., and Endfield, G. H. (2012). Critical perspectives on historical collapse. Proc. Natl. Acad. Sci. 109 (10), 3628–3631. doi:10.1073/pnas.1114772109
Caldwell, J. R. (1967). “Investigations at tal-i-iblis,” in Illinois State Museum preliminary reports.
Carolin, S. A., Walker, R. T., Day, C. C., Ersek, V., Sloan, R. A., Dee, M. W., et al. (2019). Precise timing of abrupt increase in dust activity in the Middle East coincident with 4.2 ka social change. Proc. Natl. Acad. Sci. 116 (1), 67–72. doi:10.1073/pnas.1808103115
Chauhan, O. S. (2003). Past 20,000-year history of Himalayan aridity: evidence from oxygen isotope records in the Bay of Bengal. Curr. Sci., 84 (1), pp. 90–93.
Clark, P. U., Shakun, J. D., Baker, P. A., Bartlein, P. J., Brewer, S., Brook, E., et al. (2012). Global climate evolution during the last deglaciation. Proc. Natl. Acad. Sci. 109 (19), E1134–E1142. doi:10.1073/pnas.1116619109
Colburn, H. P. (2013). Connectivity and communication in the Achaemenid empire. J. Econ. Soc. Hist. Orient 56 (1), 29–52. doi:10.1163/15685209-12341278
Cronin, S. (2021). Social histories of Iran: modernism and marginality in the Middle East. Cambridge University Press. doi:10.1017/9781108120289
Cullen, H. M., Demenocal, P. B., Hemming, S., Hemming, G., Brown, F. H., Guilderson, T., et al. (2000). Climate change and the collapse of the Akkadian empire: evidence from the deep sea. Geology 28 (4), 379–382. doi:10.1130/0091-7613(2000)028<0379:ccatco>2.3.co;2
Dansgaard, W., White, J. W., and Johnsen, S. J. (1989). The abrupt termination of the Younger Dryas climate event. Nature 339 (6225), 532–534. doi:10.1038/339532a0
Daryaee, T. (2023). Sasanian Persia: the rise and fall of an empire. New York, USA: Bloomsbury Publishing.
Daryaee, T. (2012). The Oxford Handbook of Iranian History. Oxford, United Kingdom: Oxford University Press.
Degroot, D., Anchukaitis, K., Bauch, M., Burnham, J., Carnegy, F., Cui, J., et al. (2021). Towards a rigorous understanding of societal responses to climate change. Nature 591 (7851), 539–550.
Delougaz, P., Kantor, H. J., and Alizadeh, A. (1996). Chogha mish volume 1: the first five seasons of excavations 1961-1971. Chicago: Oriental Institute Publications, 101.
De Miroschedji, P. (2003). ‘Susa and the Highlands: major trends in the history of Elamite civilisation’, in N. F. Miller, and K. Abdi (eds) Yeki bud, yeki nabud: essays on the archaeology of Iran in honor of william M. Sumner. Los Angeles: Cotsen Institute of Archaeology Monograph, 17–38.
Denton, G. H., Alley, R. B., Comer, G. C., and Broecker, W. S. (2005). The role of seasonality in abrupt climate change. Quat. Sci. Rev. 24 (10-11), 1159–1182. doi:10.1016/j.quascirev.2004.12.002
Desset, F., Vidale, M., and Soleimani, N. A. (2013). Mahtoutabad III (province of Kerman, Iran): an “Uruk-related” material assemblage in eastern Iran. Iran 51 (1), 17–54. doi:10.1080/05786967.2013.11834722
Dixit, Y., Hodell, D. A., Sinha, R., and Petrie, C. A. (2014). Abrupt weakening of the Indian summer monsoon at 8.2 kyr BP. Earth Planet. Sci. Lett. 391, 16–23. doi:10.1016/j.epsl.2014.01.026
Djamali, M., Akhani, H., Andrieu-Ponel, V., Braconnot, P., Brewer, S., de Beaulieu, J. L., et al. (2010a). Indian Summer Monsoon variations could have affected the early-Holocene woodland expansion in the Near East. Holocene 20 (5), 813–820. doi:10.1177/0959683610362813
Djamali, M., de Beaulieu, J. L., Andrieu-Ponel, V., Berberian, M., Miller, N. F., Gandouin, E., et al. (2009b). A late Holocene pollen record from Lake Almalou in NW Iran: evidence for changing land-use in relation to some historical events during the last 3700 years. J. Archaeol. Sci. 36 (7), 1364–1375. doi:10.1016/j.jas.2009.01.022
Djamali, M., De Beaulieu, J. L., Miller, N. F., Andrieu-Ponel, V., Ponel, P., Lak, R., et al. (2009a). Vegetation history of the SE section of the Zagros Mountains during the last five millennia; a pollen record from the Maharlou Lake, Fars Province, Iran. Veg. Hist. Archaeobotany 18, 123–136. doi:10.1007/s00334-008-0178-2
Djamali, M., de Beaulieu, J. L., Shah-hosseini, M., Andrieu-Ponel, V., Ponel, P., Amini, A., et al. (2008). A late Pleistocene long pollen record from Lake Urmia, NW Iran. Quat. Res. 69 (3), 413–420. doi:10.1016/J.YQRES.2008.03.004
Djamali, M., Ponel, P., Andrieu-Ponel, V., de Beaulieu, J. L., Guibal, F., Miller, N. F., et al. (2010b). Notes on arboricultural and agricultural practices in ancient Iran based on new pollen evidence. Paléorient 36, 175–188. doi:10.3406/paleo.2010.5394
Dykoski, C. A., Edwards, R. L., Cheng, H., Yuan, D., Cai, Y., Zhang, M., et al. (2005). A high-resolution, absolute-dated Holocene and deglacial Asian monsoon record from Dongge Cave, China. Earth Planet. Sci. Lett. 233 (1-2), 71–86. doi:10.1016/j.epsl.2005.01.036
Emberling, G., Robb, J., Speth, J. D., and Wright, H. T. (2002). Kunji cave: early Bronze age burials in luristan. Iran. Antiq. 37, 47–104. doi:10.2143/IA.37.0.118
Eskandari, N. (2018). A reappraisal of the chronology of the Chalcolithic period in SE Iran: absolute and relative chronology of Tepe Dehno and Tepe East Dehno, Shahdad. J. Res. Archaeom. 4 (1), 23–35. doi:10.29252/jra.4.1.23
Eskandari, N. (2021a). Mokhtarabad: a solitaire large late chalcolithic-early Bronze age site in the dasht-e Lut and its implication for understanding the communication networks. Iran. J. Archaeol. Stud. 11 (1), 129–132. doi:10.22111/IJAS.2021.6862
Eskandari, N. (2021b). Dig it Up: a Reconsideration of old excavations at the urban center of Shahdad. J. Archaeol. Stud. 12 (4), 23–45.
Eskandari, N., Shafiee, M., Abedi, A., and Javadi, M. (2014). Keshit: an early Bronze age urban center on the western edge of the Lut Desert, south-eastern Iran. J. Antiq. 341, 88.
Fallah, B., Sodoudi, S., Russo, E., Kirchner, I., and Cubasch, U. (2017). Towards modeling the regional rainfall changes over Iran due to the climate forcing of the past 6000 years. Quat. Int. 429, 119–128. doi:10.1016/j.quaint.2015.09.061
Fazeli Nashli, H., and Matthews, R. (2013). “The neolithisation of Iran: patterns of change and continuity,” in The neolithisation of Iran. Editors R. Matthews, and H. Fazeli Nashli (Oxford: Oxbow), 1–13.
Fiorentino, G., Caracuta, V., Calcagnile, L., D'Elia, M., Matthiae, P., Mavelli, F., et al. (2008). Third millennium BC climate change in Syria highlighted by carbon stable isotope analysis of 14C-AMS dated plant remains from Ebla. Paleogeogr. Palaeoclimatol. Palaeoecol. 266 (1-2), 51–58. doi:10.1016/j.palaeo.2008.03.034
Fleitmann, D., Burns, S. J., Mangini, A., Mudelsee, M., Kramers, J., Villa, I., et al. (2007). Holocene ITCZ and Indian monsoon dynamics recorded in stalagmites from Oman and Yemen (Socotra). Quat. Sci. Rev. 26, 170–188. doi:10.1016/j.quascirev.2006.04.012
Fleitmann, D., Burns, S. J., Mudelsee, M., Neff, U., Kramers, J., Mangini, A., et al. (2003). Holocene forcing of the Indian monsoon recorded in a stalagmite from southern Oman. Science 300 (5626), 1737–1739. doi:10.1126/science.1083130
Fleitmann, D., Haldon, J., Bradley, R. S., Burns, S. J., Cheng, H., Edwards, R. L., et al. (2022). Droughts and societal change: the environmental context for the emergence of Islam in late Antique Arabia. Science 376 (6599), 1317–1321. doi:10.1126/science.abg4044
Flohr, P., Fleitmann, D., Matthews, R., Matthews, W., and Black, S. (2016). Evidence of resilience to past climate change in Southwest Asia: early farming communities and the 9.2 and 8.2 ka events. Quat. Sci. Rev. 136, 23–39. doi:10.1016/j.quascirev.2015.06.022
Frumkin, A., Ford, D. C., and Schwarcz, H. P. (2000). Paleoclimate and vegetation of the last glacial cycles in Jerusalem from a speleothem record. Glob. Biogeochem. Cycles 14 (3), 863–870. doi:10.1029/1999gb001245
Gala, M., and Tagliacozzo, A. (2014). The role of birds in the settlement of Shahr-i Sokhta (Sistan, Iran) during the 3rd millennium BC. Int. J. Osteoarchaeol. 24 (3), 319–330. doi:10.1002/oa.2389
Gasse, F., and Van Campo, E. (1994). Abrupt post-glacial climate events in West Asia and North Africa monsoon domains. Earth Planet. Sci. Lett. 126 (4), 435–456. doi:10.1016/0012-821x(94)90123-6
Giesche, A., Hodell, D. A., Petrie, C. A., Haug, G. H., Adkins, J. F., Plessen, B., et al. (2023). Recurring summer and winter droughts from 4.2-3.97 thousand years ago in north India. Commun. Earth Environ. 4 (1), 103. doi:10.1038/s43247-023-00763-z
Giesche, A., Staubwasser, M., Petrie, C. A., and Hodell, D. A. (2019). Indian winter and summer monsoon strength over the 4.2 ka BP event in foraminifer isotope records from the Indus River delta in the Arabian Sea. Clim. Past 15 (1), 73–90. doi:10.5194/cp-15-73-2019
Gopnik, H., and Rothman, M. S. (2011). On the high road: the history of Godin Tepe, 1. Iran: Mazda Publ.
Griffiths, H. I., Schwalb, A., and Stevens, L. R. (2001). Evironmental change in southwestern Iran: the Holocene ostracod fauna of Lake Mirabad. Holocene 11 (6), 757–764. doi:10.1191/09596830195771
Gupta, A. K., Anderson, D. M., and Overpeck, J. T. (2003). Abrupt changes in the asian southwest monsoon during the Holocene and their links to the North atlantic ocean. Nature 421 (6921), 354–357. doi:10.1038/nature01340
Gupta, A. K., Das, M., and Anderson, D. M. (2005). Solar influence on the Indian summer monsoon during the Holocene. Geophys. Res. Lett. 32 (17). doi:10.1029/2005GL022685
Gurjazkaite, K., Routh, J., Djamali, M., Vaezi, A., Poher, Y., Beni, A. N., et al. (2018). Vegetation history and human-environment interactions through the late Holocene in Konar Sandal, SE Iran. Quat. Sci. Rev. 194, 143–155. doi:10.1016/j.quascirev.2018.06.026
Haggis, D. C. (1993). Intensive survey, traditional settlement patterns and dark age crete: the case of early Iron age kavousi. J. Mediterr. Archaeol. 6 (2), 131–174. doi:10.1558/jmea.v6i2.131
Haldon, J., Mordechai, L., Newfield, T. P., Chase, A. F., Izdebski, A., Guzowski, P., et al. (2018). History meets palaeoscience: consilience and collaboration in studying past societal responses to environmental change. Proc. Natl. Acad. Sci. 115 (13), 3210–3218. doi:10.1073/pnas.1716912115
Hamzeh, M. A., Gharaie, M. H. M., Lahijani, H. A. K., Djamali, M., Harami, R. M., and Beni, A. N. (2016). Holocene hydrological changes in SE Iran, a key region between Indian Summer Monsoon and Mediterranean winter precipitation zones, as revealed from a lacustrine sequence from Lake Hamoun. Quat. Int. 408, 25–39. doi:10.1016/j.quaint.2015.11.011
Helwing, B., Vidale, M., and Fazeli Nashli, H. (2019). “Radiocarbon dates and absolute chronology,” in Sistan, Iran: stratigraphy, formation processes and chronology of a suburban site of shahr-i Sokhta. Editors H. A. Kavosh, M. Vidale, H. Fazeli Nashli, and T. Graziani (Roma: Antilia), 151–155.
Hoff, U., Rasmussen, T. L., Stein, R., Ezat, M. M., and Fahl, K. (2016). Sea ice and millennial-scale climate variability in the Nordic seas 90 kyr ago to present. Nat. Commun. 7 (1), 12247. doi:10.1038/ncomms12247
Hole, F. (2007). “Cycles of settlement in the Khorramabad Valley in luristan, Iran,” in Settlement and society: essays dedicated to robert McCormick adams. Editor E. Stone (Los Angeles: Cotsen Institute of Archaeology, University of California), 63–82.
Issar, A. S., and Zohar, M. (2007). Climate change: environment and history of the near east, 2nd ed., Springer, New York.
Jones, M., Djamali, M., Stevens, L., Heyvaert, V., Askari, H., Noorollahi, D., et al. (2013). “Mid-Holocene environmental and climatic change in Iran,” in Ancient Iran and its neighbours: local developments and long-range interactions in the 4th millennium BC. British institute for Persian studies. Editor C. Petrie (Oxford: Oxbow Books), 400.
Jones, M. D., Abu-Jaber, N., Al Shdaifat, A., Baird, D., Cook, B. I., Cuthbert, M. O., et al. (2019). 20,000 years of societal vulnerability and adaptation to climate change in southwest Asia. Wiley Interdiscip. Rev. Water 6 (2), e1330. doi:10.1002/WAT2.1330
Jones, M. D., Djamali, M., Holmes, J., Weeks, L., Leng, M. J., Lashkari, A., et al. (2015). Human impact on the hydroenvironment of Lake Parishan, SW Iran, through the late Holocene. Holocene 25 (10), 1651–1661. doi:10.1177/0959683615594242
Jones, M. D., and Roberts, C. N. (2008). Interpreting lake isotope records of Holocene environmental change in the Eastern Mediterranean. Quat. Int. 181 (1), 32–38. doi:10.1016/j.quaint.2007.01.012
Kaniewski, D., Paulissen, E., Van Campo, E., Weiss, H., Otto, T., Bretschneider, J., et al. (2010). Late second–early first millennium BC abrupt climate changes in coastal Syria and their possible significance for the history of the Eastern Mediterranean. Quat. Res. 74 (2), 207–215. doi:10.1016/j.yqres.2010.07.010
Kay, P. A., and Johnson, D. L. (1981). Estimation of Tigris-Euphrates streamflow from regional paleoenvironmental proxy data. Clim. Change 3 (3), 251–263. doi:10.1007/bf02423218
Kehl, M. (2009). Quaternary climate change in Iran—the state of knowledge. Erdkunde 63, 1–17. doi:10.3112/erdkunde.2009.01.01
Kirsten, F., Dallmeyer, A., Bernbeck, R., Böhmer, T., Busch, R., Hessari, M., et al. (2023). Were climatic forcings the main driver for mid-Holocene changes in settlement dynamics on the Varamin Plain (Central Iranian Plateau)? Plos one 18 (10), e0290181. doi:10.1371/journal.pone.0290181
Kotlia, B. S., Singh, A. K., Joshi, L. M., and Bisht, K. (2018). Precipitation variability over Northwest Himalaya from∼ 4.0 to 1.9 ka BP with likely impact on civilization in the foreland areas. J. Asian Earth Sci. 162, 148–159. doi:10.1016/j.jseaes.2017.11.025
Kutzbach, J. E., Chen, G., Cheng, H., Edwards, R. L., and Liu, Z. (2014). Potential role of winter rainfall in explaining increased moisture in the Mediterranean and Middle East during periods of maximum orbitally-forced insolation seasonality. Clim. Dyn. 42, 1079–1095. doi:10.1007/s00382-013-1692-1
Kuzucuoğlu, C., Dörfler, W., Kunesch, S., and Goupille, F. (2011). Mid-to late-Holocene climate change in central Turkey: the Tecer Lake record. Holocene 21 (1), 173–188. doi:10.1177/0959683610384163
Laskar, A. H., and Bohra, A. (2021). Impact of Indian summer monsoon change on ancient Indian civilizations during the Holocene. Front. Earth Sci. 9, 709455. doi:10.3389/feart.2021.709455
Lawler, A. (2011). Did the first cities grow from marshes? Science 331 (6014), 141. doi:10.1126/science.331.6014.141
Lawrence, D., Philip, G., and de Gruchy, M. W. (2022). Climate change and early urbanism in Southwest Asia: a review. Wiley Interdiscip. Rev. Clim. Change 13 (1), e741. doi:10.1002/wcc.741
Lemcke, G., and Sturm, M. (1997). “δ18O and trace element measurements as a proxy for the reconstruction of climate changes at Lake Van (Turkey): preliminary results,” in Third millennium BC climate change and Old World collapse (Berlin, Heidelberg: Springer Berlin Heidelberg), 653–678. doi:10.1007/978-3-642-60616-8_29
Madjidzadeh, Y., and Pittman, H. (2008). “Excavations at konar sandal in the region of Jiroft in the Halil basin: first preliminary report (2002–2008),” 46. 69–103. doi:10.1080/05786967.2008.11864738
Magee, P. (2013). “Iron age southeastern Iran,” in The oxford handbook of ancient Iran. Editor D. T. Potts (Oxford: OUP), 493–499.
Mancini-Lander, D. J. (2009). A history of Iran: empire of the mind by michael axworthy. American Journal of Islam and Society, 26, pp. 121–123. doi:10.35632/ajis.v26i4.1371
Manning, S. W., Lorentzen, B., Welton, L., Batiuk, S., and Harrison, T. P. (2020). Beyond megadrought and collapse in the Northern Levant: The chronology of Tell Tayinat and two historical inflection episodes, around 4.2 ka BP, and following 3.2 ka BP. PLoS One 15 (10), p.e0240799.
Marchetti, N., Bortolini, E., Menghi Sartorio, J. C., Orrù, V., and Zaina, F. (2024). Long-term urban and population trends in the Southern Mesopotamian floodplains. J. Archaeol. Res. 33, 117–158. doi:10.1007/s10814-024-09197-3
Maresca, G. (2018). Between ‘Early’ and ‘Late’ Iron Age in south-eastern Iran: notes on the possibility to evaluate the ‘Achaemenid impact’ on the area. Vicino Oriente 22, 197–211. doi:10.53131/vo2724-587x2018_14
Marsh, A., Fleitmann, D., Al-Manmi, D. A. M., Altaweel, M., Wengrow, D., and Carter, R. (2018). Mid-to late-Holocene archaeology, environment and climate in the northeast Kurdistan region of Iraq. Holocene 28 (6), 955–967. doi:10.1177/0959683617752843
Mashkour, M., Mohaseb, A., and Debue, K. (2006). ‘Towards a specialized subsistence economy in the marv Dasht plain: preliminary zooarchaeological analysis of tall-e Mushki, tall-e jari A and B, and tall-e Bakun A and B,’ in A. Alizadeh (ed.) The origins of state organizations in prehistoric highland Fars, Excavations at tall-e Bakun, 128. Southern Iran, Chicago: Oriental Institute Publications, pp. 101–105.
Mashkour, M., Tengberg, M., Shirazi, Z., and Madjidzadeh, Y. (2013). Bio-archaeological studies at Konar sandal, Halil Rud basin, southeastern Iran. Environ. Archaeol. 18 (3), 222–246. doi:10.1179/1749631413y.0000000006
Matthews, R., and Fazeli Nashli, H. (2022). The archaeology of Iran from the palaeolithic to the Achaemenid empire. London: Routledge, 613. doi:10.4324/9781003224129
Matthews, W. (2013). “Contexts of Neolithic interaction: geography, palaeoclimate, and palaeoenvironment of the Central Zagros,” in The earliest neolithic of Iran: 2008 excavations at sheikh-e abad and jani. Editors R. Matthews, W. Matthews, and Y. Mohammadifar (Oxford: Oxbow and BIPS), 13–19.
Mayewski, P. A., Meeker, L. D., Twickler, M. S., Whitlow, S., Yang, Q., Lyons, W. B., et al. (1997). Major features and forcing of high-latitude northern hemisphere atmospheric circulation using a 110,000-year-long glaciochemical series. J. Geophys. Res. Oceans 102 (C12), 26345–26366. doi:10.1029/96jc03365
McDonough, S. (2011). “The legs of the throne: kings, elites, and subjects in Sasanian Iran,” in The roman empire in context: historical and comparative perspectives. Editors J. P. Arnason, and K. A. Raaflaub (Malden: Wiley-Blackwell), 290–321.
McGee, D., Broecker, W. S., and Winckler, G. (2010). Gustiness: the driver of glacial dustiness? Quat. Sci. Rev. 29 (17-18), 2340–2350. doi:10.1016/j.quascirev.2010.06.009
McGee, D., deMenocal, P. B., Winckler, G., Stuut, J. B. W., and Bradtmiller, L. I. (2013). The magnitude, timing and abruptness of changes in North African dust deposition over the last 20,000 yr. Earth Planet. Sci. Lett. 371, 163–176. doi:10.1016/j.epsl.2013.03.054
Melville, C. (1984). Meteorological hazards and disasters in Iran: a preliminary survey to 1950. Iran 22, 113–150. doi:10.2307/4299740
Middleton, G. D. (2018). Bang or whimper? Science 361 (6408), 1204–1205. doi:10.1126/science.aau8834
Middleton, G. D. (2024). Collapse studies in archaeology from 2012 to 2023. J. Archaeol. Res. 33, 57–115. doi:10.1007/s10814-024-09196-4
Migowski, C., Stein, M., Prasad, S., Negendank, J. F., and Agnon, A. (2006). Holocene climate variability and cultural evolution in the Near East from the Dead Sea sedimentary record. Quat. Res. 66 (3), 421–431. doi:10.1016/j.yqres.2006.06.010
Miller, N. F. (1985). Paleoethnobotanical evidence for deforestation in ancient Iran: a case study of urban Malyan. J. Ethnobiol. 5 (1), 1.
Mutin, B. (2022). Les premiers villages agricoles de l’est du plateau Iranien à la vallée de l’Indus: état de la question. L'anthropologie 126 (3), 103050. doi:10.1016/j.anthro.2022.103050
Mutin, B., and Garazhian, O. (2019). Iranian-French archaeological mission in Bam, kerman: summary of field-seasons 2016-2017. Archaeol. J. 3 (4), 93–106.
Mutin, B., and Lamberg-Karlovsky, C. C. (2013). The proto-elamite settlement and its neighbors: Tepe Yahya period IVC Oxbow books. United States: American School of Prehistoric Research Publications.
Mutin, B., and Minc, L. (2019). The formative phase of the Helmand civilization, Iran and Afghanistan: new data from compositional analysis of ceramics from Shahr-i Sokhta, Iran. J. Archaeol. Sci. 23, 881–899. doi:10.1016/j.jasrep.2018.11.020
Neumann, F. H., Kagan, E. J., Schwab, M. J., and Stein, M. (2007). Palynology, sedimentology and palaeoecology of the late Holocene Dead Sea. Quat. Sci. Rev. 26 (11-12), 1476–1498. doi:10.1016/j.quascirev.2007.03.004
Neumann, J., and Parpola, S. (1987). Climatic change and the eleventh-tenth-century eclipse of Assyria and Babylonia. J. Near East. Stud. 46 (3), 161–182. doi:10.1086/373244
Ng, H. C., Robinson, L. F., McManus, J. F., Mohamed, K. J., Jacobel, A. W., Ivanovic, R. F., et al. (2018). Coherent deglacial changes in western Atlantic Ocean circulation. Nat. Commun. 9 (1), 2947. doi:10.1038/s41467-018-05312-3
Nicoll, K., and Küçükuysal, C. (2013). Emerging multi-proxy records of Late Quaternary palaeoclimate dynamics in Turkey and the surrounding region. Turkish J. Earth Sci. 22 (1), 126–142. doi:10.3906/yer-1109-7
Nishiaki, Y. (2010). A radiocarbon chronology for the neolithic settlement of tall-i Mushki, marv Dasht plain, Fars, Iran. Fars, Iran. Iran. 48 (1), 1–10. doi:10.1080/05786967.2010.11864769
Olszewski, D. I. (2012). The zarzian in the context of the epipaleolithic Middle East. Int. J. Humanit. 19 (3), 1–20.
Overpeck, J., Anderson, D., Trumbore, S., and Prell, W. (1996). The southwest Indian monsoon over the last 18 000 years. Clim. Dyn. 12, 213–225. doi:10.1007/s003820050104
Palmisano, A., Lawrence, D., de Gruchy, M. W., Bevan, A., and Shennan, S. (2021). Holocene regional population dynamics and climatic trends in the Near East: a first comparison using archaeo-demographic proxies. Quat. Sci. Rev. 252, 106739. doi:10.1016/j.quascirev.2020.106739
Paulette, T. (2012). Domination and resilience in Bronze Age Mesopotamia. Surviving sudden environmental change. Answers Archaeol. 167, 196. doi:10.2307/j.ctt1wn0rbs.12
Peasnall, B. L. (2002). “Iranian neolithic,” in Encyclopedia of prehistory: volume 8: south and southwest Asia (Boston, MA: Springer US), 215–235. doi:10.1007/978-1-4615-0023-0_22
Petrie, C. A. (2011). “Culture’, innovation and interaction across southern Iran from the neolithic to the Bronze age (6500–3000 BC),” in Investigating archaeological cultures: material culture, variability and transmission. Editors B. Roberts, and M. Vander Linden (Springer), 151–182.
Petrie, C. A., and Thomas, K. D. (2012). The topographic and environmental context of the earliest village sites in western South Asia. Antiquity 86 (334), 1055–1067. doi:10.1017/S0003598X00048249
Petrie, C. A., and Weeks, L. (2018). “The Iranian plateau and the Indus river basin,” in Climate Changes in the Holocene: Impacts and Human Adaptation (Boca Raton, Florida: CRC Press), 293–326. doi:10.1201/9781351260244-14
Petrie, C. A., Weeks, L. R., Potts, D. T., and Roustaei, K. (2009). “Perspectives on the cultural sequence of Mamasani,” in The Mamasani archaeological Project stage 1: the ICHTO/sydney university archaeological research Project in the Mamasani region, Fars province, Iran. Editors D. T. Potts, K. Roustaei, C. A. Petrie, and L. R. Weeks (Oxford: Archaeopress), 169–192.
Pfälzner, P., and Soleimani, N. A. (2017). The ICAR-University of Tübingen South-of-Jiroft Archaeological Surveys (SOJAS): results of the first season 2015. Archäologische Mittl. aus Iran Turan-Berlin. 47.
Pfälzner, P., Soleimani, N. A., and Karimi, M. (2019). SOJAS 2015–2018: a résumé of four seasons of archaeological survey south of Jiroft. Archaeology. J. Iran. Cent. Archaeol. Res. 2 (2), 107–124.
Pittman, H. (2013). “Eastern Iran in the early Bronze age,” in The oxford handbook of ancient Iran. Editor D. T. Potts (Oxford: OUP), 304–324.
Pollard, A. M., Fazeli Nashli, H., Davoudi, H., Sarlak, S., Helwing, B., and Saeidi, F. (2013). A new radiocarbon chronology for the North Central Plateau of Iran from the late neolithic to the Iron age. Archäologische Mittl. aus Iran Turan 45, 27–50.
Pollock, S., Bernbeck, R., and Eberhardt, G. (2023). “Resilience as an approach to lifeways in the Iranian highlands: an introduction,” in Coming to terms with the future: concepts of resilience for the study of early Iranian societies. Editors R. Bernbeck, G. Eberhardt, and S. Pollock (Leiden: Sidestone), 7–20.
Potts, D. T. (2001). Excavations at Tepe Yahya, Iran, 1967-1975: the third millennium. Peabody museum of archaeology and ethnology, harvard university publications department, 45. Cambridge, Massachusetts: Peabody Museum Press.
Prickett, M. (1986a). Man, land, and water: settlement distribution and the development of irrigation agriculture in the upper rud-i gushk drainage. Southeast. Iran. PhD Diss.
Prickett, M. (1986b). “Settlement during the early periods,” in Excavations at Tepe Yahya, Iran 1967-1976: the early periods, American school of prehistoric research bulletin. Editor T. W. Beale (Cambridge: Peabody Museum of Archaeology and Ethnology), 38, 215–246.
Rafiei-Alavi, B., Kehl, M., and Lahijani, H. A. K. (2023). “The impact of climate on human occupations in Iran from the neolithic to the early Iron age: an attempt to link archaeological and paleoclimate records,” in Coming to terms with the future: concepts of resilience for the study of early Iranian societies. Editors R. Bernbeck, G. Eberhardt, and S. Pollock (Leiden: Sidestone), 39–64.
Roberts, N., Eastwood, W. J., Kuzucuoğlu, C., Fiorentino, G., and Caracuta, V. (2011). Climatic, vegetation and cultural change in the eastern Mediterranean during the mid-Holocene environmental transition. Holocene 21 (1), 147–162. doi:10.1177/0959683610386819
Roberts, N., Jones, M. D., Benkaddour, A., Eastwood, W. J., Filippi, M. L., Frogley, M. R., et al. (2008). Stable isotope records of Late Quaternary climate and hydrology from Mediterranean lakes: the ISOMED synthesis. Quat. Sci. Rev. 27 (25-26), 2426–2441. doi:10.1016/j.quascirev.2008.09.005
Robinson, S. A., Black, S., Sellwood, B. W., and Valdes, P. J. (2006). A review of palaeoclimates and palaeoenvironments in the Levant and Eastern Mediterranean from 25,000 to 5000 years BP: setting the environmental background for the evolution of human civilisation. Quat. Sci. Rev. 25 (13-14), 1517–1541. doi:10.1016/j.quascirev.2006.02.006
Rodrigo-Gámiz, M., Martínez-Ruiz, F., Jiménez-Espejo, F. J., Gallego-Torres, D., Nieto-Moreno, V., Romero, O., et al. (2011). Impact of climate variability in the western Mediterranean during the last 20,000 years: oceanic and atmospheric responses. Quat. Sci. Rev. 30 (15-16), 2018–2034. doi:10.1016/j.quascirev.2011.05.011
Rosenberg, M. (2003). The epipalaeolithic in the marvdasht, in N. F. Miller, and K. Abdi (eds) Yeki bud, yeki nabud: essays on the archaeology of Iran in honor of william M. Sumner. 48. Los Angeles: Cotsen Institute of Archaeology, UCLA, pp. 98–108.
Safaierad, R., Matthews, R., Dupont, L., Zolitschka, B., Marinova, E., Djamali, M., et al. (2023). Vegetation and climate dynamics at the dawn of human settlement: multiproxy palaeoenvironmental evidence from the Hashilan Wetland, western Iran. J. Quat. Sci. 38 (8), 1289–1304. doi:10.1002/jqs.3557
Safaierad, R., Mohtadi, M., Zolitschka, B., Yokoyama, Y., Vogt, C., and Schefuß, E. (2020). Elevated dust depositions in West Asia linked to ocean–atmosphere shifts during North Atlantic cold events. Proc. Natl. Acad. Sci. 117 (31), 18272–18277. doi:10.1073/pnas.2004071117
Sarkar, A., Mukherjee, A. D., Bera, M. K., Das, B., Juyal, N., Morthekai, P., et al. (2016). Oxygen isotope in archaeological bioapatites from India: implications to climate change and decline of Bronze Age Harappan civilization. Sci. Rep. 6 (1), 26555. doi:10.1038/srep26555
Schmidt, A., Quigley, M., Fattahi, M., Azizi, G., Maghsoudi, M., and Fazeli Nashli, H. (2011). Holocene settlement shifts and palaeoenvironments on the Central Iranian Plateau: investigating linked systems. Holocene 21 (4), 583–595. doi:10.1177/0959683610385961
Schulman, B., Bar-Matthews, M., Almogi-Labin, A., and Luz, B. (2001). Global climate instability reflected by Eastern Mediterranean marine records during the late Holocene. Palaeogeogr. Palaeoclimatol. Palaeoecol. 176 (1-4), 157–176. doi:10.1016/S0031-0182(01)00336-4
Shahsavari, M., Xin, W., and Eskandari, N. (2024). Tom -e gavan: unveiling the early Iron age in the Jiroft plain, southeastern Iran. Archaeol. Res. Asia 37, 100500. doi:10.1016/j.ara.2024.100500
Shakun, J. D., Burns, S. J., Fleitmann, D., Kramers, J., Matter, A., and Al-Subary, A. (2007). A high-resolution, absolute-dated deglacial speleothem record of Indian Ocean climate from Socotra Island, Yemen. Earth Planet. Sci. Lett. 259 (3-4), 442–456. doi:10.1016/j.epsl.2007.05.004
Sharifi, A., Murphy, L. N., Pourmand, A., Clement, A. C., Canuel, E. A., Beni, A. N., et al. (2018). Early-Holocene greening of the Afro-Asian dust belt changed sources of mineral dust in West Asia. Earth Planet. Sci. Lett. 481, 30–40. doi:10.1016/j.epsl.2017.10.001
Sharifi, A., Pourmand, A., Canuel, E. A., Ferer-Tyler, E., Peterson, L. C., Aichner, B., et al. (2015). Abrupt climate variability since the last deglaciation based on a high-resolution, multi-proxy peat record from NW Iran: the hand that rocked the Cradle of Civilization? Quat. Sci. Rev. 123, 215–230. doi:10.1016/j.quascirev.2015.07.006
Sharma, S., Joachimski, M., Sharma, M., Tobschall, H. J., Singh, I. B., Sharma, C., et al. (2004). Lateglacial and Holocene environmental changes in ganga plain, northern India. Quat. Sci. Rev. 23 (1-2), 145–159. doi:10.1016/j.quascirev.2003.10.005
Shoaee, M. J., Nasab, H. V., and Petraglia, M. D. (2021). The Paleolithic of the Iranian Plateau: hominin occupation history and implications for human dispersals across southern Asia. J. Anthropol. Archaeol. 62, 101292. doi:10.1016/j.jaa.2021.101292
Singarayer, J. S., Valdes, P. J., and Roberts, W. H. (2017). Ocean dominated expansion and contraction of the late Quaternary tropical rainbelt. Sci. Rep. 7 (1), 9382. doi:10.1038/s41598-017-09816-8
Sinha, A., Kathayat, G., Weiss, H., Li, H., Cheng, H., Reuter, J., et al. (2019). Role of climate in the rise and fall of the Neo-Assyrian Empire. Sci. Adv. 5 (11), eaax6656. doi:10.1126/sciadv.aax6656
Sirocko, F., Sarnthein, M., Erlenkeuser, H., Lange, H., Arnold, M., and Duplessy, J. C. (1993). Century-scale events in monsoonal climate over the past 24,000 years. Nature 364 (6435), 322–324. doi:10.1038/364322a0
Soleimani, M., Nadimi, A., Koltai, G., Dublyansky, Y., Carolin, S., and Spötl, C. (2023). Stalagmite evidence of Last Glacial Maximum to early Holocene climate variability in southwestern Iran. J. Quat. Sci. 38 (3), 308–318. doi:10.1002/jqs.3478
Stager, J. C., Ryves, D. B., Chase, B. M., and Pausata, F. S. (2011). Catastrophic drought in the Afro-Asian monsoon region during Heinrich event 1. Science 331 (6022), 1299–1302. doi:10.1126/science.1198322
Staubwasser, M., Sirocko, F., Grootes, P. M., and Segl, M. (2003). Climate change at the 4.2 ka BP termination of the Indus Valley civilization and Holocene South Asian monsoon variability. Geophys. Res. Lett. 30 (8). doi:10.1029/2002GL016822
Staubwasser, M., and Weiss, H. (2006). Holocene climate and cultural evolution in late prehistoric–early historic West Asia. Quat. Res. 66 (3), 372–387. doi:10.1016/j.yqres.2006.09.001
Stevens, L. R., Ito, E., Schwalb, A., and Wright, H. E. (2006). Timing of atmospheric precipitation in the Zagros Mountains inferred from a multi-proxy record from Lake Mirabad, Iran. Quat. Res. 66 (3), 494–500. doi:10.1016/j.yqres.2006.06.008
Stevens, L. R., Ito, E., and Wright, H. E. (2008). Variations in effective moisture at Lake Zeribar, Iran, during the last glacial period and Holocene, inferred from the δ18O values of authigenic calcite. Diatom Monogr. 8, 377.
Stevens, L. R., Wright Jr, H. E., and Ito, E. (2001). Proposed changes in seasonality of climate during the lateglacial and Holocene at Lake Zeribar, Iran. Holocene 11 (6), 747–755. doi:10.1191/09596830195762
Sumner, W. M. (1986). Achaemenid settlement in the Persepolis plain. Am. J. Archaeol. 90 (1), 3–31. doi:10.2307/505980
Sumner, W. M. (1989). “Anshan in the Kaftari phase: patterns of settlement and land use,” in Archaeologia Iranica et Orientalis. Editors L. De Meyer, and E. Haerinck (Ghent, Belgium: Peeters Press), 135–161.
Sumner, W. M. (1990). “Full-coverage regional archaeological survey in the Near East: an example from Iran,” in The archaeology of regions. A case for full-coverage survey. Washington, DC: Smithsonian Institution Press, 87–115.
Sumner, W. M. (1994). “The evolution of tribal society in the southern Zagros mountains, Iran,” in Chiefdoms and early states in the near east: the organizational dynamics of complexity. Monographs in world archaeology 18. Editors M. S. Rothman, and G. Stein (Madison: Prehistory Press), 47–65.
Taylor, H. (2015). Cultural integration in intermontane zones: the emergence of social complexity in southwestern Iran. Cambridge, England: University of Cambridge. [Doctoral Thesis].
Tengberg, M. (2012). Beginnings and early history of date palm garden cultivation in the Middle East. J. Arid Environ. 86, 139–147. doi:10.1016/j.jaridenv.2011.11.022
Thompson, W. R. (2006). Climate, water, and crises in the Southwest asian Bronze age. Nat. Cult. 1 (1), 88–132. doi:10.3167/155860706780271999
Thornton, C. P. (2012). “Iran,”. A companion to the archaeology of the ancient near east. Editors D. T. Potts (Chichester: John Wiley & Sons), 596–606. doi:10.1002/9781444360790.ch31
Toucanne, S., Soulet, G., Freslon, N., Jacinto, R. S., Dennielou, B., Zaragosi, S., et al. (2015). Millennial-scale fluctuations of the European ice sheet at the end of the last glacial, and their potential impact on global climate. Quat. Sci. Rev. 123, 113–133. doi:10.1016/j.quascirev.2015.06.010
Tsuneki, A. (2013). “Proto-Neolithic caves and neolithisation in the southern Zagros,” in The neolithisation of Iran: the formation of new societies. Editors R. Matthews, and H. Fazeli Nashli (Oxford: Oxbow Books), 84–96.
Turchin, P., Adams, J. M., and Hall, T. D. (2006). East-west orientation of historical empires and modern states. J. World-Systems Res., 219–229. doi:10.5195/jwsr.2006.369
Vaezi, A., Ghazban, F., Tavakoli, V., Routh, J., Beni, A. N., Bianchi, T. S., et al. (2019). A Late Pleistocene-Holocene multi-proxy record of climate variability in the Jazmurian playa, southeastern Iran. Palaeogeogr. Palaeoclimatol. Palaeoecol. 514, 754–767. doi:10.1016/j.palaeo.2018.09.026
Vaezi, A., Routh, J., Djamali, M., Gurjazkaite, K., Tavakoli, V., Beni, A. N., et al. (2022). New multi-proxy record shows potential impacts of precipitation on the rise and ebb of Bronze Age and imperial Persian societies in southeastern Iran. Quat. Sci. Rev. 298, 107855. doi:10.1016/J.QUASCIREV.2022.107855
Vahdati Nasab, H., Shirvani, S., and Rigaud, S. (2019). The northern Iranian Central Plateau at the end of the Pleistocene and early Holocene: the emergence of domestication. J. World Prehistory 32, 287–310. doi:10.1007/s10963-019-09133-0
Vallini, L., Zampieri, C., Shoaee, M. J., Bortonlini, E., Marciani, G., Aneli, S., et al. (2024). The Persian plateau served as hub for Homo sapiens after the main out of Africa dispersal. Nat. Commun. 15, 1882. doi:10.1038/s41467-024-46161-7
Van Zeist, W., and Bottema, S. (1977). Palynological investigations in western Iran. Palaeohistoria, 19–85.
Van Zeist, W., and Wright Jr, H. E. (1963). Preliminary pollen studies at Lake Zeribar, Zagros mountains, southwestern Iran. Science 140 (3562), 65–67. doi:10.1126/science.140.3562.65
Verheyden, S., Nader, F. H., Cheng, H. J., Edwards, L. R., and Swennen, R. (2008). Paleoclimate reconstruction in the Levant region from the geochemistry of a Holocene stalagmite from the Jeita cave, Lebanon. Quat. Res. 70 (3), 368–381. doi:10.1016/j.yqres.2008.05.004
Vidale, M., and Desset, F. (2013). “Mahtoutabad I (Konar Sandal South, Jiroft): preliminary evidence of occupation of a Halil Rud site in the early fourth millennium BC,” in Ancient Iran and its neighbours: local developments and long-range interactions in the fourth millennium BC. Editor C. A. Petrie (Oxford: Oxbow), 233–251.
Wang, Y. J., Cheng, H., Edwards, R. L., An, Z. S., Wu, J. Y., Shen, C. C., et al. (2001). A high-resolution absolute-dated late Pleistocene monsoon record from Hulu Cave, China. Science 294 (5550), 2345–2348. doi:10.1126/science.1064618
Wasylikowa, K., and Witkowski, A. (2008). The palaeoecology of Lake Zeribar and surrounding areas, western Iran, during the last 48,000 years. Ruggell, Liechtenstein: Gantner. 8.
Weeks, L. R. (2013). “The development and expansion of a neolithic way of life,” in The oxford handbook of ancient Iran.
Weiss, B. (1982a). The decline of Late Bronze Age civilization as a possible response to climatic change. Clim. Change 4, 173–198. doi:10.1007/bf00140587
Weiss, B. (1982b). The decline of Late Bronze Age civilization as a possible response to climatic change. Clim. Change 4 (2), 173–198. doi:10.1007/BF02423389
Weiss, H. (2016). Global megadrought, societal collapse and resilience at 4.2-3.9 ka BP across the Mediterranean and west Asia. 9 ka BP across Mediterr. west Asia 24, 62–63. doi:10.22498/pages.24.2.62
Weiss, H., and Bradley, R. S. (2001). What drives societal collapse? Science 291 (5504), 609–610. doi:10.1126/science.1058775
Weiss, H., Courty, M. A., Wetterstrom, W., Guichard, F., Senior, L., Meadow, R., et al. (1993). The genesis and collapse of third millennium north Mesopotamian civilization. Science 261 (5124), 995–1004. doi:10.1126/science.261.5124.995
Wick, L., Lemcke, G., and Sturm, M. (2003). Evidence of Late glacial and Holocene climatic change and human impact in eastern Anatolia: high-resolution pollen, charcoal, isotopic and geochemical records from the laminated sediments of Lake Van, Turkey. Holocene 13 (5), 665–675. doi:10.1191/0959683603hl653rp
Keywords: palaeoclimate, aeolian, monsoon, westerlies, settlements, Persian empires
Citation: Vaezi A, Weeks L and Routh J (2025) From the Last Glacial Maximum to the Late Holocene: Unraveling the dynamic complexities of climate and human history in Western and Southeastern Iran. Front. Earth Sci. 13:1477290. doi: 10.3389/feart.2025.1477290
Received: 07 August 2024; Accepted: 17 February 2025;
Published: 17 March 2025.
Edited by:
Davide Tiranti, Agenzia Regionale per la Protezione Ambientale del Piemonte (Arpa Piemonte), ItalyReviewed by:
Blas Lorenzo Valero Garcés, Spanish National Research Council (CSIC), SpainCopyright © 2025 Vaezi, Weeks and Routh. This is an open-access article distributed under the terms of the Creative Commons Attribution License (CC BY). The use, distribution or reproduction in other forums is permitted, provided the original author(s) and the copyright owner(s) are credited and that the original publication in this journal is cited, in accordance with accepted academic practice. No use, distribution or reproduction is permitted which does not comply with these terms.
*Correspondence: Joyanto Routh, am95YW50by5yb3V0aEBsaXUuc2U=; Alireza Vaezi, YWwudmFlemlAeWFob28uY29t
Disclaimer: All claims expressed in this article are solely those of the authors and do not necessarily represent those of their affiliated organizations, or those of the publisher, the editors and the reviewers. Any product that may be evaluated in this article or claim that may be made by its manufacturer is not guaranteed or endorsed by the publisher.
Research integrity at Frontiers
Learn more about the work of our research integrity team to safeguard the quality of each article we publish.