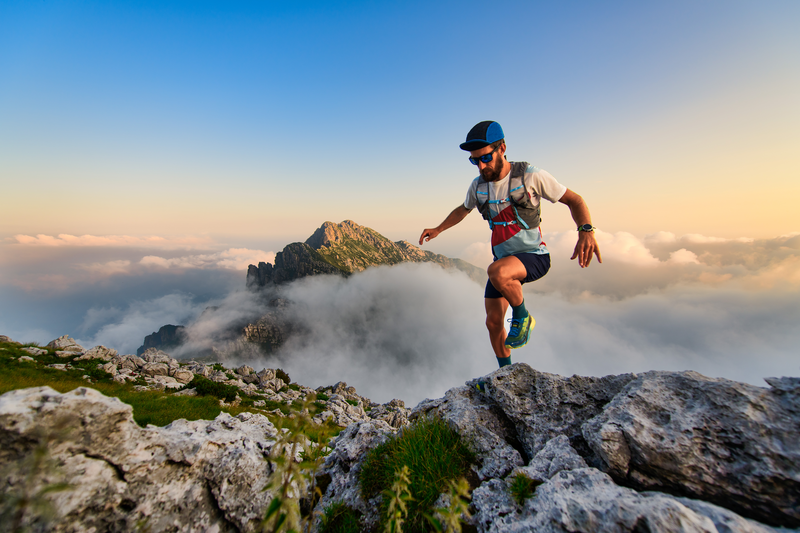
95% of researchers rate our articles as excellent or good
Learn more about the work of our research integrity team to safeguard the quality of each article we publish.
Find out more
ORIGINAL RESEARCH article
Front. Earth Sci. , 03 April 2025
Sec. Solid Earth Geophysics
Volume 13 - 2025 | https://doi.org/10.3389/feart.2025.1456257
This article is part of the Research Topic Experimental and Numerical Simulations of Rock Physics View all 17 articles
The Changling fault depression in the southern Songliao Basin hosts volcanic rock reservoirs with significant oil and gas potential, yet their complex pore structures require advanced characterization to optimize resource exploration. To address this, we employed multi-scale computed tomography (CT) imaging combined with mercury intrusion porosimetry (MIP) to analyze three representative volcanic rock samples through three-tier micrometer CT scanning. This integrated approach enabled the construction of a multi-scale digital rock model, facilitating qualitative and quantitative evaluations of pore-throat structures across diverse storage spaces. Results revealed detailed three-dimensional distributions of porosity, connectivity, and pore-throat radii, demonstrating pronounced heterogeneity in reservoir properties. The results demonstrate that integrating multi-scale CT scanning with mercury intrusion data provides a robust method for characterizing the three-dimensional pore distribution effectively. This research provides an in-depth understanding of the microscopic characteristics of volcanic rock reservoirs and establishes a scientific and technical foundation for advancing the exploration and development of oil and gas resources.
Reservoir characterization has traditionally relied on methods such as core observation, thin section identification, mercury intrusion porosimetry (MIP), and scanning electron microscopy (SEM) (Anovitz and Cole, 2015; Dong et al., 2024). Each of these techniques, however, has inherent limitations. Core observation is restricted to external features and lacks the capability to investigate the internal pore structures. Thin section identification, while valuable for detailed mineralogical and textural analysis, is confined to two-dimensional assessments and cannot capture the three-dimensional pore architecture (Bai et al., 2013; Liu et al., 2020). Similarly, SEM provides high-resolution imagery of micropore morphology and dimensions but is also limited to two-dimensional analysis (Bera and Shah, 2021; Chalmers et al., 2012). MIP quantifies accessible porosity and pore-throat sizes through mercury penetration under pressure, but only addresses interconnected pore spaces, neglecting isolated pores (Kuila, 2013; Njiekak et al., 2018; Qajar and Arns, 2022). In contrast, computed tomography (CT) imaging represents a significant advancement in reservoir characterization by enabling non-destructive, three-dimensional visualization of rock samples (Dong et al., 2019; Cnudde and Boone, 2013; Ma et al., 2016; Mees et al., 2003). CT imaging allows for the extraction of detailed microstructural pore-throat parameters across a continuum of scales (Ma and Liu, 2011; Bai et al., 2013; Ma et al., 2016; Li et al., 2016; Liu X. F. et al., 2021). This facilitates a comprehensive spatial and quantitative evaluation of reservoir quality, critical for accurate reservoir characterization (Ma et al., 2017; Remeysen and Swennen, 2008; Dong et al., 2018). However, due to the heterogeneity in pore sizes within core samples, ranging from nanometers to millimeters, capturing the full spectrum of pore characteristics requires multiple resolutions during CT scanning.
The Changling fault depression of the Southern Songliao Basin holds immense potential for oil and gas resources, with multiple volcanic gas reservoirs already discovered in the Yingcheng and Huoshiling formations (Zou et al., 2012; Wang et al., 2015; Miao et al., 2020; Li et al., 2023). Despite the promising resource potential, complex geological structures and uncertainties in reservoir microscopic features pose substantial obstacles to oil and gas exploration and development in the region (Zheng et al., 2021; Qu et al., 2022; Li et al., 2023). Hence, advancing reservoir studies, particularly on pore structure characteristics, is essential for effectively evaluating and predicting reservoirs (Liu et al., 2017; Liu B. et al., 2021). This is vital for achieving breakthroughs in the exploration of volcanic oil and gas resources in the Songliao Basin. The Flint Ridge Formation, studied herein, developed in the early stage of fault basin formation and was filled during the fault’s initial stages. It lies in unconformity contact with the underlying strata and exhibits deposition thicknesses exceeding 800 m. The lithology is predominantly gray-black mudstone interspersed with gray fine sandstone in the lower part, transitioning to volcanic breccia, andesite, and tuff in the upper part. While multi-scale CT imaging has been successfully applied to sedimentary reservoirs (Dong et al., 2019; Ma et al., 2017), its integration with mercury intrusion data for characterizing volcanic rock reservoirs—particularly in the Songliao Basin—remains underexplored. This study represents the first systematic application of this approach to address the unique challenges posed by the heterogeneity of volcanic pore networks in the Changling fault depression.
To address the complex exploration challenges in the Changling fault depression, this study employed a three-scale micrometer-resolution CT scanning protocol on three representative volcanic rock reservoir samples. By integrating CT imaging with MIP, a multi-scale pore network digital core model was developed, significantly enhancing understanding of the reservoir’s microstructural characteristics.
Qualitative analyses provided a detailed description of the three-dimensional pore-throat structures across various types of storage spaces within these volcanic rocks. Quantitative evaluations calculated critical parameters such as porosity, connectivity, and pore-throat radii distribution. This combined approach enabled a comprehensive characterization of the microscopic features of volcanic rock reservoirs.
This study not only advances the understanding of microstructural attributes influencing fluid flow and trapping mechanisms in volcanic reservoirs but also provides a scientific and technical basis for optimizing exploration and development strategies in the Changling fault depression. Moreover, it demonstrates the value of integrating multi-scale imaging and analytical techniques to gain critical insights into reservoir quality and performance, offering a robust foundation for oil and gas exploration and development.
In this study, 64 core samples were obtained from 11 wells drilled in the Huoshiling Formation of the Changling fault depression. These cores encompass lithologies such as tuff, tuffite, breccia, and andesite. Three representative samples—rhyolitic volcanic breccia, crystal tuff, and tuffite—were selected based on (1) lithological abundance (>60% of the Huoshiling Formation’s volcanic sequences), (2) distinct pore structure characteristics, and (3) resource significance. Although andesite is present in the formation, it was excluded due to its limited abundance (<15%) and low porosity (<2% in preliminary helium tests). Samples were drilled from texturally homogeneous zones to minimize intra-lithology variability.
The analysis began with a macroscopic examination of the full-diameter samples via initial CT scanning. This step provided an overview of the structural composition and facilitated preliminary identification of heterogeneities within the samples. Subsequently, thin sections were prepared to aid in accurate rock typing and the identification of pore types, further refining the geological characterization of the reservoirs.
Following these macroscopic and microscopic assessments, centimeter plugs were drilled from the primary samples for quantitative evaluation. Helium porosity measurements were performed on centimeter plugs (samples ①–③) using a ECK-III porosimeter following GB/T 29172-2012. Helium porosity measurements conducted on these plugs revealed porosities of 8.21%, 3.57%, and 0.34% for samples ①, ②, and ③, respectively. These values highlight the significant variability in porosity among the samples, which reflects their distinct textural and compositional properties.
To further explore pore-throat size distributions and connectivity, high-pressure mercury intrusion tests were performed. High-pressure mercury intrusion tests utilized a Micromeritics AutoPore IV 9505 on subsamples from the CT-scanned plugs, with pore-throat radii calculated via the Washburn equation.
This study employed a comprehensive methodology to characterize volcanic rock features at multiple scales using advanced imaging techniques. The workflow included multi-scale core CT imaging, precise image registration, multi-scale image segmentation, and multi-scale pore network modeling and analysis.
The primary equipment utilized in this study is the nanoVoxel-3502E CT scanner, manufactured by Tianjin Sanying Precision Instruments Co., Ltd., China. This state-of-the-art scanner has a maximum spatial resolution of 0.5 µm and can hold sample diameters of up to 30 cm. This equipment can carry out a multi-scale CT scanning across a diverse range of sample sizes, thereby enabling detailed and accurate visualization of geological samples from the macro to the micro scale.
Each sample undertook an initial CT scanning at a resolution of 80 µm. This scanning provided a comprehensive view of the entire core, capturing macroscopic features that were essential for initial assessments and further detailed studies. Following the full diameter scanning, a standard plug of 2.5 cm diameter was extracted from the core for higher resolution scanning at 16 µm. This step was essential for examining finer structural details that were not discernible at lower resolutions. To get even greater details, particularly focusing on the microporosity of the core, a 4 mm diameter millimeter plug sample was drilled using a precision millimeter drill bit. These smaller samples were scanned at an ultra-high resolution of 2.6 µm, enhancing the visibility of the smaller scale pore structures. This level of detail is pivotal for accurately characterizing the microporosity and determining the connectivity and distribution of pore-throats within the volcanic rock.
To align CT images across three distinct scales—millimeter plugs, centimeter plugs, and full diameter cores—a hybrid registration method is utilized. This method combines algorithms of image features and grayscale information to optimize the accuracy of alignment (Cui et al., 2017; Cui et al., 2020). This dual approach allows for effective handling of the different resolutions and complexities encountered in multi-scale imaging, ensuring that features across scales are accurately matched and the continuity of geological features is maintained. Image registration was implemented with MATLAB R2021a (MathWorks) using custom scripts based on the hybrid registration method. Image processing and analysis were performed using Avizo 9.0 (Thermo Fisher Scientific) for 3D visualization.
Multi-scale image segmentation plays a crucial role in the multi-scale characterization process, allowing for a detailed analysis of porosity and structural features across different scales. This step ensures that corresponding features across the scales are accurately matched, facilitating a coherent analysis across different resolutions. To simplify the computational process and optimize the analysis, the largest square area within the cylindrical scanned data is extracted for segmentation. This approach focuses the segmentation process on the core area of the sample, minimizing edge effects and ensuring the most representative data is analyzed. Segmentation is performed sequentially, starting from the smallest scale (millimeter plug) to the largest (full diameter core). This hierarchical approach allows for detailed analysis at smaller scales to inform the broader, more generalized segmentation at larger scales.
The segmentation process is structured in several steps to effectively differentiate between various rock components such as pores and minerals: (1) Initially, threshold segmentation was applied to high-resolution images using Otsu’s method (Otsu, 1979) to distinguish pores from the matrix; (2) In the registered overlapping image regions, a low-resolution voxel corresponds to N3 voxels at a higher resolution (where N is the resolution ratio). By analyzing the proportion of components within these regions, the grayscale values of the low-resolution images are calibrated against a curve that represents the average distribution of the grayscale-component proportion. This calibration is crucial for accurately scaling up detailed pore and mineral information to larger voxel scales; (3) Utilizing the established grayscale-porosity distribution curve, porosity values are assigned to each voxel in the low-resolution images, ranging from 0 to 1, to depict the distribution of porosity accurately; (4) Following the methodology used for porosity, grayscale values are similarly used to assign values to different mineral components across the scales. These steps are applied progressively to achieve multi-scale image segmentation from millimeter plugs to centimeter plugs to full diameter cores.
The modeling of the pore network also follows a structured multi-scale approach (Jiang et al., 2012; Jiang et al., 2013; Ma et al., 2017), which was implemented through the following steps: (1) Multi-scale model extraction. Pore network models were extracted at each scale using the maximal ball algorithm (Dong and Blunt, 2009). This algorithm identifies pores as maximal spheres fitting within void spaces and connects them via throats (narrowest constrictions between pores). At each resolution, the extracted networks captured pore-throat radii distributions, coordination numbers, and spatial connectivity specific to their scale. (2) Fine-scale model generation. For sub-resolution pores below the CT detection limit, a stochastic modeling approach was adopted. Based on MIP data, we generated synthetic pore networks, matching the cumulative pore size distribution derived from MIP curves. This hybrid approach ensured representation of both resolved and unresolved porosity. (3) Cross-scale model coupling. Statistical distributions of throat properties from finer-scale models are used to bridge coarse scale pores and fine scale pores.
The multi-scale scale CT imaging of the rhyolitic volcanic breccia reveal significant insights into the internal structure and porosity of the rock at varying scales (Figure 1).
Figure 1. Three-scale CT scan images of rhyolitic volcanic breccia (A, D): show the large-scale structure of the full diameter sample; (B, E): show the mineral and part of the pore structure of centimeter plug sample; (C, F): show finer mineral and pore structure of millimeter plug sample.
The initial full diameter scanning at 80 µm resolution provides a broad view of the rhyolitic volcanic breccia, showcasing its clastic lava structure. The largest observable clasts measure approximately 3 cm, indicating a heterogeneously distributed coarser grain structure typical of breccia. At this scale, some areas of pore development are visible, offering initial insights into the porosity of the sample. However, due to the relatively coarse resolution, finer details of pore structures are not discernible, which may underrepresent the total porosity and complexity of the pore network.
The increase in resolution in the centimeter plug scanning allows for a better identification of larger pores. This mid-scale imaging provides a more detailed view of the porosity that was only partially visible in the full diameter core scans. These images are critical for assessing the connectivity and size distribution of the larger pores, which are essential factors in evaluating the potential fluid flow and storage capacity of the reservoir.
The highest resolution imaging of the millimeter plugs reveals the finest details of the rock’s internal structure. This level of detail is crucial for understanding the micro-scale features that influence the overall properties of volcanic rocks. The scans clearly display the distribution of cracks and smaller-sized pores. These features significantly impact the mechanical properties of the rock, including its permeability and structural integrity. At this scale, the shapes and boundaries of clastic structures become more distinct.
The results from the three-scale CT imaging of the crystal tuff provide detailed insights into the structural and porosity characteristics of the rock, revealing significant variations at different scales (Figure 2).
Figure 2. Three-scale CT scan images of crystal tuff. (A, D): show the large-scale structure of the full diameter sample; (B, E): show the mineral and part of the pore structure of centimeter plug sample; (C, F): show finer mineral and pore structure of millimeter plug sample.
The CT images of the full diameter core indicate that the crystal tuff exhibits a good level of homogeneity, with no large or clearly visible pores. This suggests a dense matrix with potentially minor macroscopic porosity, which could imply limited macroscopic fluid flow paths within this scale. The lack of large pores might indicate a relatively intact rock structure, which is beneficial for certain types of structural stability but might limit reservoir capacity at this scale.
At this intermediate scale of the centimeter plug CT scanning, the emergence of some large pores becomes evident. These features were not visible at the lower resolution of the full diameter scan, showcasing the importance of examining different scales to capture varying pore sizes. The presence of these larger pores could enhance the potential fluid storage and permeability of the rock, indicating more favorable reservoir properties than initially suggested by the full diameter core scan.
The highest resolution scan of the millimeter plug reveals the development of micropores, predominantly characterized as intracrystalline dissolution pores. These types of pores are typically formed by the chemical alteration of minerals, particularly feldspar, within the rock matrix. The clear visibility of angular feldspar grains within the matrix underscores the mineralogical composition of the tuff. Feldspar, being a primary component, plays a significant role in the rock’s overall behavior, including its reaction to geochemical processes and its mechanical properties.
The CT imaging results from the three-scale examination of the Tuffite provide critical insights into the structural integrity and porosity characteristics of this type of volcanic rock (Figure 3).
Figure 3. Three-scale CT scan images of tuffite. (A, D): show the large-scale structure of the full diameter sample; (B, E): show the mineral and part of the pore structure of centimeter plug sample; (C, F): show finer mineral and pore structure of millimeter plug sample.
The full diameter core CT images reveal a high degree of homogeneity with a notable absence of large pores. This suggests a compact rock matrix with limited macroscopic porosity, which could imply restricted large-scale fluid flow within this sample at this resolution.
The centimeter plug images uncover the development of horizontal fractures, which appear as vertical fractures in the CT images. The presence of these fractures is crucial as they could represent potential pathways for fluid flow despite the overall low porosity observed. The same images display hardly any visible pores, emphasizing the rock’s structural density and the likely reliance on fractures for any significant permeability.
The millimeter plug CT imaging suggest pores are not developed, reinforcing the findings from larger scales regarding the compact nature of the rock. However, the detailed imaging reveals mineral debris particles, predominantly quartz and feldspar, with varying degrees of rounding. A significant portion of the rock matrix is bound by clay cement, with clay content constituting about 30% of the composition. This high clay content could significantly impact the mechanical properties of the rock, potentially influencing its brittleness and reducing permeability.
The CT images of the crystal tuff sample at different scales (full diameter, centimeter plug, and millimeter plug) are aligned using image registration techniques as an example (Figure 4). After the imaging process, we get the CT grayscale images and pore segmentation results of rhyolitic volcanic breccia, crystal tuff, and Tuffite at three scales (Figures 5–7). The segmentation at the millimeter scale is precise, capturing detailed pore structures and microporosity. This high-resolution segmentation provides crucial insights into the finest porosity features, which are essential for understanding fluid flow at the microscopic level. At the centimeter scale, the segmentation is probabilistic, reflecting the variation and distribution of pores as observed in the higher resolution data. This intermediate scale bridges the detailed microporosity and the more general macroscopic features, offering a balanced view of porosity distribution. The largest scale employs a probabilistic segmentation approach as well, which captures the overall three-dimensional distribution of porosity. While this scale provides less detail, it is crucial for understanding the general porosity trends and guiding macroscopic analyses and interpretations.
Figure 4. Registration results of CT images of crystal tuff. The same view of (A): Full diameter sample; (B): Centimeter plug sample; (C): Millimeter plug sample.
Figure 5. CT grayscale images and pore segmentation results of rhyolitic volcanic breccia at three scales (A, D): Full diameter sample; (B, E): Centimeter plug sample; (C, F): Millimeter plug sample.
Figure 6. CT grayscale images and pore segmentation results of crystal tuff at three scales. (A, D): Full diameter sample; (B, E): Centimeter plug sample; (C, F): Millimeter plug sample.
Figure 7. CT grayscale images and pore segmentation results of tuffite at three scales. (A, D): Full diameter sample; (B, E): Centimeter plug sample; (C, F): Millimeter plug sample.
In order to construct a multi-scale integrated digital rock core model, it is necessary to calculate the porosity proportions at different pore sizes. Using gas-measured porosity as the standard for total porosity, the porosity proportions of three sample groups are statistically analyzed across four intervals: less than 0.1 µm, 0.1–1 μm, 1–10 μm, and greater than 10 μm, in terms of their contribution to the total porosity (Table 1).
The digital rock core pore network model is progressively integrated across different scales. In the millimeter pore network digital rock core model, nanoscale pores reflected by mercury intrusion data are filled using a random filling method. This model serves as the input to fill the centimeter pore network digital rock core model, and ultimately the full diameter model. The final multi-scale pore network digital rock core model integrates mercury intrusion and three different CT scale pore data (Figure 8), aligning the digital rock core porosity with the gas-measured porosity.
Figure 8. Multi-scale integrated pore network model (A) Rhyolitic volcanic breccia (B) Crystal tuff (C) Tuffite.
Based on the integrated multi-scale pore network model, the full-scale pore radius distribution is calculated (Figure 9). The pore radius here represents equivalent spherical radius, calculated using the maximal inscribed sphere algorithm (Dong and Blunt, 2009). The pore radius distributions of the three sample groups all show a multimodal distribution, with detailed pore and throat parameters presented in Table 2. The integrated model provides parameters such as connected porosity, number of pores, pore radius distribution, number of throats, and throat radius distribution. Based on this model, the effectiveness and distribution of porosity can be accurately assessed. Combined with the frequency distribution of storage spaces, this can provide a robust basis for reservoir identification and prediction.
The segmentation results and subsequent analyses of the three sample groups reveal intricate details about the relationship between reservoir lithology and pore distribution. Each type of volcanic tuff exhibits unique pore characteristics that significantly affect the reservoir’s storage capacity and flow dynamics.
This sample predominantly exhibits intergranular, fissure, and dissolution pores. Porosity measurements across scales (2.1%–2.4%) indicate a relatively uniform micrometer-scale pore distribution. However, these account for only 30% of the gas-measured porosity, suggesting that significant nanometer-scale pores remain undetected by CT imaging. The pore distribution is unimodal and skewed, with micrometer-scale pores significantly larger than nanometer-scale ones, as depicted by the normalized pore radius distribution curve (Figure 10A).
Figure 10. Pore radius distribution based on mercury intrusion and CT imaging. (A) Rhyolitic volcanic breccia (B) Crystal tuff (C) Tuffite.
The primary storage spaces in this sample are devitrification and dissolution pores (Figure 10B). The core porosity is extremely low (0.4%) compared to gas-measured porosity, indicating a substantial presence of nanometer-scale pores. The unimodal and skewed distribution curve reveals a wide range of pore sizes, with devitrification and dissolution processes playing critical roles in pore formation.
This sample is characterized by sparse development of storage spaces, primarily interstitial pores among clay minerals, with occasional fractures (Figure 10C). The very low porosity (0.01%) accounts for only 3% of the gas-measured porosity, highlighting the dominance of nanometer-scale pores. The multimodal, skewed distribution reflects a complex interplay between minimal interstitial porosity and sporadic larger fractures.
The lithological differences among rhyolitic, crystal tuffs, and tuffite significantly influence pore characteristics and reservoir behavior. Rhyolitic tuffs feature relatively higher micrometer-scale porosity, while crystal tuff and tuffite exhibit predominantly nanometer-scale pores and limited macroscopic porosity. These variations are critical for reservoir modeling and fluid flow predictions.
The presence of substantial nanometer-scale pores across all samples emphasizes the importance of using complementary techniques, such as MIP, alongside CT imaging. This dual approach captures both micrometer- and nanometer-scale porosity, enabling a comprehensive understanding of reservoir properties. Effective reservoir management must incorporate these insights to optimize fluid flow modeling and recovery strategies.
In conclusion, integrating CT imaging with MIP provides a robust framework for assessing volcanic rock reservoirs. The lithological control on pore distribution is a key factor in defining reservoir quality, performance, and potential exploitation strategies.
The application of multi-scale CT imaging provides a comprehensive view of volcanic rock structural and porosity characteristics, from macroscopic to microscopic scales. Such as, the presence of micropores and intracrystalline dissolution pores indicates significant secondary porosity, which is crucial for evaluating reservoirs where primary porosity is limited. These features are often critical in volcanic rocks where primary porosity is limited. The lack of significant pore development and high clay content in tuffite samples limit its natural porosity. However, the presence of fractures could offset these limitations by offering alternative fluid flow pathways.
This approach offers critical insights into the interplay between rock texture, porosity development, and reservoir potential. It enhances the geological and petrophysical characterization of volcanic rock reservoirs, aiding in the development of more effective exploration and extraction strategies. By providing a detailed understanding of reservoir architecture, multi-scale imaging improves predictions of fluid flow behavior and reservoir quality, particularly in complex volcanic formations.
The integration of high-resolution imaging techniques, such as CT scans, MIP, and nanoscale imaging, enables detailed characterization of reservoir pore and throat networks across various scales. Multi-scale models offer a precise depiction of porosity, permeability, and structural integrity, facilitating reliable simulations of fluid dynamics within reservoirs. Understanding fluid movement across interconnected pore networks enables the prediction of hydrocarbon behavior during extraction, essential for optimizing recovery techniques.
Comprehensive models guide well placement, production rates, and enhanced recovery strategies. By identifying high-permeability and high-porosity zones, these models support targeted resource deployment, improving yield potential.
The detailed spatial and quantitative analysis provided by multi-scale models significantly reduces the uncertainties traditionally associated with reservoir evaluations. This improved predictive capability is crucial for both upstream exploration decisions and ongoing reservoir management, helping to minimize financial risks and optimize operational strategies.
Multi-scale models are particularly valuable for evaluating unconventional reservoirs, such as shale and tight gas formations, where pore structures are highly heterogeneous. The models aid in tailoring hydraulic fracturing and other recovery methods to the unique characteristics of each reservoir.
The complex datasets generated by multi-scale models are ideal for machine learning and artificial intelligence applications. These technologies enhance data interpretation, automate trend detection, and refine predictive reservoir behavior models under various operational scenarios.
In conclusion, multi-scale integrated models represent a paradigm shift in reservoir evaluation. By offering a detailed understanding of pore structures and fluid dynamics, these models enhance the efficiency, sustainability, and profitability of hydrocarbon extraction projects. They provide a foundation for informed decision-making, robust resource management, and improved recovery strategies, transforming the approach to exploring and managing volcanic rock reservoirs.
(1) Multi-scale scanning technology enables the detailed three-dimensional characterization of pore distribution across scales, from millimeter-sized samples to full-diameter cores. However, in tight reservoirs, a significant portion of pores, particularly at the nanometer scale, may remain undetected, necessitating complementary methods for a comprehensive analysis.
(2) Among the three analyzed volcanic rock types—rhyolitic volcanic breccia, crystal tuff, and tuffite—the rhyolitic volcanic breccia exhibits the best physical properties and the highest pore connectivity, making it the most favorable reservoir. Crystal tuff ranks second in reservoir quality, while tuffite demonstrates the poorest characteristics, with limited porosity and connectivity.
(3) The integration of multi-scale CT scanning with MIP and nanoscale imaging facilitates the construction of a full-scale digital pore network model. This model provides a visual representation of the morphology and spatial distribution of pores in three dimensions, enabling the quantitative analysis of pore and throat characteristics. Key parameters, including pore count, radius distribution, connectivity, and throat dimensions, are quantitatively evaluated, offering a robust foundation for assessing reservoir effectiveness and guiding exploration and development strategies.
The original contributions presented in the study are included in the article/supplementary material, further inquiries can be directed to the corresponding author.
JZ: Writing–original draft, Writing–review and editing. YL: Writing–review and editing. ZH: Writing–original draft.
The author(s) declare financial support was received for the research, authorship, and/or publication of this article. This study was supported by the Hainan Province Science and Technology Special Fund (ZDYF2023GXJS009) and the SINOPEC Key Science and Technology Project ‘Integrated Technology Research and Application for the Volcanic Gas Reservoirs in the Songnan Fault Depression’ (P21104) and the Open fund of SINOPEC Key Laboratory of Geophysics (33550006-22-FW0399-0017). The authors declare that this study received funding from SINOPEC. The funder was not involved in the study design, collection, analysis, interpretation of data, the writing of this article, or the decision to submit it for publication.
Authors JZ and YL were employed by Sinopec Northeast Oil and Gas Branch.
The remaining author declares that the research was conducted in the absence of any commercial or financial relationships that could be construed as a potential conflict of interest.
All claims expressed in this article are solely those of the authors and do not necessarily represent those of their affiliated organizations, or those of the publisher, the editors and the reviewers. Any product that may be evaluated in this article, or claim that may be made by its manufacturer, is not guaranteed or endorsed by the publisher.
Anovitz, L. M., and Cole, D. R. (2015). Characterization and analysis of porosity and pore structures. Rev. Mineralogy Geochem. 80, 61–164. doi:10.2138/rmg.2015.80.04
Bai, B., Zhu, R. K., Wu, S. T., Yang, W. J., Jeff, G., Allen, G., et al. (2013). Multi-scale method of nano(micro)-CT study on microscopic pore structure of tight sandstone of yanchang formation, ordos basin. Petroleum Explor. Dev. 40 (3), 354–358. doi:10.1016/S1876-3804(13)60042-7
Bera, A., and Shah, S. (2021). A review on modern imaging techniques for characterization of nanoporous unconventional reservoirs: challenges and prospects. Mar. Petroleum Geol. 133, 105287. doi:10.1016/j.marpetgeo.2021.105287
Chalmers, G. R., Bustin, R. M., and Power, I. M. (2012). Characterization of gas shale pore systems by porosimetry, pycnometry, surface area, and field emission scanning electron microscopy/transmission electron microscopy image analyses: examples from the Barnett, Woodford, Haynesville, Marcellus, and Doig units. AAPG Bull. 96, 1099–1119. doi:10.1306/10171111052
Cnudde, V., and Boone, M. N. (2013). High-resolution X-ray computed tomography in geosciences: a review of the current technology and applications. Earth-Science Rev. 123, 1–17. doi:10.1016/j.earscirev.2013.04.003
Cui, L. K., Sun, J. M., Yan, W. C., and Dong, H. M. (2020). Multi-scale and multi-component digital core construction and elastic property simulation. Appl. Geophys. 17 (1), 26–36. doi:10.1007/s11770-019-0789-7
Cui, L. K., Sun, J. M., Yan, W. C., Gao, Y. S., Wang, H. J., and Song, L. Y. (2017). Construction of multi-scale and -component digital cores based on fusion of different resolution core images. J. Jilin Univ. Sci. Ed. 47 (6), 1904–1912. doi:10.13278/j.cnki.jjuese.201706307
Dong, H., and Blunt, M. J. (2009). Pore-network extraction from micro-computerized-tomography images. Phys. Rev. E 80 (3), 036307. doi:10.1103/PhysRevE.80.036307
Dong, H. M., Luo, B., Dang, C. Y., Xu, S., Wang, F., and Chi, P. (2024). Quantitative characterization of the carbonate rock microstructure considering topological features: a case study from the gaoshiti–moxi block of the sichuan basin. Front. Earth Sci. 12 (March), 1375637. doi:10.3389/feart.2024.1375637
Dong, H. M., Sun, J. M., Cui, L. K., Naser, G., and Yan, W. C. (2019). Characteristics of the pore structure of natural gas hydrate reservoir in the qilian mountain permafrost, northwest China. J. Appl. Geophys. 164 (May), 153–159. doi:10.1016/j.jappgeo.2019.03.005
Dong, H. M., Sun, J. M., Naser, G., Cui, L. K., Jiang, L. M., Yan, G. L., et al. (2018). A method to construct high-precision complex pore digital rock. J. Geophys. Eng. 15 (6), 2695–2703. doi:10.1088/1742-2140/aae04e
Jiang, Z., Van Dijke, M. I. J., Sorbie, K. S., and Couples, G. D. (2013). Representation of multiscale heterogeneity via multiscale pore networks: representation of multiscale heterogeneity. Water Resour. Res. 49 (9), 5437–5449. doi:10.1002/wrcr.20304
Jiang, Z., van Dijke, M. I. J., Wu, K., Couples, G. D., Sorbie, K. S., and Ma, J. (2012). Stochastic pore network generation from 3D rock images. Transp. Porous Media 94 (2), 571–593. doi:10.1007/s11242-011-9792-z
Kuila, U. (2013). Measurement and interpretation of porosity and pore-size distribution in mudrocks: the hole story of shales. Colorado School of Mines: Colorado, United States.
Li, R. L., Yang, L. Y., Zhu, J. F., Liu, Y. H., Xu, W., Li, Z. B., et al. (2023). Volcanic reservoir characteristics and hydrocarbon accumulation control factors of rift depressions in southern Songliao Basin. Earth Sci. Front. 30 (4), 100. doi:10.13745/j.esf.sf.2022.10.24
Li, Y. L., Zhang, Y. F., Cong, L., Xie, Z., Yan, M., and Tian, X. X. (2016). Application of X-CT scanning technique in the characterization of micro pore structure of tight sandstone reservoir: an example from fuyu oil layer in daan oilfield. J. Jilin Univ. Sci. Ed. 46 (2), 379–387. doi:10.13278/j.cnki.jjuese.201602107
Liu, B., He, S. L., Wang, H. X., Fu, X., and Gong, L. (2021). Sealing mechanisms in volcanic faulted reservoirs in xujiaweizi extension, northern Songliao Basin, northeastern China. AAPG Bull. 105 (8), 1721–1743. doi:10.1306/03122119048
Liu, B., Yang, Y. Q., Li, J. T., Chi, Y. A., Li, J. H., and Fu, X. F. (2020). Stress sensitivity of tight reservoirs and its effect on oil saturation: a case study of lower cretaceous tight clastic reservoirs in the hailar basin, northeast China. J. Petroleum Sci. Eng. 184 (January), 106484. doi:10.1016/j.petrol.2019.106484
Liu, X. F., Wang, J. F., Ge, L., Hu, F. L., Li, C. L., Li, X., et al. (2017). Pore-scale characterization of tight sandstone in Yanchang Formation Ordos Basin China using micro-CT and SEM imaging from nm-to cm-scale. Fuel 209, 254–264. doi:10.1016/j.fuel.2017.07.068
Liu, X. F., Yan, J. X., Zhang, X. W., Zhang, L. T., Ni, H., Zhou, W., et al. (2021). Numerical upscaling of multi-mineral digital rocks: electrical conductivities of tight sandstones. J. Petroleum Sci. Eng. 201, 108530. doi:10.1016/j.petrol.2021.108530
Ma, L., Fauchille, A. L., Patrick, J. D., Fernando, F. P., Loic, C., Kevin, G. T., et al. (2017). Correlative multi-scale imaging of shales: a review and future perspectives, 454. London: Geological Society, 175–199. Special Publications.
Ma, L., Kevin, G. T., Peter, D. L., Katherine, J. D., Patrick, J. D., and Loic, C. (2016). Novel 3D centimetre-to nano-scale quantification of an organic-rich mudstone: the carboniferous bowland shale, northern england. Mar. Petroleum Geol. 72 (April), 193–205. doi:10.1016/j.marpetgeo.2016.02.008
Ma, W. G., and Liu, A. X. (2011). The study of the pore structure parameters in rocks by CT scanning technology. Sino-Global Energy 16 (7), 54–56.
Mees, F., Swennen, R., Geet, M. V., and Jacobs, P. (2003). Applications of X-ray computed tomography in the geosciences, 215. London: Geological Society, 1–6. Special Publications.
Miao, C. S., Wen, X., Liu, Y. H., and Xie, R. X. (2020). Characteristics of volcanic reservoirs in southern Songliao Basin. J. Jilin Univ. Sci. Ed. 50 (2), 635–643. doi:10.13278/j.cnki.jjuese.20190253
Njiekak, G., Schmitt, D. R., and Kofman, R. S. (2018). Pore systems in carbonate formations, Weyburn field, Saskatchewan, Canada: micro-tomography, helium porosimetry and mercury intrusion porosimetry characterization. J. Petroleum Sci. Eng. 171, 1496–1513. doi:10.1016/j.petrol.2018.08.029
Otsu, N. (1979). A threshold selection method from gray-level histograms. IEEE Transactions on Systems, Man, and Cybernetics 1 (9), 62–66. doi:10.1109/TSMC.1979.4310076
Qajar, J., and Arns, C. H. (2022). A comparative study of micro-CT and mercury intrusion techniques for predicting permeability and surface area evolution during chemical dissolution. Adv. Water Resour. 168, 104301. doi:10.1016/j.advwatres.2022.104301
Qu, X. Y., Miao, C. S., Li, R. L., Zhu, J. F., Xu, W., and Liu, Y. N. (2022). Influencing factors of tight clastic reservoir physical properties and main controlling factors of high-quality reservoirs:taking the Yingcheng Formation of longfengshan sub-sag in changling fault depression of Songliao Basin as an example. Nat. Gas. Geosci. 33 (7), 1036–1048. doi:10.11764/j.issn.1672-1926.2022.02.005
Remeysen, K., and Swennen, R. (2008). Application of microfocus computed tomography in carbonate reservoir characterization: possibilities and limitations. Mar. Petroleum Geol. 25, 486–499. doi:10.1016/j.marpetgeo.2007.07.008
Wang, P. J., Chen, C. Y., Zhang, Y., Gao, Y. F., Qu, X. J., and Yi, J. (2015). Characteristics of volcanic reservoirs and distribution rules of effective reservoirs in the changling fault depression, Songliao Basin. Nat. Gas. Ind. B 2 (5), 440–448. doi:10.1016/j.ngib.2015.09.020
Zheng, J., Wang, P. J., Zhu, J. F., Bian, W. H., Hong, Y. M., Cheng, Z. Y., et al. (2021). Characteristics and main controlling factors of pyroclastic reservoirs: a case study in longfengshan area, changling fault depression, southern Songliao Basin. World Geol. 40, 399–407.
Keywords: volcanic rock reservoir, multi-scale CT imaging, microscopic characterization, pore network model, oil and gas exploration
Citation: Zhu J, Liu Y and Hu Z (2025) Multi-scale CT imaging technique characterization of volcanic rock reservoirs: a study case from the Changling fault depression of the southern Songliao Basin. Front. Earth Sci. 13:1456257. doi: 10.3389/feart.2025.1456257
Received: 28 June 2024; Accepted: 06 March 2025;
Published: 03 April 2025.
Edited by:
Yihuai Zhang, University of Glasgow, United KingdomReviewed by:
Lin Ma, The University of Manchester, United KingdomCopyright © 2025 Zhu, Liu and Hu. This is an open-access article distributed under the terms of the Creative Commons Attribution License (CC BY). The use, distribution or reproduction in other forums is permitted, provided the original author(s) and the copyright owner(s) are credited and that the original publication in this journal is cited, in accordance with accepted academic practice. No use, distribution or reproduction is permitted which does not comply with these terms.
*Correspondence: Jianfeng Zhu, emh1amYuZGJzakBzaW5vcGVjLmNvbQ==
Disclaimer: All claims expressed in this article are solely those of the authors and do not necessarily represent those of their affiliated organizations, or those of the publisher, the editors and the reviewers. Any product that may be evaluated in this article or claim that may be made by its manufacturer is not guaranteed or endorsed by the publisher.
Research integrity at Frontiers
Learn more about the work of our research integrity team to safeguard the quality of each article we publish.