- 1Department of Biogeochemical Processes, Max Planck Institute for Biogeochemistry, Jena, Germany
- 2Hydrogeology, Institute of Geosciences, Friedrich Schiller University, Jena, Germany
- 3Aquatic Geomicrobiology, Institute of Biodiversity, Friedrich Schiller University, Jena, Germany
- 4German Center for Integrative Biodiversity Research (iDiv) Halle-Jena-Leipzig, Leipzig, Germany
- 5Cluster of Excellence Balance of the Microverse, Friedrich Schiller University Jena, Jena, Germany
Snowfall/cover and snowmelt are essential determinants of winter soil processes/events that may force the biogeochemical dynamics of soils in temperate regions. Increasing variability of the European hydroclimate is expected to lead to more frequent intermittent warm periods, which cause snowmelt during winter and rapidly mobilize large amounts of dissolved organic matter (DOM). This study, conducted at the Hainich Critical Zone Exploratory in Germany, seeks to address a significant gap in understanding the molecular impacts of snowmelt-induced DOM flows and their ability to alter soil ecosystems rapidly. During two snowmelt events between January and March 2021, we observed that DOM concentration and composition varied more in forest soil seepage than in grassland soil seepage. Forest seepage showed a pronounced DOM flux peak and synchronous increases in the relative abundances of aromatic DOM components, indicating surface-derived transport of plant litter carbon. In the grassland, however, peak DOM fluxes were characterized by a marked increase in nitrogen-containing (N-containing) DOM components, indicating a predominance of microbial carbon. Notably, the unique DOM components specific to each ecosystem increased during peak fluxes in the forest but decreased in the grassland. We suggest that an overall higher molecular richness and the broader functional metabolic potentials in grassland may account for its relatively greater DOM stability compared to the forest during peak snowmelt events.
1 Introduction
Snowfall, cover, and snowmelt during winter play crucial roles in shaping soil biogeochemistry and microbial activity (Brooks et al., 2011). Europe’s hydroclimate is projected to become more variable and snow cover is expected to become more irregular as a result (Räisänen, 2016). The impact of more frequent snow melting during warmer winter periods on soil biogeochemistry remains unclear.
Snow cover increases decomposition rates of plant litter compared to uncovered soil, providing large amounts of bioavailable carbon and nutrients to soil microbial communities and shaping the environmental conditions for plant growth during spring (Groffman et al., 2001; Sebestyen et al., 2008; Kaiser and Kalbitz, 2012; Burns et al., 2016; Chen et al., 2021). A simulated snowmelt treatment documented litter layer carbon losses of up to 36.4%, contributing more carbon to soil than roots (Uselman et al., 2007). Litter layer mass loss during winter accounted for 26% of the annual mass loss in a temperate broad-leaf forest in Japan (Uchida et al., 2005). In addition to surface-derived plant carbon, snowmelt can mobilize soil organic matter, contributing up to half of the carbon in water-extractable organic matter during snowmelt (Nakanishi et al., 2014). Since soil microbial communities can utilize available carbon to grow under snow even at temperatures below zero, snowmelt is considered a critical condition for soil microbial activity (Dibbern et al., 2014; Kuzyakov and Blagodatskaya, 2015; Gavazov et al., 2017; Isobe et al., 2018; Sorensen et al., 2020). Increased carbon availability by increased water flow during snowmelt provides favorable conditions for microbial metabolic processes, thus commonly leading to increases in microbial biomass (Rindt et al., 2023). Microbial metabolic processes, in turn, influence the concentration and composition of dissolved organic matter (DOM) and enhance the turnover of nitrogen, including mineralization and nitrification processes, as well as the biosynthesis of organic N-containing substances (Campbell and Laudon, 2019).
DOM is a crucial medium for investigating the impacts of short-term fluxes such as snowmelt on soil ecosystems, as it is the mobile and bioavailable carbon pool in soil (Roth et al., 2019). While previous studies have mainly focused on sum parameters or optical properties of DOM (e.g., Rindt et al., 2023; Meingast et al., 2023), we employed ultrahigh-resolution mass spectrometry (HR-MS), a proven state-of-the-art technique to reveal the relative abundances of thousands of molecular entities within the DOM, spanning from plant-derived compounds such as aromatic molecules or lignin phenols to microbial-derived substances such as peptides or proteins (Benk et al., 2019; Schroeter et al., 2022). In this study, we investigated two snowmelt events between January and March 2021 in forest and grassland sites within the Hainich Critical Zone Exploratory (CZE) located in central Germany (Küsel et al., 2016; Kohlhepp et al., 2017). Tension-controlled lysimeters (Lehmann et al., 2021) installed at the topsoil-subsoil-boundary (∼30 cm soil depth) continuously collected soil seepage during snowmelt. Samples were collected from the lysimeters within ∼6 h during high-volume flux periods. High temporal resolution sampling allowed us to reveal previously unseen short-term dynamics of DOM during snowmelt on a molecular level. Forest and grassland are two major ecosystems in Europe (Frost Europe, 2020; Pazúr et al., 2024), and both provide a range of valuable ecosystem services. However, due to the inherent differences in carbon and nutrients between frost and grassland soil ecosystems, these two ecosystems are expected to respond differently to short-term flux changes. Therefore, we chose these two ecosystems to study the DOM changes. As a previous study described a locally more significant contribution of bacterial carbon to total organic carbon in grassland (Herrmann et al., 2023), we hypothesized that the difference in carbon input and subsequent response may be reflected at the molecular level of DOM during snowmelt, as bacterial carbon may shape the DOM dynamics of grassland seepage, whereas forest seepage DOM is expected to mainly reflect contributions from litter layer leaching. The differing carbon inputs resulting from the diverse characteristics of different ecosystems may give rise to distinct short-term soil carbon dynamics. This is a crucial element that must be considered in order to gain a deeper understanding of the soil biogeochemical processes.
2 Materials and methods
2.1 Study site and seepage sampling
Two sets of soil seepage samples were collected during two snowmelt events in 2021 at two different sampling sites (managed deciduous forest and grassland) at the Hainich Critical Zone Exploratory in central Germany (51°04′46″N, 10°27′08″E). Both sampling sites are located on the Hainich low mountain hilltop (Reckenbühl site, Lehmann et al., 2021) with minimal slope impact. The climate in this region is characterized by an average annual air temperature of 7.5°C–8°C, with precipitation fluctuating between 900 and 1,000 mm, mainly during the winter months (Küsel et al., 2016).
High-resolution sampling of snowmelt events was carried out in 2021 from January 19th to 25th and February 15th to March 3rd at a managed deciduous forest site and at a grassland site. Sampling was initiated when the temperature was about to rise above 0°C following the nearest local snowfall. Sampling at the grassland in January 2021 started after the temperature had risen above 0°C, and sampling at the forest in February 2021 started 1 day before the temperature reached 0°C. Grassland sampling lasted for 7 days. Forest sampling lasted for 16 days.
The soil seepage samples were collected using tension-controlled lysimeters (Lehmann et al., 2021) installed at approximately 30 cm depth at both the grassland site and the forest site, representing the topsoil-subsoil boundary. Soils are rendzic leptosols developed from loess that overly the chromic cambisols developed from the carbonate bedrock (Lehmann et al., 2021). At both locations, two lysimeters were installed for the purpose of serving as replicates. However, due to the soil heterogeneity, the results obtained from the two replicate lysimeters did not fully exhibit the anticipated statistical similarity (Mann-Whitney U test p < 0.05 for Dissolved organic carbon (DOC) concentration between two lysimeters in grassland). Nevertheless, the principal components analysis (PCA) based on the molecular composition of samples shows a big difference between forest and grassland (see Supplementary Figure S1). The differences between lysimeters were also minimal, exhibiting a comparable pattern within the ecosystem. All lysimeters were well-maintained and used for regular fortnightly soil seepage monitoring (Lehmann et al., 2021). The sampling frequency was elevated to every 6 h during high flux periods. Collected samples were promptly transported to the lab and stored at −20°C until analysis. A total of 16 soil seepage subsamples were taken from the grassland site and ten subsamples from the forest site for comprehensive DOM analysis on a molecular level.
2.2 DOC concentration
Subsamples underwent filtration using regenerated cellulose membrane filters with a pore size of 0.45 μm (Chromafil Xtra RC-45/25, MACHEREY-NAGEL, Germany), filters were rinsed three times with Milli-Q water before use to remove any contaminations. After acidifying the samples with hydrochloric acid (32%, Merck KGaA), DOC concentration was measured using a total organic carbon analyzer (vario TOC cube, Elementar, Germany) (Tanunchai et al., 2023).
2.3 DOM extraction and mass spectrometric analysis
Solid phase extraction of DOM was performed using PPL (styrene-divinylbenzene polymer) cartridges (Agilent Technologies, Germany) following the procedure by Dittmar et al. (2008). Two operational blanks using pH 2 acidified ultrapure water were included with sample extraction. For HR-MS measurements, samples were adjusted to a DOC concentration of 20 mg/L in 50% methanol (HiPerSolv CHROMANORM, VWR, Germany) and 50% ultrapure water (Milli-Q IQ 7000, Merck). An Orbitrap Elite (Thermo Fisher Scientific) was used for HR-MS analysis (Benk et al., 2019; Orme et al., 2022). 100 μL of each sample were directly injected into the MS via autosampler along with 20 μL/min continuous flow (H2O: MeOH = 1:1) and then ionized in negative mode at an electrospray ionization (ESI) needle voltage of 3 kV. Averaged mass spectra of 100 scans with m/z ranging from 100 to 1,000 Da were exported from Thermo Xcalibur (Version 3.0.63). A signal-to-noise ratio >5 cutoff was employed before further processing.
2.4 Data analysis
Data analysis and visualization were performed using R (version 4.2.3) with the following packages: vegan, stats, ggplot2, ggpubr, dplyr. The open-source software tool ThermoRawFileParser (Hulstaert et al., 2020) was used to convert Thermo RAW files to mzML format. Internal calibration and molecular formula assignment were performed in R using the MFAssignR 1.0.2 package (Schum et al., 2020). Mass peaks from the procedural blanks were sorted by decreasing intensity, and all large peaks were deleted from the data set until 95% of the total intensity of the blanks was reached. By doing so, the main contaminations from sample processing were expected to be removed. The elements considered for molecular formula assignment included C, H, O, N, P, and S, with detailed settings as follows: C ≤ 60; H ≤ 120; O ≤ 30; N ≤ 4; S ≤ 2; P ≤ 1. This resulted in 7,614 formulae being assigned from 26 samples. Normalization to the sum of all formula intensities per sample was employed to account for the known effects of competitive ionization. Molecular classes were assigned as follows: aromatic substances with aromaticity index values between 0.5 and 0.66 (Koch and Dittmar, 2006; Koch and Dittmar, 2016) and aliphatic substances with H/C ≥ 1.5 (Waggoner and Hatcher, 2017). Functional metabolic potentials of DOM were evaluated by matching the annotated molecular formulae with the KEGG database (Kanehisa and Goto, 2000) via the KEGGREST package (Tenenbaum, 2017). Specifically, we focused on metabolic pathways annotated in the KEGG database that reflect “Metabolism” or “Environmental Information Processing”. Within the group of “Metabolism”, we considered only those pathways “active” that had at least ten distinct molecular formula matches and a coverage of at least 25% of the total length of the pathway. To compare the relative abundances of the metabolic pathways, i.e., “degradation of aromatic compounds” and “biosynthesis of various antibiotics”, all relative intensities of matching molecular formulae were summed, allowing each molecular formula to contribute its relative abundance only once per pathway in the case of multiple matches due to isomers. The relationship between DOC flux and the count of unique formulae was examined through Spearman’s rank correlation analysis. Furthermore, the Wilcoxon rank-sum test was utilized to determine the statistical significance of variations in the number of potential metabolic pathways and formulae counts across two ecosystems.
3 Results
3.1 Variation of DOC concentration during the snowmelt event
DOC concentrations in soil seepage at 30 cm soil depth varied between 3.11 mg/L and 9.78 mg/L at the forest site and between 5.55 mg/L and 10.97 mg/L at the grassland site (Figure 1). The first samples at temperatures just above or approaching 0°C were in tiny volumes (hourly water flux less than 10 mL/h) with DOC concentrations up to 10.97 mg/L, which decreased at the next two time points in both ecosystems and was followed by strong increases in forest and lesser increases in grassland. Over the same period, DOC fluxes increased, driven mainly by increasing water fluxes and only minor influences by the temporarily decreased DOC concentration. Peak DOC concentrations and DOC fluxes were reached after 8 days in the forest and after 3 days in the grassland. After peak DOC fluxes were reached, both DOC fluxes and concentrations gradually decreased.
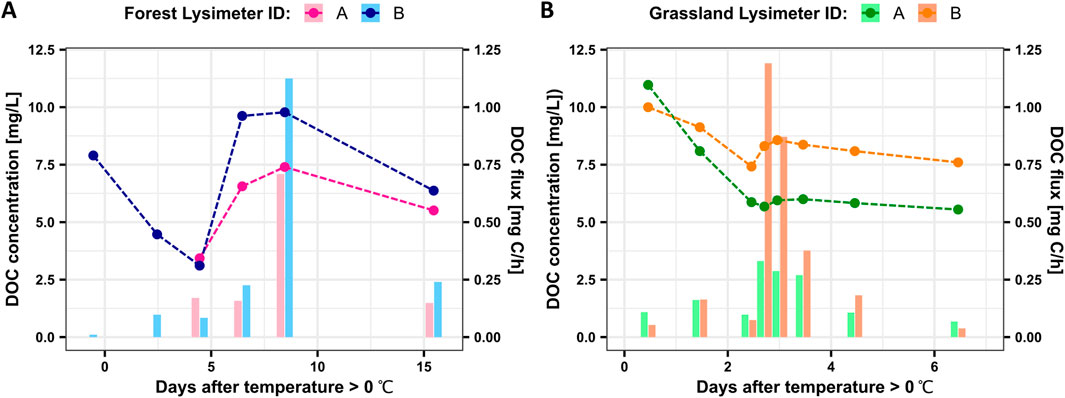
Figure 1. DOC concentration (dashed line charts) in soil seepage and hourly DOC fluxes (bar charts) at 30 cm soil depth over two snowmelt events at the Hainich Critical Zone Exploratory in Germany. (A) Forest site (sampled from 15th February to 3rd March 2021); (B) Grassland site (sampled from 19th January to 25th January 2021).
For discussion purposes, we divided the entire series into three phases based on the observed concentration changes: the initial flux period spans from the onset of snowmelt (when the temperatures rose above 0°C) until the DOC concentrations reached their minimum; the peak flux period includes the rise in DOC concentrations from the minimum to the maximum DOC concentration; and the late flux period is defined as the declining phase after the maximum DOC concentrations were reached.
Soil seepage DOC concentrations in the forest were more variable than in grassland. The average deviations from the mean were 1.84 mg/L for forest and 1.35 mg/L for grassland. During the peak flux period, forest DOC concentrations showed a more than 2-fold increase, whereas DOC concentrations in grassland rose only by ∼15%.
At the grassland site, peak DOC fluxes were reached 3 days after temperatures rose above 0°C (Figure 1B). In forest it took 8 days until peak fluxes were reached (Figure 1A). Interestingly, peak fluxes occurred before the DOC concentration peak in grassland. In contrast, forest DOC concentrations peaked earlier than fluxes. DOC fluxes ranged between ∼0.03 and 1.2 mg C/h in forest and grassland (Figure 1). The replicate lysimeters recorded marked flux differences, though, with peak fluxes reaching 0.7 or 1.1 mg C/h in forest and 0.3 or 1.2 mg C/h in grassland, pointing to important effects of local soil heterogeneity. Generally, higher peak fluxes were higher in DOC concentration as well. During the late flux period, forest showed higher fluxes than grassland, even though the forest site was sampled for 15 days after the initial snowmelt, compared to only 6 days of sampling in grassland.
3.2 Changes in the molecular composition of DOM
In forest, the relative abundance of aliphatic DOM compounds increased from 8.2% to 11.4% during the initial flux period and decreased to 8.9% during the peak flux period (Figure 2). Simultaneously, the relative abundance of aromatic and N-containing DOM compounds displayed trends opposite to those of aliphatic DOM compounds. Relative abundances of aromatics decreased from 10.8% to 7.5% during the initial flux period and sharply increased to 13.4% during peak DOC fluxes. N-containing DOM compounds initially decreased in their relative abundance from 12.8% to 9.4 and then increased to 14.7% during the peak flux period. In the late flux period, the relative abundances of all three DOM compound classes decreased. The last recorded composition in forest was 8.2% aliphatics, 9.8% aromatics and 11.6% N-containing DOM. Forest lysimeter A lacked sufficient water volumes for complete analysis during the initial flux period but showed similar distribution patterns during the peak and late flux periods.
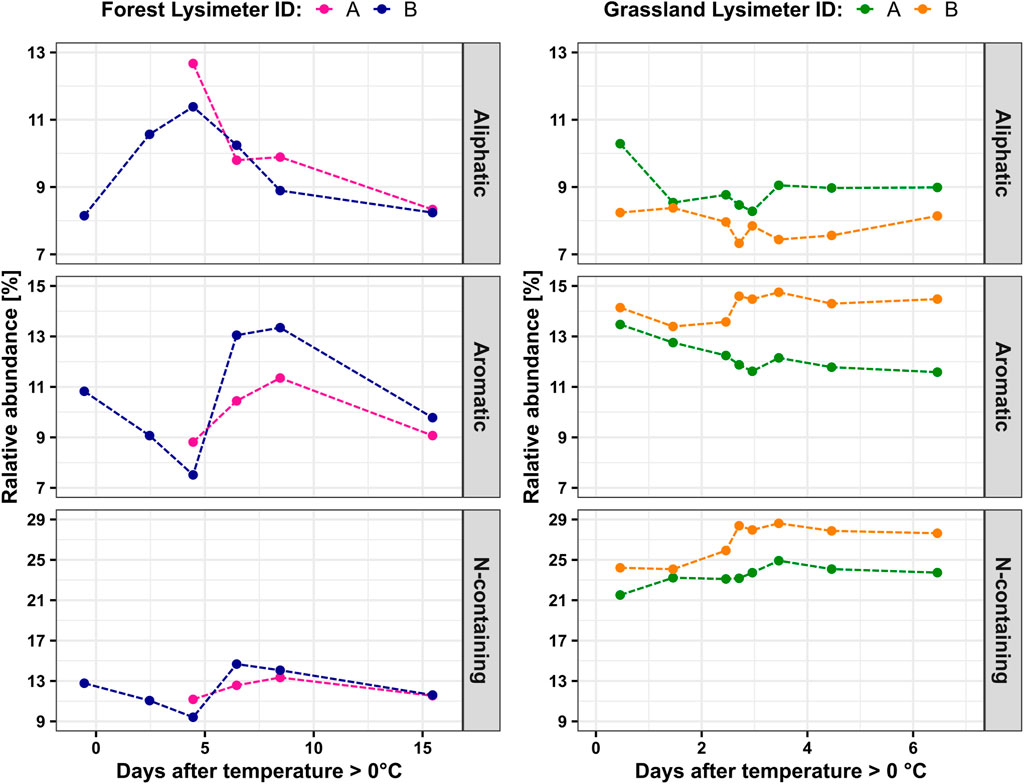
Figure 2. Changes in the relative abundance of aliphatic, aromatic, and N-containing DOM components in two ecosystems.
In grassland, relative abundances of aliphatic and aromatic DOM compounds did not show any distinct patterns during the initial and peak flux periods, varying by less than 2.5% in each lysimeter. N-containing DOM compounds increased in relative abundance during the initial and peak flux periods. During the late flux period, N-containing DOM compounds remained at elevated relative abundance levels with only minimal decreases towards the end of the sampling period. Notably, N-containing DOM compounds were approximately twice as abundant in grassland compared to forest, with mean relative abundances of 25% and 12% in grassland and forest, respectively. Mean relative abundances of aromatics and aliphatics were markedly more similar between forest and grassland, differing by ∼2 percentage points.
3.3 Compositional differences in the molecular richness and uniqueness
There were 6,309 and 7,223 molecular formulae assigned to both lysimeters in forest (lysimeter A + B) and both lysimeters in grassland (lysimeter A + B) DOM samples, respectively (Figure 3A). 5,919 formulae (77.7%) were shared between forest and grassland (Figure 3B). Here, we refer to non-shared formulae as unique formulae. Forest DOM contained fewer unique formulae (390) than grassland DOM (1,305) (Figure 3A). Molecular formulae uniquely detected in grassland were predominantly composed of N-containing substances (Figure 3C). Most showed element ratios of H/C < 1.5 and O/C < 0.5. Conversely, molecular formulae unique to the forest had more compounds containing no nitrogen (Figure 3C). Their elemental ratios were mostly either H/C > 1.5 or O/C > 0.5. The number of unique molecular formulae detected in forest and grassland was significantly correlated to DOC fluxes (Spearman correlation, p < 0.05) (Figure 3D). At the forest site, this correlation was positive; at the grassland site, it was negative.
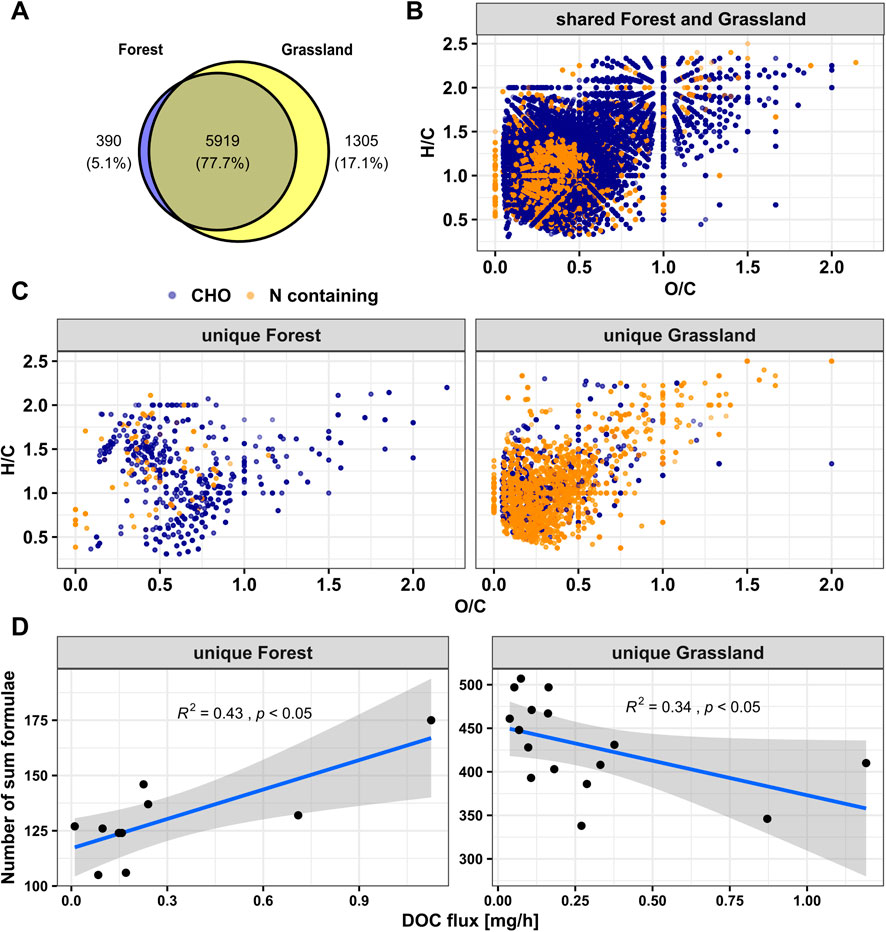
Figure 3. The molecular richness and unique formulae in two ecosystems during snowmelt; (A) Venn diagram illustrating the number of molecular formulae in two ecosystems; (B) Van Krevelen diagram displaying molecular formulae present in both ecosystems, with different colors signifying various chemical classes: blue dots representing CHO- formulae, and orange dots denoting those formulae that containing nitrogen; (C) VK diagram demonstrating molecular formulae unique to the forest and grassland; (D) number of unique formulae with hourly DOC flux for the forest and grassland ecosystem; spearman’s rank correlation analysis are used to do the correlation for figures in panel (D).
3.4 Changes in the potential metabolic pathways
Out of 7,614 total molecular formulae detected in our dataset, 11.2% (849) molecular formulae could be assigned to metabolic pathways in KEGG within the groups “Metabolism” and “Environmental information processing”. The relative abundances of the metabolic pathway degradation of aromatic compounds were around 3% in both forest and grassland. Their changes with time closely followed the patterns described above for aromatic DOM, albeit at lower relative abundance levels (Figures 2, 4A). Relative abundances of the pathway biosynthesis of antibiotics were lower in forest than in grassland. Their changes in forest were highly similar between lysimeter replicates, reaching a maximum during the onset of the peak DOC flux period. Grassland DOM showed distinct differences between both lysimeters with lysimeter A showing no effect and lysimeter B revealing a sharp increase and decline of potential antibiotics biosynthesis around the peak flux period. The number of distinct potential metabolic pathways that were detected within the group “Metabolism” was significantly greater in grassland than in forest, with a median of 42 in grassland and 36 in forest (p < 0.01, Figure 4B). Grassland DOM also contained a significantly greater number of molecular formulae annotated to “Environmental information processing” in KEGG (p<0.01, Figure 4C).
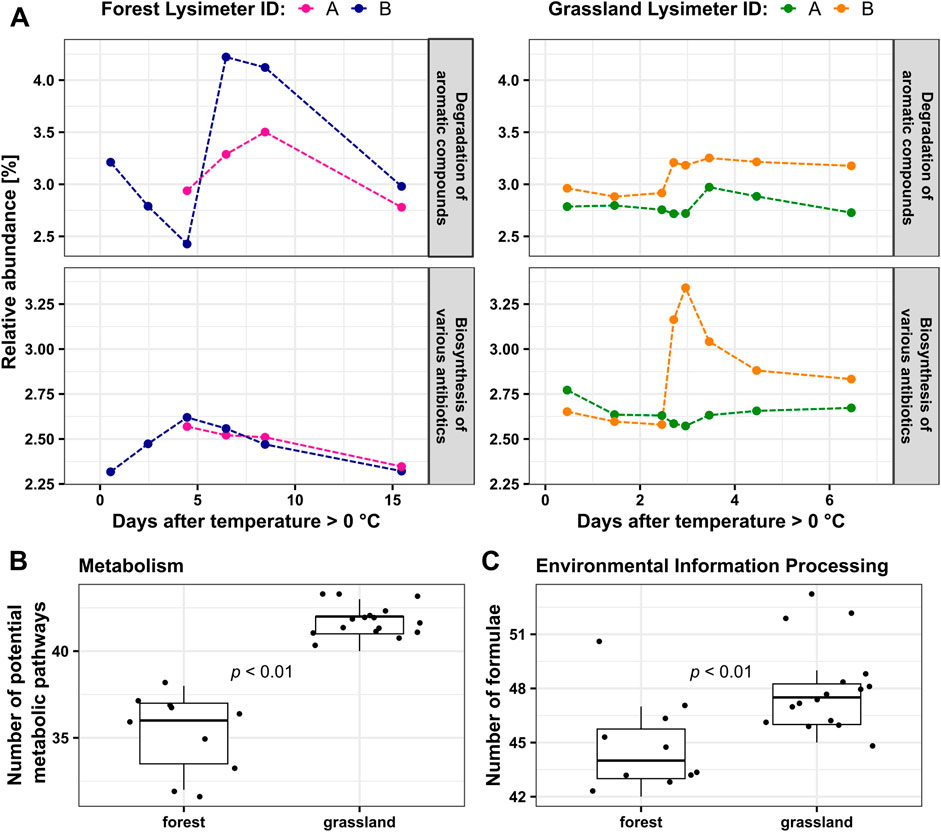
Figure 4. (A) Summed relative abundances of all formulae annotated in KEGG to “Degradation of aromatic compounds” and “Biosynthesis of various antibiotics” pathways (B) Number of potential metabolic pathways detected within the group “Metabolism” in KEGG; (C) Number of molecular formulae detected within the group “Environmental information processing” in KEGG. Wilcoxon rank-sum tests are used to do the significant test in (B, C).
4 Discussion
During the initial flux period, the DOC concentration of the collected samples decreased, suggesting that the initial water fluxes carried less organic matter than earlier soil seepage under snow cover (Figure 1). This reduction in DOC concentration can be attributed to a combination of factors. Firstly, this suggests that the contribution of low-DOC snowmelt water (<0.5 mg/L) increased, thereby diluting the overall DOC concentration of soil solution, as previously observed (Lehmann et al., 2021). Secondly, prior research also showed high microbial biomass and activity under snow cover, leading to potential carbon limitation (Gavazov et al., 2017; Isobe et al., 2018). Supporting evidence for substantial microbial degradation of carbon includes the observed decrease in the relative abundances of aromatic compounds and the increase in the relative abundances of aliphatic DOM in the forest (Figure 2), which is also in line with previous results (Khatami et al., 2019). Thirdly, the percolation of snowmelt water through the soil can lead to partial retention and reaction of DOM on mineral surfaces. Previous column experiments demonstrated that a considerable fraction of DOM exhibits reactive flow behavior in soil (Weigand and Totsche, 1998; Kalbitz et al., 2005; Kaiser and Kalbitz, 2012). This adsorption effect likely further reduced DOC concentrations during the initial flux period. Considering that forest and grassland showed similar decreases in DOC concentrations of ∼50% during the initial flux period, we concluded that initial dilution effects did not significantly differ between both ecosystems.
Most DOC transport occurred during the peak flux period, with up to 65% of the total sampled DOC in forest and 43% in grassland. While grassland and forest showed similar peak DOC fluxes of ∼1.1 mg C/h (Figure 1), their molecular composition was highly different. In forest, a synchronous peak of DOC concentration (Figure 1) and the proportion of aromatic DOM (Figure 2) most likely reflected the transport of litter layer-derived carbon (Roth et al., 2019). Grassland DOM did not show a pronounced peak in aromatic compounds (Figure 2), and changes in DOC concentration were considerably more minor than in forest (Figure 1). This difference is most likely driven by a thick forest litter layer rich in lignin, considering major litter layer mass losses and DOC leaching reported in forests during winter (Uchida et al., 2005; Uselman et al., 2007; Chen et al., 2017; Hensgens et al., 2020). Peak fluxes in grassland were instead characterized by increased proportions of N-containing compounds (Figure 2). Given that the majority of soil organic nitrogen is proteinaceous material and N-heterocycles of microbial origin, our findings suggest a stronger microbial control on soil DOM dynamics in grassland than in forest (Schulten and Schnitzer, 1997). Higher decomposition rates of both grassland litter and roots potentially led to a substantial accumulation of microbially processed carbon under snow cover compared to forest (John et al., 2011; Solly et al., 2014). The mobilization of microbially processed carbon during peak DOC fluxes likely stimulated soil microbial communities in grassland more strongly than aromatic litter layer-leaching in forest (Zhou et al., 2021). An alternative explanation of the observed increases in N-containing DOM during the peak flux period includes the transport of microbial necromass from the soil surface below the snow cover. Previous investigations highlighted rapid collapses of microbial communities upon snow melting, releasing large amounts of nitrogen (Lipson and Schmidt, 2004; Christopher et al., 2008; Isobe et al., 2018; Sorensen et al., 2020). In forest, the relative abundance changes of N-containing DOM were highly similar to those of aromatic DOM (Figure 2), rising during peak DOC fluxes and decreasing in the late DOC flux phase. This suggests the co-transport of microbial necromass and microbial-related carbon derived from the degradation of the plant products from the litter layer. In grassland, however, relative abundances of N-containing DOM remained high throughout the late flux period (Figure 2), indicating longer-term nitrogen cycling and retention compared to forest. A previous investigation of the Hainich CZE found greater mobility of bacterial cells in grassland than in forest soils, especially during winter (Herrmann et al., 2023). We speculate that differences in cellular- and necromass-derived substances in seepage can be used to describe the variation of the observed patterns of N-containing DOM in grassland and forest. Necromass-derived substances transported during peak DOC fluxes in forest were likely rapidly decomposed. The declining microbial processing of aromatic substances may have led to the decline in N-containing DOM during the late flux period. Enhanced transport of cells and derived substances in grassland could have resulted in higher persistence of N-containing DOM post-peak DOC fluxes.
We performed a prediction of potential metabolic pathways to further elucidate the role of microbially-sourced DOM based on the KEGG database. The similarity of the relative patterns between aromatic DOM (Figure 2) and the predicted “degradation of aromatic compounds” (Figure 4) indicates that the molecular formulae that matched with KEGG are a representative subset of bulk DOM. Our findings reflect the considerable role of soil bacteria in lignin degradation (Phale et al., 2007; Bugg et al., 2011; Brown and Chang, 2014). The metabolic potentials for the “biosynthesis of various antibiotics” provide an exciting perspective on short-term microbial responses to snowmelt (Figure 4A). The predicted compounds were likely sourced from bacterial-bacterial and fungal-bacterial antagonism (Bahram et al., 2018). Forest DOM revealed minor increases in the biosynthesis of antibiotics during the onset of the peak flux period (Figure 4A), followed by gradual decreases, indicating slow responses in competitive microbial community actions. The spike in relative abundance in grassland lysimeter B indicates rapid competitive responses to snowmelt among soil microbial communities in grassland (Sorensen et al., 2020; Broadbent et al., 2021). With no effect recorded in grassland lysimeter A, we speculate that local soil heterogeneity and preferential flow majorly contributed to the observed spike. This is supported by lysimeter B recording threefold higher DOC fluxes than lysimeter A (Figure 1). Our findings suggest that soil microbial communities were sensitive to snowmelt DOC fluxes, with a likely faster and greater potential for response in grassland than in forest. A significantly greater number of potential metabolic pathways annotated within the group “Metabolism” in grassland than in forest (Figure 4B) indicates that the observed greater molecular richness in grassland (Figure 3A) translates to more diverse metabolic potentials. The same is likely true for the number of formulae potentially involved in “Environmental Information Processing”, which was also significantly higher in grassland than forest (Figure 4C). More diverse metabolic potentials of microbial communities in grassland compared to forest are a likely reason for their greater resistance to the disturbance introduced by snowmelt and their ability to adapt rapidly (Wu et al., 2020; Huang et al., 2021).
Most unique molecular formulas in grassland were N-containing (Figure 3C), and their number correlated negatively with DOC fluxes (Figure 3D). This indicates that snowmelt transported typical topsoil- and litter-layer-derived compounds, likely triggering rapid bacterial community adaptation to facilitate decomposition. We speculate that more significant contributions of aromatic litter layer leachates in forest drove the observed greater variability in DOC fluxes and DOM composition. Primary decomposition of lignin in forest is majorly facilitated by fungi, which have been shown to grow and adapt more slowly to changes in substrate (Isobe et al., 2018; Yu et al., 2022). Fungal pre-processing might explain the lower molecular richness of DOM in forest and the comparably lesser capability of forest soil bacterial communities to respond and adapt to snowmelt DOC fluxes. Taken together, the lower variability of DOC fluxes and DOM composition in grassland paired with greater proportions of N-containing substances, greater molecular richness, and more pronounced responses in bacterial metabolism suggest that grassland soil microbial communities majorly contributed to stabilizing grassland soil seepage against changeover by snowmelt fluxes.
5 Conclusion
Our case study from the Hainich CZE emphasizes the potential of high-resolution DOM sampling combined with molecular composition analyses to provide novel insights into short-term soil biogeochemical processes. Snowmelt rapidly reshapes soil seepage DOM composition and surface carbon sources, preferencial flow, and the metabolic potential of local microbial communities together controlled the molecular dynamic of DOM in soil seepage. The lesser variability of grassland DOM compared to forest highlights the need for detailed investigations of the metabolic potentials of soil microbial communities to stabilize against short-term environmental forcings. The diversity of community-level metabolic functions and capabilities for environmental information processing seemed likely candidates for supporting DOM stability. In conclusion, our results highlight the importance of considering ecosystem-specific characteristics when assessing the impacts of climate variability on soil biogeochemical processes and understanding these processes becomes crucial for predicting and managing the implications for soil health and ecosystem services in temperate regions.
Data availability statement
The raw data supporting the conclusions of this article will be made available by the authors, without undue reservation.
Author contributions
CH: Formal Analysis, Investigation, Methodology, Writing–original draft. SS: Formal Analysis, Methodology, Supervision, Writing–review and editing. KL: Conceptualization, Investigation, Writing–review and editing. MH: Writing–review and editing. KT: Conceptualization, Writing–review and editing. GG: Conceptualization, Formal Analysis, Supervision, Writing–review and editing.
Funding
The author(s) declare that financial support was received for the research, authorship, and/or publication of this article. This study is part of the Collaborative Research Centre AquaDiva of the Friedrich Schiller University Jena, funded by the Deutsche Forschungsgemeinschaft (DFG, German Research Foundation)—SFB 1076—Project Number 218627073. CH would like to thank the China Scholarship Council (CSC) for the stipend (No. 202204910086).
Acknowledgments
We thank the routine measurement and analysis group of I. Hilke for measurement contributions. We thank the AquaDiva scientific coordinators. CH would like to thank the support from the International Max Planck Research School for Global Biogeochemical Cycles (IMPRS-gBGC), and Integrated Research Training Group (IRTG) AquaDiva.
Conflict of interest
The authors declare that the research was conducted in the absence of any commercial or financial relationships that could be construed as a potential conflict of interest.
Publisher’s note
All claims expressed in this article are solely those of the authors and do not necessarily represent those of their affiliated organizations, or those of the publisher, the editors and the reviewers. Any product that may be evaluated in this article, or claim that may be made by its manufacturer, is not guaranteed or endorsed by the publisher.
Supplementary material
The Supplementary Material for this article can be found online at: https://www.frontiersin.org/articles/10.3389/feart.2024.1458322/full#supplementary-material
References
Bahram, M., Hildebrand, F., Forslund, S. K., Anderson, J. L., Soudzilovskaia, N. A., Bodegom, P. M., et al. (2018). Structure and function of the global topsoil microbiome. Nature 560, 233–237. doi:10.1038/s41586-018-0386-6
Benk, S. A., Yan, L., Lehmann, R., Roth, V.-N., Schwab, V. F., Totsche, K. U., et al. (2019). Fueling diversity in the subsurface: composition and age of dissolved organic matter in the critical Zone. Front. Earth Sci. 7, 296. doi:10.3389/feart.2019.00296
Broadbent, A. A. D., Snell, H. S. K., Michas, A., Pritchard, W. J., Newbold, L., Cordero, I., et al. (2021). Climate change alters temporal dynamics of alpine soil microbial functioning and biogeochemical cycling via earlier snowmelt. ISME J. 15, 2264–2275. doi:10.1038/s41396-021-00922-0
Brooks, P. D., Grogan, P., Templer, P. H., Groffman, P., Öquist, M. G., and Schimel, J. (2011). Carbon and nitrogen cycling in snow-covered environments. Geogr. Compass 5, 682–699. doi:10.1111/j.1749-8198.2011.00420.x
Brown, M. E., and Chang, M. C. (2014). Exploring bacterial lignin degradation. Curr. Opin. Chem. Biol. 19, 1–7. doi:10.1016/j.cbpa.2013.11.015
Bugg, T. D., Ahmad, M., Hardiman, E. M., and Singh, R. (2011). The emerging role for bacteria in lignin degradation and bio-product formation. Curr. Opin. Biotechnol. 22, 394–400. doi:10.1016/j.copbio.2010.10.009
Burns, M. A., Barnard, H. R., Gabor, R. S., McKnight, D. M., and Brooks, P. D. (2016). Dissolved organic matter transport reflects hillslope to stream connectivity during snowmelt in a montane catchment. Water Resour. Res. 52, 4905–4923. doi:10.1002/2015WR017878
Campbell, J. L., and Laudon, H. (2019). Carbon response to changing winter conditions in northern regions: current understanding and emerging research needs. Environ. Rev. 27, 545–566. doi:10.1139/er-2018-0097
Chen, S., Yoshitake, S., Iimura, Y., Asai, C., and Ohtsuka, T. (2017). Dissolved organic carbon (DOC) input to the soil: DOC fluxes and their partitions during the growing season in a cool-temperate broad-leaved deciduous forest, central Japan. Ecol. Res. 32, 713–724. doi:10.1007/s11284-017-1488-6
Chen, X., Gong, L., Zhao, J., and Zhu, H. (2021). Litter decomposition, microbial community dynamics and their relationships under seasonal snow cover. Ecol. Eng. 159, 106089. doi:10.1016/j.ecoleng.2020.106089
Christopher, S. F., Shibata, H., Ozawa, M., Nakagawa, Y., and Mitchell, M. J. (2008). The effect of soil freezing on N cycling: comparison of two headwater subcatchments with different vegetation and snowpack conditions in the northern Hokkaido Island of Japan. Biogeochemistry 88, 15–30. doi:10.1007/s10533-008-9189-4
Dibbern, D., Schmalwasser, A., Lueders, T., and Totsche, K. U. (2014). Selective transport of plant root-associated bacterial populations in agricultural soils upon snowmelt. Soil Biol. biochem. 69, 187–196. doi:10.1016/j.soilbio.2013.10.040
Dittmar, T., Koch, B., Hertkorn, N., and Kattner, G. (2008). A simple and efficient method for the solid-phase extraction of dissolved organic matter (SPE-DOM) from seawater. Limnol. Oceanogr.: Methods. 6, 230–235. doi:10.4319/lom.2008.6.230
Gavazov, K., Ingrisch, J., Hasibeder, R., Mills, R. T. E., Buttler, A., Gleixner, G., et al. (2017). Winter ecology of a subalpine grassland: effects of snow removal on soil respiration, microbial structure and function. Sci. Total Environ. 590 (591), 316–324. doi:10.1016/j.scitotenv.2017.03.010
Groffman, P. M., Driscoll, C. T., Fahey, T. J., Hardy, J. P., Fitzhugh, R. D., and Tierney, G. L. (2001). Colder soils in a warmer world: a snow manipulation study in a northern hardwood forest ecosystem. Biogeochemistry 56, 135–150. doi:10.1023/A:1013039830323
Hensgens, G., Laudon, H., Peichl, M., Gil, I. A., Zhou, Q., and Berggren, M. (2020). The role of the understory in litter DOC and nutrient leaching in boreal forests. Biogeochemistry 149, 87–103. doi:10.1007/s10533-020-00668-5
Herrmann, M., Lehmann, K., Totsche, K. U., and Küsel, K. (2023). Seepage-mediated export of bacteria from soil is taxon-specific and driven by seasonal infiltration regimes. Soil Biol. biochem. 187, 109192. doi:10.1016/j.soilbio.2023.109192
Huang, R., Crowther, T. W., Sui, Y., Sun, B., and Liang, Y. (2021). High stability and metabolic capacity of bacterial community promote the rapid reduction of easily decomposing carbon in soil. Commun. Biol. 4, 1376. doi:10.1038/s42003-021-02907-3
Hulstaert, N., Shofstahl, J., Sachsenberg, T., Walzer, M., Barsnes, H., Martens, L., et al. (2020). ThermoRawFileParser: modular, scalable, and cross-platform RAW file conversion. J. Proteome Res. 19, 537–542. doi:10.1021/acs.jproteome.9b00328
Isobe, K., Oka, H., Watanabe, T., Tateno, R., Urakawa, R., Liang, C., et al. (2018). High soil microbial activity in the winter season enhances nitrogen cycling in a cool-temperate deciduous forest. Soil Biol. biochem. 124, 90–100. doi:10.1016/j.soilbio.2018.05.028
John, M. G. S., Orwin, K. H., and Dickie, I. A. (2011). No ‘home’ versus ‘away’ effects of decomposition found in a grassland–forest reciprocal litter transplant study. Soil Biol. biochem. 43, 1482–1489. doi:10.1016/j.soilbio.2011.03.022
Kaiser, K., and Kalbitz, K. (2012). Cycling downwards – dissolved organic matter in soils. Soil Biol. biochem. 52, 29–32. doi:10.1016/j.soilbio.2012.04.002
Kalbitz, K., Schwesig, D., Rethemeyer, J., and Matzner, E. (2005). Stabilization of dissolved organic matter by sorption to the mineral soil. Soil Biol. biochem. 37, 1319–1331. doi:10.1016/j.soilbio.2004.11.028
Kanehisa, M., and Goto, S. (2000). KEGG: kyoto encyclopedia of genes and genomes. Nucleic Acids Res. 28, 27–30. doi:10.1093/nar/28.1.27
Khatami, S., Deng, Y., Tien, M., and Hatcher, P. G. (2019). Formation of water-soluble organic matter through fungal degradation of lignin. Org. Geochem. 135, 64–70. doi:10.1016/j.orggeochem.2019.06.004
Koch, B. P., and Dittmar, T. (2006). From mass to structure: an aromaticity index for high-resolution mass data of natural organic matter. Rapid Commun. Mass Spectrom. 20, 926–932. doi:10.1002/rcm.2386
Koch, B. P., and Dittmar, T. (2016). From mass to structure: an aromaticity index for high-resolution mass data of natural organic matter. Rapid Commun. Mass Spectrom. 30, 250. doi:10.1002/rcm.7433
Kohlhepp, B., Lehmann, R., Seeber, P., Küsel, K., Trumbore, S. E., and Totsche, K. U. (2017). Aquifer configuration and geostructural links control the groundwater quality in thin-bedded carbonate–siliciclastic alternations of the Hainich CZE, central Germany. Hydrol. Earth Syst. Sci. 21, 6091–6116. doi:10.5194/hess-21-6091-2017
Küsel, K., Totsche, K. U., Trumbore, S. E., Lehmann, R., Steinhäuser, C., and Herrmann, M. (2016). How deep can surface signals Be traced in the critical Zone? Merging biodiversity with biogeochemistry research in a central German muschelkalk landscape. Front. Earth Sci. 4. doi:10.3389/feart.2016.00032
Kuzyakov, Y., and Blagodatskaya, E. (2015). Microbial hotspots and hot moments in soil: concept and review. Soil Biol. biochem. 83, 184–199. doi:10.1016/j.soilbio.2015.01.025
Lehmann, K., Lehmann, R., and Totsche, K. U. (2021). Event-driven dynamics of the total mobile inventory in undisturbed soil account for significant fluxes of particulate organic carbon. Sci. Total Environ. 756, 143774. doi:10.1016/j.scitotenv.2020.143774
Lipson, D. A., and Schmidt, S. K. (2004). Seasonal changes in an alpine soil bacterial community in the Colorado rocky mountains. Appl. Environ. Microbiol. 70, 2867–2879. doi:10.1128/AEM.70.5.2867-2879.2004
Meingast, K. M., Kane, E. S., Marcarelli, A. M., Wagenbrenner, J. W., and Beltrone, V. G. (2023). Seasonal trends of DOM character in soils and stream change with snowmelt timing. Water Resour. Res. 59, e2022WR032014. doi:10.1029/2022WR032014
Nakanishi, T., Atarashi-Andoh, M., Koarashi, J., Saito-Kokubu, Y., and Hirai, K. (2014). Seasonal and snowmelt-driven changes in the water-extractable organic carbon dynamics in a cool-temperate Japanese forest soil, estimated using the bomb-14C tracer. J. Environ. Radioact. 128, 27–32. doi:10.1016/j.jenvrad.2013.10.028
Orme, A. M., Lange, M., Schroeter, S. A., Wicke, M., Kolle, O., Pohnert, G., et al. (2022). Drought reduces release of plant matter into dissolved organic matter potentially restraining ecosystem recovery. Front. Soil Sci. 2, 904259. doi:10.3389/fsoil.2022.904259
Pazúr, R., Nováček, J., Bürgi, M., Kopecká, M., Lieskovský, J., Pazúrová, Z., et al. (2024). Changes in grassland cover in Europe from 1990 to 2018: trajectories and spatial patterns. Reg. Environ. Change 24, 51. doi:10.1007/s10113-024-02197-5
Phale, P. S., Basu, A., Majhi, P. D., Deveryshetty, J., Vamsee-Krishna, C., and Shrivastava, R. (2007). Metabolic diversity in bacterial degradation of aromatic compounds. OMICS J. Integr. Biol. 11, 252–279. doi:10.1089/omi.2007.0004
Räisänen, J. (2016). Twenty-first century changes in snowfall climate in Northern Europe in ENSEMBLES regional climate models. Clim. Dyn. 46, 339–353. doi:10.1007/s00382-015-2587-0
Rindt, O., Rosinger, C., Bonkowski, M., Rixen, C., Brüggemann, N., Urich, T., et al. (2023). Biogeochemical dynamics during snowmelt and in summer in the Alps. Biogeochemistry 162, 257–266. doi:10.1007/s10533-022-01005-8
Roth, V.-N., Lange, M., Simon, C., Hertkorn, N., Bucher, S., Goodall, T., et al. (2019). Persistence of dissolved organic matter explained by molecular changes during its passage through soil. Nat. Geosci. 12, 755–761. doi:10.1038/s41561-019-0417-4
Schroeter, S. A., Eveillard, D., Chaffron, S., Zoppi, J., Kampe, B., Lohmann, P., et al. (2022). Microbial community functioning during plant litter decomposition. Sci. Rep. 12, 7451. doi:10.1038/s41598-022-11485-1
Schulten, H.-R., and Schnitzer, M. (1997). The chemistry of soil organic nitrogen: a review. Biol. Fertil. Soils 26, 1–15. doi:10.1007/s003740050335
Schum, S. K., Brown, L. E., and Mazzoleni, L. R. (2020). MFAssignR: molecular formula assignment software for ultrahigh resolution mass spectrometry analysis of environmental complex mixtures. Environ. Res. 191, 110114. doi:10.1016/j.envres.2020.110114
Sebestyen, S. D., Boyer, E. W., Shanley, J. B., Kendall, C., Doctor, D. H., Aiken, G. R., et al. (2008). Sources, transformations, and hydrological processes that control stream nitrate and dissolved organic matter concentrations during snowmelt in an upland forest: stream nitrate and dom sources. Water Resour. Res. 44. doi:10.1029/2008WR006983
Solly, E. F., Schöning, I., Boch, S., Kandeler, E., Marhan, S., Michalzik, B., et al. (2014). Factors controlling decomposition rates of fine root litter in temperate forests and grasslands. Plant Soil 382, 203–218. doi:10.1007/s11104-014-2151-4
Sorensen, P. O., Beller, H. R., Bill, M., Bouskill, N. J., Hubbard, S. S., Karaoz, U., et al. (2020). The snowmelt niche differentiates three microbial life strategies that influence soil nitrogen availability during and after winter. Front. Microbiol. 11, 871. doi:10.3389/fmicb.2020.00871
Tanunchai, B., Ji, L., Schroeter, S. A., Wahdan, S. F. M., Hossen, S., Delelegn, Y., et al. (2023). FungalTraits vs. FUNGuild: comparison of ecological functional assignments of leaf- and needle-associated fungi across 12 temperate tree species. Microb. Ecol. 85, 411–428. doi:10.1007/s00248-022-01973-2
Uchida, M., Mo, W., Nakatsubo, T., Tsuchiya, Y., Horikoshi, T., and Koizumi, H. (2005). Microbial activity and litter decomposition under snow cover in a cool-temperate broad-leaved deciduous forest. Agric. For. Meteorol. 134, 102–109. doi:10.1016/j.agrformet.2005.11.003
Uselman, S. M., Qualls, R. G., and Lilienfein, J. (2007). Contribution of root vs. Leaf litter to dissolved organic carbon leaching through soil. Soil Sci. Soc. Am. J. 71, 1555–1563. doi:10.2136/sssaj2006.0386
Waggoner, D. C., and Hatcher, P. G. (2017). Hydroxyl radical alteration of HPLC fractionated lignin: formation of new compounds from terrestrial organic matter. Org. Geochem. 113, 315–325. doi:10.1016/j.orggeochem.2017.07.011
Weigand, H., and Totsche, K. U. (1998). Flow and reactivity effects on dissolved organic matter transport in soil columns. Soil Sci. Soc. Am. J. 62, 1268–1274. doi:10.2136/sssaj1998.03615995006200050017x
Wu, Y., Rong, X., Zhang, C., Zhang, R., He, T., Yu, Y., et al. (2020). Response of the intertidal microbial community structure and metabolic profiles to zinc oxide nanoparticle exposure. Int. J. Environ. Res. Public. Health 17, 2253. doi:10.3390/ijerph17072253
Yu, K., van den Hoogen, J., Wang, Z., Averill, C., Routh, D., Smith, G. R., et al. (2022). The biogeography of relative abundance of soil fungi versus bacteria in surface topsoil. Earth Syst. Sci. Data 14, 4339–4350. doi:10.5194/essd-14-4339-2022
Keywords: high resolution mass spectrometry (HR-MS), molecular formula assignment, soil processes, event, lysimeter, climate impact
Citation: Huang C, Schroeter SA, Lehmann K, Herrmann M, Totsche KU and Gleixner G (2024) Snowmelt seepage fluxes of dissolved organic matter in forest and grassland – a molecular-level case study from the Hainich Critical Zone Exploratory, Germany. Front. Earth Sci. 12:1458322. doi: 10.3389/feart.2024.1458322
Received: 02 July 2024; Accepted: 07 October 2024;
Published: 21 October 2024.
Edited by:
Evan S. Kane, Michigan Technological University, United StatesReviewed by:
Zhimin Song, Michigan Technological University, United StatesJessica Rush, University of Colorado Boulder, United States
Copyright © 2024 Huang, Schroeter, Lehmann, Herrmann, Totsche and Gleixner. This is an open-access article distributed under the terms of the Creative Commons Attribution License (CC BY). The use, distribution or reproduction in other forums is permitted, provided the original author(s) and the copyright owner(s) are credited and that the original publication in this journal is cited, in accordance with accepted academic practice. No use, distribution or reproduction is permitted which does not comply with these terms.
*Correspondence: Simon A. Schroeter, c2ltb24uc2Nocm9ldGVyQGJnYy1qZW5hLm1wZw==; Gerd Gleixner, Z2VyZC5nbGVpeG5lckBiZ2MtamVuYS5tcGcuZGU=