- 1Chongqing Gas Field, PetroChina Southwest Oil and Gasfield Company, Chongqing, China
- 2Southwest Oil and Gas Field Company Development Department, Chengdu, China
- 3School of Economics, Sichuan University of Science and Engineering, Yibin, China
Recent exploration efforts have revealed significant industrial gas flow from the Wujiaping Formation marine shale in the Longmen–Wushankan area of the eastern Sichuan Basin, underscoring its considerable exploration potential. In this study, the reservoir characteristics and exploration potential of the Wujiaping Formation shale gas in this area are further evaluated. On the basis of well rock electrical properties, experimental analyses, and depositional and structural patterns in the eastern region, we characterize the reservoir properties and identify the primary factors controlling shale gas accumulation. The results indicate that the Wujiaping shale is characterized by a high organic matter content, favorable pore types, high porosity, and a high content of brittle minerals, which are conducive to subsequent development and fracturing. A positive correlation is observed between the total organic carbon (TOC) content, porosity, brittle mineral content, and shale gas content. The TOC content is strongly correlated with the gas content (the correlation coefficient is 0.75). The high shale gas yield of the Wujiaping Formation is attributed to a combination of favorable sedimentary environments, pore conditions, roof and floor conditions, and fracturing capabilities. However, compared with the Longmaxi Formation in the Sichuan Basin, the Wujiaping Formation shale is characterized by a lower porosity, thinner shale, and deeper burial, posing challenges for exploration and development. In this study, criteria for evaluating Wujiaping Formation shale gas are established, and four favorable exploration areas are identified. Overall, the Permian Wujiaping Formation marine shale in the Longmen–Wushankan area holds promising exploration and development potential. Further exploration and an enhanced understanding of this formation will provide valuable guidance for future marine shale gas exploration and development in this area.
1 Introduction
The southern Sichuan Basin has emerged as a focal point for shale gas exploration and production in China, highlighted by the recent establishment of a supergiant shale gas field with an annual production capacity exceeding 200 × 108 m3 (Nie et al., 2018; Tang et al., 2019; Li, 2022). This remarkable development stems from successful exploration and production efforts targeting the Silurian Longmaxi Formation, which is widely recognized as a prolific shale gas reservoir. Success in the southern Sichuan Basin has prompted broader exploration activities, which have delineated shallow shale gas production zones (at depths less than 3,500 m) in key areas, such as Fuling, Weiyuan, Changning, and Zhaotong (Zhang et al., 2015; Chen et al., 2024). These shallower zones typically target organic-rich shales within the Longmaxi Formation and are characterized by favorable combinations of the total organic carbon (TOC) content, thermal maturity, thickness, and gas content. Building on these successes, exploration efforts have expanded into deeper portions of the Sichuan Basin (at depths of 3,500–4,500 m), with areas such as Luzhou showing significant promise for deep shale gas discoveries. These deeper plays often target the Longmaxi Formation and the underlying Permian Wujiaping Formation. The exceptional exploration and production potential of the marine shales in the Sichuan Basin and its adjacent areas can be attributed to a combination of favorable geological factors: depositional environments conducive to the accumulation of organic-rich sediments, suitable burial histories leading to optimal thermal maturation of organic matter, the presence of extensive overpressure, and the development of natural fractures enhancing permeability (Li et al., 2021; Yang et al., 2021; Yang et al., 2023). These factors, along with ongoing technological advancements in drilling and completion techniques, have positioned these marine shales as the primary target for shale gas exploration and production in China (Zou et al., 2022; Wang et al., 2022a).
Recent exploration has revealed that the HY1H and DY1H wells deployed and implemented by Sinopec and the CNPC in the Wujiaping Formation, respectively, yielded gas at rates of 8.9 × 104 m3/d and 32.06 × 104 m3/d, respectively (Feng et al., 2016; Zhu et al., 2019; Li, 2023; Smedley et al., 2024). This finding reveals new blocks and stratigraphic layers for marine shale gas exploration in the Sichuan Basin beyond the Ordovician Longmaxi Formation, Cambrian Danshancheng Formation, Cambrian Qiongzhusi Formation, Permian Dalong Formation, and Triassic Xujiahe Formation (Chen et al., 2019; Chen et al., 2021; Li et al., 2019; Dang et al., 2022; He et al., 2022a; He et al., 2022b; Jiang et al., 2023). Previous studies have investigated the distribution, sedimentary environment, and main factors controlling shale gas enrichment in the Wujiaping Formation in the western Hubei Province in depth (Zhai et al., 2019; Zeng et al., 2020; Wang et al., 2022b; Wang et al., 2024; Li et al., 2022a; Chen et al., 2022). Liang and Li (2021) clarified the geological conditions and exploration potential of shale gas in the Wujiaping Formation in eastern Chongqing–West Hubei, whereas Bao et al. identified the main factors controlling the high productivity of shale gas in the Hongxing area of western Hubei (Bao et al., 2023; Cao et al., 2021) defined the characteristics of organic-rich siliceous shale reservoirs in the Wujiaping Formation in the Lichuan area of western Hubei. However, the Wujiaping Formation in the Kaijiang‒Liangping trough in the eastern Sichuan Basin has not received much attention. Existing studies have focused mostly on areas of non-deep-water carbonate facies along the Kaijiang‒Liangping trough in northern and northwestern Sichuan (Liu and Xie, 2017; Zhang et al., 2022; Du et al., 2023) or have focused on the extension and expansion of the DY1H well. Limited detailed research has focused on the deep-water shale adjacent to the area of the DY1H well (He et al., 2021c). In this study, the characteristics of shale reservoirs in the Wujiaping Formation of the Nanya Syncline, eastern Sichuan Basin, are examined, drawing upon the electrical properties of well rocks and the sedimentary background of the eastern Sichuan Basin. In this research, the relationship between shale reservoir characteristics and the gas content is investigated, key factors controlling these reservoirs are identified, and they are compared to those of the Longmaxi Formation in the Sichuan Basin. By providing a clearer understanding of the Wujiaping Formation shale, in this study, insight into the exploration and exploitation of new shale gas resources in the Sichuan Basin is provided.
2 Geological setting
2.1 Structural setting
The eastern Sichuan Basin is part of the high and steep fold zone of eastern Sichuan, a relatively active tectonic area within the stable block. NE–SW-trending barrier-type folds have developed across the area from west to east. The Longtan and Wujiaping Formations are extensively developed in the eastern Sichuan Basin (Chen et al., 2020; Luo et al., 2024). These two formations represent isochronous heterotopic deposits. The Wujiaping Formation is exposed in northeastern Sichuan and adjacent areas and is characterized by marine carbonate rock deposits. In some parts of the eastern Sichuan Basin, thin to thick layers of shale and Emeishan basalts are interbedded and exposed (Wang et al., 2021). From the Guang’an–Chongqing area southeastward, the Wujiaping Formation gradually transitions into the Longtan Formation.
The study area is located mainly in the Nanya Syncline, which is a secondary structural unit of the high and steep fold zone in the eastern Sichuan Basin. The structural position is stable. Within the syncline, burial depths range from 4,000 m to 5,500 m, with predominant NE–SW-trending structures. The structural width gradually decreases from north to south, forming an overall elongated shape with significant folding intensity. The Datianchi structural belt and the eastern boundary of the Nanmenchang structural belt mark the western boundary. The southern part features a concealed Longmen structure, whereas the northern part features a concealed Wushankan structure (Figure 1A). Owing to the impact of the Dongwu Movement, the top of the Maokou Formation in the study area has been completely eroded, with only remnants of the Gufeng Member remaining (Wang et al., 2021). With fluctuating sea levels, coastal swamp deposits with interbedded thin coal seams and volcanic rocks subsequently developed in the lower part of the Wujiaping Formation in the early late Permian. This unit is in disconformable contact with the underlying Gufeng Member of the Maokou Formation. The upper part of the Wujiaping Formation is characterized by interbedded shale and limestone, which developed predominantly in a shelf facies environment (Yang et al., 2021; Yang et al., 2023).
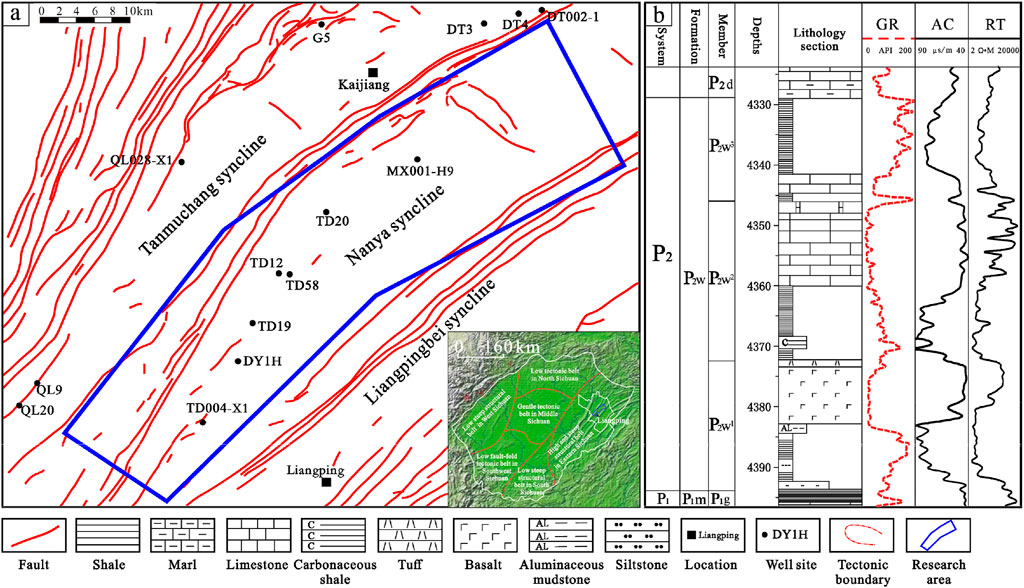
Figure 1. Structural location of the study area (A) and lithological profile of the Wujiaping Formation (B).
2.2 Stratigraphy
On the basis of the electrical, geochemical, and paleontological characteristics observed in well logs from wells in the study area, the Wujiaping Formation can be divided into three members. The shale and argillaceous mudstone at the base of the first member of the Wujiaping Formation unconformably overlie the carbonaceous and siliceous mudstone of the Gufeng Member (uppermost Maokou Formation) (Tang et al., 2020; Fan et al., 2024a; Li et al., 2024a). The shale and argillaceous mudstone (or thin coal seams) at the base of the second member of the Wujiaping Formation conformably overlie the volcanic rocks of the first member. The shale and marl at the base of the third member of the Wujiaping Formation conformably overlie the limestone with siliceous chert nodules of the second member. Finally, the marl and siliceous rocks at the base of the Changxing/Dalong Formation conformably overlie the shale and carbonaceous–calcareous–siliceous shale of the uppermost third member of the Wujiaping Formation (Figure 1B). The boundaries between these three members are distinct and easily recognizable, supporting the validity of this subdivision.
3 Samples and methods
3.1 Samples
In this study, we conducted core observations of well DY1H and collected more than 80 core samples. These core samples were subjected to thin section and scanning electron microscopy (SEM) observations, total organic carbon (TOC) analysis, porosity measurements, X-ray diffraction (XRD) analysis, and gas content determination (Table 1). All the experiments were conducted at the Analytical Laboratory of the Exploration and Development Research Institute of PetroChina Southwest Oil & Gasfield Company.
3.2 Methods
3.2.1 TOC analysis
TOC analysis was conducted via the acid dissolution method. The samples were ground to particle sizes smaller than 0.2 mm and then heated to 60°C–80°C after 3 mL of dilute hydrochloric acid was added (Zhang et al., 2023a; Tan et al., 2020). After a thorough reaction, the samples were washed with water and dried. The TOC content was subsequently measured via a LECO CS230 carbon‒sulfur analyzer. The measurement method followed the Chinese National Standard GB/T19145-2003 “Determination of TOC in Sedimentary Rocks.”
3.2.2 SEM observations
SEM was used to characterize the pore structures and types in the shale samples (Li et al., 2024b). The procedure involved the following steps: shale samples were cut into 10 mm × 5 mm × 5 mm cubes along the axial line via a water-free cutting technique and then embedded in cubic epoxy resin molds. Next, the samples were polished under vacuum conditions using a high-energy argon ion beam (Leica EM RES 102). Finally, the polished samples were imaged and analyzed via SEM (Zhao et al., 2022; Zhang et al., 2023b).
3.2.3 Characterization of the whole-rock mineral composition
A Rigaku X-ray diffractometer (Model TTR, 08070158) was used for mineral composition analysis (Shan et al., 2022; Xia et al., 2023). The steps involved the following: 50 g of the prepared sample was crushed to a size of 1 mm via an iron mortar. The crushed sample (50 g) was then placed in a porcelain mortar and mixed with a small amount of distilled water, followed by repeated grinding. The mixture was transferred to a beaker and mixed with 500 mL of distilled water. After stirring for approximately 10 min, the mixture was allowed to settle for at least 8 h. The clay fraction of the suspension (<2 μm) was extracted via a centrifuge. Approximately 40 mg of the clay fraction was mixed with 7 mL of distilled water and stirred thoroughly. The mixture was then spread evenly on a frosted glass slide and allowed to air dry. Finally, the sample was scanned via XRD to determine the mineral composition. The analysis followed the standard procedure outlined in the “Method for X-ray Diffraction Analysis of Clay Minerals in Sedimentary Rocks” (SY/T5163-2018), published by the China National Petroleum Corporation.
3.2.4 Isothermal adsorption
An isothermal adsorption experiment was conducted in which the collected shale samples were crushed and heated to remove adsorbed shale gas (Shan et al., 2018; Qi et al., 2022). The shale samples were subsequently placed in a sealed container. The temperature was kept constant, and the pressure inside the container was gradually increased. The amount of natural gas adsorbed by the experimental samples was observed and measured. Finally, the experimental data were fitted via the Langmuir equation to obtain the isothermal adsorption curve. In this study, nitrogen isothermal adsorption was employed to analyze the pore size distribution. This method effectively characterizes mesoporous structures with a measurement range of 1.5–100 nm and a sensitivity range of 2–50 nm.
4 Results
4.1 Organic matter abundance
Organic matter abundance is a crucial indicator for evaluating the hydrocarbon generation potential of shale (Dessouky, 2024; Xu et al., 2024). The TOC content was used to evaluate the abundance of organic matter in the shale. According to the experimental results, 64% of the samples presented TOC values exceeding 5%, whereas 28.5% had TOC values less than 2%. Notably, wells TD19 and TD58 had the highest TOC values, reaching 7.4%. The Wujiaping Formation exhibited high TOC contents, indicating significant hydrocarbon generation potential. Additionally, the TOC values in the deep-water shelf facies samples were significantly higher than those in the shallow-water shelf facies samples. The TOC value in well TD19 (7.4%), located in the deep-water shelf facies, was 5.2% higher than the TOC value in well TD4 (2.2%), located in the shallow-water shelf facies. The average TOC content in the deep-water shelf facies was 6.2%, whereas the average TOC content in the shallow-water shelf facies was 1.8%, indicating a significant difference (Figure 2A). From a vertical perspective, the TOC enrichment and high values in the third member of the Wujiaping Formation were significantly greater than those in the other members. From a planar distribution perspective, the TOC contents ranged from 3.0% to 7.0%, and the high-TOC zones were consistent with the morphology of trough development, with the average TOC generally exceeding 4.0% within the trough (Figure 2B). Overall, the TOC content in the study area rapidly changed, with high values occurring along the trough, indicating a significant patchy trend of enrichment.
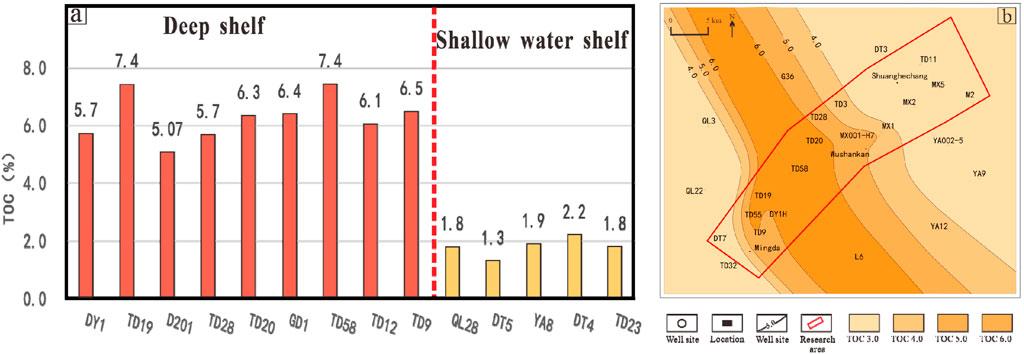
Figure 2. TOC contents shown in column format and plan view of the study area. (A) TOC contents of the main wells in the study area; (B) TOC contents of the third member of the Wujiaping Formation in the study area.
4.2 Spatial and physical characteristics of the reservoir
Three types of nanoscale pores were observed in the third member of the Wujiaping Formation: inorganic pores, organic pores, and microfractures (Figure 3). In accordance with the International Union of Pure and Applied Chemistry (IUPAC) pore diameter classification standard, these pores are classified into micropores (diameters < 2 nm), mesopores (diameters 2–50 nm), and macropores (diameters > 50 nm) (Guo et al., 2019; Li, 2022). The pores exhibited irregular shapes, with elliptical and circular shapes being the most common (Figure 3).
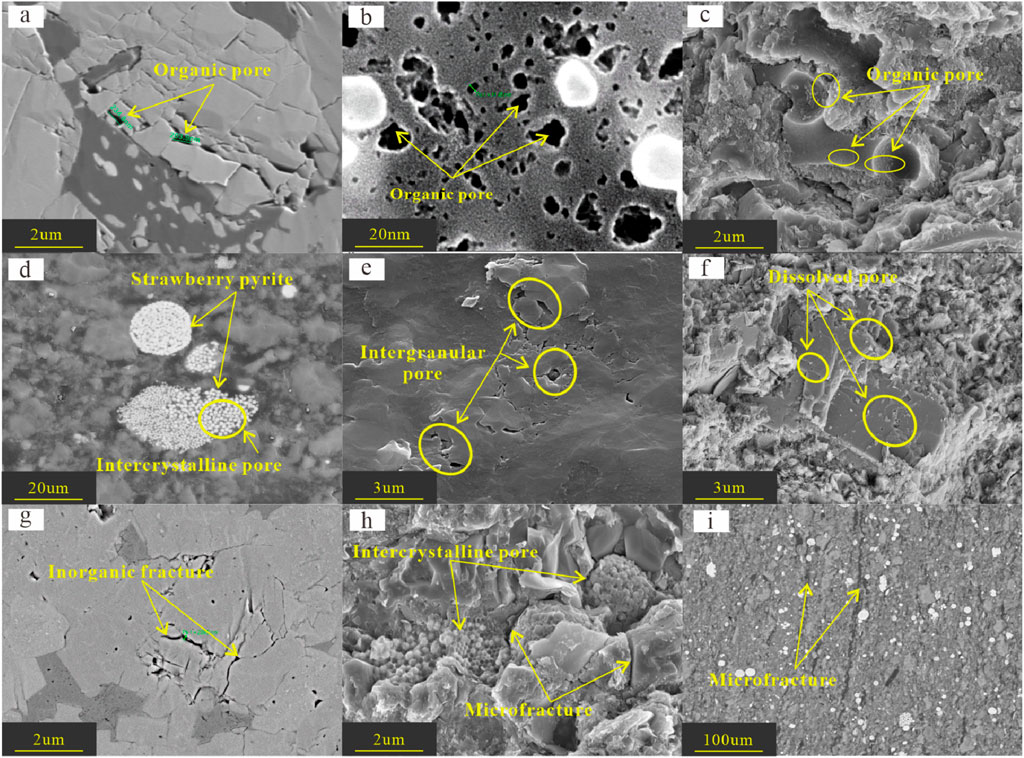
Figure 3. Micropore types in shale reservoirs of the Wujiaping Formation. (A) DY1H, 4,335.76 m, organic pores; (B) DY1H, 4,338.21 m, abundant interconnected organic pores developed from organic matter; (C) D201, 4,567.71 m, rounded organic pores; (D) DY1H, 4,337.20 m, intercrystalline pores developed in strawberry-shaped pyrite; (E) DY1H, 4,337.20 m, intergranular micropores, and microfractures; (F) D201, 4,568.53 m, a few dissolution pores developed in microcrystalline dolomite; (G) FT1, 4,578 m, microfractures; (H) D201, 4,564.12 m, concentrated distribution of strawberry-shaped pyrite, with microfractures developed at the edges; (I) DY1H, 4,334.15 m, bedding microfractures filled with organic matter.
The third member of the Wujiaping Formation in the study area was dominated by meso- and macropores, with a pore size distribution ranging from 20 to 200 nm (Figure 4B). The average porosity values were between 4.0% and 5.0%. Significant high-value zones, similar in morphology to the trough, were observed in the central and southern areas (Figure 4C). Additionally, the correlation between porosity and permeability was weak when the porosity was less than 6%. However, the correlation became more prominent when the porosity exceeded 6%, and the permeability was greater than 520 nD, reaching a correlation coefficient of 0.98 (Figure 4A). This suggests that the shale in the study area has a strong gas storage capacity with good pore connectivity.
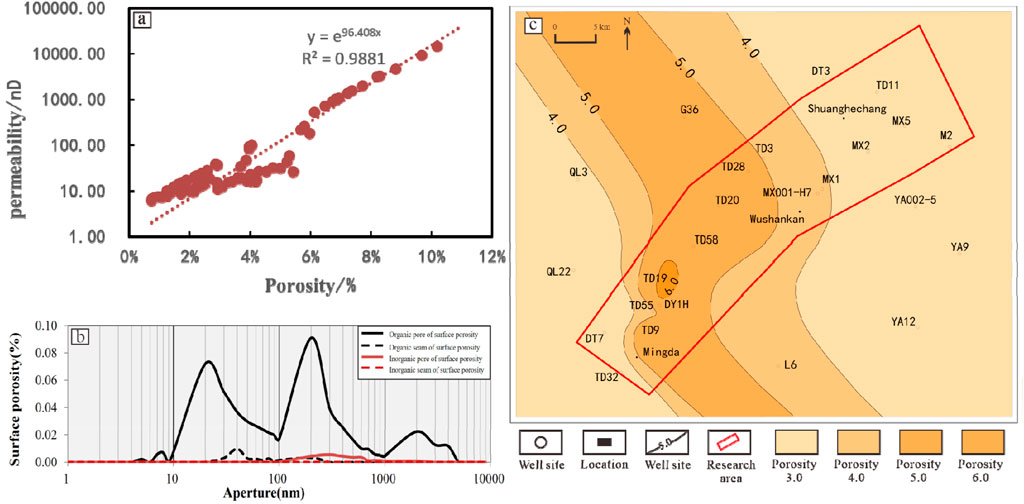
Figure 4. Shale porosity and distribution of the Wujiaping Formation. (A) Correlation between shale porosity and permeability in well DY1H; (B) Pore size characteristics of the shale samples; (C) Planar distribution of porosity.
4.3 Brittle mineral content
The variation in the brittle mineral content directly affects the mechanical properties of shale. A higher brittle mineral content indicates that shale is more prone to fracturing under the same stress conditions, leading to the formation of fractures, which are beneficial for hydraulic fracturing and gas desorption (Yin et al., 2018a; Yin et al., 2018b; Li, 2022). The brittle mineral content in the study area was calculated via the following equation.
where VBM is the brittle mineral content (%), Vquartz is the silicate content (%), Vcalcite is the carbonate content (%), Vpyrite is the pyrite content (%), and Vclay is the clay content (%).
The shale in the third member of the Wujiaping Formation in well DY1H was primarily composed of quartz, carbonate, and clay minerals, with minor amounts of feldspar and pyrite (Figure 5A). Planarly, a high-value zone formed around well DY1H, with values exceeding 80%. Vertically, the shale in the Wujiaping Formation exhibited some heterogeneity, with silicate minerals consistently exceeding 40%. Statistical analysis revealed that the brittle mineral contents ranged from 60.1% to 87.2%, with an average value of 77.54% (Figure 5B).
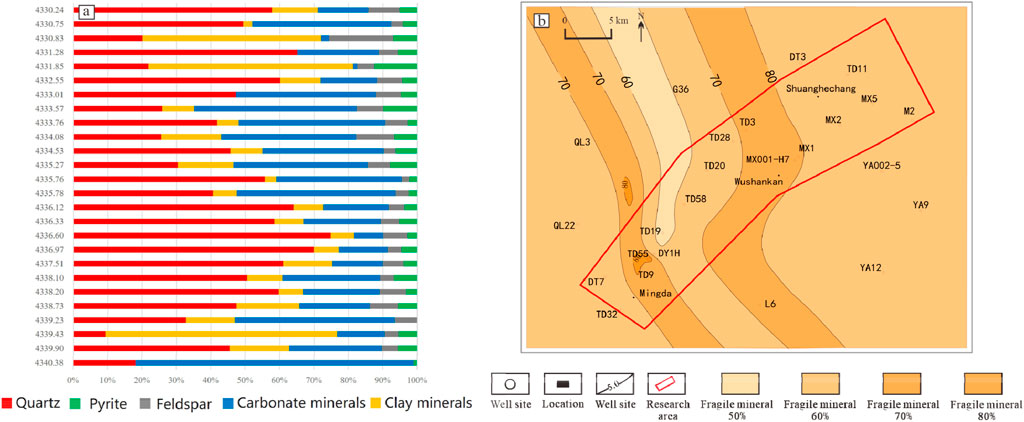
Figure 5. Characteristics and planar distribution of brittle minerals in the third member of the Wujiaping Formation. (A) Mineral composition in well DY1H; (B) Contour map showing the brittle mineral distribution.
4.4 Gas content
The gas content is the most direct parameter for evaluating the commercial viability of shale gas reservoirs. The total gas content in the main wells of the study area was generally high, averaging approximately 3.5 m3/t. The gas contents in wells DY1H and G18 reached 6.3 m3/t and 4.9 m3/t, respectively (Figure 6A). The third member of the Wujiaping Formation had the highest total gas content, with that in DY1H reaching 9.3 m3/t (Figure 7). Planarly, the total gas content reached high values along the trough zone, exhibiting a banded distribution (Figure 6B).
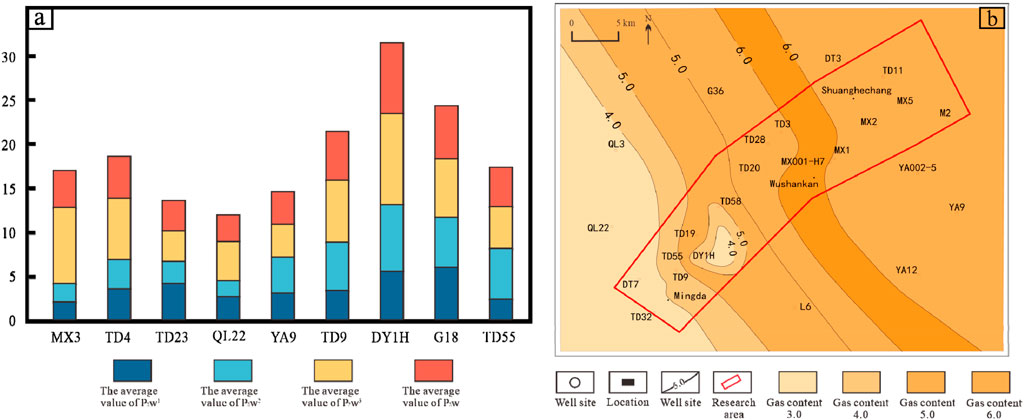
Figure 6. Column diagram of the gas contents in the study area and isogram of the gas contents of the third member of the Wujiaping Formation. (A) Column of the gas contents of some wells in the study area; (B) Isocontour plot of the gas contents in the study area.
5 Discussion
5.1 Main factors controlling shale gas reservoirs
Shale gas, a hydrocarbon resource found in organic-rich shale layers or interlayers, exists in free, adsorbed, or dissolved states, respectively, and migrates in situ or over short distances to form reservoirs (Tian et al., 2024; Fan et al., 2024; Lascelles, 2024). In the Longmen–Wushankan area of the eastern Sichuan Basin, deepwater shelf shale gas within the Wujiaping Formation is significantly influenced by the TOC content, porosity, and brittle mineral content. The presence of clay minerals in the lower Wujiaping Formation results in high experimental porosity but low effective porosity in the clay-rich shale. Consequently, the correlation between the porosity and gas content in the Wujiaping Formation is only 0.04 (Figure 8A). However, within the third member of the Wujiaping Formation, a positive correlation of 0.55 is observed between the porosity and shale gas content (Figure 8B).
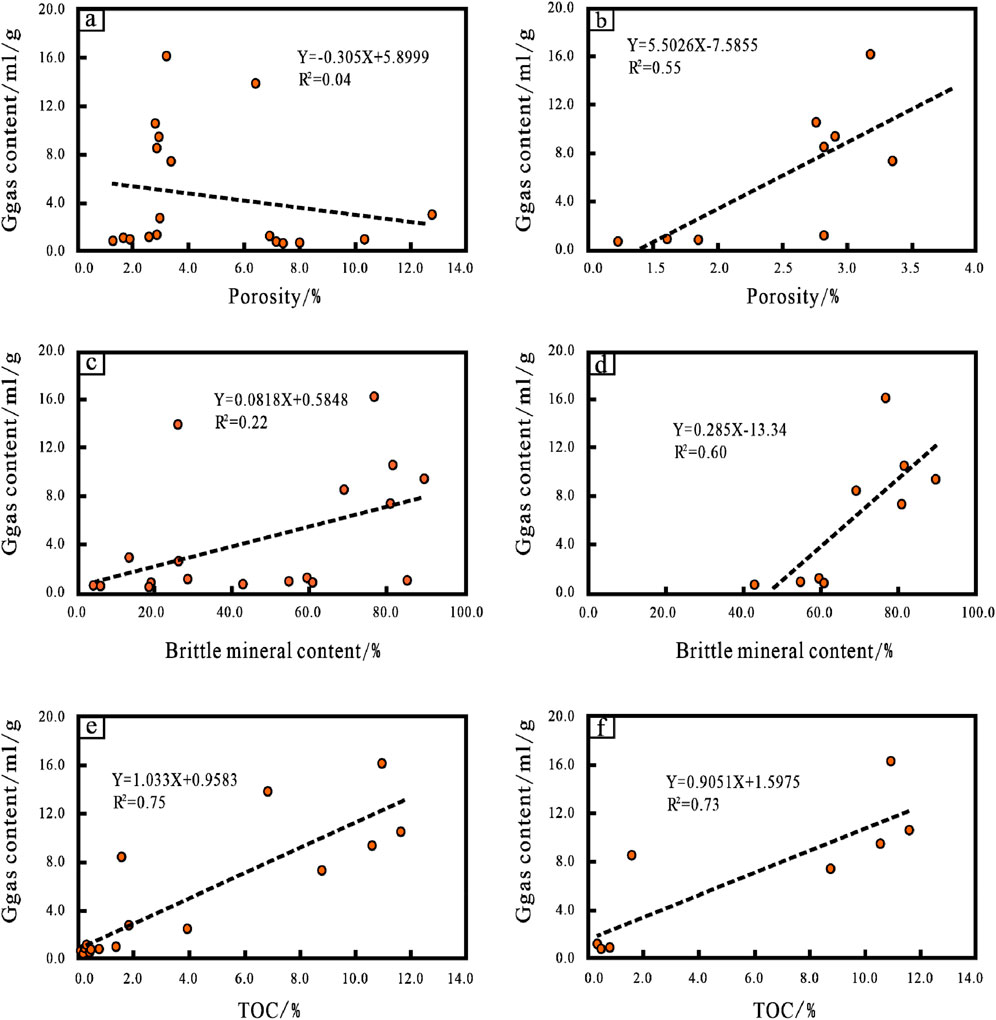
Figure 8. Relationship between reservoir parameters and the gas content. (A) Porosity and gas content of the Wujiaping Formation. (B) Porosity and gas content of the third member of the Wujiaping Formation. (C) Brittle mineral content and gas content of the Wujiaping Formation. (D) Brittle mineral content and gas content of the third member of the Wujiaping Formation. (E) TOC content and gas content of the Wujiaping Formation. (F) TOC content and gas content of the third member of the Wujiaping Formation.
Similarly, the high clay mineral content and low brittle mineral content in the lower Wujiaping Formation lead to a weak correlation of 0.22 between the brittle mineral and gas contents for the entire formation (Figure 8C). In contrast, the third member has a stronger positive correlation of 0.60 between the brittle mineral content and gas content (Figure 8D), suggesting that the high brittle mineral content in this member facilitates fracture development, thereby increasing shale gas accumulation. A strong positive correlation exists between the TOC content and gas content in the shale gas, with correlations of 0.75 and 0.73 for the entire Wujiaping Formation and its third member, respectively (Figures 8E, F).
Considering the three influential factors, the presence of clay minerals in the lower Wujiaping Formation means that a positive correlation with the gas content is observed only for the porosity and brittle mineral content of the third member. However, the TOC content positively correlates with the gas content throughout the Wujiaping Formation. Therefore, while the porosity, brittle mineral content, and TOC content are positively correlated with the shale gas content in the Wujiaping Formation, the TOC content is the most influential factor. This suggests that the TOC content is a key factor driving the high shale gas content observed in the Wujiaping Formation.
5.2 Main factors controlling shale gas enrichment and high yield
Multiple factors influence the enrichment and preservation of shale gas (Li et al., 2022b). The study area is located in the depositional center of the Kaijiang‒Liangping Trough in the eastern Sichuan Province and is characterized by deepwater shelf facies. This deepwater environment, which was typically anoxic and reducing, positively impacted the enrichment and preservation of shale gas. Within the study area, the TOC content exhibits noticeable heterogeneity and longitudinal differentiation. Shales deposited in the trough’s deepwater shelf facies display relatively high TOC contents, particularly those formed in the deepwater reducing environment of the third member of the Wujiaping Formation. These findings suggest that the deepwater shelf sedimentary environment provided the conditions for the development of organic-rich shales.
The Wujiaping Formation in the study area has average porosities ranging from 4% to 5%. These porosities, characterized by diverse pore types, contribute to shale gas preservation. Furthermore, the Wujiaping Formation’s roof and floor lithologies are crucial for gas preservation. The roof comprises dense limestone or marl belonging to the Dalong or Changxing Formations, whereas the floor consists of thickly layered limestone of the Maokou Formation in the eastern area. These bounding layers provide effective sealing capacity, sandwiching the Wujiaping Formation shale and further facilitating shale gas preservation.
Finally, the fracturing capacity is crucial for achieving high shale gas yields. The Wujiaping Formation shale contains abundant brittle minerals, as exemplified in well DY1H, and it has an average brittle mineral content of 77.54%. This high brittle mineral content enhances the effectiveness of later-stage fracturing operations, ultimately increasing shale gas production.
In summary, the high yield of shale gas in the Wujiaping Formation is controlled by a combination of factors: the sedimentary environment, pore conditions, roof and floor conditions, and fracturing ability. The sedimentary environment provides the material basis, pore conditions provide reservoir space, favorable preservation conditions govern shale gas enrichment, and the fracturing ability enables high production rates.
5.3 Comparison with shale gas in other areas of the Sichuan Basin
The brittle mineral content affects the effectiveness of hydraulic fracturing. The Wujiaping Formation shale has a high overall brittle mineral content, reaching a maximum of 94%, which is significantly higher than those in other areas, such as western Chongqing (maximum of 68%), Luzhou (maximum of 72%), and Weiyuan (maximum of 71%) (Figure 9A). The range of TOC contents of the Wujiaping Formation is broad, with contents varying from 2.8% to 6.7%, with an average exceeding 4.0% within the trough. These values are also significantly higher than those in other areas in the Sichuan Basin, indicating that the Wujiaping Formation has a high TOC content, which is conducive to hydrocarbon generation and results in high-quality shale gas (Figure 9B). The organic matter maturity (Ro) of the Wujiaping Formation is comparable to that in other areas (Figure 9D). However, in terms of the porosity, shale thickness, and burial depth, the Wujiaping Formation shale is slightly inferior to the Longmaxi Formation shale. The porosity values of the Wujiaping Formation shale range from 5.6% to 6.8%, which are slightly lower than those in areas such as Changning and Weiyuan (Figure 9C). The shale thickness is generally less than that of the Longmaxi Formation. Although the Wujiaping Formation shale is widely distributed, it interlayers with limestone vertically, resulting in significant variations in shale thickness. The single-layer shale thicknesses range from 1 to 45 m (generally less than 20 m), which are less than the 20–66 m thickness of the Longmaxi Formation (Figure 9E). With respect to the burial depth, the area of the Longmaxi Formation with mature rocks is buried between 2,000 and 4,500 m, with a relatively small thickness. However, the Wujiaping Formation in the eastern Sichuan Basin is generally buried between 3,000 and 6,000 m. The study area predominantly comprises deep shale formations buried at depths exceeding 4,500 m, posing significant challenges for exploration and development (Figure 9F).
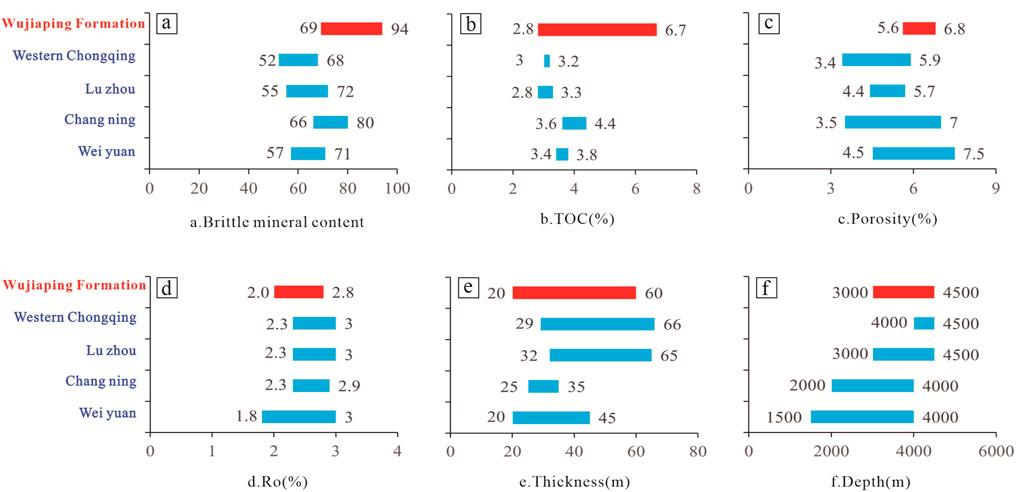
Figure 9. The characteristics of the Wujiaping Formation in the eastern Sichuan Basin are compared with those of the Longmaxi Formation shale in other major areas of the Sichuan Basin. (A) Brittle mineral content; (B) TOC; (C) Porosity; (D) Ro; (E) Thickness; (F) Burial depth.
The gas content is a direct indicator of shale gas production. The adsorbed gas content in the third member of the Wujiaping Formation in well DY1H is 4.2 m3/t, which is higher than that in the Longmaxi Formation in areas such as Changning (1.7 m3/t to 3.4 m3/t), Luzhou (1.5 m3/t to 2.0 m3/t), and Weiyuan (2.2 m3/t to 2.5 m3/t), indicating that the shale reservoir in the third member of the Wujiaping Formation has a strong adsorption capacity. The free gas content is 6.2 m3/t, which is slightly higher than those in Changning (2.5 m3/t to 4.4 m3/t), Luzhou (2.4 m3/t to 4.7 m3/t), and Weiyuan (4.3 m3/t to 6.1 m3/t). The total gas contents range from 7.2 m3/t to 10.67 m3/t, which are significantly greater than that of the Longmaxi Formation. Overall, the gas content in the third member of the Wujiaping Formation in the study area is relatively high, with significant exploration potential (Figure 10).
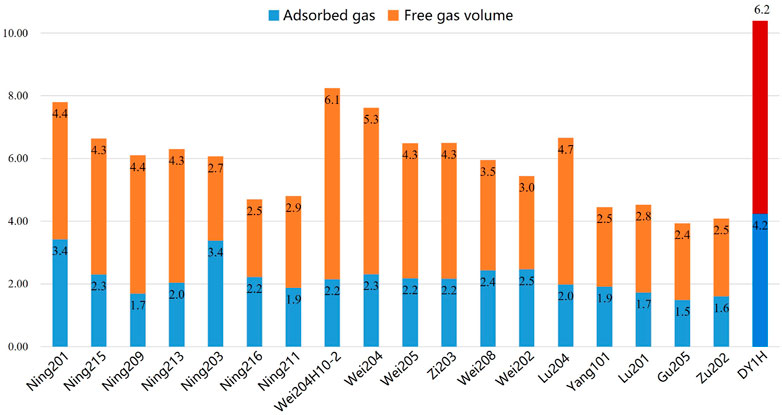
Figure 10. Gas content of the Wu3 Member in the study area compared with that of the Longmaxi Formation shale in other areas of the Sichuan Basin.
In general, the shale of the Permian Wujiaping Formation in the eastern Sichuan Basin has brittle mineral contents as high as 94% and a high organic matter content, which is conducive to shale gas formation. However, compared with the Longmaxi Formation, the Wujiaping Formation has no obvious advantage in terms of organic matter maturity, and it has a slightly poorer porosity, smaller shale thickness, and deeper burial depth, so exploration and development are more difficult (Yang et al., 2022; Du et al., 2022). However, the shale reservoir in the third member of the Wujiaping Formation has a strong adsorption capacity, and the total gas content is higher than that in the Longmaxi Formation, so the study interval has great development potential.
5.4 Classification criteria for favorable zones
Currently, the Longmaxi Formation shale in areas such as Weiyuan, Changning, Luzhou, and western Chongqing in the Sichuan Basin exhibits promising exploration results, with some areas demonstrating good value for commercial exploration and development (Fan et al., 2020). The Wujiaping Formation has certain similarities with the Longmaxi Formation shale. Therefore, we can utilize the evaluation criteria for the Longmaxi Formation shale to conduct a comprehensive assessment of shale gas.
The CNPC Southwest Oil & Gasfield Company has classified the Longmaxi Formation shale on the basis of well logging evaluation via four categories: source rocks, physical properties, gas contents, and rock mechanics. The classification used four indicators: total organic carbon (TOC), porosity, free and adsorbed gas contents, and brittle mineral content. Three reservoir types were defined: Type I reservoirs (TOC contents >2%, porosity values >5%, gas contents >2 m3/t, and brittle mineral contents >55%); Type II reservoirs (TOC contents between 1% and 2%, porosity values between 3% and 5%, gas contents between 1 m3/t and 2 m3/t, and brittle mineral contents between 35% and 55%); and Type III reservoirs (TOC contents <1%, porosity values <3%, gas contents <1 m3/t, and brittle mineral contents <35%).
Bai et al. (2023) evaluated the shale gas in the Wujiaping Formation of the northeastern Sichuan Basin. They established favorable zones of Type I and Type II reservoirs based on the shale effective thickness, TOC, Ro, gas content, distance to the erosion line, and burial depth (Table 2). The Type I favorable zone characteristics are as follows: effective thickness >20 m, TOC >2%, Ro >1.1%, gas content >1 m3/t, distance to the erosion line >5 km, burial depths between 1,000 and 4,000 m; the Type II favorable zone characteristics are as follows: effective thickness >10 m, TOC >1%, Ro >1.1%, gas content >0.7 m3/t, distance to the erosion line >5 km, burial depths between 4,000 and 4,500 m.
Organic-rich shale, deposited in favorable sedimentary environments, is widely distributed in the Longmen–Wushankan area. The overall depositional setting was a shelf environment, with the deep-water shelf facies of the third member of the Wujiaping Formation being the most favorable for shale gas. Multiple factors influence the enrichment and preservation of shale. In this study, evaluation criteria for high-quality shale in the study area are established on the basis of multiple indicators, including source rocks, physical properties, rock mechanics, and gas contents. The criteria are based on the evaluation standards for shale gas in the Longmaxi Formation in the Sichuan Basin and Bai et al.'s evaluation standards for shale gas in the Wujiaping Formation in the northeastern Sichuan Basin, while also incorporating industry standards NB/T 10398-2020 (Table 3).
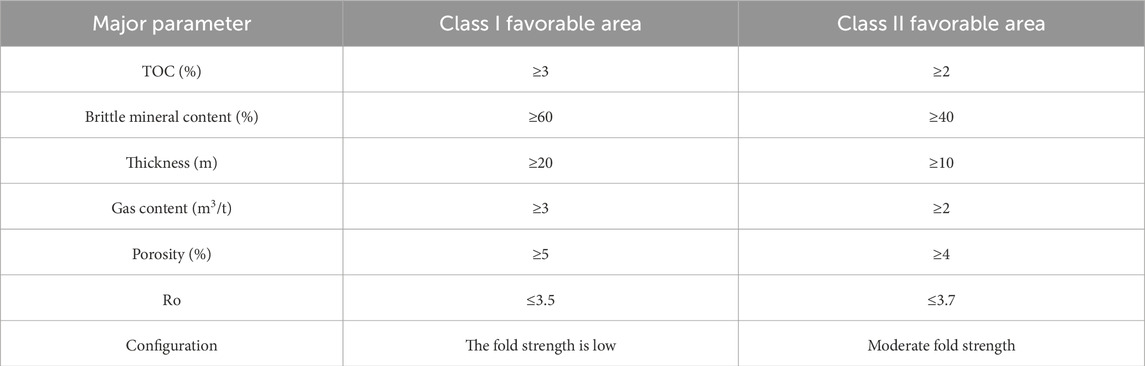
Table 3. Classification and evaluation criteria for high-quality shale from the Wujiaping Formation.
5.5 Prospects of shale gas exploration and development
Within the “Kaijiang–Liangping Trough,” the favorable zone of the Wujiaping Formation (buried between 4,500 and 5,000 m) can be divided into four synclinal belts from west to east: the Tanmuchang Syncline, the Nanya Syncline, the Liangping North Syncline, and the Yunyang–Wanxian Syncline (Yang et al., 2023). The shale reservoir in the deep-water shelf facies in the Wujiaping Formation in the Nanya Syncline, which is the southeastern end of the “Kaijiang–Liangping Trough,” exhibits stable lateral continuity. The third member of the Wujiaping Formation shale has good gas contents, high TOC contents, good compressibility, and high porosity. Organic-rich shale, with a generally low drilling density, is widely distributed in this area. The marine shale gas in the Wujiaping Formation in the Nanya Syncline has good exploration and development prospects. The area shallower than 5,000 m in the Wujiaping Formation covers more than 450 km2, with estimated geological resources of approximately 1,650 × 1012 m3.
Considering factors such as reservoirs, engineering, preservation, and surface conditions, the favorable zone in the Longmen–Wushankan area can be divided into four (1∼4) units of development (Figure 11).
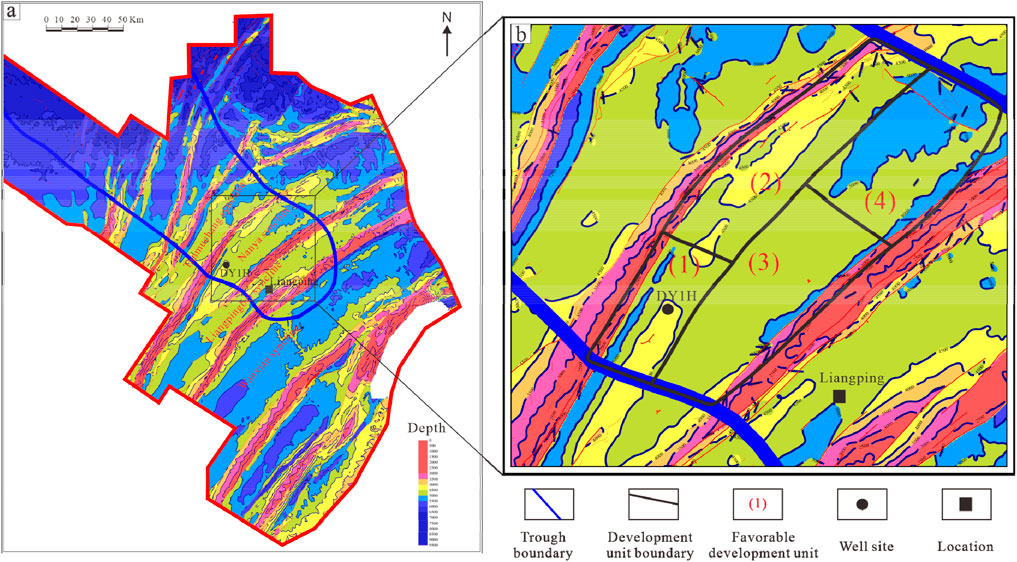
Figure 11. Location of the surface structure (A) and division of favorable areas for shale gas exploration (B) in the Nanya Syncline.
1) Located on a structural slope in the southwestern portion of the Nanya Syncline, this unit contains well DY1H at its center. It is characterized by a single secondary fault, indicating low fracture risk. The reservoir has consistent thicknesses of 15–20 m, a stable distribution, and burial depths ranging from 4,000 to 4,500 m. 2) Located on a structural slope in the northwestern portion of the Nanya Syncline, this unit is bound to the west by the Datianchi #1 fault. Two secondary faults are present in the northeast. The reservoir is characterized by a consistent thickness greater than 20 m, a stable distribution, and burial depths ranging from 4,000 to 5,000 m. 3) Located within the main stable area of the Nanya Syncline, this unit is characterized by a relatively gentle structural setting. With the Nanmenchang #1 fault as its eastern boundary, this unit is not internally faulted. The reservoir has consistent thicknesses of 15–20 m and is buried at depths ranging from 4,300 to 5,000 m. 4) Located in the northeastern portion of the Nanya Syncline, this unit is characterized by a relatively gentle structural setting within the stable synclinal area. Two secondary faults are present at the northeastern margin. Burial depths predominantly range from 4,300 to 5,000 m, locally exceeding 5,000 m.
6 Conclusion
(1) The Wujiaping Formation shale in the Longmen–Wushankan area exhibits favorable characteristics for shale gas accumulation, including high TOC contents, abundant pore types (organic pores, inorganic pores, and microfractures), high porosities, and significant brittle mineral contents. These properties are particularly pronounced within the third member of the Wujiaping Formation, highlighting its potential for shale gas exploration and development. While positive correlations exist between the TOC content, porosity, brittle mineral content, and shale gas content, the TOC content emerges as the dominant controlling factor.
(2) The high shale gas yield observed in the Wujiaping Formation is attributed to a combination of factors: a favorable sedimentary environment (providing source material), suitable pore characteristics (governing storage capacity), effective roof and floor conditions (enhancing gas preservation), and good fracability (enabling high production rates). However, compared with the well-established Longmaxi Formation in the Sichuan Basin, the Wujiaping Formation presents challenges because of its lower porosity, reduced thickness, and deeper burial depths.
(3) In this study, a framework for evaluating the Wujiaping Formation shale gas potential is established, drawing upon existing evaluation standards for both the Longmaxi and Wujiaping Formations and incorporating the unique characteristics of the study area. In this evaluation, four favorable exploration areas within the Nanya Synclinorium are identified, highlighting its potential for future exploration. Development strategies should leverage the high-yield model of well DY1H, focusing on replicating its design within a pilot test platform for a well group and gradually expanding development within a small, representative test area.
Data availability statement
The original contributions presented in the study are included in the article/supplementary material, further inquiries can be directed to the corresponding authors.
Author contributions
WT: Conceptualization, Data curation, Formal Analysis, Methodology, Resources, Writing–original draft. CT: Conceptualization, Data curation, Formal Analysis, Methodology, Resources, Writing–original draft. SM: Conceptualization, Data curation, Methodology, Resources, Writing–review and editing. YY: Data curation, Formal Analysis, Investigation, Methodology, Resources, Writing–review and editing. DL: Conceptualization, Formal Analysis, Methodology, Resources, Writing–original draft, Writing–review and editing. XY: Conceptualization, Formal Analysis, Resources, Writing–review and editing. LY: Conceptualization, Data curation, Resources, Writing–review and editing. HL: Conceptualization, Methodology, Investigation, Project administration, Writing–original draft, Writing–review and editing.
Funding
The authors declare that financial support was received for the research, authorship, and/or publication of this article. This study was financially supported by the scientific research project of Southwest Oil and Gas Field Company (20230304-10).
Conflict of interest
Authors WT, CT, YY, DL, and XY were employed by PetroChina Southwest Oil and Gasfield Company. Authors SM and LY were employed by Southwest Oil and Gas Field Company Development Department.
The remaining author declares that the research was conducted in the absence of any commercial or financial relationships that could be construed as a potential conflict of interest.
Publisher’s note
All claims expressed in this article are solely those of the authors and do not necessarily represent those of their affiliated organizations, or those of the publisher, the editors and the reviewers. Any product that may be evaluated in this article, or claim that may be made by its manufacturer, is not guaranteed or endorsed by the publisher.
References
Bai, W., Sun, X., and Han, H. (2023). Hydrocarbon generation potential and favorable area selection of shale in Wujiaping Formation of Permian in north Eastern Sichuan. IFEDC, 255–241. doi:10.26914/c.cnkihy.2023.059446
Bao, H. Y., Zhao, S., Liang, B., Zhou, L., and Liu, H. T. (2023). Enrichment and high yield of shale gas in the Permian Wujiaping Formation in Hongxing area of Eastern Sichuan and its exploration implications. China Petrol. expl. 28 (1), 71–82. doi:10.3969/j.issn.1672-7703.2023.01.007
Cao, T. T., Deng, M., Liu, H., Cao, Q. G., and Liu, G. X. (2021). Reservoir characteristics and methane adsorption capacity of the Wujiaping formation shale: a case study in Lichuan area, western Hubei. J. China Univ. Min. Tech. 50 (1), 138–153. doi:10.13247/j.cnki.jcumt.001245
Chen, F. R., Wei, X. F., Liu, Z. J., Ao, M. C., and Yan, J. H. (2020). Pore development characteristics and main controlling factors of the Permian marine-continent transitional shale in the Sichuan Basin. Nat. Gas. Geosci. 31 (11), 1593–1602. doi:10.11764/j.issn.1672-1926.2020.04.028
Chen, Y., Tang, S. H., Tang, H. M., Xi, Z., Zhao, N., Sun, S., et al. (2024). Pore structure heterogeneity of deep marine shale in complex tectonic zones and its implications for shale gas exploration. Energy Fuel 38 (3), 1891–1905. doi:10.1021/acs.energyfuels.3c03882
Chen, Y. H., Liu, S. H., Zhu, Z. W., Wang, Z., Sun, X., and Xu, T. (2021). Geochemical characteristics and sedimentary setting of chang 9 shale in the upper triassic yanchang Formation of southEastern ordos basin (NW China). J. Petrol. Sci. Eng. 196, 108081. doi:10.1016/j.petrol.2020.108081
Chen, Y. H., Zhu, Z. W., and Zhang, L. (2019). Control actions of sedimentary environments and sedimentation rates on lacustrine oil shale distribution, an example of the oil shale in the Upper Triassic Yanchang Formation, south Eastern Ordos Basin (NW China). Mar. Petrol. Geol. 102, 508–520. doi:10.1016/j.marpetgeo.2019.01.006
Chen, Z. P., Liao, Y., Li, L., Chen, L., Wang, P., Zuo, Y., et al. (2022). Implication of alkane carbon and hydrogen isotopes for genesis and accumulation of over-mature shale gas: a case study of Longmaxi Formation shale gas in Upper Yangtze area. Front. Earth Sci. 10, 901989. doi:10.3389/feart.2022.901989901989
Dang, W., Nie, H. K., Zhang, J. C., Tang, X., Jiang, S., Wei, X., et al. (2022). Pore-scale mechanisms and characterization of light oil storage in shale nanopores: new method and insights. Geosci. Front. 13 (5), 101424. doi:10.1016/j.gsf.2022.101424
Dessouky, O. K. (2024). Geochemical characteristics and genesis of niobium–yttrium–fluorine granite pegmatite from Hmrat El Sorwhyia area, North Eastern Desert, Egypt. Appl. Earth Sci. Online First. doi:10.1177/25726838241262934
Du, W., Yang, W., Li, X. Y., Shi, F., Lin, R., Wang, Y., et al. (2022). Differential reservoir-forming mechanisms of the lower paleozoic wufeng-longmaxi and niutitang marine gas shales in northern Guizhou Province, SW China: theories and models. Energies 15 (4), 5137. doi:10.3390/en15145137
Du, Y., Wang, X. Z., Tang, R. F., Zhu, Y., Yang, C., Zhou, H., et al. (2023). Extraordinarily high organic matter enrichment in upper permian Wujiaping Formation in the Kaijiang-Liangping trough, Sichuan Basin. Energies 16 (1), 349. doi:10.3390/en16010349
Fan, C. H., Li, H., Qin, Q. R., He, S., and Zhong, C. (2020). Geological conditions and exploration potential of shale gas reservoir in Wufeng and Longmaxi Formation of southeastern Sichuan Basin, China. J. Petrol. Sci. Eng. 191, 107138. doi:10.1016/j.petrol.2020.107138
Fan, C. H., Nie, S., Li, H., Pan, Q. C., Shi, X. C., Qin, S. M., et al. (2024a). Geological characteristics and major factors controlling the high yield of tight oil in the Da’anzhai member of the western Gongshanmiao in the central Sichuan basin, China. Geomech. Geophys. Geol. 10 (1), 67. doi:10.1007/s40948-024-00783-9
Fan, C. H., Nie, S., Li, H., Radwan, A. E., Pan, Q. C., Shi, X. C., et al. (2024). Quantitative prediction and spatial analysis of structural fractures in deep shale gas reservoirs within complex structural zones: a case study of the Longmaxi Formation in the Luzhou area, southern Sichuan Basin, China. J. Asian Earth Sci. 263, 106025. doi:10.1016/j.jseaes.2024.106025
Feng, J. H., Cai, X. Y., Mou, Z. H., and Gao, S. L. (2016). Oil and gas exploration of China petroleum and chemical corporation during the 12th Five-Year Plan and the prospect for the 13th Five-Year Plan. China Petrol. expl. 21 (3), 1–13. doi:10.3969/j.issn.1672-7703.2016.03.001
Guo, X. B., Huang, Z. L., Zhao, L. B., Han, W., Ding, C., Sun, X., et al. (2019). Pore structure and multi-fractal analysis of tight sandstone using MIP, NMR and NMRC methods: a case study from the Kuqa depression, China. J. Petrol. Sci. Eng. 178, 544–558. doi:10.1016/j.petrol.2019.03.069
He, S., Li, H., Qin, Q. R., and Long, S. X. (2021c). Influence of mineral compositions on shale pore development of Longmaxi Formation in the Dingshan area, southEastern Sichuan Basin, China. Energy Fuel 35 (13), 10551–10561. doi:10.1021/acs.energyfuels.1c01026
He, S., Qin, Q. R., Li, H., and Wang, S. L. (2022b). Deformation differences in complex structural areas in the southern Sichuan Basin and its influence on shale gas preservation: a case study of Changning and Luzhou area. Front. Earth Sci. 9, 818155. doi:10.3389/feart.2021.818155
He, S., Qin, Q. R., Li, H., and Zhao, S. X. (2022a). Deformation differences in complex structural areas in the southern Sichuan Basin and its influence on shale gas preservation: a case study of changning and Luzhou areas. Front. Earth Sci. 9, 818543. doi:10.3389/feart.2021.818534
Jiang, S. L., Zhou, Q. H., Li, Y. J., and Yang, R. L. (2023). Fracability evaluation of the middle–upper Permian marine shale reservoir in well HD1, western Hubei area. Sci. Rep. 13, 14319. doi:10.1038/s41598-023-40735-z
Lascelles, D. F. (2024). Plate tectonics caused the demise of banded iron formations. Appl. Earth Sci. 122 (4), 230–241. doi:10.1179/1743275814Y.0000000043
Li, H. (2022). Research progress on evaluation methods and factors influencing shale brittleness: a review. Energy Rep. 8, 4344–4358. doi:10.1016/j.egyr.2022.03.120
Li, H. (2023). Coordinated development of shale gas benefit exploitation and ecological environmental conservation in China: a mini review. Front. Ecol. Evol. 11, 1232395. doi:10.3389/fevo.2023.1232395
Li, H., Tang, H. M., Qin, Q. R., Wang, Q., and Zhong, C. (2019). Effectiveness evaluation of natural fractures in Xujiahe Formation of Yuanba area, Sichuan basin, China. Arab. J. Geosci. 12 (6), 194. doi:10.1007/s12517-019-4292-5
Li, H., Wang, Q., Qin, Q. R., and Ge, X. Y. (2021). Characteristics of natural fractures in an ultradeep marine carbonate gas reservoir and their impact on the reservoir: a case study of the Maokou Formation of the JLS Structure in the Sichuan Basin, China. Energy Fuel 35 (16), 13098–13108. doi:10.1021/acs.energyfuels.1c01581
Li, H., Zhou, J. L., Mou, X. Y., Guo, H., Wang, X., et al. (2022a). Pore structure and fractal characteristics of the marine shale of the Longmaxi Formation in the changning area, southern Sichuan Basin, China. Front. Earth Sci. 10, 1018274. doi:10.3389/feart.2022.1018274
Li, J., Li, H., Jiang, W., Cai, M., He, J., Wang, Q., et al. (2024a). Shale pore characteristics and their impact on the gas-bearing properties of the Longmaxi Formation in the Luzhou area. Sci. Rep. 14, 16896. doi:10.1038/s41598-024-66759-7
Li, H., He, S., Radwand, A. E., Xie, J. T., and Qin, Q. R. (2024b). Quantitative analysis of pore complexity in lacustrine organic-rich shale and comparison to marine shale: Insights from experimental tests and fractal theory. Energy Fuel. 38 (17), 16171–16188. doi:10.1021/acs.energyfuels.4c03095
Li, J. J., Qin, Q. R., Li, H., and Wan, Y. F. (2022b). Numerical simulation of the stress field and fault sealing of complex fault combinations in Changning area, Southern Sichuan Basin, China. Energy Sci. Eng. 10 (2), 278–291. doi:10.1002/ese3.1044
Liang, X., and Li, L. (2021). Geological conditions and exploration potential for shale gas in upper permian Wujiaping Formation in the eastern of western Hubei-Eastern Chongqing. Petrol. Geol. Exp. 43 (3), 386–394. doi:10.11781/sysydz202103386
Liu, C., and Xie, Q. B. (2017). Depositional, sedimentary, and diagenetic controls on reservoir quality in carbonate successions: a case study from the carbonate gas reservoirs of the Lower Triassic Feixianguan Formation, Eastern Sichuan Basin, China. J. Petrol. Sci. Eng. 163, 484–500. doi:10.1016/j.petrol.2017.12.063
Luo, T., Guo, X. W., He, Z. L., Dong, T., Tao, Z., Yang, R., et al. (2024). Fluid evolution and gas enrichment in the Wufeng-Longmaxi shale reservoirs of the Eastern Sichuan Basin, SW China. J. Asian Earth Scie. 259, 105905. doi:10.1016/j.jseaes.2023.105905
Nie, H. K., Jin, Z. J., and Zhang, J. C. (2018). Characteristics of three organic matter pore types in the Wufeng-Longmaxi shale of the Sichuan Basin, southwest China. Sci. Rep. 8, 7014. doi:10.1038/s41598-018-25104-5
Qi, R., Qin, X., Lu, C., Ma, C., Mao, W., and Zhang, W. (2022). Experimental study on the isothermal adsorption of methane gas in natural gas hydrate argillaceous silt reservoir. Adv. Geo-Energy Res. 6 (2), 143–156. doi:10.46690/ager.2022.02.06
Shan, C., Ye, C., Zhang, Z., Zou, C., He, F., Zhang, H., et al. (2022). Reservoir characteristics and resource potential analysis of transitional shale gas in Southern Sichuan Basin. Front. Earth Sci. 10, 909469. doi:10.3389/feart.2022.909469
Shan, C., Zhang, T., Liang, X., Zhang, Z., Zhu, H., Yang, W., et al. (2018). Influence of chemical properties on CH 4 adsorption capacity of anthracite derived from southern Sichuan Basin, China. China. Mar. Petrol. Geol. 89, 387–401. doi:10.1016/j.marpetgeo.2017.10.008
Smedley, P. L., Allen, G., Baptie, B. J., Fraser-Harris, A., Ward, R., Chambers, R., et al. (2024). Equipping for risk: lessons learnt from the UK shale-gas experience on assessing environmental risks for the future geoenergy use of the deep subsurface. Sci. Total Environ. 921, 171036. doi:10.1016/j.scitotenv.2024.171036
Tan, B., Cheng, G., Zhu, X. M., and Yang, X. B. (2020). Experimental study on the physisorption characteristics of O2 in coal powder are effected by coal nanopore structure. Sci. Rep. 10, 6946. doi:10.1038/s41598-020-63988-4
Tang, L., Song, Y., Jiang, S., Li, L. X., Li, Z., Li, Q. W., et al. (2020). Sealing mechanism of the roof and floor for the Wufeng-Longmaxi shale gas in the southern Sichuan Basin. Energy Fuel 34 (6), 6999–7018. doi:10.1021/acs.energyfuels.0c00983
Tang, X. L., Jiang, Z. X., Jiang, S., Cheng, L. J., Zhong, N. N., Tang, L., et al. (2019). Characteristics, capability, and origin of shale gas desorption of the Longmaxi Formation in the southeastern Sichuan Basin, China. Sci. Rep. 9, 1035. doi:10.1038/s41598-018-37782-2
Tian, H. N., Tang, J. P., Zhang, S. P., and Zhang, X. (2024). Adsorption-desorption characteristics of coal-bearing shale gas under three-dimensional stress state studied by low field nuclear magnetic resonance spectrum experiments. Sci. Rep. 14, 5566. doi:10.1038/s41598-024-54532-9
Wang, E. Z., Guo, T. L., L, B., et al. (2022a). Lithofacies and pore features of marine-continental transitional shale and gas enrichment conditions of favorable lithofacies: a case study of Permian Longtan Formation in the Lintanchang area, southeast of Sichuan Basin, SW China. Petrol. explor. Dev. 49 (6), 1310–1322. doi:10.11698/PED.20220273
Wang, J., Zhu, C. G., Li, X., Wang, B., and Zhang, Y. T. (2024). Experimental study on triaxial disturbance and failure characteristics of rock-like specimens. J. Min. Strata Control Eng. 6 (2), 023034. doi:10.13532/j.jmsce.cn10-1638/td.20240015.004
Wang, P., Liu, Z., Li, X., et al. (2022b). Development of the upper permian wujiaping shale in hongxing area, eastern Sichuan Basin, and its significance to shale gas enrichment. Oil Gas. Geol. 43 (5), 1102–1114. doi:10.11743/ogg20220508
Wang, X. Z., Li, B., Yang, X. Y., et al. (2021). Characteristics of “Guangyuan-Wangcang” trough during late Middle Permian and its petroleum geological significance in northern Sichuan Basin, SW China. Petrol. Expl. Dev. 48 (3), 562–574. doi:10.11698/PED.2021.03.11
Xia, Y., Xu, S., Lu, C., Andersen, P. Ø., and Cai, J. (2023). Characterization and capillary pressure curve estimation of clayey-silt sediment in gas hydrate reservoirs of the South China Sea. Adv. Geo-Energy Res. 10 (3), 200–207. doi:10.46690/ager.2023.12.06
Xu, S., Wen, J., Liu, K., Shi, X., and Dong, T. (2024). Brittle minerals, mechanical properties and fracability evaluation of shales. Adv. Geo-Energy Res. 214 (1), 8–11. doi:10.46690/ager.2024.10.03
Yang, W., Wang, Y. H., Du, W., Song, Y., Jiang, Z., Wang, Q., et al. (2022). Behavior of organic matter-hosted pores within shale gas reservoirs in response to differential tectonic deformation: potential mechanisms and innovative conceptual models. Gas. Sci. Eng. 102, 104571. doi:10.1016/j.jngse.2022.104571
Yang, Y., Chen, Y., Liu, S., et al. (2021). Status, potential and prospect of shale gas exploration and development in the Sichuan Basin and its periphery. Nat. Gas. Ind. 41 (1), 42–58. doi:10.3787/j.issn.1000-0976.2021.01.004
Yang, Y., Wang, H., Xie, J., et al. (2023). Exploration breakthrough and prospect of permian marine shale gas in the kaijiang-liangping trough, Sichuan Basin. Nat. Gas. Ind. 43 (11), 19–27. doi:10.3787/j.issn.1000-0976.2023.11.003
Yin, S., Jia, Q., and Ding, W. L. (2018b). 3D paleotectonic stress field simulations and fracture prediction for marine-continental transitional facies forming a tight-sandstone reservoir in a highly deformed area. J. Geophys. Eng. 15 (4), 1214–1230. doi:10.1088/1742-2140/aaad97
Yin, S., Lv, D. W., Jin, L., and Ding, W. L. (2018a). Experimental analysis and application of the effect of stress on continental shale reservoir brittleness. J. Geophys. Eng. 15 (2), 478–494. doi:10.1088/1742-2140/aaa5d2
Zeng, Q., Hu, X., Qu, H., et al. (2020). Division of strata and distribution of favorable reservoir rocks of the Upper Permian Wujiaping formation in the northwestern Sichuan Basin. Nat. Gas. Expl. Dev. 43 (4), 33–47. doi:10.12055/gaskk.issn.1673-3177.2020.04.004
Zhai, G. Y., Li, J., Jiao, Y., Wang, Y., Liu, G., Xu, Q., et al. (2019). Applications of chemostratigraphy in a characterization of shale gas sedimentary microfacies and predictions of sweet spots -taking the Cambrian black shales in Western Hubei as an example. Mar. Petrol. Geol. 109, 547–560. doi:10.1016/j.marpetgeo.2019.06.045
Zhang, H., Wu, Y. P., and Xie, P. S. (2023a). Experimental study on evolution law of compressive mechanical properties of interbedded roofrock. J. Min. Strata Control Eng. 5 (1), 013011. doi:10.13532/j.jmsce.cn10-1638/td.20220923.001
Zhang, P. W., Hu, L. M., Meegoda, J., and Gao, S. Y. (2015). Micro/nano-pore network analysis of gas flow in shale matrix. Sci. Rep. 5, 13501. doi:10.1038/srep13501
Zhang, Y., Huang, H. S., Zeng, Q., et al. (2022). Microfacies of limestones from upper permian Wujiaping Formation at gediba section in the northwestern Sichuan, southwest China. J. Earth Sci. Env. 44 (2), 287–297. doi:10.19814/j.jese.2021.08007
Zhang, Y. X., Pan, H. F., Bai, Y. B., Chen, G., and Luo, J. (2023b). Pore characteristics, oil contents and factors influencing laminated shale in the first member of the Qingshankou Formation in the Gulong sag, northern Songliao basin. Minerals 13 (9), 1220. doi:10.3390/min13091220
Zhao, J. P., Chen, H., Zhang, J. Y., Zhang, W. J., and Chen, G. (2022). Quantitative characterization of organic and inorganic pores in shale based on FIB-SEM. Front. Earth Sci. 10, 994312. doi:10.3389/feart.2022.994312
Zhu, W. C., Niu, L. L., Li, S. H., and Li, S. (2019). Creep-impact test of rock: status-of-the-art and prospect. J. Min. Strata Control Eng. 1 (1), 013003. doi:10.13532/j.jmsce.cn10-1638/td.2019.02.007
Keywords: shale gas, reservoir characteristics, geological conditions, Nanya Syncline, Wujiaping Formation, Longmen-Wushankan area
Citation: Tang W, Tuo C, Ma S, Yao Y, Liu D, Yang X, Yang L and Li H (2024) Shale reservoir characterization and implications for the exploration and development of the upper Permian Wujiaping Formation, Longmen–Wushankan area, eastern Sichuan Basin. Front. Earth Sci. 12:1453098. doi: 10.3389/feart.2024.1453098
Received: 22 June 2024; Accepted: 16 August 2024;
Published: 13 September 2024.
Edited by:
Gang Rao, Southwest Petroleum University, ChinaReviewed by:
Jingshou Liu, China University of Geosciences Wuhan, ChinaWei Yang, China University of Petroleum, Beijing, China
Copyright © 2024 Tang, Tuo, Ma, Yao, Liu, Yang, Yang and Li. This is an open-access article distributed under the terms of the Creative Commons Attribution License (CC BY). The use, distribution or reproduction in other forums is permitted, provided the original author(s) and the copyright owner(s) are credited and that the original publication in this journal is cited, in accordance with accepted academic practice. No use, distribution or reproduction is permitted which does not comply with these terms.
*Correspondence: Dongxi Liu, ldxi@petrochina.com.cn; Hu Li, lihu860628@126.com, lihu@suse.edu.cn