- 1School of Resources and Environmental Engineering, Ludong University, Yantai, China
- 2Key Laboratory of Marine Geology and Metallogeny, First Institute of Oceanography, Ministry of Natural Resources, Qingdao, China
In order to distinguish terrestrial material sources in the Okinawa Trough (OT), this study analyzed four surface sediment samples from the northern, middle, and southern parts of the OT using detrital zircon U-Pb age analysis. The detrital zircon age distributions in the northern Okinawa Trough (N-OT) predominantly fall into 1,000−2,300 Ma and 100−600 Ma age groups. In contrast, in the middle Okinawa Trough (M-OT) and southern Okinawa Trough (S-OT), the detrital zircon age groups of 100−600 Ma and 600–1,000 Ma increase significantly, while the age groups of 1,000−2,300 Ma and >2,300 Ma decrease. Detrital zircons from the N-OT primarily originate from the Yellow River and mixed with the Old Yellow River, while those from the M-OT come mainly from the Yellow River, Yangtze Rivers, and East China Sea. And Zhuoshui River maybe a potential source for the M-OT. The detrital zircons from the S-OT are mainly influenced by the Yangtze River, with some effects from the Lanyang River. This study utilizes detrital zircon U-Pb age analysis to gain a better understanding of the terrestrial material sources in various regions of the OT. Most of the zircon grain are coarse, with grain sizes of 40–150 μm long and 30–100 μm wide in the N-OT, smaller in the M-OT and S-OT in this study, which are probably not modern deposits, but the products in the low sea level period of last glaciation mixed with modern sediments. The transport and sedimentation processes reflect the comprehensive influence of sea level change, Kuroshio Current, river input, East Asian monsoon and other factors from last glaciation to present.
1 Introduction
The Okinawa Trough (OT) lies between the continental shelf of the East China Sea (ECS) and the Ryukyu Island Arc, marking it as a typical marginal basin in the northwest Pacific Ocean. As a transition between the shallow continental shelf and the deep ocean, the OT’s seabed, which is covered by thousands of meters of sediments, encapsulates records of sea level fluctuations, tectonic activities, climate change, and paleoenvironmental evolution from the late Quaternary (Liu et al., 1999; Li et al., 2005; Dou et al., 2012; Dou et al., 2015; Wang et al., 2015; Zhao et al., 2019). Thus, the OT offers a prime location for investigating land-sea interactions and the paleoenvironment at the northwestern Pacific Ocean’s periphery (Li and Chang, 2009; Dou et al., 2010a). Sediment studies within the OT remain a focal point in marginal sea research. Identifying sediment sources is crucial to addressing these issues about sea level fluctuations, tectonic activities, climate change.
Researchers have employed various methods to identify the source of sediments in the OT, such as mineralogy, rare earth elements, and Sr-Nd isotopes, as well as environmental magnetism (Chen et al., 1979; Zhao and Yan, 1992; Liu J. et al., 2007; Dou et al., 2010b; Dou et al., 2012; Li et al., 2015; Wang et al., 2015; Dou et al., 2016; Zhao et al., 2018; Hu et al., 2020; Zou et al., 2021; Li et al., 2023). These studies suggested that the terrigenous sediment sources of the OT predominantly include direct supply from major rivers, particularly the Yangtze River and Yellow River (Katayama and Watanabe, 2003) and lateral transport via the ECS shelf (Iseki et al., 2003; Oguri et al., 2003). Rivers in Taiwan also discharge terrestrial sediments into the OT (Liu et al., 2008). In addition, widespread fallout tephra layers in the northern OT (N-OT) (Machida, 1999), submarine hydrothermal activity in the middle (M-) and southern OT (S-OT) (Zhai et al., 2001; Zeng et al., 2017; Yang et al., 2021), seafloor earthquakes (Huh et al., 2004) provide siliciclastic sediments into the trough.
Therefore, the origin of sediments in the OT is still unresolved. Whether the Yangtze and/or Yellow River-derived particulate materials can directly reach the OT in the present day or during the Last Glacial Maximum (LGM) remains controversial (Oguri et al., 2003; Dou et al., 2010a). The Kuroshio Current strengthened and its mainstream returned to the OT at about 7 ka (Jian et al., 2000), which likely reduced the sediment transport at the bottom from the continental shelf of the ECS to the trough (Guo et al., 2001). Secondly, in the M-OT, most researchers suggest that sediments are predominated derived from the Yangtze River and ECS shelf before ∼7.0 ka when the sea level was lower, the East Asian winter monsoon was stronger (Katayama and Watanabe, 2003; Dou et al., 2010a; Dou et al., 2010b). However, several recent studies have shown that the Yellow River (Xu et al., 2014; Li et al., 2015) might make contribution to the N-OT and even the M-OT (Wang et al., 2015) during the LGM. Thirdly, river sediments from Taiwan island might be transported into the OT by the Kuroshio Current (Dou et al., 2012; Zheng et al., 2014). However, whether and how far the terrigenous sediments from Taiwan Island can be transported northward into the M-OT and N-OT is a challenging question (Hsu et al., 2004; Xu et al., 2017).
However, these tracer indices have the problem of end-member overlap which often leads to multiple solutions when source identification is performed. The major elements of eastern Taiwan river sediments overlap with the Yangtze River sediments (Hu et al., 2020). There is overlap of rare earth elements in Yangtze River, Yellow River and Taiwan river sediments (Yang et al., 2018; Zhao et al., 2018). The Sr-Nd isotopic compositions of eastern Taiwan river sediments overlap with the Yellow River sediments (Hu et al., 2020). It is difficult to distinguish Sr-Nd isotopes in western Taiwan river sediments from those in northeastern Taiwan rivers sediments (Dou et al., 2016). In addition, illite crystallinity is similar between the Yellow and Yangtze River sediments (Wang et al., 2015). Therefore, the use of these indicators inevitably leads to challenges in accurately defining the source. In this study, detrital zircon chronology was used to identify the sediment sources from the N-OT to the S-OT.
Zircon is a highly stable mineral in nature, characterized by its durability, stable physical and chemical attributes. With a low concentration of common lead, zircon is enriched in U and Th. Its low ionic diffusion rate, high sealing temperature, and minimal late disturbance make it an ideal medium for U-Pb isotope dating (Wang et al., 2013). Recently, researchers have proposed innovative approaches and methodologies utilizing detrital zircons to study the provenance and climatic evolution based on modern river sediments and loess (Thomas et al., 2004; Lease et al., 2007; Xie et al., 2012; He et al., 2013; Deng et al., 2017). However, limited studies have focused on detrital zircons in marine sediments from the OT. Only detrital zircon age of sediments from the S-OT has been previously documented (Zhu and Zeng, 2022).
In order to discern the terrestrial material sources in various OT regions, four surficial sediment samples from the northern, middle, and southern sections of the OT (N-OT: N1; M-OT: M1, and M2; S-OT: S1) were subjected to detrital zircon U-Pb age analysis (Figure 1). In addition, sediments from the potential provenance, Yellow River Estuary, Old Yellow River, Yangtze Estuary, ECS, Lanyang River Estuary, and Zhuoshui River Estuary were introduced to make comparative analysis. This study represents the first comprehensive analysis of the detrital zircon U-Pb age of sediments from N-OT to S-OT. This study introduces the method for tracing marine sediment sources, aiming to gain a better understanding of the terrestrial material sources in various regions of the OT.
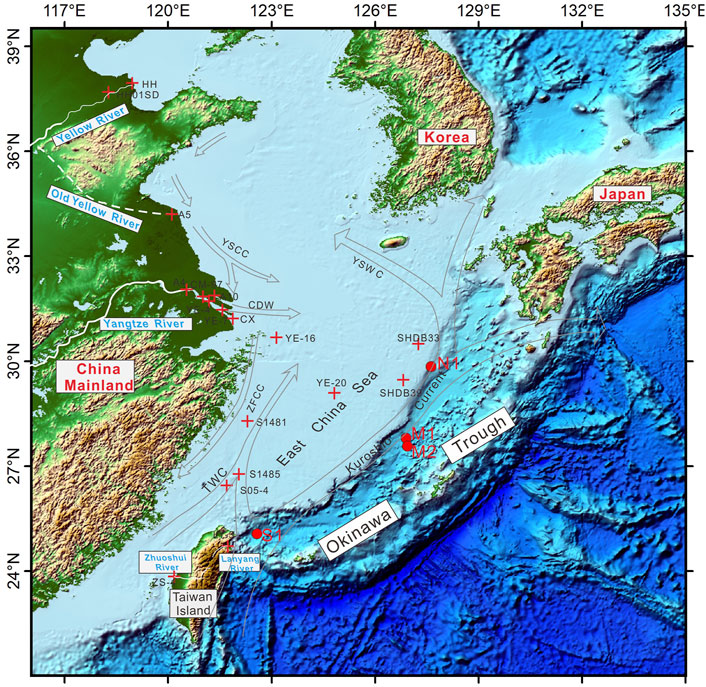
Figure 1. A schematic map illustrating the China mainland, Yellow River, Old Yellow River, Yangtze River, Lanyang River, Zhuoshui River, East China Sea, and the topography of the Okinawa Trough. The Old Yellow River entered the Yellow Sea from 1,128 to 1855 AD (dashed course). Red dots indicate the locations of the sampling sites for the surface sediments from the Okinawa Trough in this study. Red crosses indicate locations of sediments in the potential provenances. References are listed in Supplementary Appendix B. The abbreviations here are ZFCC, Zhenjiang and Fujian Coastal Current; TWC, Taiwan Warm Current; YSCC, Yellow Sea Coastal Current; YSWC, Yellow Sea Warm Current; CDW, Changjiang Diluted Water. The topography of the Okinawa Trough is sourced from Global topographic and geomorphic data (https://www.ncei.noaa.gov/products/etopo-global-relief-model). The oceanic circulations are derived from Li et al. (2015) and Dou et al. (2012).
2 Regional setting
The OT is a back-arc basin in the Northwest Pacific, extending in a NE-SW direction between the Taiwan Province and Kyushu. The trough spans approximately 1,200 km in length and 100–150 km in width, covering a total area of about 140,000 km2. Its topography is characterized by deep depths in the south and shallower depths in the north, with its deepest point reaching up to 2,700 m in the southwest and gradually becoming shallower towards its northern edge at around 800 m. The OT can be divided into three main components: west slope, trough, and east slope. The west slope represents an extension of the ECS shelf on its eastern side. It features significant variations in water depth and complex topography, including numerous raised platforms, deep gullies, and stepped landforms. A submarine canyon-fan system is widely developed on the west slope, with submarine canyons primarily distributed along the slope of M-OT and S-OT. These canyons tend to become increasingly complex from north to south, with their scale gradually expanding (Zhao et al., 2009; Li et al., 2022). On the other hand, the east slope is the Ryukyu’s western island slope. The bottom of the trough is slow. The terrain is complex with some isolated seamounts, raised highlands and depressions.
The OT is situated on the north side of the Taiwan-Luzon collision zone, within the convergence belt of the Pacific Plate and the Eurasian plate. It is also located on the western side of the Ryukyu Trench and Ryukyu volcanic arc. The OT is an active back-arc basin within the trench-arc-basin system of the Northwest Pacific continental margin. (Sibuet et al., 1998). The tectonic activity began in the late Miocene, and the present tectonic pattern was basically established in the early Pleistocene or late Pliocene (Li et al., 1998). In addition to the NNE-trending parallel faults extending along the trough, there are a series of transverse faults that are oblique or orthogonal to the trough in the NW direction. The parallel fault system extends NNE trending in the north section, NE trending in the middle section and EW trending in the south section. The large transverse faults include the Tokala fault zone and the Kerama fault zone, which divide the trough into three sections: the north (N-OT), middle (M-OT), and south OT (S-OT) (Jin and Yu, 1987) (Figure 1).
The OT exhibits a variety of shallow and deep sedimentary environments. Due to its distance from the mainland, the supply of terrigenous materials is limited, and the barrier effect of the Ryukyu Island arc impacts its interaction with the ocean. The presence of turbidity currents and submarine volcanic activities significantly enriches the sediment species within the trough (Pan and Shi, 1986). Another prominent characteristic of the trough sediments is their diverse sources, including terrigenous debris, bioclasts, volcanic debris, and a small amount of authigenic sediments. Terrigenous debris predominates in the trough but gradually diminishes from west to east and from southwest to northeast. Conversely, bioclasts increase notably towards the north and become increasingly prevalent. However, pyroclast distribution is relatively limited and associated with submarine volcanism (Yuan et al., 1987).
The ECS is a vast marginal sea in the northwest Pacific, and its current system is quite complex, which can be generally divided into two broad categories. 1) External current system composed of Kuroshio and its branches; 2) Coastal current system includes Zhenjiang and Fujian Coastal Current (ZFCC), Taiwan Warm Current (TWC), Yellow Sea Coastal Current (YSCC), Yellow Sea Warm Current (YSWC), Changjiang Diluted Water (CDW). According to the temporal and spatial distribution of the current system, from the mainland coast to the Okinawa Trough, there are: the CDW moving northeast in summer; 2) ZFCC, which flows southward along the mainland coast in winter; 3) The TWC moving north along the shelf; 4) Kuroshio flowing northeastward along the edge and slope of the shelf (Dou et al., 2012; Li et al., 2015) (Figure 1).
The study area is situated within the renowned East Asian monsoon region, where the seasonal variation of the monsoon exerts a significant influence on sediment transport in the ECS (Bian et al., 2010). The sediment transport in the ECS exhibits a distinct “storage in summer and transport in winter” pattern under the influence of both monsoons and ocean currents (Yang et al., 1992; Yanagi et al., 1996). During summer, the ECS experiences dominance by a southwest monsoon with speeds of up to 5 m/s, which aligns with both the TWC and the Kuroshio. Furthermore, this monsoon causes a southward shift in the branch point of the Kuroshio, thereby intensifying its flow. As a result, most sediment is prevented from being transported to deeper sea areas during summer, leading to substantial accumulation on the shelf. In winter, the ECS is dominated by the northwest monsoon. On the one hand, the monsoon controlled the location of the Kuroshio branch points in the Bashi Channel, which weakened the Kuroshio (Qu and Lukas, 2003). On the other hand, down currents generated by winter storms allowed sediment to be transported to the OT.
3 Materials and methods
3.1 Surface sediment samples
Four surface sediment samples were collected by the Kexue Yi hao and KEXUE research vessels from the N-, M-, and S-OT regions (Figure 1) between 2013 and 2016 during the HOBAB1-4 cruises. The water depths of the four samples, N1, M1, M2, and S1, were 288 m, 1,039 m, 1,589 m and 1,039 m, respectively, in which N1 was located on the continental slope and the other samples were located in the trough (Table 1). All samples were procured using TV-grabs. The samples, which appear gray, have minimal foraminifer content.
3.2 Grain size analysis
Grain size was analyzed for the bulk sediments. Organic matters, carbonate and silica were removed using 30% H2O2, 1 mol/L dilute hydrochloric acid and 2 mol/L sodium carbonate, respectively. Grain size measurements of the samples were conducted using a laser diffraction particle size analyzer Mastersizer 2000 at Key Laboratory of Marine Geology and Metallogeny, MNR, China. The analytical precision is better than 2%. The moment method by McManus (1988) is used to calculate the mean particle size, sorting coefficient, skewness and kurtosis. The classification method by Folk et al. (1970) was used to name the surface sediments. The results are shown in Table 2.
3.3 Detrital zircon selection
Initially, detrital zircon grains were extracted by conventional heavy liquid separation techniques at Key Laboratory of Marine Geology and Metallogeny, MNR, China. Roughly 200 g of the wet sample was sieved. Subsequently, heavy liquid CHBr3 (specific gravity range d420 = 2.889−2.891) was employed to differentiate between heavy and light minerals. The isolated heavy minerals were then chosen for zircon examination under a stereomicroscope. Given the distance of the OT from the Asian continent, the transported detrital zircon grains in the trough are minuscule. These zircons predominantly fall within a grain size range of 0.032–0.063 mm.
Afterward, the zircon grains were affixed and polished for U-Pb age testing. They were carefully attached to a double-sided adhesive under binoculars, then covered in a mixture of epoxy resin and coagulant. After this application, the samples were set on a hot plate to dry and solidify. They were later polished.
Finally, the zircon grains underwent photography sessions under transmitted and reflected light. A scanning electron microscope facilitated cathodoluminescence examinations, revealing the grains’ internal structure and morphological features. This included the visibility of ring zones, fissures, inclusions, and inheritance nuclei. Suitable locations for dating were then chosen and labeled for laser ablation analysis.
3.4 U-Pb age dating
Detrital zircon U-Pb dating was conducted at the State Key Laboratory of Continental Dynamics, Northwest University. The employed laser ablation system was the GeoLas200M, a product of MicroLas, Germany. The system features a ComPex102 ArF excimer laser (wavelength 193 nm) from Lambda Physik, Germany, paired with an optical configuration devised by MicroLas. The adjustable spot diameter ranges from 4 to 120 μm. The single pulse’s energy is 200 MJ, and the highest repetition rate reaches 20 Hz, with an average power of 4 W. When adjusted and focused by the optical system, the energy density reaches 20 J/cm2. The ICP-MS used is the Agilent 7500a, produced by Agilent, United States.
Both the zircon samples and the standard materials (zircons 91,500 and GJ-1) along with artificial silicate glass standard NIST SRM 610 were attached to a double-sided adhesive. These were then secured with a clear epoxy resin and polished until a flat surface was exposed. Before measurement, the sample surface underwent cleaning with 3% (v/v) HNO3 to eliminate contamination.
The laser beam chosen for laser ablation had a diameter of 24 μm and a frequency of 10 Hz. The chosen sampling method was single-point erosion, utilizing helium as the erosion material. The backgrounds for 204Pb and 202Hg are below 100 cps due to the pure quality of the Ar and He gases. Dwell times were set at 15 ms (for isotopes 204Pb, 206Pb, 207Pb, and 208Pb) and 10 ms (for Th and U). Standard materials zircons 91,500 (1,057 Ma), GJ-1 (600 Ma) and silicate glass NIST SRM 610 were measured after every five sample points. Each sample point had a gas background acquisition time of 30 s and a signal acquisition period of 40 s.
The raw ICP-MS data were exported and processed using Glitter (version 4.0, Macquarie University) (Achterberg et al., 2001) software. Isotope ratios and ages were corrected through replicate analyses of standard zircons 91,500 and GJ-1. 1% error (1σ) is assigned to the isotope ratios for the standard. Common Pb correction was calculated following the method of Andersen (2002). Element content calculations used NIST SRM 610 as the external standard, with Si as the internal standard. The average age computation and related graphical representations for each sample were done using Isoplot (version 2.49) (Ludwig, 2001). According to Compston et al. (1992) 206Pb/238U ages are used for zircons younger than 1.0 Ga, while 207Pb/206Pb ages were used for zircons older than 1.0 Ga.
4 Results
4.1 The morphological characteristics of zircon grains from the Okinawa Trough
The grain size of N1 is relatively coarse compared to the other samples, which are more uniformly fine. Grain size analysis reveals that N1 is silty sand, and M1, M2, S1 are sandy silt, silt, and sandy silt, respectively (Table 2). The majority of zircon grains in sample N1 are relatively large, measuring 40–150 μm long and 30–100 μm wide. In contrast, most zircon grains in sample M1 and M2 are rounded and fragmented, with dimensions of 40–100 μm long and 30–50 μm wide. Similarly, the majority of zircon grains in sample S1 are also rounded with measurements of 40–120 μm long and 30–80 μm wide (Figure 2). The detrital zircons from the four surface sediment samples in this study predominantly exhibit a round-circular shape, suggesting that these zircon grains have experienced long-distance transportation. A minor proportion of the zircons retains a complete crystal form, implying their derivation from proximal volcanic rock masses. Cathodoluminescence (CL) images reveal that a majority of the detrital zircons display pronounced oscillatory zoning, while a limited number exhibit absent or weak zoning, indicative of their igneous origin (Figure 2). The Th/U ratio of zircons serves as an estimator of their origin: igneous zircons typically present Th/U ratios >0.3, whereas metamorphic zircons show Th/U ratios <0.1. In this research, most zircons exhibited Th/U ratios >0.3, and only a few displayed Th/U ratios <0.1 (Figure 3), supporting the assertion that most zircons have an igneous origin, consistent with the CL findings.
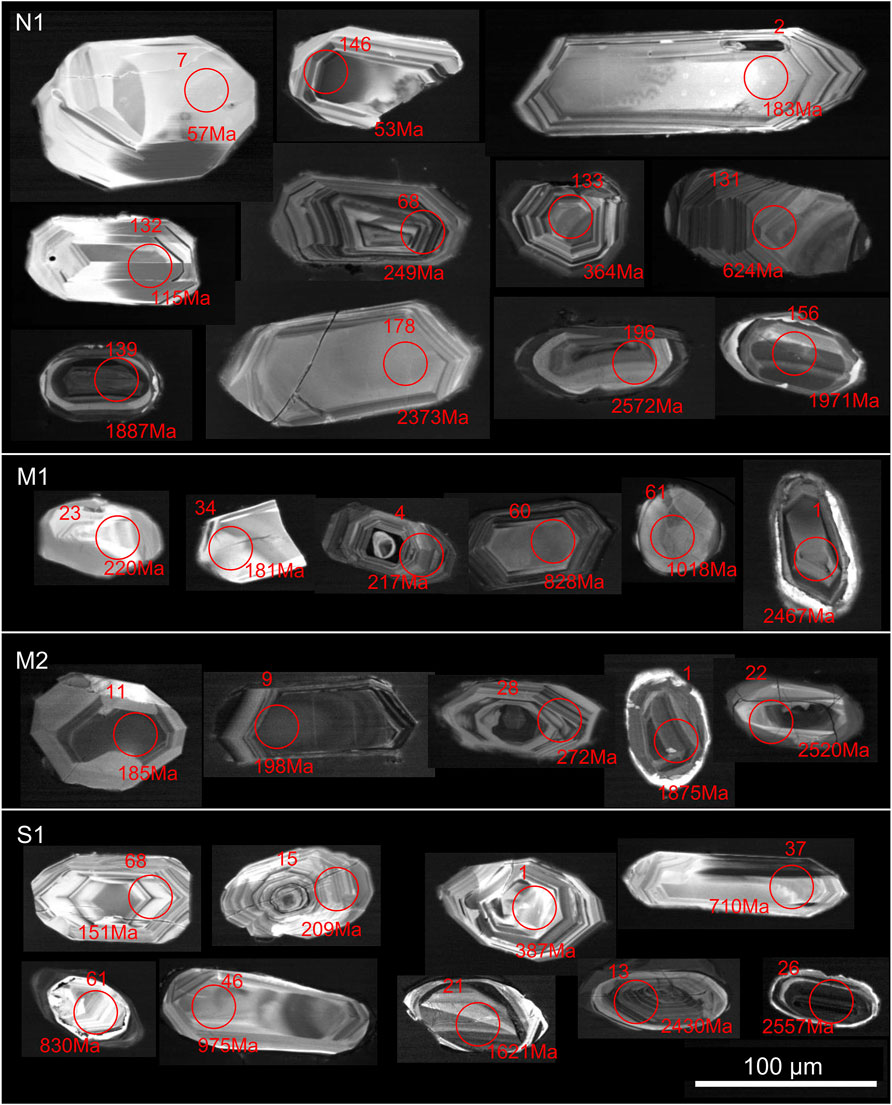
Figure 2. Cathodoluminescence images of the detrital zircons in the sediments from the Okinawa Trough.
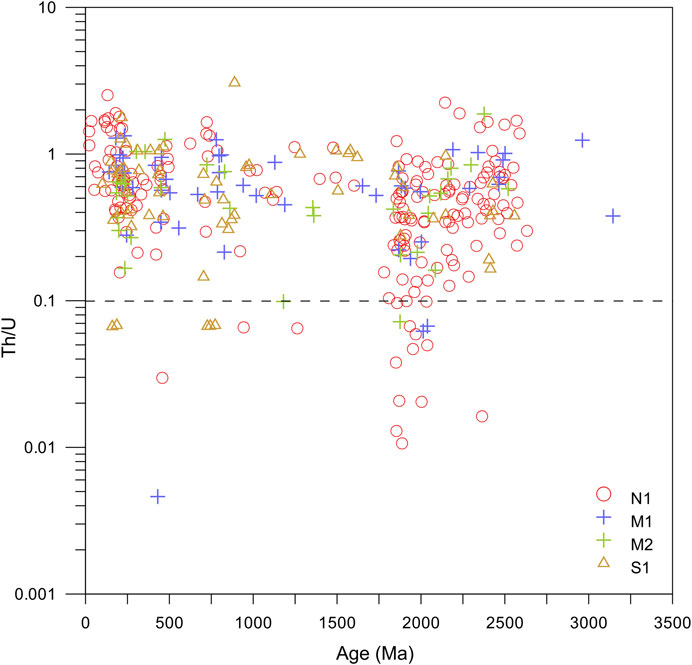
Figure 3. A plot representing Th/U ratios versus U-Pb ages of concordant detrital zircons from the Okinawa Trough sediments.
4.2 U–Pb geochronology of the sediment samples from the Okinawa Trough
Zircon U-Pb age data points with a concordance >90% were selected for further analysis. A total of 342 concordant age data points were identified, with sample N1 contributing 196 points, M1 providing 50 points, M2 accounting for 33 points, and S1 contributing 63 points (Table 1). The U-Pb age data are given in Supplementary Appendix A.
Based on the detrital zircon U-Pb age distribution, the detrital zircons of the sediment samples from the OT can be categorized into six groups: <100 Ma (∼Cenozoic); 100−250 (∼Mesozoic); 250−600 (∼Paleozoic); 600−1,000 (∼Neoproterozoic); 1,000−2,300 (∼Mesozoic-Paleozoic); and >2,300 Ma (∼Archeozoic) zircons (Table 3). The U-Pb age distributions are depicted in the Kernel density estimation (KDE) plots (Figure 4). The age population for N1 presents four prominent peaks at approximately 209, 1883, 2,170, and 2,400 Ma. Detrital zircon U-Pb ages of M1, M2 and S1 fall into the last five age groups. M1 showcases age peaks at approximately 209, 439, 783, 1932, 2,473, and 3,056 Ma, while M2 displays age peaks at about 217, 824, 1,333, and 2088 Ma. Age peaks for S1 are observed at approximately 209, 430, 775, 1,547, 1875, 2,137, and 2,408 Ma (Table 4). These age groups and age peaks correspond to the significant tectonic-magmatic events in China mainland (Deng et al., 2017, and references therein), including the Yanshanian Orogeny (YS, 180−90 Ma), Indosinian Orogeny (IS, 270−195 Ma), (WY, 465−415 Ma), Jinningian Orogeny (JN, 1,000−750 Ma), Lüliangian Orogeny (LL, 2,400−1800 Ma), Fuping-Wutai Orogeny (FW, 3,000−2,400 Ma) (Figure 4).
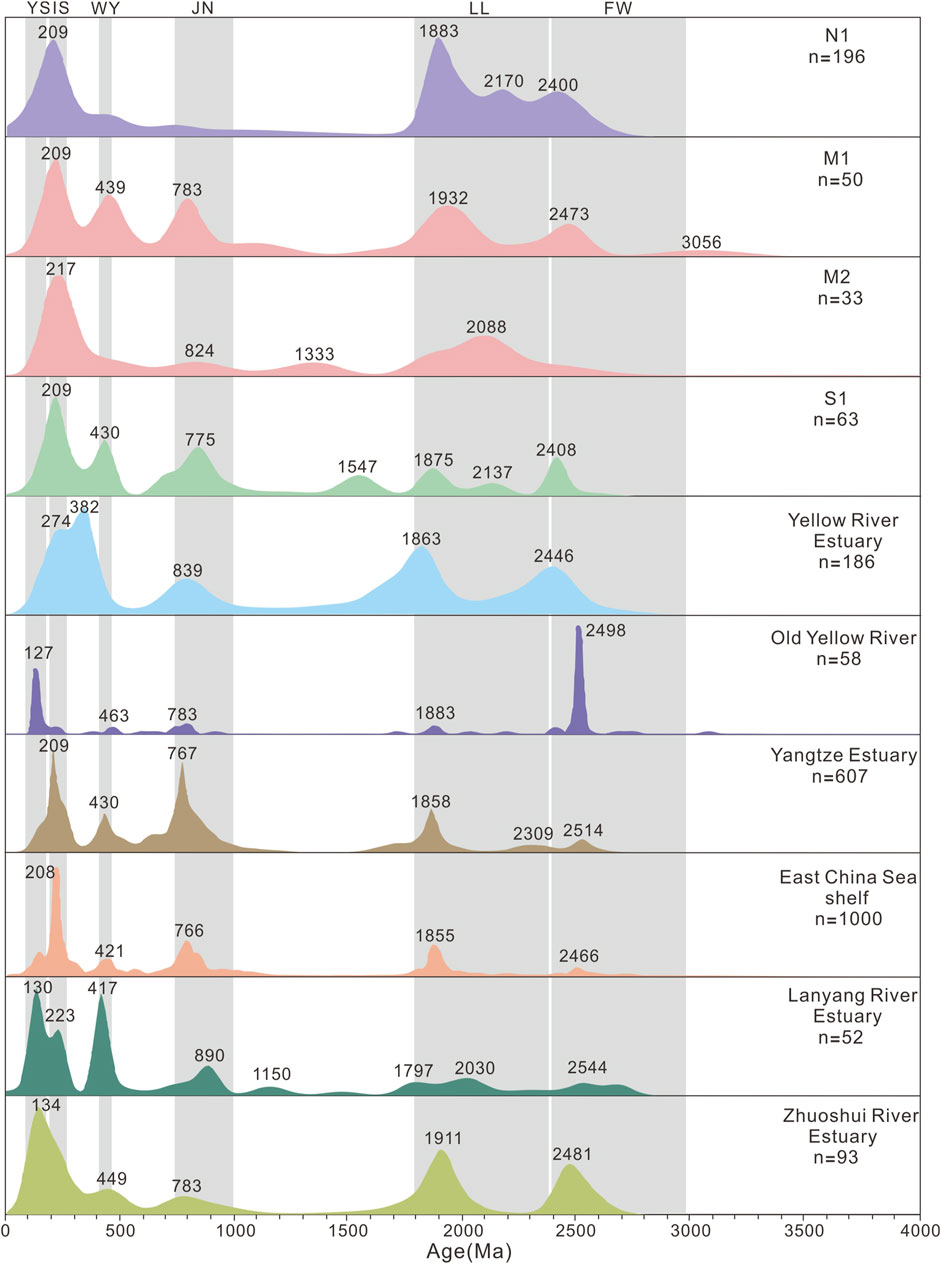
Figure 4. Kernel density estimation (KDE) plots presenting the zircon U-Pb ages of sediments from the Okinawa Trough in this study, in comparison to those from the Yellow River Estuary (Yang et al., 2009; Zheng et al., 2013), Old Yellow River (Su et al., 2018), Yangtze Estuary (He et al., 2013; Huang et al., 2020; Su et al., 2018), East China Sea shelf (Huang et al., 2020), Lanyang River Estuary (Deng et al., 2017), and Zhuoshui River Estuary (Deng et al., 2017). The gray shaded bars highlight tectonic-magmatic events in China mainland (Deng et al., 2017, and references therein). The abbreviations here are YS, Yanshanian Orogeny (180−90 Ma); IS, Indosinian Orogeny (270−195 Ma); WY, Wuyi-Yunkai Orogeny (465−415 Ma); JN, Jinningian Orogeny (1,000−750 Ma); LL, Lüliangian Orogeny (2,400−1800 Ma); FW, Fuping-Wutai Orogeny (3,000−2,400 Ma).
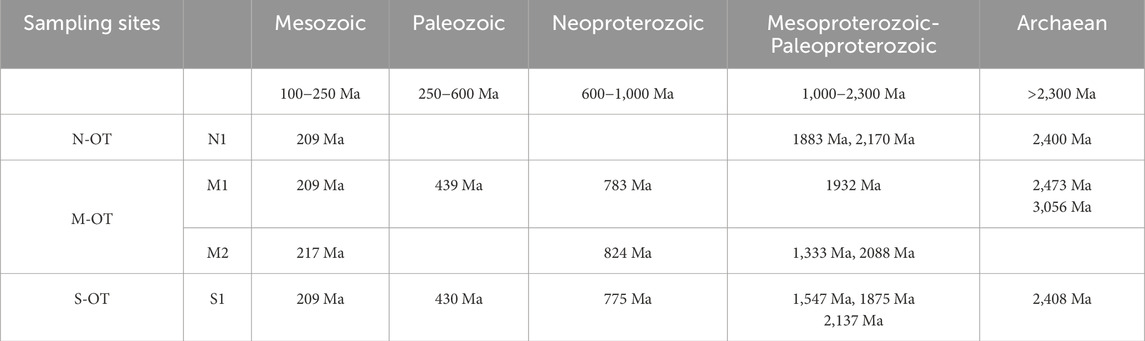
Table 4. Approximated age peaks of detrital zircons in the surface sediments from the Okinawa Trough.
Pie charts are also introduced in Figure 5 to depict the percentage for different age groups. In sample N1, age group 1,000–2,300 Ma has the highest proportion (45%), followed by age group 100−600 Ma (30%), and age group 600−1,000 Ma has a significantly lower proportion (6%). In sample M1, the proportion of age group 100−600 Ma and 1,000−2,300 Ma is similar (38% and 32%), followed by age groups of 600−1,000 Ma (16%). In sample M2, the proportion of age group 100−600 Ma and 1,000−2,300 Ma is higher than those of M1. In sample S1, age group100−600 Ma has the highest proportion (43%), followed by age groups 600−1,000, 1,000−2,300 Ma with same percentage of 24%.
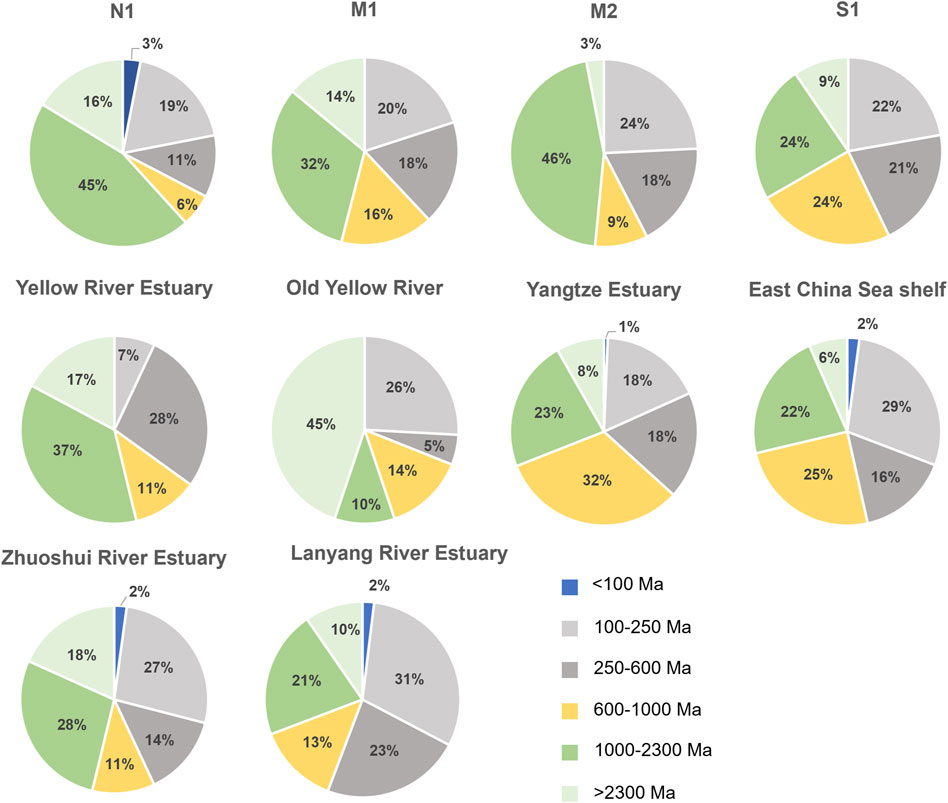
Figure 5. Pie charts depicting detrital zircon U-Pb ages of sediment samples from the Okinawa Trough and from the potential provenances.
By comparing the detrital zircon U-Pb age group among N-OT, M-OT, and S-OT, the U-Pb age for N1 is mainly focusing on the Paleoproterozoic and Mesoproterozoic (1,000−2,300 Ma, 45%) and Mesozoic-Paleozoic (100−600 Ma, 30%). However, in the M-OT, the percentage for the Mesozoic and Paleozoic zircons (100−600 Ma, 38%−42%) and Neoproterozoic zircons (600−1,000 Ma, 6%−16%) are considerably increased. The age distribution for S1 is similar to that for M1. However, in the S-OT, the percentage for the age group 1,000−2,300 Ma is reduced, while that for the age group 600−1,000 Ma is considerably increased.
5 Discussion
5.1 Previous studies: U–Pb geochronology of the potential provenances
U-Pb age distributions of detrital zircons from the potential provenances are listed in Supplementary Appendix B and are shown in Figure 4. U-Pb age of detrital zircons from the Yellow River Estuary reveals four major age groups: 200−500, 500−1,300, 1,400−2,300, and >2,300 Ma, with age peaks at approximately 274, 382, 839, 1863, and 2,446 Ma (Yang et al., 2009; Zheng et al., 2013). U-Pb age of detrital zircons from the Old Yellow River is mainly focused in two age groups: 100–200 Ma and 2,400–2,600 Ma, with peaks at ages of 127, 2,498 Ma (Su et al., 2018). U-Pb age of detrital zircons from the Yangtze Estuary exhibits five major age groups: 100−300, 400−550, 700−1,000, 1800−2000, and >2,300 Ma, with peaks around ages of 209, 430, 767, 1858, and 2,514 Ma (He et al., 2013; Su et al., 2018; Huang et al., 2020). Detrital zircons U-Pb age distribution of the ECS shelf is similar to that of the Yangtze Estuary, presenting five major age groups with peaks at ages of 208, 421, 766, 1855, and 2,466 Ma (Huang et al., 2020). U-Pb age of detrital zircons from the Lanyang River Estuary shows five major age groups: 100−300, 300−550, 700−900, 1700−2,300, and 2,400−2,600 Ma. The sediments in this river present a relatively focused zircon age distribution, dominated by Phanerozoic zircons with distinct peaks at ages of 130, 223, and 417 Ma (Deng et al., 2017). U-Pb age of detrital zircons from the Zhuoshui River presents five major age groups: 100−300, 300−550, 700−900, 1700−2000, and 2,400−2,600 Ma. The peaks are at ages about 134, 449, 783, 1911, and 2,481 Ma (Deng et al., 2017).
Pie charts are also presented in Figure 5 to depict the age percentage for the potential provenances. The detrital zircons for the Yellow River sediments show high percentage in age groups 100−600 Ma (35%) and 1,000−2,300 Ma (37%), and low percentage in age group 600−1,000 Ma (11%). The detrital zircon U-Pb age distribution is similar to the Yangtze River and ECS shelf. The detrital zircon U-Pb age of sediments from the Yangtze Estuary and ECS shelf are with the characteristics of high percentage in age groups 100–600 Ma (36−45%) and 600–1,000 Ma (25−32%), and low percentage in age group 1,000−2,300 Ma (22−23%), which are different from the Yellow River sediments. The detrital zircon U-Pb age distribution of the Lanyang River and Zhuoshui River is obviously different. The detrital zircons for Zhuoshui River sediments show higher percentage in older age groups 1,000−2,300 Ma and >2,300 Ma, and lower percentage in younger age group 100−600 Ma than those of Lanyang River. More than half of the detrital zircons in the Lanyang River sediments are younger Mesozoic-Paleozoic (100−600 Ma, 54%) zircons. The age distribution of Zhuoshui River sediments is similar to that of the Yellow River sediments with high percentage in age groups 100−600, 1,000−2,300 Ma and low percentage in age group 600−1,000 Ma. The Old Yellow River sediments are characterized by significantly higher percentage of age groups 100−600 Ma and >2,300 Ma.
5.2 Identification for sedimentary provenances in the Okinawa Trough
In the N-OT, the detrital zircon U-Pb age characteristics of sample N1 show a high proportion in the age group 1,000−2,300 Ma, and a low proportion in age group 600−1,000 Ma. KDE plots show that the age peaks of approximately 209, 1883, and 2,400 Ma match those of the Yellow River sediments (Figure 4). However, N1 exhibits lower age peaks of approximately 431 and 775 Ma, which are predominant in the Yellow River sediments (Figure 4). Pie charts also show the percentage of each age group in N1 compared to that in the Yellow River sediments. However, the proportion of age groups 100−600 Ma and 600−1,000 Ma in N1 is slightly lower than that in the Yellow River sediments. This indicates the contribution of other potential sources to the N-OT. The Yangtze River and ECS shelf sediments have a high age peak of 766/767 Ma, and high percentage of age group 600−1,000 Ma which is obviously different from the age distribution of sample N1. The detrital zircon U-Pb age for Lanyang River sediments show high percentage in age group 100−600 Ma and low percentage in age group 1,000−2,300 Ma which is also different from the age distribution of sample N1. Therefore, the contribution of Yangtze River, ECS shelf, and Lanyang River sediments to sample N1 may be small. Although the KDE plot can represent the occurrence and duration of geological events, it is difficult to visualize the proportions in different age groups. Therefore, the cumulative probability plots (CPPs) are introduced in this study to highlight differences in proportions of similar ages across various age spectrums (Gehrels, 2011). By comparing the CPPs of zircon age between OT and potential river sediments (Figure 6), the CPPs show that N1 lies between the Yellow River, Old Yellow River and Zhuoshui River sediments, and away from the Yangtze River sediments. This suggests that the Yellow River, Old Yellow River and Zhuoshui River may be the potential sources of detrital zircons in the N-OT, while the contribution of the Yangtze River may be small. The percentage of each age group of M1, M2 and these potential sources can also provide evidence. The Yellow River sediments show relatively lower proportion of age group 100−250 Ma, while the Old Yellow River and Zhuoshui River sediments are just the opposite. Therefore, the higher proportion of age group 100−250 Ma in N1 may be due to a mixture from the Yellow River and Old Yellow River sediments. And Zhuoshui River can be another potential source for N1. Zhao et al. (2018) reported that sediments in the N-OT were mainly supplied by the Yellow River middle reaches at ∼34‒8 ka. The low sea level and proximity of paleo-Yellow River mouth to the N-OT were the causes of the dominant Yellow River input. After ∼8 ka, a retreated Yellow River mouth coupled with the blocking effect of the Kuroshio Current and its branches resulted in relatively strong sediment input from Yellow River upper reaches. Xu et al. (2014) reported since 7.3 kyr BP, Taiwan materials might have been transported northward to the N-OT by the fully evolved Tsushima Warm Current, characterized by higher chlorite/kaolinite ratios. The lower reaches of the Yellow River change frequently, alternately feeding into the Bohai Sea and the Yellow Sea, the most recent enter the Yellow Sea in 1,128−1855. The Old Yellow River enters the sea in the northern part of Jiangsu Province and rapidly advances to the sea at its estuary, which provides a large amount of sediment into the Yellow Sea (Xue et al., 2003; Xue et al., 2004). Therefore, the Old Yellow River can be a potential source of the N-OT. The U-Pb age group less than 100 Ma (21.6−75.2 Ma) is only found in sample N1 from the N-OT, and this age group’s percentage is minimal, constituting approximately 3%. The OT represents a back-arc basin associated with plate subduction since the late Miocene (9−6 Ma), and its depositional evolution can be categorized into three stages: stage I from the late Miocene (9−6 Ma) to the late Pliocene (2 Ma); stage II from the late Pliocene (2 Ma) to the late Pleistocene (130 ka); and stage III from the Pleistocene to the present (Li et al., 2004). The youngest zircon in sample N1 (21.6 Ma) predates the basement rocks of the OT, indicating that the Cenozoic zircons in sample N1 did not originate from the basement of OT and the Ryukyu Arc. When compared to the Yellow River, Old Yellow River, and Zhuoshui River sediments, detrital zircons from the Zhuoshui River show a small proportion of age group less than 100 Ma (2%) which is more likely the source for the detrital zircons with age group of less than 100 Ma in the N-OT. Therefore, as discussed above, the detrital zircons in N1 may be the mixture by the Yellow River and Old Yellow River sediments. And Zhuoshui River may be a potential source for N1. In addition, there are other potential sources for detrital zircons with age group less than 100 Ma in N1. The Korean Peninsula is mountainous, with the terrain gradually decreasing from northeast to southwest. Most rivers in the Korean Peninsula flow west and south into the Yellow Sea and provide sediments for the Yellow Sea (Choi et al., 2016; Huang et al., 2020). U-Pb analyses on the detrital zircons from modern river sediments in South Korea show that zircon ages range from 3,566 to 48 Ma with predominance of Mesozoic (249–79 Ma) and Paleoproterozoic (2,491–1,691 Ma). The age distribution is similar to N1. In particular, the younger zircons from modern river sediments in South Korea may be the potential source for detrital zircons with age group less than 100 Ma in sample N1. However, it is worth noting that the annual sediment discharge of rivers in the Korean Peninsula is around 20 Mt (Yang et al., 2003), which is equivalent to that of the Zhe-Min rivers at 23 Mt (Zhang and Liu, 2002), indicating that they are relatively less important compared to Taiwan (300 Mt) (Dadson et al., 2003) and Yangtze River (about 500 Mt) (Yang et al., 2005). This suggests that while rivers in the Korean Peninsula may provide some sediment to OT, they are not its main source.
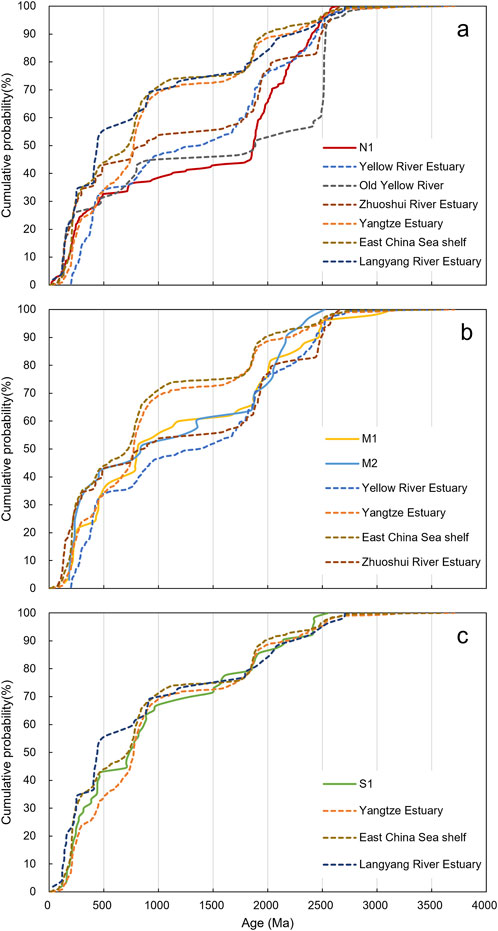
Figure 6. Cumulative probability plots highlighting the zircon U-Pb ages of sediment samples (A) N1, (B) M1, M2, and (C) S1 from the Okinawa Trough in this study and from the potential provenances.
In the M-OT, compared to N1, the detrital zircon U-Pb ages of M1, M2 exhibit an obviously higher proportion of age group 600−1,000 Ma, lower proportion of age groups 1,000−2,300 Ma and >2,300 Ma. The age peaks, especially M1 are similar to those of the Yangtze River and ECS shelf, with significant peaks at ∼ 420−440 Ma and ∼760−790 Ma. However, pie charts show that M1, M2 display higher proportion in the age group 1,000−2,300 Ma and lower proportion in the age group 600–1,000 Ma than those of Yangtze River and ECS shelf (Figure 5), which indicates the contribution of other potential sources to the M-OT. The CPPs show that M1 and M2 are intermediate between the Yellow River, Yangtze River, and ECS shelf sediments. Meanwhile, the CPPs show that M1 and M2 are more similar to the Zhuoshui River sediments and farther away from the Lanyang River sediments. This suggests that the detrital zircons from M1 and M2 are a mixture of the Yellow River and the Yangtze River, and perhaps the Zhuoshui River, while the contribution from the Lanyang River is less. The percentage of each age group of M1, M2 and these potential sources can also provide evidence. The detrital zircon U-Pb ages of Yangtze River and ECS shelf sediments are characterized by higher proportion in the age group 600−1,000 Ma and slightly lower proportion in the age group of 1,000−2,300 Ma, while the age distribution of Yellow River is just the opposite. Therefore, compared with N1, the slightly higher percentage of age group 600−1,000 Ma and lower percentage of age group 1,000−2,300 Ma may be a mixture from the Yangtze River, ECS shelf, and the Yellow River sediments. Moreover, the U-Pb age distribution and percentage of each age group of Zhuoshui River sediments are more similar to the those of M1 and M2. Therefore, in addition to the Yangtze River, ECS shelf and Yellow River, Zhuoshui River is probably another important source in the M-OT.
KDE plots show that the age peaks of S1 approximately 209, 430, 775, 1875, and 2,408 Ma are similar to the age peaks of the Yangtze River and ECS shelf (Figure 4). Meanwhile, pie charts display similar percentage of each age group between S1 and Yangtze River, ECS shelf sediments (Figure 5). The differences are that the proportion of age group 600−1,000 Ma is relatively lower, and the proportion of age group 250−600 Ma is higher which indicates another potential source may transport to the S-OT. CCP plots show that S1 lies between the Yangtze River, ECS shelf and Lanyang River, more closely aligning with the Yangtze River and ECS shelf sediments. The Lanyang River shows lower age group 600−1,000 Ma and higher age group 250−600 Ma which maybe another source of S1. This indicates that detrital zircons in the S1 are mainly derived from the Yangtze River and ECS shelf. Secondly, the Lanyang River may also have a small contribution to S1. This is consistent with the previous study that the detrital zircons from the southern Okinawa Trough are dominated from the ECS shelf, Yangtze River and a small amount from the Lanyang River (Zhu and Zeng, 2022).
5.3 Indication for transport and sedimentation in the Okinawa Trough
Detrital zircons from the N-OT are primarily from the Yellow River and mixed with the Old Yellow River. In contrast, detrital zircons from the M-OT are mainly from the Yangtze River, ECS shelf and Yellow River. Moreover, it is possible that the Zhuoshui River in the western Taiwan may also be a potential source for both the M-OT and N-OT. Detrital zircons from the S-OT are predominantly from the ECS shelf, Yangtze River with some influence from the Lanyang River.
Regarding sample depths, N1 was located on the continental slope at a depth of 288 m, while samples M1, M2, and S1 were situated in trough at depths of 1,039 m, and 1,222 m respectively (Table 1).
Based on the dating results of outer ECS shelf and recent studies (Chen, 2008; Wang et al., 2012), it is indicated that the coarse-grained sediments found in the outer continental shelf are not modern sediments, but rather formed during the low sea level period of the last glaciation. This resulted in an outward extension of the ancient Yangtze River estuary, leading to a significant deposition of sediment on the outer shelf. Subsequently, as sea levels rose, the Yangtze River estuary retreated and was continuously affected by the newly formed Taiwan Warm Current and Kuroshio Current. These processes caused difficulties in depositing minerals with low density and modern fine-grained sediments (Zou et al., 2021).
The grain size of N1 is comparatively coarse, whereas the other samples are more uniformly fine. Grain size analysis reveals that N1 is silty sand, and M1, M2, S1 are sandy silt, silt, and sandy silt, respectively (Table 2). The majority of zircon grains in sample N1 are relatively large, ranging from 40 to 150 μm long and 30–100 μm wide. In contrast, most zircon grains in samples M1 and M2 are rounded and fragmented, measuring 40–100 μm long and 30–50 μm wide. Similarly, the majority of zircon grains in sample S1 are also rounded with dimensions of 40–120 μm long and 30–80 μm wide (Figure 2). The grain sizes of these zircons are coarse, and the sampling position of some bulk sediment sample are near the outer continental shelf. This indicates that these zircon grains in the OT are probably not modern deposits, but rather products from the low sea level period during the last glaciation mixed with modern sediments.
The transport and sedimentation process in the OT reflects the comprehensive influence of sea level changes, Kuroshio evolution, river input, East Asian monsoon and other factors. Fluctuations in sea level result in shifts in coastlines and estuaries, while the intensity of the Kuroshio significantly impacts material input from Taiwan. Additionally, monsoon rainfall plays a crucial role in influencing river runoff. The combined effect of all these factors ultimately leads to provenance changes within the OT.
During the period of 16–10 kyr BP, the estuaries of the Ancient Yangtze River and the Yellow River were in closer proximity to the OT when the sea level was low. This allowed for more sediments derived from them to be transported to the OT (Meng et al., 2007). As sea levels rose, it is believed that the tidal current of the ECS shelf was stronger than it is today (Uehara and Saito, 2003), leading to potential erosion and transportation of materials from the shelf to the OT (Dou et al., 2010b; Xu et al., 2017). During deglaciation, the deposition rate of the M-OT reached 40∼50 cm/ka due to input from both the Yangtze River and the ECS shelf, which was higher than that in the Holocene.
Therefore, most of the detrital zircons in the N-OT and M-OT originate from the Yellow River and the Yangtze River. These zircons are believed to have been directly transported to the continental slope and trough during the low sea level of the last glaciation.
With the rise of sea levels during the Holocene period, the formation of the Taiwan Warm Current and the strengthening of the Kuroshio, along with the influence of East Asian monsoon, significantly impacted the input of modern continental sources into the Trough (Guo et al., 2001). Based on modern observations and sediment capture studies, it is observed that in summer, the ECS is influenced by the southeast monsoon. During this time, the direction of the monsoon aligns with that of both the Kuroshio and Taiwan Warm Current. The water barrier effect of these currents prevents sediments from rivers such as Yangtze River and Yellow River, as well as from ECS shelf to transport to the OT, resulting in most sediments accumulating in estuaries and the ECS shelf (Yanagi et al., 1996; Iseki et al., 2003; Liu J. P. et al., 2007). In winter, the ECS is controlled by the northeast monsoon and its storm surge (Yuan et al., 2008). During this season, northeast monsoons move opposite to that of Kuroshio and Taiwan Warm Current. As a result, there is a weakening or disappearance in water barrier effect caused by Kuroshio (Yang et al., 1992). Additionally, since winter monsoons dictates location branch points for Kuroshio current, it also influences the intensity of the Kuroshio (Qu and Lukas, 2003). Consequently, sediments accumulated in continental shelf and estuary during summer can be transported to the trough through bottom nepheloid layer or upper water body (Iseki et al., 2003; Oguri et al., 2003).
In addition, numerous canyons develop on the western slope of the OT. The head of the canyons begins at an isobaric position of approximately −120 m on the shelf, while the tail of the canyons extends to a water depth of up to 1800 m (Zhao et al., 2009; Li et al., 2022). Previous research has demonstrated that the valley serves as a important channel connecting the shelf and trough, with its deposition rate being significantly higher than that of adjacent slope deposition (Hung et al., 1999). The combination of stronger winter monsoon and weaker Kuroshio facilitates sediment transport from the ECS shelf to the trough along the continental slope or canyon.
Overall, since the Holocene, the sediment transport capacity from the Yangtze River and the ECS shelf to the trough has been weakened in summer due to the water barrier effect of the Kuroshio and Taiwan warm current. However, they are still able to transport materials to the trough in winter under the influence of wind monsoon and canyon passage. Additionally, with the strengthening of the Kuroshio, there is an enhanced transport capacity of materials from Taiwan to the trough. Recent studies have indicated that a significant amount of material from Taiwan can be carried by Kuroshio and deposited in the S-OT (Wang et al., 2015; Dou et al., 2016; Chen et al., 2017), and even reach as far as middle and northern parts of OT (Dou et al., 2010a; Wang et al., 2015; Yang et al., 2018).
In this study, the detrital zircons from the N-OT are primarily sourced from the Yellow River and are mixed with those from the Old Yellow River. As for the detrital zircons from the M-OT, they mainly originate from the Yangtze River, ECS shelf, and Yellow River. Additionally, it is possible that the Zhuoshui River in western Taiwan Island could serve as a potential source for both the M-OT and N-OT.
The Zhuoshui River in western Taiwan has a high sediment transport capacity (Kao and Milliman, 2008). Research indicates that the majority of sediments carried by the Zhuoshui River since 10 kyr BP have been deposited in the Taiwan Strait, forming Clinoform, as well as in the coastal plain extending northward from the estuary (Liu et al., 2008). The Taiwan Warm Current has the ability to transport sediments from the western river northward to the ECS (Uehara and Saito, 2003). During winter, a combination of coastal current and weaker Kuroshio may potentially transport Taiwan’s western river sediments to the middle and even northern trough. Additionally, there is a possibility of subsurface sediment gravity flow transport during summer. The strong waves generated by tropical storms such as powerful typhoons during summer can result in re-suspension of continental bottom materials, leading to gravity flow down the continental slope. The local cold water on the shelf is very low, which may sink to sea bottom, be transported through Kuroshio water from bottom to deep sea level, and also bring suspension, that is the “leakage” phenomenon (Yang et al., 1992).
Therefore, whether in winter or summer, it is likely that river sediments from western Taiwan, which are stored on the ECS shelf, will be re-transported to the M-OT or even N-OT. This phenomenon serves as a key factor contributing to the presence of river materials from western Taiwan in the northern and middle regions of the Okinawa Trough, as observed in this study.
The Lanyang estuary in the northeast corner of Taiwan directly leads to the OT. The river experiences large annual rainfall and a high rate of erosion, resulting in an annual runoff of the Lanyang River reaching 2,773 m3 × 106 m3 and carrying about 191 Mt of materials to the ECS every year. Most of these materials are deposited on the Yilan shelf and the southern part of the OT. Additionally, a significant portion of sediment deposited on the shelf is transported to the OT through late hydrodynamic transport. Hsu et al. (2004) demonstrated a positive correlation between Lanyang River runoff and sediment flux on continental slope and trough, indicating its contribution to the trough. Furthermore, due to obstruction on the northern slope of OT, Kuroshio current deflects after entering OT and forms a counterclockwise vortex over Cotton Canyon and North Cotton Canyon. This vortex carries materials from northeastern Taiwan and ECS shelf down into the trough, potentially leading to extremely thick sedimentary layers (1–3 km) with an alarming sedimentation rate (0.18–0.87 cm/a) in the S-OT. However, it is noted that such a high sedimentation rate cannot be solely attributed to Lanyang River alone as Yangtze River also plays a crucial role as an important sediment supplier to ECS by injecting 478 Mt of sediment into ECS annually. However, the high sedimentation rate provided by the Lanyang River alone is insufficient. The Yangtze River plays a crucial role as an important sediment supplier to the ECS, providing 478 Mt of sediment into the ECS annually. Approximately 40% of this sediment is deposited on the inner shelf and estuary, while the remainder is carried by coastal currents and strong winter downdraft to the southern trough (Zheng et al., 2014). This also explains why detrital zircons in the S-OT in this study are primarily derived from the ECS shelf and are influenced by materials from the Lanyang River.
6 Conclusion
The detrital zircon U-Pb age for sample N1 from the N-OT are mainly concentrated in the Paleoproterozoic-Mesoproterozoic age groups (1,000−2,300 Ma, 45%) and Mesozoic-Paleozoic age groups (100−600 Ma, 30%). However, in samples M1 and M2 from the M-OT, the percentage of the Mesozoic and Paleozoic zircons (100−600 Ma, 38%−42%) and Neoproterozoic zircons (600−1,000 Ma, 6%−16%) has considerably increased. The age distribution for S1 is similar to that of M1. However, in the S-OT, the percentage of the age group 1,000−2,300 Ma has reduced, while the percentage of the age group 600−1,000 Ma has considerably increased.
Comparative analysis between the surface sediments and potential provenances using kernel density estimation plots, age peaks of each group, pie charts, and cumulative probability plots shows that detrital zircons from the N-OT are mainly sourced from the Yellow River and mixed with the Old Yellow River. Detrital zircons from the M-OT are mainly derived from the Yangtze River, ECS shelf and Yellow River. In addition, the Zhuoshui River from the western Taiwan island maybe a potential source for the M-OT and even for the N-OT. Detrital zircons in the S-OT are predominantly derived from the ECS shelf and Yangtze River, with some influence from the Lanyang River.
Most of the zircon grain are coarse, with grain sizes of 40–150 μm long and 30–100 μm wide in the N-OT, smaller in the M-OT and S-OT in this study, which are probably not modern deposits, but the products in the low sea level period of last glaciation mixed with modern sediments. The transport and sedimentation processes reflect the comprehensive influence of sea level change, Kuroshio, river input, East Asian monsoon and other factors from last glaciation to present.
Data availability statement
The original contributions presented in the study are included in the article/Supplementary Material, further inquiries can be directed to the corresponding author.
Author contributions
B-JY: Investigation, Writing–original draft, Writing–review and editing. J-HL: Funding acquisition, Writing–review and editing, Resources. Y-HW: Project administration, Writing–review and editing, Investigation.
Funding
The author(s) declare that financial support was received for the research, authorship, and/or publication of this article. This research project was financially supported by the National Natural Science Foundation of China (No. 42106080), the Laboratory for Marine Geology, the China Ocean Mineral Resources R&D Association project (No. DY135-S2-2–03), Shandong Province Natural Science Foundation (No. ZR2020QD074), Ludong University for introduce talents research start-up funding project.
Acknowledgments
Thanks to all the crew for their hard work during the sampling processes in HOBAB1-4 voyages.
Conflict of interest
The authors declare that the research was conducted in the absence of any commercial or financial relationships that could be construed as a potential conflict of interest.
Publisher’s note
All claims expressed in this article are solely those of the authors and do not necessarily represent those of their affiliated organizations, or those of the publisher, the editors and the reviewers. Any product that may be evaluated in this article, or claim that may be made by its manufacturer, is not guaranteed or endorsed by the publisher.
Supplementary material
The Supplementary Material for this article can be found online at: https://www.frontiersin.org/articles/10.3389/feart.2024.1450319/full#supplementary-material
References
Achterberg, E. V., Ryan, C., Jackson, S. E., and Griffin, W. L. (2001). Data reduction software for LA-ICP-MS. Laser Ablation ICP-MS Earth Sci. 29, 239–243.
Andersen, T. (2002). Correction of common lead in U–Pb analyses that do not report 204Pb. Chem. Geol. 192, 59–79. doi:10.1016/S0009-2541(02)00195-X
Bian, C., Jiang, W., and Song, D. (2010). Terrigenous transportation to the Okinawa Trough and the influence of typhoons on suspended sediment concentration. Cont. Shelf Res. 30, 1189–1199. doi:10.1016/j.csr.2010.03.008
Chen, C.-T. A., Kandasamy, S., Chang, Y.-P., Bai, Y., He, X., Lu, J.-T., et al. (2017). Geochemical evidence of the indirect pathway of terrestrial particulate material transport to the Okinawa Trough. Quat. Int. 441, 51–61. doi:10.1016/j.quaint.2016.08.006
Chen, L., Xu, W., and Shen, S. (1979). Mineral composition and their distribution patterns in the sediments of the East China Sea (in Chinese with English abstract). Chin. Sci. Bull. (in Chinese) 24, 709–712. doi:10.1360/csb1979-24-15-709
Choi, T., Lee, Y., and Orihashi, Y. (2016). Crustal growth history of the Korean Peninsula: constraints from detrital zircon ages in modern river sediments. Geoscience frontiers 7, 707–714. doi:10.1016/j.gsf.2016.05.003
Compston, W., Williams, I. S., Kirschvink, J. L., Zhang, Z., and Ma, G. (1992). Zircon U-Pb ages for the early cambrian time-scale. Journal of the Geological Society 149, 171–184. doi:10.1144/gsjgs.149.2.0171
Dadson, S. J., Hovius, N., Chen, H., Dade, W. B., Hsieh, M.-L., Willett, S. D., et al. (2003). Links between erosion, runoff variability and seismicity in the Taiwan orogen. Nature 426, 648–651. doi:10.1038/nature02150
Deng, K., Yang, S., Li, C., Su, N., Bi, L., Chang, Y.-P., et al. (2017). Detrital zircon geochronology of river sands from Taiwan: implications for sedimentary provenance of Taiwan and its source link with the east China mainland. Earth-Sci. Rev. 164, 31–47. doi:10.1016/j.earscirev.2016.10.015
Dou, Y., Yang, S., Li, C., Shi, X., Liu, J., and Bi, L. (2015). Deepwater redox changes in the southern Okinawa Trough since the last glacial maximum. Prog. Oceanogr. 135, 77–90. doi:10.1016/j.pocean.2015.04.007
Dou, Y., Yang, S., Liu, Z., Clift, P. D., Shi, X., Yu, H., et al. (2010a). Provenance discrimination of siliciclastic sediments in the middle Okinawa Trough since 30ka: constraints from rare earth element compositions. Mar. Geol. 275, 212–220. doi:10.1016/j.margeo.2010.06.002
Dou, Y., Yang, S., Liu, Z., Clift, P. D., Yu, H., Berne, S., et al. (2010b). Clay mineral evolution in the central Okinawa Trough since 28ka: implications for sediment provenance and paleoenvironmental change. Palaeogeogr., Palaeoclimatol., Palaeoecol. 288, 108–117. doi:10.1016/j.palaeo.2010.01.040
Dou, Y., Yang, S., Liu, Z., Shi, X., Li, J., Yu, H., et al. (2012). Sr–Nd isotopic constraints on terrigenous sediment provenances and Kuroshio Current variability in the Okinawa Trough during the late Quaternary. Palaeoclimatol., Palaeoecol. 365-366, 38–47. doi:10.1016/j.palaeo.2012.09.003
Dou, Y., Yang, S., Shi, X., Clift, P. D., Liu, S., Liu, J., et al. (2016). Provenance weathering and erosion records in southern Okinawa Trough sediments since 28ka: geochemical and Sr–Nd–Pb isotopic evidences. Chem. Geol. 425, 93–109. doi:10.1016/j.chemgeo.2016.01.029
Folk, R., Andrews, P., and Lewis, D. (1970). Detrital sedimentary rock classification and nomenclature for use in New Zealand. N. Z. J. Geol. Geophys 13, 937–968. doi:10.1080/00288306.1970.10418211
Gehrels, G. (2011). Detrital Zircon U-Pb Geochronology: Current Methods and New Opportunities. Tectonics of Sedimentary Basins, 45–62. doi:10.1002/9781444347166.ch2
Guo, Z., Yang, Z., and Lei, K. (2001). The distribution and composition of suspended matters and their influencing factors in the central-southern area of Okinawa Trough and its adjacent shelf sea. Haiyang Xuebao 23, 66–72. doi:10.3321/j.issn:0253-4193.2001.01.009
He, M., Zheng, H., and Clift, P. D. (2013). Zircon U–Pb geochronology and Hf isotope data from the Yangtze River sands: implications for major magmatic events and crustal evolution in Central China. Chem. Geol. 360-361, 186–203. doi:10.1016/j.chemgeo.2013.10.020
Hsu, S.-C., Lin, F.-J., Jeng, W.-L., Chung, Y.-c., Shaw, L.-M., and Hung, K.-W. (2004). Observed sediment fluxes in the southwesternmost Okinawa Trough enhanced by episodic events: flood runoff from Taiwan rivers and large earthquakes. Deep Sea Research Part I Oceanographic Research Papers 51, 979–997. doi:10.1016/j.dsr.2004.01.009
Hu, S., Zeng, Z., Fang, X., Yin, X., Chen, Z., Li, X., et al. (2020). Increasing terrigenous sediment supply from Taiwan to the southern Okinawa Trough over the last 3000 years evidenced by SrNd isotopes and geochemistry. Sediment. Geol. 406, 105725. doi:10.1016/j.sedgeo.2020.105725
Huang, X., Song, J., Yue, W., Wang, Z., Mei, X., Li, Y., et al. (2020). Detrital zircon U-Pb ages in the East China seas: implications for provenance analysis and sediment budgeting. Minerals 10, 398. doi:10.3390/min10050398
Huh, C.-A., Su, C.-C., Liang, W.-T., and Ling, C.-Y. (2004). Linkages between turbidites in the southern Okinawa Trough and submarine earthquakes. Geophys. Res. Lett. 31. doi:10.1029/2004GL019731
Hung, J. J., Lin, C. S., Hung, G. W., and Chung, Y. C. (1999). Lateral transport of lithogenic particles from the continental margin of the southern east China sea. Estuar. Coast. Shelf Sci. 49, 483–499. doi:10.1006/ecss.1999.0520
Iseki, K., Okamura, K., and Kiyomoto, Y. (2003). Seasonality and composition of downward particulate fluxes at the continental shelf and Okinawa Trough in the East China Sea. DSR 50, 457–473. doi:10.1016/S0967-0645(02)00468-X
Jian, Z., Wang, P., Saito, Y., Wang, J., Pflaumann, U., Oba, T., et al. (2000). Holocene variability of the Kuroshio Current in the Okinawa Trough, northwestern Pacific Ocean. Earth. Planet. Sci. Lett. 184, 305–319. doi:10.1016/S0012-821X(00)00321-6
Jin, X., and Yu, P. (1987). The tectonic characteristics and evolution of the Okinawa Trough. Science in China Series B (in Chinese) 17, 86–93. doi:10.1360/zb1987-17-2-196
Kao, S. J., and Milliman, J. D. (2008). Water and sediment discharge from small mountainous rivers, Taiwan: the roles of lithology, episodic events, and human activities. The Journal of Geology 116, 431–448. doi:10.1086/590921
Katayama, H., and Watanabe, Y. (2003). The Huanghe and Changjiang contribution to seasonal variability in terrigenous particulate load to the Okinawa Trough. Deep-Sea Research 50, 475–485. doi:10.1016/S0967-0645(02)00469-1
Lease, R., Burbank, D., Gehrels, G., Wang, Z., and Yuan, D. (2007). Signatures of mountain building: detrital zircon U/Pb ages from northeastern Tibet. Geology 35, 239. doi:10.1130/G23057A.1
Li, D., Liu, X., Cheng, H., Liang, J., Xu, S., Dong, G., et al. (2022). Development of submarine canyons on the continental slope of the Okinawa Trough with potential origin related to methane seepage. Ore Geology Reviews 149, 105088. doi:10.1016/j.oregeorev.2022.105088
Li, D., Xu, X., Liu, X., Cheng, H., Xu, S., and Jiang, X. (2023). Grain size and geochemistry characteristics of Core S01-10 from the central Okinawa Trough since 14 ka: indications for sediment source and the East Asian winter monsoon. Mar. Geol. 460, 107053. doi:10.1016/j.margeo.2023.107053
Li, N., Jiang, L., and Li, C. (1998). A study on the nature of crustal structures of the Okinawa Trough. Oceanologia et limnologia sinica (in Chinese) 29, 441–450. CNKI:SUN:HYFZ.0.1998-04-015.
Li, T., and Chang, F. (2009). Paleoceanography in the Okinawa Trough. Beijing, China: China Ocean Press.
Li, T., Xiang, R., Sun, R., and Cao, Q. (2005). Benthic foraminifera and bottom water evolution in the middle-southern Okinawa Trough during the last 18 ka. Science in China Series D Earth Sciences 48, 805–814. doi:10.1360/03yd0222
Li, T., Xu, Z., Lim, D., Chang, F., Wan, S., Jung, H., et al. (2015). Sr–Nd isotopic constraints on detrital sediment provenance and paleoenvironmental change in the northern Okinawa Trough during the late Quaternary. Palaeogeogr., Palaeoclimatol., Palaeoecol. 430, 74–84. doi:10.1016/j.palaeo.2015.04.017
Li, X.-s., Zhao, Y.-x., Liu, B.-h., Liu, C.-g., Zheng, Y.-p., and Wang, K.-y. (2004). Spatio-temporal characteristics of depositional evolution of the Okinawa Trough (abstract in English). Advances in Marine Science 22, 472–479.
Liu, J., Zhu, R., Li, T., Li, A., and Li, J. (2007a). Sediment−magnetic signature of the mid-Holocene paleoenvironmental change in the central Okinawa Trough. Mar. Geol. 239, 19–31. doi:10.1016/j.margeo.2006.12.011
Liu, J. P., Liu, C. S., Xu, K. H., Milliman, J. D., Chiu, J. K., Kao, S. J., et al. (2008). Flux and fate of small mountainous rivers derived sediments into the Taiwan Strait. Mar. Geol. 256, 65–76. doi:10.1016/j.margeo.2008.09.007
Liu, J. P., Xu, K. H., Li, A. C., Milliman, J. D., Velozzi, D. M., Xiao, S. B., et al. (2007b). Flux and fate of Yangtze River sediment delivered to the East China sea. Geomo 85, 208–224. doi:10.1016/j.geomorph.2006.03.023
Liu, Z., Saito, Y., Li, T., Berne, S., Chen, Z., Li, P., et al. (1999). Millennial-scale paleoceanography in Okinawa Trough during late quaternary period. Chin. Sci. Bull. 44, 1705–1709. doi:10.1007/BF03183496
Ludwig, K. R. (2001). User’s manual for isoplot/ex (rev. 2.49): a geochronological toolkit for microsoft excel. Berkeley Geochronology Center, Special Publication 1a.
Machida, H. (1999). The stratigraphy, chronology and distribution of distal marker-tephras in and around Japan. Global and Planetary Change - GLOBAL PLANET CHANGE 21, 71–94. doi:10.1016/S0921-8181(99)00008-9
Meng, X., Du, D., and Liu, Y. (2007). Terrestrial flux in sediments from the Okinawa Trough and its response to climate changes over the past 35 000 a. Haiyang Xuebao (in Chinese) 29, 74–80. doi:10.3321/j.issn:0253-4193.2007.05.009
Oguri, K., Matsumoto, E., Yamada, M., Saito, Y., and Iseki, K. (2003). Sediment accumulation rates and budgets of depositing particles of the East China Sea. DSR 50, 513–528. doi:10.1016/S0967-0645(02)00465-4
Pan, Z., and Shi, S. (1986). Study on sediments and sedimentation in Okinawa Trough. Marine Geology and Quaternary Geology (in Chinese) 6, 17–29.
Qu, T., and Lukas, R. (2003). The bifurcation of the north equatorial current in the pacific. J. Phys. Oceanogr. 33, 5–18. doi:10.1175/1520-0485(2003)033<0005:TBOTNE>2.0.CO;2
Sibuet, J.-C., Deffontaines, B., Hsu, S.-K., Thareau, N., Forma, J.-P. L., Liu, C.-S., et al. (1998). Okinawa trough backarc basin: early tectonic and magmatic evolution. JGR 103, 30245–30267. doi:10.1029/98jb01823
Su, J., Rao, W., Wang, Y., and Mao, C. (2018). Detrital zircon geochronology of the radial sand ridge system of Jiangsu coast, east China: implication for sediment provenance. Journal of Earth Science 29, 144–154. doi:10.1007/s12583-017-0769-x
Thomas, W. A., Astini, R. A., Mueller, P. A., Gehrels, G. E., and Wooden, J. L. (2004). Transfer of the Argentine precordillera terrane from laurentia: constraints from detrital-zircon geochronology. Geology 32, 965–968. doi:10.1130/G20727.1
Uehara, K., and Saito, Y. (2003). Late Quaternary evolution of the Yellow/East China Sea tidal regime and its impacts on sediments dispersal and seafloor morphology. Sediment. Geol. 162, 25–38. doi:10.1016/S0037-0738(03)00234-3
Wang, J., Li, A., Xu, K., Zheng, X., and Huang, J. (2015). Clay mineral and grain size studies of sediment provenances and paleoenvironment evolution in the middle Okinawa Trough since 17ka. Mar. Geol. 366, 49–61. doi:10.1016/j.margeo.2015.04.007
Wang, L., Griffin, W. L., Yu, J., and O'Reilly, S. Y. (2013). U–Pb and Lu–Hf isotopes in detrital zircon from Neoproterozoic sedimentary rocks in the northern Yangtze Block: implications for Precambrian crustal evolution. Gondwana Research 23, 1261–1272. doi:10.1016/j.gr.2012.04.013
Wang, Z., Yang, S., and Zhang, Z. (2012). The grain size compositions of the surface sediments in the East China Sea: indication for sedimentary environments. Oceanologia et limnologia sinica (in Chinese) 43, 1039–1049. doi:10.11693/hyhz201206003003
Xie, J., Yang, S., and Ding, Z. (2012). Methods and application of using detrital zircons to trace the provenance of loess. Sci. China Earth Sci. 55, 1837–1846. doi:10.1007/s11430-012-4428-x
Xu, Z., Li, T., Chang, F., Wan, S., Choi, J., and Lim, D. (2014). Clay-sized sediment provenance change in the northern Okinawa Trough since 22 kyr BP and its paleoenvironmental implication. Palaeogeography Palaeoclimatology Palaeoecology 399, 236–245. doi:10.1016/j.palaeo.2014.01.016
Xu, Z., Li, T., Clift, P. D., Lim, D., Nan, Q., Wan, S., et al. (2017). Sediment provenance and paleoenvironmental change in the middle Okinawa Trough during the last 18.5 ky: clay mineral and geochemical evidence. Quaternary International 440, 139–149. doi:10.1016/j.quaint.2016.07.058
Xue, C., Zhou, Y., and Wang, G. (2003). Reviews of the Yellow River delta superlobes since 700 BC. Marine Geology and Quaternary Geology (in Chinese) 23, 23–29.
Xue, C., Zhou, Y., and Zhu, X. (2004). The huanghe river course and delta from end of late Pleistocene. Acta Oceanologica Sinica (in Chinese) 26, 48–61. doi:10.3321/j.issn:0253-4193.2004.01.006
Yanagi, T., Takahashi, S., Hoshika, A., and Tanimoto, T. (1996). Seasonal variation in the transport of suspended matter in the East China Sea. J. Oceanogr. 52, 539–552. doi:10.1007/BF02238320
Yang, B., Wu, Y., Liu, J., Liu, Y., Zhang, H., Wang, X., et al. (2018). Elemental geochemistry of surface sediments in Okinawa Trough and its implications for provenance and hydrothermal activity. Marine Geology and Quaternary Geology (in Chinese) 38, 25–37. doi:10.16562/j.cnki.0256-1492.2018.02.003
Yang, B., Wu, Y., Wang, X., Zhang, Y., Cui, J., Yu, M., et al. (2021). Mineralogical and geochemical characteristics and ore-forming mechanism of hydrothermal sediments in the middle and southern Okinawa Trough. Mar. Geol. 437, 106501. doi:10.1016/j.margeo.2021.106501
Yang, J., Gao, S., Chen, C., Tang, Y., Yuan, H., Gong, H., et al. (2009). Episodic crustal growth of North China as revealed by U–Pb age and Hf isotopes of detrital zircons from modern rivers. Geochim. Cosmochim. Acta. 73, 2660–2673. doi:10.1016/j.gca.2009.02.007
Yang, S. L., Zhang, J., Zhu, J., Smith, J. P., Dai, S. B., Gao, A., et al. (2005). Impact of dams on Yangtze River sediment supply to the sea and delta intertidal wetland response. Journal of Geophysical Research Earth Surface 110. doi:10.1029/2004JF000271
Yang, S. Y., Jung, H. S., Lim, D. I., and Li, C. X. (2003). A review on the provenance discrimination of sediments in the Yellow Sea. Earth-Sci. Rev. 63, 93–120. doi:10.1016/S0012-8252(03)00033-3
Yang, Z., Guo, Z. G., and Wang, Z. X. (1992). Basic pattern of transport of suspended matter from the Yellow Sea and East China Sea to the eastern deep seas. Acta Oceanol. Sin. 14, 81–90. doi:10.1007/BF02677081
Yuan, D., Zhu, J., Li, C., and Hu, D. (2008). Cross-shelf circulation in the Yellow and East China Seas indicated by MODIS satellite observations. J. Mar. Syst. 70, 134–149. doi:10.1016/j.jmarsys.2007.04.002
Yuan, Y., Chen, G., Yang, W., and Zhu, C. (1987). Characteristics of sediments in the Okinawa Trough. Acta Oceanologica Sinica (in Chinese) 9, 353–359.
Zeng, Z., Chen, S., Ma, Y., Yin, X., Wang, X., Zhang, S., et al. (2017). Chemical compositions of mussels and clams from the Tangyin and Yonaguni Knoll IV hydrothermal fields in the southwestern Okinawa Trough. Ore Geology Reviews 87, 172–191. doi:10.1016/j.oregeorev.2016.09.015
Zhai, S., Xu, S., Yu, Z., Qin, Y., and Zhao, Y. (2001). Two possible hydrothermal vents in the northern Okinawa Trough. Chin. Sci. Bull. 46, 943–945. doi:10.1007/BF02900472
Zhang, J., and Liu, C. L. (2002). Riverine composition and estuarine geochemistry of particulate metals in China—weathering features, anthropogenic impact and chemical fluxes. Estuar. Coast. Shelf Sci. 54, 1051–1070. doi:10.1006/ecss.2001.0879
Zhao, D., Wan, S., Clift, P. D., Tada, R., Huang, J., Yin, X., et al. (2018). Provenance, sea-level and monsoon climate controls on silicate weathering of Yellow River sediment in the northern Okinawa Trough during late last glaciation. Palaeogeogr., Palaeoclimatol., Palaeoecol. 490, 227–239. doi:10.1016/j.palaeo.2017.11.002
Zhao, D., Wan, S., Jiang, S., Shen, S., Ren, X., Révillon, S., et al. (2019). Quaternary sedimentary record in the northern Okinawa Trough indicates the tectonic control on depositional environment change. Palaeogeogr., Palaeoclimatol., Palaeoecol. 516, 126–138. doi:10.1016/j.palaeo.2018.12.001
Zhao, Y., Liu, B., Li, X., Liu, C., Pei, Y., Wu, J., et al. (2009). Distributions of different submarine canyons on the East China sea slope and their tectonic response (in Chinese). Advances in Marine Science 27, 460–468. doi:10.3969/j.issn.1671-6647.2009.04.007
Zhao, Y. Y., and Yan, M. C. (1992). Abundance of chemical elements in sediments from the Huanghe River, the Changjiang River and the continental shelf of China. Chin. Sci. Bull. 37, 1991–1994. doi:10.1360/sb1992-37-23-1991
Zheng, P., Li, D., Chen, Y., Hou, K., and Liu, C. (2013). Zircon U-Pb ages of clastic Sediment from the outfall of the Yellow River and their geological significance. Geoscience (in Chinese) 27, 79–90. doi:10.3969/j.issn.1000-8527.2013.01.008
Zheng, X., Li, A., Wan, S., Jiang, F., Kao, S.-J. I., and Johnson, C. (2014). ITCZ and ENSO pacing on East Asian winter monsoon variation during the Holocene: sedimentological evidence from the Okinawa Trough. Journal of Geophysical Research Oceans 119, 4410–4429. doi:10.1002/2013JC009603
Zhu, B., and Zeng, Z. (2022). Characteristic of detrital zircon U-Pb geochronology in the southern Okinawa Trough and its implication for sediment provenance. Marine Geology Frontiers (in Chinese) 38, 14–23. doi:10.16028/j.1009-2722.2020.190
Keywords: Okinawa Trough, sediments, detrital zircons, geochronology, provenance
Citation: Yang B-J, Liu J-H and Wu Y-H (2024) Detrital zircon U-Pb geochronology of sediments from the Okinawa Trough: implications for sedimentary provenance. Front. Earth Sci. 12:1450319. doi: 10.3389/feart.2024.1450319
Received: 17 June 2024; Accepted: 19 August 2024;
Published: 05 September 2024.
Edited by:
Marta Wlodarczyk-Sielicka, Maritime University of Szczecin, PolandCopyright © 2024 Yang, Liu and Wu. This is an open-access article distributed under the terms of the Creative Commons Attribution License (CC BY). The use, distribution or reproduction in other forums is permitted, provided the original author(s) and the copyright owner(s) are credited and that the original publication in this journal is cited, in accordance with accepted academic practice. No use, distribution or reproduction is permitted which does not comply with these terms.
*Correspondence: Bao-Ju Yang, bjyang0535@163.com