- 1Gasfield Company of Shaanxi Yanchang Petroleum Group Co., Ltd., Yan’an, Shaanxi, China
- 2College of Geoscience and Surveying Engineering, China University of Mining and Technology, Beijing, China
The Yan’an gas field in the Ordos Basin is a typical deep coalbed methane field with tremendous resource potential. Evaluation methods for gas content in deep coal seams are urgently required to be established. This study is aimed at quantitatively analyzing the gas content of coalbed methane in deep coalbed methane reservoirs and revealing its influencing factors. With the coal rock samples of typical deep coalbed methane wells in the Yan’an gas field of the Ordos Basin as the research objects, the gas-bearing characteristics of deep coal rocks were analyzed, and the main controlling factors of gas-bearing properties were explored. The research results indicate that (1) the deep coal seams in the Yan’an gas field have a considerable thickness, a high total organic carbon content, and the potential of pyrolysis hydrocarbon generation is generally elevated, presenting excellent hydrocarbon generation potential. (2) Various types of pores and fractures in the deep coal rocks of the Yan’an gas field are well-developed, providing a favorable preservation space and migration channel for deep coalbed methane. (3) The total gas content of on-site analysis of deep coalbed methane in the Yan’an gas field is relatively high, mainly existing in the form of free gas, and has significant exploration and development potential. (4) The gas content of deep coal rocks in the Yan’an gas field is jointly controlled by multiple factors such as the total organic carbon content, minerals, and pore structure. In conclusion, the deep coal seams in the Yan’an gas field have favorable reservoir-forming conditions and great exploration and development potential.
1 Introduction
The seventy-fifth session of the United Nations General Assembly put forward the ‘double carbon’ target. The exploration and development of clean energy is facing great challenges. Different from other types of natural gas, the development and utilization of coalbed methane has multiple attributes of ‘economy, safety, and environmental protection’. The geological resources of coalbed methane in China are approximately 30.37 trillion m3 at a buried depth of 1,500–3,000 m, which is twice the amount of coalbed methane resources above 1,500 m (Chen, 2016). However, at present, the buried depth of the target coal seam for coalbed methane exploration and development in China and major countries in the world is concentrated at 1,500 m. According to the distribution characteristics of coalbed methane resources, the study shows that the deep coalbed methane resources are more abundant and have important strategic significance (Geng et al., 2018; Zhao et al., 2011). In recent years, the exploration and development of deep coalbed methane in China has attracted great attention, and the exploration field of coalbed methane has been expanded from a high coal rank to a middle and low coal rank, and the development depth of coalbed methane has been expanded from shallow to middle and deep layers (Xu et al., 2021; Sun et al., 2011).
In recent years, after preliminary estimation, China’s 2,000-m and deep coalbed methane resources can reach 40.71 trillion m3, of which recoverable resources are 10.01 trillion m3, including the Ordos Basin, Junggar Basin, and Turpan-Hami–Santanghu Basin (Qin et al., 2022). The Ordos Basin is rich in coalbed methane resources. Compared with shallow coal seams, deep coalbed methane reservoirs have the characteristics of relatively high temperature, high pressure, high gas content, and high saturation (Niu et al., 2023). Under deep conditions, the coal reservoir is in complex geological conditions of high temperature, high pressure, and high ground stress, and the dynamic equilibrium processes of adsorption/desorption, diffusion, and seepage of coalbed methane become complicated. The exploration and development of deep coalbed methane cannot simply apply the exploration and development theory of shallow coalbed methane. Coalbed methane mainly exists in the pores and fissures of coal rock in the form of adsorption and free state, and only a small part exists in the form of dissolved state (Song et al., 2020). The adsorbed gas is mainly adsorbed on the surface of the coal matrix and clay mineral particles, and the free gas is mainly free from the macropores and cracks in coals (Mou et al., 2021). Different from shallow coalbed methane, the occurrence state of deep coalbed methane is complex, and the ratio of adsorbed gas to free gas has always been controversial. The free gas content is affected by factors such as pore and formation temperature. The pore determines the size of the gas storage space and directly affects the free gas content. Temperature and pressure affect the gas compression state and the adsorption or free state of methane, which, in turn, affects the gas content. In the process of coalbed methane development, only the influence of micron-scale fracture development scale and connectivity on the permeability of coal reservoirs is usually emphasized, while the influence of nano-scale pore-fracture development characteristics and connectivity on gas desorption and migration is ignored (Zhang et al., 2017). With the progress of coalbed methane exploration and development technology in recent years and the increase in the domestic energy demand, the development of deep coalbed methane has become possible and imperative. China’s deep coalbed methane resources are huge. If economic exploitation and utilization can be realized, it will effectively promote the development of China’s coalbed methane industry into a new stage and help China’s unconventional natural gas exploration and development to a new level.
Aiming at the difference in the gas-bearing property of deep coal in the Yichuan area of Yan’an gas field in southeastern Ordos Basin, based on the exploration data on the study area, the geochemical characteristics, mineral composition, and distribution law of deep coal were analyzed, the physical properties of deep coal were analyzed, and the microscopic reservoir space characteristics of coal were studied. At the same time, combined with on-site analytical experiments and carbon isotope fractionation experiments, the quantitative characterization and evaluation of adsorption–desorption of key wells were carried out, and the gas-bearing variation law of carbon isotope fractionation in the Yichuan area was analyzed.
2 Geological overview
The Ordos Basin is the second largest sedimentary basin in China. It is an important coal-bearing basin in China, with an area of approximately 25×104 km3, spanning Shanxi, Inner Mongolia, Shaanxi, Gansu, and Ningxia provinces. The Ordos Basin is mainly composed of six secondary tectonic units, namely, Yimeng uplift, western margin thrust belt, Tianhuan depression, Yishan slope, Jinxi flexural fold belt, and Weibei uplift (Wang, 1996). The southeastern Ordos Basin is roughly located in the east of Zhidan, the west of the Yellow River, the south of Suide, and the north of Luochuan, with an area of 1.07×104 km2. Its main body is located in the secondary tectonic unit of the Yishan slope (Figure 1).
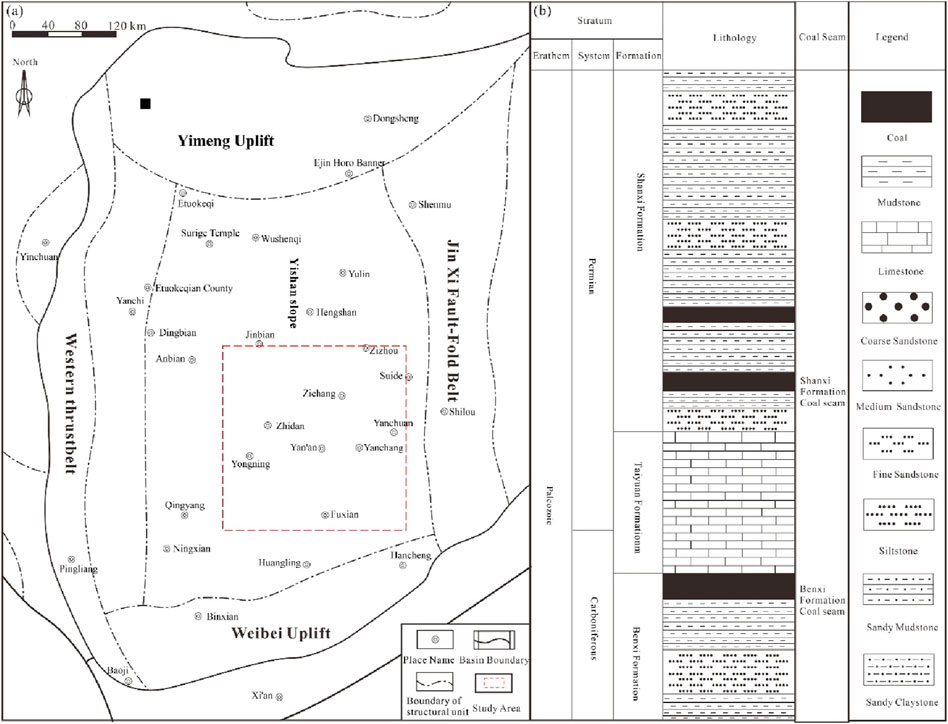
Figure 1. (A): Yan’an gas field is located in the Ordos Basin, China, and the sample locations used in this study are indicated by red circles. (B): Stratigraphic characteristics of the Paleozoic in the Yan’an gas field (Yu et al., 2017).
The Upper Paleozoic in the southeastern part of the Ordos Basin mainly develops the Upper Permian Shiqianfeng Formation, the Middle Permian Shihezi Formation and the Lower Permian Shanxi Formation, Taiyuan Formation, and the Upper Carboniferous Benxi Formation (Li et al., 2014). The Upper Paleozoic of the Yan’an gas field is divided into the Benxi Formation of Upper Carboniferous, Taiyuan Formation and Shanxi Formation of Lower Permian, Lower Shihezi Formation and Upper Shihezi Formation of Middle Permian, and Shiqianfeng Formation of Upper Permian from bottom to top. The Upper Paleozoic Middle–Upper Carboniferous to Permian strata are continuous and well-integrated, forming continuous and stable sedimentary strata from the Benxi Formation to Shiqianfeng Formation (Zhao et al., 2012). The Benxi Formation develops a set of barrier-coastal sedimentary mudstone and sandstone interbedded rock series with unequal thickness. The upper part is thick coal, and the bottom is black mudstone or a bauxite layer, which is in pseudo-conformity contact with the underlying Lower Ordovician Majiagou Formation. Multi-layer thin fine sandstone and coal seam interlayers are developed in the Shanxi Formation, mainly various types of mud shale. The coalbed methane of the Benxi Formation and Shanxi Formation has a good exploration prospect, which is one of the main exploration target strata of the Upper Paleozoic in the southeast part of the Ordos Basin. The source supply in the study area is relatively sufficient, and it is in the mixed source area where the north and south sources converge. The source mainly comes from the northern Yinshan area and the southern Qinling orogenic belt. In contrast, the northern source has a larger range of influence (Zhao et al., 2023; Zhang et al., 2023). The research block of this paper is the Yan’an gas field located in the southeast of the Ordos Basin, and the field gas content test of six wells is complete.
3 Samples and experimental methods
3.1 Sample selection
In this paper, the deep coal rock samples of six key exploration wells in the Yan’an gas field in the southeastern Ordos Basin are taken as the research object. The development horizons include the Shanxi Formation and Benxi Formation, with a total of 18 samples. Coal and rock samples are quickly sealed in a sealed bag after collection in order to minimize pollution and oxidation. The coal structure of the selected coal samples is primary structural coal, and the coal rock types are mainly semi-bright coal and semi-dark coal. The maximum reflectance of vitrinite varies from 1.81% to 4.01%, with an average of 2.84%, which belongs to high-rank coal (Figure 2). The industrial analysis shows that the moisture content of the air-dried base of the coal mining rock is 0.22–1.61%, with an average of 0.82%, which is an ultra-low moisture coal. The dry base ash is 6.20–80.73%, with an average of 20.81%, which is ultralow-to-low-ash coal. The volatile content is 5.71–42.04%, with an average of 12.21%, which is low-volatile coal. Elemental analysis showed that the coal samples accounted for the vast majority of carbon; the content was 39.44–84.37%, and the average was 68.24%. In addition, the contents of oxygen and hydrogen in the samples are also high, which are 0.24–5.91% and 1.22–6.97%, respectively. The second is sulfur; the content is 0.34–5.03%, and the nitrogen content is the least, which is 0.22–1.06%. The total sulfur content of coal samples in the Yan’an gas field ranges from 0.20% to 5.15%, with an average of 1.86%, which belongs to medium sulfur coal. The sulfur in coal samples mainly exists in the form of organic sulfur.
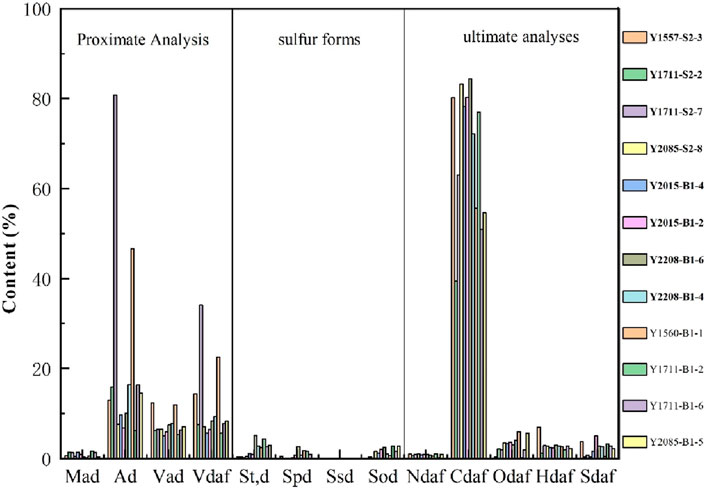
Figure 2. Analysis of the coal quality characteristics of coal rock samples based on proximate analysis.
3.2 Experimental methods
The main experimental methods in this study are field desorption and isotope fractionation experiments. The on-site gas content analysis experiment was carried out in combination with the measurement standard and the actual situation of the drilling site. The ground temperature is set to 70°C. This is because the overall average ground temperature gradient in the Ordos Basin is 2.50–2.90°C/100 m. The buried depth of the on-site analytical sample is between 3,238 and 3,296 m, which is converted into a vertical depth between 2,778 and 2,829 m. The ground temperature of the on-site analytical sample is calculated to be 69.45–70.73°C, with a ground temperature gradient of 2.50°C/100 m. In order to unify the on-site analytical experimental conditions, the ground temperature is set to 70°C. The high-temperature condition is set to 90°C. This is because the test uses an easy-to-operate, high-precision water bath heating box device. When the temperature reaches 90–100°C, water vapor will be generated to affect the analytical effect. Therefore, the high-temperature condition is controlled at 90°C, and the residual gas analysis effect of the sample under high-temperature conditions can be achieved. According to these three temperatures, the field analysis process can be divided into three stages, namely, the normal temperature stage, the low temperature stage, and the high temperature stage. The basic process of analysis is to accurately record the key moment during the drilling process. After the core is lifted to the wellhead, it is quickly loaded into the sealing tank. The desorption gas volume in the coal seam is measured under normal temperature and simulated formation temperature conditions, and then, the residual gas volume in the coal seam is measured under high-temperature conditions. The square root of the measured desorption gas volume and desorption time is used to calculate the lost gas volume.
According to field analysis and isotope fractionation theory, we know that the carbon isotope value (δ13C1) of methane gradually becomes heavier with the increase in analysis time. In the early stage, the free gas in the desorption gas of coal and rock is dominant. With the increase in desorption temperature and the passage of desorption time, the adsorbed gas in coal and rock is gradually desorbed. Until the final stage, the free gas has been almost completely desorbed, and the remaining is mainly adsorbed gas. Based on the field analytical experiment, we selected gas samples of different representative temperatures for each sample to measure and obtained the methane carbon isotope value (δ13C1) of the coal sample at the normal temperature stage, the low temperature stage, and the high temperature stage. According to the formula, the content of adsorbed gas and free gas is calculated, and then, the adsorption free ratio is obtained.
4 Development characteristics of deep coal rock
4.1 Coal seam distribution characteristics
The main coal-bearing strata in the southeastern Ordos Basin are the Benxi Formation and Shanxi Formation. The buried depth of Benxi Formation coal rock and Shanxi Formation coal rock in the study area shows a trend of gradual increase from east to west and is generally greater than 2,000 m. Some coal seams are buried more than 3,000 m, which belongs to a typical deep coalbed methane field (Figure 3). The coal seam of the Benxi Formation in the study area is controlled by the continental river delta, mainly open overlying water floating swamp, and the thickness of the coal seam is thin. The coal seam of the Shanxi Formation in the study area is near the coastal plain environment, which is developed by high swamps, and the thickness of the coal seam is large. The thickness of the Benxi Formation coal seam in the Yan’an gas field is mainly between 0.50 and 7.60 m, with an average of 1.60 m. The thickness of the Shanxi Formation coal seam in the Yan’an gas field is between 0.50 m and 10.00 m, with an average of 2.30 m. The thickness of the Benxi Formation coal seam is slightly smaller than that of the Shanxi Formation coal seam. In general, the distribution of coal seam thickness in the study area is that the coal seams are thinning from north to south and east to west (Figure 4).
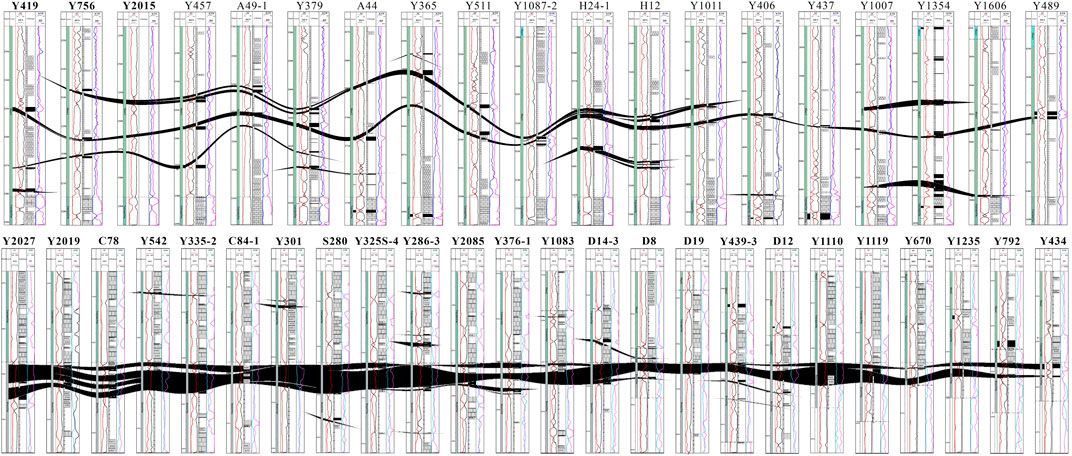
Figure 4. Coal seam profile comparison between the Benxi Formation and Shanxi Formation in the Yan’an gas field research area.
4.2 Coal rock characteristics
Macroscopic coal rock types are usually divided according to the combination form of the coal rock composition and its gloss strength. Medium–high-rank humic coal is widely developed in the study area, and its formation process is related to the peat swamp environment (Lu et al., 2014). The macroscopic coal rock types of the main coal seams in the study area are mainly semi-bright coal and semi-dark coal (Figure 5). Among them, the coal rock of the Shanxi Formation is mainly semi-bright coal and semi-dark coal; the coal rock of the Benxi Formation is mainly semi-bright coal and bright coal. Bright coal has the strongest metallic luster and a uniform composition; usually, the banded structure is not obvious, with a shell-like fracture and endogenous fracture development; the semibright coal has strong luster, from which we can see the lineation, lens, and strip formed by specular coal. Semidull coal, gray–black color, part of the fracture has luster (Cui, 2022). From the point of view of coal structure, Shanxi Formation coal rock, the whole coal rock is dominated by primary structure coal. Generally, bright coal and semi-bright coal with a primary structure have developed micro-fractures and cleats. The seams in the study area are well developed, most of the coal seam cracks are filled with calcite and pyrite, and the reservoir performance is good (Zhao et al., 2024).
The macerals in the coal rock samples of the Yan’an gas field are mainly vitrinite and inertinite, containing a small amount of the lipid group (Figure 6). The content of vitrinite in the Shanxi Formation and Benxi Formation is 51.30–71.60% and 42.50–85.90%, respectively. The inertinite content ranges between 2.90–41.70% and 2.40–32.70%, respectively. The content range of the lipid group was between 1.20%–2.80% and 0.20–3.00%, respectively. The content of vitrinite reflects the depth of water body and the degree of reduction of sedimentary environment during the deposition of coal-forming sediments (Zhang, 2022). It can also better reflect the physical conditions of coalbed methane reservoirs. From the perspective of the content of macerals in the study area, the overall study area is a strong reduction environment. The vitrinite reflectance of the Shanxi Formation ranges from 1.81% to 2.96%, with an average of 2.64%. The vitrinite reflectance of the Benxi Formation ranges from 1.96% to 4.01%, with an average of 3.07%. All samples have Ro>1.80%, which belongs to the evolution degree of medium and high coal rank. In addition to the high proportion of vitrinite, the other components change significantly, indicating that the heterogeneity of coal rock is strong.
5 Gas-bearing characteristics of deep coal seams
5.1 On-site desorption gas content of coal rock
In the process of the field analysis of coalbed gas, coalbed gas can be divided into three parts (Figure 7), namely, the amount of natural gas that escapes and is difficult to measure before the sample is loaded into the desorption tank (lost gas), the amount of gas released by coal rock under a simulated formation temperature (desorption), and the amount of natural gas that cannot be obtained by desorption under a simulated formation temperature (residual gas) (Zhang et al., 2024). First, the free coalbed methane in the pores or cracks of coal rock is desorbed at the normal temperature stage, and the analytical gas volume curve gradually decreases and tends to be gentle with the increase in time. However, since the free gas content accounts for about 10% of the total content in coalbed methane reservoirs (Hou et al., 2016; Jiao et al., 2014), this part of the analytical gas volume accounts for a small proportion. The second part is based on the desorption of the remaining free gas and the adsorbed gas from the surface of organic matter or minerals at the formation temperature. The analytical gas volume increases rapidly and gradually becomes gentle with the increase in temperature. The third part is desorption of the adsorbed gas at the high temperature stage, and the analytical gas volume gradually increases and eases with the increase in temperature. The adsorbed gas in coalbed methane reservoirs accounts for more than 85% of the total content (Hou et al., 2016). In the desorption experiment, the second and third parts of analytical gas account for a large proportion, and the adsorbed gas is the main gas.
According to the results of field analysis of coal samples, the gas content of coal seams in the Yan’an area is relatively high. The total gas content of coal seams in the Shanxi Formation is 6.02–28.51 m3/t, and the average gas content is 20.46 m3/t. Among them, the total gas content of coal seam in the Y1557 well is 19.95 m3/t, and the average gas content is 19.95 m3/t. The total gas content of the coal seam in the Y1711 well is 20.45–21.37 m3/t, and the average gas content is 20.91 m3/t. The total gas content of the coal seam in the Y2085 well is 6.02–28.51 m3/t, and the average gas content is 20.33 m3/t. The total gas content of the Benxi Formation coal seam is 13.40–23.86 m3/t, and the average gas content is 18.91 m3/t. The total gas content of the coal seam in the Y2015 well is 17.73–20.50 m3/t, and the average gas content is 19.30 m3/t. The total gas content of the coal seam in the Y2208 well is 14.15–17.07 m3/t, and the average gas content is 15.61 m3/t. The total gas content of the coal seam in the Y1560 well is 17.19–21.14 m3/t, and the average gas content is 19.17 m3/t. The total gas content of the coal seam in the Y1711 well is 17.62–21.49 m3/t, and the average gas content is 19.56 m3/t. The total gas content of the coal seam in the Y2085 well is 13.40–23.86 m3/t, and the average gas content is 20.11 m3/t. The gas content of some samples in the Shanxi Formation is higher than 20.46 m3/t, and the gas content of the Shanxi Formation coal seam is slightly higher than the Benxi Formation on the whole.
Among them, the gas structure of the Yan’an gas field is shown in Figure 8, in which the desorption gas content is the highest, the loss gas is the second, and the residual gas is the least. The contents of desorption, lost gas, and residual gas in the Shanxi Formation were 4.86–20.19 m3/t, 0.58–5.67 m3/t, and 0.58–1.61 m3/t, and the mean values were 14.75 m3/t, 4.56 m3/t, and 1.48 m3/t, respectively. The contents of desorption, loss gas, and residual gas in the Benxi Formation were 8.68–17.84 m3/t, 0.54–8.35 m3/t, and 0.55–4.22 m3/t, respectively, and the mean values were 13.60 m3/t, 3.22 m3/t, and 2.08 m3/t, respectively. On the whole, the gas content of coalbed methane in the study area is high, and it has great potential for exploration and development.
5.2 Characterization of the gas-bearing structure based on field analysis
Like shale gas, coalbed methane is also divided into three parts: lost gas, desorbed gas, and residual gas during on-site analysis. When the coal rock stratum is opened by the drill and the rock core is lifted to the surface, due to the change in temperature and pressure conditions, the free state of natural gas escapes directly under the condition of pressure reduction and finally constitutes the lost gas. At the same time, under certain conditions, it can also become the main contributor to desorption gas. The adsorbed gas gradually begins to desorb with the extension of time and the increase in temperature. In addition to a part of the desorbed gas, it is still the main body of the residual gas (Zhang et al., 2021). Therefore, it is easy to obtain the parameters or the aspirational ratio to characterize the relative ratio of free gas and adsorbed gas in coal rock. The flow-suction ratio is a gas bearing structural parameter of coal rock which is different from gas bearing. The gas-suction ratio represents the characteristics of the occurrence state of shale gas. The higher the value, the easier shale gas is to be produced.
Zhang Jinchuan (2010) put forward the concept of gas-bearing structure and defined the content, proportion, and relationship of natural gas in each phase as the gas-bearing structure. The gas-bearing structural parameters such as the total amount of natural gas, the proportion of each, and the ratio of each other in different ways or under different conditions in coal–rock strata, especially the gas occurrence state and its proportion, directly affect the recovery rate of coal–rock gas recovery (Zhang et al., 2024). The simultaneous presence of adsorbed and free natural gas leads to complex changes in the gas-bearing structure and recoverable capacity of coal rock. The average proportion of coal and rock samples in the study area is 72.12%, the average proportion of lost gas is 18.65%, and the average proportion of residual gas is 9.18%. In the total gas content, the proportion of desorption is the highest, the average proportion of lost gas is the lowest, and the average proportion of residual gas is the lowest (Figure 9).
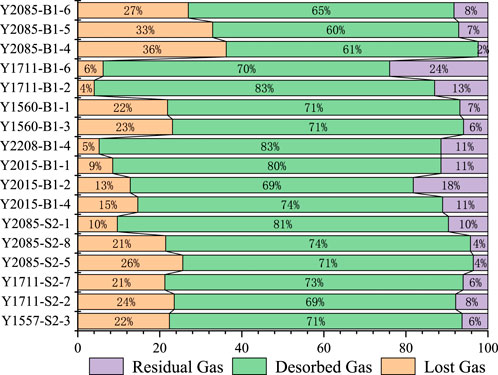
Figure 9. Proportion of the gas-bearing structure of samples from the research area of the Yan’an gas field.
5.3 Adsorption-free ratio based on isotope fractionation
Controlled by the pore structure of the reservoir, the main occurrence modes of methane in coal rock are dissociation and adsorption. As an important parameter affecting the evaluation of coal–rock gas resources, the ratio of free gas to adsorbed gas is always the focus of research. The flow-suction ratio is a key parameter to evaluate the producibility and economic value of shale gas reservoirs. The quantitative evaluation of gas content by predecessors mainly focuses on experimental testing and analysis of gas content and lacks systematic analysis and research on the proportional relationship between free gas and adsorbed gas. Most research results are qualitative analysis and lack quantitative calculation.
The content of adsorbed gas mainly depends on the adsorption capacity. The stronger the adsorption capacity of the rock, the more significant the isotope fractionation during the desorption process (Xia and Tang, 2012). Free state is an important occurrence state of deep coalbed methane. It is of great significance to analyze the main controlling factors affecting free gas content for the evaluation of favorable areas and the formulation of exploration and development plans. The isotope fractionation characteristics of coalbed methane in the field analysis process are closely related to the gas content and the ratio of adsorbed gas/free gas, which provides a new way for the accurate evaluation of gas-bearing parameters of coal rock (Li et al., 2022; Li et al., 2023). The methane carbon isotope values of free gas and adsorbed gas in coalbed methane are obviously different. Usually, the methane carbon isotope value of free gas is lighter than that of adsorbed gas (Liu, 2017). Some scholars have analyzed the production data on shale gas production wells and the carbon isotope data on methane. It is believed that with the extension of development time, the production of free gas decreases, and the carbon isotope of methane tends to increase. Coalbed methane has the same characteristics (Xia and Tang, 2012; Li et al., 2020).
In this study, the carbon isotope value of methane showed significant isotope fractionation during the analysis of coalbed methane (Figure 10). As the analysis time increases, the carbon isotope value of methane gradually becomes heavier. According to the on-site analytical data on coal seams, different samples show the phenomenon of carbon isotope fractionation at different analytical stages. The value of δ13C1 in the Yan’an gas field is distributed in −35.47∼-29.36% at the normal temperature stage, with an average value of −32.20%. It is distributed in −32.47∼-22.99% at the geothermal δ13C1 stage, with an average value of −28.10%. It is distributed in −28.55∼-20.17% at high temperature stage, with an average value of −24.50%. In other words, δ13C1 has an increasing trend with the increase in analytical time and temperature and has a good correlation.
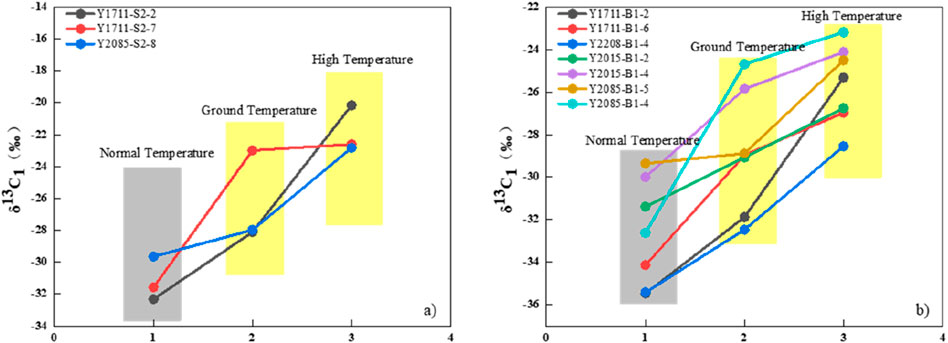
Figure 10. Changes in methane isotopes in different analytical stages of coal and rock in the Yan’an gas field.
Carbon isotope fractionation is a common phenomenon in the development of coalbed methane and shale gas. According to this phenomenon, some research has established prediction models for parameters such as the gas content and desorption ratio (Liu et al., 2016; Liu, 2017). In order to verify the method of estimating the occurrence state of coalbed methane by combining the above isotope data with the field desorption experiment, we applied this method to six key exploration wells in the Yan’an gas field. We know that in the process of field analysis, the lost gas escaped from the core drilling to the surface until the process of filling the tank should also be dominated by free gas.
It is assumed that the gas in the first desorption stage during the on-site analysis process is mainly free gas, so the lost gas that the core escapes during the process of lifting the drill to the surface until the tank is filled should also be dominated by free gas. Based on this assumption, the free gas content of the total gas content can be calculated using Equation 1:
It is assumed that in the first stage of the field desorption process, that is, the normal temperature stage, the gas released by the core is mainly free gas, so the methane carbon isotope value measured at this stage is the isotope value of free gas. In the second stage, that is, the geothermal stage, we believe that it is mainly a mixture of free gas and adsorbed gas, so the carbon isotope value of methane measured at this stage is the isotope value of the mixture. In the third stage, that is, the high temperature stage, we believe that it is mainly composed of adsorbed gas, and the carbon isotope value of methane measured in this stage is the isotope value of adsorbed gas. We can calculate the proportion of free gas and adsorbed gas in desorption according to the carbon isotope values of methane in these three stages according to Equation 2.
In the analysis stage of the six key exploration wells, the proportion of free gas is 4.24–90.31%, with an average of 48.04%; the free gas content in the total gas content is 0.76–15.76 m3/t, the average content is 8.33 m3/t, the ratio of free gas to adsorption is between 0.04 and 9.32, and the average wing-to-suction ratio is 1.95 (Figure 11), which can show the main occurrence state of coalbed methane in coal rock; the results show that the coal seam is rich in free gas, basically consistent with the on-site analytical curve. Free gas accounted for most of the total gas content. Different from shallow coal rock, with the deepening of buried depth, the gas content of coal seam changes from adsorbed gas greater than free gas to free gas greater than adsorbed gas. When the total gas content is high and the swing-to-suction ratio is large, it undoubtedly constitutes the best development conditions. The research results show that the Yan’an gas field has a high total gas content and a large desorption ratio, indicating that the deep coalbed methane in the Yan’an gas field has good development conditions.
6 Main controlling factors of the gas-bearing property of deep coal rock
Gas content in coal reservoir is the key geological factor to calculate the amount of coalbed methane resources. The level of gas content is directly related to the potential of coalbed methane exploration and development. The evaluation of the gas content of deep coalbed methane has always been highly valued as a key content. Coalbed methane exists in coal rock in three forms, namely, free, adsorbed, and dissolved, among which adsorbed gas dominates deep coalbed methane in the Ordos Basin, and some of it is stored in the form of free gas in the pores and fractures of coal seams, while a small part exists in the form of dissolved gas in formation fluids (Song et al., 2020; Mou et al., 2021). The geochemical characteristics, mineral content, and reservoir characteristics of coal rock affect the occurrence state of coalbed methane, which, in turn, has an important impact on the gas content of coalbed methane. By exploring the main controlling factors affecting the gas content and suction and drainage ratio of coalbed methane, the gas content of deep coal seam is evaluated.
6.1 Effect of organic geochemical on the gas-bearing property
The total organic carbon content of deep coal and rock in the Shanxi Formation of the Ordos Basin is between 26.37 and 85.75%, with an average of 65.20%. The total organic carbon content of deep coal and rock in the Benxi Formation is between 53.97 and 93.39%, with an average of 75.60%. The average total organic carbon content of coal and rock in the Benxi Formation is higher than that in the Shanxi Formation. The total organic carbon of coal and rock is generally high, and the abundance of organic matter is high. The pyrolysis hydrocarbon generation potential (S1 + S2) of coal rock samples in the Shanxi Formation is mainly between 3.22 and 10.00 mg/g, and the pyrolysis hydrocarbon generation potential (S1 + S2) of individual samples is as high as 55.45 mg/g. The pyrolysis hydrocarbon generation potential (S1 + S2) of Benxi Formation coal rock samples is mainly between 4.66 and 26.87 mg/g. In general, the pyrolysis hydrocarbon generation potential of Shanxi Formation coal rock and Benxi Formation coal rock is generally high, both of which have good hydrocarbon generation potential. The vitrinite reflectance is between 1.81% and 2.96%, which is generally in the high-over mature stage, and its overall metamorphic degree is high, mainly bituminous coal. Through the comprehensive analysis of rock pyrolysis experiments and optical macerals, the organic matter type of the Shanxi Formation coal seam and Benxi Formation coal seam is mainly type III of the partial humic type, and the coal seam has great potential for gas generation.
In the process of burial diagenesis, organic matter is the main internal factor controlling the adsorption performance of coal rock. The total gas content of coal rock samples on the whole increases with the increase in the TOC content (Figure 12), indicating that the higher the organic matter content, the higher the gas content of coal rock samples, indicating that the TOC content contributes greatly to the pore development of coal rock. Because kerogen has a strong ability to adsorb gas and the higher the maturity of organic matter, the more developed the pores involved in the formation of kerogen and the higher the gas content. With the increase in the TOC content, the adsorption ratio of coal and rock samples increases as a whole, which indicates that with the increase in the organic matter evolution degree of coal and rock samples, the main gas in coal seam samples gradually changes to the coexistence of adsorbed gas and free gas. The relationship between the TOC and wing-to-suction ratio of the Y2085-B1-5 coal sample is obviously inconsistent with the rule, mainly because the TOC content is low, the vitrinite content is small, and its micropores are relatively undeveloped, so the free gas content is high.
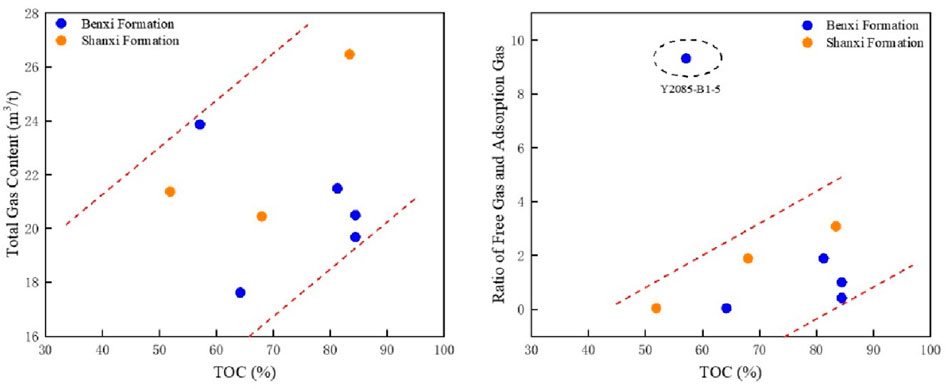
Figure 12. Relationship between the total gas content and migration ratio of coal rock samples and geochemical parameters.
6.2 Effect of minerals on the gas-bearing property
The mineral composition of deep coal rock in the Yan’an gas field of the Ordos Basin is mainly clay minerals and quartz. The content of clay minerals is high, ranging from 12.80% to 91.1%, with an average of 56.32%, and the range of variation is large. The composition of clay minerals usually reflects the diagenetic evolution stage and sedimentary environment of coal rock, indicating that its sedimentary environment and source supply change greatly. The content of clay minerals plays an important role in the study of gas adsorption capacity and gas content of coal rock. On one hand, clay minerals have a certain ability to adsorb methane gas. On the other hand, clay minerals are fine in particle size, which will fill in the pores formed by quartz and other minerals, reduce porosity, and then affect the gas content. The quartz content of brittle minerals is between 0.90 and 30.5%, with an average of 5.24%. The content of brittle minerals is low, indicating that the brittleness of coal and rock in the study area is low and not easy to break. The clay mineral content of coal and rock in the Yan’an gas field of the Ordos Basin is mainly illite, kaolinite, illite–montmorillonite mixed layer, and chlorite. The content of kaolinite is the highest, ranging from 5 to 100%, with an average of 55%, and the content of kaolinite in some clay minerals reaches 100% (Figure 13).
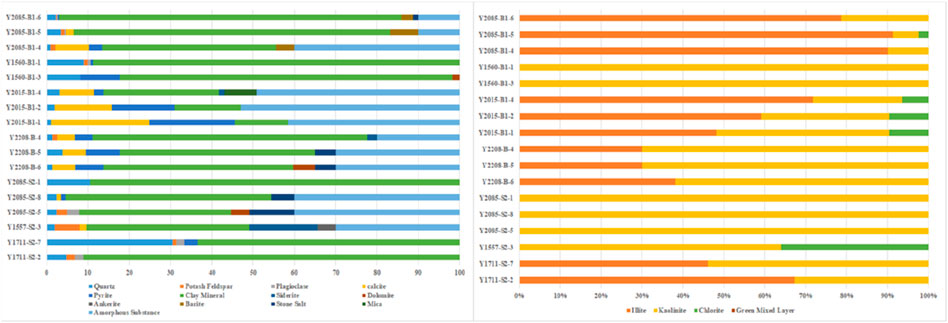
Figure 13. Coal and rock mineral composition (%) and clay mineral composition (%) in the study area.
According to the analysis of the correlation between different mineral components and gas content (Figure 14), the total gas content of coal rock shows a downward trend with the increase in the clay mineral content, which is because clay minerals will fill some pores, reducing the adsorption space of coalbed methane, thereby reducing the gas content of coal rock (Zhu et al., 2021; Gao et al., 2023). The correlation between clay minerals and the migration ratio is not obvious, which may be due to the fact that the migration ratio is controlled by a variety of factors, and coalbed methane is mainly in the form of adsorbed gas and free gas in the deep coal rock. The content of the two is always in a dynamic equilibrium process. The change in any factor will lead to the change in the content of adsorbed gas and free gas, which will lead to the change in the migration ratio. The total gas content of coal rock increases first and then decreases with the increase in the quartz content. This is mainly because quartz can support pore space in coal rock. The higher the content of quartz, the greater the space provided for free gas storage and the higher the gas content. With the progress in metamorphism, quartz may also fill pores and occupy the space for free gas storage, resulting in a decrease in the total gas content of coal rock. The gas content of coal rock increases first with the increase in illite content and decreases with the decrease in kaolinite content. This is because the specific surface area of illite is large, and the adsorbed coalbed methane content is higher, so the gas content increases with the increase in the illite content. The specific surface area of illite is larger than that of kaolinite, so the total gas content of coalbed methane decreases with the increase in the kaolinite content. The relationship between sample Y2085-S2-8 illite, kaolinite and total gas content, and the migration ratio is obviously not in accordance with the law, mainly because the clay minerals of the selected samples are all kaolinite, resulting in the development of sample pores and the increase in the total gas content.
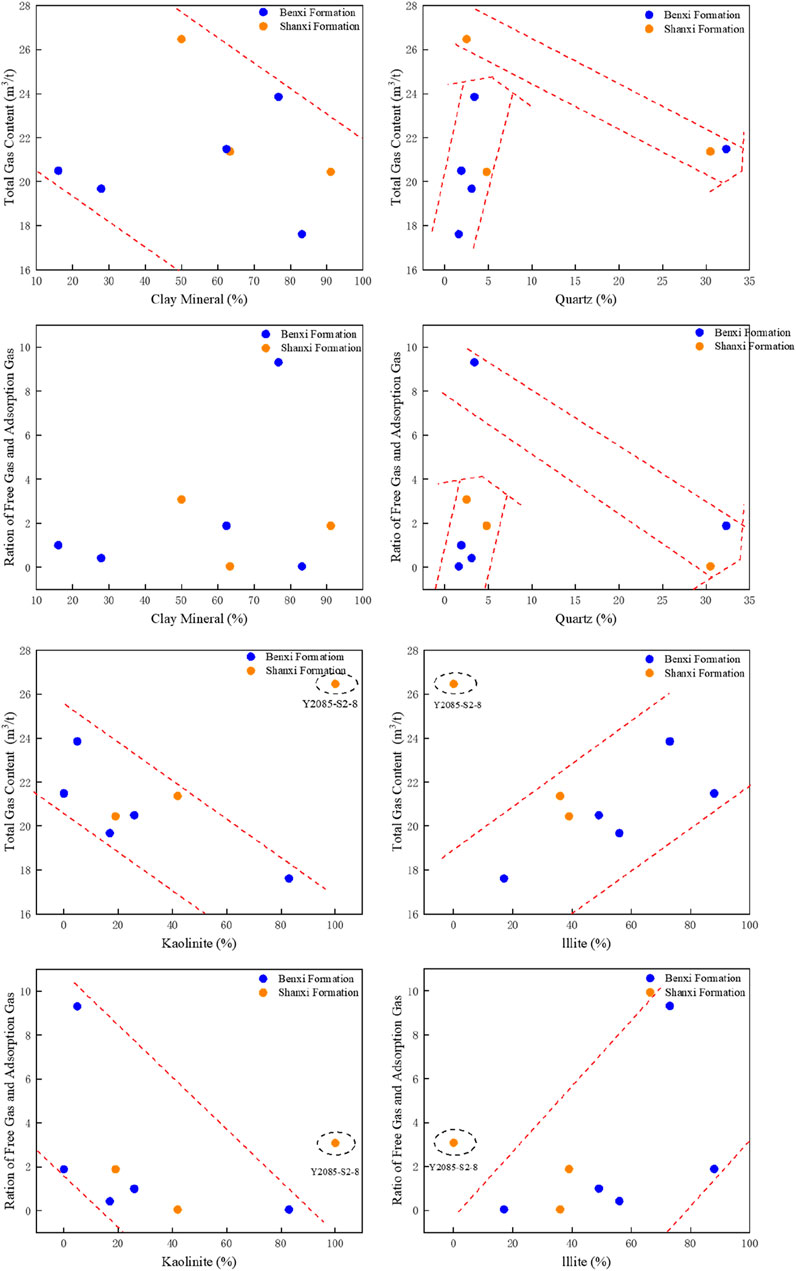
Figure 14. Relationship between the content of mineral components in coal and rock and the total gas content and the ratio of migration and absorption in the study area.
6.3 Effect of reservoir space on the gas-bearing property
Scanning electron microscopy (SEM) was used to observe the coal rocks in the study area, and it was found that microcracks, solution pores, intergranular pores, and a large number of pores were mainly developed (Figure 15). Dissolution pores are generally developed in the interior and edge of minerals. They are mainly pores formed by the dissolution of clay minerals and brittle minerals in the process of sedimentary burial and diagenesis. The shape is irregular, triangular, and angular. The pore size is generally in nanometer. The pore size is distributed from tens of nanometers to several microns and generally has good connectivity. The development of micro-fractures is the result of both internal and external factors (Zhang et al., 2018; Li et al., 2022; Zhao et al., 2024). With the development of micro-fractures, the free gas volume tends to increase. Micro-fractures can provide a large amount of reservoir space and provide space conditions for the enrichment of deep coalbed methane (Deng et al., 2024). The pores in coal rock are mainly the pores left by gas escape during coalification, which have a great influence on the pore volume of coal rock, and exist in coal and coal rock components of various metamorphic degrees. Generally, it appears in a single form and can be densely grouped when the gas-forming effect is strong. It is disorderly arranged in different sizes, and the outline is smooth. The shape is mostly circular and elliptical, and the larger one can be harbor-shaped. There are mainly mesopores and macropores and some transition pores (Huo et al., 2024). Because vitrinite is the main microcoal-rock type in the Yan’an gas field and vitrinite has strong hydrocarbon generation capacity, pores are more common in vitrinite. The pores not only provide the main adsorption space for coalbed methane but can also accommodate a large amount of free gas.
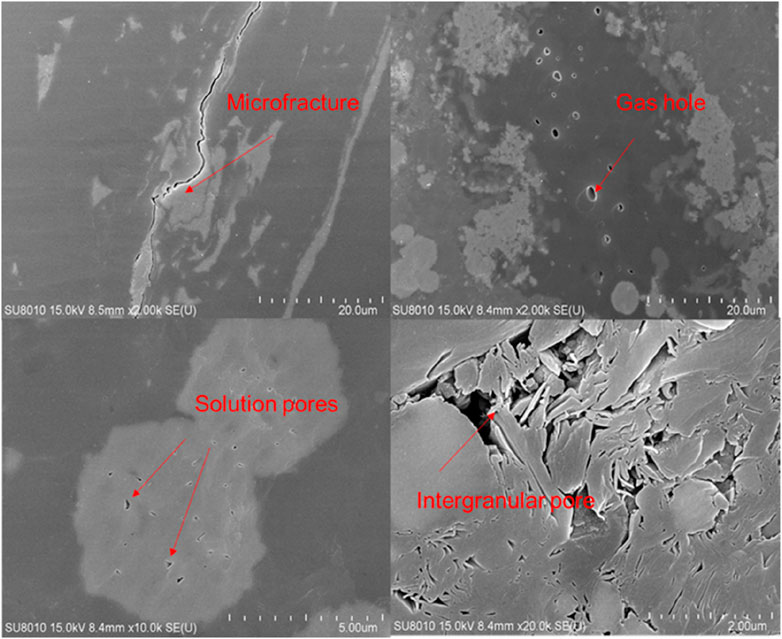
Figure 15. SEM image of common pore cracks in samples from the research area of the Yan’an gas field.
The research on the pore structure mainly includes three commonly used methods: the mercury intrusion method, nitrogen adsorption method, and carbon dioxide adsorption method (Li et al., 2019; Zeng et al., 2019). Previous studies have also shown that the mercury intrusion method mainly characterizes the pore size of >50 nm, the nitrogen adsorption method mainly characterizes the pore size of 2–50 nm, and the carbon dioxide adsorption method mainly characterizes the pore size of <2 nm. Mercury intrusion experiments, nitrogen adsorption experiments, and carbon dioxide adsorption experiments can quantitatively characterize the microscopic pore structure of coalbed methane reservoirs. However, due to different principles of different experimental methods, their test ranges and results are also different. By effectively fusing the pore size information on different scales represented by these experimental results, the pore size distribution curve that objectively characterizes the microscopic pore structure of the reservoir can be obtained.
The mercury intrusion method is suitable for the determination of the pore structure of various massive coal rock samples. From the pressure-cumulative mercury saturation curve of coal samples in the study area, the shape of the curve can reflect the distribution characteristics of each pore throat and the quality of pore connectivity (Lin et al., 2019; Ma et al., 2016). The overall curve shape is basically the same. The mercury injection curve of coal sample has the characteristics of gentle front end and steep back end (Figure 16). When the mercury injection pressure is less than 10 MPa, the mercury injection speed is slow, and the corresponding pore diameter is mainly developed. Micron-sized pore fissures indicate that the pore fissures in this section are relatively undeveloped; when the pressure reaches approximately 100 MPa, the amount of mercury intake increases rapidly, indicating that there are a large number of nano-scale pores in the coal sample. The maximum mercury removal efficiency is between 24.89 and 91.17%, with an average of 69.38%. The higher the mercury removal efficiency, the better the pore connectivity of coal. The mercury removal efficiency of coal samples in the study area is higher, indicating that the pores in coal samples are mostly open pores and have good connectivity. The pore separation coefficient of coal is between 1.12 and 4.97, with an average of 3.08. The smaller the pore separation coefficient is, the more uniform the pore throat is. The pore separation coefficient of the Ordos Basin is larger, and the pore throat distribution is not uniform. The pore size of coal samples is mainly developed between 10 and 100 nm. The pore structure of coal reservoirs in this area is complex and characterized by the development of large- and medium-sized pores. The porosity and permeability of coal and rock in the study area are tested. The porosity of coal and rock is between 5.45 and 7.40%, with an average of 6.53%. The permeability is between 0.18 and 10.79 mD, with an average of 3.02 mD. The porosity and permeability of the whole coal rock are small, which is conducive to the enrichment, accumulation, and extraction of coal seam methane.
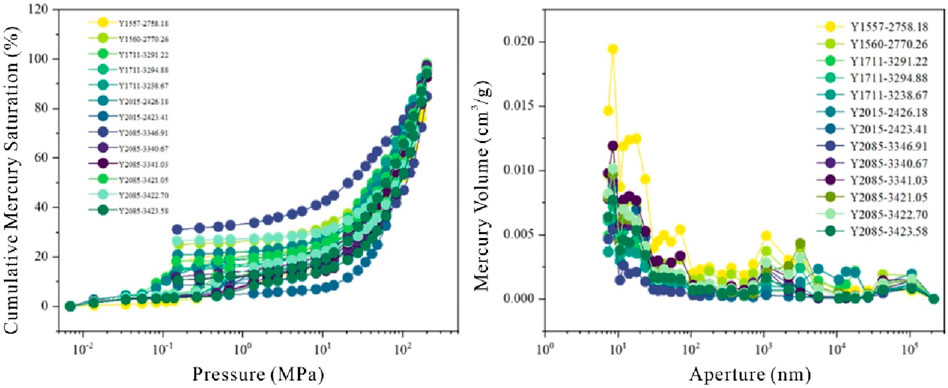
Figure 16. Mercury inlet and outlet curve and pore diameter distribution curve of samples from the Yan’an gas field.
The high-pressure mercury injection experiment can characterize the distribution characteristics and pore connectivity of the pore channel. Figure 17 shows that with the increase in the mercury removal efficiency, the total gas content of coal rock increases, indicating that with the increase in pore connectivity of coal rock, coalbed gas is more likely to enter the pores and be adsorbed, resulting in the increase in the total gas content. However, with the increase in rock porosity, the total gas content of coal rock decreases, which indicates that with the increase in porosity, the pore space of coal rock increases, and the heterogeneity is enhanced, and the coalbed methane is easy to escape, thus escaping from the pores and reducing the total gas content of coal rock. The correlation between pore structure parameters and the ratio is not obvious. This is mainly because the content of free gas and adsorbed gas is dynamic, which is affected by many factors. The ratio will maintain a relatively stable change, so the influence of pore structure parameters on the ratio is relatively not obvious. The relationship between the pore structure parameters of the Y2085-B1-5 coal sample and the suction ratio is obviously not in accordance with the law, which is mainly because the vitrinite content of the coal sample is less, and its micropores are relatively underdeveloped, so the free gas content is higher.
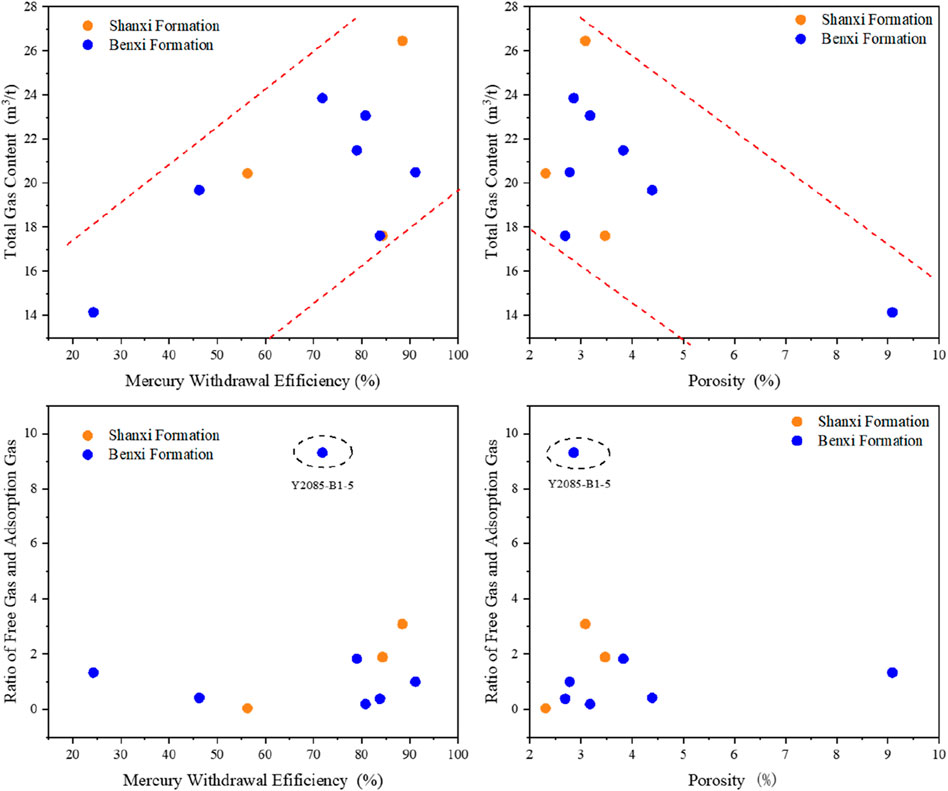
Figure 17. Correlation diagram of pore structure parameters with a total gas content and suction ratio in the Yan’an gas field.
From the low-temperature N2 adsorption–desorption curve of coal samples in the study area, the adsorption curves of all coal samples are slightly different in shape, but the whole is inverted ‘S' type (IUPA, 1994). Previous studies have found that the pore structure development of coal rock can be inferred according to the nitrogen adsorption–desorption curve and the shape of the hysteresis loop. When p/p0>0.5, the nitrogen adsorption branch line and desorption branch line do not coincide, forming a hysteresis loop. The nitrogen adsorption branch of coal samples in the study area is very steep at the saturated vapor pressure (p0), while the desorption branch is similar to the adsorption branch, and it is also very steep at the saturated vapor pressure (p0). When p/p0<0.5, the desorption branch and the adsorption branch tend to overlap, and the hysteresis loop between the two is narrow. This kind of curve is close to the H3 type proposed by IUPAC and has the characteristics of the H4 type at the same time. It shows that a large number of nano-scale pores are developed in the coal and rock of the Shanxi Formation and Benxi Formation in the Ordos Basin, and the pore types are mainly conical pores, ink bottle pores, and slit pores.
Coal rock is a kind of porous adsorption rock with strong heterogeneity. Pore volume and pore-specific surface area are key indicators for the coal mesoporous structure, and their distribution characteristics are of great significance for reservoir research and coalbed methane exploration. The specific surface area is the pore area size of unit mass coal, which is an important parameter to reflect the adsorption performance of coal and rock to methane. The specific surface area is positively correlated with the adsorption capacity of methane, and the larger the area, the greater the adsorption capacity. The low-temperature N2 adsorption data were analyzed based on the DFT model (Figure 18). The results show that the pore volume and pore-specific surface area of the coal samples in the pore size range of 1–36 nm are mainly provided by 1–7-nm pores. The DET pore volume range of coal rock in the study area is 0.02–0.09 cm3/g, with an average of 0.06 cm3/g. The total specific surface area range is 54.12–256.91 m2/g, with an average of 175.44 m2/g, which is mainly composed of the micropore volume and small pore volume (Figure 18). As shown in Figure 19, the specific surface area and total pore volume of deep coal rock are positively correlated with the total gas content, that is, with the increase in the total specific surface area and total pore volume of coal rock, the total gas content shows an increasing trend. This is because with the increase in the total specific surface area and total pore volume of coal rock, the adsorption sites and adsorption spaces of gas in coal rock also increase. The content of adsorbed gas and free gas increased, resulting in a positive correlation between the total gas content and total specific surface area and total pore volume. The correlation between the specific surface area and total pore volume of deep coal and rock is not obvious, which is because the macropores and micropores of coal and rock in the study area provide the main storage space for coalbed methane.
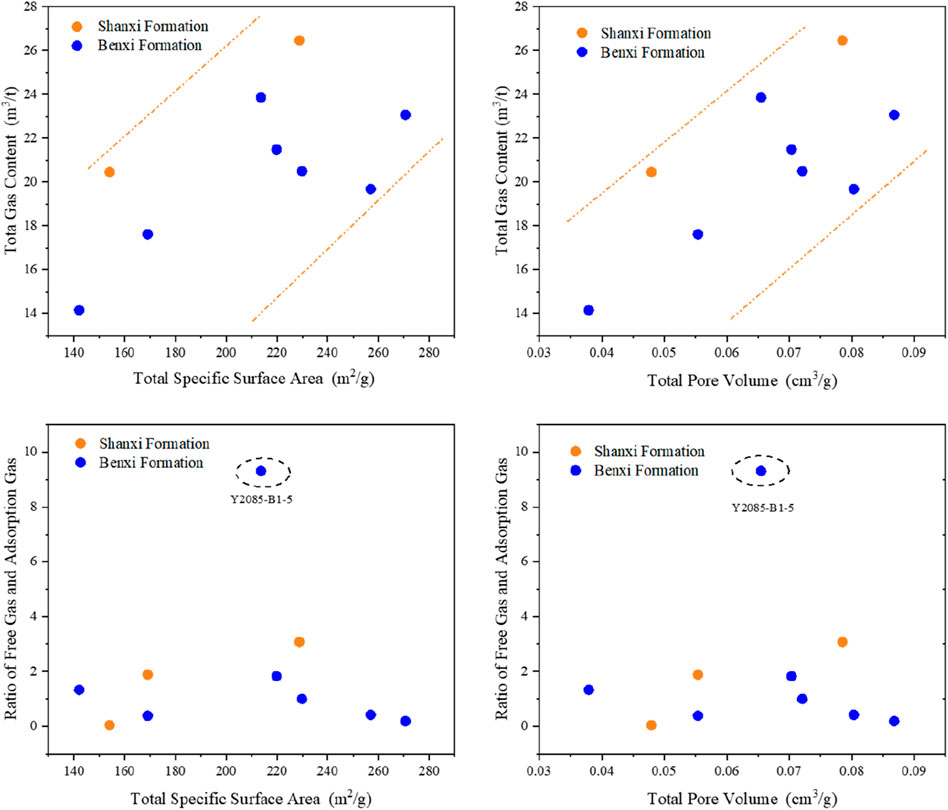
Figure 19. Correlation diagram of pore structure parameters with a total gas content and suction ratio in the Yan’an gas field.
Previous studies on the adsorption capacity of coal have focused on mesopores and macropores (Wang et al., 2023) but have not paid enough attention to micropores with pore size less than 2 nm. The experimental test of carbon dioxide adsorption on coal samples was carried out. The pore volume and pore-specific surface area parameters of coal were obtained based on DFT theory, and the coal samples of the Shanxi Formation and Benxi Formation in the Ordos Basin were analyzed. The results show that the specific surface area and total pore volume of coal seam micropores are mainly provided by 0.50–0.70 nm pores, and the total pore volume distribution range is 0.0007–0.0056 cm3/g, with an average of 0.0016 cm3/g. The total specific surface area distribution range is 0.1845–2.3741 m2/g, with an average of 0.7233 m2/g. The micropore development of coal samples is generally multimodal, with peaks distributed in 0.30–0.40 nm, 0.40–0.70 nm, and 0.80–0.90 nm (Figure 20).
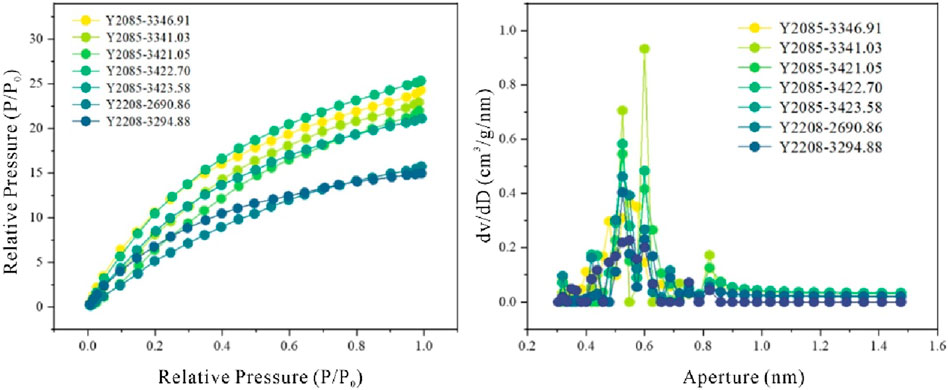
Figure 20. Isothermal curve and pore diameter distribution curve of carbon dioxide adsorption and desorption samples in the Yan’an gas field.
The total gas content of coal and rock is negatively correlated with the total pore volume and specific surface area (Figure 21). This is because the occurrence state of deep coalbed methane in Yan’an gas field is mainly free gas. With the development of micropores in coal and rock, the pores in this part have a large specific surface area, providing a large number of adsorption sites for gas occurrence. The free gas in deep coal rock will be more converted into adsorbed gas, the relative proportion of free gas will be reduced, and the total resolved gas content will be reduced. However, the change law of the swing-absorption ratio is relatively not obvious because the content of adsorbed gas and free gas in deep coal rocks is dynamically changing, and the pore structure of deep coal rocks is complex, which has a relatively insignificant influence on the gas content of deep coal rocks.
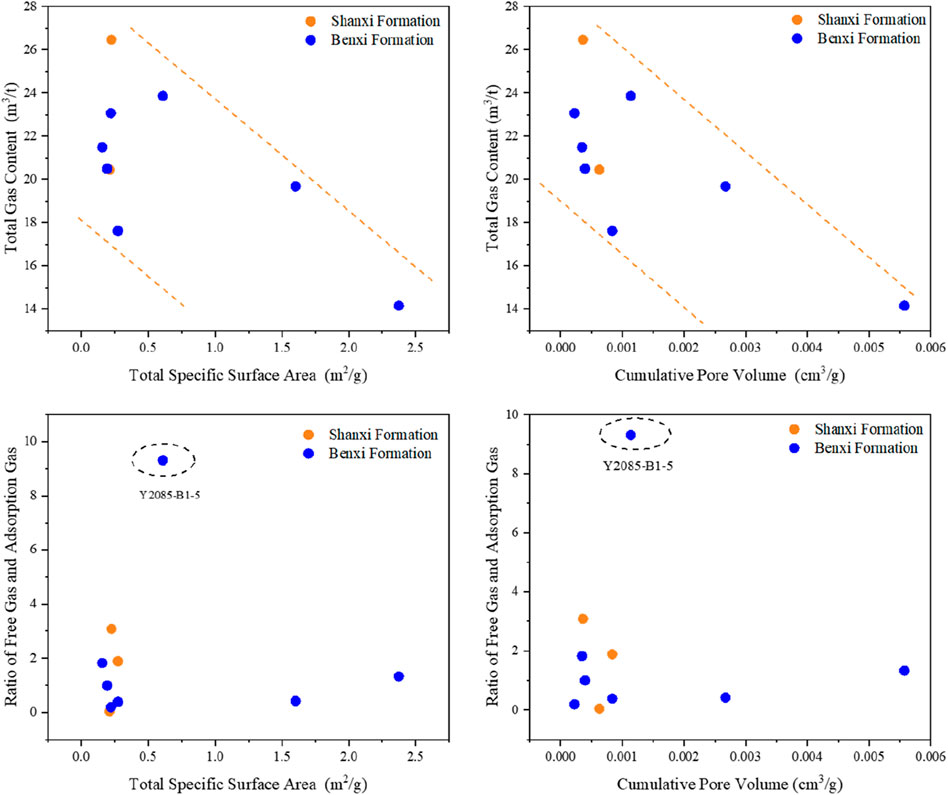
Figure 21. Correlation of pore structure parameters with a total gas content and suction ratio in the Yan’an gas field.
The pore structure of the Y2085-B1-5 coal rock sample is complex, and its gas content is affected by the coupling of macropores, mesopores, and micropores. This multi-stage pore structure not only increases the gas storage capacity of coal rock but also makes the adsorption and migration of coalbed methane more complicated. It is worth noting that the organic matter content of the sample is relatively low, which may affect its natural gas generation potential. However, what is inconsistent with the organic matter content is its abnormally high free gas content, which may be due to the fact that the mesopores and macropores of coal rock samples are more developed than the micropores, resulting in the abnormally high free gas content. This discovery has some practical significance because it suggests that even in coal seams with low organic matter content, there may be rich recoverable natural gas resources.
7 Conclusion
This study selects the deep coal rock of the Yan’an gas field in the southeast part of the Ordos Basin as the research subject and accomplishes the gas-bearing assessment of deep coal rock. The following insights have been acquired:
(1) The Yan’an gas field predominantly develops two sets of primary coal seams in the Shanxi Formation and Benxi Formation, with a burial depth exceeding 2,000 m, representing a typical deep coalbed methane field. The mineral constituents of the coal rock in the Yan’an gas field mainly consist of quartz and clay minerals. The total organic carbon content is high, the pyrolysis hydrocarbon generation potential is relatively elevated, and the overall status is within the stage of high maturity to over-maturity, featuring good hydrocarbon generation potential.
(2) Microfractures, dissolution pores, and intergranular pores are predominantly developed in the deep coal rocks of the Yan’an gas field. Dissolution pores are generally developed within and at the edges of minerals, with pore sizes ranging from tens of nanometers to several microns. Microfractures are relatively well-developed, which constitute a favorable preservation space and migration channel for deep coalbed methane.
(3) The total gas content of the coal rock in the deep Yan’an gas field is high. By combining the carbon isotope distribution model and gas content distribution model, the proportion and content of free gas in coalbed methane were calculated, and the free adsorption ratio was obtained, revealing the main occurrence state of coalbed methane in the deep coal rocks, which was largely consistent with the field analytical curve, and the free gas constituted the majority of the total gas content.
(4) Multiple factors influence the gas content of deep coal rocks in the Yan’an gas field. The content of organic matter exhibits a favorable positive correlation with the gas content of deep coal rocks. Mineral components affect the gas content by participating in pores. There is also a correlation between the reservoir space and the gas content. With the increase in the pore connectivity of coal rocks, coalbed methane is more likely to enter the pores, be adsorbed, and also be more likely to escape. The adsorption sites and adsorption spaces of coal and rock increase with the increase in mesoporous-specific surface area and total pore volume. The development of micropores facilitates the conversion of free gas into adsorbed gas more easily, thereby reducing the proportion of free gas. Various geological factors impact the gas content of deep coal rocks in the Yan’an gas field.
Data availability statement
The data analyzed in this study are subject to the following licenses/restrictions: only for this study. Requests to access these datasets should be directed to Shuangbiao Han,YmpjdXBoYW5AMTYzLmNvbQ==.
Author contributions
YW: writing–original draft. ZW: writing–original draft. DH: writing–review and editing. YW: writing–review and editing. MH: writing–review and editing. XM: writing–review and editing. SH: writing–review and editing.
Funding
The author(s) declare that financial support was received for the research, authorship, and/or publication of this article. This research was supported by the Fundamental Research Funds for the Central Universities (Grant No. 2023ZKPYDC07).
Acknowledgments
The editors and reviewers are gratefully acknowledged.
Conflict of interest
Authors WY, W, and H were employed by Gasfield Company of Shaanxi Yanchang Petroleum Group Co., Ltd.
The remaining authors declare that the research was conducted in the absence of any commercial or financial relationships that could be construed as a potential conflict of interest.
Publisher’s note
All claims expressed in this article are solely those of the authors and do not necessarily represent those of their affiliated organizations, or those of the publisher, the editors, and the reviewers. Any product that may be evaluated in this article, or claim that may be made by its manufacturer, is not guaranteed or endorsed by the publisher.
References
Chen, G. (2016). Adsorption characteristics and influencing factors of deep low rank coal in Cainan area of Junggar Basin. Coal Geol. Explor 44, 50–54. doi:10.3969/j.issn.1001-1986.2016.02.010
Cui, C. (2022). Logging identification and quantitative evaluation of macroscopic coal rock types. Beijing: China University of Geosciences. doi:10.27493/d.cnki.gzdzy.2021.001597
Deng, Z., Wang, H., Jiang, Z., Ding, R., Li, Y., and Wang, T. (2024). The influence of pore and fracture structure of deep coal reservoir on coalbed methane occurrence-Taking Daning-Jixian block in the eastern margin of Ordos Basin as an example. Coal Sci. Technol., 1–18. doi:10.12438/cst.2023-1334
Gao, J., Li, X., Cheng, G., Luo, H., and Zhu, H. (2023). Structural evolution and characterization of organic-rich shale from macroscopic to microscopic resolution: the significance of tectonic activity. Adv. Geo-Energy Res. 10, 84–90. doi:10.46690/ager.2023.11.03
Geng, M., Chen, H., Chen, Y., Zeng, L., Chen, S., and Jiang, X. (2018). The fourth round of national CBM resource evaluation methods and results. Coal Sci. Technol. 46, 64–68. doi:10.13199/j.cnki.cst.2018.06.011
Hou, W., Zhang, J., and Ma, Z. (2016). The occurrence and accumulation of the coal-bed methane. Ground Water 38, 231–234. doi:10.3969/j.issn.1004-1184.2016.02.088
Huo, Z., Xi, Z., Guo, J., and Wang, D. (2024). Pore characteristics of tectonic coal based on nitrogen adsorption and SEM. Coal Eng. 56, 149–156. doi:10.11799/ce202403023
IUPAC (International Union of Pure and Applied chemistry). (1994). “Physical chemistry division commission on colloid and surface chemistry, subcommittee on characterization of porous solids: recommendations for the characterization of porous solids (technical report)”, in paper present at the Pure Appl Chem Meeting. 66, 1739–1758.
Jiao, K., Yao, S. P., Liu, C., Gao, Y. Q., Wu, H., Li, M., et al. (2014). The characterization and quantitative analysis of nanopores in unconventional gas reservoirs utilizing FESEM-FIB and image processing: an example from the lower Silurian Longmaxi Shale, upper Yangtze region, China. Int. J. Coal Geol. 128, 1–11. doi:10.1016/j.coal.2014.03.004
Li, W., Lu, S., Li, J., Wei, Y., Zhao, S., Zhang, P., et al. (2022). Advances in isotope fractionation during shale gas/coalbed methane migration. Pet. Explor. Dev. 49, 929–942. doi:10.11698/PED.20220225
Li, W., Lu, S., Li, J., Zhang, P., Wang, S., Feng, W., et al. (2020). Carbon isotope fractionation during shale gas migration: mechanism, characterization and significance. CSCD 50, 553–569. doi:10.1360/N072019-0156
Li, X., Gao, J., Zhang, S., Li, Y., Wang, M., and Lu, W. (2022). Combined characterization of coal pore structure based on scanning electron microscopy, pore-fracture analysis system and gas adsorption. J. China Univ. Geosci. 47, 1876–1889. doi:10.3799/dqkx.2021.195
Li, Y., Gao, S., Wu, P., Xu, L., Ma, L., Hu, W., et al. (2023). Evaluation and correction of prediction model for free gas content in deep coalbed methane-a case study of deep coal seams in the eastern margin of Ordos Basin. Acta Pet. Sin. 44, 1892–1902. doi:10.7623/syxb202311011
Li, Y., Zhang, J., Li, J., He, J., Sun, G., and Qiao, Y. (2014). Sedimentary microfacies characteristics of Carboniferous Benxi Formation in southeastern Ordos Basin and its control on natural gas enrichment. Northwest. Geol. 47, 216–222. doi:10.3969/j.issn.1009-6248.2014.02.027
Li, Z. T., Liu, D. M., Cai, Y. D., W, Y., and J, T. (2019). Adsorption pore structure and its fractal characteristics of coals by N2 adsorption/desorption and FESEM image analyses. Fuel 257, 116031. doi:10.1016/j.fuel.2019.116031
Lin, H., Bu, J., Yan, M., and Bai, Y. (2019). Combined analysis of pore structure characteristics of medium and low rank coal by nitrogen adsorption method and mercury intrusion method. J. Xi'an Univ. Sci. Technol. 39, 1–8. doi:10.13800/j.cnki.xakjdxxb.2019.0101
Liu, Y. (2017). Geochemical genetic model and application of natural gas in high-over mature shale. Beijing: China University of Geosciences.
Liu, Y., Zhang, J. C., and Tang, X. (2016). Predicting the proportion of free and adsorbed gas by isotopic geochemical data: a case study from lower Permian shale in the southern North China basin (SNCB). Int. J. Coal Geol. 156, 25–35. doi:10.1016/j.coal.2016.01.011
Lu, J., Shao, L., Yang, M., Li, Y., Zhang, Z., Wang, S., et al. (2014). Coal facies evolution, sequence stratigraphy and paleoenvironment of swamp system in continental basin. J. China Coal Soc. 39, 2473–2481. doi:10.13225/j.cnki.jccs.2013.1779
Ma, Y., Pan, Z., Zhong, N., Connell, L. D., Down, D. I., Lin, W. L., et al. (2016). Experimental study of anisotropic gas permeability and its relationship with fracture structure of Longmaxi shales, Sichuan Basin, China. Fuel 180, 106–115. doi:10.1016/j.fuel.2016.04.029
Mou, P., Pan, J., Niu, Q., Wang, Z., Li, Y., and Song, D. (2021). Coal pores: methods, types, and characteristics. Energy fuels. 35, 7467–7484. doi:10.1021/acs.energyfuels.1c00344
Niu, X., Zhao, W., Shi, Y., Hu, X., and Du, X. (2023). Natural gas accumulation conditions and exploration potential of Benxi Formation in Ordos Basin. Acta Pet. Sin. 44, 1240–1257. doi:10.7623/syxb202308004
Qin, Y., Shen, J., and Shi, R. (2022). Strategic value and strategic choice of coal measure gas industry construction in China. J. China Coal Soc. 47, 371–387. doi:10.13225/j.cnki.jccs.YG21.1616
Song, D., Ji, X., Li, Y., Zhao, H., Song, B., and He, K. (2020). Heterogeneous development of micropores in medium-high rank coal and its relationship with adsorption capacity. Int. J. Coal Geol. 226, 103497. doi:10.1016/j.coal.2020.103497
Sun, Q., Zhao, Q., Jiang, X., Mu, F., Kang, L., Wang, M., et al. (2011). Prospects and countermeasures of coalbed methane exploration and development in China under the new situation. J. China Coal Soc. 46, 65–76. doi:10.13225/j.cnki.Jccs.2020.1579
Wang, S. (1996). Coal accumulation law and coal resource evaluation in Ordos Basin. Coal Industry Press.
Wang, T., Tian, F. H., Deng, Z., and Hu, H. Y. (2023). The characteristic development of micropores in deep coal and its relationship with adsorption capacity on the eastern margin of the Ordos Basin, China. Minerals 13, 302. doi:10.3390/min13030302
Xia, X. Y., and Tang, Y. C. (2012). Isotope fractionation of methane during natural gas flow with coupled diffusion and adsorption/desorption. Geochim. Cosmochim. Acta 77, 77489–77503. doi:10.1016/j.gca.2011.10.014
Xu, Y., Wang, B., Zhao, X., Yun, J., Zhang, S., Wang, Y., et al. (2021). Thoughts and suggestions on promoting the high-quality development of China 's CBM business under the goal of ' double carbon '. China Pet. Explor 2, 9–18. doi:10.3969/j.issn.1672-7703.2021.03.002
Yu, X., Wang, X., Wang, N., Shan, X., Zhou, J., Han, X., et al. (2017). Upper Paleozoic sequence stratigraphic framework and sedimentary evolution characteristics of gas-bearing sand bodies in southeastern Ordos Basin. J. Palaeogeogr. 19, 935–954. doi:10.7605/gdlxb.2017.06.073
Zeng, W., Zhou, G., and Song, Z. (2019). Influence of pore structure on the shale oil accumulation of the Qingshankou Formation in the Songliao Basin. Geochimica 48, 632–643. doi:10.19700/j.0379-1726.2019.06.011
Zhang, J., Li, X., Wei, Q., Sun, K., Zhang, G., and Wang, F. (2017). Characterization of full-sized pore structure and fractal characteristics of marine-continental transitional longtan formation shale of sichuan basin, south China. Energy fuels. 31, 10490–10504. doi:10.1021/acs.energyfuels.7b01456
Zhang, J., Liu, S., Wei, X., Tang, X., and Liu, Y. (2021). Method for shale gas content evaluation. Oil Gas. Geol. 42, 28–40. doi:10.11743/ogg20210103
Zhang, J., Wang, X., Li, Z., Liu, S., Niu, J., Yuan, T., et al. (2024). Progress and development trend of field testing technology for shale gas content. Earth Sci. Front. 31, 315–326.
Zhang, P., Lu, S., Li, J., Xue, H., Li, W., and Wang, S. (2018). Quantitative characterization of microscopic pore structure of shale based on scanning electron microscopy. J. China Univ. Pet. 42, 19–28. doi:10.3969/j.issn.1673-5005.2018.02.003
Zhang, Q. (2022). Analysis of main controlling geological factors of deep coal seam gas production. Beijing: China University of Geosciences. doi:10.27493/d.cnki.gzdzy.2016.000183
Zhang, R., Wang, L., Liu, L., Hu, J., Du, J., Chen, H., et al. (2024). Source-to-sink filling process and paleogeographic pattern of the late carboniferous Benxi Formation in the eastern Ordos Basin. Acta Sedimentol. Sin., 1–22. doi:10.14027/j.issn.1000-0550.2023.099
Zhao, L., Wu, H., Huang, L., Zhao, J., Zhao, H., Er, C., et al. (2023). Provenance analysis of Shan 2 member of Shanxi Formation in Daning-Jixian block and its adjacent area, eastern margin of Ordos Basin. Northwest. Geol. 56, 322–331. doi:10.12401/j.nwg.2023132
Zhao, Q., Sun, F., and Li, W. (2011). Geological theory and practice of coalbed methane exploration and development. Petroleum Industry Press, 5–11.
Zhao, W., Liu, H., Wang, H., Liu, D., and Li, X. (2024). Discussion on pore characteristics and genesis of Benxi Formation coal in deep Ordos Basin-Taking 8 # coal of Yulin M172 well as an example. Nat. Gas. Geosci. 35, 02–216. doi:10.11764/j.issn.1672-1926.2023.10.012
Zhao, Z., Guo, Y., Wang, Y., and Lin, D. (2012). Research progress on tectonic evolution and paleogeographic characteristics of Ordos Basin. Spec. Oil Gas. Reserv. 19, 15–20+151. doi:10.3969/j.issn.1006-6535
Zhao, Z., Xu, W., Zhao, Z., Yi, S., Yang, W., Zhang, Y., et al. (2024). Geological characteristics and exploration breakthrough of coal-rock gas in Benxi Formation of carboniferous in Ordos Basin. Pet. Explor. Dev. 51, 234–247+259. doi:10.11698/PED.20230679
Zhu, H., Huang, C., Ju, Y., Bu, H., Li, X., Yang, M., et al. (2021). Multi-scale multidimensional characterization of clay-hosted pore networks of shale using FIBSEM, TEM, and X-raymicro-tomography: implications for methane storage and migration. Appl. Clay Sci. 213, 106239. doi:10.1016/j.clay.2021.106239
Keywords: Yan’an gas field, deep coalbed methane, gas-bearing characteristics, isotope fractionation, on-site analysis
Citation: Wan Y, Wang Z, Hu D, Wang Y, Huo M, Mu X and Han S (2024) Gas-bearing evaluation of deep coal rock in the Yan’an gas field of the Ordos Basin. Front. Earth Sci. 12:1438834. doi: 10.3389/feart.2024.1438834
Received: 26 May 2024; Accepted: 22 August 2024;
Published: 11 September 2024.
Edited by:
Hongjian Zhu, Yanshan University, ChinaReviewed by:
Ruyue Wang, State Key Laboratory of Shale Oil and Gas Enrichment Mechanisms and Efficient Development, ChinaFuhua Shang, Inner Mongolia University of Technology, China
Bhupati Neupane, Institute of Tibetan Plateau Research, Chinese Academy of Sciences (CAS), China
Copyright © 2024 Wan, Wang, Hu, Wang, Huo, Mu and Han. This is an open-access article distributed under the terms of the Creative Commons Attribution License (CC BY). The use, distribution or reproduction in other forums is permitted, provided the original author(s) and the copyright owner(s) are credited and that the original publication in this journal is cited, in accordance with accepted academic practice. No use, distribution or reproduction is permitted which does not comply with these terms.
*Correspondence: Zhenchuan Wang, MTI0NTkzMDUyQHFxLmNvbQ==; Shuangbiao Han, YmpjdXBoYW5AMTYzLmNvbQ==