- 1Key Laboratory of Land and Sea Ecological Governance and Systematic Regulation, Shandong Academy for Environmental Planning, Jinan, China
- 2Frontiers Science Center for Deep Ocean Multispheres and Earth System, and Key Laboratory of Marine Chemistry Theory and Technology, Ministry of Education, Ocean University of China, Qingdao, China
- 3Shandong Blue Ocean Technology Co., Ltd., Yantai, China
- 4College of Marine Geo-Science, Ocean University of China, Qingdao, China
- 5Qingdao Marine Geological Engineering Survey Institute Co., Ltd., Qingdao, China
- 6Qingdao Boyan Marine Environment Technology Co., Ltd., Qingdao, China
Introduction: The rapid advances in marine engineering projects are exacerbating environmental pressures on bay ecosystems. This study utilized the MIKE 21 model to evaluate the impacts of such projects in Laizhou Bay (LZB) on hydrodynamic conditions and the spread of dissolved inorganic nitrogen (DIN) from riverine inputs.
Results: The results indicated an expansion of 80.77 km2 in areas with DIN concentrations surpassing 0.5 mg/L 2 months after input from the Yellow River, with increased levels in the southern Yellow River Delta. Decreased flow velocities adjacent to the wave barriers at the Xiaoqing River estuary impeded lateral DIN dispersion, resulting in a 0.93 mg/L increase in DIN concentrations at the river mouth. After the construction of marine engineering projects (2020), significant alterations in the coastline of LZB have markedly modified hydrodynamic characteristics near marine structures, altering DIN dispersion patterns.
Conclusion: This study provides crucial information for the management of pollutants at estuaries, understanding dispersion mechanisms, and evaluating the feasibility and environmental compatibility of marine engineering projects.
1 Introduction
Marine bay areas are often abundant in natural resources, making them some of the most densely populated and economically active regions (Shen et al., 2016; Halpern et al., 2008). As population and economic growth accelerate, the exploitation and utilization of these bays have intensified, establishing them as dynamic centers for marine construction activities. The impact of human activities on these bays is increasingly evident (Bishop et al., 2017; Shan and Li, 2020; Li et al., 2020; Shen et al., 2022). While marine construction projects—such as land reclamation, port facilities, breakwaters, and artificial islands—offer significant benefits to humanity, they also pose considerable threats to the marine environment (Wibowo, 2018; Imalpen et al., 2024; Hasan et al., 2023; Yamamoto et al., 2024).
Numerous studies of marine construction projects globally indicate that their environmental impacts are complex and multifaceted. These projects can alter the hydrodynamic conditions of bays, leading to reduced tidal influx and diminished water exchange capacity. For example, reclamation efforts in Jakarta Bay (Indonesia), Sanmen Bay (China), and Kuwait Bay have significantly decreased the water exchange capacity between these bays and their surrounding environments, resulting in a marked reduction in hydrodynamic activity in adjacent waters (Ihsan, 2020; Yamamoto et al., 2024; Han et al., 2024). Additionally, alterations in hydrodynamics can affect the transport of pollutants, such as inorganic nitrogen and heavy metals, in nearby marine areas, leading to a decline in water quality. The construction of ports and docks has been linked to the accumulation of heavy metals and eutrophication in marine areas adjacent to Richards Bay Harbour (South Africa), Asaluyeh Port (northern Persian Gulf), Haikou Port (China), and Artificial Shihwa Bay (Korea) (Mehlhorn et al., 2021; Delshab et al., 2017; Zeng et al., 2023; Lee et al., 2018).
Moreover, the changes in hydrodynamics and water quality resulting from marine engineering projects can indirectly affect the marine ecological environment. For instance, reclamation projects in locations such as the Ayer Islands (Singapore), the Tianjin Binhai New Area (China), and the coast of Hong Kong have altered habitats for marine organisms, leading to habitat destruction and a decline in biodiversity (Tay et al., 2018; Li et al., 2010; Lai et al., 2016; Chusov et al., 2012). The artificial island reclamation project in Jakarta Bay, Indonesia, has modified the direction and velocity of seawater currents, which in turn affects nutrient supply and impacts the growth of mangroves within the bay (Nugroho and Magdalena, 2020). Therefore, marine engineering projects not only modify the hydrodynamic conditions of bays, impacting water exchange capabilities, but also influence the dispersion of pollutants, resulting in significant effects on marine ecosystems and biodiversity.
Current international research on the impact of marine engineering on the marine environment predominantly utilizes methodologies such as in-situ observations and numerical simulations. In-situ observations provide the most direct means of assessing environmental impacts; however, the sampling and monitoring techniques employed only capture environmental conditions at specific locations and times. Long-term, continuous, and large-scale monitoring is both time-consuming and labor-intensive, rendering comprehensive observations particularly challenging (Shen et al., 2022). The evaluation of long-term environmental changes in bays resulting from marine engineering activities has attracted considerable attention from researchers.
In recent years, previous studies have employed various models, including the DELFT3D hydrodynamic model, the MIKE 21 hydrodynamic model, high-resolution hydrodynamic-passive tracer models, and coupled hydrodynamic-ecosystem models (Nugroho and Magdalena, 2020; Wang et al., 2021a; Mazoyer et al., 2020; Wakelin et al., 2015), to investigate the effects of reclamation on water exchange capacity, pollutant dispersion, and bay ecosystem dynamics (Muller et al., 2020; Imalpen et al., 2024; Xiangyang et al., 2023; Sharaan et al., 2022). These studies have made significant advancements in the numerical simulation of the impacts of marine engineering projects. However, there is a paucity of research assessing changes in these parameters before and after marine engineering initiatives. Consequently, the effects of marine engineering projects, such as reclamation and dam construction, on hydrodynamics and the dispersion of riverine pollutants into bays warrant further investigation.
Laizhou Bay (LZB), one of the three major bays of the Bohai Sea, is located in the northwestern part of the Shandong Peninsula (Zhang et al., 2022). Considering its geographical advantages and beneficial environmental conditions, LZB has become a hotspot of human activities such as those related to ports and aquaculturing. Coastal marine engineering constructions in the LZB area drive population growth and economic development but also have a considerable impact on the ecological environment (Zhang et al., 2022; Zhao et al., 2010), especially in terms of polluting the estuary and nearshore waters (Zhang et al., 2012). Studies have shown that with the construction of coastal projects, the natural shoreline in LZB has been gradually shortened, and the proportion of artificial shorelines is increasing (Wang et al., 2021b; Wang et al., 2014). Influenced by the characteristics of the semi-enclosed Bohai Sea, the seawater exchange capacity in LZB is low, and the ecosystem is extremely fragile (Su et al., 2021). Changes in the topography and shoreline of the bay affect the hydrodynamic conditions and water exchange processes, thus reducing the self-purification capacity of the bay (Zhang et al., 2023; Shen et al., 2015; Wang et al., 2010). In addition, the Yellow and Xiaoqing rivers have the highest runoff and land-based pollutant input among the many seagoing rivers feeding into LZB, which negatively impacts the bay’s ecosystems (Peng et al., 2021; Liu et al., 2012). Among the various factors, continuous nutrient input is a significant contributor to the deterioration of water quality in LZB, and dissolved inorganic nitrogen (DIN) is the predominant pollutant in this area (Wei et al., 2022). Therefore, studying the influence of marine engineering construction on the diffusion of pollutants from the rivers entering LZB is essential for informing conservation efforts in the bay ecosystem.
In this study, we employed the MIKE 21 mathematical model to simulate and analyze the characteristics of the tidal field and the diffusion patterns of DIN from major rivers into LZB before and after a marine engineering project. Our study provides a reference for pollutant control and the management of future marine engineering projects, which may help prevent further deterioration of the bay’s ecological environment.
2 Materials and methods
2.1 Coastline data
Remote sensing images from February 2002 Landsat7 EMT and February 2020 Landsat8 EMT of LZB were downloaded from BIGEMAP and the United States Geological Survey website with spatial resolutions of 15 m and 30 m. After that, the remote sensing images were enhanced using ENVI. Then, a 1:50,000 topographic map was used as a reference to rectify these images. In the geo-referencing process, control points were selected from the remote sensing images, and the geometric correction, fusion, cropping, and mosaic were performed on the remotely sensed images using alignment methods of “image-map” and “image-image” (Zhu et al., 2018). The coastline of the study area adopts the general high-tide line. For the coastline of artificial, bedrock, and sandy coasts, the coastline was extracted by directly tracing the coastal boundary. For the artificial structures such as breakwaters, jetties, and pilot dikes that protrude into the sea, the root line connecting them with the land area was used as the coastline. A comparison of the coastline of LZB in 2002 and 2020 is shown in Figure 1C.
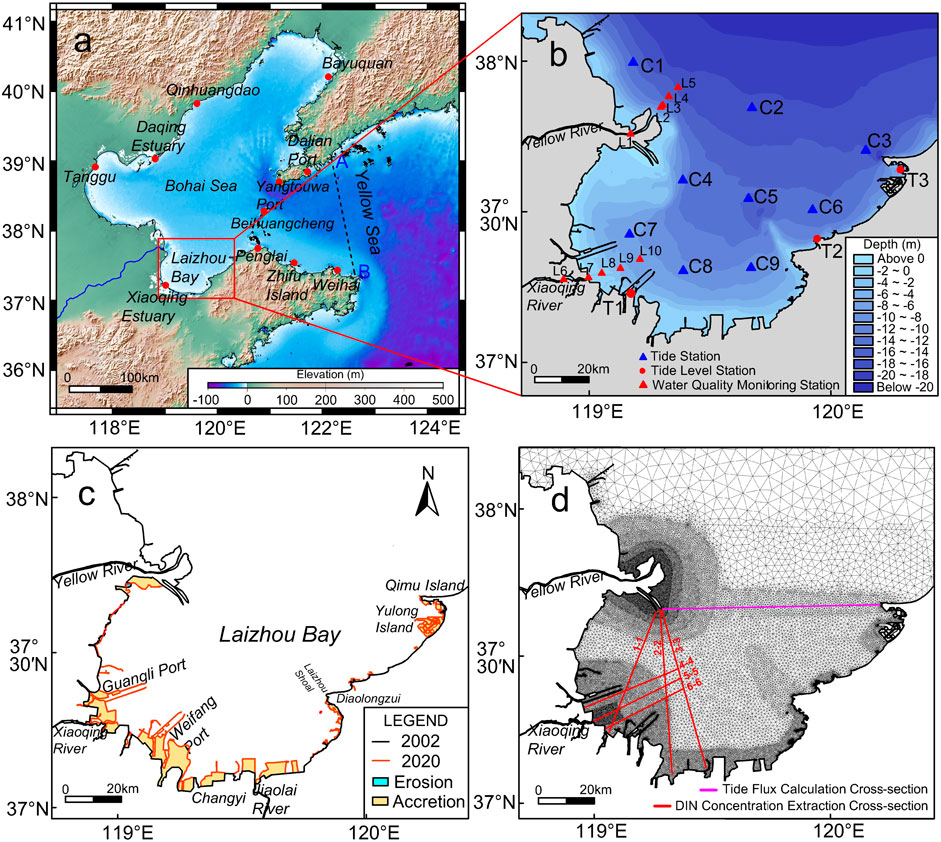
Figure 1. Region of study: (A) Map of the Laizhou Bay (LZB) and its adjacent coastal regions. (B) Bathymetry map of LZB, tidal validation, and water quality validation stations. (C) Coastline comparison of LZB in 2002 and 2020. (D) Grid map of the calculation domain of LZB, DIN concentration extraction cross-section, and tidal flux calculation.
2.2 Numerical simulation
2.2.1 Hydrodynamic model
The hydrodynamic module in MIKE 21 FM (DHI, 2017) was used to simulate the tidal field changes in LZB before (2002) and after (2020) the main period of marine construction in the area. The model has been commonly used for hydrodynamic and water quality simulations and has demonstrated its efficiency in simulating flow movement and water quality changes (Chi et al., 2020; Yang et al., 2023).
The model used a non-structural triangular grid to dissect the computational domain. The computational domain range is shown in Figure 1D. To better compare the tidal field changes in LZB before and after the main period of marine construction in the area, the grid of other sea areas outside LZB was set to be consistent. Concurrently, to clearly understand the tidal conditions in the nearshore area of LZB, a grid of this area, especially the area near the port, was locally encrypted. Other settings of the model were as follows:
2.2.1.1 Water depth and shore boundary
The water depth and shore boundary were based on the 1:1 million charts (No. 10011), 1:150,000 charts (Nos 11370, 11570, 11710, 11770, 11840, and 11910), 1:30,000 charts (Nos 11873 and 2011), 1:25,000 charts (No. 11892, 11872), the bathymetric topographic survey data near the project in 2013 and of the Lower Yangtze River Hydrographic and Water Resources Survey Bureau of the Hydrographic Bureau of the Yangtze River Commission in March 2007 in the Mi River (1:2000), Xiaoqing River (1:5,000) and its estuary (1:10,000), as well as the latest measured bathymetric topographic data of the sea area near the project. Furthermore, the shoreline boundary before and after the project was determined based on the satellite image data in 2002 and 2020, respectively.
2.2.1.2 Large calculation domain grid and calculation of time step
The large calculation domain grid comprised 42,169 nodes and 80,516 triangular units before the marine engineering project (2002) and 38,532 nodes and 70,065 triangular units thereafter (2020). The minimum grid spacing was approximately 20 m.
The model calculation time step was dynamically adjusted according to the Courant-Friedrich-Lévy (CFL) condition to ensure the model calculation was stable, and the minimum time step was 0.4 s. The CFL formula (Equation 1) was as follows:
where h is the total water depth, u and v are the velocity components in the x- and y-direction, respectively, g is the gravitational acceleration, Δx and Δy are a characteristic length scale in the x- and y-direction, respectively, for an element, and Δt is the time step interval.
2.2.1.3 Model water boundary input
The formula of open boundary was calculated using Equation 2. The reconciliation constants of the four major tidal constituents, M2, S2, K1, and O1, which were obtained by reconciling multi-year tide level observation data from Densha River in Liaoning (point A), Zhongnan, Zhongbei, and Jiming Island in Shandong (point B), were quoted for calculation:
where σi is the angular velocity of the ith tidal constituents (used in this study: M2, S2, O1, and K1); Hi and Gi are the reconciliation constants, which are the tidal amplitudes and phases of the tidal constituents, respectively.
Closed boundary: The large sea area and the surrounding shoreline were used as the closed boundary.
2.2.1.4 Bottom bed roughness and horizontal eddy viscosity coefficient
The bottom bed roughness was controlled by the Manning coefficient. The Manning coefficient n was 51–94 m1/3/s.
The formula of Smagorinsky (1963) was used to calculate the horizontal eddy viscosity coefficient (Equation 3). The calculation formula was as follows:
where cs is a constant, and this simulation calculation takes 0.28, l is the characteristic mixing length, and Sij is calculated from
2.2.2 River pollutant discharge model
The transport module in MIKE 21 FM was used to simulate the pollutant dispersion in the Yellow and Xiaoqing rivers for 2 months (July and August). The main pollutant in LZB was DIN; thus, we selected DIN as a main factor for pollutant dispersion analysis to focus on the diffusion changes of DIN discharged from the Yellow and Xiaoqing rivers into the bay under the influence of tidal currents before and after the marine engineering project in LZB. The pollutant transport formula was calculated by Equation 4.
The vertically averaged material transport equation was:
where P is the pollutant concentration; u and v are the flow rate components in x and y directions, respectively; Dx and Dy are the diffusion coefficients in x and y directions, respectively; S is the discharge rate of pollutants per unit time; H is the water depth; k is the attenuation coefficient.
To ensure the reliability of the simulation results, hydrological and water quality data from the hydrological station of the Yellow River in Lijin, the Xiaoqing River Shicun hydrological station, and the cross-section of the Xiaoqing River in Yangkou were collected to obtain the runoff flow and water quality monitoring data of the Yellow and Xiaoqing rivers during the abundant water period. We set the runoff parameter of the Yellow River to 1,366.4 m3/s and that of the Xiaoqing River to 81.3 m3/s based on the statistical runoff data. According to the water quality monitoring data, the source strength of total nitrogen in the Yellow River was selected as 2.69 mg/L, and that in the Xiaoqing River was selected as 7.89 mg/L. The ratio of DIN to total nitrogen was 0.95 in the Yellow River and 0.73 in the Xiaoqing River based on the monitored DIN content and the corresponding total nitrogen content. Then, the source strength of DIN was determined to be 2.556 mg/L for the Yellow River and 5.782 mg/L for the Xiaoqing River.
The collected data on runoff volume and DIN source strength of the Yellow and Xiaoqing rivers were used as input parameters for the river pollutant discharge model (Table 1). As this study analyzed the changes in pollutant dispersion under different coastline conditions, the parameters were set consistently before and after the marine engineering project. We only considered the concentration increment of river pollutant discharge on the water quality of the sea without considering the background value of water quality in the study area. The attenuation coefficient was uncertain because the DIN attenuation is affected by various factors; according to the conservative calculation of the maximum possible impact range of DIN, DIN attenuation is not considered in this simulation, and DIN is simulated as a conservative variable. Furthermore, this study mainly investigated the diffusion characteristics of DIN from rivers into the sea under the action of the tide before and after the construction of marine engineering, and the model did not consider the biochemical reaction processes and the nitrogen transformation or reaction. In subsequent research, we will consider 3D models to simulate research, taking wind-driven and density-driven currents into account, as well as biochemical reaction processes and the nitrogen transformation or reaction.
2.3 Pollutant contribution calculation
Many rivers feed into LZB, and river input accounts for most of the terrigenous input into the sea. To examine the influence of river input of pollutants into the sea on water quality, we controlled the variables based on the initial parameters (Run 1) and set up four additional working conditions (Table 2). With constant runoff, pollutant source intensity increased by 50% (Run 2) and decreased by 50% (Run 3). The source strength of pollutants was controlled to be constant, and the runoff increased by 50% (Run 4) and decreased by 50% (Run 5). The following formula was used to calculate pollutant contribution:
where W is the pollutant contribution, C is the pollutant concentration, A is the grid area, and h is the water depth.
2.4 Tide flux calculation
The tidal flux refers to the volume of tidal water that accumulates through a given cross-section at a given time, and the tidal flux entering the mouth of a bay can indicate the self-purification capacity at the corresponding time. The equation was:
where Si is the area of grids, h1i and h2i represent the high-tide and low-tide levels of the elements, respectively, and n is the total number of grids.
3 Results
3.1 Model validation
3.1.1 Hydrodynamic model validation
To ensure the accuracy of the hydrodynamic model and verify whether the simulated tide levels and tides can better reproduce the actual tide levels and conditions, we collected historical observations from 11 tide stations in Dalian, Yangtouwa, Bayuquan, Qinhuangdao, Daqing Estuary, Tanggu, Xiaoqing Estuary, Penglai, Zhifu Island, Weihai, and Beihuangcheng and used the Fourier analysis method (Huang and Huang, 2005) to reconcile the analysis. The stations are shown in Figure 1A. Then, four reconciliation constants of M2, S2, K1, and O1 were chosen to forecast the tide levels during the high tide period, which were verified with the calculated results. Simultaneously, the actual tide level observation data of LZB was verified with the tide level calculation results during the spring tide period. Owing to space limitations, only the measured tide level verification curves (Bayuquan, Penglai, T1, T2, and T3) in the study area are listed in this study (Figure 2). To further interpret the accuracy of the model results, the mean absolute errors (MAEs) (Zhu et al., 2018) between the simulation and observation at the five tidal level stations were calculated (Table 3). As shown in Table 3, the MAEs for the tidal level ranged from 0.01 to 0.17 m. These errors may have occurred because the tidal constituents in the model mainly take M2, S2, O1, and K1, whereas the actual tidal changes can be decomposed into multiple tidal constituents. In general, the simulated results accurately captured the tidal level variations in terms of range and phase.
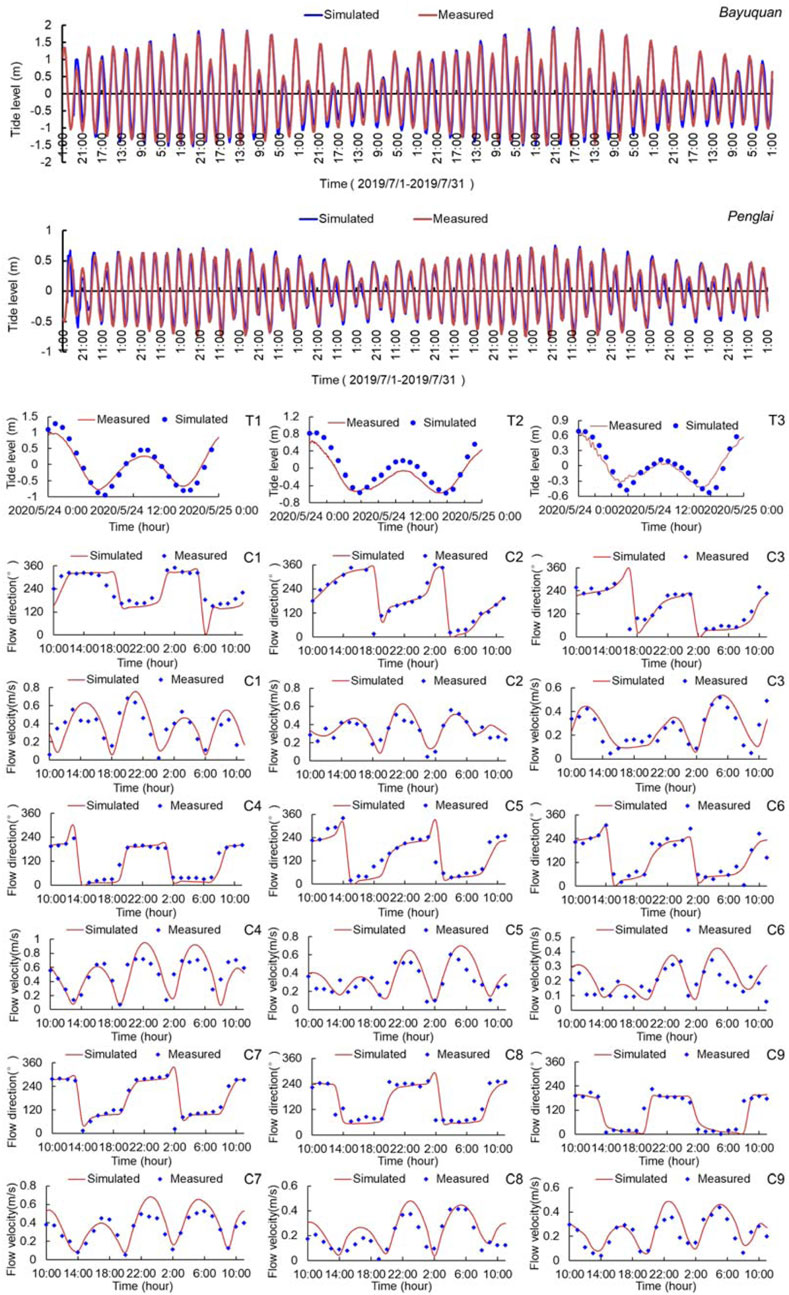
Figure 2. Comparison of measured and simulated results of tide level (Bayuquan, Penglai and T1–T3), flow direction and velocity (C1–C9).
The tidal current observation data from C1–C9 stations collected by the Ocean University of China in the sea area of LZB from 24 May 2020, at 10:00 a.m. to 25 May 2020, at 11:00 a.m. (spring tide period) were used for tidal current verification. The stations are shown in Figure 1B. Then, the hydrodynamic model was used to simulate the tidal field of LZB in May 2020, and the flow velocity and direction of C1–C9 stations were extracted and compared with the measured tide. The tidal validation curve is shown in Figure 2. As shown in Table 4, the MAE ranges of flow direction and velocity were 3°–24° and 0.01–0.06 m/s at C1–C9 stations, respectively. These errors may be due to the fact that the model only considered the tidal dynamic factor, without accounting for the effect of wind-driven and density-driven currents, and there was a certain spatial deviation between the grid point and the measured point (Panda et al., 2015; Chen et al., 2019). In general, the model predictions of current direction and velocity followed the general variation pattern and reasonably matched the observation. Thus, the model can be used for further simulations.
3.1.2 River pollutant discharge model validation
To ensure the reliability of the river pollutant discharge model, this study collected water quality data from the water quality monitoring stations deployed by the Ocean University of China in May 2020 at the estuaries of the Yellow and Xiaoqing rivers into the sea. The stations are shown in Figure 1B. We simulated the DIN dispersion of the Yellow and Xiaoqing river input into LZB in May 2020 and extracted the simulation results (average value in May) from the water quality monitoring stations of the Yellow and Xiaoqing rivers estuaries to compare with the field monitoring data for verification.
Figure 3 shows the verification curve, indicating that the simulated results aligned well with the measured data, albeit with slightly lower values. Atmospheric nitrogen deposition constitutes a significant contributor to DIN input into the Bohai Sea (Shou et al., 2018; Zheng et al., 2020). Anthropogenic nitrogen emissions are transported via the atmosphere and deposited onto ocean surfaces through wet and dry deposition, leading to an increase in seawater nitrogen content (Chen and Huang, 2021; Xie et al., 2021), which may explain the higher measured DIN concentrations.
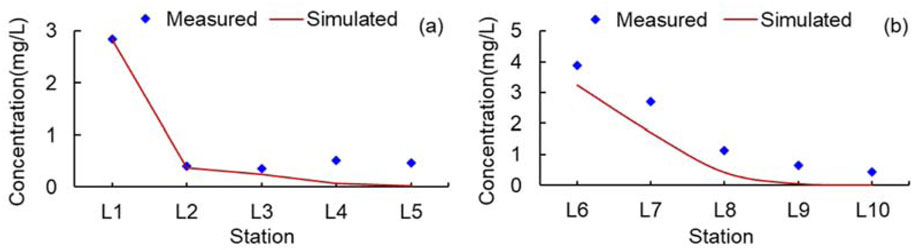
Figure 3. River pollutant discharge model validation curve. Comparison of measured and simulated DIN concentration results of the Yellow (A) and Xiaoqing (B) river estuary stations in May 2020.
3.2 Change in tidal current field caused by the marine engineering project
To study the influence of the marine engineering project on the tidal field, this study simulated the tidal field at the moment of flood and ebb tides before and after the marine engineering project in LZB during the spring tide period (Figures 4A–D). The simulation of the tidal field before the marine engineering project showed that the tidal current in the calculation domain at the moment of flood tide flowed from outside LZB to inside the bay (Figures 4A, B), and overall, the closer to the coastline, the lower was the velocity. On the east side of the sea, the tidal flow near the top of the bay was southward and turned to the southwest at Laizhou Shoal. After passing Laizhou Shoal, the tidal flow turned southward and southeastward. On the west side of the sea, the tidal flow near the top of the bay was southeastward, and the tidal flow around the mouth of the Yellow River to the south side of the Yellow River Delta turned from southeastward to westward and northwestward. During ebb tide, the tidal current flowed from the inside to the outside of LZB, and the flow direction distribution was roughly opposite to that during flood tide. The overall flow velocity in LZB during ebb tide was greater than that during flood tide. After marine engineering construction (Figures 4C, D), the tidal current characteristics of the sea area near the project changed significantly, which was mainly manifested by the noticeable deflection of the tidal current flow in the sea area near the project, such as the Yulong Island and Weifang Port breakwaters.
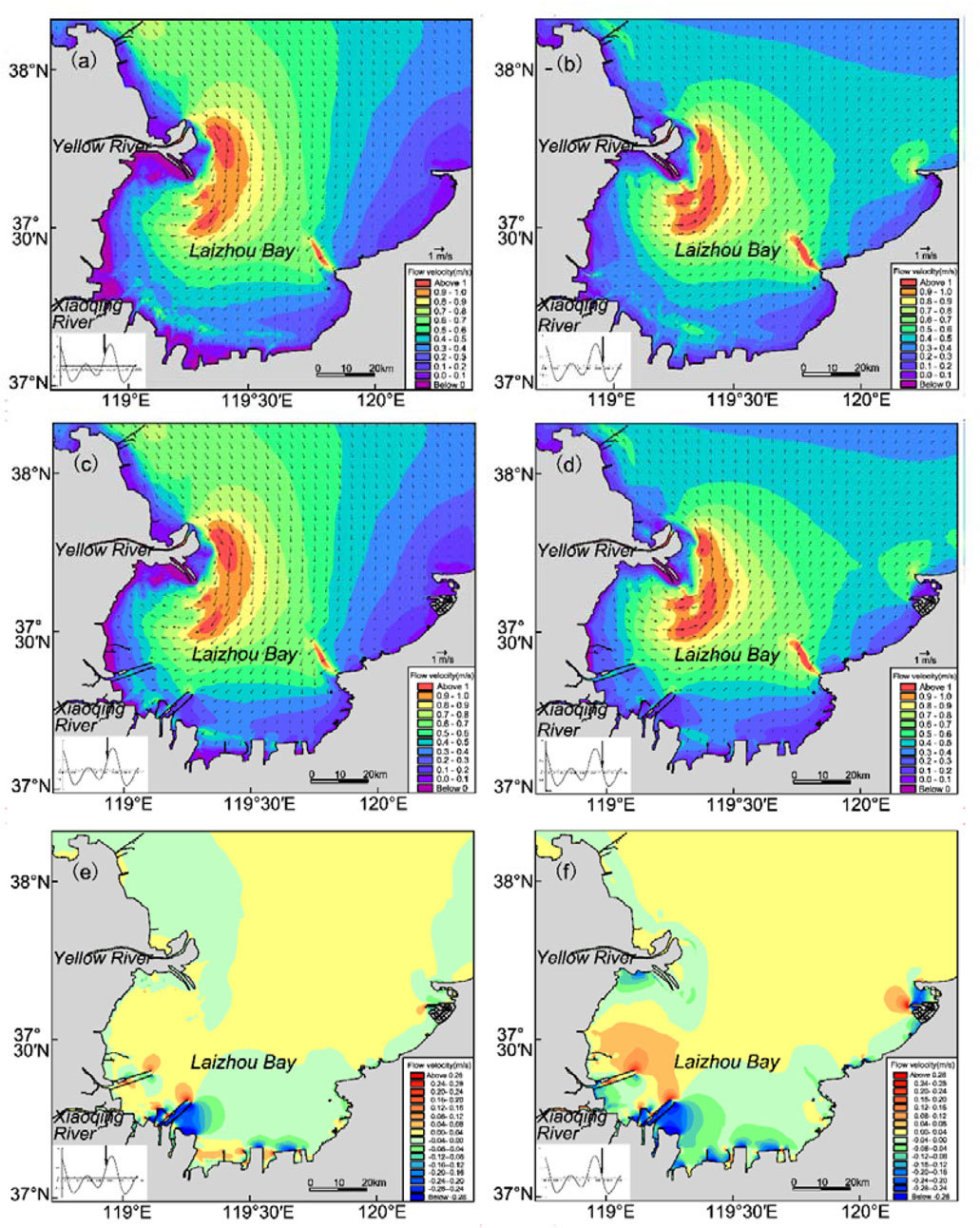
Figure 4. Tidal field distribution characteristics and flow rate comparison chart (spring tide period). Tidal current distribution at the flood (A) and ebb (B) tides before the project. Tidal current distribution at the flood (C) and ebb (D) tides after the project. Comparison of flow rate at the flood (E) and ebb (F) tides before and after the project.
To elucidate the tidal field changes caused by marine engineering, we selected the tidal current field during flood and ebb tides before and after marine engineering in the spring tide period to compare the flow rates. The flow rate change is shown in Figures 4E, F. The rate change during ebb tide was more pronounced than during flood tide, and the change was between 10 and 50 cm/s. The flow rate increased significantly at the head of the breakwater of Guangli and Weifang ports and the west side of the breakwater of Yulong Island owing to the effect of provoking flow (Zhu et al., 2004), and the increase was between 12 and 34 cm/s. The decreased flow rate was evident in the sea areas on the south side of the Yellow River Delta, the east side of Weifang Port, near the dyke on the north side of Changyi, near the salt fields on both sides of Jiao Lai River mouth, and the north side of Yulong Island, with a decrease between 16 and 46 cm/s. The blocking effect of marine engineering may be one of the reasons for the decrease in tidal current velocity near the project (Xu et al., 2017; Kuang et al., 2024). The closer to the project, the more evident was the decrease in current velocity. The change range of the current velocity during flood tide decreased compared with that during ebb tide, and the change value was 0–0.35 m/s. Except for the tidal current velocity near the dike on the north side of Changyi, which showed an increase compared with that during ebb tide, the overall velocity change area was the same as that during ebb tide. Marine construction at the LZB inshore site has significantly altered the surrounding hydrodynamic environment and the original tidal patterns.
Several studies have investigated the effects of marine engineering on the hydrodynamics of bays. For example, Gong et al. (2019) used a two-dimensional numerical model to simulate the effects of reclamation projects on the hydrodynamic environment of Dongshan Bay and found that such projects had a large impact on the bay mouth and caused different degrees of decrease in the tidal prism and water exchange rate. Yanagu and Onishi (1999) investigated the tidal changes caused by a reclamation project in Tokyo Bay using three-dimensional numerical simulations. The reclamation project led to a decrease in the flow velocity and water exchange volume in the mouth of the bay by approximately 20% and 35%, respectively. Gao et al. (2014) established a three-dimensional model to study the effects of sea reclamation in Jiaozhou Bay and reported that the reclamation project led to the weakening of tidal motion in the bay mouth. The above studies showed that marine engineering projects cause changes in the original coastline, topography, and area of bays and cause changes in the tidal current field, usually weakening the tidal current in the bay (Okada et al., 2011; Zhang et al., 2021). However, the weakening of tidal currents and the reduction of the bay area reduce the environmental and pollution-carrying capacity of the bay (Chi et al., 2020).
3.3 Characteristics of residual current fields before and after the marine engineering project
The simulation of the residual current field in LZB before and after the marine project is shown in Figure 5. The residual velocity in LZB was small overall, and the average residual current velocities within LZB were approximately 2.10 cm/s before and 2.27 cm/s after the marine engineering project. The south of the Yellow River Delta has a clockwise circulation with high residual velocities. There was an anti-clockwise circulation on the west of Diaolongzui and Qimu Island, and a clockwise circulation on the north of Diaolongzui. After the construction of the marine engineering project, there exists a clockwise circulation with smaller residual velocity and range radius in the sea area near the east side of Weifang Port breakwater, and an anti-clockwise circulation with a smaller range radius in the sea area west of Yulong Island.
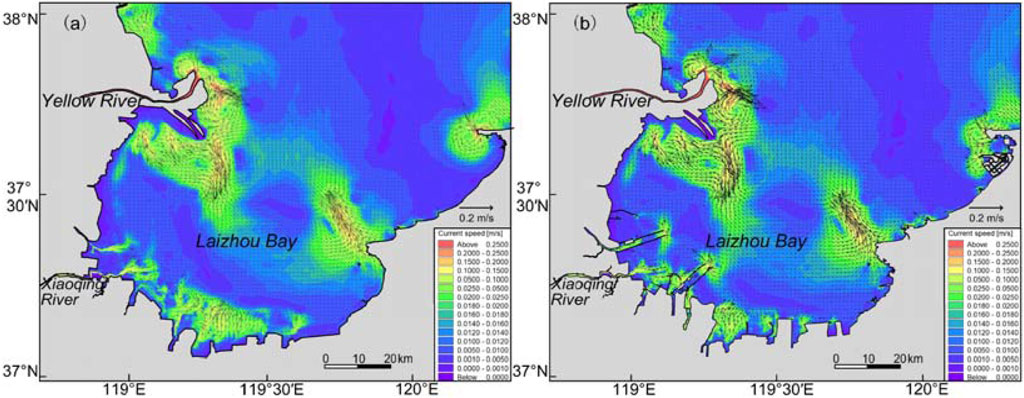
Figure 5. Characteristics of residual current fields before (A) and after (B) the marine engineering project.
3.4 Effects of the marine engineering project on the dispersion of DIN from rivers to sea
We simulated the diffusion of DIN input into LZB during the abundant water period for the Yellow and Xiaoqing rivers, respectively. The simulation results are shown in Figure 6. The diffusion range of the Yellow River and Xiaoqing rivers into LZB gradually increased with increasing simulation time. Figures 6A–H shows the simulation results of DIN diffusion from the Yellow River into the sea before and after the marine engineering project. The DIN input from the Yellow River into LZB diffused into the bay in a “tongue-like” manner. The diffusion range of DIN reached its maximum extent at 60 days. DIN with an increment exceeding 0.5 mg/L affected the diffusion to the middle of LZB, and DIN with an increment exceeding 0.1 mg/L almost spread to the middle and west of LZB.
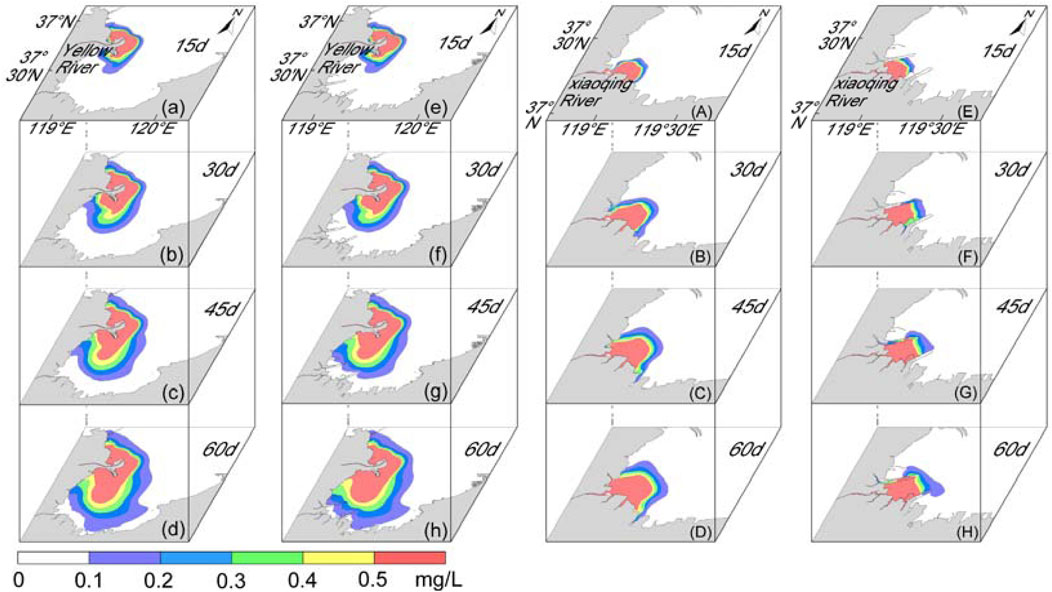
Figure 6. Diffusion plane distribution of DIN from the Yellow and Xiaoqing rivers into Laizhou Bay before and after marine engineering construction (from top to bottom, the simulation duration is 15, 30, 45, and 60 d, respectively). Diffusion range of DIN from the Yellow River into Laizhou Bay before (A–D) and after (E–H) the marine engineering project. Diffusion range of DIN from the Xiaoqing River into Laizhou Bay before (A–D) and after (E–H) the marine engineering project.
A comparison of the diffusion area of DIN in the Yellow River into LZB before and after the marine engineering project (Table 5) showed that the diffusion area of ≥0.5 mg/L DIN increased after the marine engineering project, and it increased by 80.77 km2 when simulated to 60 days. The average DIN concentration in the sea area near the Yellow River estuary has recently exceeded the national seawater quality standard of category IV, showing an excess of DIN (Wang et al., 2018; Zhang et al., 2008; Shan et al., 2000). This is relatively consistent with the results of numerical simulations showing a larger spread of DIN above the seawater quality standard of category IV.
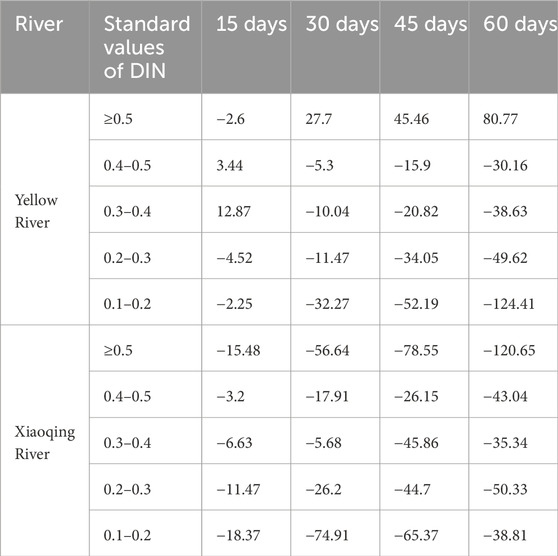
Table 5. Area difference of DIN diffusion in the Yellow and Xiaoqing rivers before and after the marine engineering project.
The diffusion area of DIN increased after marine engineering construction when the simulation was up to 15 days (Figure 7A); however, the diffusion area of DIN input from the Yellow River into LZB significantly decreased when the simulation was up to 30, 45, and 60 days. As the DIN gradually diffused to the nearshore waters in the southwestern part of LZB, it was blocked by artificial structures, such as Guangli and Weifang ports, resulting in a change in the diffusion range of DIN near the shore and a significant decrease in the diffusion area.
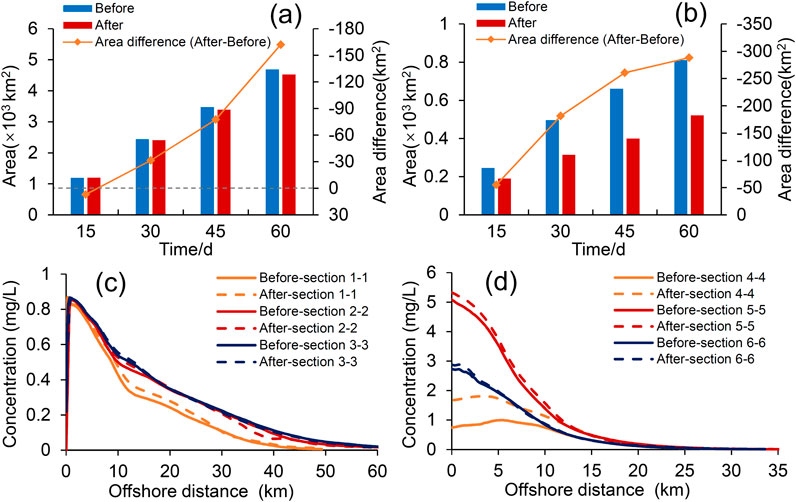
Figure 7. Calculation results of diffusion area of DIN in Yellow and Xiaoqing rivers before and after the marine engineering project and the average concentration of each section. Comparison of the diffuse area of the Yellow (A) and Xiaoqing (B) rivers before and after the marine engineering project. Comparison of the concentration of cross-sections 1-1, 2-2, 3-3 (C) and cross-sections 4-4, 5-5, 6-6 (D) before and after the marine engineering project.
Figures 6A–H shows the simulation results of DIN diffusion from the Xiaoqing River into the sea before and after the marine engineering project. By comparing the planar distribution of DIN diffusion before and after the marine engineering project, we found that Guangli and Weifang ports blocked the DIN diffusion to the southeast–northwest direction, resulting in a significant decrease in the DIN diffusion range. Simultaneously, the diffusion area of DIN input from the Xiaoqing River into LZB significantly decreased after marine engineering construction (Table 5; Figure 7B).
To further analyze the degree of influence of the marine engineering project on the diffusion of DIN in seagoing rivers of LZB, we extended three sections outward from the estuary of the Yellow River and the estuary of the Xiaoqing River. The location of the sections is shown in Figure 1D. The average concentrations of each section before and after the marine engineering project were extracted separately. The results of the concentration comparison are shown in Figures 7C, D. The concentration gradually decreased with the diffusion of DIN to the inner side of LZB.
The DIN concentrations in cross-sections 1-1, 2-2, 3-3 changed little after the marine engineering construction (Figure 7C). Only the concentration in cross-section 1-1 increased in the middle part of the section (from the mouth of the Yellow River to the middle sea area of the mouth of the Xiaoqing River), indicating that marine engineering in LZB had a small impact on the diffusion concentration of DIN in the Yellow River. In contrast, the DIN concentrations in cross-sections 4-4, 5-5, 6-6 changed significantly after the marine engineering construction, mainly showing a significant increase at the mouth of the Xiaoqing River (Figure 7D).
4 Discussion
4.1 Influence of changing hydrodynamic conditions on the diffusion of DIN
Hydrodynamic conditions significantly influence the transport and accumulation of substances within oceanic environments, with regions exhibiting weaker hydrodynamic activity being more susceptible to nutrient accumulation. For example, a comparative study on eutrophication in Victoria Harbour and Tolo Harbour in Hong Kong, which possess distinct hydrodynamic characteristics, revealed that Tolo Harbour, characterized by weaker hydrodynamics, exhibited elevated nutrient levels (Xu et al., 2010). In Malaysia, the complex topography of the Setiu Lagoon restricts the exchange of substances between water bodies, thereby promoting the accumulation of nutrients within the lagoon (Zainol et al., 2020).
Analysis of the residual current field in LZB (Figure 5) indicates that higher residual velocities in the southern section of the Yellow River Delta facilitate the transport of elevated concentrations of DIN to this area, resulting in increased DIN levels. Following the construction of marine engineering projects flanking the Xiaoqing River estuary, a reduction in flow velocity was observed, leading to an increase in DIN concentration at the estuary. Additionally, the diffusion rate of DIN was found to be enhanced in regions of high flow velocity, while it diminished in areas of lower flow velocity.
In addition, the transformation of nitrogen in water is an important part of the nitrogen cycle in the ecosystem, which is mainly divided into three processes: nitrogen fixation, nitrification, and denitrification (Hudon and Carignan, 2008; Hudon et al., 2017). The denitrification process typically occurs when hydrodynamic conditions are weak, with slow currents and lack of oxygen, reducing excess nitrate nitrogen in the water to nitrite nitrogen and ammonia nitrogen, thus maintaining the balance of nitrogen in seawater (Bradley et al., 2010; Liu et al., 2016). Regions of high flow velocities also have an influence on nitrogen transformation and cycling. Jiang et al. (2020) simulated the cycles and budgets of DIN and particulate organic nitrogen (PON) in the Yellow Sea, showing that the bottom friction stress is greater than 2 N/m2 along the Shoal coast of North Jiangsu Province and part of the Korean Peninsula, and the annual sedimentary flux of PON is very low. This indicates that enhanced sediment resuspension processes in regions of higher flow velocities affect nitrogen deposition and resuspension fluxes, thereby altering the pathways and rates of nitrogen transformation; however, the mechanism of nitrogen transformation in water is a complex process, which involves several links and factors, including oxygen, light, temperature, and bacteria (Percuoco et al., 2015; Wei et al., 2017; Chen et al., 2018). In the future, we will collect further data to thoroughly investigate the process and mechanism of nitrogen transformation in seawater.
4.2 Influence of the marine engineering project on the water quality of the bay
Marine engineering construction has occupied the original sea area and changed the length and morphology of the coastline, causing the coastline to continuously advance toward the sea (Tian et al., 2018; Lv et al., 2017). This has resulted in a pronounced decline in the natural properties of the nearshore sea area of LZB, of which the Xiaoqing River estuary was highly artificialized after 2010 (Fan et al., 2017), which subsequently led to the estuary exhibiting poor self-regulation ability and resilience (Dafforn et al., 2015). Meanwhile, the construction of levees in Guangli and Weifang ports reduced the flow velocity between the two levees (Figure 4F) and increased the water depth. In addition, the diffusion of DIN to the outer sea area is hampered when it is blocked by the levees, thus intensifying the accumulation of DIN and resulting in severe water pollution near the estuary of the Xiaoqing River (Liu, 2013; Xu and You, 2017).
Over the past 2 decades, numerous major coastal cities worldwide have initiated marine construction projects, including land reclamation, resulting in the addition of over 250,000 ha to the global coastline (Sengupta et al., 2023). Such construction activities, including dam and reclamation projects, can significantly alter the hydrodynamic environment and water exchange capacity of adjacent marine areas. This alteration can lead to nutrient enrichment and the accumulation of other substances, ultimately causing a deterioration in water quality (Meng et al., 2017; Lie et al., 2008).
For instance, the construction of coastal dams in Shihwa, South Korea (Lee et al., 2018), the artificial island reclamation project in Jakarta Bay, Indonesia (Ihsan, 2020), the reclamation projects at Jebel Ali Harbour in Dubai and the beaches of Makassar City in Indonesia (Maraqa et al., 2007; Wibowo, 2018), the land reclamation along the Yeonggwang coast in South Korea (Kim et al., 2019), the reclamation projects in Jiaozhou Bay (Zhang et al., 2017; Lin et al., 2015), and the reclamation project in Qinzhou Bay (Lyu et al., 2022) have all been associated with increased concentrations of nutrients, such as BOD and inorganic nitrogen, in nearby marine areas, leading to a decline in water quality.
Additionally, bridge and port constructions also significantly impact the water quality of bays. For example, the cross-sea bridge in Kuwait Bay has restricted water exchange between the bay and the open sea, resulting in a differential distribution of nutrients in the seawater on either side of the bridge (Yamamoto et al., 2024). In the northern Persian Gulf, near the port of Asaluyeh, industrial activities and shipping operations have led to the accumulation of sewage that is challenging to disperse into the open ocean, with heavy metal concentrations in the seawater exceeding global averages, particularly in proximity to oil refineries (Delshab et al., 2017).
Furthermore, aquaculture practices can adversely affect water quality. For instance, aquaculture facilities in Dapeng Bay on the southwest coast of Taiwan, China, have occupied extensive marine areas, significantly reducing the water exchange rate within the bay. This situation has resulted in a continuous influx of nutrients and organic matter into the seawater, leading to severe eutrophication. However, following the removal of these aquaculture facilities, the water exchange time decreased, and subsequent improvements in water quality were observed (Hung et al., 2020; Hung et al., 2013; Hung and Hung, 2003).
Considering the results of the above studies and those of the current study, it can be concluded that the construction of marine engineering projects leads to the reduction of space in the marine area where the project is located, thus reducing the tidal flow and weakening the water exchange capacity and self-purification capacity of the bay. These factors, together with pollutants such as domestic sewage, oily sewage, aquaculture wastewater, and other pollutants produced during the operation of the marine engineering project, will negatively impact the bay’s water quality, thus aggravating water pollution; however, because the negative effects on water quality are caused by the artificial structures, the hydrodynamic and ecological environment of the sea area near the project can be gradually restored by removing abandoned breeding ponds, dikes, and other engineering facilities, as well as restricting the reclamation of land for aquaculture and the use of salt fields.
4.3 Influence of pollutant sources
In addition to the significant effects of marine engineering construction on the marine water environment, the introduction of land-based pollutants from human activities—both industrial and domestic—can severely impact marine ecosystems. This pollution can lead to issues such as eutrophication, chronic hypoxia, and harmful algal blooms (Zhang et al., 2017; Chi et al., 2020). For instance, the excessive use of commercial inorganic fertilizers in agriculture to boost crop yields has elevated pollutant levels in offshore waters. Research has indicated that the overapplication of agricultural fertilizers is a primary contributor to increased nitrogen fluxes in the estuarine waters of the Yangtze River (Zhang, 1996; Jiang et al., 2015).
Moreover, industrial wastewater and domestic waste from industrial activities are often directly discharged into the ocean or transported to offshore waters via rivers, resulting in heightened pollutant fluxes and significant pollution of coastal aquatic environments (Pan and Wang, 2012; Xu and Zhang, 2007). The combined effects of land-based pollution and land reclamation can synergistically diminish the marine environment’s carrying capacity and degrade water quality. For example, ocean acidification in coastal regions such as the Netherlands (Provoost et al., 2010), Bengal Bay (Sridevi and Sarma, 2021), Buzzards Bay in the United States (Rheuban et al., 2019), the Gulf of Mexico (Osborne et al., 2022), and Tokyo Bay in Japan (Yamamoto-Kawai et al., 2021) is closely associated with nutrient inflow from adjacent rivers. Additionally, elevated concentrations of heavy metals in seawater along the coast of Yanbu, Saudi Arabia (El-Sorogy et al., 2023), in shipbreaking industrial areas of Bangladesh (Hasan et al., 2023), Karachi, Pakistan (Chan et al., 2021), Suva, Fiji (Arikibe and Prasad, 2020), Port Klang, Malaysia (Sany et al., 2013), and the Gulf of Suez, Egypt (Nour and El-Sorogy, 2020) are directly linked to human activities such as sewage discharge. Therefore, the influence of pollution sources, including river runoff and anthropogenic activities, on the water quality of bays warrants careful consideration.
By varying the pollutant fluxes (Table 2), the diffusion characteristics of pollutants that are translocated into LZB jointly by the Yellow and Xiaoqing rivers were simulated, and the contribution of DIN (increment greater than 0.1 mg/L) and the diffusion area were calculated for five working conditions under a simulation duration of 60 days. Table 6 shows that the pollutant contribution (Equation 5) and diffusion area under the five operating conditions were reduced after marine engineering construction compared with those before, which was consistent with the results of this study as discussed above. When the pollutant flux (Run 2 and Run 4) was increased, the contribution of pollutants and the diffusion area of the Yellow and Xiaoqing rivers into LZB increased significantly (Figure 8). When the pollutant source strength (Run 2) increased, the contribution (Figure 8A) and diffusion area (Figure 8B) of the pollutants into LZB changed to a greater extent than when the runoff volume (Run 4) increased, indicating that the negative impact of increasing the source intensity of pollutants into LZB on water quality is greater. Correspondingly, when the pollutant flux (Run 3 and Run 5) was decreased, the contribution (Figure 8A) of pollutants and the diffusion area (Figure 8B) within LZB from the Yellow and Xiaoqing rivers were significantly reduced. When the pollutant input flux was reduced, the change in diffuse area was approximately twice as large as when the pollutant input flux was increased (Figure 8B). This indicates that the pollutant dispersion area significantly decreased when the pollutant flux into LZB was reduced, implying that the pollutant dispersion area in LZB can be effectively controlled by reducing the pollutant flux into the sea from the river. Therefore, the water quality of the bay can be effectively improved by controlling the total amount of pollutants discharged at the source by controlling the point source pollution of the river.
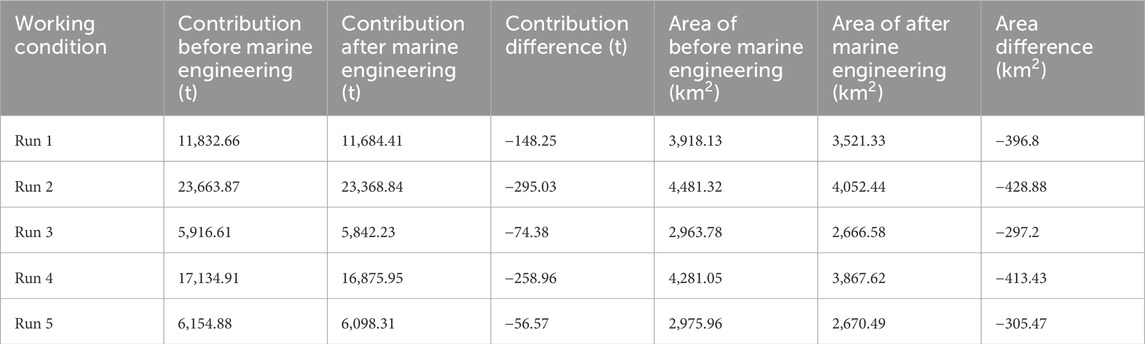
Table 6. Calculation of DIN contribution and diffusion area for different working conditions before and after the marine engineering project.
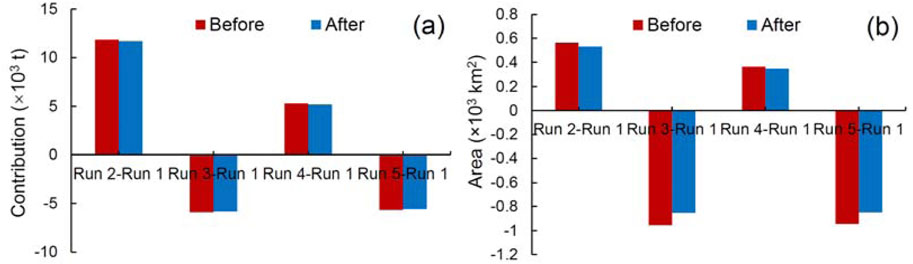
Figure 8. Variation of DIN contribution and diffusion area under different working conditions. (A) Changes in DIN contribution. (B) Changes in DIN diffusion area.
4.4 Forecast of future possibilities and control measures
The activities of sea use in LZB, such as land reclamation, sea-farming, port construction, and the salt industry, have brought great environmental pressure to the bay, such as the destruction of seafloor substrates and biological habitats and input of nutrients and pollutants (Liu et al., 2018; Tian et al., 2016). If this is not controlled, marine engineering construction exerts more severe adverse effects on the sea environment of LZB (Zhu et al., 2016). Therefore, through the control variables, we assumed that the coastal area in the southeast of LZB continues to build reclamation projects, its coastline continues to advance to the sea, and other conditions remain consistent with the current situation to simulate its hydrodynamic force and DIN diffusion. We compared the changes in tidal flux and DIN diffusion in the past, present, and predicted future (Figure 9). The formula of tidal flux (W) was calculated using Equation 6. The tidal flux decreased significantly when the reclamation area continued to increase, and the ebb tide flux at the entrance of LZB in 1 month decreased from 3.95 × 1011 to 3.90 × 1011 m3. The flood tide flux decreased from 3.98 × 1011 to 3.93 × 1011 m3. The DIN diffusion distribution curves and concentration percentage pie charts at the simulation time of 60 days also showed that the diffusion area of DIN decreased, but the concentration increased. The proportion of DIN with concentration increments greater than 0.5 mg/L increased by 2% compared to the past under continued reclamation in the future. The results showed that continued reclamation would aggravate the reduction of hydrodynamics in LZB and cause an increase in DIN content, leading to serious environmental pollution of the water body.
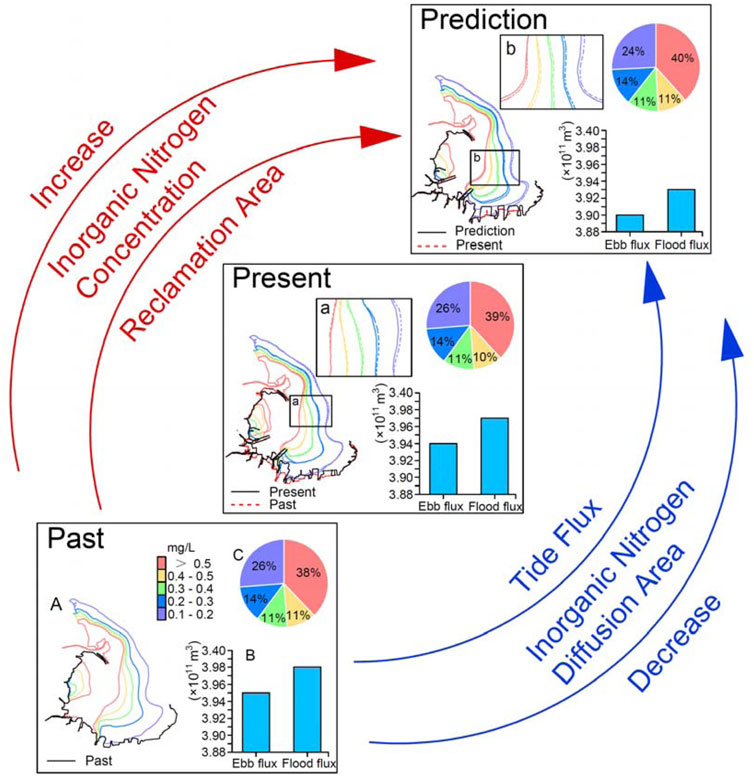
Figure 9. Comparison of past, present, and projected future changes in tidal flux and dissolved inorganic nitrogen (DIN) diffusion in Laizhou Bay. (A) Diffusion range of DIN discharges from the Yellow and Xiaoqing rivers during the simulation duration of 60 d. (B) One-month flood and ebb tide fluxes in Laizhou Bay. (C) The proportion of DIN in the total diffusion range for each standard concentration. (A) Present and past DIN diffusion range local distribution map. (B) Prediction and present DIN diffusion range local distribution map.
Therefore, in the distant future, the outlook for the marine environment of LZB is not optimistic if the reclamation activities are not controlled. Fortunately, the Chinese government has increased its focus on coastal ecosystems and environmental protection and tightened the approval process for coastal reclamation plans. Limiting land reclamation and strengthening environmental assessments in coastal areas by local governments is vital to ensure ecological functionality and ecosystem services (Duan et al., 2016; Wang et al., 2014; Miao and Xiu, 2021).
5 Conclusion
We employed the MIKE21 model to develop a two-dimensional hydrodynamic-pollutant transport model, investigating the impact of marine engineering projects on hydrodynamics and dispersion of DIN from rivers entering the LZB.
Under the same riverine input conditions, after the construction of marine engineering projects (in 2020), the dispersion area of high-concentration DIN entering LZB from the Yellow River increased. The flow velocity on both sides of the wave-breaking walls at the Xiaoqing River estuary decreased, and the dispersion of DIN to the sides was obstructed, resulting in an increase of 0.93 mg/L in DIN concentration at the Xiaoqing River mouth. Additionally, human activities such as land-based inputs and marine aquaculture can exacerbate water pollution in the bay. Therefore, if marine engineering construction is not controlled, it will have more severe negative impacts on the marine environment of LZB.
Furthermore, we did not consider the effects of factors such as seawater background values, biology, and chemistry, which presents certain limitations. In future research, the impact of marine engineering projects on pollutant dispersion under the combined action of multiple factors can be simulated. Overall, our results indicate that marine engineering projects have modified the hydrodynamic conditions in the nearshore regions of the bay, with projects in proximity to estuaries significantly influencing DIN dispersion and intensifying marine water quality pollution.
The findings from this study advance our comprehension of how marine engineering projects can affect hydrodynamics and pollutant dispersion, offering valuable guidance for the planning of future marine engineering projects to prevent ecological degradation in bays.
Data availability statement
The raw data supporting the conclusions of this article will be made available by the authors, without undue reservation.
Author contributions
ZW: Writing–original draft, Resources. RB: Funding acquisition, Project administration, Supervision, Writing–review and editing. WW: Funding acquisition, Supervision, Writing–review and editing. WL: Conceptualization, Writing–review and editing. XL: Methodology, Software, Writing–review and editing. JL: Resources, Writing–review and editing. WZ: Investigation, Writing–review and editing.
Funding
The author(s) declare that financial support was received for the research, authorship, and/or publication of this article. This study was financially supported by the Sub-project of Basic Condition Investigation and Evaluation of Bohai Region in Shandong (402001202000006_001) and the National Natural Science Foundation of China (NSFC; No. 42076037).
Acknowledgments
We would like to thank the Ocean University of China and the Qingdao Boyan Marine Environment Technology Co. for the current observation data, the Shandong Yellow River Administration Bureau of Yellow River Conservancy Commission for the Yellow River runoff data, the Shandong Provincial Hydrological Center for the Xiaoqing River hydrological data, and the Shandong Provincial Department of Ecology and Environment for the water quality data. We would like to thank Editage (www.editage.cn) for English language editing.
Conflict of interest
Author WW was employed by Shandong Blue Ocean Technology Co., Ltd. Author WL was employed by Qingdao Marine Geological Engineering Survey Institute Co., Ltd. Author WZ was employed by Qingdao Boyan Marine Environment Technology Co., Ltd.
The remaining authors declare that the research was conducted in the absence of any commercial or financial relationships that could be construed as a potential conflict of interest.
Publisher’s note
All claims expressed in this article are solely those of the authors and do not necessarily represent those of their affiliated organizations, or those of the publisher, the editors and the reviewers. Any product that may be evaluated in this article, or claim that may be made by its manufacturer, is not guaranteed or endorsed by the publisher.
References
Arikibe, J. E., and Prasad, S. (2020). Determination and comparison of selected heavy metal concentrations in seawater and sediment samples in the coastal area of Suva, Fiji. Mar. Pollut. Bull. 157, 111157. doi:10.1016/j.marpolbul.2020.111157
Bishop, M. J., Mayer-Pinto, M., Airoldi, L., Firh, L. B., Morris, R. L., Loke, L. H. L., et al. (2017). Effects of ocean sprawl on ecological connectivity: impacts and solutions. J. Exp. Mar. Biol. Ecol. 492, 7–30. doi:10.1016/j.jembe.2017.01.021
Bradley, P. B., Sanderson, M. P., Frischer, M. E., Brofft, J., Booth, M. G., Kerkhof, L. J., et al. (2010). Inorganic and organic nitrogen uptake by phytoplankton and heterotrophic bacteria in the stratified Mid-Atlantic Bight. Coast. Shelf Sci. 88 (4), 429–441. doi:10.1016/j.ecss.2010.02.001
Chan, M. W. H., Hasan, K. A., Balthazar-Silva, D., Mirani, Z. A., and Asghar, M. (2021). Evaluation of heavy metal pollutants in salt and seawater under the influence of the Lyari River and potential health risk assessment. Mar. Pollut. Bull. 166, 112215. doi:10.1016/j.marpolbul.2021.112215
Chen, H. Y., and Huang, S. Z. (2021). Composition and supply of inorganic and organic nitrogen species in dry and wet atmospheric deposition: use of organic nitrogen composition to calculate the Ocean's external nitrogen flux from the atmosphere. Cont. Shelf Res. 213, 104316. doi:10.1016/j.csr.2020.104316
Chen, N. W., Krom, M. D., Wu, Y. Q., Yu, D., and Hong, H. S. (2018). Storm induced estuarine turbidity maxima and controls on nutrient fluxes across river-estuary-coast continuum. Sci. Total Environ. 628-629, 1108–1120. doi:10.1016/j.scitotenv.2018.02.060
Chen, Y. Y., Song, D. H., Bao, X. W., and Yan, Y. H. (2019). Impact of the cross-bay bridge on water exchange in Jiaozhou Bay, Qingdao, China. Oceanol. Limnologia Sinica 50 (4), 12. doi:10.11693/hyhz20180900211
Chi, W. Q., Zhang, X. D., Zhang, W. M., Bao, X. W., Liu, Y. L., Xiong, C. B., et al. (2020). Impact of tidally induced residual circulations on chemical oxygen demand (COD) distribution in Laizhou Bay, China. Mar. Pollut. Bull. 151, 110811. doi:10.1016/j.marpolbul.2019.110811
Chusov, A., Lednova, J., and Shilin, M. (2012). Ecological assessment of dredging in the eastern Gulf of Finland. IEEE, 1–4. doi:10.1109/BALTIC.2012.6249169
Dafforn, K. A., Mayer-Pinto, M., Morris, R. L., and Waltham, N. J. (2015). Application of management tools to integrate ecological principles with the design of marine infrastructure. J. Environ. Manag. 158, 61–73. doi:10.1016/j.jenvman.2015.05.001
Delshab, H., Farshchi, P., Mohammadi, M., and Moattar, F. (2017). Preliminary assessment of heavy metal contamination in water and wastewater from Asaluyeh Port (Persian Gulf). Iran. J. Sci. Technol. Trans. A Sci. 41, 363–373. doi:10.1007/s40995-016-0031-x
DHI (2017). MIKE21 and MIKE 3 flow model FM hydrodynamic and transport module: scientific documentation. Hørsholm, Denmark: Danish Hydraulic Institute.
Duan, H. B., Zhang, H., Huang, Q. F., Zhang, Y. K., Hu, M. W., Niu, Y. N., et al. (2016). Characterization and environmental impact analysis of sea land reclamation activities in China. Ocean and Coast. Manag. 130, 128–137. doi:10.1016/j.ocecoaman.2016.06.006
El-Sorogy, A. S., Youssef, M., and Al-Hashim, M. H. (2023). Water quality assessment and environmental impact of heavy metals in the red sea coastal seawater of Yanbu, Saudi Arabia. Water 15 (1), 201. doi:10.3390/w15010201
Fan, X. Z., Dai, X. Y., Yang, G. J., Jia, Z. R., Liu, L. F., and Sun, N. (2017). Detecting artificialization process and corresponding state changes of estuarine ecosystems based on naturalness assessment. Ocean and Coast. Manag. 146, 178–186. doi:10.1016/j.ocecoaman.2017.07.007
Gao, G. D., Wang, X. H., and Bao, X. W. (2014). Land reclamation and its impact on tidal dynamics in Jiaozhou Bay, Qingdao, China. Estuar. Coast. Shelf Sci. 151, 285–294. doi:10.1016/j.ecss.2014.07.017
Gong, X. D., Yu, J., and Lan, Y. Y. (2019). Effects of reclamation on hydrodynamic environment in Semi-closed Bay. J. Huaqiao Univ. Nat. Sci. 40 (01), 72–78. doi:10.11830/ISSN.1000-5013.201808015
Halpern, B. S., Walbridge, S., Selkoe, K. A., Kappel, C. V., Micheli, F., D'Agrosa, C., et al. (2008). A global map of human impact on marine ecosystems. Science 319 (5865), 948–952. doi:10.1126/science.1149345
Han, H. H., Gao, F., Ding, D., and Ma, L. (2024). Influence of reclamation project on tidal volume and water exchange in Sanmen Bay. Mar. Geol. Front. 40 (5), 40–50. doi:10.16028/j.1009-2722.2022.237
Hasan, A. B., Reza, A. H. M. S., Siddique, M. A. B., Akbor, M. A., Nahar, A., Hasan, M., et al. (2023). Spatial distribution, water quality, human health risk assessment, and origin of heavy metals in groundwater and seawater around the ship-breaking area of Bangladesh. Environ. Sci. Pollut. Res. 30 (6), 16210–16235. doi:10.1007/s11356-022-23282-4
Huang, Z. K., and Huang, L. (2005). Tidal theory and calculation. Qingdao, China: China Ocean University Press.
Hudon, C., and Carignan, R. (2008). Cumulative impacts of hydrology and human activities on water quality in the St. Lawrence River (Lake Saint-Pierre, Quebec, Canada). Can. J. Fish. Aquatic Sci. 65 (6), 1165–1180. doi:10.1139/F08-069
Hudon, C., Gagnon, P., Rondeau, M., Hébert, S., Gilbert, D., Hill, B., et al. (2017). Hydrological and biological processes modulate carbon, nitrogen and phosphorus flux from the St. Lawrence River to its estuary (Quebec, Canada). Biogeochemistry 135 (3), 251–276. doi:10.1007/s10533-017-0371-4
Hung, J. J., Huang, W. C., and Yu, and C. S. (2013). Environmental and biogeochemical changes following a decade's reclamation in the Dapeng (Tapong) Bay, southwestern Taiwan. Estuar. Coast. Shelf Sci. 130, 9–20. doi:10.1016/j.ecss.2013.03.018
Hung, J. J., Hung, C. S., Wann, C. K., Hung, P. Y., and Kuo, F. (2020). Mercury distribution and speciation in two lagoons with different pollution and eutrophication conditions in Taiwan. Mar. Pollut. Bull. 156, 111096. doi:10.1016/j.marpolbul.2020.111096
Hung, J. J., and Hung, P. Y. (2003). Carbon and nutrient dynamics in a hypertrophic lagoon in southwestern Taiwan. J. Mar. Syst. 42 (3-4), 97–114. doi:10.1016/S0924-7963(03)00069-1
Ihsan, Y. N. (2020). Marine macro debris transport based on hydrodynamic model before and after reclamation in Jakarta Bay, Indonesia. Malays. J. Appl. Sci. 5 (2), 100–111. doi:10.37231/myjas.2020.5.2.241
Imalpen, I., Prartono, T., Rastina, R., Koropitan, A. F., and Yuliardi, A. Y. (2024). HYDRODYNAMICS hydrodynamics modeling in kendari bay, soucheast sulawesi, Indonesia. Int. J. Remote Sens. Earth Sci. (IJReSES) 21 (1), 54–65. doi:10.30536/j.ijreses.2024.v21.a3906
Jiang, H., Zhao, L., and Zhang, J. (2020). Budgets and transformation of dissolved inorganic and particulate organic nitrogen in the Yellow Sea: a model study. China Environ. Sci. 40 (9), 3981–3991. doi:10.19674/j.cnki.issn1000-6923.2020.0444
Jiang, T. T., Pan, J. F., Pu, X. M., Wang, B., and Pan, J. J. (2015). Current status of coastal wetlands in China: degradation, restoration, and future management. Estuar. Coast. Shelf Sci. 164, 265–275. doi:10.1016/j.ecss.2015.07.046
Kim, R., Kim, J., Ryu, J., and Koh, D. (2019). Hydrogeochemical characteristics of groundwater influenced by reclamation, seawater intrusion, and land use in the coastal area of Yeonggwang, Korea. Geosciences J. 23 (4), 603–619. doi:10.1007/s12303-018-0065-5
Kuang, C. P., Wang, D., Wang, G., Liu, J. T., Han, X. J., and Li, Y. (2024). Impact of reclamation projects on water quality in jinmeng bay, China. Estuar. Coast. Shelf Sci. 300, 108719. doi:10.1016/j.ecss.2024.108719
Lai, R. W. S., Perkins, M. J., Ho, K. K. Y., Astudillo, J. C., Yung, M. M. N., Russell, B. D., et al. (2016). Hong Kong’s marine environments: history, challenges and opportunities. Regional Stud. Mar. Sci. 8, 259–273. doi:10.1016/j.rsma.2016.09.001
Lee, J., Park, K. T., Lim, J. H., Yoon, J. E., and Kim, I. N. (2018). Hypoxia in Korean coastal waters: a case study of the natural Jinhae Bay and artificial Shihwa Bay. Front. Mar. Sci. 5, 70. doi:10.3389/fmars.2018.00070
Li, F. X., Ding, D. D., Chen, Z. J., Chen, H. H., Shen, T., Wu, Q. L., et al. (2020). Change of sea reclamation and the sea-use management policy system in China. Mar. Policy 115, 103861. doi:10.1016/j.marpol.2020.103861
Li, K., Liu, X., Zhao, X., and Guo, W. (2010). Effects of reclamation projects on marine ecological environment in Tianjin harbor industrial zone. Procedia Environ. Sci. 2, 792–799. doi:10.1016/j.proenv.2010.10.090
Lie, H. J., Cho, C. H., Lee, S., Kim, E. S., Koo, B. J., and Noh, J. H. (2008). Changes in marine environment by a large coastal development of the Saemangeum reclamation project in Korea. Ocean Polar Res. 30 (4), 475–484. doi:10.4217/OPR.2008.30.4.475
Lin, L., Liu, Z., Xie, L., Gao, H. W., Cai, Z. Y., Chen, Z. Y., et al. (2015). Dynamics governing the response of tidal current along the mouth of Jiaozhou Bay to land reclamation. J. Geophys. Res. Oceans 120 (4), 2958–2972. doi:10.1002/2014JC010434
Liu, B. J., Wu, X. Q., Du, P. P., Gao, M., and Du, X. Y. (2018). Assessment of potential pressure caused by sea use activities on the eco-environment of bays: a case study in Laizhou Bay, China. J. Mar. Sci. 36 (03), 76–83. doi:10.3969/j.issn.1001-909X.2018.03.008
Liu, J. Q. (2013). Numerical study on the environment effects of marine engineering on the mouth of Xiaoqing River in Laizhou Bay. Qingdao, China: Ocean University of China. doi:10.7666/d.y2158213
Liu, S. M., Li, L. W., Zhang, G. L., Liu, Z., Yu, Z., and Ren, J. L. (2012). Impacts of human activities on nutrient transports in the Huanghe (Yellow River) estuary. J. Hydrology 430-431, 103–110. doi:10.1016/j.jhydrol.2012.02.005
Liu, S. M., Qi, X. H., Li, X. N., Ye, H. R., Wu, Y., Ren, J. L., et al. (2016). Nutrient dynamics from the changjiang (Yangtze River) estuary to the east China sea. J. Mar. Syst. 154, 15–27. doi:10.1016/j.jmarsys.2015.05.010
Lv, T., Su, B., Wang, J. Y., Jin, Y., He, X., Yu, H. M., et al. (2017). The hydrodynamic environment variability of Laizhou Bay response to the marine engineering. Mar. Environ. Sci. 36 (04), 571–577. doi:10.13634/j.cnki.mes.2017.04.014
Lyu, H., Song, D. H., Zhang, S. F., Wu, W., and Bao, X. W. (2022). Compound effect of land reclamation and land-based pollutant input on water quality in Qinzhou Bay, China. Sci. Total Environ. 826, 154183. doi:10.1016/j.scitotenv.2022.154183
Maraqa, M. A., Ali, A., and Khan, N. (2007). Modeling selected water quality parameters at Jebel Ali harbour, dubai-UAE. J. Coast. Res. 50, 794–799. doi:10.2112/jcr-si50-148.1
Mazoyer, C., Vanneste, H., Dufresne, C., Ourmières, Y., Magaldi, M. G., and Molcard, A. (2020). Impact of wind-driven circulation on contaminant dispersion in a semi-enclosed bay. Estuar. Coast. Shelf Sci. 233, 106529. doi:10.1016/j.ecss.2019.106529
Mehlhorn, P., Viehberg, F., Kirsten, K., Newman, B., Frenzel, P., Gildeeva, O., et al. (2021). Spatial distribution and consequences of contaminants in harbour sediments–A case study from Richards Bay Harbour, South Africa. Mar. Pollut. Bull. 172, 112764. doi:10.1016/j.marpolbul.2021.112764
Meng, W. Q., Hu, B. B., He, M. X., Liu, B. Q., Mo, X. Q., Li, H. Y., et al. (2017). Temporal-spatial variations and driving factors analysis of coastal reclamation in China. Estuar. Coast. Shelf Sci. 191, 39–49. doi:10.1016/j.ecss.2017.04.008
Miao, D. D., and Xue, Z. H. (2021). The current developments and impact of land reclamation control in China. Mar. Policy 134, 104782. doi:10.1016/j.marpol.2021.104782
Muller, J. R. M., Chen, Y. P., Aarninkhof, S. G. J., Chan, Y. C., Piersma, T., van Maren, D. S., et al. (2020). Ecological impact of land reclamation on Jiangsu coast (China): a novel ecotope assessment for Tongzhou Bay. Water Sci. Eng. 13 (1), 57–64. doi:10.1016/j.wse.2020.04.001
Nour, H. E., and El-Sorogy, A. S. (2020). Heavy metals contamination in seawater, sediments and seashells of the Gulf of Suez, Egypt. Environ. Earth Sci. 79 (11), 274. doi:10.1007/s12665-020-08999-0
Nugroho, A., and Magdalena, I. (2020). Hydrodynamic model for investigating the impact of reclamation islands in Jakarta Bay to mangrove ecosystem in the area. AIP Conf. Proc. 2268, 050003. doi:10.1063/5.0016899
Okada, T., Nakayama, K., Takao, T., and Furukawa, K. (2011). Influence of freshwater input and bay reclamation on long-term changes in seawater residence times in Tokyo bay, Japan. Hydrol. Process. 25 (17), 2694–2702. doi:10.1002/hyp.8010
Osborne, E., Hu, X., Hall, E. R., Yates, K., Vreeland-Dawson, J., Shamberger, K., et al. (2022). Ocean acidification in the Gulf of Mexico: drivers, impacts, and unknowns. Prog. Oceanogr. 209, 102882. doi:10.1016/j.pocean.2022.102882
Pan, K., and Wang, W. X. (2012). Trace metal contamination in estuarine and coastal environments in China. Sci. Total Environ. 421-422, 3–16. doi:10.1016/j.scitotenv.2011.03.013
Panda, U. S., Mahanty, M. M., Ranga Rao, V., Patra, S., and Mishra, P. (2015). Hydrodynamics and water quality in chilika lagoon-A modelling approach. Procedia Eng. 116, 639–646. doi:10.1016/j.proeng.2015.08.337
Peng, H., Geng, H., Mao, X., Shi, J., and Lv, X. (2021). Study on total control of total nitrogen in the laizhou bay. WaterSwitzerl. 13 (17), 2439. doi:10.3390/w13172439
Percuoco, V. P., Kalnejais, L. H., and Officer, L. V. (2015). Nutrient release from the sediments of the great bay estuary, N.H. USA. Estuar. Coast. Shelf Sci. 161, 76–87. doi:10.1016/j.ecss.2015.04.006
Provoost, P., van Heuven, S., Soetaert, K., Laane, R. W. P. M., and Middelburg, J. J. (2010). Seasonal and long-term changes in pH in the Dutch coastal zone. Biogeosciences 7 (11), 3869–3878. doi:10.5194/bg-7-3869-2010
Rheuban, J. E., Doney, S. C., McCorkle, D. C., and Jakuba, R. W. (2019). Quantifying the effects of nutrient enrichment and freshwater mixing on coastal ocean acidification. J. Geophys. Res. Oceans 124 (12), 9085–9100. doi:10.1029/2019JC015556
Sany, S. B. T., Salleh, A., Sulaiman, A. H., Sasekumar, A., Rezayi, M., and Tehrani, G. M. (2013). Heavy metal contamination in water and sediment of the Port Klang coastal area, Selangor, Malaysia. Environ. Earth Sci. 69 (6), 2013–2025. doi:10.1007/s12665-012-2038-8
Sengupta, D., Choi, Y. R., Tian, B., Brown, S., Meadows, M., Hackney, C. R., et al. (2023). Mapping 21st century global coastal land reclamation. Earth's Future 11 (2), e2022EF002927. doi:10.1029/2022EF002927
Shan, J. Z., and Li, J. M. (2020). Valuing marine ecosystem service damage caused by land reclamation: insights from a deliberative choice experiment in Jiaozhou Bay. Mar. Policy 122, 104249. doi:10.1016/j.marpol.2020.104249
Shan, Z. X., Zheng, Z. H., Xing, H. Y., Liu, X. J., Liu, X. B., and Liu, Y. H. (2000). Study on eutrophication in laizhou bay of Bohai Sea. Trans. Oceanol. Limnol. 2000 (02), 41–46. doi:10.13984/j.cnki.cn37-1141.2000.02.008
Sharaan, M., Lebleb, A. A., ElZahar, M. M. H., and Iskander, M. (2022). Studying the tidal-induced water circulation pattern within EL-Burullus fishing harbor, Egypt, using CMS-PTM numerical modeling. Mar. Environ. Res. 180, 105726. doi:10.1016/j.marenvres.2022.105726
Shen, C. C., Shi, H. H., Li, F., Peng, S. T., and Ding, D. W. (2016). Study on the cumulative impact of reclamation activities on ecosystem health in coastal waters. Mar. Pollut. Bull. 103 (1-2), 144–150. doi:10.1016/j.marpolbul.2015.12.028
Shen, C. C., Zheng, W., Shi, H. H., Ding, D. W., and Wang, Z. L. (2015). Assessment and regulation of ocean health based on ecosystem services: case study in the Laizhou Bay, China. Acta Oceanol. Sin. 34 (12), 61–66. doi:10.1007/s13131-015-0777-6
Shen, Y. M., Zhang, H. X., and Tang, J. (2022). Hydrodynamics and water quality impacts of large-scale reclamation projects in the Pearl River Estuary. Ocean. Eng. 257, 111432. doi:10.1016/j.oceaneng.2022.111432
Shou, W. W., Zong, H. B., Ding, P. X., and Hou, L. J. (2018). A modelling approach to assess the effects of atmospheric nitrogen deposition on the marine ecosystem in the Bohai Sea, China. Coast. Shelf Sci. 208, 36–48. doi:10.1016/j.ecss.2018.04.025
Sridevi, B., and Sarma, V. V. S. S. (2021). Role of river discharge and warming on ocean acidification and pCO2 levels in the Bay of Bengal. Tellus B Chem. Phys. Meteorology 73 (1), 1971924. doi:10.1080/16000889.2021.1971924
Su, B., Zhu, J. L., Wang, J. Y., Zhao, Y. T., Wang, L. M., and Ma, Y. Q. (2021). A simulation study on influence of the construction of Jiaolai Canal on the water exchange and marine environment in the Laizhou Bay. Coast. Eng. 40 (01), 48–58. doi:10.3969/j.issn.1002-3682.2021.01.006
Tay, J. Y., Wong, S. K., Chou, L. M., and Todd, P. A. (2018). Land reclamation and the consequent loss of marine habitats around the Ayer Islands, Singapore. Nat. Singap. 11, 1–5. doi:10.24192/nis.2018.11.001
Tian, B., Wu, W. T., Yang, Z. Q., and Zhou, Y. X. (2016). Drivers, trends, and potential impacts of long-term coastal reclamation in China from 1985 to 2010. Estuar. Coast. Shelf Sci. 170, 83–90. doi:10.1016/j.ecss.2016.01.006
Tian, Y., Yu, D. Y., and Li, Y. L. (2018). Research on the accumulation of marine environment in Laizhou Bay. Periodical Ocean Univ. China 48 (01), 117–124. doi:10.16441/j.cnki.hdxb.20150266
Wakelin, S. L., Artioli, Y., Butenschön, M., Icarus Allen, J., and Holt, J. T. (2015). Modelling the combined impacts of climate change and direct anthropogenic drivers on the ecosystem of the northwest European continental shelf. J. Mar. Syst. 152, 51–63. doi:10.1016/j.jmarsys.2015.07.006
Wang, C. Y., Lv, Y. C., and Li, Y. W. (2018). Riverine input of organic carbon and nitrogen in water-sediment system from the Yellow River estuary reach to the coastal zone of Bohai Sea, China. Cont. Shelf Res. 157, 1–9. doi:10.1016/j.csr.2018.02.004
Wang, L., Chen, Y., Zhang, L. J., Ma, S. Q., Xu, Y. D., Lou, A. G., et al. (2021a). A study on effects of reclamation on tidal flow field and tidal prism of Zhifu Bay. Trans. Oceanol. Limnol. 43, 32–39. doi:10.13984/j.cnki.cn37-1141.2021.02.005
Wang, W., Liu, H., Li, Y. Q., and Su, J. L. (2014). Development and management of land reclamation in China. Ocean and Coast. Manag. 102, 415–425. doi:10.1016/j.ocecoaman.2014.03.009
Wang, X., Chen, W. Q., Zhang, L. P., Jin, D., and Lu, C. Y. (2010). Estimating the ecosystem service losses from proposed land reclamation projects: a case study in Xiamen. Ecol. Econ. 69 (12), 2549–2556. doi:10.1016/j.ecolecon.2010.07.031
Wang, Z. C., Gao, Z. Q., Shang, W. T., and Jiang, X. P. (2021b). Land use change and its influence on coastline change along Laizhou Bay during 1987-2017. Bull. Soil Water Conservation 41 (01), 309–316. doi:10.13961/j.cnki.stbctb.2021.01.042
Wei, Y. Q., Cui, H. W., Hu, Q. J., Bai, Y., Qu, K. M., Sun, J., et al. (2022). Eutrophication status assessment in the laizhou bay, Bohai Sea: further evidence for the ecosystem degradation. Mar. Pollut. Bull. 181, 113867. doi:10.1016/j.marpolbul.2022.113867
Wei, Y. Q., Liu, H. J., Zhang, X. D., Xue, B., Munir, S., and Sun, J. (2017). Physicochemical conditions in affecting the distribution of spring phytoplankton community. Chin. J. Oceanol. Limnol. 35 (6), 1342–1361. doi:10.1007/s00343-017-6190-6
Wibowo, M. (2018). Study on the effect of reclamation to seawater quality in makasar city with numerical modeling. IOSR J. Environ. Sci. 12 (11), 80–89. doi:10.9790/2402-1211018089
Xiangyang, T. U., Wenbin, L. V., and Menwu, W. U. (2023). Numerical simulation study on water exchange capacity of colombo port engineering under the control of ocean current and tidal power. Int. Conf. Energy Environ. Sci., 49–62. doi:10.1007/978-3-031-32068-2_5
Xie, L., Gao, X. L., Liu, Y. L., Yang, B., Lv, X. Q., and Zhao, J. M. (2021). Perpetual atmospheric dry deposition exacerbates the unbalance of dissolved inorganic nitrogen and phosphorus in coastal waters: a case study on a mariculture site in North China. Mar. Pollut. Bull. 172, 112866. doi:10.1016/j.marpolbul.2021.112866
Xu, D. X., and Zhang, G. X. (2007). Impact of human activities on coastal wetlands in China. Wetl. Sci. 5 (03), 282–288. doi:10.13248/j.cnki.wetlandsci.2007.03.013
Xu, J., Yin, K., Liu, H., Lee, J. H. W., Anderson, D. M., Ho, A. Y. T., et al. (2010). A comparison of eutrophication impacts in two harbours in Hong Kong with different hydrodynamics. J. Mar. Syst. 83, 276–286. doi:10.1016/j.jmarsys.2010.04.002
Xu, T., and You, X. Y. (2017). Effects of large-scale embankments on the hydrodynamics and salinity in the Oujiang River Estuary, China. J. Mar. Sci. Technol. 22, 71–84. doi:10.1007/s00773-016-0394-x
Xu, Y., Cai, Y. P., Sun, T., Yin, X. A., and Tan, Q. (2017). Development of an integrated indicator system to assess the impacts of reclamation engineering on a river estuary. Mar. Pollut. Bull. 199 (2), 50–59. doi:10.1016/j.marpolbul.2017.03.015
Yamamoto, T., Madhusoodhanan, R., Sarkar, A., Alghunaim, A., Al-Said, T., Fernandes, L., et al. (2024). Response of hydrodynamic and physico-chemical conditions to engineered forcing in an enclosed bay. Estuar. Coast. Shelf Sci. 305, 108854. doi:10.1016/j.ecss.2024.108854
Yamamoto-Kawai, M., Ito, S., Kurihara, H., and Kanda, J. (2021). Ocean acidification state in the highly eutrophic Tokyo Bay, Japan: controls on seasonal and interannual variability. Front. Mar. Sci. 8, 642041. doi:10.3389/fmars.2021.642041
Yanagu, T., and Onishi, K. (1999). Change of tide, tidal current, and sediment due to reclamation in Tokyo Bay. Oceanogr. Jpn. 8 (6), 411–415. doi:10.5928/kaiyou.8.411
Yang, H. Y., Hu, Y. F., Li, J. H., Wang, H. Q., Liu, Z. H., and Li, G. Y. (2023). Investigation of push-flow aeration on improving water quality in urban lakes. Environ. Technol. and Innovation 32, 103247. doi:10.1016/j.eti.2023.103247
Zainol, Z., Akhir, M. F., and Abdullah, S. (2020). Hydrodynamics, nutrient concentrations, and phytoplankton biomass in a shallow and restricted coastal lagoon under different tidal and monsoonal environmental drivers. Regional Stud. Mar. Sci. 38, 101376. doi:10.1016/j.rsma.2020.101376
Zeng, W. T., Zhang, D. Q., Liu, B., Yang, Y. P., Zhang, H. F., Wu, D. Y., et al. (2023). Distribution, main controlling factors and pollution assessment of heavy metals in surface seawater of the Northern Bay of Hainan Island, south China. J. Trop. Oceanogr. 42 (6), 156–167. doi:10.11978/2023004
Zhang, H., Shen, Y., and Tang, J. (2021). Hydrodynamics and water renewal in the Pearl River Estuary, China: a numerical study from the perspective of water age. Ocean. Eng. 237, 109639. doi:10.1016/j.oceaneng.2021.109639
Zhang, H. X., Shen, Y. M., and Tang, J. (2023). Numerical investigation of successive land reclamation effects on hydrodynamics and water quality in Bohai Bay. Ocean. Eng. 268, 113483. doi:10.1016/j.oceaneng.2022.113483
Zhang, J. (1996). Nutrient elements in large Chinese estuaries. Cont. Shelf Res. 16 (8), 1023–1045. doi:10.1016/0278-4343(95)00055-0
Zhang, J. M., Liu, S., Zhang, Q., and Liu, Y. T. (2008). Nutrient distribution and eutrophication assessment for the adjacent waters of the Yellow River Estuary. Mar. Sci. Bull. 27 (05), 65–72. doi:10.3969/j.issn.1001-6392.2008.05.010
Zhang, M., Lu, Q. Y., Wang, D. W., Ding, D. S., Cui, Z. G., and Shi, H. H. (2022). Spatiotemporal evolution of nutrients and the influencing factors in Laizhou Bay over the past 40 years. Mar. Pollut. Bull. 184, 114186. doi:10.1016/j.marpolbul.2022.114186
Zhang, P., Su, Y., Liang, S. K., Li, K. Q., Li, Y. B., and Wang, X. L. (2017). Assessment of long-term water quality variation affected by high-intensity land-based inputs and land reclamation in Jiaozhou Bay, China. Ecol. Indic. 75, 210–219. doi:10.1016/j.ecolind.2016.12.035
Zhang, X., Zhang, L. J., Hou, Z. L., and Liao, W. (2012). Spatio-temporal variability of principal pollutants in Laizhou Bay from 1980 to 2008. Periodical Ocean Univ. China 42 (11), 91–98. doi:10.16441/j.cnki.hdxb.2012.11.015
Zhao, P., Jiang, W. S., Mao, X. Y., Gao, H. W., and Guo, X. Y. (2010). Salinity change and influencing factor in the Laizhou Bay from 2000 to 2005. Oceanol. Limnologia Sinica 41 (01), 12–23. doi:10.11693/hyhz201001002002
Zheng, L. W., Zhai, W. D., Wang, L. F., and Huang, T. (2020). Improving the understanding of central Bohai Sea eutrophication based on wintertime dissolved inorganic nutrient budgets: roles of north Yellow Sea water intrusion and atmospheric nitrogen deposition. Environ. Pollut. 267, 115626. doi:10.1016/j.envpol.2020.115626
Zhu, G. R., Xie, Z. L., Xu, X. G., Ma, Z. W., and Wu, Y. L. (2016). The landscape change and theory of orderly reclamation sea based on coastal management in rapid industrialization area in Bohai Bay, China. Ocean and Coast. Manag. 133, 128–137. doi:10.1016/j.ocecoaman.2016.09.016
Zhu, L. H., Hu, R. J., Zhu, H. J., Jiang, S. H., and Wang, N. (2018). Modeling studies of tidal dynamics and the associated responses to coastline changes in the Bohai Sea, China. Ocean. Dyn. 2018 (1), 1625–1648. doi:10.1007/s10236-018-1212-2
Keywords: Laizhou Bay, marine engineering projects, MIKE 21, hydrodynamics, DIN
Citation: Wang Z, Bao R, Wang W, Li W, Leng X, Liang J and Zhang W (2024) Impacts of a marine engineering project on hydrodynamics and diffusion characteristics of fluvial pollutants in Laizhou Bay, China. Front. Earth Sci. 12:1432090. doi: 10.3389/feart.2024.1432090
Received: 13 May 2024; Accepted: 24 September 2024;
Published: 16 October 2024.
Edited by:
Vlad Constantin Manea, National Autonomous University of Mexico, MexicoReviewed by:
Changchun Huang, Nanjing Normal University, ChinaMarta Rodrigues, National Laboratory for Civil Engineering, Portugal
Copyright © 2024 Wang, Bao, Wang, Li, Leng, Liang and Zhang. This is an open-access article distributed under the terms of the Creative Commons Attribution License (CC BY). The use, distribution or reproduction in other forums is permitted, provided the original author(s) and the copyright owner(s) are credited and that the original publication in this journal is cited, in accordance with accepted academic practice. No use, distribution or reproduction is permitted which does not comply with these terms.
*Correspondence: Weizhong Wang, bHp3d3oxMzlAMTI2LmNvbQ==