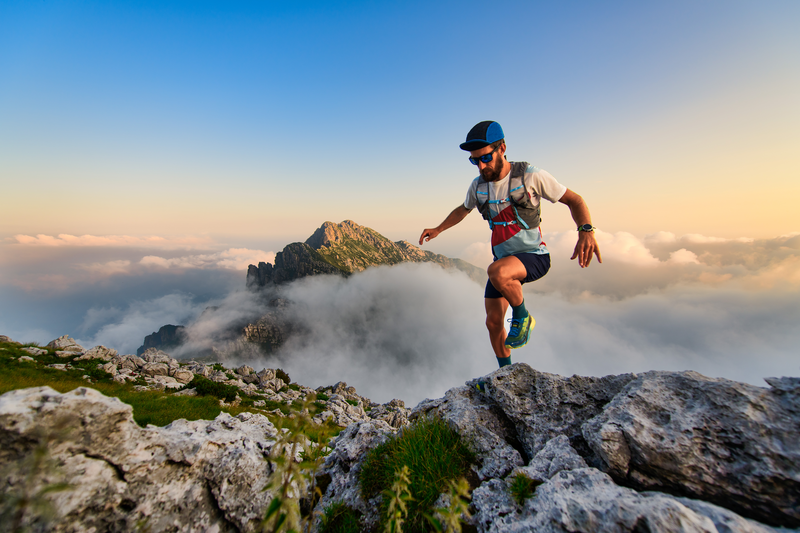
94% of researchers rate our articles as excellent or good
Learn more about the work of our research integrity team to safeguard the quality of each article we publish.
Find out more
ORIGINAL RESEARCH article
Front. Earth Sci. , 02 August 2024
Sec. Structural Geology and Tectonics
Volume 12 - 2024 | https://doi.org/10.3389/feart.2024.1430091
This article is part of the Research Topic Research Progress on the Formation Mechanism, Controlling Factors and Hydrocarbon Accumulation Patterns of Unconventional Oil and Gas Reservoirs Worldwide View all 9 articles
Natural fractures are widely developed and distributed in tight sandstone reservoirs in the western Sichuan Basin, China, influenced by complex tectonic movements and diagenetic processes. These natural fractures serve as the main flow channels and important storage spaces in such reservoirs. Understanding their development characteristics and controlling factors determines the positioning and production efficiency of tight gas wells. This paper first explores the types and characteristics of natural fractures in the second member of the Upper Triassic Xujiahe Formation (T3x2) in the western Sichuan Basin through outcrop investigations, core observations, image log interpretations, and thin section analyses. We study the main influencing factors controlling the development and distribution of tectonic shear fractures by combining the characterization of fracture attributes. The results show that the tight sandstone reservoirs in the T3x2 mainly contain tectonic fractures, diagenetic fractures, and overpressure fractures, among which shear fractures in tectonic fractures are the main types. There are four sets of tectonic shear fractures in the study area, oriented in the E-W, N-S, NE-SW, and NW-SE directions. The dip angles of fractures range from 20° to 80°, with an average dip angle of 53°, predominantly consisting of high-angle fractures. The lengths of fractures are mainly within 100 cm, with a mean value of 47.53 cm, and 76.2% of fractures have lengths less than 50 cm. Fracture apertures range from 2.51 to 163.19 μm, with an average of 30.54 μm. The proportion of effective fractures in tectonic shear fractures reaches 85.7%. The development of tectonic shear fractures is primarily influenced by lithology, rock mechanics stratigraphy, diagenetic processes and facies, and faults. Lithology stands as the fundamental factor influencing the degree of fracture development in tight reservoirs. In rocks with the same composition, as the grain size decreases, the degree of fracture development increases. Simultaneously, with the rise in muddy content, fracture development diminishes in siltstone, argillaceous siltstone, silty mudstone, and mudstone. The formation and distribution of fractures are governed by rock mechanics stratigraphy, primarily manifested as a decrease in fracture density with an increase in rock mechanics layer thickness within a certain range. Diagenetic processes affect the abundance of tectonic shear fractures by influencing the mechanical properties of rocks. The stronger the compaction and cementation, the poorer the physical properties, and the higher the brittleness of the rocks, resulting in a greater abundance of tectonic shear fractures. Rocks with strong compaction and robust cementation facies exhibit high brittleness, rendering them more susceptible to fracturing under identical stress conditions. Fractures are highly prevalent in both the hanging wall and footwall of faults. As the distance from the fault increases, the linear density of fractures significantly decreases, owing to the uneven distribution of stress perturbation caused by fault activity.
Tight sandstone reservoirs play an important role in the global production of hydrocarbons (Khlaifat et al., 2011; Wang et al., 2023). Currently, commercial development of tight oil and gas resources has been achieved in multiple basins worldwide. For example, in North America, this includes the Williston, San Juan, Alberta, and Appalachian basins (Law, 2002; Hart, 2006; Zou et al., 2011; Friesen et al., 2017). In China, significant progress has been made in the Ordos, Junggar, Songliao, and Western Sichuan basins (Zou et al., 2012; Wang et al., 2023; Jiang et al., 2023), all of which still hold considerable potential for hydrocarbon production. However, the low structural and compositional maturity of the original sediment, coupled with deep burial depths (mostly exceeding 4,000 m) and intense diagenesis (primarily compaction and cementation), lead to the low matrix properties and strong heterogeneity of tight sandstone, posing significant challenges for exploration and development (Olson et al., 2009; Gong et al., 2019b; Zeng et al., 2023b; Zou et al., 2023).
Influenced by multiple stages of tectonic deformation and diagenetic evolution, various types and scales of natural fractures are widely developed in tight sandstone reservoirs (Zeng et al., 2023a). These natural fractures serve as crucial storage spaces and primary flow channels in tight reservoirs, controlling the accumulation and migration of tight oil and gas resources (Zeng et al., 2010; Zhang et al., 2021a). Additionally, natural fractures influence the extent and scope of hydraulic fracturing, determining the pathways and production capacity of hydrocarbons (Chang et al., 2020; Fu and Dehghanpour, 2020). Therefore, understanding the characteristics, formation mechanisms, and distribution patterns of natural fractures is crucial for the exploration and development of tight oil and gas resources (Lee et al., 2018; Lyu et al., 2019).
The controlling factors of fractures can be summarized as internal, dynamic, heterogeneity, and evolution factors (Faulkner et al., 2010; Fossen, 2010; Ameen et al., 2012; Ferrill et al., 2021). Internal factors are reflected in the differences in fracture development intensity caused by lithology, sedimentary microfacies, and diagenetic facies (Fossen and Bale, 2007; Laubach and Diaz-Tushman, 2009; Gale et al., 2014). Dynamic factors include structure and pore pressure (Torabi and Berg, 2011; Zanella et al., 2015). Heterogeneity factors involve the impact of the heterogeneity of rock mechanical properties and preexisting fractures on fracture development (Zeng et al., 2023a). Evolution factors are represented by the states of vertical principal stress (σV), maximum horizontal principal stress (σHmax), and minimum horizontal principal stress (σHmin), which determine the formation of fractures (Ferrill et al., 2021). Analyzing the controlling factors of fractures is a key aspect of predicting and evaluating reservoir fractures, as it can improve the predictability of fracture distribution (Olson et al., 2009). However, under different geological conditions, there are significant differences in the development intensity of fractures in tight sandstone reservoirs, and there are many controlling factors affecting fracture development (Zeng and Li, 2009; Gong et al., 2019a; Lyu et al., 2019). Therefore, it is particularly important to clarify the controlling factors of fractures for specific study areas.
The western Sichuan Basin is an important area for natural gas production in China (Xu et al., 2015). After half a century of continuous exploration practices, significant achievements have been made in natural gas exploration successively in the Triassic Leikoupo and Xujiahe formations, as well as the Jurassic Shaximiao Formation (Deng et al., 2022; Wang et al., 2022; Su et al., 2023). The Xujiahe Formation in the western Sichuan region has demonstrated tremendous exploration and development potential. By the end of 2023, the geological reserves of natural gas in this formation had reached 10200 × 108 m3 (Xiong et al., 2024). The matrix porosity and permeability of reservoirs in the Xujiahe Formation in the western Sichuan region are generally poor. Storage space primarily comprises secondary pores (approximately 75%) and primary pores (approximately 25%), with natural fractures being widespread in the reservoirs (Zhang et al., 2021b; Yang et al., 2021). Due to the combined influence of complex sedimentary evolution characteristics, multiple tectonic backgrounds, and multi-stage diagenetic processes, the reservoirs in the Xujiahe Formation in the western Sichuan region are tight, highly heterogeneous, and exhibit complex relationships in the distribution of natural gas and water (Zou et al., 2009). Currently, through studies on tectonic features, sequence and sedimentary characteristics, hydrocarbon source rocks and reservoir features, as well as the conditions and distribution patterns of oil and gas accumulation, valuable insights have been gained for predicting sweet spots within the region and adjusting well deployment schemes (Yang et al., 2012). Previous studies have analyzed the natural fracture characteristics, genesis mechanisms, controlling factors, and their impacts on oil and gas productivity within the Xujiahe Formation in specific areas such as Xinchang, Qiongxi, Pingluoba, and Jiange (Zeng, 2010; Lyu et al., 2017; Zhang et al., 2021b). However, there is a lack of comprehensive understanding regarding the development characteristics and controlling factors of natural fractures in the Xujiahe Formation in the western Sichuan region, which will impede the efficient exploration and development of tight gas in the western Sichuan region.
This paper analyzes the types of natural fractures in the T3x2 tight sandstone reservoirs in the western Sichuan Basin using outcrop, core, imaging logging, and thin-section data, clarifying the development characteristics of fractures. Additionally, it discusses the controlling factors affecting natural fractures in tight sandstone reservoirs through statistical and experimental data on fracture attribute parameters. The research findings can provide a geological basis for the accurate evaluation and efficient development of tight reservoirs in the western Sichuan Basin. This aims to support a deeper understanding of the development characteristics and distribution patterns of natural fractures in tight reservoirs.
The Sichuan Basin, located in the southwest of China, is one of the most significant hydrocarbon-rich regions. The Western Sichuan Basin is situated to the east of the Longmen Mountain thrust belt, connected to the Micangshan thrust belt in the north, the Emei-Wanshan faulted and folded zone in the south, and the Middle Sichuan Uplift in the east (Figure 1). Since its formation in the Late Triassic, the basin has undergone multiple tectonic movements, including the Indosinian, Yanshan, and Xishan events (Wang et al., 2020). These multiple phases of activity have resulted in the formation of several structural movement interfaces within the basin, as well as major structures trending in a NE-SW direction and a nearly N-S direction (Li et al., 2011).
Figure 1. Locations of (A) the Western Sichuan Basin in China, (B) wells and outcrops in the Western Sichuan Basin (modified from Zeng, 2010).
During the early Late Triassic, the Ma’antang and Xiaotangzi formations in the western part of the basin, along with the Middle Triassic Leikoupo Formation, were deposited in marine or transitional marine-continental environments. From the Late Triassic Xujiahe Formation to the thick marine-continental transitional and continental strata of the Cretaceous (Yu et al., 2016), sedimentary environments of the clastic sediments of the Xujiahe Formation include alluvial fans, braided deltas, shallow lakes, and braided rivers (Zhu et al., 2009). The Xujiahe Formation can be divided into five members from bottom to top, with the sixth member eroded due to uplift from the Indosinian late-stage movement (Lin et al., 2022). The first, third, and fifth members of the Xujiahe Formation, characterized by dark mudstone, coal, and siltstone, serve as the main hydrocarbon source rocks (Figure 2). The second and fourth members, composed of sandstone, are the main reservoirs and serve as important target layers for Upper Triassic natural gas exploration (Figure 2). In the Western Sichuan Basin, the second member of the Xujiahe Formation has a thickness ranging from 479 to 780 m. Its lower part consists of medium and fine sandstones interbedded with minor mudstones, its middle part is characterized by interbedded mudstones, siltstones, and fine sandstones with minor coal seams, and its upper part comprises fine and medium sandstones interbedded with siltstones and mudstones (Guo et al., 2012).
Figure 2. The stratigraphic framework of the Western Sichuan Basin (modified from Gong et al., 2016; He et al., 2019).
This study analyzed the developmental characteristics of natural fractures in the tight sandstone reservoirs of the T3x2 in the western Sichuan Basin using outcrop, core, imaging logging, and thin-section data (Table 1). Specifically, favorable outcrop conditions of the T3x2 tight sandstone reservoirs were observed in the northwest part of the western Sichuan Basin, where the development of fractures along a cumulative 125.5 m geological profile was analyzed. Fracture features of 1059.99 m of core from 18 wells were observed, and 150 samples were selected for thin-section preparation (sized at 30 mm × 45 mm × 30 mm). Additionally, interpretation data from 11 imaging logging wells, totaling 2459.47 m, was analyzed to further evaluate attributes such as fracture orientation, aperture, and density.
Through field outcrop observations and core analysis, key attributes of fractures such as orientation, dip, scale, density, and filling degree were directly measured using tools like tape measures, compasses, and goniometers. Additionally, the types of fracture fillings were identified using diluted hydrochloric acid. Fracture density in cores and outcrops is represented by line density, indicating the number of fractures intersecting a straight line perpendicular to the fracture trend divided by the length of this line, expressed in m-1.
Imaging logging provides high-resolution 360° circumferential two-dimensional images of the rock formation, displaying differences in resistivity or acoustic impedance caused by lithology, fractures, pores, and bedding features as different color codes on the image (Lai et al., 2018). One type of imaging logging is Electrical Borehole Imaging Logs (X-tended Range Micro Imager (XRMI)). In XRMI images, resistive rocks appear bright, while conductive materials appear dark. XRMI can be used to characterize natural fractures when the fractures are filled with electrically contrasting fluids and solids (Lyu et al., 2017). For fracture analysis, imaging can distinctly reflect attributes such as orientation, aperture, density, and filling of individual fractures (Khoshbakht et al., 2012).
Microscopic fracture analysis requires the use of a microscope (Anders et al., 2014). We utilized a Leica DM4500P polarizing microscope for identification and strictly followed the standards outlined in the Chinese petroleum and gas industry standard SY/T5368—2000. In thin sections, fracture density is characterized by surface density, which is the ratio of the cumulative length of fractures measured directly on the thin section to the total area of the thin section, expressed in cm/m2.
Outcrop surveys, core observations, and interpretations from imaging logging reveal extensive fracture development within the T3x2 tight sandstone reservoirs in the western Sichuan Basin. These fractures have been categorized into three types based on their geological origins: tectonic fractures, diagenetic fractures, and overpressure fractures. Among these, tectonic fractures emerge as the dominant natural fracture type in the study area.
Fractures formed and distributed under the direct or indirect influence of local tectonic events or stress fields are referred to as tectonic fractures (Aguilera, 1988). Combining the stress properties of rocks, tectonic fractures can be further divided into tectonic shear fractures and tensile fractures (Zeng, 2008). Tectonic shear fractures are formed by shear forces, with fractures distributed along the maximum shear stress plane at a certain acute angle to the maximum principal stress direction, and the displacement direction of the fracture is parallel to the fracture surface. Stable orientations characterize tectonic shear fractures and have a large extension range (Figures 3A, B, 5A). The surfaces of these fractures are smooth (Figure 3C), resembling mirror surfaces, but significant shear features such as scratches and steps may also appear. Some of these fractures cut through rock layers, while others develop within them (Figures 3A,B). Additionally, tectonic shear fractures may be filled with minerals such as calcite and quartz. In imaging logging images, tectonic shear fractures typically appear as dark sinusoidal shapes (Figure 4).
Figure 3. The natural fractures in outcrops and cores of the Upper Triassic Xujiahe Formation in the western Sichuan Basin. (A) Tectonic shear fractures in outcrops, cutting across rock layers, B1 outcrop field section; (B) Tectonic shear fractures in outcrops, constrained by rock layers B2 outcrop field section; (C) Surface of tectonic shear fractures and bedding fractures, A1 well, depth 3826.13 m; (D) Tensile fractures, A2 well, depth 3738.38 m. See Figure 1B for the outcrop and well locations.
Figure 4. Natural fractures and their orientations in the imaging logging data (XRMI) of the T3x2 tight sandstone reservoirs in the western Sichuan Basin, from well A3. See Figure 1B for the well location.
Tensile fractures are a type of tectonic fracture formed when the tensile stress within the rock exceeds the tensile strength of the rock (Ding et al., 2015). These fractures are distributed along planes formed by the maximum and intermediate principal stresses, perpendicular to the direction of the minimum principal stress, and the displacement direction of the fracture is perpendicular to the fracture surface. These fractures exhibit unstable orientations, have a limited extension range, and appear rough and irregular. They typically have a morphology characterized by a wider middle section and narrower sides, resembling lenses, and are often filled with asphalt (Figure 3D).
During the processes of sedimentation and diagenesis, fractures that form within rock layers due to various geological processes are referred to as diagenetic fractures (Zeng et al., 2022). Among these, fractures that occur nearly horizontally along bedding planes due to compaction and pressure dissolution during diagenesis are called bedding fractures, which are the most predominant type of diagenetic fractures in the study area. These fractures typically exhibit a discontinuous distribution along bedding planes and tend to bypass mineral grains, often branching at their ends (Figure 5B). Fractures resulting from compaction-induced particle breakage or linear contact between particles are known as intergranular and grain-edge fractures (Figure 5C). Intergranular fractures primarily develop within brittle grains, appearing as micro-cracks within quartz, feldspar, or calcite grains without cutting through mineral grains. Grain-edge fractures, on the other hand, are mainly located near the edges of linearly contacting grains.
Figure 5. Different types of microfractures in the T3x2 tight sandstone reservoirs in the western Sichuan Basin. (A) Tectonic fractures, from well A4, depth 3896.5 m; (B) Bedding fracture, from well A5, depth 4257.3 m; (C) Intragranular fractures and grain-edge fractures, from well A6, depth 4384.4 m; (D) Overpressure fractures, from well A7, depth 3995.7 m. See Figure 1B for the well locations.
Overpressure fractures are formed due to the action of abnormal formation pressure, with their specific stress state mainly influenced by abnormal high-pressure fluids (Zeng, 2010). These fractures typically appear in clusters, exhibiting mixed occurrences, indefinite orientations, limited extension lengths, and uneven apertures, among other typical characteristics (Figure 5D). These fractures are often completely or partially filled with asphalt, thereby limiting their effectiveness.
Various types of natural fractures develop in the T3x2 tight sandstone reservoirs of the western Sichuan Basin. The developmental characteristics of these different types of fractures directly influence their contribution to the petrophysical properties of the tight sandstone reservoirs. Compared to other types of fractures present in the study area, tectonic shear fractures exhibit larger scale, higher density, and wider apertures. In the study area, tectonic shear fractures demonstrate better effectiveness in controlling reservoir fluid flow capacity and enhancing hydrocarbon production efficiency compared to tectonic tensile fractures, diagenetic fractures, and overpressure fractures. Therefore, this paper focuses on quantitatively characterizing the developmental characteristics of tectonic shear fractures.
Outcrop surveys, core observations, and imaging logging interpretations indicate that in the T3x2 tight sandstone reservoirs in the western Sichuan Basin, there are mainly four sets of tectonic shear fractures, including those in the E-W, N-S, NE-SW, and NW-SE directions (Figure 6A). These four sets of tectonic shear fractures account for 77.9% of the total number of fractures, while fractures in other directions are less common. The dip angles of the tectonic shear fractures range from 20° to 80°, with an average dip angle of 53°. High-angle fractures (>60°) account for 47.98% of all fractures, while medium-angle (30°–60°) fractures account for 24.06%, and low-angle (<30°) fractures account for 27.96% (Figure 6B). The T3x2 tight sandstone reservoirs in the western Sichuan Basin have a high proportion of low-angle tectonic shear fractures. This is due to the compressional tectonic forces in the foreland basin, resulting in thrust faulting or interbedded sliding, which has formed a large number of low-angle tectonic shear fractures (Zeng et al., 2009).
Figure 6. Occurrence parameters of tectonic shear fractures in the T3x2 tight sandstone reservoirs, western Sichuan Basin. (A) Strike rose diagram of tectonic shear fractures derived from field outcrop measurements and imaging logging interpretations (N = 1360). (B) Frequency histogram of the dip angle distribution of tectonic shear fractures derived from field outcrop measurements, core observations, and imaging logging interpretations (N = 1978).
Fracture scale can be evaluated using the aperture and length of the fractures. Fracture length refers to the longitudinal extension length of the fractures in the direction of their orientation. Outcrop survey results indicate that approximately 60.2% of the tectonic shear fractures are shorter than 50 cm, about 24.3% range between 50 and 100 cm, and around 15.5% exceed 100 cm in length (Figure 7). Imaging logging interpretation results show that approximately 75.2% of the fractures are shorter than 50 cm, about 14.7% range between 50 and 100 cm, and around 10.1% exceed 100 cm in length (Figure 7). Core observation results reveal that approximately 93.2% of the fractures are shorter than 50 cm, about 5.7% range between 50 and 100 cm, and around 1.1% exceed 100 cm in length (Figure 7). Overall, the lengths of tectonic shear fractures in the study area are mainly distributed within 100 cm, with a mean value of 47.53 cm. Additionally, 76.2% of the fractures have lengths less than 50 cm (Figure 7). Some fractures exhibit a wider range of extension lengths, with approximately 8.9% of fractures having lengths exceeding 100 cm (Figure 7). These statistical results are obtained from outcrop observations and imaging logging interpretations used to interpret larger-scale fractures. Due to limitations in core sampling, fractures observed in the cores are expected to have larger scales.
Figure 7. Frequency histogram of the length distribution of tectonic shear fractures in the T3x2 tight sandstone reservoirs in the western Sichuan Basin.
Fracture aperture refers to the vertical distance between the two walls of a fracture. Although fracture aperture can be directly measured from core samples, the extracted cores release the pressure of overlying formations at the surface, leading to values that significantly exceed the true underground aperture. Therefore, this study utilizes fracture aperture obtained from more reliable imaging logging interpretations. The imaging interpretation results indicate that fracture apertures in the study area range from 2.51 to 163.19 μm, with an average of 30.54 μm (Figure 8). Fractures with apertures <30 μm account for 61.1% of the total, while fractures with apertures greater than 50 μm also represent a significant proportion, approximately 7.7% (Figure 8).
Figure 8. Frequency histogram of the aperture distribution of tectonic shear fractures in the T3x2 tight sandstone reservoirs in the western Sichuan Basin. The data is sourced from imaging logging interpretations (N = 738).
Following the formation of natural fractures, they may undergo a filling process wherein minerals occupy the effective space within them, thereby influencing the capacity of the reservoir for hydrocarbon accumulation and fluid flow properties. Consequently, the filling characteristics directly determine the effectiveness of natural fractures. Based on the proportion of space occupied by filling minerals within fractures, tectonic shear fractures can be categorized as fully filled, partially filled, and unfilled fractures, with corresponding degrees of filling decreasing and effectiveness increasing. Statistical analyses reveal that partially filled and unfilled fractures in the cores account for 83.5%, whereas filled fractures constitute 16.5% (Figure 9). In thin sections, partially filled and unfilled fractures represent 69.7%, while filled fractures comprise 30.3% (Figure 9). The types of filling minerals include calcite, quartz, and carbonaceous matter. Fractures completely filled with minerals make minimal contributions to gas migration and are deemed ineffective, whereas partially filled and unfilled fractures serve as effective conduits for gas migration.
Figure 9. Filling degree of tectonic shear fractures in the T3x2 tight sandstone reservoirs in the western Sichuan Basin.
The density of fractures reflects their degree of development. According to statistical results from observations of 18 cored wells and interpretations of 11 imaging logging data, the macroscopic tectonic shear fractures in the study area exhibit a density distribution ranging from 0.2 to 2.0 m−1, with an average linear density of 0.72 m−1 (Figure 10). Results from 150 thin sections indicate that the fracture surface density ranges from 0.01 to 1.26 cm/cm2, with an average surface density of 0. 55 cm/cm2 (Figure 10).
(1) The relationship between lithology and the development of fractures
Figure 10. Boxplot of macro and micro tectonic shear fracture density in the T3x2 tight sandstone reservoirs in the western Sichuan Basin.
The petrological analysis reveals that the sedimentary rock types in the T3x2 tight sandstone reservoirs include coarse sandstone, medium sandstone, fine sandstone, siltstone, argillaceous siltstone, silty mudstone, and mudstone. Observations from core and thin section data indicate that the development of sandstone fractures in the tight reservoir is greater than that of mudstone, with the highest degree observed in siltstone and fine sandstone (Figure 11). Conversely, the development of fractures is relatively lower in medium sandstone and coarse sandstone, reflecting an increase in fracture development with decreasing grain size of the same lithology (Figure 11).
(2) The relationship between rock mechanics stratigraphy and the development of fractures
Figure 11. Boxplot of tectonic shear fracture density in different lithologies in the T3x2 tight sandstone reservoirs in the western Sichuan Basin.
Outcrop surveys, core observations, and logging interpretations show that within a certain range of rock layer thickness, there is a good power-law relationship between fracture density and the average thickness of fractured rock layers (Figure 12). As the thickness of mechanical stratigraphy increases, the density of fractures tends to decrease following a power-law trend. Fractures generally do not develop when the thickness of a single layer exceeds 2.5 m, and their correlation becomes less significant.
(3) The relationship between diagenetic processes, facies, and the development of fractures
Figure 12. Relationship between the line density of tectonic shear fractures and the thickness of mechanical stratigraphy in the T3x2 tight sandstone reservoirs in the western Sichuan Basin.
Based on diagenetic process types and rock brittleness, the diagenetic facies in the study area can be divided into strong cementation compaction-dominant, medium cementation dissolution-dominant, and weakly cementation dissolution-dominant types (Zeng et al., 2016) (Figure 13). Analysis of core and thin section data indicates that tectonic fractures are most abundant in the strong cementation compaction-dominant diagenetic facies, followed by the medium cementation dissolution-dominant diagenetic facies, with the lowest abundance in the weakly cementation dissolution-dominant diagenetic facies (Figure 13).
(4) The relationship between faults and the development of fractures
Figure 13. Boxplot of tectonic shear fracture surface density in different lithofacies in the T3x2 tight sandstone reservoirs in the western Sichuan Basin.
The linear density of fractures in the hanging wall and footwall of the fault shows a significant difference (Figure 14A). The linear density of fractures in the hanging wall ranges from 0.31 to 1.75 m⁻1, with an average value of approximately 0.88 m⁻1. In contrast, the linear density of fractures in the footwall ranges from 0.09 to 1.24 m⁻1, with an average value of approximately 0.58 m⁻1 (Figure 14B). This phenomenon indicates that the linear density of fractures in the hanging wall is greater than that in the footwall. The relationship between fault distance and tectonic shear fracture abundance shows that as the distance from the fault increases, the linear density of fractures decreases significantly, exhibiting an exponential decay trend with increasing distance from the fault plane (Figure 14B).
Figure 14. Relationship between the linear density of tectonic shear fractures and distance from fault planes in the Xujiahe Formation, B3 outcrop field section, the western Sichuan Basin. See Figure 1B for the outcrop location.
Previous studies have shown significant variations in the degree of fracture development across different regions (Fossen et al., 2007; Laubach et al., 2019; Oliveira et al., 2022). Even within the same region, notable differences in fracture development exist between different locations within the same stratigraphic unit and within the same location at different stratigraphic levels (Zeng et al., 2023a). The heterogeneity in fracture development exceeds that of the reservoir and even surpasses the variability of sedimentary microfacies, severely constraining the exploration and development of fractured gas reservoirs (Zeng, 2008). Based on the analysis of the development characteristics of tectonic fractures in the Xujiahe Formation in the study area, this paper explores the control exerted by lithology, rock mechanics stratigraphy, diagenetic processes and facies, and faults on the development and distribution of natural fractures.
Due to the varying deformation and fracturing abilities exhibited by different rock types under similar stress conditions (Shi et al., 2024). The reduction in rock grain size leads to increased density and fracture strength, causing relatively higher fracture development in dense reservoirs with lower grain size under smaller strain conditions (Fossen et al., 2007; Gong et al., 2019b; Oliveira et al., 2022). Additionally, an increase in mud content results in a decrease in fracture development in siltstone, muddy siltstone, silty mudstone, and mudstone (Figure 11). This is attributed to the correlation between fracture development and rock brittleness (Shi et al., 2020). The increase in mud content reduces rock brittleness, resulting in lower fracture development under the same stress conditions compared to rocks with lower mud content (e.g., hard sandstone with high elastic modulus and brittleness). Overall, fracture development is closely related to rock composition and sorting, with better-sorted and purer sandstones exhibiting higher fracture development compared to poorly-sorted mudstones.
The development characteristics of tectonic shear fractures indicate that these fractures typically intersect mechanical interfaces of rock layers perpendicularly or at high angles, develop within stratigraphic units, and terminate at mechanical interfaces, rarely penetrating them (Figures 3A,B). The height of the fractures generally equals the thickness of the fractured rock layer. The stratigraphic units and mechanical interfaces together constitute a mechanical layer (Laubach et al., 2009; Lyu et al., 2022). Therefore, the rock mechanics stratigraphy is an important factor influencing the development of tectonic shear fractures. Tectonic shear fractures typically develop within stratigraphic units, while mechanical interfaces limit the extension of fractures. With the increase in the thickness of mechanical stratigraphy, the abundance of fractures decreases, but their scale increases (Figure 12).
The tight sandstone reservoirs of the Xujiahe Formation in the western Sichuan Basin have undergone various types of diagenetic processes, resulting in the complex evolution of reservoir quality, primarily including compaction, dissolution, and cementation processes (Liu et al., 2019). Compaction and cementation are key factors determining the densification of the target layers in the study area, leading to the destruction of primary reservoir porosity, with compaction being the dominant influence (Liu et al., 2018). Diagenetic facies represent the material manifestations in specific sedimentary and diagenetic physicochemical environments, reflecting the lithological characteristics, reservoir properties, and petrophysical features of diagenetic environments (Zhou et al., 2019; Wu et al., 2020). With enhanced compaction, porosity and permeability of the reservoir decrease, and rock brittleness significantly increases (Zhang et al., 2020). Cementation processes replace air or water in the pores with cementitious particles, forming a rock framework, reducing porosity and permeability, increasing the bulk density of the main rock, and imparting higher brittleness (Lavenu et al., 2014; Palhano et al., 2023). Therefore, rocks with strong compaction and cementation exhibit higher brittleness and are more prone to fracturing under similar stress conditions. In summary, diagenetic processes influence the abundance of tectonic fractures by affecting the mechanical properties of rocks, with stronger compaction and cementation resulting in poorer physical properties and higher rock brittleness, leading to a greater abundance of tectonic fractures (Figure 13).
Faults are important structural types that affect the development and distribution of tectonic fractures. Due to the influences of the Indo-Indochina Movement, Yanshan Movement, and Xishan Movement, a series of NE-SW-trending and nearly N-S-trending faults have developed in the western Sichuan Basin. In the stress perturbation zones near the faults, tectonic shear fractures typically intersect with the faults at small angles or are subparallel to them, indicating a correlation between the development of these fractures and the faults (Mao et al., 2022). Additionally, statistical analysis reveals that although there is a clear decreasing trend in fracture linear density with increasing distance from the fault on both the hanging wall and footwall, there are also differences in fracture development on either side of the fault (Figure 14). Specifically, the degree of fracture development on the hanging wall is significantly greater than that on the footwall, as the hanging wall is often the active side where stress perturbations are more pronounced.
(1) Natural fractures are widely developed in the second member of the Upper Triassic Xujiahe Formation tight sandstone reservoirs in the western Sichuan Basin, with tectonic shear fractures being the main type. There are primarily four sets of tectonic shear fractures in the study area, oriented in the E–W, N–S, NE-SW, and NW-SE directions. The dip angle of fractures ranges from 20° to 80°, with an average dip angle of 53°, mainly consisting of high-angle fractures. Fracture lengths are mainly distributed within 100 cm, with a mean length of 47.53 cm, and 76.2% of fractures have lengths less than 50 cm. The aperture of fractures ranges from 2.51 to 163.19 μm, with an average of 30.54 μm. Among them, the proportion of effective fractures reaches 85.7%.
(2) Lithology is the fundamental factor affecting the development of fractures in tight reservoirs. In rocks with the same composition, the finer the grain size, the higher the degree of fracture development. Moreover, with increasing muddy content, the degree of fracture development decreases in the siltstone, argillaceous siltstone, silty mudstone, and mudstone.
(3) Fracture formation and distribution are influenced by rock mechanics stratigraphy, mainly manifested as a decrease in fracture density with the increasing thickness of rock mechanics stratigraphy within a certain range of thickness.
(4) Diagenesis affects the abundance of tectonic fractures by influencing the mechanical properties of rocks. The stronger the compaction and cementation, the poorer the physical properties and the higher the brittleness of the rocks, leading to a greater abundance of tectonic fractures. This is evidenced by the fact that rocks with strong compaction and cementation have higher brittleness and are more prone to fracturing under the same stress conditions.
(5) Due to the uneven distribution of stress caused by fault activity, fractures are highly developed near both the footwall and hanging wall of faults. There is a typical distribution pattern where the linear density of fractures decreases significantly with increasing distance from the fault plane.
The original contributions presented in the study are included in the article/Supplementary Material, further inquiries can be directed to the corresponding author.
YZ: Writing–original draft, Writing–review and editing, Formal Analysis, Investigation. RZ: Funding acquisition, Writing–review and editing. LQ: Conceptualization, Writing–review and editing. HW: Conceptualization, Software, Writing–review and editing. QD: Conceptualization, Investigation, Writing–review and editing. ZZ: Data curation, Writing–review and editing. TS: Software, Writing–review and editing. RH: Data curation, Writing–review and editing.
The author(s) declare that financial support was received for the research, authorship, and/or publication of this article. This study was financially supported by the China Postdoctoral Science Foundation (No. 2023MD744255), the Natural Science Basic Research Program of Shaanxi (No. 2024JC-YBQN-0353), the Scientific Research Program Funded by Shaanxi Provincial Education Department (No. 23JK0600), the Shaanxi Postdoctoral Science Foundation (No. 2023BSHEDZZ324), the Key Research and Development Program of Shaanxi (No. 2021KW-10), the Innovation Capability Support Program of Shaanxi (No. 2022PT-08), and the State Key Laboratory of Shale Oil and Gas Enrichment Mechanisms and Effective Development (No. 33550000-22-ZC0613-0326).
We are particularly grateful to the anonymous reviewers for their constructive comments and suggestions, which significantly improved this manuscript.
The authors declare that the research was conducted in the absence of any commercial or financial relationships that could be construed as a potential conflict of interest.
All claims expressed in this article are solely those of the authors and do not necessarily represent those of their affiliated organizations, or those of the publisher, the editors and the reviewers. Any product that may be evaluated in this article, or claim that may be made by its manufacturer, is not guaranteed or endorsed by the publisher.
Aguilera, R. (1988). Determination of subsurface distance between vertical parallel natural fractures based on core data. Am. Assoc. Pet. Geol. Bull. 72, 845–851.
Ameen, M. S., MacPherson, K., Al-Marhoon, M. I., and Rahim, Z. (2012). Diverse fracture properties and their impact on performance in conventional and tight-gas reservoirs, Saudi Arabia: the Unayzah, South Haradh case study. Am. Assoc. Pet. Geol. Bull. 96, 459–492. doi:10.1306/06011110148
Anders, M. H., Laubach, S. E., and Scholz, C. H. (2014). Microfractures: a review. J. Struct. Geol. 69, 377–394. doi:10.1016/j.jsg.2014.05.011
Chang, X., Zhao, H., and Cheng, L. (2020). Fracture propagation and coalescence at bedding plane in layered rocks. J. Struct. Geol. 141, 104213. doi:10.1016/j.jsg.2020.104213
Deng, J., Liu, M., Ji, Y., Tang, D., Zeng, Q., Song, L., et al. (2022). Controlling factors of tight sandstone gas accumulation and enrichment in the slope zone of foreland basins: the Upper Triassic Xujiahe Formation in Western Sichuan Foreland Basin, China. J. Pet. Sci. Eng. 214, 110474. doi:10.1016/j.petrol.2022.110474
Ding, W., Wang, X., Hu, Q., Yin, S., Cao, X., and Liu, J. (2015). Progress in tight sandstone reservoir fractures research. Adv. Earth Sci. 30, 737–750. (In Chinese with English abstract).
Faulkner, D. R., Jackson, C. A. L., Lunn, R. J., Schlische, R. W., Shipton, Z. K., Wibberley, C. A. J., et al. (2010). A review of recent developments concerning the structure, mechanics and fluid flow properties of fault zones. J. Struct. Geol. 32, 1557–1575. doi:10.1016/j.jsg.2010.06.009
Ferrill, D. A., Smart, K. J., Morris, A. P., and Cawood, A. J. (2021). The fold-thrust belt stress cycle: superposition of normal, strike-slip, and thrust faulting deformation regimes. J. Struct. Geol. 148, 357791. doi:10.1130/abs/2020am-357791
Fossen, H. (2010). Deformation bands formed during soft-sediment deformation: observations from SE Utah. Mar. Pet. Geol. 27, 215–222. doi:10.1016/j.marpetgeo.2009.06.005
Fossen, H., and Bale, A. (2007). Deformation bands and their influence on fluid flow. Am. Assoc. Pet. Geol. Bull. 91, 1685–1700. doi:10.1306/07300706146
Fossen, H., Schultz, R. A., Shipton, Z. K., and Mair, K. (2007). Deformation bands in sandstone: a review. J. Geol. Soc. Lond. 164, 755–769. doi:10.1144/0016-76492006-036
Friesen, O. J., Dashtgard, S. E., Miller, J., Schmitt, L., and Baldwin, C. (2017). Permeability heterogeneity in bioturbated sediments and implications for waterflooding of tight-oil reservoirs, Cardium Formation, Pembina Field, Alberta, Canada. Mar. Pet. Geol. 82, 371–387. doi:10.1016/j.marpetgeo.2017.01.019
Fu, Y., and Dehghanpour, H. (2020). How far can hydraulic fractures go? A comparative analysis of water flowback, tracer, and microseismic data from the Horn River Basin. Mar. Pet. Geol. 115, 104259. doi:10.1016/j.marpetgeo.2020.104259
Gale, J. F. W., Laubach, S. E., Olson, J. E., Eichhubl, P., and Fall, A. (2014). Natural fractures in shale: a review and new observations. Am. Assoc. Pet. Geol. Bull. 101, 2165–2216. doi:10.1306/08121413151
Gong, L., Fu, X., Wang, Z., Gao, S., Jabbari, H., Yue, W., et al. (2019a). A new approach for characterization and prediction of natural fracture occurrence in tight oil sandstones with intense anisotropy. Am. Assoc. Pet. Geol. Bull. 103, 1383–1400. doi:10.1306/12131818054
Gong, L., Su, X., Gao, S., Fu, X., Jabbari, H., Wang, X., et al. (2019b). Characteristics and formation mechanism of natural fractures in the tight gas sandstones of Jiulongshan gas field, China. J. Pet. Sci. Eng. 175, 1112–1121. doi:10.1016/j.petrol.2019.01.021
Gong, L., Zeng, L., Gao, Z., Zhu, R., and Zhang, B. (2016). Reservoir characterization and origin of tight gas sandstones in the upper triassic Xujiahe Formation, western Sichuan Basin, China. J. Pet. Explor. Prod. Technol. 6, 319–329. doi:10.1007/s13202-015-0203-9
Guo, Y., Pang, X., Chen, D., Leng, J., and Tian, J. (2012). Evolution of continental formation pressure in the middle part of the Western Sichuan Depression and its significance on hydrocarbon accumulation. Pet. Explor. Dev. 39, 457–465. doi:10.1016/S1876-3804(12)60062-7
Hart, B. S. (2006). Seismic expression of fracture-swarm sweet spots, Upper Cretaceous tight-gas reservoirs, San Juan Basin. Am. Assoc. Pet. Geol. Bull. 90, 1519–1534. doi:10.1306/05020605171
He, D., Ma, Y., Li, Y., and Wu, S. (2019). New directions in an established gas play: promising dolomite reservoirs in the middle triassic leikoupo formation of the Sichuan Basin, China. Am. Assoc. Pet. Geol. Bull. 103, 1–29. doi:10.1306/05111816502
Jiang, L., Zhao, W., Bo, D. M., Hong, F., Gong, Y. J., and Hao, J. Q. (2023). Tight sandstone gas accumulation mechanisms and sweet spot prediction, Triassic Xujiahe Formation, Sichuan Basin, China. Pet. Sci. 20, 3301–3310. doi:10.1016/j.petsci.2023.07.008
Khlaifat, A., Qutob, H., and Barakat, N. (2011). Tight gas sands development is critical to future world energy resources. Soc. Pet. Eng. - SPE Middle East Unconv. Gas. Conf. Exhib. 2011, UGAS, 143–154. doi:10.2118/142049-ms
Khoshbakht, F., Azizzadeh, M., Memarian, H., Nourozi, G. H., and Moallemi, S. A. (2012). Comparison of electrical image log with core in a fractured carbonate reservoir. J. Pet. Sci. Eng. 86 (87), 289–296. doi:10.1016/j.petrol.2012.03.007
Lai, J., Wang, G., Wang, S., Cao, J., Li, M., Pang, X., et al. (2018). Review of diagenetic facies in tight sandstones: diagenesis, diagenetic minerals, and prediction via well logs. Earth-Science Rev. 185, 234–258. doi:10.1016/j.earscirev.2018.06.009
Laubach, S. E., and Diaz-Tushman, K. (2009). Laurentian palaeostress trajectories and ephemeral fracture permeability, Cambrian Eriboll formation sandstones west of the moine thrust zone, NW Scotland. J. Geol. Soc. Lond. 166, 349–362. doi:10.1144/0016-76492008-061
Laubach, S. E., Lander, R. H., Criscenti, L. J., Anovitz, L. M., Urai, J. L., Pollyea, R. M., et al. (2019). The role of chemistry in fracture pattern development and opportunities to advance interpretations of geological materials. Rev. Geophys. 57, 1065–1111. doi:10.1029/2019rg000671
Laubach, S. E., Olson, J. E., and Cross, M. R. (2009). Mechanical and fracture stratigraphy. Am. Assoc. Pet. Geol. Bull. 93, 1413–1426. doi:10.1306/07270909094
Lavenu, A. P. C., Lamarche, J., Salardon, R., Gallois, A., Marié, L., and Gauthier, B. D. M. (2014). Relating background fractures to diagenesis and rock physical properties in a platform-slope transect. Example of the Maiella Mountain (central Italy). Mar. Pet. Geol. 51, 2–19. doi:10.1016/j.marpetgeo.2013.11.012
Law, B. E. (2002). Basin-centered gas systems. Am. Assoc. Pet. Geol. Bull. 86. doi:10.1306/61eeddb4-173e-11d7-8645000102c1865d
Lee, H. P., Olson, J. E., and Schultz, R. A. (2018). Interaction analysis of propagating opening mode fractures with veins using the Discrete Element Method. Int. J. Rock Mech. Min. Sci. 103, 275–288. doi:10.1016/j.ijrmms.2018.01.005
Li, Z., Liu, S., Chen, H., Sun, D., Lin, J., and Tang, C. (2011). Structural superimposition and conjunction and its effects on hydrocarbon accumulation in the Western Sichuan Depression. Pet. Explor. Dev. 38, 538–551. doi:10.1016/S1876-3804(11)60054-2
Lin, L., Yu, Y., Nan, H., and Chen, H. (2022). Petrologic and geochemical characteristics of carbonate cements in the Upper Triassic Xujiahe Formation tight gas sandstone, western Sichuan Basin, China. Am. Assoc. Pet. Geol. Bull. 106, 461–490. doi:10.1306/10042119129
Liu, Y., Hu, W., Cao, J., Wang, X., Tang, Q., Wu, H., et al. (2018). Diagenetic constraints on the heterogeneity of tight sandstone reservoirs: a case study on the Upper Triassic Xujiahe Formation in the Sichuan Basin, southwest China. Mar. Pet. Geol. 92, 650–669. doi:10.1016/j.marpetgeo.2017.11.027
Liu, Y., Hu, W., Cao, J., Wang, X., Zhu, F., Tang, Q., et al. (2019). Fluid–rock interaction and its effects on the Upper Triassic tight sandstones in the Sichuan Basin, China: insights from petrographic and geochemical study of carbonate cements. Sediment. Geol. 383, 121–135. doi:10.1016/j.sedgeo.2019.01.012
Lyu, W., Zeng, L., Lyu, P., Yi, T., Dong, S., Wang, S., et al. (2022). Insights into the mechanical stratigraphy and vertical fracture patterns in tight oil sandstones: the Upper Triassic Yanchang Formation in the eastern Ordos Basin, China. J. Pet. Sci. Eng. 212, 110247. doi:10.1016/j.petrol.2022.110247
Lyu, W., Zeng, L., Zhang, B., Miao, F., Lyu, P., and Dong, S. (2017). Influence of natural fractures on gas accumulation in the Upper Triassic tight gas sandstones in the northwestern Sichuan Basin, China. Mar. Pet. Geol. 83, 60–72. doi:10.1016/j.marpetgeo.2017.03.004
Lyu, W., Zeng, L., Zhou, S., Du, X., Xia, D., Liu, G., et al. (2019). Natural fractures in tight-oil sandstones: a case study of the upper triassic yanchang formation in the southwestern ordos basin, China. Am. Assoc. Pet. Geol. Bull. 103, 2343–2367. doi:10.1306/0130191608617115
Mao, Z., Zeng, L., Liu, G., Liu, G., Tian, H., Dong, S., et al. (2022). Controls of fault-bend fold on natural fractures: insight from discrete element simulation and outcrops in the southern margin of the Junggar Basin, Western China. Mar. Pet. Geol. 138, 105541. doi:10.1016/j.marpetgeo.2022.105541
Oliveira, L. S. B., Nogueira, F. C. C., Vasconcelos, D. L., Balsamo, F., Bezerra, F. H. R., and Pérez, Y. A. R. (2022). Mechanical stratigraphy influences deformation band pattern in arkosic sandstones, Rio do Peixe Basin, Brazil. J. Struct. Geol. 155, 104510. doi:10.1016/j.jsg.2022.104510
Olson, J. E., Laubach, S. E., and Lander, R. H. (2009). Natural fracture characterization in tight gas sandstones: integrating mechanics and diagenesis. Am. Assoc. Pet. Geol. Bull. 93, 1535–1549. doi:10.1306/08110909100
Palhano, L. C., Nogueira, F. C. C., Marques, F. O., Vasconcelos, D. L., Bezerra, F. H., Souza, J. A. B., et al. (2023). Influence of hydrothermal silicification on the physical properties of a basin-boundary fault affecting arkosic porous sandstones, Rio do Peixe Basin, Brazil. Mar. Pet. Geol. 148, 106062. doi:10.1016/j.marpetgeo.2022.106062
Shi, J., Zeng, L., Zhao, X., Zhang, Y., and Wang, J. (2020). Characteristics of natural fractures in the upper Paleozoic coal bearing strata in the southern Qinshui Basin, China: implications for coalbed methane (CBM) development. Mar. Pet. Geol. 113, 104152. doi:10.1016/j.marpetgeo.2019.104152
Shi, J., Zhao, X., Zhang, Y., You, Y., Shang, M., and Zhu, Z. (2024). Characteristics and controlling factors of natural fractures in deep carbonates of the Puguang area, Sichuan Basin, China. ACS Omega 9, 16071–16083. doi:10.1021/acsomega.3c09699
Su, N., Chen, Z., Yang, W., Wang, L., Li, W., Yang, C., et al. (2023). Features and favorable exploration direction of normal faults in Jurassic strata in northern central Sichuan Basin, China. J. Nat. Gas. Geosci. 8, 413–426. doi:10.1016/j.jnggs.2023.11.003
Torabi, A., and Berg, S. S. (2011). Scaling of fault attributes: a review. Mar. Pet. Geol. 28, 1444–1460. doi:10.1016/j.marpetgeo.2011.04.003
Wang, G., Song, X., Liu, Y., Meng, X., and Long, K. (2022). Accumulation characteristics and exploration prospects of the 4th member of Leikoupo Formation in the western Sichuan Basin, China. J. Nat. Gas. Geosci. 7, 199–210. (In Chinese with English abstract). doi:10.1016/j.jnggs.2022.08.004
Wang, M., Feng, W., Jiang, D., Yan, B., Chen, Z., and Song, G. (2020). Interactions between thin- and thick-skinned tectonics at the western Sichuan Basin, China. Tectonophysics 796, 228628. doi:10.1016/j.tecto.2020.228628
Wang, W., Yue, D., Eriksson, K. A., Li, S., Zhang, Y., Zhang, J., et al. (2023a). Spatial variations in diagenetic facies in tight sandstones from sublacustrine-fan deposits: upper Triassic Yanchang formation, Ordos Basin, China. Mar. Pet. Geol. 156, 106446. doi:10.1016/j.marpetgeo.2023.106446
Wang, Z., Lu, S., Zhou, N., Liu, Y., Lin, L., Shang, Y., et al. (2023b). Diagenesis as a control on the tight sandstone reservoir quality of the upper Carboniferous strata in the northeastern Ordos basin, China. Mar. Pet. Geol. 158, 106565. doi:10.1016/j.marpetgeo.2023.106565
Wu, D., Liu, S., Chen, H., Lin, L., Yu, Y., Xu, C., et al. (2020). Investigation and prediction of diagenetic facies using well logs in tight gas reservoirs: evidences from the Xu-2 member in the xinchang structural belt of the western Sichuan Basin, western China. J. Pet. Sci. Eng. 192, 107326. doi:10.1016/j.petrol.2020.107326
Xiong, L., Long, K., Cao, Q., and Zhang, S. (2024). Multilayer accumulation conditions and key technologies for exploration and development of the West Sichuan gas field in Sichuan Basin. Acta Pet. Sin. 45, 595–614. (In Chinese with English abstract). doi:10.7623/syxb202403009
Xu, C., Gehenn, J. M., Zhao, D., Xie, G., and Teng, M. K. (2015). The fluvial and lacustrine sedimentary systems and stratigraphic correlation in the Upper Triassic Xujiahe Formation in Sichuan Basin, China. Am. Assoc. Pet. Geol. Bull. 99, 2023–2041. doi:10.1306/07061514236
Yang, K., Zhu, H., Ye, J., Zhang, K., and Ke, G. (2012). The geological characteristics of tight sandstone gas reservoirs in west Sichuan Basin. Science Press. (In Chinese).
Yang, P., Zhang, L., Liu, K., Cao, B., Gao, J., and Qiu, G. (2021). Diagenetic history and reservoir evolution of tight sandstones in the second member of the Upper Triassic Xujiahe Formation, western Sichuan Basin, China. J. Pet. Sci. Eng. 201, 108451. doi:10.1016/j.petrol.2021.108451
Yu, Y., Lin, L. B., and Gao, J. (2016). Formation mechanisms and sequence response of authigenic grain-coating chlorite: evidence from the Upper Triassic Xujiahe Formation in the southern Sichuan Basin, China. Pet. Sci. 13, 657–668. doi:10.1007/s12182-016-0125-2
Zanella, A., Cobbold, P. R., Ruffet, G., and Leanza, H. A. (2015). Geological evidence for fluid overpressure, hydraulic fracturing and strong heating during maturation and migration of hydrocarbons in Mesozoic rocks of the northern Neuquén Basin, Mendoza Province, Argentina. J. South Am. Earth Sci. 62, 229–242. doi:10.1016/j.jsames.2015.06.006
Zeng, L. (2008). Formation and distribution of fractures in low-permeability sandstone reservoirs. Beijing: Science Press House. (In Chinese).
Zeng, L. (2010). Microfracturing in the upper triassic Sichuan Basin tight-gas sandstones: tectonic, overpressure, and diagenetic origins. Am. Assoc. Pet. Geol. Bull. 94, 1811–1825. doi:10.1306/06301009191
Zeng, L., Gong, L., Zhang, Y., Dong, S., and Lyu, W. (2023a). A review of the genesis, evolution, and prediction of natural fractures in deep tight sandstones of China. Am. Assoc. Pet. Geol. Bull. 107, 1687–1721. doi:10.1306/07052322120
Zeng, L., Jiang, J., and Yang, Y. (2010). Fractures in the low porosity and ultra-low permeability glutenite reservoirs: a case study of the late Eocene Hetaoyuan formation in the Anpeng Oilfield, Nanxiang Basin, China. Mar. Pet. Geol. 27, 1642–1650. doi:10.1016/j.marpetgeo.2010.03.009
Zeng, L., and Li, X. (2009). Fractures in sandstone reservoirs with ultra-low permeability: a case study of the upper Triassic Yanchang Formation in the Ordos Basin, China. Am. Assoc. Pet. Geol. Bull. 93, 461–477. doi:10.1306/09240808047
Zeng, L., Lü, W., Xu, X., Tian, H., Lu, S., and Zhang, M. (2022). Development characteristics, formation mechanism and hydrocarbon significance of bedding fractures in typical tight sandstone and shale. Acta Pet. Sin. 43, 180–191. (In Chinese with English abstract). doi:10.7623/syxb202202002
Zeng, L., Song, Y., Liu, G., Tan, X., Xu, X., Yao, Y., et al. (2023b). Natural fractures in ultra-deep reservoirs of China: a review. J. Struct. Geol. 175, 104954. doi:10.1016/j.jsg.2023.104954
Zeng, L., Wang, Z., Xiao, S., and Zhang, G. (2009). The origin and geological significance of low dip-angle fractures in the thrust zones of the western basins of China. Acta Pet. Sin. 30, 56–60. (In Chinese with English abstract).
Zeng, L., Zhu, R., Gao, Z., Gong, L., and Liu, G. (2016). Structural diagenesis and its petroleum geological significance. Pet. Sci. Bull. 1, 191–197. (In Chinese with English abstract).
Zhang, Y., Zeng, L., Luo, Q., Zhu, R., Lyu, W., Liu, D., et al. (2021a). Influence of natural fractures on tight oil migration and production: a case study of permian lucaogou Formation in Jimsar Sag, Junggar basin, NW China. J. Earth Sci. 32, 927–945. doi:10.1007/s12583-021-1442-y
Zhang, Y., Zeng, L., Luo, Q., Zhu, R., Pan, S., Dai, Q., et al. (2020). Effects of diagenesis on natural fractures in tight oil reservoirs: a case study of the Permian Lucaogou Formation in Jimusar Sag, Junggar Basin, NW China. Geol. J. 55, 6562–6579. doi:10.1002/gj.3822
Zhang, Y., Zeng, L., Lyu, W., Sun, D., Chen, S., Guan, C., et al. (2021b). Natural fractures in tight gas sandstones: a case study of the upper Triassic Xujiahe Formation in xinchang gas field, western Sichuan Basin, China. Geol. Mag. 158, 1543–1560. doi:10.1017/S001675682100008X
Zhou, X., Zhang, C., Zhang, Z., Zhang, R., Zhu, L., and Zhang, C. (2019). A saturation evaluation method in tight gas sandstones based on diagenetic facies. Mar. Pet. Geol. 107, 310–325. doi:10.1016/j.marpetgeo.2019.05.022
Zhu, R., Zhao, X., Liu, L., Wang, X., Zhang, N., Guo, H., et al. (2009). Depositional system and favorable reservoir distribution of Xujiahe Formation in Sichuan Basin. Pet. Explor. Dev. 36, 46–55. (In Chinese with English abstract). doi:10.1016/S1876-3804(09)60110-5
Zou, C., Tao, S., Zhu, R., Yuan, X., Li, W., Zhang, G., et al. (2009). Formation and distribution of “continuous” gas reservoirs nd their giant gas province: a case from the Upper riassic Xujiahe Formation giant gas province, Sichuan Basin. Pet. Explor. Dev. 36, 307–319. (In Chinese with English abstract). doi:10.1016/S1876-3804(09)60128-2
Zou, C., Yang, Z., Zhang, G., Zhu, R., Tao, S., Yuan, X., et al. (2023). Theory, technology and practice of unconventional petroleum geology. J. Earth Sci. 34, 951–965. doi:10.1007/s12583-023-2000-8
Zou, C., Zhu, R., Bai, B., Yang, Z., Wu, S., Su, L., et al. (2011). First discovery of nano-pore throat in oil and gas reservoir in China and its scientific value. Acta Petrol. Sin. 27, 1857–1864. (In Chinese with English abstract).
Keywords: tight sandstone reservoirs, natural fractures, development characteristics, reservoir controlling, western Sichuan basin
Citation: Zhang Y, Zhang R, Qu L, Wu H, Dai Q, Zhang Z, Shen T and He R (2024) Development characteristics of natural fractures in tight sandstone reservoirs and their controlling factors: upper Triassic Xujiahe Formation, western Sichuan Basin. Front. Earth Sci. 12:1430091. doi: 10.3389/feart.2024.1430091
Received: 09 May 2024; Accepted: 16 July 2024;
Published: 02 August 2024.
Edited by:
Wenlong Ding, China University of Geosciences, ChinaReviewed by:
Xiangwen Kong, Research Institute of Petroleum Exploration and Development (RIPED), ChinaCopyright © 2024 Zhang, Zhang, Qu, Wu, Dai, Zhang, Shen and He. This is an open-access article distributed under the terms of the Creative Commons Attribution License (CC BY). The use, distribution or reproduction in other forums is permitted, provided the original author(s) and the copyright owner(s) are credited and that the original publication in this journal is cited, in accordance with accepted academic practice. No use, distribution or reproduction is permitted which does not comply with these terms.
*Correspondence: Rongjun Zhang, cmp6aGFuZ0B4c3l1LmVkdS5jbg==
Disclaimer: All claims expressed in this article are solely those of the authors and do not necessarily represent those of their affiliated organizations, or those of the publisher, the editors and the reviewers. Any product that may be evaluated in this article or claim that may be made by its manufacturer is not guaranteed or endorsed by the publisher.
Research integrity at Frontiers
Learn more about the work of our research integrity team to safeguard the quality of each article we publish.