- 1Qinghai Traffic Construction Management Co., Ltd., Xining, China
- 2Guizhou Geological Engineering Investigation Design and Research Institute Co., Ltd., Guizhou, China
In high-cold regions, the instability of precarious rock masses in open-pit mines is often exacerbated by the effects of freeze-thaw cycles, posing a significant threat to the continuous production of open-pit mining operations. To address this issue effectively, we conducted an in-depth study on the precarious rock masses in the near-slope of a mining area in a high-cold region using a fracture mechanics-based stability analysis method. We analyzed the impact of freeze-thaw cycles on the engineering stability. Introducing a novel approach, we established the temperature field at different time points to determine whether the freeze-thaw depth influences the generation of freeze-thaw forces on the controlling structural surfaces. Employing the maximum circumferential stress criterion, we conducted a comprehensive analysis of tension cracks in the slope and derived corresponding safety factor expressions. Focusing on retrogressive rock slopes, we divided the slope's precarious rock masses into n potentially unstable approximate rectangular rock bodies. Based on this, we developed a fracture mechanics-based slope stability calculation method considering the combined effects of freeze-thaw forces, crack water pressure, and gravity. Through relevant numerical examples, we successfully calculated the safety factors of the segmented rock bodies, revealing the varying influence of freeze-thaw forces on rock mass stability. By integrating the calculation results with practical engineering considerations, we validated the feasibility of our proposed method. Lastly, aligning with pertinent precarious rock stability assessment criteria, we provided corresponding remediation measures based on the distinct stability conditions of the rock masses. Through comprehensive research and an effective computational approach, we offer a scientifically viable solution for the stability of precarious rock masses in open-pit mines in high-cold regions, thereby providing robust technical support for the sustainable production of mining enterprises.
1 Introduction
Engineering projects in cold regions are subjected to the distinctive conditions of freeze-thaw cycles, which exert a prolonged influence on the geological aspects of these projects. In such environments, rock masses frequently experience the effects of rainfall or the accumulation of groundwater, initiating internal water-ice phase transition phenomena (Foresti et al., 2018; Salimbeni et al., 2018). This phenomenon has a direct impact on the internal structure of rock masses, primarily manifested through the expansion of cracks. The consequence of this crack propagation is a reduction in the locked segment length on potential slip surfaces, ultimately leading to slope instability.
Freeze-thaw cycles represent a significant characteristic in cold region engineering, wherein groundwater undergoes water-ice phase transition within the internal structure of rock masses. This phase transition involves the process of water transitioning from a liquid state to a solid ice state at low temperatures, accompanied by volumetric expansion. Particularly in cases of rainfall or groundwater enrichment, the crack propagation induced by water-ice phase transition within rock masses becomes more pronounced (Cabrera et al., 2015; Oxilia et al., 2020). The development of cracks may result from the infiltration of water, and during the cold season, this water freezes to form new ice bodies, leading to the accumulation of internal stresses. The extension of cracks directly influences the nature of potential slip surfaces. Slip surfaces are crucial parameters in slope stability analysis, and the length of locked segments on these surfaces directly relates to the overall stability of the slope. Due to crack propagation induced by water-ice phase transition, the reduction in locked segment length weakens the slope's stability. This impact is particularly pronounced in harsh geological conditions in cold environments. Slope instability is one potential outcome of crack propagation induced by water-ice phase transition (Liao et al., 2016). As locked segment length decreases, the strength of potential slip surfaces diminishes, increasing the risk of slope instability. This poses a serious challenge for the design and construction of engineering projects in cold regions, as slope failure events such as landslides and collapses may occur in unstable conditions, posing a threat to project safety. The crack propagation induced by water-ice phase transition in rock masses in cold region engineering is a complex and serious issue. This problem is directly related to slope stability and holds significant implications for enhancing the safety and stability of engineering projects in cold regions.
In recent years, substantial progress has been made in the study of rock slope stability. Some scholars have noted the limited accuracy of calculating rock slope stability using traditional limit equilibrium methods (Panpan, 2018). In this context, new research methods have emerged, attempting to enhance the accuracy of rock slope stability calculations through more detailed analyses and fracture mechanics-based approaches.
A novel research method involves dividing precarious rock masses into n potentially unstable approximate rectangular rock bodies. Subsequently, a fracture mechanics-based analysis of the force patterns acting on each rock body is conducted, delving into the stability conditions of individual rocks (Lv et al., 2017; Nisi et al., 2018; Wang et al., 2020). The innovation of this method lies in its meticulous consideration of the heterogeneity within the rock mass, providing a more reasonable model for the mechanical response of the rock mass through a more accurate subdivision approach.
In further studies, some researchers treated each approximate rectangular rock body as a cantilever beam controlled by a dominant structural surface. They developed a fracture mechanics-based analysis method for precarious rock mass stability. By making reasonable assumptions about the cantilever beam model, this method thoroughly explored the crucial influence of the dominant structural surface on rock mass stability (Chiogna et al., 2014; Sun et al., 2014; Lindstrom et al., 2015). By incorporating factors controlling the structural surface into the analysis, this method comprehensively considers the complexity of the internal structure of the rock mass.
Shear-joint composite fractures are common complex structures in rock masses and have a significant impact on slope stability. Addressing this issue, some scholars utilized the maximum circumferential stress criterion to analyze the stability of different engineering slopes (Verlaguet et al., 2011; Feakins et al., 2016; Janku-Capova et al., 2018). This method provides an in-depth perspective on understanding the microscopic characteristics of the internal structure, the evolution of fractures, and stress distribution within the rock mass, laying the foundation for a comprehensive stability analysis.
However, it is worth noting that the aforementioned studies primarily focus on rock mass stability under general engineering conditions, with limited attention to the stability of precarious rock masses in cold regions. In high-cold regions, freeze-thaw cycles are a key factor influencing the mechanical properties of rock masses (Frank et al., 2021). To comprehensively analyze the stability of precarious rock masses in cold regions, it is essential to consider freeze-thaw forces, a significant factor leading to instability in cold region engineering due to freeze-thaw slope failures (Sternai et al., 2019).
Freeze-thaw cycling experiments have revealed the significant impact of freeze-thaw forces generated by moisture in rock fractures at low temperatures on rock damage (Byas et al., 2019; Borghini et al., 2020; Chen et al., 2020; Kenner, 2020). Therefore, understanding the mechanical response of rocks under freeze-thaw cycling is a necessary prerequisite for the stability analysis of precarious rock masses in cold regions. Some scholars, through extensive rock freeze-thaw cycling experiments, have delved into the effects of freeze-thaw forces on the internal structure and strength of rocks, providing an experimental foundation for stability analysis in cold region precarious rock masses.
Furthermore, specific studies have focused on the expansion patterns of precarious rocks under freeze-thaw conditions and proposed formulas for calculating freeze-thaw forces on dominant structural surfaces under freezing conditions (Frattini et al., 2016; Li et al., 2020; Zhu et al., 2023). The establishment of this theoretical framework not only deepens the understanding of the mechanisms of freeze-thaw force impact on precarious rock masses but also provides more rigorous mathematical tools for stability analysis in precarious rock masses in cold regions.
While exploring the stability of precarious rock masses in cold regions, it is essential to consider variations under different geological conditions (Nepal et al., 2014). A comprehensive consideration of factors such as the unique freeze-thaw environment, water-ice phase transitions, fracture mechanics, and freeze-thaw forces is a crucial step in laying a solid foundation for stability research in precarious rock masses in cold regions (Chen et al., 2014; Mahdavi-Gharavi et al., 2020).
In summary, recent research has explicitly pointed out the limitations of traditional limit equilibrium methods in calculating rock slope stability, prompting scholars to explore new analytical methods (Pan et al., 2017; Wang et al., 2017; Moradian et al., 2020). Particularly, the introduction of fracture mechanics methods, enabling a more detailed subdivision and mechanical analysis of precarious rock masses, holds the potential to improve the accuracy of rock slope stability calculations. However, challenges persist in the field of stability research for precarious rock masses in cold regions, including the mechanisms of freeze-thaw force under freeze-thaw cycling conditions, stability variations under different geological conditions, and slope stability analysis methods based on fracture mechanics in cold regions.
This study targets an open-pit mine in a cold region, aiming to delve into the impact of temperature variations on freeze-thaw forces acting on dominant structural surfaces. By establishing a temperature field model spanning 360 days, we seek to provide a thorough exploration of the intricate relationship between temperature changes and freeze-thaw forces. Through meticulous analysis and systematic research, we establish a temperature field model that accurately reflects temporal and spatial variations. This model serves as the foundation for determining whether the dominant structural surface is influenced by freeze-thaw forces at specific points in time. Utilizing the temperature field analysis, the precarious rock masses on the slope are subdivided into n potentially unstable rectangular rock masses. We propose a fracture mechanics stability analysis method that comprehensively considers the interactions among freeze-thaw forces, fissure water pressure, and gravity. To validate the feasibility of the method, we conduct stability analyses on slope sections significantly affected by freeze-thaw action. Through in-depth analysis, we gain a comprehensive understanding of the mechanical response and stability conditions of rock masses under the influence of freeze-thaw action. Considering the varied stability conditions of rock masses in different regions, we introduce a tilting-type precarious rock stability assessment method and put forward corresponding remediation strategies. By offering personalized assessments, we provide specific and feasible mitigation measures for engineering practices to address the diverse challenges posed by rock masses in cold regions under the influence of freeze-thaw action.
2 Methodology
This study is based on an open-pit mine located in a high-cold region, where the underlying rock mass beneath the slopes consists predominantly of moderately weathered sandstone. During the mining phase, bench heights reach 12 m with adjacent benches overlapping at the final boundary, each bench having a height of 24 m. The platform width varies between 7 m and 9 m, and the slope angle during the Quaternary geological stage is 45°. The climatic conditions in this high-cold region exhibit significant daily and annual variations, characterized by short summers and prolonged winters. Winter temperatures can drop to as low as −34.6°C, and the maximum snow depth can reach 220 mm. Consequently, slopes containing water near the mining area experience substantial freezing during winter, followed by thawing as temperatures rise, creating a freeze-thaw cycle.
In this study, the model slope under investigation is currently in the final phase of construction, situated in an area where the underlying rock mass primarily comprises moderately weathered sandstone susceptible to freeze-thaw induced instability. To address this issue, multiple monitoring points were strategically placed along the slope, as illustrated in Figure 1.
2.1 Parameters
In conducting simulations, it is essential to establish the functional relationships between rock thermal conductivity and temperature, as well as between unfrozen volumetric water content and temperature. When constructing the full thermal material model using Geo-studio software for simulation, specific parameters of the rock must be inputted. Detailed rock thermophysical parameters required for the simulation are listed in Table 1. Key rock properties necessary for the simulation include thermal conductivity, temperature functions, and unfrozen volumetric water content, as detailed in Table 2.
The variations in water content and thermal conductivity are estimated based on empirical or semi-empirical functions, considering the annual temperature fluctuations in the region. The specific heat change in the rock mass is primarily described by the volumetric heat capacity in both unfrozen and frozen states, along with the in situ volumetric water content. It is noteworthy that the rock's thermal conductivity undergoes corresponding changes with the annual temperature variations.
Where,
In Geo-studio software,
2.2 Boundary conditions
During the simulation process, the left, right, and bottom boundaries of the slope are set as adiabatic conditions, indicating no heat exchange. However, a temperature boundary condition is applied to the upper boundary of the slope. It is crucial to emphasize that the selected open-pit mine slope in this study is situated in a high-cold region in China, characterized by significant temperature variations between winter and summer. The lowest temperatures typically occur in December, reaching approximately −34.6°C, while the peak temperatures in June can reach around 17.5°C.
Starting from January 1st and considering a one-year temperature cycle, we have chosen representative time points on the 90th, 180th, 270th, and 360th days for the simulation, capturing the temperature fields at these intervals. The annual temperature distribution in the region and the temperature fields at each selected time point are illustrated in Figures 2, 3.
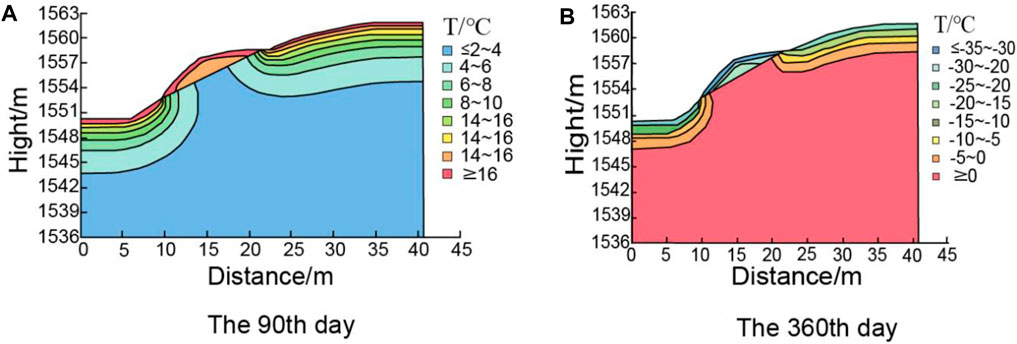
Figure 3. Cloud diagram of isotherm distribution of temperature field in an alpine open pit mine. (A) 90th day (B) 360th day.
2.3 Rock physical-mechanical model
The slope failure is attributed to the progressive expansion of fractures along the primary structural planes, resulting in overall instability. To elucidate this failure mechanism, we established a rock physics-mechanics model based on principles of fracture mechanics, considering the unique engineering geological conditions of high-cold regions. A detailed description of the model is provided in the Appendix. This model integrates freeze-thaw forces, gravity, and crack water pressure. Regarding freeze-thaw forces, we proposed a method to ascertain whether the depth of freezing and thawing affects the primary structural planes and precisely delineate which parts of the rock mass are influenced by freeze-thaw forces. In simulating the temperature field of the slope, specific boundary conditions were set to ensure the accuracy and reliability of the simulations. Specifically, thermal insulation boundary conditions were applied to the left, right, and bottom boundaries of the slope, where no thermal exchange occurs. Conversely, the top boundary of the slope was defined based on actual temperature boundary conditions.
The studied open-pit mine slope is located in a high-cold region characterized by significant temperature differences between winter and summer. Specifically, the lowest temperatures occur in December, reaching approximately −34.6°C, whereas the highest temperatures occur in June, around 17.5°C. To comprehensively simulate the temperature field changes on the slope, simulations were conducted from January 1st over a full annual temperature cycle until day 360. The temperature distribution on day 360, as depicted in Figure 4, illustrates the simulation results.
Our approach defines characteristic points by extracting intersections of the freezing temperature line with the primary structural planes. When the shortest distance from these characteristic points to the slope surface is less than the freeze-thaw depth, it indicates that the rock mass along the structural plane to the slope surface is affected by freeze-thaw forces. Conversely, if the freeze-thaw depth is less than the shortest distance from the characteristic points to the slope surface, there is no impact of freeze-thaw forces on the structural planes.
The force analysis of individual rock masses is depicted in Figure 5. Each force acting on rock mass failure corresponds to the stress intensity factor at the crack tip. Calculation of stress intensity factors using the superposition principle in Figure 5 demonstrates their significant variation under combined effects of moment, compressive stress, shear stress, and average crack water pressure.
Consultation of stress intensity factor manuals reveals the substantial influence of crack water pressure, compressive stress, shear stress, moment, and freeze expansion on stress intensity factors in the fracture mechanics model proposed in this study. These factors not only reflect the complexity and diversity of stress fields at crack tips but also provide crucial mechanical parameters for evaluating crack propagation and fracture behavior. Comprehensive consideration of these factors is essential for accurate prediction and assessment of crack propagation and fracture behavior.
3 Results and discussion
To thoroughly explore the applicability of the aforementioned theories in practical engineering applications, this study takes a high-cold region open-pit mine as a research background and selects a slope relying on a rock face for freeze-thaw induced instability analysis.
Due to the presence of unstable rocks at this site, posing potential safety risks, we chose the freeze-thaw conditions after 360 days as a representative case for the study. Based on detailed geological surveys, the freeze-thaw depth of the rock layers in this area reaches 0.12 m after 360 days of freeze-thaw cycles. With a freezing temperature of −33°C and rock density
According to the results in Table 3, the unstable rock mass is categorized as I∼V. Upon calculation, it is observed that, without the influence of freeze-thaw forces, the safety factor of rock mass I is 1.52. However, after considering the impact of freeze-thaw forces, its safety factor decreases by 18%. Rock masses II∼III are in an unstable state with stability coefficients less than 1 and should undergo immediate engineering reinforcement. As for rock masses I, IV, and V, with stability coefficients ranging between 1 and 1.5, they are in a quasi-stable state and should undergo strengthened monitoring. Additionally, local surface drainage or engineering governance is recommended. Considering the actual conditions of the open-pit mine, no overall landslide has occurred in this slope area.
Through on-site investigations, it was observed that during the final relying process of the slope in this mine, the rock masses in the relying slope were significantly affected by freeze-thaw forces, and unstable rock masses were identified, posing a serious threat to the mining operations of the open-pit mine. The underlying rock mass in this area is mainly composed of moderately weathered sandstone. Due to multiple tectonic movements in this geological region, the geological structure is complex, with intense folding of rock layers and well-developed faults and fractures. Additionally, the mine often experiences groundwater leakage issues. Coupled with the large daily and yearly temperature variations in this region, common freeze-thaw phenomena in winter result in crack expansion, rock cutting, and ultimately trigger slope instability.
Field survey results indicate that only a small number of tension cracks appeared at the front edge of the slope, while significant deformations were observed, especially in the upper part of the slope at the rear edge. This suggests that the actual observations align closely with the calculated results.
4 Conclusion
This study, grounded in rock fracture mechanics, integrates a time-evolving temperature field model and innovatively proposes a new method for assessing slope engineering stability in high-cold regions. Against the backdrop of the unique climatic conditions of high-cold regions, this research deeply considers multiple factors such as freeze-thaw forces, crack water pressure, and gravity, constructing a comprehensive temperature field analysis model. Through systematic theoretical analysis and numerical simulations, this method effectively evaluates and predicts the stability of relevant unstable rock masses.
Experimental results demonstrate that freeze-thaw forces significantly impact the stability of rock masses in high-cold region slope engineering. Specifically, under the influence of freeze-thaw forces, the safety factor of rock mass I# decreases by 18% compared to conditions without freeze-thaw effects. This finding not only underscores the critical role of freeze-thaw forces in the assessment of rock mass stability but also offers new perspectives and references for the design, construction, and maintenance of slope engineering in high-cold regions.
Furthermore, the method proposed in this study exhibits unique advantages and potential in practical engineering applications. Firstly, by comprehensively considering factors such as temperature fields, freeze-thaw forces, and crack water pressure, this method can more comprehensively and accurately assess the stability of rock masses in high-cold region slopes, providing a more scientific basis for engineering design. Secondly, the method enables early warning and risk assessment of unstable rock masses, thereby enhancing engineering safety. Finally, the findings of this study are crucial for guiding the protection and reinforcement of slope engineering in high-cold regions, contributing to the durability and safety of engineering structures.
Looking ahead, we will continue to deepen the research and application of this method, aiming to make greater contributions to the safety and stability of slope engineering in high-cold regions.
Data availability statement
The original contributions presented in the study are included in the article/Supplementary Material, further inquiries can be directed to the corresponding author.
Author contributions
YZ: Methodology, Writing–original draft. KH: Investigation, Resources, Writing–review and editing. DH: Investigation, Resources, Writing–review and editing. YL: Investigation, Resources, Writing–review and editing. LZ: Formal Analysis, Methodology, Software, Writing–review and editing.
Funding
The author(s) declare that financial support was received for the research, authorship, and/or publication of this article. This research is financially supported by the Key R$D and Transformation Plan of Qinghai Province (2023-GX-C06).
Conflict of interest
Authors YZ, KH, DH, and YL were employed by Qinghai Traffic Construction Management Co., Ltd.
Author LZ was employed by Guizhou Geological Engineering Investigation Design and Research Institute Co., Ltd.
Publisher's note
All claims expressed in this article are solely those of the authors and do not necessarily represent those of their affiliated organizations, or those of the publisher, the editors and the reviewers. Any product that may be evaluated in this article, or claim that may be made by its manufacturer, is not guaranteed or endorsed by the publisher.
References
Borghini, G., Rampone, E., Zanetti, A., Class, C., Fumagalli, P., and Godard, M. (2020). Ligurian pyroxenite-peridotite sequences (Italy) and the role of melt-rock reaction in creating enriched-MORB mantle sources. Chem. Geol. 532, 119252. doi:10.1016/j.chemgeo.2019.07.027
Byas, A. D., Applegate, T. J., Stuart, A., Byers, S., and Frank, C. B. (2019). Thymoma-associated exfoliative dermatitis in a goat: case report and brief literature review. J. veterinary diagnostic investigation 31 (6), 905–908. doi:10.1177/1040638719884957
Cabrera, M., Senent, S., Olalla, C., and Jimenez, R. (2015). Sliding stability analysis of gravity dam founded on rock mass with impersistent joint set. Can. Geotechnical J. 52 (11), 1668–1684. doi:10.1139/cgj-2014-0307
Chen, Y. J., Tanaka, M., Tanaka, K., and Wang, H. O. (2014). Stability analysis and region-of-attraction estimation using piecewise polynomial Lyapunov functions: Polynomial fuzzy model approach. IEEE Trans. Fuzzy Syst. 23 (4), 1314–1322. doi:10.1109/tfuzz.2014.2347993
Chen, Y. X., Demeny, A., Schertl, H. P., Zheng, Y. F., Huang, F., Zhou, K., et al. (2020). Tracing subduction zone fluids with distinct Mg isotope compositions: Insights from high-pressure metasomatic rocks (leucophyllites) from the Eastern Alps. Geochimica Cosmochimica Acta 271, 154–178. doi:10.1016/j.gca.2019.12.025
Chiogna, G., Santoni, E., Camin, F., Tonon, A., Majone, B., Trenti, A., et al. (2014). Stable isotope characterization of the Vermigliana catchment. J. Hydrology 509, 295–305. doi:10.1016/j.jhydrol.2013.11.052
Feakins, S. J., Bentley, L. P., Salinas, N., Shenkin, A., Blonder, B., Goldsmith, G. R., et al. (2016). Plant leaf wax biomarkers capture gradients in hydrogen isotopes of precipitation from the Andes and Amazon. Geochimica Cosmochimica Acta 182, 155–172. doi:10.1016/j.gca.2016.03.018
Foresti, L., Sideris, I. V., Panziera, L., Nerini, D., and Germann, U. (2018). A 10-year radar-based analysis of orographic precipitation growth and decay patterns over the Swiss Alpine region. Q. J. R. Meteorological Soc. 144 (716), 2277–2301. doi:10.1002/qj.3364
Frank, S., Goeppert, N., and Goldscheider, N. (2021). Improved understanding of dynamic water and mass budgets of high-alpine karst systems obtained from studying a well-defined catchment area. Hydrol. Process. 35 (4), e14033. doi:10.1002/hyp.14033
Frattini, P., Riva, F., Crosta, G. B., Scotti, R., Greggio, L., Brardinoni, F., et al. (2016). Rock-avalanche geomorphological and hydrological impact on an alpine watershed. Geomorphology 262, 47–60. doi:10.1016/j.geomorph.2016.03.013
Janku-Capova, L., Sutherland, R., Townend, J., Doan, M. L., Massiot, C., Coussens, J., et al. (2018). Fluid flux in fractured rock of the Alpine Fault hanging-wall determined from temperature logs in the DFDP-2B Borehole, New Zealand. Geochem. Geophys. Geosystems 19 (8), 2631–2646. doi:10.1029/2017gc007317
Kenner, R. (2020). Mass wasting processes affecting the surface of an alpine talus slope: Annual sediment budgets 2009–2018 at Flüelapass, eastern Swiss Alps. Land Degrad. Dev. 31 (4), 451–462. doi:10.1002/ldr.3462
Li, Z. J., Li, Z. X., Fan, X. J., Wang, Y., Song, L. L., Gui, J., et al. (2020). Transformation mechanism of ions on different waters in alpine region. Chemosphere 248, 126082. doi:10.1016/j.chemosphere.2020.126082
Liao, Y., Liu, Z., Zhang, G., and Xiang, C. (2016). Vehicle-grid system modeling and stability analysis with forbidden region-based criterion. IEEE Trans. Power Electron. 32 (5), 3499–3512. doi:10.1109/tpel.2016.2587726
Lindström, E., Norén, V., and Madsen, H. (2015). Consumption management in the Nord Pool region: A stability analysis. Appl. energy 146, 239–246. doi:10.1016/j.apenergy.2015.01.113
Lv, Q., Liu, Y., and Yang, Q. (2017). Stability analysis of earthquake-induced rock slope based on back analysis of shear strength parameters of rock mass. Eng. Geol. 228, 39–49. doi:10.1016/j.enggeo.2017.07.007
Mahdavi-Gharavi, M., Mehdian, H., and Hajisharifi, K. (2020). Local stability analysis of interface region of astrophysical viscous shear flows with a gradual velocity gradient. Adv. Space Res. 65 (6), 1607–1614. doi:10.1016/j.asr.2019.12.020
Moradian, M., Ashtiani, F., and Khonsari, A. (2020). Stability region and delay analysis of a SWIPT-based two-way relay network with opportunistic network coding. IEEE Trans. Veh. Technol. 69 (12), 15682–15693. doi:10.1109/tvt.2020.3031662
Nepal, S., Krause, P., Flügel, W. A., Fink, M., and Fischer, C. (2014). Understanding the hydrological system dynamics of a glaciated alpine catchment in the Himalayan region using the J2000 hydrological model. Hydrol. Process. 28 (3), 1329–1344. doi:10.1002/hyp.9627
Nisi, L., Hering, A., Germann, U., and Martius, O. (2018). A 15-year hail streak climatology for the Alpine region. Q. J. R. Meteorological Soc. 144 (714), 1429–1449. doi:10.1002/qj.3286
Oxilia, G., Bortolini, E., Badino, F., Bernardini, F., Gazzoni, V., Lugli, F., et al. (2021). Exploring late Paleolithic and Mesolithic diet in the Eastern Alpine region of Italy through multiple proxies. Am. J. Phys. Anthropol. 174 (2), 232–253. doi:10.1002/ajpa.24128
Pan, Y., Liu, F., Chen, L., Wang, J., Qiu, F., Shen, C., et al. (2017). Towards the robust small-signal stability region of power systems under perturbations such as uncertain and volatile wind generation. IEEE Trans. Power Syst. 33 (2), 1790–1799. doi:10.1109/tpwrs.2017.2714759
Panpan, W. (2018). Research on the design of the box traction substation in the alpine-cold region. Electr. Eng.
Salimbeni, S., Malusà, M. G., Zhao, L., Guillot, S., Pondrelli, S., Margheriti, L., et al. (2018). Active and fossil mantle flows in the western Alpine region unravelled by seismic anisotropy analysis and high-resolution P wave tomography. Tectonophysics 731, 35–47. doi:10.1016/j.tecto.2018.03.002
Sternai, P., Sue, C., Husson, L., Serpelloni, E., Becker, T. W., Willett, S. D., et al. (2019). Present-day uplift of the European Alps: Evaluating mechanisms and models of their relative contributions. Earth-Science Rev. 190, 589–604. doi:10.1016/j.earscirev.2019.01.005
Sun, F., Lü, Y., Fu, B., Ma, Z., and Yao, X. (2014). Spatial explicit soil moisture analysis: pattern and its stability at small catchment scale in the loess hilly region of China. Hydrol. Process. 28 (13), 4091–4109. doi:10.1002/hyp.9940
Verlaguet, A., Goffé, B., Brunet, F., Poinssot, C., Vidal, O., Findling, N., et al. (2011). Metamorphic veining and mass transfer in a chemically closed system: a case study in Alpine metabauxites (western Vanoise). J. Metamorph. Geol. 29 (3), 275–300. doi:10.1111/j.1525-1314.2010.00918.x
Wang, L., Yin, Y., Huang, B., and Dai, Z. (2020). Damage evolution and stability analysis of the Jianchuandong dangerous rock mass in the Three Gorges Reservoir Area. Eng. Geol. 265, 105439. doi:10.1016/j.enggeo.2019.105439
Wang, X., Blaabjerg, F., and Loh, P. C. (2017). Passivity-based stability analysis and damping injection for multiparalleled VSCs with LCL filters. IEEE Trans. Power Electron. 32 (11), 8922–8935. doi:10.1109/tpel.2017.2651948
Zhu, M., Huang, B., Liu, Z., Wang, Y., Teng, J., Tian, X., et al. (2023). The distribution, effectiveness and environmental threshold of soil aggregate phosphorus fractions in the sub-alpine region of Southwest China. Land Degrad. Dev. 34 (1), 3–15. doi:10.1002/ldr.4401
Appendix
Rock mechanics model
To analyze the specific stability conditions of each unstable rock mass affected by the structural plane cutting, we made the following assumptions in our model:
1. Due to the reduced stiffness of the rock mass at the back caused by the structural plane cutting, we assume the rock mass as a rigid body, considering changes only at the structural plane.
2. To simplify the model and focus on the effects of structural plane cutting, we exclude the vibrational impact of rock collapse on other rock masses.
3. When rock mass collapses, it experiences rotation around the centroid of the unpenetrated section of the structure at the back. This assumption considers the mechanical behavior of rock mass collapse, emphasizing the impact of structural plane cutting on rock mass stability.
These assumptions contribute to establishing a simplified yet practically feasible model for an in-depth analysis of the specific effects of structural plane cutting on the stability conditions of each unstable rock mass.
Based on geological cross-sectional data (as shown in Figure 1), we constructed a retrogressive rock slope model, as illustrated in Figure 6.
This slope is influenced by the cutting of structural planes in various rock masses, and the stability of the slope is controlled by the structural planes in multiple rock masses. We subdivided the analyzed unstable rock masses into n potential unstable approximate rectangular rock masses, where the thickness of each rock mass is
We perform force analysis on the nth-1, nth, and nth+1 rock masses, and their force models are detailed in Figure 7.
In this model, the loads borne by the rock mass primarily include self-weight G, interlayer water pressure
Taking the nth rock mass as an example, we decompose its self-weight, splitting it into components along the structural plane's normal and tangential directions, obtaining the tangential stress (
Keywords: high-cold region, precarious rock mass, freeze-thaw forces, stress intensity factor, engineering stability
Citation: Zhao Y, Hu K, Han D, Lang Y and Zhang L (2024) A novel approach to assessing precarious rock instability in high-cold regions considering freeze-thaw forces. Front. Earth Sci. 12:1422093. doi: 10.3389/feart.2024.1422093
Received: 23 April 2024; Accepted: 12 July 2024;
Published: 29 July 2024.
Edited by:
Luqi Wang, Chongqing University, ChinaReviewed by:
Cihang Huang, Purdue University, United StatesPeng Xu, Ningxia University, China
Limin Duan, Henan University, China
Copyright © 2024 Zhao, Hu, Han, Lang and Zhang. This is an open-access article distributed under the terms of the Creative Commons Attribution License (CC BY). The use, distribution or reproduction in other forums is permitted, provided the original author(s) and the copyright owner(s) are credited and that the original publication in this journal is cited, in accordance with accepted academic practice. No use, distribution or reproduction is permitted which does not comply with these terms.
*Correspondence: Lin Zhang, bGluejY1ODMwQGdtYWlsLmNvbQ==