- 1School of Earth Sciences and Engineering, Xi’an Shiyou University, Xi’an, China
- 2Shaanxi Key Laboratory of Petroleum Accumulation Geology, Xi’an Shiyou University, Xi’an, China
- 3Key Laboratory of Petroleum Resources Research, Lanzhou, China
- 4CNOOC (China) Limited Tianjin branch, Tianjin, China
- 5Northwest Institute of Eco-Environment and Resources, Chinese Academy of Sciences, Lanzhou, China
- 6Institute of Petroleum Exploration and Development, PetroChina Tarim Oilfield Company, Korla, China
Extensional strike-slip basins have unique structural features that influence both sub-sags and traps. Despite previous investigations into the extension-strike-slip fault system, there remains a gap in the comprehensive analysis of its influence on sub-sag formation and trap development. The Huanghekoudong and Miaoxi’nan sub-sags within the Bohai Bay Basin, intersected by the Tan-Lu Fault Zone, offer exemplary cases for examining the interplay between strike-slip and extensional tectonic regimes. The neotectonic strike-slip movements along the Tan-Lu Fault Zone have given rise to various extensional strike-slip overlap zones in these sub-sags, rendering these locales ideal for investigating the dynamics of strike-slip and extensional tectonic processes. Employing three-dimensional seismic data, well logs, and a newly constructed sequence stratigraphic framework, our research delineates the geometric and kinematic phenomena characteristic of the extensional strike-slip fault system, including the dynamics of extensional fault deformation and interaction through time. By scrutinizing the fault system’s vital role in shaping sub-sag evolution and trap genesis, we present a comprehensive model that significantly contributes to our understanding of structural trapping dynamics. This model not only fine-tunes existing trapping models but also offers invaluable insights for future exploration strategies within the Bohai Bay Basin and other similar extensional strike-slip basins worldwide. Our findings highlight the novel and significant implications of the extensional strike-slip fault system in controlling sub-sag and trap features, thus bridging a notable gap in existing geotechnical knowledge.
1 Introduction
The concept of the extensional strike-slip fault system delineates a multifaceted fault arrangement arising from the overlay of pronounced late-stage strike-slip movements upon early-stage extensional faults (Li et al., 2020b; Li et al., 2021). This system plays a pivotal role in dictating the tectonic framework of several hydrocarbon-rich sedimentary basins, including the Bohai Bay Basin, Qiongdongnan Basin, Yinggehai Basin, and Liaohe Basin (Ren et al., 2011; Liu et al., 2019; Li et al., 2020a; Li et al., 2023).
These basins exhibit unique structural traits and mechanisms controlling sub-sag formation and trap development. While existing literature has extensively explored the structural aspects of strike-slip faults and their correlation with hydrocarbon occurrences (Tong et al., 2008; Liu et al., 2010; Teng et al., 2016; Ma et el., 2018; Wang et al., 2020; Deng et al., 2022; Deng et al., 2022; Yang et al., 2022), a profound comprehension of the extensional strike-slip architectures and their control mechanisms on sub-sag and trap formations remains elusive.
Focusing on the southern Bohai Sea’s Huanghekoudong (HHKD) sub-sag and MXN (Miaoxi’nan) sub-sag, areas of pronounced oil and gas exploration potential intersected by the Tan-Lu Fault Zone, this study aims to unravel the complexity of superimposed deformation characterized by initial extension followed by strike-slip activity. The presence of abundant and illustrative extensional strike-slip faults renders this area an exemplary site for dissecting the evolution of extensional strike-slip fault systems alongside their implications on depression development and trap genesis. Employing the latest three-dimensional seismic data, augmented by recent drilling, logging, and other analytical outcomes, this research delves into the fault combination characteristics and evolutionary patterns within the strike-slip fault zone, utilizing techniques such as variance slicing. Through this analysis, the study advances our understanding of the control mechanisms exerted by the extensional strike-slip fault system on depression formation, trap configuration, and overall structural trapping paradigms, thereby offering valuable insights for future exploration strategies in the study area and serving as a benchmark for related investigations within extensional strike-slip basins.
2 Geological setting
The study area comprises of the HHKD and MXN sub-sags, and the location of the study area is shown in Figure 1C.
2.1 The MXN sub-sag
The MXN sub-sag, nestled within the prolific hydrocarbon terrain of the Bohai Sea area, forms an integral part of the sedimentary tectonic framework of the Bohai Bay Basin (BBB). This Cenozoic basin, stretched along China’s eastern coast, encompasses a vast expanse, estimated at approximately 200,000 km2, and is noted for its complex rifted structure shaped through multi-phase tectonic evolutions (Lu et al., 1997; Chi and Zhao, 2000; Hao et al., 2007; 2009; Schlische and Withjack, 2009; Henza et al., 2010; Yin, 2010; Zuo et al., 2011; Xu et al., 2014; Chang et al., 2018; Song et al., 2019; Jin et al., 2020; Ye et al., 2020; Yu et al., 2020) (Figure 1A). Enveloped by ancient uplifts such as Yan Shan, Taihang Shan, Jiaoliao, and Luxi, the BBB is dissected into multiple subbasins and uplifts, including Liaodong Bay, Liaohe, Bozhong, and others, housing significant hydrocarbon reserves (Hao et al., 2009; 2011; Li et al., 2017; Shi et al., 2019; Yu et al., 2020; Zeng et al., 2022).
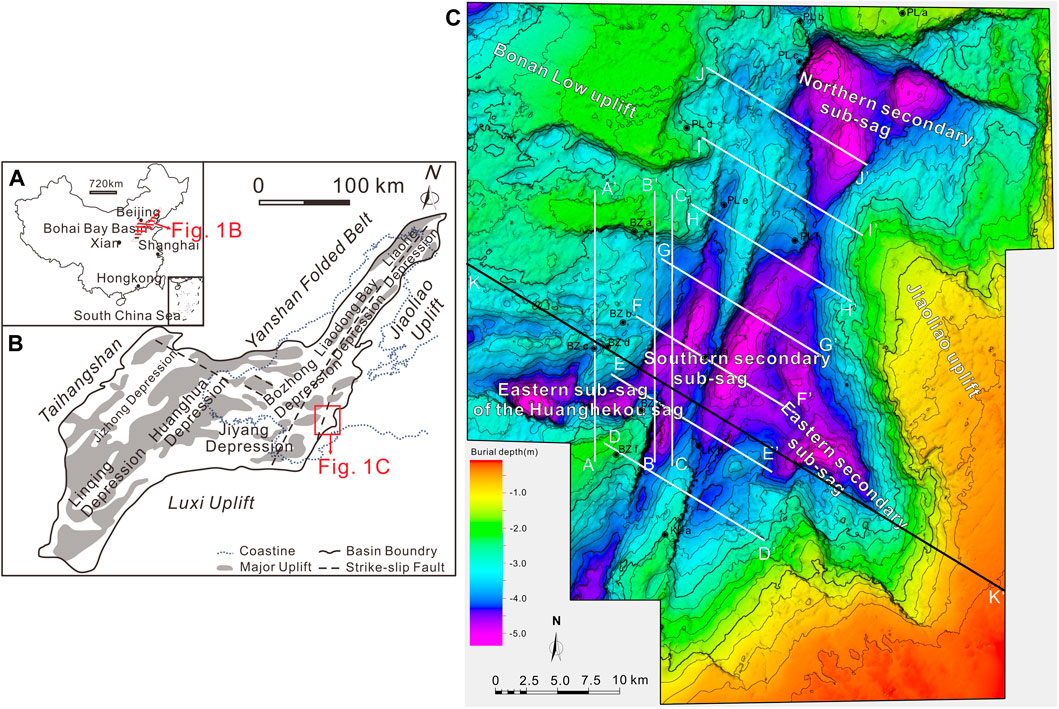
Figure 1. (A) Tectonic location of the Bohai Bay Basin in China. (B) Tectonic location of the southern sub-sag of the HHKD and MXN sub-sags in the Bohai Bay Basin. (C) Structural unit sketch map of the study area, displaying the present structural units of the HHKD and MXN sub-sags. The figure also includes seismic lines and wells used in this paper.
Residing at the basin’s margin, the MXN sub-sag is a testament to the regional geological complexity, flanked by prominent mountains and demonstrating substantial hydrocarbon accumulations with crude oil and natural gas proven reserves (Jiang et al., 2016; Hou and Hari, 2014). As a Cenozoic sub-sag, it is demarcated by key geological structures, including the Jiaoliao uplift and Bonan Low uplift, and is directly linked to neighboring sub-sags, illustrating a northeastward strip-shaped distribution across 1200 km2 (Qi, 2004; Zhou et al., 2010; Zong et al., 2012; Huang et al., 2018) (Figure 1B).
The structural intricacy of the MXN sub-sag is further exemplified by the east branch of the Laizhouwan section within the Tan-Lu Fault Zone (TLFZ), which bisects the sub-sag, creating distinct geological divisions that have played a crucial role in its formation and hydrocarbon potential, including significant oilfields such as Bozhong 36–1 and Penglai 25–6 (Gong et al., 2007; Zhao et al., 2020).
2.2 The HHKD sub-sag
The HHKD sub-sag is located in the eastern Bohai Bay Basin, NE China, sandwiched between the Bonan and Laibei uplifts to the north and south, and connected with the MXN sub-sag to the east (Figure 1B). Two major tectonic systems of east–west extension and northeastward strike–slip structures control the basic tectonic framework of the region (Hsiao et al., 2004; 2010; Liu et al., 2010; Qi and Yang, 2010; Tong et al., 2012; Li et al., 2013; Liu et al., 2015). The structural appearance resembles a graben reworked by slip–strike faults; it is steeper in the south and gentle to the north, higher in the west, and buried deeper in the east (Sun et al., 2017; Liu et al., 2018; Lan and Liu, 2022).
Stratigraphically, the study area hosts both Paleogene and Neogene deposits, with the Paleogene characterized by formations rich in fan- and braided-deltas and lacustrine deposits, while the Neogene features fluvial and deltaic sequences, highlighting the dynamic sedimentary processes that have shaped its geological history (Hou et al., 2001) (Figure 2). The amalgamation of these structural and stratigraphic elements underscores the study area’s significance within the broader context of the BBB’s geological and hydrocarbon framework.
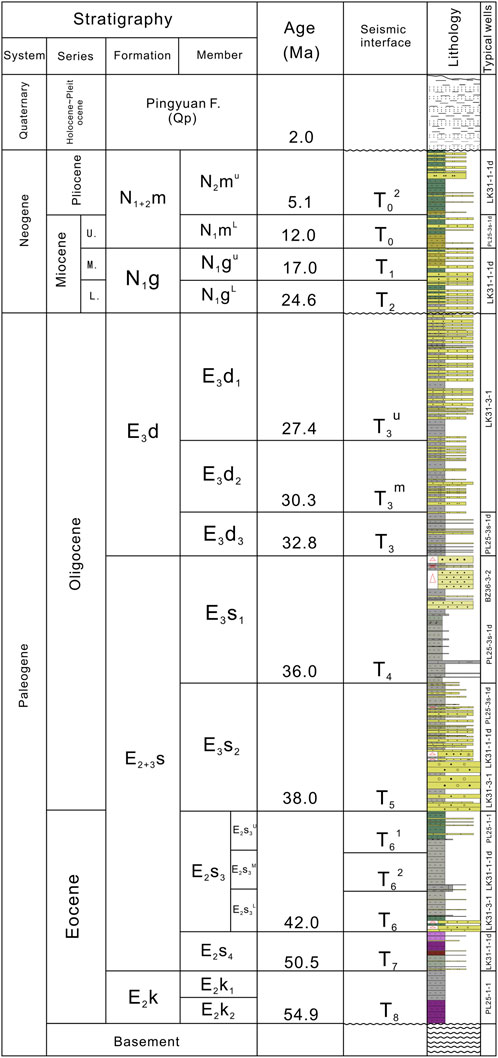
Figure 2. Tectono-stratigraphic log of the HHKD and MXN sub-sags (modified after Sun, 2017) (Abbreviations: N1+2m=Mianghuazhen Formation, N2mU=Upper member of the Minghuazhen Formation, N2mL=Lower member of the Minghuazhen Formation, N1g=Guantao Formation, N1gU= Upper member of the Guantao Formation, N1gL= Lower member of the Guantao Formation, E3d=Dongying Formation, E3d1=First member of the Dongying Formation, E3d2=Second member of the Dongying Formation, E3d3=Third member of the Dongying Formation, E2+3s=Shahejie Formation, E3s1=First member of the Shahejie Formation, E3s2=Second member of the Shahejie Formation, E3s3= Third member of the Shahejie Formation, E2s3U=Upper section of the Third member of the Shahejie Formation, E2s3M=Middle section of the Third member of the Shahejie Formation, E2S3L= Lower section of the Third member of the Shahejie Formation, E3s4= Fourth member of the Shahejie Formation; E2k=Kongdian Formation, E2k1=First member of the Kongdian Formation, E2k2=Second member of the Kongdian Formation).
3 Data acquisition and analytical methodology
3.1 Acquisition of data
This investigation integrated multidimensional seismic datasets, borehole recordings, and auxiliary geological insights specifically from the HHKD and MXN sub-sags area. A comprehensive spatial coverage was achieved through a high-resolution 3D seismic survey. Detailed lithological interpretations were derived from 15 strategically positioned wells, leveraging an array of logging techniques. The suite of well log data predominantly included gamma-ray (GR) logs, spontaneous potential (SP) logs, acoustic time (AC) logs, among other crucial datasets, providing insights into the primary lithological frameworks within the MXN subregion. The acquisition of both seismic and borehole datasets was facilitated through collaboration with CNOOC (China) Co. Ltd., Tianjin branch.
This method provides thorough geological and spatial knowledge, but it has drawbacks due to seismic resolution limits and the subjective nature of well log interpretation. Seismic data may inaccurately represent stratigraphic features due to complex geology, and well log analysis can be biased by the geologists’ expertise.
3.2 Analytical techniques
Our methodology encompassed a rigorous examination of the spatial and structural dynamics of extensional strike-slip fault systems within the sub-sag. This involved a meticulous interpretation of three-dimensional seismic datasets, including the analysis of sequence boundary variance slices and representative vertical seismic profiles within the established sequence stratigraphic framework. To elucidate the tectono-stratigraphic evolution of the sub-sag, we applied back-stripping analysis. This technique facilitated the construction of balanced cross-sections, enabling a detailed visualization of the basin’s architectural and stratigraphic heterogeneity. Furthermore, our study delved into assessing the impact of extensional strike-slip faulting on the stratigraphic framework, spatial configuration of sub-sags, and the consequent hydrocarbon potential.
The employed analytical methods are robust but have limitations. Seismic data interpretation relies on data quality, which varies and can create uncertainty in fault and boundary analysis. Back-stripping, useful for studying basin evolution, depends on unverifiable assumptions about geological history, which can affect results. The analysis’s success also hinges on the accuracy of seismic and well log data inputs.
4 Main fault characteristics
The southern Bohai area is characterized by nearly east-west trending extensional faults and NNE trending strike-slip faults, dividing the area into multiple tectonic units. The HHKD and MXN sub-sags are developed with extensional fault systems, strike-slip fault systems, and extensional strike-slip fault systems (Table 1). Due to the nature and activity intensity of the faults, there are significant differences in the structural characteristics of the faults.
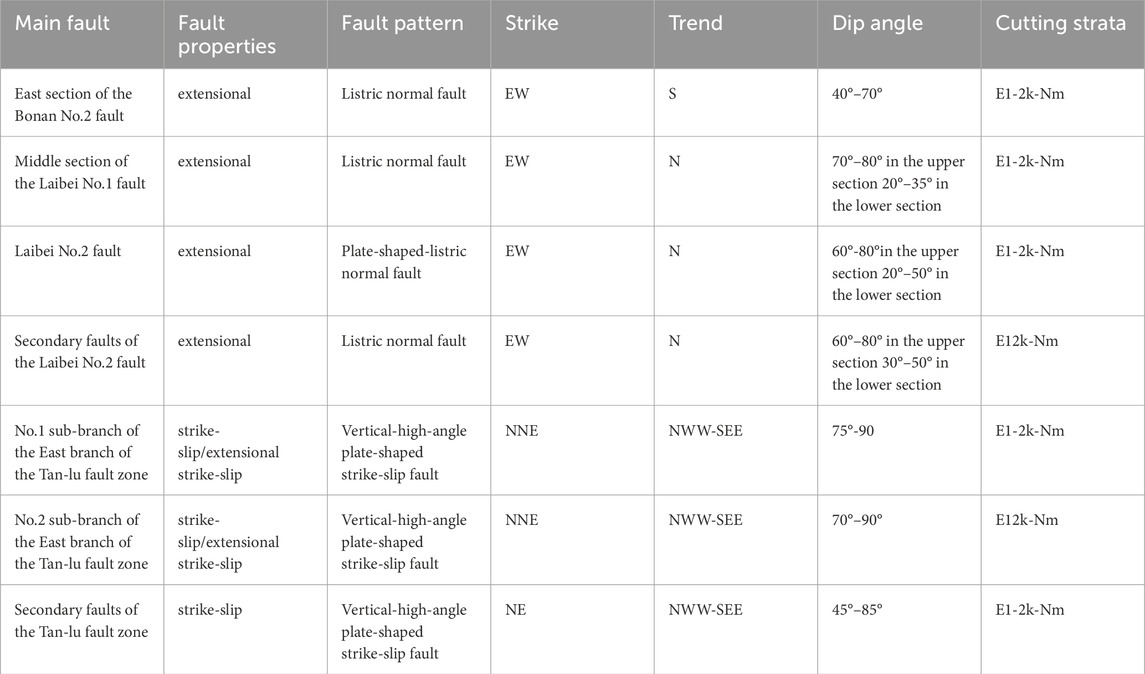
Table 1. Elements of the typical faults in eastern sub-sag of the Huanghekou sag and Miaoxinan sub-sag.
4.1 Extensional fault system overview
The extensional fault system in the HHKD and MXN sub-sags features predominantly EW-NW trending faults, exhibiting significant control over sedimentary and structural patterns. These faults display bifurcation and arc shapes, with echelon or feather-like intersections with secondary faults, observed in various combinations (Figure 3). Their listric and shovel-like profiles cut through deep strata, with near-surface “y” and inverted “y” shapes formed by interactions with secondary faults. The system’s activity varies across sections and periods.
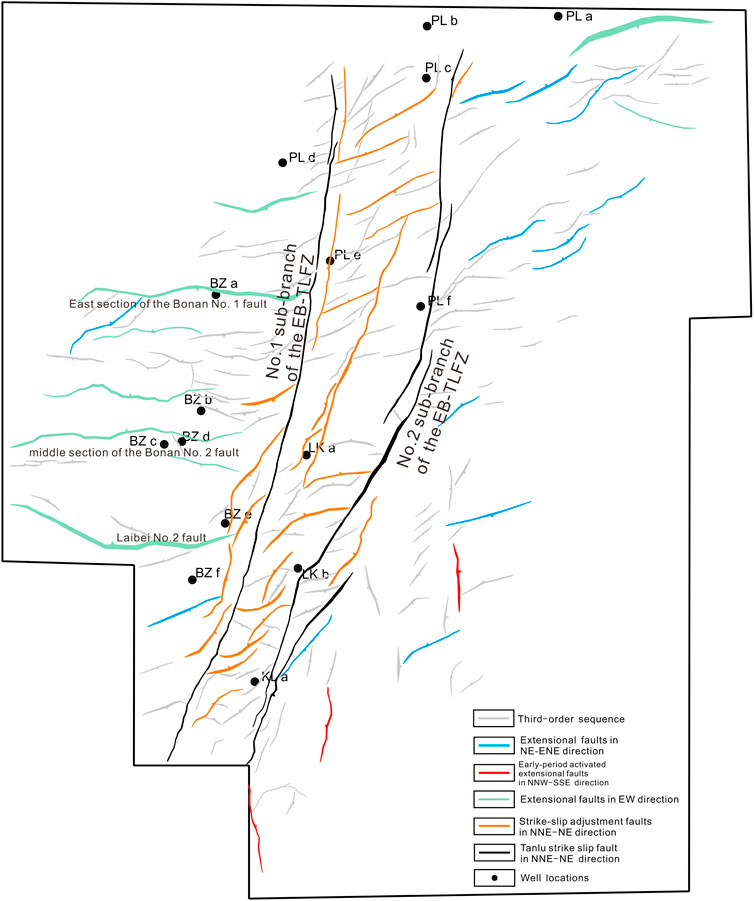
Figure 3. Planar distribution of the fault systems in T3 (the interface between E3s1 and E3d) of the HHKD and MXN sub-sags.
4.1.1 East section of the Bonan No. 1 fault (BNF)
The Eastern Section of the Bonan No. One Fault displays EW-NW trending extensional faults with bifurcations and arc shapes. Notably, the eastern section intersects with the EB-TLFZ and extends over 13 km. Fault profiles reveal steep, shovel-like dips in the subsurface and “y” shaped formations near-surface. Survey lines AA’, BB’, and CC’ highlight variations in fault geometry, with angles ranging from 30° to 50° at depth to 75°–85° at shallow levels. Secondary faults create flower structures, particularly near the eastern strike-slip transition zone (Figures 4–6).
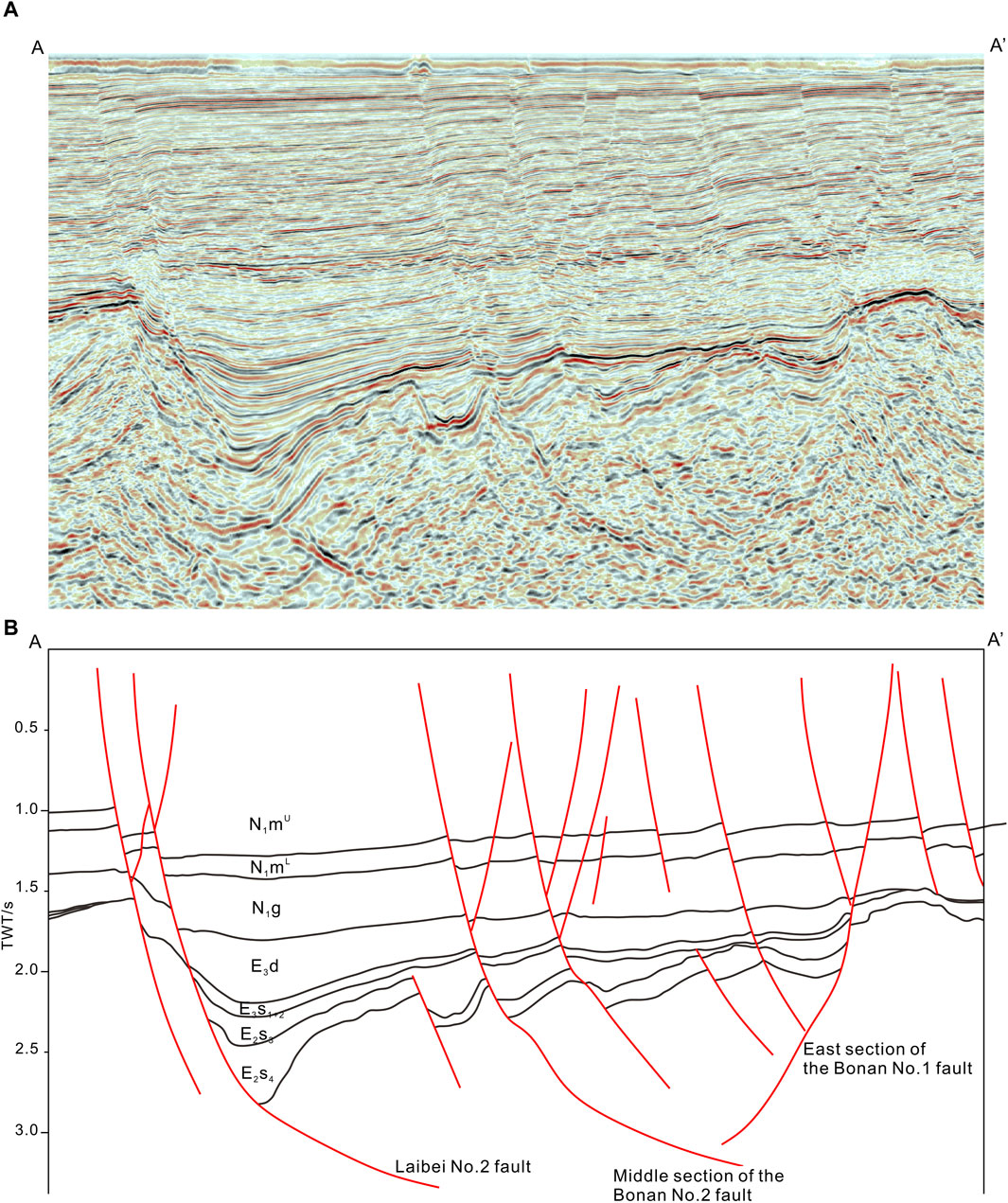
Figure 4. Interpreted seismic section AA’, showing the vertical distribution of extensional fault system. (A) Original seismic section AA’, (B) Interpreted section AA’ (see its location in Figure 1).
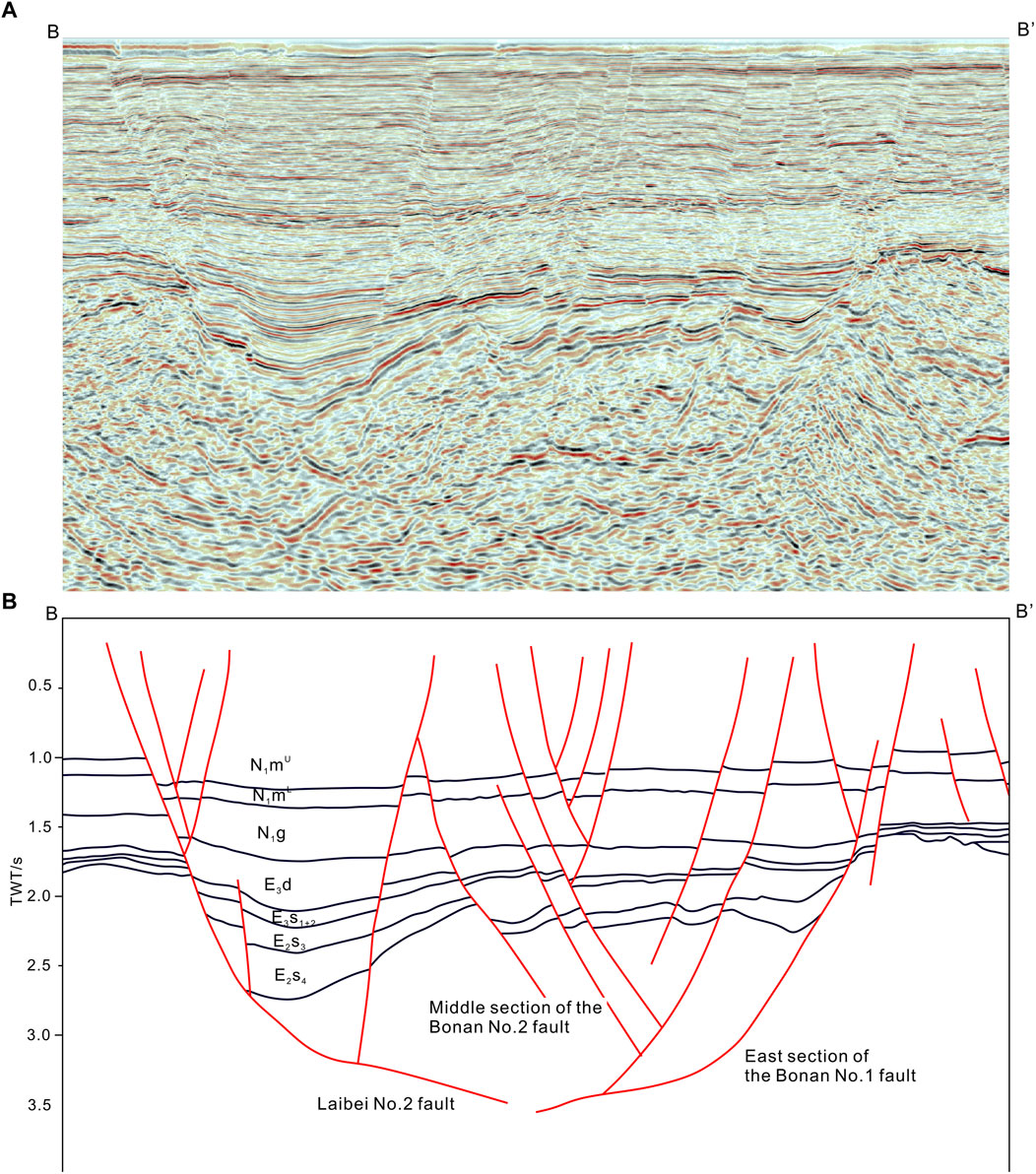
Figure 5. Interpreted seismic section BB’, showing the vertical distribution of extensional fault system. (A) Original seismic section BB’, (B) Interpreted section BB’ (its location is shown in Figure 1).
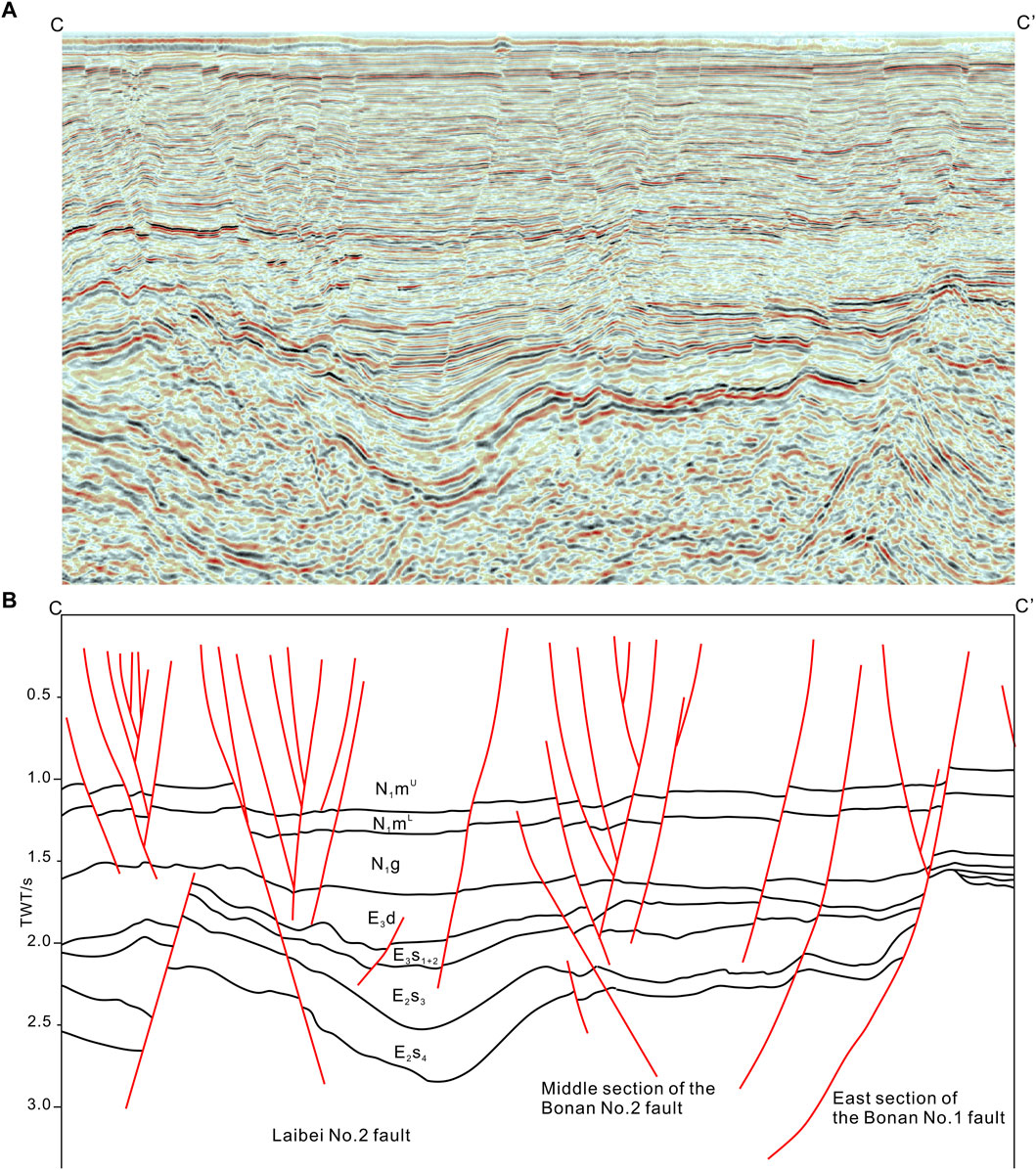
Figure 6. Interpreted seismic section CC, showing the vertical distribution of extensional fault system. (A) Original seismic section CC’, (B) Interpreted section CC’ (see its location in Figure 1).
4.1.2 Middle section of the Bonan No. 2 fault
The Middle Section of the Bonan No. 2 Fault characterizes steep shovel-like normal faults in the profile, with deep angles of 25°–35° and shallower angles of 70°–80°. Listric normal faults appear near the eastern end, and secondary faults generate inverted “y” and flower-like structures, forming a fault scarp zone on the northern side (Figures 4–6).
4.1.3 Laibei No. 2 fault
The Laibei No. 2 Fault is marked by steep shovel-like normal faults with deep angles of 25°–40° and shallow angles of 70°–80°. It features continuous main fault sections with developed secondary faults near the surface, forming inverted “y” and flower-like structures (Figures 4–6).
4.2 Strike-slip fault system overview
The eastern branch of the Tan-Lu fault delineates the HHKD and MXN sub-sags, running NNE with notable continuity and variability (Figure 3). Vertical main faults slice through deep strata, with their interactions with secondary faults resulting in complex profiles.
4.2.1 EB-TLFZ sub-branches
The EB-TLFZ delineates the eastern and western borders of the HHKD and MXN sub-sags, stretching approximately 71 km in length and 4 km in width, trending NNE. It comprises mainly the No. 1 and No. 2 sub-branches, exhibiting nearly vertical high-angle listric fault profiles which penetrate deep strata down to the Cenozoic base, showing varying orientations from NWW in the north to SEE in the south, which causes a ribbon effect. This zone is characterized by large-scale secondary fault formations at shallower depths under strong strike-slip stress, presenting various flower-like structures alongside the main faults. Notably, the middle sections of both sub-branches exhibit extensional strike-slip features, significantly influencing the area’s structural integrity (Figures 3, 7, 8).
Seismic analysis across different sections of the EB-TLFZ reveals distinct fault characteristics:
Section JJ’ shows the No. 1 sub-branch with steep shallow and gentler deep angles, and the No. 2 with generally gentler angles but nearly vertical at depth. Secondary faults are notably developed on the No. 1 sub-branch, mainly on the southeast, displaying flower-like structures, while the No. 2 sub-branch has secondary faults on both sides, forming an inverted flower-like structure (Figures 7G, 8G).
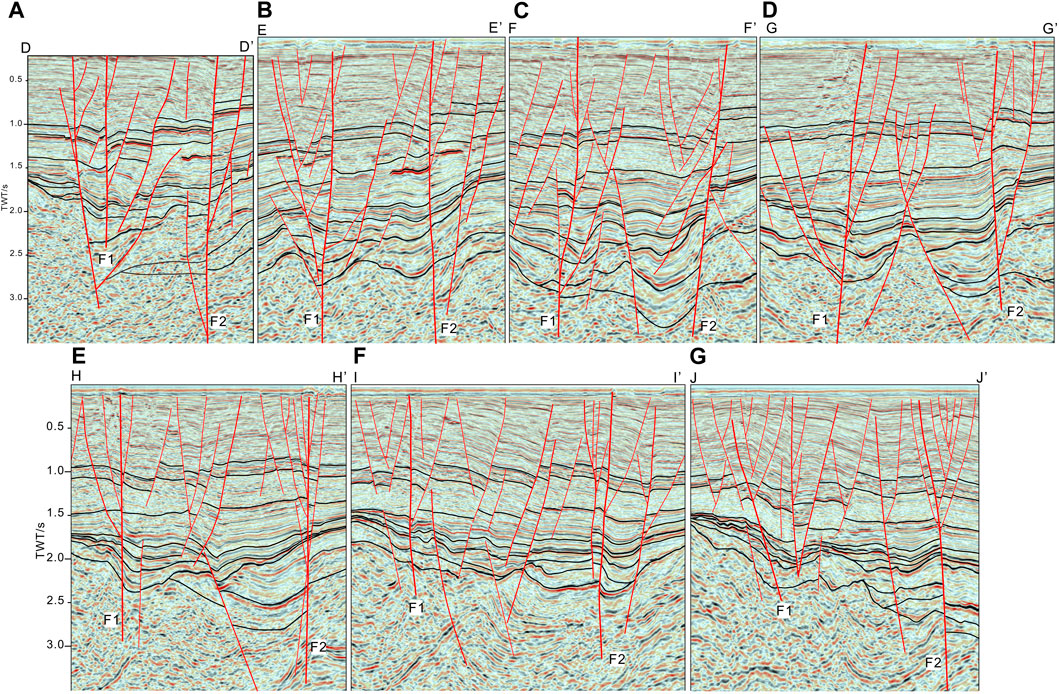
Figure 7. Interpreted inline seismic sections showing the vertical distribution of strike-slip and extensional strike-slip fault system. (A) Interpreted seismic section DD’, (B) Interpreted seismic section EE’, (C) Interpreted seismic section FF’, (D) Interpreted seismic section GG’, (E) Interpreted seismic section HH’, (F) Interpreted seismic section II’, (G) Interpreted seismic section JJ’ (see their locations in Fig.1). Notes: F1: No. 1 sub-branch of EB-TLFZ, F2: No. 2 sub-branch of EB-TLFZ.
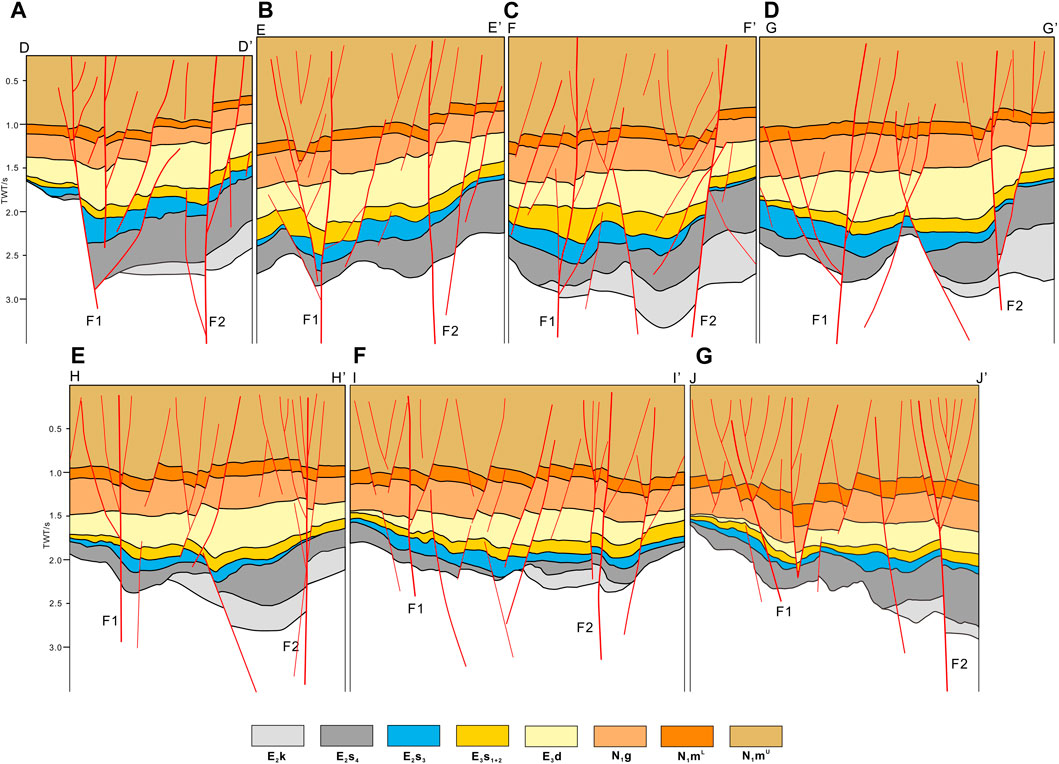
Figure 8. Geological sections showing the vertical distribution of strike-slip and extensional strike-slip fault system. (A) Geological section DD’, (B) Geological section EE’, (C) Geological section FF’, (D) Geological section GG’, (E) Geological section HH’, (F) Geological section II’, (G) Geological section JJ’ (see their locations in Figure 1).
Section HH’ indicates both sub-branches’ main faults are nearly vertical, with the No. 1 sub-branch displaying developed secondary faults on both sides forming an inverted flower-like structure, and the No. 2 sub-branch having fewer secondary faults, forming a flower-like structure (Figures 7E, 8E).
Section FF’ highlights the No. 1 sub-branch’s nearly vertical main fault cutting down to the Mesozoic base and developing numerous secondary faults under intense strike-slip stress, creating an inverted flower-like structure. The No. 2 sub-branch’s main fault has a dip angle of 70°–80°, with fewer secondary faults forming a semi-flower-like structure at shallow levels (Figures 7C, 8C).
4.2.2 Eastern branch secondary faults
The eastern branch secondary faults, within the strike-slip transition zone of the east, extend northeastward for about 20 km, connecting to the first and second sub-branches of the EB-TLFZ at their southwestern and northeastern ends, respectively. These faults have a continuous presence at depth but are less continuous closer to the surface (Figure 3). Their profiles reveal steep listric shapes with angles between 70° and 80°, and they cut through to the base of the Cenozoic stratum. Adjacent to the main fault, particularly on the northeastern side, is an area of enhanced secondary faulting, which results in a floral structural pattern (Figures 7D, 8D), influenced by strike-slip stress.
4.3 Extensional strike-slip fault system overview
The study area features extensional strike-slip faults primarily within the No. 2 sub-branch of the east branch of the Tan-Lu fault zone, characterized by complex structures akin to inverted or semi-flower patterns superposed on listric and shovel-like normal faults. Seismic section HH’ reveals a concentration of these faults in the same sub-branch, where they exhibit simple patterns in deep layers but complex combinations of derivative faults in shallow layers due to extensional, strike-slip, and compressional interactions. The inline 5200 intersects this sub-branch and shows the main fault with a shallow dip angle of 80°–90° that steepens with depth, maintaining strong continuity. In the shallow portions, secondary faults form flower-like structures with the main fault, while at greater depths, they create “Y” shaped configurations (refer to Figures 7E, 8E).
5 The structural pattern of the fault systems in HHKD and MXN sub-sags
The Huanghekou sag and Miaoxi Sag are secondary negative structural units in the southern section of the Bonan area, both controlled by boundary extensional faults that influence the sag sedimentation, with internal extensional fault systems controlling the sag pattern. The Huanghekou sag features a “north-faulted, south-overlapped” fan-shaped structure, while the Miaoxi sag’ sub-sags are variably affected by the Tan-Lu Fault Zone; the northern and eastern sub-sags are mainly reformed by the extensional fault system, and the southern sub-sag is mainly reformed by the east branch strike-slip fault zone of the Tan-Lu Fault, with the northern part being a graben structure and the southern part being a fan-shaped fault sag. The structural pattern of the sags is due to the differentiation of faults (Table 2). The extensional faults of both sags are nearly EW-oriented, and their profile morphologies are mostly listric and shovel-shaped normal faults. Strike-slip faults mainly develop in the central strike-slip transition zone and east branch strike-slip transition zone, with significant morphological differences; the east branch strike-slip fault’s main section is more upright compared to the central branch. The same extensional fault shows morphological differences at different locations, and the combination patterns of secondary faults also vary. Superposed strike-slip faults are primarily distributed in the middle of the central branch strike-slip fault and have similar morphological features on profiles.
The Structural Pattern refers to a specific combination of profile morphology and strain mechanism that are closely related (Xu et al., 2014). Based on the analysis of seismic profiles in the southern Bonan area, fault characteristics, and combination patterns, three types of Structural Patterns can be identified: extensional Structural Pattern, strike-slip Structural Pattern, and superposed strike-slip Structural Pattern. Now, the internal Structural Patterns of the HHKD and MXN sub-sags will be analyzed and compared.
5.1 Extensional structural pattern
The extensional structural pattern is a structural combination formed under tensional forces. In the study area, this style is mainly composed of normal faults, forming “Y,” inverted “Y,” and flower-like structures. The extensional faults in the HHKD and MXN sub-sags trend nearly EW (Table 2), with secondary faults arranged in echelon or intersecting the main faults in a feather-like pattern. The HHKD sub-sag has three major extensional faults: the Laibei No. 2 fault, the central section of Bonan No. 2, and the eastern section of Bonan No. 1.
5.2 Strike-slip structural pattern
The strike-slip structural pattern results from compressional or tensional shear stress, leading to a combination of strike-slip faults. The study area’s strike-slip style is mainly characterized by negative flower structures, flower-like structures, and half-flower structures (Table 2). Strike-slip faults mainly distribute along the eastern branch of the Tan-Lu Fault Zone, trending NNE and controlling the boundary of the HHKD sub-sag. The northern part of the eastern strike-slip fault extends to the Miaoxi northern sub-depression, and the southern part to the Laizhou Bay Depression. Negative flower structures have branching faults upward, forming Y-shaped structures, with the main fault appearing as upright or high-angle listric normal faults. The eastern branch No. One strike-slip fault is primarily characterized by negative flower structures, while the eastern branch No. 2 develops a few such structures (Table 2).
Flower-like structures are combinations of a main fault with multiple secondary faults on the profile. Both the eastern branch No. 2 and its secondary faults develop flower-like structures, whereas the eastern branch No. 1 has fewer flower-like structures. In addition, internal structures within the two sub-depressions also develop, mainly in areas with abundant shallow secondary faults, forming flower-like structures in combination with the main faults locally, as Laibei No. 2 and Bonan No. 1 faults. Half-flower structures are combinations with multiple derivative faults on one side of the main fault, with the derivative faults being normal faults. In the eastern branch strike-slip fault zone, local areas of the eastern branch No. 2 fault develop half-flower structures, with the main fault being steep and the overall fault profile being clear (Table 2).
5.3 Extensional strike-slip structural pattern
The overlapping strike-slip structural pattern is a combination of faults formed under both extensional and strike-slip tectonic deformation. Overlapping strike-slip structures are later strike-slip tectonic movements superimposed on early extensional faults, manifesting as flower structures and multi-level “Y”-shaped combination styles. The overlapping strike-slip area is distributed in the central part of the eastern branch strike-slip fault zone between the HHKD and MXN sub-sags, mainly superimposing strike-slip movements on listric and listric normal faults (Table 2).
All three structural patterns are developed in the study area. Among them, the extensional structural pattern is more widespread, mainly distributed along the edges and interiors of the two sub-depressions, trending nearly EW, with long extensions; strike-slip and overlapping strike-slip structural patterns mainly develop along the eastern branch strike-slip fault zone on the edge of the HHKD sub-sag, trending NNE and extending long. The main extensional structures in the HHKD and MXN sub-sags are listric and listric normal faults, with shallow secondary faults forming “Y,” inverted “Y,” and flower-like structures in combination with the main faults; the main strike-slip faults on the profile appear as nearly upright listric faults, combined with shallow secondary faults to form negative flower, half-flower, and flower-like structures; overlapping strike-slip develops in the overlapping area of extension and strike-slip, mainly distributed in the central part of the eastern branch strike-slip transition zone, with extensional structures on both sides being more developed, leading to overlapping, mainly characterized by strike-slip movements superimposing on listric and listric normal faults in the strike-slip zone (Table 2).
6 Discussion
6.1 Tectono-stratigraphic evolution of the study area
Throughout the faulting phase, the boundary faults of the MXN sub-sag were subject to intense activation, markedly influencing sedimentary processes and leading to distinct segmentation within the sub-sags. Initial formation of the MXN sub-sag was observed during the E2k depositional phase, signified by the rise of the eastern secondary sub-sag. Sedimentary accumulation predominantly occurred within the southern and eastern secondary sub-sags, with sedimentary focus centralized in the eastern section. The E2k stratigraphy was shaped by three critical faults, notably with the northwest fault acting as the eastern segmental offshoot of the EB-TLFZ (Figure 9).
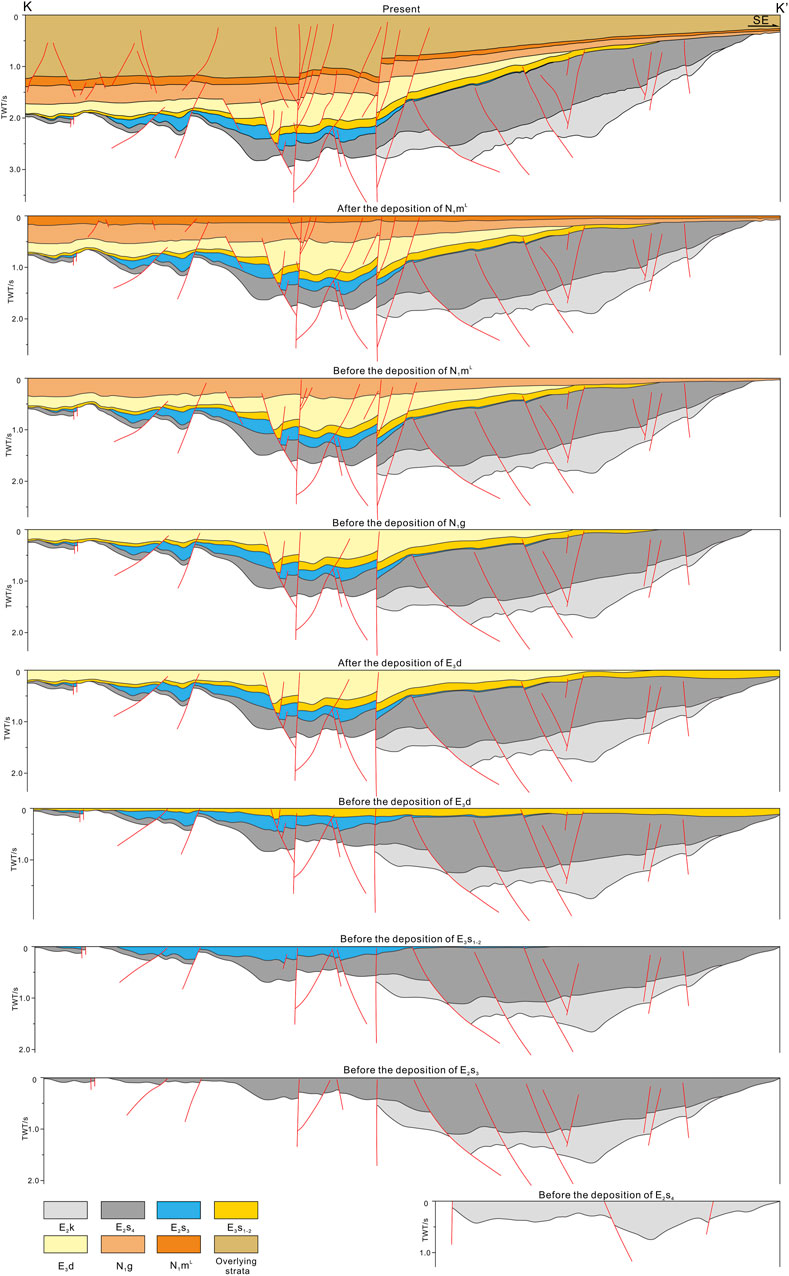
Figure 9. Balanced section KK’ illustrating the stratigraphic evolution characteristics of the southern secondary sub-sag of the MXN sub-sag (the location of seismic section KK’ are shown in Figure 1).
In the E2s4 depositional phase, expansion of the MXN sub-sag was notable, especially within its eastern secondary section. The sedimentary focus gradually migrated northwestward, remaining within the eastern secondary sub-sag. This phase was predominantly governed by a syndepositional fault, with the eastern secondary branch of the EB-TLFZ continuing its evolution and the commencement of its western secondary branch. Additionally, seven distinct faults were instrumental in shaping the development of the eastern secondary sub-sag, marking the initial formation of the southern secondary sub-sag with the sedimentary focus retained in the eastern section (Figure 9).
During the E2s3 phase, development of the eastern secondary sub-sag halted, while the southern secondary sub-sag’s evolution proceeded. The period was characterized by the continued evolution of two major strike-slip faults and the influence of seven syndepositional normal faults along with the west secondary branch of the EB-TLFZ, steering the development direction. The sedimentary focus shifted northwestward (Figure 9).
The E3s1-2 phase saw the entire region undergoing stable deposition, with the southern secondary sub-sag undergoing adjustments due to two major strike-slip and several normal faults, which also modulated other secondary sub-sags (Figure 9).
Transitioning from the faulting to the depression phase, boundary faults maintained a significant control over sediment deposition, leading to a stabilization of regional depositional thickness and a trend towards uniformity (Figure 9).
In the E3d phase, the southern secondary sub-sag experienced rapid development and substantial N1g strata deposition, with a slight southeastward migration of the sedimentary focus. The deposition was primarily controlled by one syndepositional fault alongside two major strike-slip faults (Figure 9).
Post E3d deposition saw the southeast sag undergoing uplift and erosion, with E2s4, E3s1-2, and N1d strata facing differential erosion - E3s1-2 experiencing the most severe erosion, followed by E2s4, and N1d experiencing the least (Figure 9).
During the depression phase, Neogene deposition was recorded across all secondary sub-sags, with the sedimentary focus in the northern secondary sub-sag. The N1g phase featured the thickest strata centrally and in the northwest, tapering towards the southeast, governed by the continued evolution of two major strike-slip faults and several normal faults, which directed sediment deposition in the southern secondary sub-sag. The northern secondary sub-sag was influenced by a singular normal fault (Figure 9).
The N2mL phase witnessed uniform deposition across the region, modulated by seven normal faults, with the eastern secondary branch of the EB-TLFZ directing the southern secondary sub-sag.
Presently, post- N2mL deposition is characterized by its thickness centrally and in the northwest, thinning towards the southeast, controlled by two major strike-slip faults and adjusted by several normal faults in the eastern secondary sub-sag and other secondary sub-sags. In essence, the MXN sub-sag has undergone a progressive migration of the depositional and subsiding centers westward and northward (Figure 9).
6.2 The control of extensional strike-slip fault system on sub-sag development
During the E2k depositional period, NW-oriented initial oblique extension faults were formed, and the strike-slip faults at this stage exist latently in the basement. During this period, the east, and north secondary sub-sag of the MXN sub-sag were formed. The east secondary sub-sag is mainly controlled by the oblique extension and the pre-existing basement strike-slip fault of the East sub-branch, showing a spindle-shaped distribution; while the north secondary sub-sag is controlled by the EW-extension fault (Figure 10A).
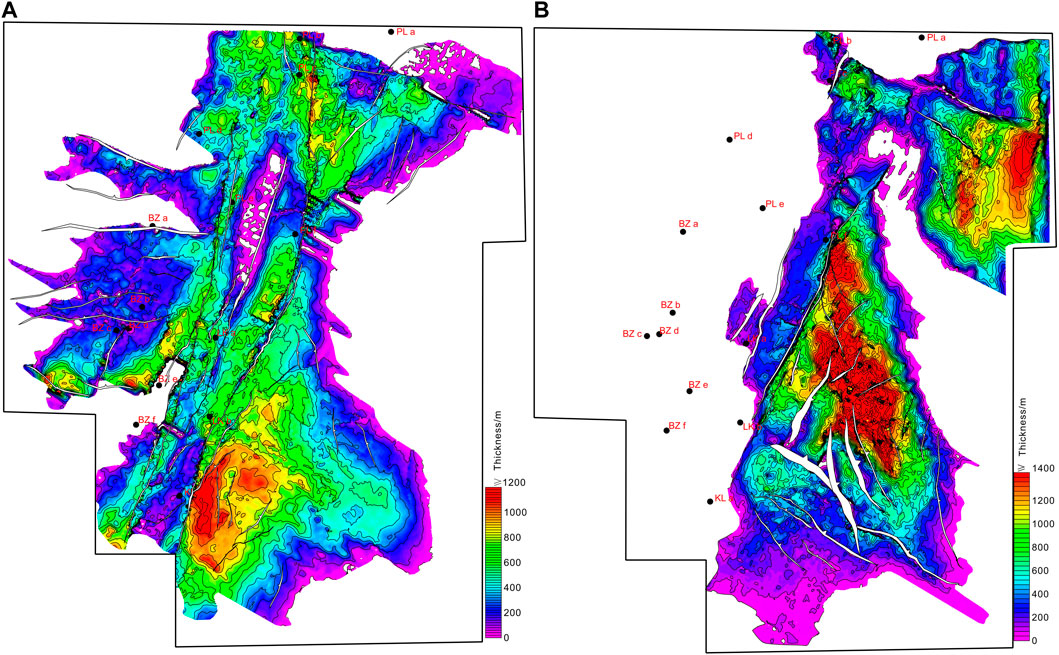
Figure 10. The overlap map of the strata isopach map and the planar distribution of fault systems, indicating the control of fault systems on the sub-sag development (A) overlap map of the E2k isopach map and the planar distribution of fault systems in T7 (the top surface of E2k), (B) overlap map of the E2s4 isopach map and the planar distribution of fault systems in T6 (the top surface of E2s4).
During the E2s4 depositional period, with the increase of extension displacement, the NE-oriented oblique extension faults continued to develop. At the same time, the East sub-branch strike-slip fault became significantly active under the action of this stress field, showing a right-lateral stepover fault combination mode. It generally bends in an arc shape from south to north, and the end of the segmented fault in the north is accompanied by near EW-oriented secondary extension faults. The west sub-branch strike-slip fault was still latent and inactive during this period. Therefore, in addition to the NE-oblique growth fault controlling the sporadic depression of the hanging wall, in the right-lateral strike-slip deformation of the southern bend of the East sub-branch strike-slip fault, a tensional release type negative unit was formed, that is, a “spindle-shaped” depression was formed. And in the joint part of the two faults in the northern part of the East sub-branch strike-slip fault, controlled by the secondary tensional stress field accompanied by strike-slip shear displacement, a “L-shaped” depression was formed, that is, the North secondary sub-sag of MXN (Figure 10B).
During the E2s3 depositional period, the range of fault activity expanded, including the intense activity of the west sub-branch strike-slip fault. But there are still active segments, specifically manifested in the western part of the end of the segmented activity of the strike-slip fault in the HHKD sub-sag, which is accompanied by several relatively large near EW-extension faults, thereby controlling the formation of the “L-shaped” HHKD sub-sag (Figure 11A).
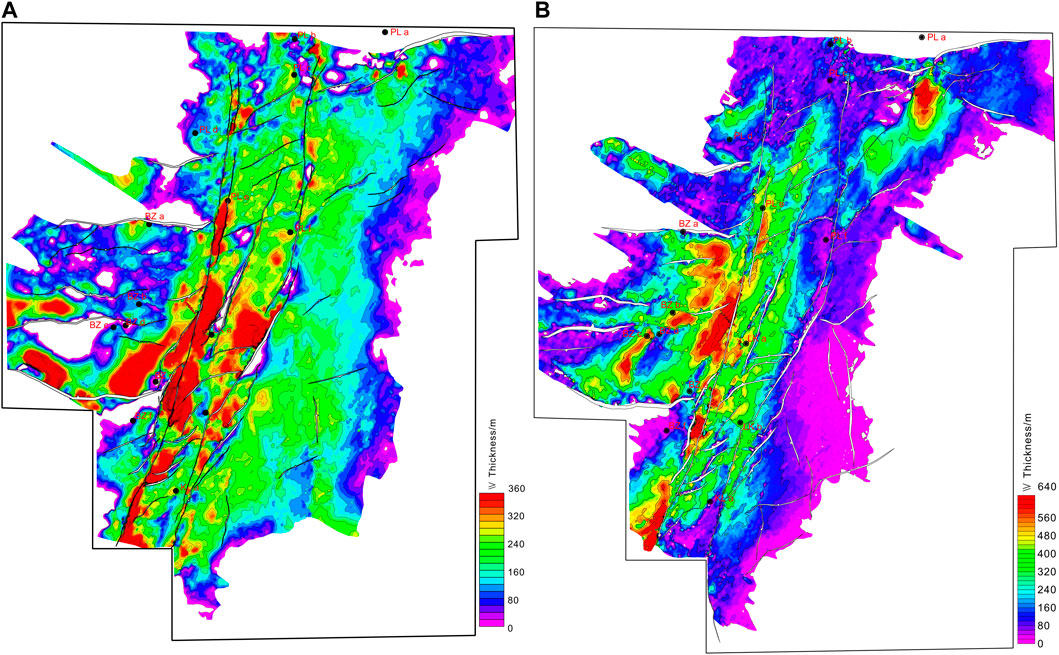
Figure 11. The overlap map of the strata isopach map and the planar distribution of fault systems, indicating the control of fault systems on the sub-sag development (A) overlap map of the E2s3 isopach map and the planar distribution of fault systems in T5 (the top surface of E2s3), (B) overlap map of the E3s1-2 isopach map and the planar distribution of fault systems in T3 (the top surface of E3s1-2).
During the depositional period from E3s1-E3s2 to E3d, the activity of the strike-slip fault dominated. The East and West sub-branch strike-slip faults penetrated the entire area of the MXN sub-sag and even a larger area of the Bohai Sea. The West sub-branch strike-slip fault showed a complete through-type combination, and the East sub-branch strike-slip fault had a higher degree of connection, extended in a through-type and retained the bifurcated fault traces left by the previous left-step combination mode. Therefore, during this period, the “diamond-shaped” depression, namely, the south secondary sub-sag, was mainly formed in the release bending parts of the two strike-slip faults in the middle and south, similar to the strike-slip fault bend basin morphology (Figures 11B, 12).
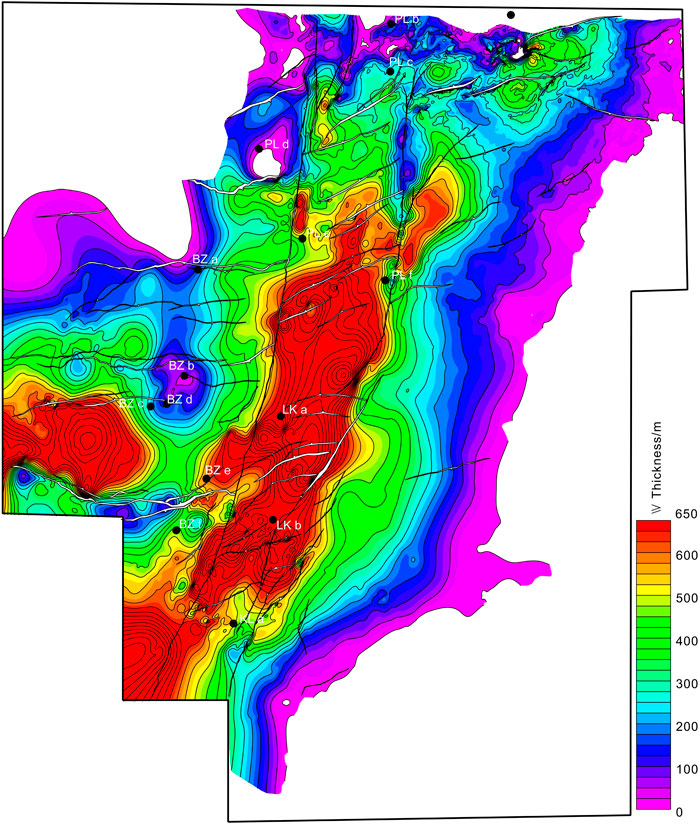
Figure 12. The overlap map of the strata isopach map and the planar distribution of fault systems, indicating the control of fault systems on the sub-sag development. Overlap map of the E3d isopach map and the planar distribution of fault systems in T2 (the top surface of E3d).
Looking at the transformation relationship of different evolution stages of strike-slip faults and oblique extension faults and their control on the characteristics of the depressions formed, in the early stage of basin evolution, that is, the E2k -E2s4 stage and E2s3 stage, mainly formed branch “L-shaped” sub-sag and depressurization bending “spindle-shaped” sub-sag, the depression is mainly controlled by the segmentation expansion mechanism of the strike-slip fault, and the scale of the depression is relatively large. In the middle period of basin evolution, that is, E3s1, E3s2, and E3d stages, the main formation is depressurization “diamond-shaped” depression, and the scale of the depression is also relatively large (Figure 13).
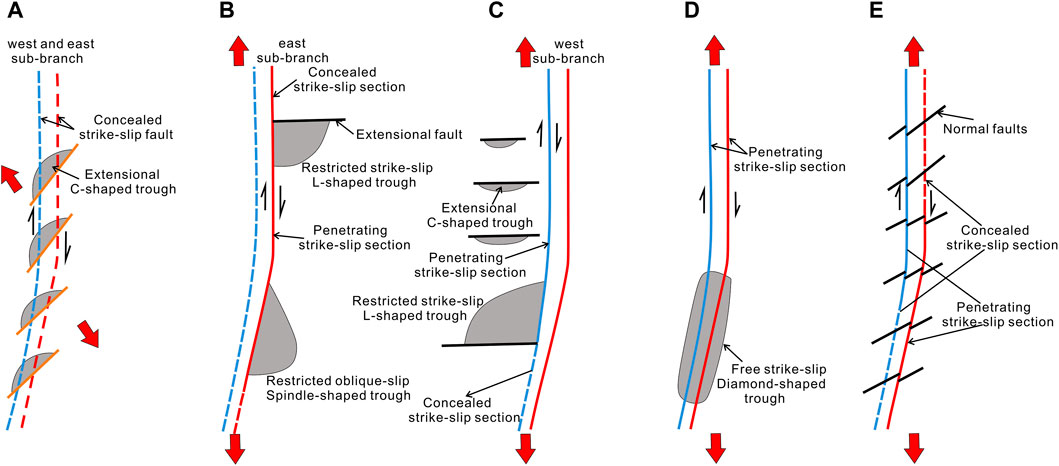
Figure 13. Interaction relationship between strike-slip and extensional fault at different stages and model diagram of sub-sag types in the eastern sub-sag Huanghekou sag and MXN sub-sag. (A) E2k deposition period, concealed strike-slip (B) E3s4 deposition period, segmented strike-slip faulting with terminal extension (C) E2s3 deposition period, segmented penetrative strike-slip faulting with terminal extension (D) E3s1-2 and E3d deposition period, monolithic penetrative strike-slip faulting (E) N1mL deposition period, conversion of concealed strike-slip to penetrative strike-slip faulting.
6.3 The control of extensional strike-slip fault system on the formation and distribution of the traps
Since the Cenozoic era, the southwestern depression of the temple has been profoundly transformed by the forces of early extensional fault stretching, numerous phases of tectonic uplift, and late-stage strike-slip faulting. It has developed a series of strike-slip faults atop a foundation of pre-existing faults from the Paleogene period, resulting in a collection of blocks with compressional and torsional characteristics, alongside groups of fault-related folds and reverse faulted anticlines (Figure 14). Influenced by the differing features of the eastern and western branches of the strike-slip faults, the genesis and types of structural closures within various tectonic zones exhibit considerable variation.
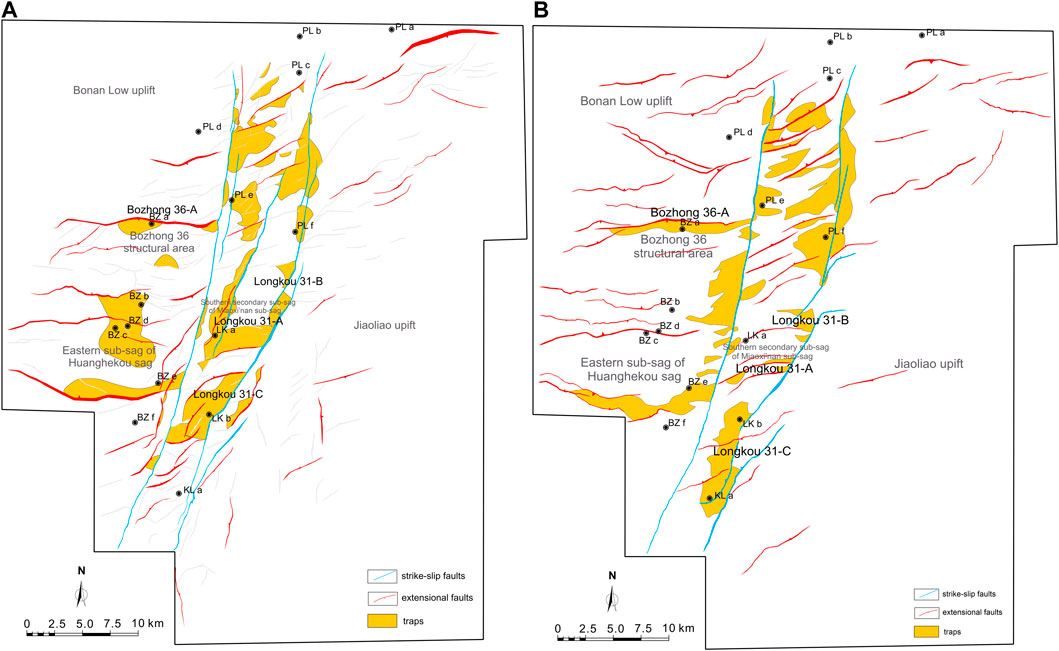
Figure 14. The overlap map of the traps distribution and the planar distribution of fault systems. (A) The overlap map of the traps distribution and the planar distribution of fault systems in top surface of E3s1, indicating the control of fault systems on the trap development in deep layer. (B) The overlap map of the traps distribution and the planar distribution of fault systems in top surface of N1g, indicating the control of fault systems on the trap development in deep layer. (the distribution of traps was revised from Zhao et al., 2020).
Notably, the eastern branch of the strike-slip fault zone is distinguished by a fan-shaped arrangement of faults, with clear signs of early extensional and late compressional-torsional deformations. The strike-slip faults, with their curved segments and “right-lateral left-stepping” overlap zones, have given rise to a series of significant faulted anticlines and faulted block closure groups. For example, the Longkou 31-C structural zone in the southern part of the depression is developed against the backdrop of the southward extension of the Jiao-Liao uplift to the secondary depression, situated in the dual overlap zone of the strike-slip faults. Late-stage strike-slip adjusting faults and configurations have led to the formation of inherited faulted anticline closures at both deep and shallow levels (Figure 14).
The Longkou 31-B structure, located within the depression area, falls within the strike-slip pressure transition zone, forming a “right-lateral left-stepping” block closure adjacent to the eastern branch’s strike-slip fault. The Penglai 25-A structural zone in the north of the eastern branch of the strike-slip fault lies within the overlap zone of the fault, where the Paleogene has experienced multiple episodes of compression, uplift, and erosion. Since the initiation of the new tectonic movement, intense clockwise compressional strike-slip actions have facilitated the emergence of large faulted anticline closures in the area’s shallow layers (Figure 14).
The eastern and western sides of the western branch of the strike-slip fault display pronounced differences in bedding and closure characteristics. For instance, the Penglai 31 structural zone on the western side of the strike-slip fault comprises a block closure group formed through the combined effects of the strike-slip fault and its adjusting faults, presenting a “toothbrush-shaped” configuration. The Penglai 25 west structural zone in the northern part of the strike-slip fault features Paleogene structures with compressional and torsional properties, forming a collection of fault blocks and faulted anticline closures. Additionally, the deep-layer development above the central structural ridge in the southern depression is controlled by the Longkou 31-A block and faulted anticline closures, influenced by early (strike-slip nature) faults. This structural zone is adjacent to the center of the depression, characterized by a thick overlying layer and excellent preservation conditions (Figure 14).
6.4 The relationship between the faults systems and hydrocarbon accumulation
In the Bohai Bay Basin, the fault system is quite complex, making faults the main pathways for oil and gas migration. For a fault to be a favorable source of oil, its active periods must coincide with the hydrocarbon expulsion periods. Otherwise, it is considered a poor oil source fault, such as the nearly east-west trending extensional faults like Bonan No. 1 and Laibei No. 2 faults and the eastern branch strike-slip faults, which are good oil source faults. Most oil and gas reservoirs in the study area were formed when large faults provided pathways for early hydrocarbon source rocks to accumulate within traps, forming large oil and gas fields.
Since faults serve as channels for hydrocarbon movement, their effectiveness in aiding oil and gas accumulation depends on the timing of their activity and hydrocarbon expulsion. Extensional faults create structural traps for hydrocarbons due to the stretching of the earth’s crust, and their formation periods are critical for hydrocarbon migration and trapping. Strike-slip faults allow for horizontal strata displacement and enhance vertical hydrocarbon migration to traps, with secondary faults formed by this movement also acting as migration pathways. Extensional Strike-slip Faults facilitate hydrocarbon migration but require suitable reservoir-cap rock combinations to effectively trap hydrocarbons. In summary, the control of various fault systems on hydrocarbon migration and trapping is a critical factor in the development of oil and gas reservoirs in the Bohai Bay Basin.
6.4.1 The relationship between extensional faults and hydrocarbon accumulation
The relationship between extensional faults and hydrocarbons is exemplified by the BZ34- 9–1 well on the south side of the HHKD sub-sag. The Lai Bei No. 2 is a controlling fault for the sag in the southern sub-sag of the Huanghekou sag. During the early rift stage, thick hydrocarbon source rocks were rapidly deposited, and the E2s3 and E3s1- E3s2 formations provided hydrocarbons for the internal oil and gas reservoirs of the sag. Drilling data show that due to factors such as depositional environment, diagenesis, and sediment composition, good reservoirs developed in the E3s, E3d, N1g, and N1+2m, which can store oil and gas. The main traps in this area are block and anticlinal traps formed by the upper-derived faults of the Lai Bei 2 fault. The BZ34-9 structure has developed a good set of reservoir-cap combinations: braided river delta front sheet sand bodies and lacustrine mudstone of the E3s2- E3s1 Formations; the same for the E3d2L down to the E3d3L; the N1g; and shallow delta sand bodies and thick lacustrine mudstones of the lower part of the N1+2m. During the N1+2m, the E2s3 and E3s1- E3s2 source rocks entered the hydrocarbon expulsion period. During this period, extensional faults developed inheritedly, strike-slip movement was significant, and secondary faults developed, facilitating the connection between source rocks and reservoirs, opening pathways for oil and gas migration. Some hydrocarbons accumulated in situ in the E2s3, while the rest migrated stepwise to the E3s2, E3s1 Formations, E3d, N1g, and N1+2m. From the N1g to the present, the Huanghekou sag and the MXN sub-sag have been in a stage of weak extension and strong strike-slip. Some secondary faults provide favorable channels for the continuous migration of oil and gas, forming oil and gas reservoirs in the shallow N1+2m and N1g. The BZ34-9 structure is located at a structurally high position and is a long-term favorable migration area (Figure 15A).
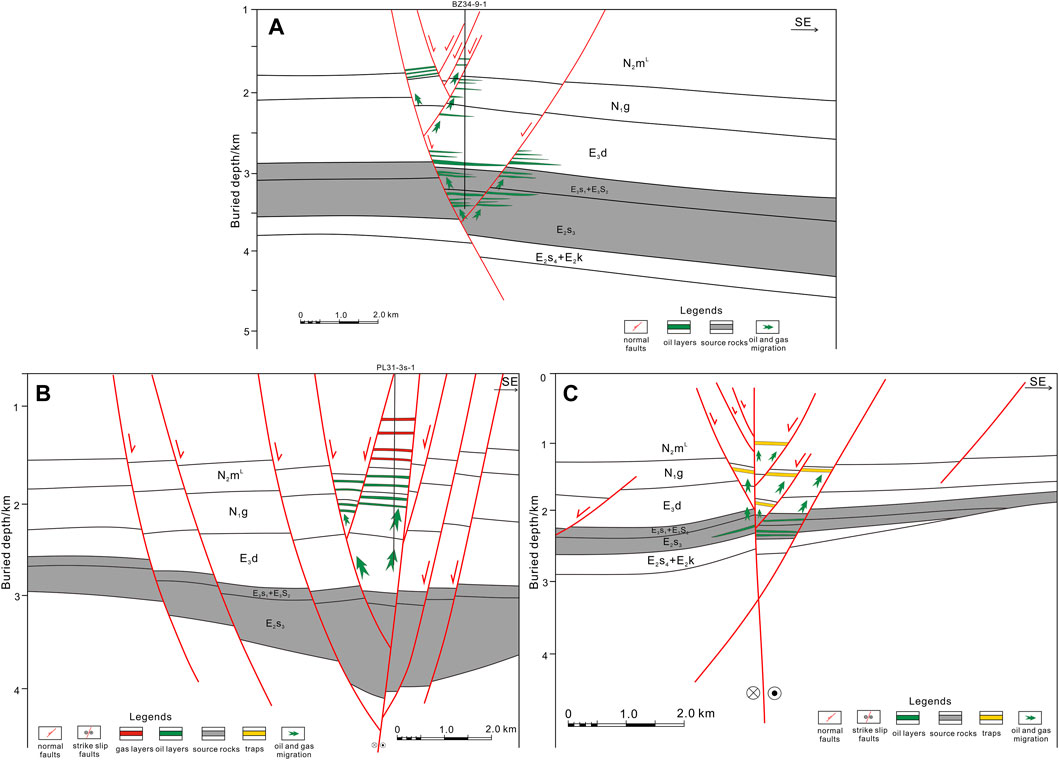
Figure 15. Hydrocarbon accumulation model map. (A) Map of extensional fault-controlled hydrocarbon accumulation model. (B) Map of strike-slip fault-controlled hydrocarbon accumulation model. (C) Map of extensional strike-slip fault-controlled hydrocarbon accumulation model.
6.4.2 The relationship between strike-slip faults and hydrocarbon accumulation
The relationship between strike-slip faults and hydrocarbons is exemplified by the well PL31-3s-1 in the HHKD sub-sag and the western part of the MXN sub-sag, which lies on the eastern branch of the Tan-Lu strike-slip fault zone. Strike-slip and secondary faults are well-developed here, providing vertical migration channels for hydrocarbons and trapping them in shallow block and (semi-) anticlinal traps. Well PL31-3s-1 is a typical oil and gas field in the study area, utilizing the strike-slip fault system for hydrocarbon transport. The main source rocks of the E2s3 and E3s2-E3s1 Formations provide good hydrocarbon sources, with depositional environments such as braided river deltas, meandering river deltas, and lacustrine sediments serving as good reservoirs. The main strike-slip fault cuts through the Cenozoic strata, extending upwards to the Quaternary, as a continuously active fault, providing favorable channels for vertical hydrocarbon migration; shallow secondary faults develop negative flower structures, providing channels for small-scale hydrocarbon accumulation. Late Cenozoic fault activity was weak, and the good sealing properties of the eastern branch can act as effective controlling faults, blocking hydrocarbon migration. Overall, PL31-3S has good source-reservoir-cap combinations, making it a favorable oil and gas area, with the N1+2m and N1g as key levels (Figure 15B).
6.4.3 The relationship between extensional strike-slip faults and hydrocarbon accumulation
The relationship between extensional strike-slip faults and hydrocarbons is exemplified by the extensional strike-slip fault (No.2 sub-branch of EB-TLFZ) on the Inline 5280 seismic line through the eastern branch of the strike-slip fault zone. The line crosses the middle part of the eastern branch strike-slip fault zone, which has high slip intensity, with the HHKD sub-sag to the west and the MXN sub-sag to the east. In the profile, the extensional strike-slip structure of the No. 2 sub-branch of EB-TLFZ is developed, providing a good channel for hydrocarbon migration. Early on, the No. 2 sub-branch of EB-TLFZ was an extensional fault, cutting the lower basement and providing a pathway for the hydrocarbons of the E3s2. During the Cenozoic, strong early extensional activity overlapped with strong strike-slip motion during the E3d depositional period, with secondary faults not only providing pathways for hydrocarbons but also associated structural traps. However, the upper reservoir-cap conditions are poor, so oil and gas reservoirs are mostly formed in the middle to deep parts (Figure 15C).
Superimposed strike-slip faults controlled the migration and accumulation of hydrocarbons in two phases. During the early Eocene, the E3s’s third and E3s1-E3s2 formations were deposited, providing two sets of high-quality source rocks. The source rocks deposited during the late Eocene and Oligocene did not enter thermal evolution stage; the hydrocarbon expulsion period occurred during the E3d and Neogene periods. The enhanced fault activity during the E3d provided good conditions for hydrocarbon migration.
During the depositional period of the E2k-E2s4, the sag-controlling faults and the Tan-Lu fault zone were active, in a strong rift stage. During the deposition of the E2s3, the main faults had inherited extensional activities, with depositional environments dominated by wide semi-deep to deep lake facies, and well-developed source rocks. In the late stage of the E3s1 Formation, the main faults continued to develop, inheriting the semi-deep to deep lake depositional environments and depositing source rocks of the E3s1-E3s2 Formations, providing a fundamental guarantee for the later transportation of hydrocarbons. During the E3d depositional period, the eastern branch of the Tan-Lu strike-slip fault became active, with derived faults starting to develop. On the plane, secondary faults are arranged in echelon or intersect with the main faults in a feather-like pattern, while on the profile, they appear as negative flower structures superimposed on early extensional faults. The development of derived faults forms channels for the later transportation of hydrocarbons and block-type traps.
At the end of the E3d to the N1g, the source rocks reached the hydrocarbon generation threshold and began to expel hydrocarbons. During this period, extensional fault activity was weak, but strike-slip activity was still strong, with secondary faults developing on the plane and obvious negative flower structures on the profile. The fault activity and the expulsion period of the source rocks coincided, allowing for the vertical and horizontal migration of hydrocarbons through faults and sand bodies, forming block and anticlinal traps. The continuous activity of the faults still provided migration channels for hydrocarbons, with sand bodies of the E3s2 serving as lateral migration channels, continuously accumulating hydrocarbons for early oil and gas reservoirs.
6.5 Comparison with similar global basins
6.5.1 Comparison of hydrocarbon accumulation models
(1) Jeanne d’Arc Basin, Canada
The basin is characterized by a combination of extensional and strike-slip faulting which has facilitated the creation of multiple hydrocarbon traps. By comparing the Jeanne d'Arc Basin with the HHKD and MXN sub-sags, the authors can discuss the implications of different types of traps and reservoir qualities influenced by similar tectonic regimes.
(2) Pannonian Basin, Central Europe
The Pannonian Basin is known for its extensional and strike-slip faulting which created accommodation space for hydrocarbon-bearing sediments. Comparing the stratigraphic sequences and the timing of hydrocarbon generation could provide insights into the petroleum system’s efficiency in different tectonic settings.
6.5.2 Comparison of fault dynamics and sedimentation
(1) East African Rift System:
The East African Rift is a contemporary analog for extensional tectonics with strike-slip components. It provides a live model for observing how rift dynamics influence sedimentation and trap formation. Comparing the sedimentary environments can shed light on the role of syn-tectonic sedimentation in hydrocarbon basin evolution.
(2) Dead Sea Transform, Middle East:
The Dead Sea Transform is a prominent strike-slip fault system that has facilitated the formation of pull-apart basins with significant hydrocarbon prospects. The sediment fill and structural traps along the transform can be compared with the HHKD and MXN sub-sags to evaluate the effects of strike-slip faulting on basin morphology and hydrocarbon potential.
7 Conclusion
The HHKD and MXN sub-sags’ extensional faults are predominantly east-west oriented, delineating the structural framework of these depressions.
The main strike-slip faults, trending nearly NNE, significantly influence the basins’ depositional centers.
Key faults (Bonan No. 1, Bonan No. 2, and Laibei No. 2) within the HHKD sub-sag are notably parallel with an east-west orientation.
(1) Main faults characteristics:
① The HHKD and MXN sub-sags feature various fault configurations such as linear, arcuate, en echelon, and feather-like.
② Fault geometries include listric, planar, “Y,” inverted “Y,” multi-tiered “Y,” and flower-like patterns.
③ Strike-slip faults show continuity at depth and form negative and semi-flower structures near the surface.
(2) Tectono-stratigraphic evolution:
① A westward and northward migration of the depocenters and subsidence axes is evident throughout the basins’ evolutionary history.
② The area’s stratigraphic architecture is primarily influenced by extensional and strike-slip fault interactions.
③ Sub-branch faults near the EB-TLFZ significantly impact sedimentary distribution.
(3) Controls of extensional strike-slip fault on sub-sags development:
① During the E2k period, “C-shaped” depressions developed due to small-scale oblique extension.
② The E2s4-E2s3 phase was dominated by “L-shaped” and “spindle-shaped” troughs, with segmented movements along the EB-TLFZ’s sub-branches.
③ The E3s2-1 to E3d period saw “diamond-shaped” depressions formed as a result of intense strike-slip fault activity, particularly around the EB-TLFZ’s sub-branches.
(4) Hydrocarbon exploration implications:
① The area exhibits complex fault interactions, including extensional, strike-slip, and combined dynamics, forming distinct geological traps.
② A series of large fault blocks, faulted anticlinal closures, and overlapping zones create valuable targets for hydrocarbon exploration.
③ Three hydrocarbon accumulation models are proposed: extensional fault-controlled, strike-slip fault-controlled, and combined extensional strike-slip fault-controlled models.
(5) Future research directions or unanswered questions:
① Quantitative analysis of fault slip would improve our understanding of fault evolution and its effects on basin deformation and hydrocarbon migration.
② Employing 3D basin modelling could simulate the basin’s tectono-stratigraphic history, shedding light on potential hydrocarbon reservoirs.
③ An integrated hydrocarbon system analysis is essential for evaluating hydrocarbon potential and identifying prime exploration sites.
④ Research should also assess the effect of sub-branch faults on reservoir quality, particularly how they impact porosity and permeability.
⑤ Lastly, it's important to study how climate change affects sedimentary processes and the stratigraphy of hydrocarbon basins.
Data availability statement
The data analyzed in this study is subject to the following licenses/restrictions: the corporation and Country’s law limits the data. Requests to access these datasets should be directed to xiaofu1241396@163.com.
Author contributions
SF: Conceptualization, Methodology, Writing–original draft. DW: Investigation, Writing–review and editing. HY: Investigation, Resources, Writing–review and editing. LS: Conceptualization, Writing–review and editing. MM: Investigation, Methodology, Writing–review and editing. KW: Investigation, Writing–review and editing.
Funding
The author(s) declare that financial support was received for the research, authorship, and/or publication of this article. The paper was supported By and Open Fund (SZDKFJJ20231008) of Key Laboratory of Petroleum Resources Research, Gansu Province, Northwest Institute of Eco-Environment and Resources, Chinese Academy of Sciences; and Natural Science Basic Research Program of Shaanxi (2023-JC-QN-0287).
Acknowledgments
Special thanks to the experts in CNOOC (China) Limited Tianjin branch for their help and support in this paper. We also extend our gratitude to all the editors and reviewers for their constructive suggestions and comments, contributing to the improvement of our paper.
Conflict of interest
Author HY was employed by the CNOOC (China) Limited Tianjin branch. Author KW was employed by the PetroChina Tarim Oilfield Company.
The remaining authors declare that the research was conducted in the absence of any commercial or financial relationships that could be construed as a potential conflict of interest.
Publisher’s note
All claims expressed in this article are solely those of the authors and do not necessarily represent those of their affiliated organizations, or those of the publisher, the editors and the reviewers. Any product that may be evaluated in this article, or claim that may be made by its manufacturer, is not guaranteed or endorsed by the publisher.
References
Chang, J., Qiu, N., Zhao, X., Shen, F., Liu, N., and Xu, W. (2018). Mesozoic and Cenozoic tectono-thermal reconstruction of the western Bohai Bay Basin (East China) with implications for hydrocarbon generation and migration. J. Asian Earth Sci. 160, 380–395. doi:10.1016/j.jseaes.2017.09.008
Chi, Y. L., and Zhao, W. Z. (2000). Strike-slip deformation during the cenozoic and its influence on hydrocarbon accumulation in the Bohai Bay Basin. Acta Pet. Sin. 21 (2), 14. (in Chinese). doi:10.3321/j.issn:0253-2697.2000.02.003
Deng, S., Zhao, R., Kong, Q., Li, Y., and Li, B. (2022). Two distinct strike-slip fault networks in the Shunbei area and its surroundings, Tarim Basin: hydrocarbon accumulation, distribution, and controlling factors. AAPG Bull. 106 (1), 77–102. doi:10.1306/07202119113
Gong, Z. S., Cai, D. S., and Zhang, G. C. (2007). Dominating action of Tanlu Fault on hydrocarbon accumulation in eastern Bohai Sea area. Acta Pet. Sin. 28 (4), 1–10. doi:10.3321/j.issn:0253-2697.2007.04.001
Hao, F., Zhou, X., Zhu, Y., Bao, X., and Yang, Y. (2009). Charging of the Neogene Penglai 19-3 field, Bohai Bay Basin, China: oil accumulation in a young trap in an active fault zone. AAPG Bull. 93 (2), 155–179. doi:10.1306/09080808092
Hao, F., Zhou, X. H., Zhu, Y. M., and Yang, Y. Y. (2011). Lacustrine source rock deposition in response to co-evolution of environments and organisms controlled by tectonic subsidence and climate, Bohai Bay Basin, China. China Org. geochem. 42 (4), 323–339. doi:10.1016/j.orggeochem.2011.01.010
Hao, F., Zou, H. Y., Gong, Z. S., and Deng, Y. H. (2007). Petroleum migration and accumulation in the Bozhong sub-basin, Bohai Bay basin, China: significance of preferential petroleum migration pathways (PPMP) for the formation of large oilfields in lacustrine fault basins. Mar. Petrol. Geol. 24 (1), 1–13. doi:10.1016/j.marpetgeo.2006.10.007
Henza, A. A., Withjack, M. O., and Schlische, R. W. (2010). Normal-fault development during two phases of noncoaxial extension: an experimental study. J. Struct. Geol. 32 (11), 1656–1667. doi:10.1016/j.jsg.2009.07.007
Hou, G., and Hari, K. R. (2014). Mesozoic-Cenozoic extension of the Bohai Sea: contribution to the destruction of north China craton. Front. Earth Sci. 8, 202–215. doi:10.1007/s11707-014-0413-3
Hou, G. T., Qian, X. L., and Cai, D. S. (2001). The tectonic evolution of Bohai Basin in mesozoic and cenozoic time. Acta Sci. Nat. Univ. Pekin. 37 (6), 845–851. (In Chinese with English Abstract). doi:10.3321/j.issn:0479-8023.2001.06.016
Hsiao, L. Y., Graham, S. A., and Tilander, N. (2004). Seismic reflection imaging of a major strike-slip fault zone in a rift system: Paleogene structure and evolution of the Tan-Lu fault system, Liaodong Bay, Bohai, offshore China. AAPG Bull. 88, 71–97. doi:10.1306/4b1ab41b-fec1-4493-8f923371c08d166a
Hsiao, L. Y., Graham, S. A., and Tilander, N. (2010). Stratigraphy and sedimentation in a rift basin modified by synchronous strike-slip deformation: southern Xialiao basin, Bohai, offshore China. Basin Res. 22 (1), 61–78. doi:10.1111/j.1365-2117.2009.00449.x
Huang, Y., Guan, D. Y., Zhao, G. X., Zhu, Y. C., and Ren, Y. P. (2018). Geological characteristics of tight oil in the Shahejie Formation in northeast subsag of Laizhou Bay Sag and its formation condition. Petroleum Geol. Recovery Effic. 25 (1), 22–29. (in Chinese with English abstract). doi:10.13673/j.cnki.cn37-1359/te.2018.01.004
Jiang, F. J., Pang, X. Q., Bai, J., Zhou, X. H., Li, J. P., and Guo, Y. H. (2016). Comprehensive assessment of source rocks in the Bohai Sea area, eastern China. AAPG Bull. 100 (6), 969–1002. doi:10.1306/02101613092
Jin, F., Huang, J., Pu, X., Ma, C., Fu, L., Leng, C., et al. (2020). Characteristics of the Cretaceous magmatism in Huanghua depression and their relationships with hydrocarbon enrichment. J. Earth Sci. 31, 1273–1292. doi:10.1007/s12583-020-1308-8
Lan, X., and Liu, H. (2022). The geochemical characteristics of the Paleogene lacustrine source rock and Cenozoic oil in the eastern Huanghekou Sag, Bohai Bay Basin, China: an oil–source rock correlation. J. Petrol. Sci. Eng. 214, 110434. doi:10.1016/j.petrol.2022.110434
Li, K., Dong, R., Xu, G. S., Liang, Q., and Yang, H. F. (2021). Development and evolutionary model of the Cenozoic strike-slip-extensional composite fault system in Laizhouwan Sag. J. Chengdu Univ. Technol. Sci. Technol. Ed. 48 (1), 11. (In Chinese with English Abstract). doi:10.3969/j.issn.1671-9727.2021.01.02
Li, F. Y., Yang, H. C., Yang, D. S., Guo, S., Sun, R., Ji, M., et al. (2020a). The extension-strike-slip composite fault belts in central Changchang Sag of Qiongdongnan Basin and their significance for oil and gas exploration. Mar. Oil Gas Geol. 25 (3), 6. (In Chinese with English Abstract). doi:10.3969/j.issn.1672-9854.2020.03.008
Li, S. W., Huang, Y. L., Feng, Y. H., Guo, Y. M., Zhang, B., and Wang, P. J. (2020b). Cenozoic extensional-strike-slip tectonics and fracture characteristics in the eastern part of the Liaohe Basin. Chin. J. Geophys. 63 (2), 612–626.
Li, S. Z., Suo, Y. H., Santosh, M., Dai, L. M., Liu, X., Yu, S., et al. (2013). Mesozoic to cenozoic intracontinental deformation and dynamics of the north China craton. Geol. J. 48 (5), 543–560. doi:10.1002/gj.2500
Li, Y., Pu, R., Zhang, G., and Fan, X. (2023). Differences of polygonal faults with irregularly polygonal geometries: a case study from the changchang sag of Qiongdongnan Basin, northern south China sea. J. Ocean Univ. China 22 (1), 119–135. doi:10.1007/s11802-023-5285-2
Li, Z., Zuo, Y., Qiu, N., and Gao, J. (2017). Meso–cenozoic lithospheric thermal structure in the Bohai Bay Basin, eastern north China craton. Geosci. Front. 8 (5), 977–987. doi:10.1016/j.gsf.2016.09.003
Liu, C. C., Dai, F. G., Yang, J., and Yang, K. C., 2010. Seismic interpretation of Eogene-Neogene geological structures and tectonic styles in the sea area of Bohai Bay basin. Chin. Geol. 37 (6), 1545–1558. (in Chinese with English abstract). doi:10.3969/j.issn.1000-3657.2010.06.001
Liu, H., Xia, Q. L., Somerville, I. D., Wang, Y., Zhou, X. H., Niu, C. M., et al. (2015). Paleogene of the Huanghekou Sag in the Bohai Bay Basin, NE China: deposition-erosion response to a slope break system of rift lacustrine basins. Geol. J. 50 (1), 71–92. doi:10.1002/gj.2527
Liu, H., Xia, Q. L., and Zhou, X. H. (2018). Geologic-seismic models, prediction of shallow-water lacustrine delta sandbody and hydrocarbon potential in the Late Miocene, Huanghekou Sag, Bohai Bay Basin, northern China. J. Palaeogeogr. 7 (1), 66–87. doi:10.1016/j.jop.2017.11.001
Liu, L., Chen, H., Wang, J., Zhong, Y., Du, X., Gan, X., et al. (2019). Geomorphological evolution and sediment dispersal processes in strike-slip and extensional composite basins: a case study in the Liaodong Bay Depression, Bohai Bay Basin, China. China. Mar. Petrol. Geol. 110, 73–90. doi:10.1016/j.marpetgeo.2019.07.023
Lu, K. Z., Qi, J. F., and Dai, J. S. (1997). Tectonic model of cenozoic petroliferous basin Bohai Bay Province. Beijing: Geological Publishing House. (in Chinese).
Ma, D. B., Wang, Z. C., Duan, S. F., Gao, J. R., Jiang, Q. C., Jiang, H., et al. (2018). Strike-slip faults and their significance for hydrocarbon accumulation in Gaoshiti–Moxi area, Sichuan Basin, SW China. Petroleum Explor. Dev. 45 (5), 851–861. doi:10.1016/s1876-3804(18)30088-0
Qi, J. F. (2004). Two tectonic systems in the Cenozoic Bohai Bay basin and their genetic interpretation. Geol. China 31 (01), 15–22. (in Chinese with English abstract).
Qi, J. F., and Yang, Q. (2010). Cenozoic structural deformation and dynamic processes of the Bohai Bay basin province, China. China. Mar. Petrol. Geol. 27 (4), 757–771. doi:10.1016/j.marpetgeo.2009.08.012
Ren, J. Y., Lei, C., Wang, S., Li, T., and Zhang, X. L. (2011). Tectonic stratigraphic framework of the Yinggehai-Qiongdongnan Basins and its implication for tectonics province division in South China Sea. Chin. J. Geophys. 54 (6), 1124–1137. doi:10.3969/j.issn.0001-5733.2011.12.028
Schlische, R. W., and Withjack, M. O. (2009). Origin of fault domains and fault-domain boundaries (transfer zones and accommodation zones) in extensional provinces: result of random nucleation and self-organized fault growth. J. Struct. Geol. 31 (9), 910–925. doi:10.1016/j.jsg.2008.09.005
Shi, H. S., Wang, Q. B., Wang, J., Liu, X. J., Feng, C., Hao, Y. W., et al. (2019). Discovery and exploration significance of large condensate gas fields in BZ19-6 structure in deep Bozhong sag. China Petrol. Explor 24 (1), 36–45. (in Chinese with English abstract). doi:10.3969/j.issn.1672-7703.2019.01.005
Song, G., Wang, H., Xu, M., Xu, J., and Sang, G. (2019). Sedimentary facies, sequence stratigraphic patterns in pre-cenozoic inland compressional basin: example from early yanshanian succession of eastern yihezhuang Salient, jiyang depression, Bohai Bay Basin, China. J. Earth Sci. 30, 194–205. doi:10.1007/s12583-018-0867-4
Sun, G. Y. (2017). Study on hydrocarbon source rocks and resource evaluation in the Miaoxinan sag. Beijing: China University of Geosciences. (Master thesis in Chinese with English Abstract).
Sun, Z., Zhu, H., Xu, C., Yang, X., Du, X., Wang, Q., et al. 2017. Pore fluid evolution influenced by volcanic activities and related diagenetic processes in a rift basin: evidence from the Paleogene medium-deep reservoirs of Huanghekou Sag, Bohai Bay Basin, China. Geofluids, 2017.
Teng, C., Hao, F., Zou, H., Zhou, X., and Xu, C. (2016). Tan-Lu fault system and its significance in oil accumulation in the central Liaodong Bay subbasin, Bohai Bay Basin, China. AAPG Bull. 100 (2), 289–314. doi:10.1306/10221515011
Tong, H., Yu, F., and Geng, C. (2008). Characteristics and evolution of strike-slip tectonics of the Liaohe western sag, Bohai Bay Basin. Petroleum Sci. 5, 223–229. doi:10.1007/s12182-008-0034-0
Tong, K. J., Zhao, C. M., Lu, Z. B., Zhang, Y. C., Zheng, H., Xu, S. N., et al. (2012). Reservoir evaluation and fracture chracterization of the metamorphic buried hill reservoir in Bohai Bay Basin. Petrol. explor. Dev. 39 (1), 62–69. doi:10.1016/s1876-3804(12)60015-9
Wang, Z., Gao, Z., Fan, T., Shang, Y., Qi, L., and Yun, L. (2020). Structural characterization and hydrocarbon prediction for the SB5M strike-slip fault zone in the Shuntuo Low Uplift, Tarim Basin. Mar. Petrol. Geol. 117, 104418. doi:10.1016/j.marpetgeo.2020.104418
Xu, J. Y., Ben-Avraham, Z., Kelty, T., and Yu, H. S. (2014). Origin of marginal basins of the NW Pacific and their plate tectonic reconstructions. Earth-Sci. Rev. 130, 154–196. doi:10.1016/j.earscirev.2013.10.002
Yang, G., Yin, H., Gan, J., Wang, W., Zhu, J., Jia, D., et al. (2022). Explaining structural difference between the eastern and western zones of the Qiongdongnan Basin, northern South China Sea: insights from scaled physical models. Tectonics 41 (2), e2021TC006899. doi:10.1029/2021tc006899
Ye, Z. Q., Zhu, H. T., Du, X. F., Yang, X. H., Yao, T. T., and Li, S. (2020). Sedimentary characteristics and model of mixed siliciclastic-carbonates of member 1 of Paleogene Shahejie formation, huanghekou sag, Bohai Bay Basin. Earth Sci. 45 (10), 3731–3745. (in Chinese with English abstract). doi:10.3799/dqkx.2020.224
Yin, A. (2010). Cenozoic tectonic evolution of Asia: a preliminary synthesis. Tectonophysics 488, 293–325. doi:10.1016/j.tecto.2009.06.002
Yu, Y., Zhou, X., Xu, C., Wu, K., Lv, D., Liu, Y., et al. (2020). Architecture and evolution of the Cenozoic offshore Bohai Bay basin, eastern China. J. Asian Earth Sci. 192, 104272. doi:10.1016/j.jseaes.2020.104272
Zeng, Z., Zhu, H., Zeng, H., Yang, X., and Xu, C. (2022). Seismic sedimentology analysis of fluvial-deltaic systems in a complex strike-slip fault zone, Bohai Bay Basin, China: implications for reservoir prediction. J. Petrol. Sci. Eng. 208, 109290. doi:10.1016/j.petrol.2021.109290
Zhao, Y., Yang, H. F., Huang, Z., Zhao, D. J., and Wang, H. (2020). Strike-slip structural characteristics and its controlling effect on hydrocarbon accumulation in Miaoxinan Sag, Bohai Sea. Petroleum Geol. Recovery Effic. 27 (4), 35–44. (in Chinese with English abstract). doi:10.13673/j.cnki.cn37-1359/te.2020.04.004
Zhou, X. H., Yu, Y. X., Tang, L. J., Lv, D. Y., and Wang, Y. B. (2010). Cenozoic offshore basin architecture and division of structural elements in Bohai sea. China Offshore Oil Gas 22 (5), 285–289. (in Chinese with English abstract).
Zong, Y., Liang, J. S., and Liu, L. F. (2012). Structural characteristics of Miaoxi sag. Lithol. Reserv. 24 (1), 36–39. (in Chinese with English abstract). doi:10.3969/j.issn.1673-8926.2012.01.007
Zuo, Y., Qiu, N., Zhang, Y., Li, C., Li, J., Guo, Y., et al. (2011). Geothermal regime and hydrocarbon kitchen evolution of the offshore Bohai Bay Basin, North China. AAPG Bull. 95 (5), 749–769. doi:10.1306/09271010079
Glossary
Keywords: extensional strike-slip fault system, Tan-Lu fault zone, tectonic evolution stage, structural pattern, the Huanghekoudong and Miaoxi’nan sub-sags, hydrocarbon accumulation
Citation: Fu S, Wang D, Yang H, Song L, Ma M and Wang K (2024) Influence of extensional strike-slip fault systems on hydrocarbon accumulation: case studies from Huanghekoudong and Miaoxi’nan sub-sags. Front. Earth Sci. 12:1407074. doi: 10.3389/feart.2024.1407074
Received: 26 March 2024; Accepted: 11 April 2024;
Published: 16 May 2024.
Edited by:
Xixin Wang, Yangtze University, ChinaReviewed by:
Chaojin Lu, University of Miami, United StatesBingshan Ma, Southwest Petroleum University, China
Shiqiang Xia, North China University of Science and Technology, China
Copyright © 2024 Fu, Wang, Yang, Song, Ma and Wang. This is an open-access article distributed under the terms of the Creative Commons Attribution License (CC BY). The use, distribution or reproduction in other forums is permitted, provided the original author(s) and the copyright owner(s) are credited and that the original publication in this journal is cited, in accordance with accepted academic practice. No use, distribution or reproduction is permitted which does not comply with these terms.
*Correspondence: Sheng Fu, xiaofu1241396@163.com; Lijun Song, Ljsong@xsyu.edu.cn