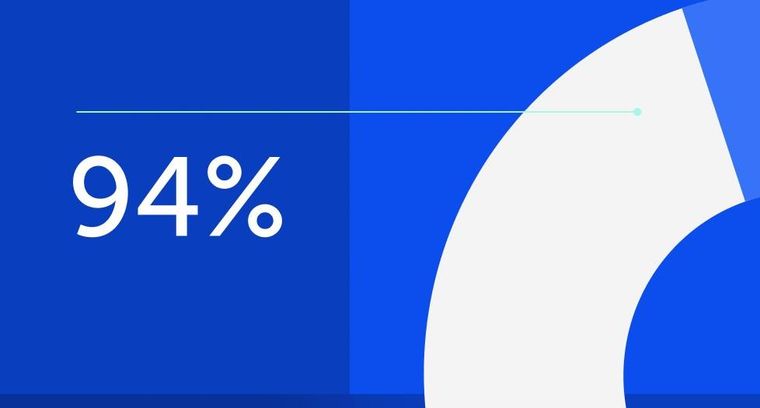
94% of researchers rate our articles as excellent or good
Learn more about the work of our research integrity team to safeguard the quality of each article we publish.
Find out more
ORIGINAL RESEARCH article
Front. Earth Sci., 03 June 2024
Sec. Geohazards and Georisks
Volume 12 - 2024 | https://doi.org/10.3389/feart.2024.1403902
Shale formations often contain a high proportion of clay minerals, which, upon contact with drilling fluid, undergo hydration expansion. This leads to wellbore instability, a problem that poses significant challenges globally. This study aims to investigate the variation of mechanical properties of shale with respect to hydration time. We employ an empirical model that relates shale strength parameters to the time of drilling through geological formations. Additionally, we consider both shear failure along the wellbore boundary and shear sliding along bedding planes in the analysis. We establish a predictive model for wellbore instability in shale formations. The model quantitatively analyzes the variation of wellbore collapse pressure with drilling time. The research findings indicate that, when the influence of bedding is considered, both the wellbore collapse pressure and the optimal well trajectory undergo significant changes, in addition, for some wellbore trajectories, the collapse pressure can increase by more than 30%. Therefore, it is essential to account for the influence of bedding in wellbore stability analysis in shale formations. As the bedding dip angle changes, both the numerical values and distribution range of wellbore collapse pressure and the optimal well trajectory change noticeably. Changes in bedding dip direction, however, do not affect the numerical values of collapse pressure but do influence the distribution region of the optimal well trajectory. Thus, in wellbore trajectory design within shale formations, it is crucial to determine the orientation of bedding and adjust the well trajectory accordingly to enhance wellbore stability. Furthermore, shale hydration does not impact the optimal well trajectory for a block, but with prolonged hydration, the minimum drilling fluid density required to maintain wellbore stability gradually increases. This suggests that hydration intensifies the weakening effect on bedding plane strength. The research results are helpful to understand the effect of hydration on shale wellbore stability and ensure shale wellbore stability during drilling cycle.
Maintaining borehole stability is a crucial issue in the oil and gas industry. The economic losses caused by borehole instability exceed $1 billion annually, with the lost time accounting for over 40% of all non-productive time in drilling operations. It is reported that shale formations account for 75% of drilled formations in the oil and gas industry, and 90% of borehole stability issues occur in shale formations (Zhang M. et al., 2023; Wang Z. M. et al., 2023; Wang, 2023; Huang et al., 2023; Fan et al., 2019).
The hydration process of shale is complex, involving multiple factors that need to be considered comprehensively. Previous studies have achieved significant progress in this area. Tang et al. (2022) established a collapse pressure calculation model considering the structural conditions of formations, wellbore trajectory, and multiple weak planes in coal seams based on the weak plane criterion. They investigated the main controlling factors and influencing patterns of collapse pressure due to multiple weak planes in coal seams. The study found that an increase in the dip of bedding mainly affects the orientation of borehole collapse, with minimal influence on the numerical value. The research results can prevent collapse of coal seam boreholes (Zhang et al., 2021a). Zhang et al. (2017) proposed a strength criterion for laminated shale considering the dual effects of anisotropy and hydration. This criterion can calculate the laminated shale strength under different dip angles, confining pressures, and water contents with minimal experimental data. The experimental testing method is simple, and the predictive results are reliable. The authors also established a wellbore stability model coupled with horizontal wellbore strengthening and found significant anisotropy in shale strength. When the wellbore inclination angle approaches 45°, the wellbore is most prone to instability (Zhang et al., 2021b). Liu et al. (2023) utilized the principle of linear superposition combined with the effects of formation seepage, pore pressure changes, and temperature field changes induced by thermal stress to establish a thermo-poro-elastic model. They developed a multi-porous elastic model and a dynamic temperature field coupling wellbore stability analysis method. The research results indicate that as circulation time increases, the excessive temperature difference between the wellbore and formation leads to an increase in superimposed thermal stress, resulting in a greater difference in the rock’s principal stresses and increasing instability (Zhang P. S. et al., 2023). Huang et al. (2023) established a transient analytical model for seepage, temperature, and stress field variations under overbalanced and underbalanced drilling conditions. This model can analyze the effects of critical drilling parameters on wellbore stability (Zhang et al., 2017; Liu et al., 2023). Qiu et al. (2023a) established a multi-field coupled finite element model for wellbore stability of underbalanced horizontal wells based on the theory of fluid-solid coupling. They analyzed the evolution law of wellbore stability in unbalanced horizontal wells with muddy sand reservoirs due to rock strength deterioration caused by rock water absorption diffusion. The study found that although wellbore pressure is the dominant factor influencing instability, with time, the impact of rock strength deterioration caused by muddy water hydration on the stability of underbalanced horizontal wellbore gradually increases, and the stability of overbalanced conditions is superior to that of near-balanced conditions, while the stability of underbalanced conditions is the worst but still meets the requirements for maintaining wellbore stability= (Tang et al., 2022).
The aforementioned studies primarily focus on research regarding wellbore stability models, considering factors such as stress, rock strength, and fluid-solid coupling, predicting the safe drilling fluid density window and collapse cycle of shale formations. Some scholars have also conducted extensive research on the inhibitory, plugging, and reinforcement effects of drilling fluids on shale formations. Wang et al. conducted relevant experiments using X-ray diffraction, electron microscopy scanning, CT scanning, and triaxial mechanics to determine coal rock mineral composition, crack width distribution, strength characteristics, etc. They designed drilling fluid formulations from three aspects: “sealing against collapse, cleaning the wellbore, and lubrication to prevent sticking,” achieving stable wellbore drilling in long horizontal sections (Zhong et al., 2023). Addressing the control difficulties of drilling fluid leakage in fractured reservoirs, Xu et al. (2020) clarified the multi-scale structure of fracture sealing layers, constructed a destabilization model of fracture sealing layers under high-temperature, high-pressure, and high-ground stress environments in deep fractured reservoirs, and revealed the destabilization mechanism of sealing layer structures (Qiu et al., 2023b). Wang (2023) evaluated the high- and low-pressure sealing performance of drilling fluid systems using a permeability plugging tester and assessed the pollution resistance of drilling fluid systems using medium pressure and high-temperature and pressure filtration loss rates. They also evaluated the reservoir protection performance of drilling fluids using the recovered value of core permeability (Li et al., 2012; Fan et al., 2019; Chen et al., 2022; Ding et al., 2022; Zhang M. et al., 2023; Li et al., 2023). The study results show that the force chain network formed by contact between plugging materials determines the macroscopic pressure stability of the sealing layer. The micro-scale strength of force chains depends on the key performance parameters of plugging materials such as microscale permeability, particle size distribution, fiber aspect ratio, friction coefficient, compressive strength, high-temperature resistance, and solubility (Liu and Li, 2012; Lin et al., 2013; Teng et al., 2017; Xu et al., 2020; Tang et al., 2021; Ma et al., 2022; Zhou et al., 2022). Tang (2011) proposed that the essence of inhibiting shale hydration is to control hydration stress, hinder fluid invasion, and pressure transmission, which can prevent/control shale hydration and promote stable wellbore in complex shale formations (Huang and Zhang, 1998; Lu et al., 1999; Tang, 2011; Hang et al., 2014).
Previous research has improved the understanding of the physicochemical properties of shale and characteristics of borehole instability, achieving good application effects (Ma, 2015; Wen, 2015; Zhang et al., 2017; Meng et al., 2019; Gao and Zhang, 2022; Zhengzheng et al., 2024a; Zhengzheng et al., 2024b; Zhang et al., 2024; Zhang et al., 2024). However, the problem of borehole instability in shale formations has not been completely solved, especially the evident delayed effect and stable time window of borehole instability in shale formations (Wang F. et al., 2023). Research in this aspect is still insufficient. This study considers the time effect of shale hydration, establishes a model for shale strength weakening after immersion in water-based drilling fluid, combines it with a wellbore stress model, and investigates the variation law of borehole collapse pressure with drilling cycles while considering weak plane characteristics such as bedding and the influence of wellbore trajectory. The aim is to predict the safe cycle of shale wellbore stability, prevent occurrences of blockage and pump-out situations, and ensure safe and efficient well construction.
To obtain the wellbore stress, it is necessary to establish the conversion relationship between the geodetic coordinates and the stress coordinates, the wellbore orthogonal polar coordinates, and the layer attitude coordinates. The relationships between the coordinates are illustrated in Figures 1, 2. In these figures, αs represents the angle between the horizontal maximum principal stress and the north direction, while βs represents the angle between the vertical stress and the direction of the axis Ze. The angle between the wellbore axis and the axis Ze direction is the wellbore inclination angle βb. The angle between the projection of the wellbore lowest point on the horizontal plane and the north direction is the wellbore azimuth angle αb. Additionally, αbp+π/2 represents the strike of the bedding plane, and βbp is the angle between the normal of the bedding plane and the plumb direction.
Figure 1. Relationship between geodetic coordinates, geo-stress coordinates and Borehole column coordinate system and polar coordinate system conversion.
Based on the in situ stress coordinates, the distribution of in situ stress around the well in the Cartesian coordinate system can be obtained through a series of coordinate transformations, which can be expressed as follows (Huang and Zhang, 1998; Tang, 2011; Li et al., 2012; Wen, 2015; Ma, 2015; Huang et al., 2023; Qiu et al., 2023a),
After obtaining the distribution tensor of the in situ stress in the wellbore orthogonal coordinate system, the wellbore stress components caused by the pressure of the drilling fluid column inside the well and the stress components caused by the hydration between the drilling fluid and the formation are superimposed. Considering the Biot’s effective stress theory, the equation for the wellbore stress in the well column coordinate system is derived as Eq. 2 (Ma et al., 2016; Liu et al., 2020; Aslannezhad et al., 2020; Qiu et al., 2023; Yang et al., 2023a; Ma et al., 2024a),
In which, σθ, σr, σz, τrθ, τrz, and τθz represent the components of the wellbore stress in the well column coordinate system, MPa. rw is the radius of the wellbore, r is the distance from any point around the well to the wellbore axis, m. Pp is the formation pressure, MPa. Α is the Biot effective stress coefficient, dimensionless. θ is the angle around the wellbore, measured counterclockwise from the axis Xb, °; Im is the membrane efficiency, T is the temperature of the shale formation, V is the partial molar volume of water, R is the gas constant, αwm is the activity of drilling mud, and αwsh is the activity of shale.
To facilitate calculations, most strength criteria typically use the form of principal stresses. Therefore, it is necessary to convert the wellbore stress into the form of principal stresses. By substituting the components of wellbore stress, as shown in Eq. 2, into Eq. 3, we can obtain the values of principal stresses around the wellbore, as shown in Eq. 3 (Ding et al., 2022; Ma et al., 2024b),
Based on the experimental research in this paper, it is known that the Jaeger single weak plane criterion, which is expressed as Eq. 4, can better reveal the strength of different shale layers with varying bedding angles (Ma, 2015; Ma and Chen, 2015; Yang et al., 2023b; Yang et al., 2023c; Yu et al., 2022). This study adopts this theory as the criterion for determining the lower limit of the wellbore safety density window.
In Eq. 4, co and φo represent the cohesion and internal friction angle of the shale matrix, measured in MPa and degrees, respectively. cbp and φbp represent the cohesion and internal friction angle of the shale bedding plane, measured in MPa and degrees, respectively. β denotes the angle between the normal of the bedding plane and the applied load, measured in degrees. β1 and β2 represent the critical angles at which the rock fails along the bedding plane, measured in degrees.
During the drilling process, shale formations often exhibit a series of issues such as mud balling, degradation of drilling fluid properties, bit balling, periodic collapses of the wellbore, and enlargement of the wellbore diameter. While increasing drilling fluid density can effectively prevent wellbore collapse, frequent occurrences of complex drilling accidents arise over time due to the weakening of mechanical parameters such as strength in shale caused by hydration reactions.
Extensive experimental studies have led researchers like Huang et al. (2023) to approximate the relationship between shale strength parameters and moisture content using a linear model. Meanwhile, the relationship between elastic modulus and moisture content follows an exponential pattern. Additionally, researchers like Ma (2015) had utilized conventional triaxial mechanical test systems to assess the strength of shale bodies under different soaking periods and direct shear test systems to evaluate the strength of shale bedding planes under similar conditions. Analysis of experimental data indicates that the weakening trend of shale strength with increasing moisture content conforms to the Logistic model, as shown in Eqs 5, 6 (Ma, 2015),
In which, Co represents the cohesion of the shale matrix, measured in MPa; φo represents the internal friction angle of the shale matrix, measured in degrees; Cw represents the cohesion of the shale bedding plane, measured in MPa; φw represents the internal friction angle of the shale bedding plane, measured in degrees; t represents the hydration time of the shale, measured in days.
According to the weakening model of shale strength with hydration time, the weakening trend of shale body and bedding plane strength with hydration time is illustrated in Figure 3. It can be observed that with increasing hydration time, the strength of shale initially decreases rapidly. However, after 6 days, the decreasing trend of cohesion and internal friction angle slows down, indicating a weakening effect of hydration on strength that diminishes over time.
Based on the study, the parameters of the research block are as follows, the maximum horizontal stress σH is 38.5479MPa, the minimum horizontal stress is 31.7977MPa, the vertical stress is 43.1887MPa, and the formation pressure is 6.0318 MPa. The direction of the minimum horizontal stress is N0°E, the Poisson’s ratio is 0.25, and the parameters of the shale matrix and bedding plane strength, as well as their variation with hydration time, are as shown in Section Three. The inputting parameters and interested depth are summarized in Table 1, which are obtained from the interpretation of well logging data and field experiment results. By inputting these parameters into the model established in this paper, the quantitative analysis will examine the influence of shale bedding, wellbore trajectory, and formation drilling time on wellbore collapse pressure.
Shale formations typically exhibit a set of parallel bedding planes, leading to significant anisotropy in their strength. The failure of wellbore confinement pressure will result in shear failure along the matrix and shear sliding failure along the bedding planes. The influence of bedding planes on the wellbore collapse pressure polar plot is depicted in Figure 4. In the figure, the polar plot transitions from blue to purple, indicating a gradual increase in collapse pressure. The circumferential direction represents the wellbore azimuth angle ranging from 0° to 360°, where 0° denotes the direction of minimum horizontal stress and 90° represents the direction of maximum horizontal stress. The radial direction indicates the wellbore inclination angle, where 0° represents vertical wells and 90° represents horizontal wells.
Figure 4A illustrates the polar plot of wellbore collapse pressure without considering bedding planes. The range of collapse pressure varies from 0.85 to 1.1 g/cm3 and symmetrically distributes along the directions of minimum horizontal stress and maximum horizontal stress. Lower collapse pressure is observed when drilling in the direction of minimum horizontal stress, indicating better wellbore stability. Additionally, within the range of 35°–45° wellbore inclination, the collapse pressure reaches its minimum value along the direction of minimum horizontal stress. Taking into account the influence of bedding planes, assuming the development of horizontal bedding in the formation, Figure 4B depicts the polar plot of wellbore collapse pressure. The polar plot still symmetrically distributes along the directions of minimum horizontal stress and maximum horizontal stress, but the collapse pressure significantly increases, ranging from 1 to 2 g/cm3. Wellbores with inclination angles below 30° exhibit the lowest collapse pressure, indicating the optimal drilling trajectory. It is evident that considering the effect of bedding planes leads to significant changes in collapse pressure and the optimal wellbore trajectory. Therefore, the influence of bedding planes should be considered in the stability analysis of shale formations.
After establishing that bedding planes have a significant impact on wellbore collapse pressure in shale formations, this study investigated the influence of bedding plane attitudes on wellbore collapse pressure. When the bedding plane strikes at 0°, the polar plots of wellbore collapse pressure under different bedding plane dip angles are shown in Figure 5. For a bedding plane dip angle of 0°, the range of wellbore collapse pressure under any wellbore trajectory varies from 1 to 2 g/cm3. When the bedding plane dip angle is 30°, the range of wellbore collapse pressure varies from 1 to 2.2 g/cm3. With a bedding plane dip angle of 40°, the range of wellbore collapse pressure varies from 0.8 to 2.4 g/cm3. At a bedding plane dip angle of 90°, the range of wellbore collapse pressure varies from 1 to 2.2 g/cm3. Comparison reveals that when the bedding plane dip angle is 30°, the distribution range and upper limit of wellbore collapse pressure are the largest, indicating that wellbore is more prone to shear sliding failure along the bedding planes at this inclination. Furthermore, as the bedding plane dip angle changes, there are significant variations in the distribution range of optimal wellbore trajectories. When the bedding plane dip angle is 0°, wellbore collapse pressure is lower for wellbore inclination angles below 30°. Conversely, when the bedding plane dip angle is 90°, high-angle wellbores drilled along the direction of minimum horizontal stress exhibit lower wellbore collapse pressure. This indicates that in the design of wellbore trajectories in shale formations, the attitude of bedding planes should be determined. Accordingly, wellbore trajectories should be adjusted based on the orientation of bedding planes to enhance wellbore stability.
Figure 5. Influence of dip angle on wellbore collapse pressure when bedding dipping direction is 0°.
When the bedding plane dip angle is fixed at 30°, the polar plots of wellbore collapse pressure under different bedding plane strikes (ranging from 0° to 360°) are shown in Figure 6. Comparison reveals that regardless of the bedding plane strike, the distribution range of wellbore collapse pressure remains unchanged at 1–2.2 g/cm3. When the bedding plane strike is at 0°, wellbores with azimuth angles between 130° and 230° and inclination angles between 0° and 40° exhibit lower wellbore collapse pressure. Similarly, when the bedding plane strike is at 60°, wellbores with azimuth angles between 190° and 290° and inclination angles between 0° and 40° also experience lower wellbore collapse pressure. This suggests that with each 60° increase in bedding plane strike, the distribution area of optimal wellbore trajectories rotates clockwise by 60°. Therefore, while changes in bedding plane strike do not affect the numerical value of collapse pressure, they do influence the distribution area of optimal wellbore trajectories.
Figure 6. The influence of dipping direction on wellbore collapse pressure when the dipping angle is 30°.
One of the main characteristics of shale formations is the delayed instability of the wellbore, which is a phenomenon known as time-dependent instability. Accurately understanding the quantitative relationship between the wellbore’s safe drilling fluid density window and the drilling time in shale formations can assist in the development of wellbore stability techniques and efficient drilling construction measures. This study investigates the influence of drilling time on the polar plot of wellbore collapse pressure in shale formations.
Without considering the influence of bedding planes, the polar plots of wellbore collapse pressure at the initial stage of formation drilling, 2 days after formation drilling, 4 days after formation drilling, and 6 days after formation drilling are shown in Figure 7. At the initial stage of formation drilling, the maximum collapse pressure for any trajectory wellbore is 1.1 g/cm3, while the minimum is 0.85 g/cm3. After 2 days of formation drilling, the distribution range of collapse pressure for any trajectory wellbore ranges from 1.1 to 1.45 g/cm3. After 4 days of formation drilling, the distribution range of collapse pressure expands to 1.2–1.5 g/cm3. Subsequently, after 6 days of formation drilling, the distribution range of collapse pressure further increases to 1.2–1.55 g/cm3. It is evident that with increasing formation drilling time, the weakening of strength due to shale hydration leads to a gradual increase in collapse pressure.
Figure 7. The influence of shale hydration time on wellbore collapse pressure without considering bedding plane.
Comparatively, it can be observed that the distribution of wellbore collapse pressure polar plots remains unchanged for different drilling times, symmetrically distributed along the directions of maximum and minimum horizontal stresses. Only the numerical values of collapse pressure change. This indicates that shale hydration does not affect the distribution of optimal wellbore trajectories in the block. However, with prolonged hydration time, the lower limit of drilling fluid density required to maintain wellbore stability gradually increases.
Assuming horizontal bedding in the formation and considering the influence of bedding planes, the variation of wellbore collapse pressure polar plots with formation drilling time in shale formations is illustrated in Figure 8. Analysis reveals that when considering the influence of bedding planes, the change in collapse pressure for low-angle wellbores is relatively small, while for high-angle wellbores, the collapse pressure significantly increases. This indicates that high-angle wellbores experience a notable increase in collapse pressure around the wellbore due to shear sliding failure along the bedding planes. Furthermore, with the extension of formation drilling time, the collapse pressure for low-angle wellbores also significantly increases. This suggests that after shale hydration, the strength of the bedding planes further decreases, making the surrounding rock more susceptible to shear sliding failure along the bedding planes.
Figure 8. The influence of shale hydration time on wellbore collapse pressure with considering the effect of bedding planes.
Further analysis of the variation patterns of wellbore stability with hydration time reveals the changes in collapse pressure for vertical and horizontal wells, as shown in Figures 9, 10. For vertical wells, collapse pressure is unaffected by bedding planes, meaning they do not experience damage caused by shear sliding along bedding planes. With increasing formation drilling time, the collapse pressure of vertical wells initially sharply increases, then stabilizes after approximately 5 days. However, when considering the influence of bedding planes, the collapse pressure along both the direction of maximum and minimum horizontal stresses significantly increases. Additionally, it increases with hydration time. The difference lies in the fact that when considering the influence of bedding planes, the collapse pressure stabilizes after approximately 5 days of formation drilling, whereas without considering bedding planes, the collapse pressure essentially stops significantly increasing after approximately 2 days of formation drilling. This indicates that the weakening effect of hydration on the strength of bedding planes is more pronounced.
Taking Well X on QY1 Platform in the western part of the deep depression in Meijiayuan Sub-depression of Qintong Depression, Subei Basin as an example, this well serves as an appraisal well. Its drilling objective is to evaluate the adaptability of development technologies for the Upper II shale oil reservoir (sublayers 5-8) in the Fu’er Formation within the QY1 area, assess the production capacity and economically recoverable reserves of the 1500 m horizontal section, and obtain various geological parameters as a basis for reserve certification. Based on neighboring well drilling data, regional geological data, and seismic data, the expected strata encountered while drilling this well from top to bottom are the Dongtai Formation of the Quaternary, Yancheng Formation of the Neogene, Sandou Formation, Dainan Formation, Funing Formation (fourth, third, second sections) of the Paleogene.
To prevent collapse of the wellbore due to shale formation hydration, oil-based drilling fluid was used initially. However, during composite drilling to a depth of 1762.8m, a kick occurred, resulting in a flow rate of 2 m3. Throttling and adjusting the drilling fluid’s properties, the flow rate increased to 2.8 m3 at 17:40, prompting the well to be shut in immediately with a shut-in pressure of 7.9 MPa. After shutting in, personnel conducted circulation and prepared kill mud. During the kill mud injection process, a slight loss of circulation was observed after injecting 20 m3 of kill mud, leading to a decrease in flow rate. The choke was immediately lowered, the choke valve fully opened, and the shut-in pressure dropped to 0 MPa. Rig activity resumed, with a hang-off weight of 135t. Attempts to release the stuck drill string were unsuccessful. Subsequent pump circulation resulted in the removal of thick slurry and clear water, totaling 32m3 of contaminated drilling fluid. Analysis indicated water contamination of the oil-based drilling fluid, causing performance degradation, leading to borehole instability, well leakage, and drill string sticking. Consequently, the drill string had to be side-tracked after explosive unblocking, resulting in significant losses.
To prevent formation water contamination of oil-based drilling fluid, a water-based drilling fluid system was utilized for the sidetracked well. The mud weight was tested at 0.78 activity. At a depth of 1762.8m, the wellbore inclination angle was 12.5°, with a maximum angle of 75° relative to the horizontal maximum stress direction. The formation consists of mud shale with horizontal bedding planes. Using the data in Table 1 and models presented in this paper, different wellbore collapse pressures under various drilling cycles and drilling fluid densities were predicted, as shown in Figure 11.
Figure 11. Variability of lower limit of safe drilling fluid density window with drilling cycle for Well X.
After designing the drilling fluid density at 1.55 g/cm³, drilling proceeded to 2,290.0 m. Due to elevated torque during lifting, a short downstroke was initiated for safety, requiring the entire section to be reamed. Torque and pump pressure remained stable throughout the reaming process, which efficiently removed a significant number of cuttings and small chunks from the wellbore wall. Overall, the drilling progress during lifting and lowering was normal. After drilling for 5 days into the formation, a blockage was encountered at 1770.0m, leading to frequent pump stalling and top drive stalling events (with a top drive torque setting of 18 kN m, while normal reaming torque ranged from 13 to 15 kN m). Thickening agents with sand were added to the well, resulting in the removal of substantial chunks ranging from 1 to 2cm, with some as large as 3–4 cm. After reaming to the bottom, drilling resumed normally after circulation. Based on the research findings in this paper, the drilling fluid density was increased to 1.86 g/cm³, resulting in smooth drilling operations with significantly reduced chunking and normal cuttings return throughout, without any incidents of sticking.
To quantitatively evaluate the weakening effect of hydration on shale strength and wellbore instability, a wellbore instability model is established with strength anisotropy considered, besides, weakening effect of hydration on strength of rock matrix and bedding plane are considered respectively. The main conclusions of the study are as follows, Firstly, bedding planes exert a significant influence on the collapse pressure and optimal wellbore trajectories in shale formations. Incorporating bedding plane considerations is crucial for wellbore stability analysis.
Secondly, at a 30° bedding plane dip angle, the risk of shear sliding failure along bedding planes increases. Varying dip angles alter collapse pressure and optimal trajectory distribution significantly. Bedding plane dip direction impacts trajectory distribution rather than collapse pressure values, highlighting the need for tailored trajectory designs based on bedding plane characteristics.
Thirdly, highly inclined wellbores are more susceptible to collapse due to shear sliding along bedding planes. Prolonged drilling time weakens bedding planes, escalating the risk of shear sliding failures. These findings enhance our ability to assess hydration’s impact on wellbore stability quantitatively.
The original contributions presented in the study are included in the article/Supplementary material, further inquiries can be directed to the corresponding author.
SL: Writing–original draft, Writing–review and editing. PZ: Conceptualization, Data curation, Investigation, Methodology, Software, Supervision, Writing–original draft. BL: Formal Analysis, Funding acquisition, Project administration, Resources, Validation, Visualization, Writing–review and editing.
The author(s) declare financial support was received for the research, authorship, publication of this article. This paper is supported by Key Technologies and Engineering Tests for Beneficial Development of Shale Gas in Guizhou Province (Major Collaborative Innovation Project for Strategic Action of Mineral Search Breakthrough in Guizhou Province [2022]ZD005); Research on Hydraulic Fracturing Technology of Shale Gas Horizontal Well in Complex Structure Area (the Science and Technology Foundation of Guizhou Province [2022]230); Study on the Enrichment and Accumulation Conditions of Wufeng Formation Shale Gas in Fenggang Area, Guizhou Province (the Science and Technology Foundation of Guizhou Province [2023]344).
Authors SL, PZ, and BL were employed by Guizhou Energy Industry Research Institute Co.
All claims expressed in this article are solely those of the authors and do not necessarily represent those of their affiliated organizations, or those of the publisher, the editors and the reviewers. Any product that may be evaluated in this article, or claim that may be made by its manufacturer, is not guaranteed or endorsed by the publisher.
Aslannezhad, M., Keshavarz, A., and Kalantariasl, A. (2020). Evaluation of mechanical, chemical, and thermal effects on wellbore stability using different rock failure criteria. J. Nat. Gas Sci. Eng. 78, 103276. doi:10.1016/j.jngse.2020.103276
Chen, X., Yong, Z. Q., Deng, B., Bao, D., Peng, X., Liang, H., et al. (2022). Study on the loss mechanism of the silurian fractured reservoir in the southern part of shunbei No. 5 fault zone. Minerals Rocks 42 (2), 112–124. doi:10.19719/j.cnki.1001-6872.2022.02.11
Ding, L., Lv, J., Wang, Z., and Liu, B. (2022). Borehole stability analysis: considering the upper limit of shear failure criteria to determine the safe borehole pressure window. J. Petroleum Sci. Eng. 212, 110219. doi:10.1016/j.petrol.2022.110219
Fan, S., Song, B., Chen, Z., Li, D., Liu, J., and Cheng, Z. (2019). Technology for enhancing pressure bearing capacity of fractured Silurian system in well Shunbei 5-8. Drill. Fluid & Complet. Fluid 36 (4), 431–436. doi:10.3969/j.issn.1001-5620.2019.04.006
Gao, X., and Zhang, M. (2022). Investigation of 3D Mohr-Coulomb criteria performance based on the least absolute deviation method. Arabian J. Geosciences 15 (17), 1457. doi:10.1007/s12517-022-10672-8
Hang, W. E. N., Mian, C. H. E. N., Yan, J. I. N., Kai, W. A. N. G., Yang, X. I. A., Dong, J., et al. (2014). A chemo-mechanical coupling model of deviated borehole stability in hard brittle shale. Petroleum Explor. Dev. 41 (6), 817–823. doi:10.1016/S1876-3804(14)60099-9
Huang, J. G., and Zhang, L. (1998). “Influence of water on some characteristics of rock friction and sliding,” in Proceedings of the 5th academic conference of the Chinese society of rock mechanics and engineering for the sustainable development strategy of the national economy.
Huang, J. J., Jiang, M. J., and Wang, H. N. (2023). Unsteady analytical model of wellbore stability in hydrate-bearing formations based on fluid-solid coupling. J. Tongji Univ. Nat. Sci. Ed. 51 (7), 1033–1043.
Li, S. G., Luo, J., Yu, Y., Ming, T., Hao, Y., and Dezhi, Z. (2023). Establishment of pressure profile and wellbore structure optimization in the southern part of shunbei fault zone well No. 5. Pet. Drill. Tech. 51 (1), 9–15. doi:10.11911/syztjs.2022037
Li, Y., Fu, Y., Tang, G., She, C., Guo, J., Zhang, J., et al. (2012). “Effect of weak bedding planes on wellbore stability for shale gas wells,” in Proceedings of the IADC/SPE asia pacific drilling technology conference and exhibition (Society of Petroleum Engineers). doi:10.2118/155666-MS
Lin, H., Deng, J. G., Hu, L. B., Yuan, J., Chen, Z., and Lan, X. (2013). Study on the influence of moisture content on rock strength and sand production. Sci. Technol. Eng. 13 (13), 37–45. doi:10.3799/dqkx.2021.073
Liu, G., Zeng, L., Han, C., Ostadhassan, M., Lyu, W., Wang, Q., et al. (2020). Natural fractures in carbonate basement reservoirs of the Jizhong Sub-Basin, Bohai Bay Basin, China: key aspects favoring oil production. Energies 13 (18), 4635–4646. doi:10.3390/en13184635
Liu, Y. P., Yue, J. P., Peng, T., Li, B., and Ping, W. (2023). Study on wellbore stability based on porous elastic model and dynamic temperature field. Oil Gas Explor. Dev. 41 (2), 93–99. doi:10.3969/j.issn.1006-5539.2023.02.013
Liu, Y. P., Yue, J. P., Peng, T., et al. (2023). Study on wellbore stability based on porous elastic model and dynamic temperature field. Oil Gas Explor. Dev. 41 (2), 93–99.
Lu, B. P., Lin, Y. X., and Zhang, C. J. (1999). Experimental study on the influence of hydration on the mechanical properties of mudstone. J. Geomechanics 5 (1), 65–70.
Ma, T., and Chen, P. (2015). A wellbore stability analysis model with chemical-mechanical coupling for shale gas reservoirs. J. Nat. Gas Sci. Eng. 26, 72–98. doi:10.1016/j.jngse.2015.05.028
Ma, T., Chen, P., Zhang, Q., and Zhao, J. (2016). A novel collapse pressure model with mechanical-chemical coupling in shale gas formations with multi-weakness planes. J. Nat. Gas Sci. Eng. 36, 1151–1177. doi:10.1016/j.jngse.2016.02.034
Ma, T., Liu, J., Fu, J., Qiu, Y., Fan, X., and Martyushev, D. A. (2024b). Fully coupled thermo-hydro-mechanical model for wellbore stability analysis in deep gas-bearing unsaturated formations based on thermodynamics. Rock Mech. Rock Eng., 1–32. doi:10.1007/s00603-023-03703-7
Ma, T., Liu, K., Su, X., Chen, P., Ranjith, P. G., and Martyushev, D. A. (2024a). Investigation on the anisotropy of meso-mechanical properties of shale rock using micro-indentation. Bull. Eng. Geol. Environ. 83 (1), 29–45. doi:10.1007/s10064-023-03510-y
Ma, T. S. (2015). Study on wellbore stability mechanism of shale gas horizontal wells[D]. Ph.D. Thesis. Southwest Petroleum University.
Ma, Y. S., Cai, X. Y., Yun, L., Li, Z. J., Li, H. L., and Deng, S. (2022). Practices and theoretical technological progress of exploration and development of super-deep carbonate reservoirs in the Shunbei area of Tarim Basin. Petroleum Explor. Dev. 49 (1), 1–17. doi:10.11698/PED.2022.01.01
Meng, M., Chen, P., and Ren, R. (2019). Statistic evaluation of failure criteria in wellbore stability with temperature effects. Fuel 252, 730–752. doi:10.1016/j.fuel.2019.04.110
Qiu, Y., Ma, T., Peng, N., Liu, Y., and Liu, J. (2023a). “Wellbore stability analysis of inclined shale wells using a fully anisotropic hydraulic-mechanical coupled FEM model,” in ARMA US rock mechanics/geomechanics symposium (ARMA). doi:10.56952/ARMA-2023-0122
Qiu, Y., Ma, T. S., Chen, Y. J., Yang, B., and Deng, C. (2023b). Evolution law of wellbore stability of underbalanced horizontal wells in argillaceous sandstone reservoirs. J. Central South Univ. Sci. Technol. 54 (3), 967–983. doi:10.11817/j.issn.1672-7207.2023.03.015
Tang, C. F., Li, Z. H., Chen, H., Xing, X. S., Shou, X., and Hu, P. J. (2022). Analysis of collapse model and law of non-continuous coal seam wellbore considering multiple weak planes. Drill. Prod. Technol. 45 (5), 8–15. doi:10.11817/j.issn.1672-7207.2023.03.015
Tang, L., Wang, J. F., Cao, J. H., Yang, M., and Li, S. G. (2021). Exploration of integrated geological engineering model of super-deep karst reservoir in shunbei area, tarim basin. Eval. Dev. Oil Gas Fields 11 (3), 329–339. doi:10.13809/j.cnki.cn32-1825/te.2021.03.007
Tang, W. Q. (2011). Study on the influence of mudstone hydration on wellbore stability[D]. Ph.D. Thesis. China University of Petroleum East China.
Teng, J. Y., Tang, J. X., Zhang, Y. N., Duan, J. C., and Wang, J. B. (2017). Damage and failure process and characteristics of layered water-bearing shale under uniaxial compression. Rock Soil Mech. 38 (6), 1629–1638. doi:10.16285/j.rsm.2017.06.011
Wang, Y. H. (2023). Research on strengthening water-based drilling fluid technology in the northern bay basin. Chem. Eng. Equip. 7, 150–152.
Wang, F., Xu, H., Liu, Y., Meng, X., and Liu, L. (2023). Mechanism of low chemical agent adsorption by high pressure for hydraulic fracturing-assisted oil displacement technology: a study of molecular dynamics combined with laboratory experiments. Langmuir 39 (46), 16628–16636. doi:10.1021/acs.langmuir.3c02634
Wang, Z. M., Chen, J. X., Shen, Y. Y., Zhu, K. L., Li, Z. W., Hou, Y., et al. (2023). Drilling technology for stable wellbore in the long horizontal section of JN1H coalbed methane wells. Drill. Fluid & Complet. Fluid 40 (3), 356–362. doi:10.12358/j.issn.1001-5620.2023.03.011
Wen, K. M. (2015). Study on the influence of mudstone hydration on wellbore stability[D]. Ph.D. Thesis. Southwest Petroleum University.
Xu, C. Y., Yan, X. P., Kang, Y. L., You, L. J., and Zhang, J. Y. (2020). Mechanism of seal layer structure instability and strengthening method in deep fractured reservoirs. Petroleum Explor. Dev. 47 (2), 399–408. doi:10.11698/PED.2020.02.19
Yang, J., Zhao, Y. J., Li, Y., and Kang, Y. L. (2023). Study on the improvement of geomechanical simulation method for deep-water wellbore stability. Oil Gas Explor. Dev. 41 (4), 52–61.
Yang, L., Hou, D. W., and Zhao, Y. (2023). Study on the influence of inclined stratum of single-layered wellbore stability on drilling technology. Drill. Prod. Technol. 46 (1), 83–92.
Yang, Y. L., Wang, T. H., and Gao, Z. L. (2023c). Study and application of sandstone formation wellbore stability in an offshore Oilfield. Petroleum Explor. Dev. 7, 123–125.
Yu, J., Zhang, X. L., and Zhang, Y. N. (2022). Analysis of wellbore stability and pressure-bearing capacity of fractured Silurian system in Shunbei 5-8 well. J. China Coal Soc. 47 (9), 2866–2877.
Zhang, B., Pan, Z., Cao, L., Xie, J., Chu, S., Jing, Y., et al. (2024). Measurement of wellbore leakage in high-pressure gas well based on the multiple physical signals and history data: method, technology, and application. Energy Sci. Eng. 12 (1), 4–21. doi:10.1002/ese3.1619
Zhang, M., Fan, X., Zhang, Q., Yang, B., Zhao, P., Yao, B., et al. (2021a). Influence of multi-planes of weakness on unstable zones near wellbore wall in a fractured formation. J. Nat. Gas Sci. Eng. 93, 104026. doi:10.1016/j.jngse.2021.104026
Zhang, M., Fan, X., Zhang, Q., Yang, B., Zhao, P., Yao, B., et al. (2021b). Parametric sensitivity study of wellbore stability in transversely isotropic medium based on poly-axial strength criteria. J. Petroleum Sci. Eng. 197, 108078. doi:10.1016/j.petrol.2020.108078
Zhang, M., Li, D., Liu, J., Zhang, D., Zhang, Y., and Cui, K. (2023). The modification of Mohr-Coulomb criteria based on shape function and determination method of undetermined parameters. Mech. Mater. 185, 104772. doi:10.1016/j.mechmat.2023.104772
Zhang, M. M., Liang, L. X., and Liu, X. J. (2017). Analysis of the influence of different rock shear failure criteria on wellbore collapse pressure. Chin. J. Rock Mech. Eng. 36 (S1), 3485–3491.
Zhang, P. S., Xu, D. Q., Yan, W., Hu, X., Li, X., and Fang, J. X. (2023). Study on the influence of post-peak cycle loading on mechanical and permeability characteristics of sandstone under different confining pressures. Coal Sci. Technol. 51 (7), 94–105. doi:10.13199/j.cnki.cst.2023-0384
Zhengzheng, C., Pengshuai, W., Zhenhua, L., and Feng, D. (2024b). Migration mechanism of grouting slurry and permeability reduction in mining fractured rock mass. Sci. Rep. 14 (1), 3446. doi:10.1038/s41598-024-51557-y
Zhengzheng, C., Qiang, S., Zhenhua, L., and Feng, D. (2024a). Abnormal ore pressure mechanism of working face under the influence of overlying concentrated coal pillar. Sci. Rep. 14 (1), 626. doi:10.1038/s41598-024-51148-x
Zhong, Z., Meng, X., and Hu, Y. J. (2023). Experimental study on seepage and its influencing factors of rock mass with single crack under coupling of rock mass and fluid. Chin. J. Rock Mech. Eng. 42 (9), 2148–2163.
Keywords: hydration, shale, wellbore stability, strength weakening, time-dependent effects
Citation: Li S, Zhou P and Lan B (2024) Study of wellbore instability in shale formation considering the effect of hydration on strength weakening. Front. Earth Sci. 12:1403902. doi: 10.3389/feart.2024.1403902
Received: 27 March 2024; Accepted: 07 May 2024;
Published: 03 June 2024.
Edited by:
Manoj Khandelwal, Federation University Australia, AustraliaReviewed by:
Jintang Wang, China University of Petroleum, ChinaCopyright © 2024 Li, Zhou and Lan. This is an open-access article distributed under the terms of the Creative Commons Attribution License (CC BY). The use, distribution or reproduction in other forums is permitted, provided the original author(s) and the copyright owner(s) are credited and that the original publication in this journal is cited, in accordance with accepted academic practice. No use, distribution or reproduction is permitted which does not comply with these terms.
*Correspondence: Shaopeng Li, cGVuZzIwMjRfc3dwdUAxNjMuY29t
Disclaimer: All claims expressed in this article are solely those of the authors and do not necessarily represent those of their affiliated organizations, or those of the publisher, the editors and the reviewers. Any product that may be evaluated in this article or claim that may be made by its manufacturer is not guaranteed or endorsed by the publisher.
Research integrity at Frontiers
Learn more about the work of our research integrity team to safeguard the quality of each article we publish.