- 1Sinopec, Northwest Oilfield Company, No.4 Oil Production Plant, Urumqi, China
- 2Jiang Su Oilfield Company, No.2 Oil 4 Production Plant, Aksu, China
- 3Key Laboratory of Exploration Technologies for Oil and Gas Resources, Ministry of Education, Yangtze University, Wuhan, China
The fault cavern type reservoir in Block 1 of Shunbei area is located in the ultra-deep layer and is classified as a volatile oil reservoir. The reservoir is characterized by very deep burial with an oil/gas ratio between 250 and 500 m3/tone and a saturation pressure range of 28–36 MPa. As of March 2021, the formation pressure has dropped to 30.5 MPa and the pressure retention rate is 35.8%. In order to effectively develop this reservoir, it was decided to use a water injection development method with a planned transition to natural gas injection in March 2022 to further enhance recovery and maintain formation pressure. Through evaluation and research, the pressure distribution, production capacity change and reservoir dynamic response during the water injection development stage were analyzed with respect to the characteristics of fracture-controlled fracture-cave type reservoir in Shunbei-1 block. On this basis, the necessity, feasibility, and expected effects of conversion to natural gas injection are explored, including improvement of displacement efficiency, reduction of fluid output, and enhancement of formation energy replenishment. In addition, this study also carries out in-depth research and prediction on the optimization of natural gas injection parameters, the selection of injection methods and possible problems, aiming to provide scientific basis and technical support for the efficient and sustainable development of this block. In order to smoothly promote the implementation of natural gas injection development, a special reservoir description technique applicable to fault cavern type reservoirs was adopted. Based on this foundation, an in-depth study was conducted on the mechanism of natural gas displacement, so as to optimize the layout of the well network and the setting of injection and extraction parameters. In addition, a set of evaluation system for natural gas injection effect in fault cavern type reservoirs was constructed. By adopting the natural gas injection development method and combining the corresponding supporting technology development and optimization measures, the reservoir development efficiency and oil (gas) production rate have been effectively improved, and the risk of gas flaring has been reduced at the same time. These research results are of great significance for ensuring the comprehensive development and effective utilization of oil and gas resources, and provide strong technical support for future development work.
1 Introduction
Block one in the Shunbei area (Figure 1) possesses a fracture-controlled fracture-cavern type reservoir with a burial depth of more than 7,500 m and an oil column thickness of more than 400 m. The reservoir has a high degree of non-homogeneity and is highly heterogeneous. The fracture and cavern structures within the reservoir are intertwined, resulting in a high degree of inhomogeneity. At the early stage of reservoir development, the elastic energy was huge, and the original static pressure was as high as 85.5 MPa. However, as the development process advances, the pressure drops rapidly and the supplementary injection pressure reaches only 40.2 MPa, making the formation pressure maintain a low level. At present, characteristic difficulties such as the typical water tampering problem have emerged during the water injection process. Therefore, it is particularly important to implement natural gas injection evaluation and research on this reservoir which is deep, complex and has been facing problems such as pressure failure (Yuan, 2018; Ji et al., 2022; Jiawei et al., 2022; Chen et al., 2023).
In order to solve the water runoff problem, it is necessary to select a suitable energy replenishment medium based on a detailed description of the reservoir. Numerical simulations of the oil replacement process using different injection media show that the injection of natural gas can achieve the highest crude oil recovery up to 46.3% (Zhu et al., 2010; Yang et al., 2018; Hou et al., 2022; Xinxing et al., 2023). In view of the rich natural gas resources in the Shunbei oil field area, it was decided to adopt the natural gas injection development method (Tang et al., 2004; Juhua et al., 2008; Ma et al., 2017; Luo et al., 2022). In this process, targeted gas injection strategy design and optimization was implemented for fracture-controlled fracture-cavity type reservoirs, including but not limited to: building accurate geological models to identify favorable gas injection channels (Baozeng et al., 2020; Zhang et al., 2022; CHEN et al., 2024). Formulate reasonable well network layout and injection and extraction parameter programs to avoid or reduce the occurrence of gas flushing; and use advanced monitoring technology to monitor the dynamic effect of gas injection and possible gas transportation behavior in real time, so as to ensure the effective utilization of natural gas and the efficient development of the reservoir (Cong et al., 2014; Liang et al., 2016; Zheng et al., 2022; Hu et al., 2023). In addition, for the complex situations that may occur after natural gas injection, such as uneven pressure distribution and formation injury, we have carried out in-depth research combining theoretical analysis and on-site practice, established a comprehensive evaluation system for natural gas injection, and formulated corresponding countermeasures and technical standards, so as to guarantee the smooth progress and long-term benefits of the natural gas injection development project for the fracture-controlled fracture-cavern-type reservoirs in Shunbei Area Block 1 (Lu and Cai, 2010; YUAN et al., 2023).
This paper, based on an analysis of the mechanisms behind natural gas injection, conducted research on the design and optimization of well patterns and injection-production parameters under natural gas injection conditions. An evaluation of the current stage of natural gas flooding was performed, and preventive policies against gas channeling were formulated. This work provides theoretical and foundational support for the development of natural gas injection in Shunbei Area Block 1.
2 Study of natural gas injection mechanism
In the Shunbei Area Block one reservoir, the original crude oil contains a high content of C2-C6 components, ranging from 11.8% to 19.4% of the total. Additionally, the reservoir has high pressure conditions, which are favorable for the formation of miscible gas flooding. Through laboratory experiments, the miscibility threshold pressure in the Shunbei Area Block one was determined to be 47 MPa, and it was confirmed that the miscible gas flooding in this area follows an evaporative multi-contact miscible flooding mechanism. The multi-contact mechanism significantly reduces the density, viscosity, and interfacial tension of the crude oil, greatly improving its flowability, and increasing the volume coefficient, thereby enhancing elastic energy. Numerical simulations conducted for different injection phase stages in the A1-12 well group indicate that miscible flooding yields better oil recovery results (as shown in Figure 2).
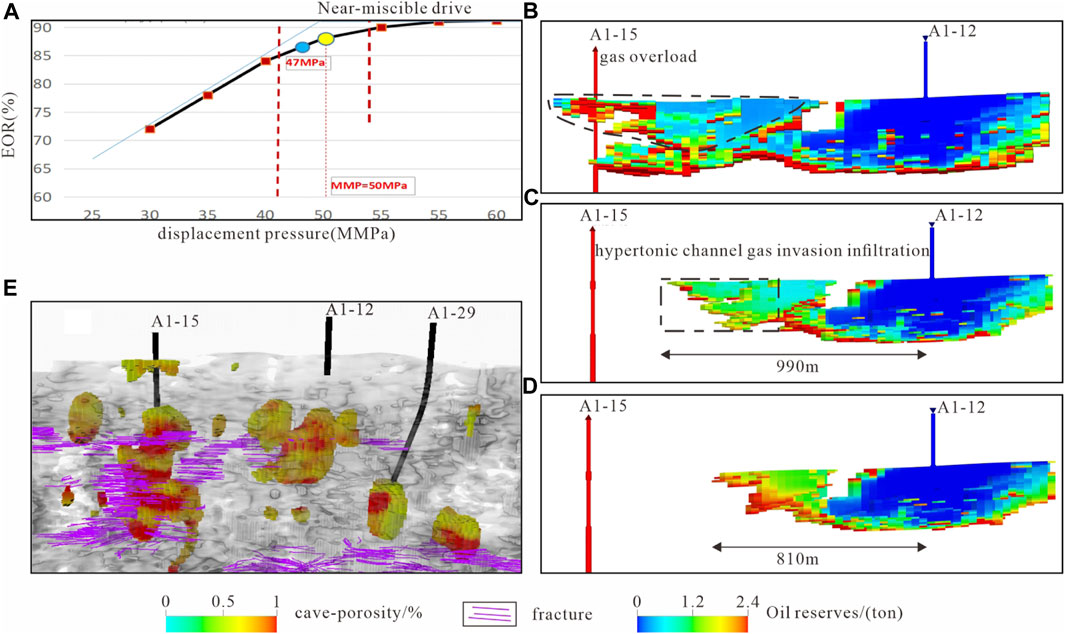
Figure 2. Residual Oil Distribution for Different Injection and Production States in Well Group A1-12 (A). diagram of the minimum mixing pressure for natural gas flooding; (B) residual oil distribution of non-miscible drive in 35MPa; (C) residual oil distribution of near-miscible drive in 45MPa; (D) residual oil distribution of mixed drive in 55MPa; (E) location of wells).
3 Injection and production well network design
3.1 Injection and production well network design
Shunbei Area one is affected by large-scale strike-slip fracture zones, and develops slit-type carbonate reservoirs, which are strongly controlled by the fracture zones, with weak karst development, and extremely non-homogeneous reservoirs. According to the characteristics of the fractured reservoir in Block one of Shunbei area, four connectivity modes are designed: segmented pulling type along the section, segmented pulling type through the body, segmented panning type along the section and segmented panning type through the body. Meanwhile, three different injection and extraction relationships were established, i.e., high injection and low extraction, low injection and high extraction, and balanced injection and extraction (see Figure 3).
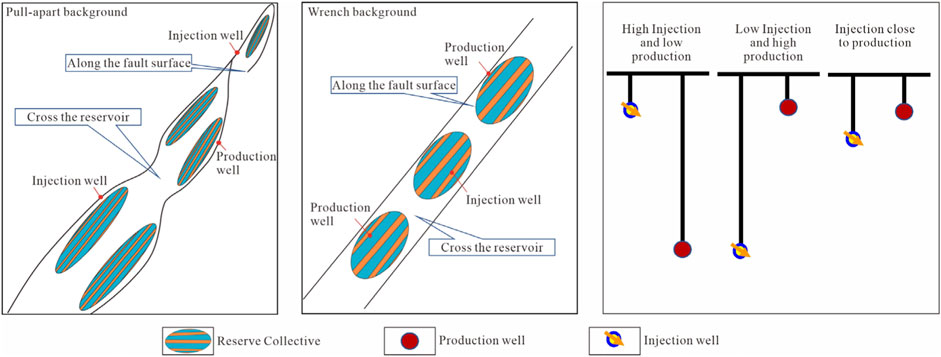
Figure 3. Connectivity mode and injection-production relationship diagram of Shunbei fault-controlled fracture-cavity reservoir.
From the numerical simulation results, it can be observed that in the “low injection and high production” well network, due to the development of high-angle fractures and a significant vertical thickness, the gravitational segregation of injected gas is pronounced. After gas injection, gas channeling is likely to occur easily, leading to a decrease in reservoir energy. Specifically, after 20 days of gas injection, the gas-oil ratio increased by 50%, after 50 days it increased by 100%, and after 90 days it increased by 150% (see Figure 4).
In contrast, the “high injection and low production” well network, due to the reduction in the producing interval in oil wells, effectively delays gas channeling (for 50 days). It also fully exploits the effects of gas injection for reservoir re-energizing and gas cap drive (see Figure 5). Specifically, after 70 days of gas injection, the gas-oil ratio increased by 50%, after 100 days it increased by 100%, and after 135 days it increased by 150%.
Therefore, it is preferable to choose the “high injection and low production” injection-production relationship, which results in a slower increase in gas-oil ratio and better reservoir re-energizing effects.
For fractured-dominated fissure-type reservoirs, the development of fractures and the effectiveness of miscible displacement are constrained by factors such as cross-sections, fractures, and reservoir types. When the injection wells are surrounded by predominantly fractured reservoirs and are directly connected to the production wells through fractures or connected through the same cross-section, it is easy to form dominant gas drive channels, leading to the occurrence of gas channeling. In such a connectivity pattern, natural gas drive predominates as the primary mechanism, with miscible displacement playing a secondary role. However, when injection wells are located in areas with developed caves, and production wells are on different cross-sections, natural gas drive is primarily based on miscible displacement. The injection gas extends over a large and uniform area, making gas channeling less likely.
Therefore, it is recommended to preferentially select the connectivity pattern with the development of cave-type reservoirs and injection-production wells on different cross-sections for injection and production operations (see Figure 6). This approach helps optimize reservoir development and enhance uniform gas distribution, reducing the likelihood of gas channeling.
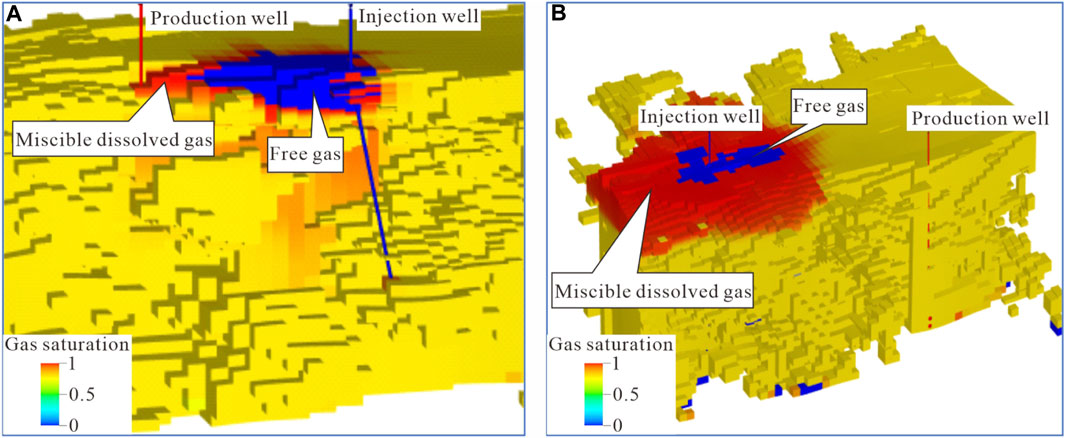
Figure 6. Oil Displacement Effects under Different Connectivity Patterns [(A) Injection well cross the fault and in the same side with the production well; (B) injection well and production well is on different side of the fault].
3.2 Injection and production parameters design
The numerical simulation results of injection and recovery parameter design show that the higher the reservoir pressure is maintained, the higher the cumulative oil production is. However, when the pressure is restored to 130% of the mixed-phase pressure, the production increase effect slows down significantly. Therefore, the optimal pressure maintenance level was determined to be 61 MPa (Figure 7).
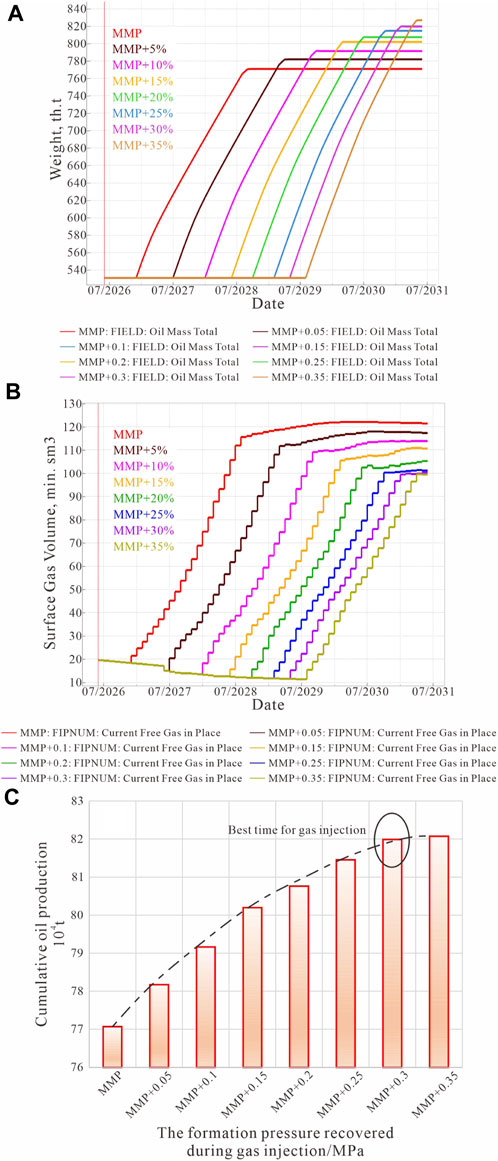
Figure 7. Comparison of the effect of gas injection to pressure increase in different formations in the fault-controlled fracture-cavity reservoir in Shunbei one area [(A) Cumulative oil production at different injection times; (B) The volume of free gas at different injection times; (C) Intersection plot of cumulative oil and swept volume].
However, physical simulation experiments simulating changes in oil recovery wells after gas injection through a laboratory setup showed relatively small increases in crude oil recovery after reaching mixed-phase drive. The discrepancy between the numerical simulations and the mixed-phase experimental results is mainly attributed to the consideration of pressure sensitivity factors of the rock. As pressure increases, connectivity and permeability between fractures and pores are enhanced. When designing the injection and extraction parameters, factors such as reservoir pressure, injected gas type and injection rate are comprehensively considered to balance the contradiction between improving oil driving efficiency and preventing gas flushing. By comparing and analyzing different injection and extraction schemes, we select the injection rate and volume that can enhance the formation pressure within the effective range and minimize the occurrence of gas flushing, so as to realize the economic and effective development strategy of natural gas drive. At the same time, in combination with the actual geological conditions, the pressure distribution during the injection phase is finely regulated, and the well network structure is optimized for the characteristics of different well groups, to ensure that the injected gas can be more evenly distributed in the target reservoir, to further reduce the risk of gas flushing and improve the overall efficiency of oil drive.
By selecting different well groups for simulation with varying injection rates, it can be observed that the optimal gas injection rate falls within the range of 90,000 to 150,000 cubic meters per day. When the injection rate exceeds 150,000 cubic meters per day, gas channeling accelerates, adversely affecting the performance (Figure 8).
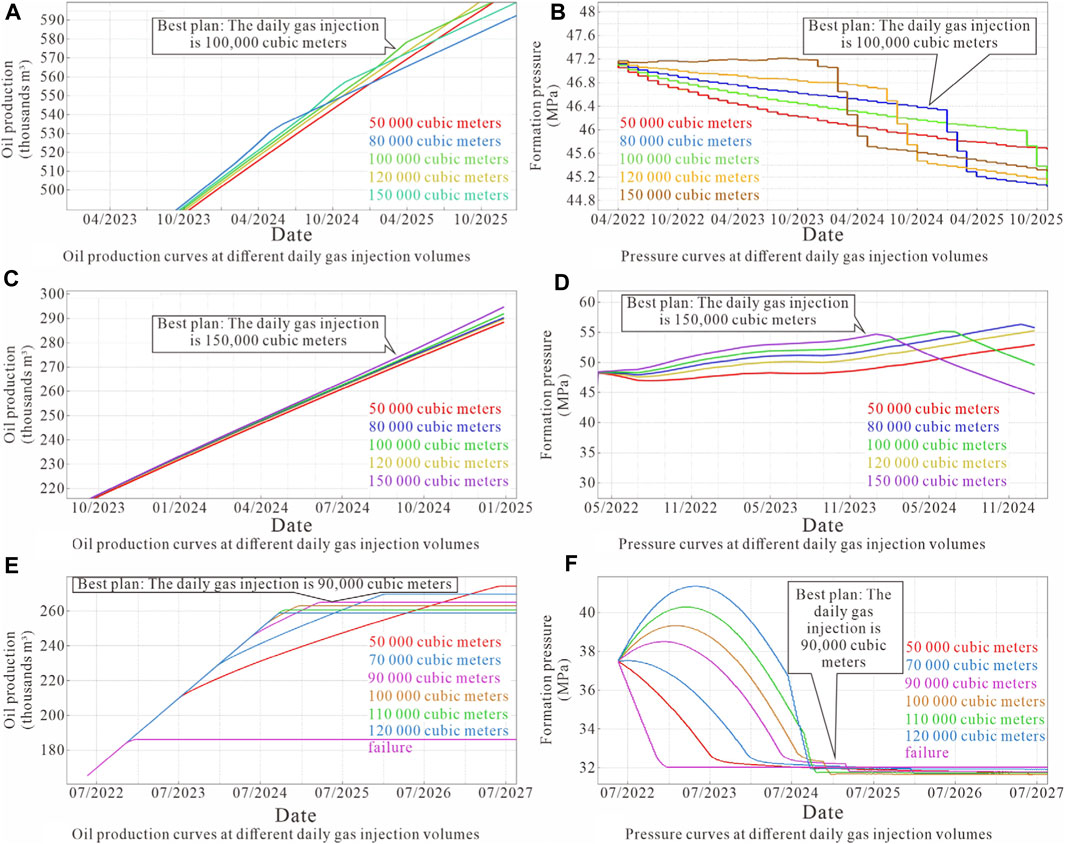
Figure 8. Comparison of the Effects of Different Injection and Production Ratios and Gas Injection Rates on Different Well Groups [(A) A1-25 well group cumulative oil production curve; (B) A1-25 Well Group Pressure Curve; (C) A1-10 well group cumulative oil production curve; (D) A1-10 Well Group Pressure Curve; (E) A1-12 well group cumulative oil production curve; (F) A1-12 Well Group Pressure Curve).
To assess the oil recovery effect at different stages with varying injection-production ratios, simulations were conducted (Yuanshuai et al., 2008; Gao et al., 2009; Rong et al., 2010) (Table 1). Before the miscible displacement, the injection-production ratio was set at 2:1, resulting in a simulated oil recovery increase of 171,000 tons. After miscible displacement, the ratio was adjusted to 1:1, with a similar simulated increase of 171,000 tons. Therefore, following the principle of ‘one well, one strategy’ and ‘optimal gas drive,’ the initial injection-production ratio can be designed as 2:1, which should transition to 1.2:1 after reaching miscible displacement. This transition should occur gradually while maintaining the reservoir pressure at 61 MPa. Additionally, dynamic optimization adjustments should be made based on wellbore safety, gas drive effectiveness, and gas channeling conditions.
4 Evaluation of natural gas drive effect
4.1 Evaluation criteria
We have selected eight key indicators to evaluate the effectiveness of natural gas drive in fissure-type reservoirs, for ease of assessment: a. Ineffective Gas Injection: The gas produced beyond the normal gas-oil ratio is considered ineffective gas injection. b. Gas Storage: It is calculated as the gas injection volume minus the ineffective gas injection. c. Gas Storage Ratio: Gas storage divided by the cumulative gas injection volume. d. Sweep Efficiency: The percentage of the reservoir volume (or area) swept by the injected displacement system relative to the total reservoir volume (or area), which is related to reservoir volume/area. The swept area by the displacement system is represented by the effective gas injection volume (gas storage), and the total reservoir volume is the geological reserves of the well group. e. Displacement Efficiency: The ratio of underground crude oil displaced by the injected displacement phase to the crude oil within the displacement range (injected gas volume). f. Enhanced Oil Recovery Degree: It is calculated as the incremental oil production divided by the geological reserves of the well group. g. Enhanced Oil Recovery Rate: Estimated incremental oil production divided by the geological reserves of the well group. h. Gas Drive Utilization Reserves: The gas consumption per unit of pressure increase for the well group divided by the liquid production per unit of pressure decrease for the well group, multiplied by the utilized reserves for the well group (Guo et al., 2004; Tang et al., 2005; Wen et al., 2011).
4.2 Evaluation results
Four different types of typical well groups subjected to natural gas drive were selected for evaluation and comparison. Comparing the injection-production relationships, for the same reservoir with through-reservoir type, the high injection and low production type has a gas storage ratio 1.14 times that of the low injection and high production type. The incremental oil production is 2.6 times higher, displacement efficiency is 1.1 times higher, estimated enhanced recovery rate is 3.7 times higher, and sweep efficiency is 4.7 times higher, and gas channeling is less likely to occur. Comparing the connectivity types, both are low injection and high production types, with through-reservoir and along-the-cross-section types. The former has an incremental oil production 3.6 times higher, and a displacement efficiency of 33.5%, which is 6.1 times higher than the latter. Therefore, overall, the through-reservoir type with high injection and low production exhibits better natural gas drive effectiveness (Figure 9; Table 2).
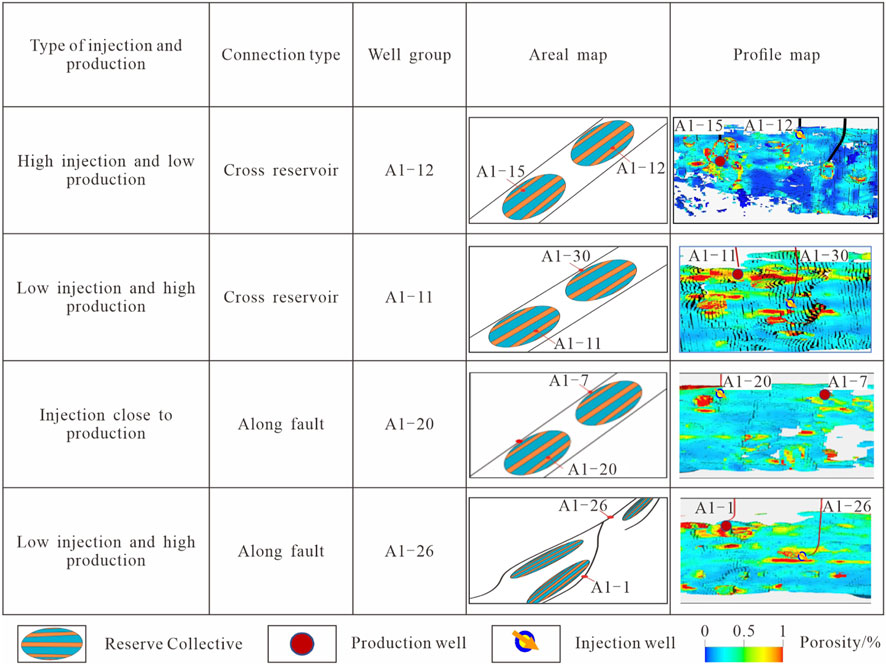
Figure 9. Comparison of the effects of different injection-production relationships on different well groups at different gas injection rates.
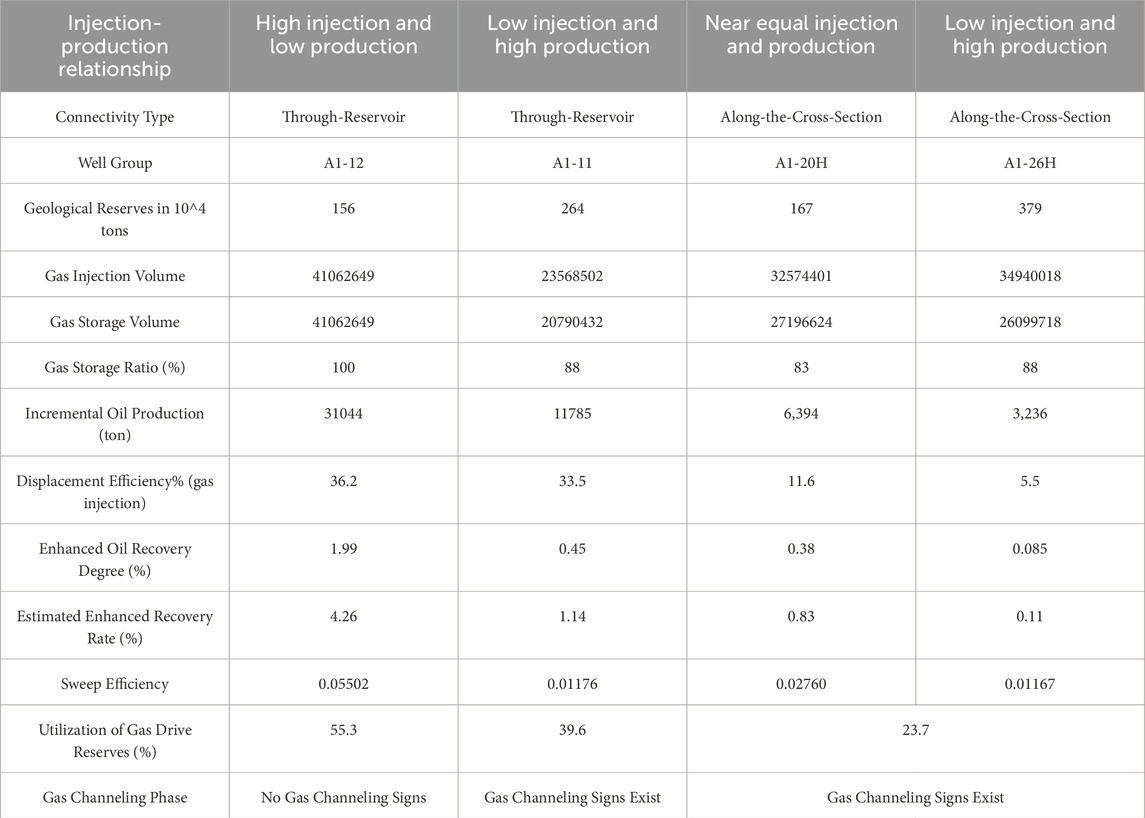
Table 2. Gas injection effect evaluation table for well groups under natural gas drive in Shunbei zone 1.
5 Gas channeling prevention policies and effects
5.1 Gas channeling evaluation indicators
Incorporating field trials in fractured control fissure-type oil reservoirs, a 1-main-3-auxiliary set of gas channeling evaluation indicators has been established, with the gas-oil ratio as the primary indicator and natural gas composition, flow pressure gradient deviation, and production changes as auxiliary indicators for the phase division of gas injection well groups. The indicators for the initial stage of gas channeling are as follows: a gas-oil ratio increase of 20%–100%, a C1 content increase of over 5% in produced gas, a decrease of over 45% in C5+ content, and a production decline within the range of 0%–5%. When the gas-oil ratio exceeds 100% or the production decline falls within the range of 5%–12%, it is considered the mid-stage of gas channeling, and when the production decline exceeds 12%, it is deemed to have entered the late stage of gas channeling (Figure 10).
Currently, three low injection-high production wells exhibit abnormal indicators, with one in the late stage of gas channeling, one in the mid-stage, and one in the early stage. Among them, the low injection-high production, along-the-cross-section connected well A1-1H has experienced a 562% increase in gas-oil ratio, a 6.4% increase in C1 content, a 52% decrease in C5+ content, and a 30% production decline, indicating it is in the late stage of gas channeling. The low injection-high production, through-reservoir connected well A1-11 has seen a 114% increase in gas-oil ratio, a 4.9% increase in C1 content, a 22% decrease in C5+ content, and a 4.0% oil production decline, placing it in the mid-stage of gas channeling. The near equal injection-production, along-the-cross-section connected well A1-7H has witnessed a 93.5% increase in gas-oil ratio, a 4.9% increase in C1 content, a −50% decrease in C5+ content, and a slight 3% production increase, indicating it is in the mid-stage of gas channeling. The high injection-low production, through-reservoir connected well A1-15 has shown a current production increase of 50%, with a stable gas-oil ratio and no signs of gas channeling observed. It is evident that in fractured control fissure-type oil reservoirs, prioritizing the construction of a high injection-low production, through-reservoir connected gas injection well network can effectively delay gas channeling and achieve better natural gas drive results (Table 3).
5.2 Gas migration control measures
For fault-controlled cavity-type oil reservoirs, gas migration management is based on the “well network model,” aiming to “improve recovery rates.” Development strategies are adjusted in stages and differentially according to different pressure types. In the early stage of gas migration, different types of well groups are adjusted differently. For low-injection, high-production well groups that have not reached miscibility, maintain an injection to production ratio of 1.5–2.0 to also accommodate energy supplementation to reach miscibility. For low-injection, high-production well groups with remaining oil at the top, utilize natural gas for its “oil-driving” and “oil-replacing” functions; for those without remaining oil at the top, use natural gas for its “oil-driving” function. In contrast, high-injection, low-production well groups should implement balanced injection and extraction, continuously using natural gas for its “oil-replacing” function. In the mid-stage of gas migration, all should carry out displacement adjustment, maintaining a 1:1 injection to production ratio to sustain production; however, for low-injection, high-production well groups at the top of the reservoir with no remaining oil and not yet reaching miscibility, a larger injection to production ratio should be maintained to achieve miscibility. In the late stage of gas migration, if it is a low-injection, high-production well network, shift the injection and production relationship to high-injection, low-production for natural gas development; if it is already a high-injection, low-production network, then proceed with depletion-type development (refer to Figure 11).
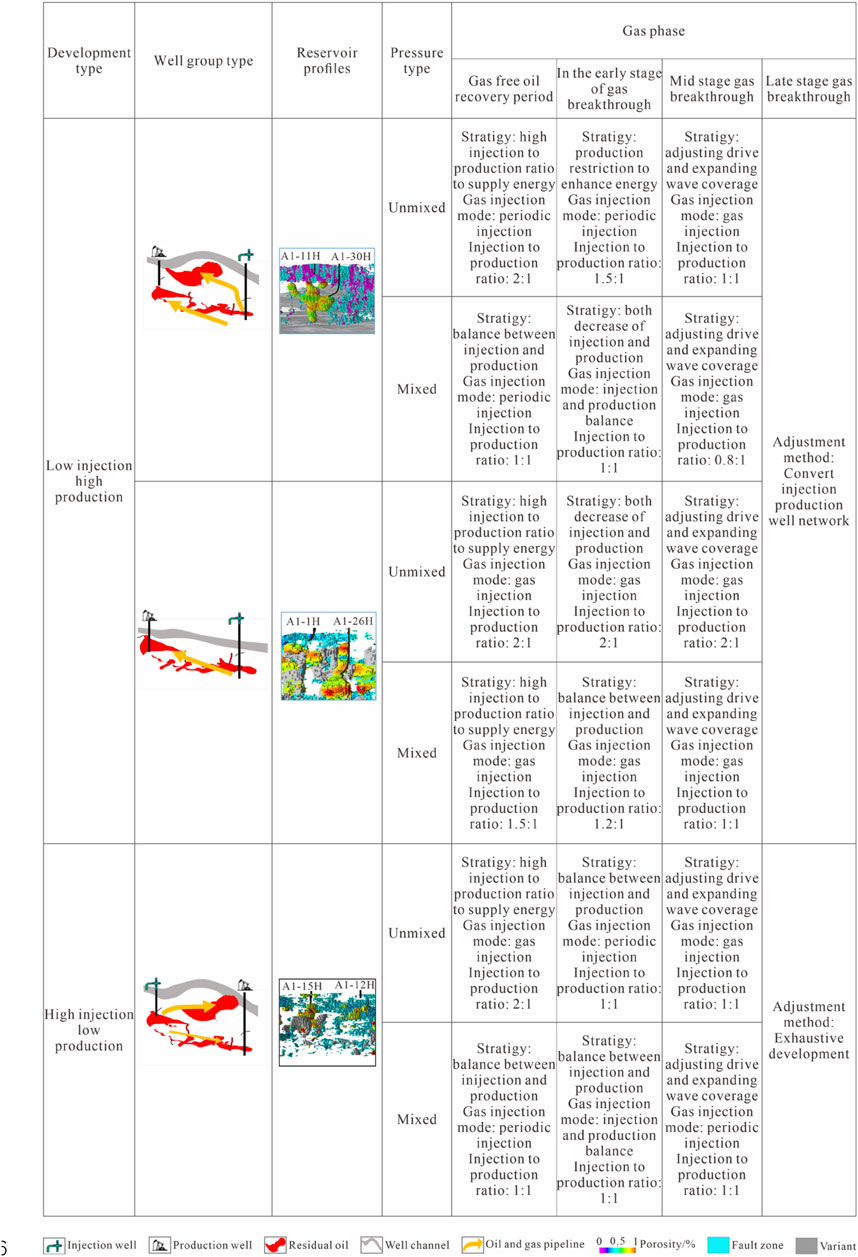
Figure 11. Development strategy diagram for different well network types at different gas appearance stages in fault-controlled fractured cavity-type oil reservoirs.
5.3 The effects of managing gas migration in a specific well group
A1-1H Well Group: This group is a low-injection, high-production well network, interconnected along the fault plane. Early numerical modeling indicated that the primary mechanism of gas drive is gravitational override, with the injected natural gas predominantly advancing along the fault plane.
Gas Migration Management: 21 days after natural gas injection into the A1-26H well, a pressure response was observed in the A1-1H well. 76 days post-injection, the gas-to-oil ratio in the A1-1H well rapidly increased, and the flowing pressure gradually declined, indicating signs of gas appearance. The well group is currently in the late stage of gas migration, with a 1-day response time for injection and production (refer to Figure 12; Table 4).
For the A1-1H well group, several techniques were employed to control gas migration, including intermittent injection, reduced injection, fluid control, increased injection, and fluid control. Overall, fluid control proved to be the most effective. Initially, after intermittent injection, the gas-to-oil ratio continued to rise by 107%, production decreased by 9.5%, formation pressure dropped by −4.3 MPa, the methane (C1) content in the produced gas continuously increased, and the C5+ content consistently decreased. Subsequently, after reduced injection, the gas-to-oil ratio continued to rise by 17.8%, production fell by 11.3%, formation pressure decreased by 1.1 MPa, the methane content still slightly increased, the C5+ content slightly decreased, and the gas storage rate dropped to its lowest at 22.14%. Finally, after fluid control, the results were better: the gas-to-oil ratio decreased by 41%, formation pressure increased by 6.2 MPa, gas storage rate rose to 76.6%, methane content in the produced gas decreased by 1%, and C5+ content increased by 16%. This indicates that injecting natural gas effectively improved the development outcomes (refer to Figure 13).
6 Conclusion
The fault-controlled fracture-cavity type reservoirs in the Shunbei Oilfield have achieved the best results in the development using natural gas injection. By rapid initial gas injection, followed by slow gas injection with phase mixing, supplemented by a balanced injection and recovery strategy, the drive process of crude oil was effectively promoted. An evaluation system was also established in the study, indicating that the optimal results are obtained with a high injection-low recovery ratio, good connectivity of the well network in non-single sections, and gas injection through collective reservoir-type wells. In addition, we have developed a gas flurry prediction system and a gas flurry control strategy. Experimental results show that controlling the liquid injection volume is more effective than optimizing the injection period and rate. By targeting different stages and types of well packs with appropriate gas transportation management measures, as shown in the case of A1-1H well pack, we can effectively improve the gas flushing situation, enhance the effect of natural gas drive, and ultimately improve the oil recovery rate and economic benefits of the whole block. This series of research results provide scientific basis and technical guidance for future oil and gas field development under similar geological conditions.
Data availability statement
The original contributions presented in the study are included in the article/Supplementary material, further inquiries can be directed to the corresponding author.
Author contributions
YNH: Writing–original draft. YL: Writing–review and editing. WC: Writing–review and editing. LC: Data curation, Writing–review and editing. YJH: Data curation, Writing–review and editing. HL: Methodology, Writing–review and editing. YS: Methodology, Writing–review and editing. LW: Writing–review and editing.
Funding
The author(s) declare that no financial support was received for the research, authorship, and/or publication of this article.
Conflict of interest
Authors YNH, YL, LC, and HL were employed by Sinopec, Northwest Oilfield Company. Authors WC, YJH, and YS were employed by Jiang Su Oilfield Company.
The remaining author declares that the research was conducted in the absence of any commercial or financial relationships that could be construed as a potential conflict of interest.
Publisher’s note
All claims expressed in this article are solely those of the authors and do not necessarily represent those of their affiliated organizations, or those of the publisher, the editors and the reviewers. Any product that may be evaluated in this article, or claim that may be made by its manufacturer, is not guaranteed or endorsed by the publisher.
References
Baozeng, L. I. U., Qi, L., Zongjie, L. I., Jun, L. I. U., Chao, HUANG, Lin, YANG, et al. (2020). Spatial characterization and quantitative description technology for ultra-deep fault-karst reservoirs in the Shunbei area. Acta Pet. Sin. 41 (4), 412–420.
Chen, Y., Zhu, L., and Liu, X. (2023). Feasibility of natural gas miscible flooding in ultra-deep Fault-Controlled reservoirs in shunbei-1 Block. Xinjiang Pet. Geol. 44 (02), 203–209.
Chen, S., Yunfeng, H. E., Wang, L., et al. (2024). Architecture characterization and 3D geological modeling of Ordovician carbonate reservoirs in Shunbei No. 1 fault zone, Tarim Basin. Lithol. Reserv. 36 (2), 124–135.
Cong, T., Peng, X., and Yang, Li (2014). Optimization of waterflooding process for Fault⁃Controlled Fracture⁃Cavity reservoir of ordovician in tahe field. Tarim. Basin 35, 703–707.
Gao, H., Hou, T., and Wu, Y. (2009). Study of EOR by natural gas injection. Drill. Prod. Technol. 32, 25.
Guo, P., Du, Z., and Zhang, M. (2004). Case analysis on hydrocarbon alternative gas miscible flooding in Pubei oil field. J. Southwest Petroleum Inst. 26, 25.
Hou, D., Liu, X., Zheng, W., et al. (2022). Problems and countermeasures of gas-oil ratio rise in gas injection development of Carboniferous oil reservoirs in Oilfield Donghe-1 of Tarim Basin. Petroleum Geol. Oilfield Dev. Daqing 41 (02), 51–58. doi:10.19597/j.issn.1000-3754.202006035
Hu, W., Li, X., Yang, M., et al. (2023). Well pattern optimization for fractured-vuggy carbonate reservoirs in tahe oilfield. Xinjiang Pet. Geol. 44 (4), 429–434.
Ji, B., Zheng, S., and Gu, H. (2022). On the development technology of fractured-vuggy carbonate reservoirs: a case study on Tahe oilfield and Shunbei oil and gas field. Oil Gas Geol. 43 (6), 1459–1465. doi:10.11743/ogg20220614
Jiawei, F. A. N., Ye, YUAN, Shaohua, L. I., et al. (2022). Geology-engineering integrated simulation technology of deep tight oil reservoir in Tarim Basin. Fault-Block Oil Gas Field 29 (2), 194–198.
Juhua, Li, Xiangfang, Li, Jiang, T., et al. (2008). Study on mechanism and features of unstable state displacement of miscible hydrocarbon-gas flooding. Petroleum Geol. oilfield Dev. Da Qing 27, 95.
Liang, CHENG, Zhang, X., Wang, J., et al. (2016). Study on periodic gas injection mechanism of condensate gas reservoirs with high to high-super content of condensate oil in Central Tarim by numerical simulation. J. Xi'an Shiyou Univ. Nat. Sci. Ed. 31 (2), 57–64.
Lu, X., and Cai, Z. (2010). A study of the paleo cavern system in fractured vuggy carbonate reservoirs and oil/gas development-taking the reservoirs in Tahe oilfield as an example. Oil Gas Geol. 31, 22–25.
Luo, Q., Chen, L., Wang, J., et al. (2022). Study on the oil-gas miscibility zone in tarim oilfield. J. Chongqing Univ. Sci. Technol. 24, 42–47.
Ma, Q., Yan, E., Wanfen, Pu, et al. (2017). Experimental study on the natural gas and CO2 injection EOR processes in tuofutai Block of tahe oilfield. Sci. Technol. Eng. 17 (08), 14–20.
Rong, Y., Liu, X., and Yang, M. A discussion on water flooding in the multi⁃well fractured⁃vuggy units of carbonate reservoirs in Tahe oilfield. Oil Gas Geol., 2010, 31, 28–32.
Tang, Y., Sun, L., Shilun, Li, et al. (2004). On evaluation method of miscible gas-flooding mechanism. Xinjiang Pet. Geol. 25 (4), 414–416.
Tang, Y., Sun, L., Zhou, Y., et al. Mechanism evaluation of condensing/vaporizing miscible flooding with hydrocarbon⁃rich gas injection.Petroleum Explor. Dev., 2005, 32, 133.
Wen, CHEN, Tang, Y., Liang, T., et al. (2011). Mechanism study of dynamic mixed-phase driving process of supercritical CO2. Drill. Prod. Technol. 34 (03), 77–80+117.
Xinxing, H. E., Huang, Z., Liming, LIAN, et al. (2023). Key technologies for enhancing the recovery of condensate gas reservoirs by gas injection–storage coordination. Nat. Gas. Ind. 43 (1), 86–95.
Yang, L. I., Zhijiang, KANG, Xue, Z., et al. (2018). Theories and practices of carbonate reservoirs development in China. Petroleum Explor. Dev. 45 (4), 669–678.
Yuan, D. (2018). EOR technology and related mechanism research of gas injection to fractured-cavity carbonate reservoir. Beijing: China University of Petroleum.
Yuan, F. Y., Wang, H. P., Yue, P., et al. (2023). Simulation analysis of influencing factors of single well gas injection in fracture-vuggy carbonate reservoir. Unconv. Oil Gas 10 (4), 86.
Yuanshuai, R., Liu, X., and Luo, J. (2008). Pilot study on waterflooding development in multi⁃well fracture⁃cavity units of Tahe oilfield. Oil Drill. Prod. Technol. 30, 83–87.
Zhang, W., Zhang, Y., Duan, T., et al. (2022). Hierarchy modeling of the Ordovician fault-karst carbonate reservoir in Tuopaitai area, Tahe oilfield, Tarim Basin, NW China. Oil&Gas Geol. 43 (1), 207–218. doi:10.11743/ogg20220117
Zheng, S., Kang, Z., Cheng, X., et al. (2022). Waterflooding characteristics and improvement measures of fracture-cavity carbonate reservoirs in Tahe Oilfield. Petroleum Geol. Recovery Effic. 29 (6), 95–104. doi:10.13673/j.cnki.cn37-1359/te.202201022
Keywords: fault-controlled fractured-cavity type, natural gas injecting, gas flooding evaluation, gas leakage, Shunbei fault zone no. 1, deep-ultra-deep oil and gas development
Citation: He Y, Liu Y, Chen W, Chi L, He Y, Li H, Sun Y and Wang L (2024) Evaluating and study of natural gas injecting in Shunbei-1 block fault-controlled fractured-cavity type reservoir. Front. Earth Sci. 12:1399921. doi: 10.3389/feart.2024.1399921
Received: 12 March 2024; Accepted: 11 April 2024;
Published: 16 May 2024.
Edited by:
Xixin Wang, Yangtze University, ChinaReviewed by:
Bo Liao, China University of Petroleum (East China), ChinaMuming Wang, University of Calgary, Canada
Pengfei Xie, University of Calgary, Canada
Copyright © 2024 He, Liu, Chen, Chi, He, Li, Sun and Wang. This is an open-access article distributed under the terms of the Creative Commons Attribution License (CC BY). The use, distribution or reproduction in other forums is permitted, provided the original author(s) and the copyright owner(s) are credited and that the original publication in this journal is cited, in accordance with accepted academic practice. No use, distribution or reproduction is permitted which does not comply with these terms.
*Correspondence: Yunfeng He, heyf.xbsj@sinopec.com