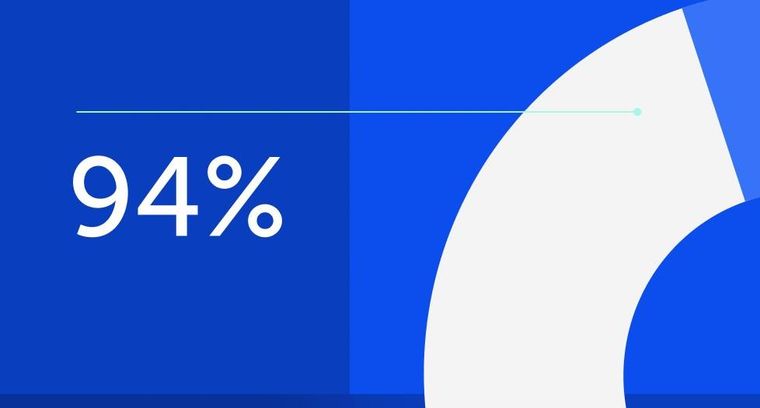
94% of researchers rate our articles as excellent or good
Learn more about the work of our research integrity team to safeguard the quality of each article we publish.
Find out more
ORIGINAL RESEARCH article
Front. Earth Sci., 02 July 2024
Sec. Geohazards and Georisks
Volume 12 - 2024 | https://doi.org/10.3389/feart.2024.1395418
This article is part of the Research TopicMonitoring, Early Warning and Mitigation of Natural and Engineered Slopes – Volume IVView all 30 articles
Introduction: The research is aimed to identify the vulnerable areas of quarry slopes through the creation of a stress-strain state model for the rock mass at the Vostochny quarry, East Saryoba field of the Zhi-landy Group of cupriferous sandstones, as well as their subsequent strengthening.
Methods: The research is based on the development of a database containing information on mining-geological, geophysical, geodetic and aerospace surveys. The authors of the research use modern geodetic, aerospace and geophysical technologies for scientifically based predicting of technogenic disasters and the rock mass stress-strain state modeling to ensure safe and optimal mining of fields in difficult mining-geological conditions.
Results: The results obtained show that the strengthening of weakened quarry slopes based on the rock mass stress-strain state modeling contributes to the scientific-practical profitability of field mining and provides safe mining in difficult mining-geological conditions.
Discussion: Novelty is in the scientific substantiation of the method for strengthening quarry slopes, which is based on the rock mass stress-strain state modeling. The research is of great practical importance, as the rock mass stress-strain state modeling increases the reliability of predicting the rock mass state during its mining. Strengthening of quarry slopes using the proposed method reduces risks and increases safety and economic efficiency of mining the solid mineral deposits in difficult mining-geological conditions.
A characteristic peculiarity of open-pit mining of mineral deposits is that as the depth of quarries increases, so does the service life of quarry slopes placed in a limiting position. In addition, mining operations are intensified and concentrated, and mining occurs in difficult mining, geological and hydrogeological conditions (Tyo and Zeitinova, 2023; Wang et al., 2023; Zhe et al., 2023). In Kazakhstan, more than 70% of quarries reach a depth of 200 m or more, sometimes up to 700 m (Kassymkanova et al., 2020; Tolovkhan et al., 2023). Therefore, in order to ensure safe and complete mining of deposits, increasing technical-economic performance of the mining enterprise in the conditions of open-pit mining, it is of course necessary to ensure the stability of quarry slopes (Pysmennyi et al., 2020; Taiwo et al., 2023). When planning, it is necessary to reliably determine the optimum parameters of quarry slopes to ensure their long-term stability while minimizing the volume of stripping operations (Rysbekov et al., 2020).
Numerous studies performed to ensure the quarry slope stability require improvement in the present realities due to the rapid development of equipment and technologies (Moldabayev et al., 2021; Bazaluk et al., 2023; Fandy et al., 2024). In Kazakhstan, geomechanical and geodynamic research using modern technologies and methods is conducted at mining enterprises, but technogenic disasters cannot be prevented (Nurpeisova et al., 2021; Tolovkhan et al., 2022; Issatayeva et al., 2023). Modern mining facilities are a kind of laboratories where geomechanical and geodynamic processes are studied using space, geodetic, geological, etc., Methods.
When mining mineral deposits, the rock mass is out of its natural position and to understand the geomechanical processes occurring in it, it is important to conduct studies of fracturing in the rock mass before and during its mining, since with increasing depth of mining operations, the mining-geological conditions become more difficult (Pivnyak et al., 2012; Kalybekov et al., 2020; Malanchuk et al., 2022; Petlovanyi et al., 2023). During large-scale mining operations at Zhezkazgan, Akbakai, Maikain, Maleevsk and other fields, there is a change in the geodynamic regime of the rock mass geological environment, as evidenced by scientific research conducted by a group of scientists of Satbayev University (Aidarbekov et al., 2021; Nurpeissova et al., 2021; Aidarbekov and Istekova, 2022; Sedina et al., 2022).
When ensuring industrial safety of subsoil mining, reliable information on the rock mass state in the subsoil is an urgent task (Bazaluk et al., 2022a; Baltiyeva et al., 2023). In this case, it is necessary to distinguish those factors that have a great influence on the geomechanical processes occurring in the rock mass, in specific mining-geological conditions (Kalybekov et al., 2015; Bazaluk et al., 2022b). Since the stability loss of quarry slopes in their limiting position leads to various kinds of deformations that destroy transport routes in the quarry, disable mining equipment and often lead to human casualties (Sdvyzhkova et al., 2022; Saik et al., 2023). Pipatpongsa et al., (Pipatpongsa et al., 2024), investigates the critical conditions leading to reverse toe sliding in laterally confined low wall slopes subjected to counterweight fill, providing novel insights into enhancing slope stability in mining operations. Modelling stands as a pivotal tool in comprehending and mitigating landslide hazards, offering a nuanced perspective on the intricate dynamics of landslide processes under various triggering factors (Fang et al., 2023).
The rock mass stress-strain state (SSS) changes under the influence of technogenic factors, as well as under the influence of natural factors–current geodynamic movements along tectonic disturbances (Pysmennyi et al., 2022). Thus, the stability of quarry slopes depends on the rock mass SSS. Therefore, control over the rock mass state can be positively solved through innovative monitoring methods, as well as by creating a database of geological, geophysical and geodetic information to model the rock mass SSS during its mining for making managerial decisions on strengthening weakened rock mass areas (Nurpeissova et al., 2020; Kassymkanova et al., 2023a; Kassymkanova et al., 2023b).
To perform the SSS modeling of quarry slopes, it is necessary to have a 3D model of the quarry with a structural characteristic of quarry slopes by the degree of their disturbance (Sdvyzhkova et al., 2020; Zerradi et al., 2023). This model can be derived from performing laser scanning of the quarry (Aukazhieva and Darkenbayeva, 2021; Pasierb et al., 2024). Unmanned aerial survey is used in the analysis of hazardous areas in open-pit mining (Cao et al., 2023; Liu et al., 2024). Laser scanning is a modern survey method that provides high accuracy and density of 3D data points containing information on the spatial structure of quarry slopes. This method is widely used in the mining industry (Li et al., 2023; Zhang et al., 2023).
Earlier terrestrial scanning systems included only laser scanners, but later GPS receivers were added to many models, allowing data to be obtained in an external coordinate system in real time and after processing (Apollo et al., 2023). In contrast, airborne and mobile laser scanning systems are more complex and require additional data preprocessing, but their accuracy is lower compared to terrestrial scanning systems (Medic et al., 2023). Typically, airborne laser scanning systems include the laser scanner itself, GPS-system and Inertial Navigation System (INS), a digital camera and a control unit (Coccia et al., 2022).
The choice of laser scanning method depends on the tasks set and desired data accuracy. Terrestrial laser scanning provides the highest accuracy within 2–5 mm over spatial distances of up to 1 km. Mobile laser scanning has an accuracy of 5 cm, while airborne laser scanning has an accuracy of 15–20 cm. Terrestrial laser scanning is ideal for creating millimeter-accurate 3D models of various objects, such as assessing the quarry slope deformations. In addition, point coordinates derived from traditional geodetic methods can serve as reference points to assess the scan data accuracy, and not only for their correction (Adebiyet et al., 2022; Castillón et al., 2022).
The Zhilandy Group of cupriferous sandstone deposits covers an extensive area and includes the Itauz, West Saryoba, East Saryoba, Kipshakpai, Karashoshak fields and the Kokdombak, Donyzauz, Kulzhan, Airanbay, Taldybulak and Konkuduk ore occurrences, confined to the wings of the northern part of the Zhezkazgan syncline (Skrinnik et al., 2020; Zeylik et al. 2021; Akpanbayeva and Issabek, 2023). About 30 points of the State Geodetic Network (SGN) are located in this area, as well as the developed surveying reference network, which includes several dozens of points. In the area of the East Saryoba field of the Zhilandy Group of cupriferous sandstone deposits, geodetic measurements are conducted at the SGN points located within and outside the East Saryoba field at a distance of about 20 km (Kuttykadamov et al., 2016). Using the satellite positioning method, measurements are performed at 5 SGN points. All coordinates and heights of ground reference marks are determined using the Global Navigation Satellite System (GNSS) by measurements from starting points at a distance of no more than 5 km. Measurements are performed in Static mode, using the network positioning method. The following necessary observation conditions are fulfilled during the work: maximum distance between receivers is 5.0 km; minimum number of observed satellites for processing–8; recording interval–5 s; elevation angle mask is 10°; accuracy of obtaining horizontal coordinates is 0.002 m; in height–0.002 m; GDOP (satellite geometry) is less than 5; duration of observations at the point is 70 min.
The GNSS measurements confirm high efficiency in solving geomechanics problems at mining enterprises in Kazakhstan. They can be used not only for discrete measurements, but also for continuous monitoring of deformations and stresses occurring both in the earth’s crust and in the near-wall rock masses during open-pit mining of mineral deposits.
This manuscript aims to present a novel and systematic approach to managing the quarry slope stability in challenging mining-geological conditions. By integrating advanced geodetic, geological, and geophysical technologies, we aim to develop a comprehensive stress-strain state model of the rock mass at the Vostochny quarry, East Saryoba field. Our methodology emphasizes the practical application of predictive modeling to identify vulnerable areas within quarry slopes and inform strategic decision-making for slope strengthening measures. By integrating multiple data sources and applying cutting-edge techniques, this research contributes to the advancement of sustainable mining practices and enhances the safety and economic viability of mineral mining.
The authors of this paper propose a five-stage modeling method for the rock mass SSS during open-pit mining of the Vostochny quarry, East Saryoba field of the Zhilandy Group of cupriferous sandstones for making managerial decisions on strengthening weakened quarry slope areas placed in a limiting position.
At the first stage, the geological and structural characteristics of Zhilandy Group deposits of cupriferous sandstones are studied according to the Republican Geological Fund reports to create a geophysical-geological information database of the studied object to identify the internal structure and geometrization of the spatial position of tectonic disturbance planes, geological dislocations, zones of heterogeneity and structural rock mass disturbance. Based on the performed analysis of mining-technical and geomechanical documentation of previously conducted research, potential rock mass zones prone to failure are identified due to the redistribution of stresses in the near-wall rock masses during mining of mineral deposits. In addition, the physical-mechanical rock mass properties of the Vostochny quarry, East Saryoba field of the Zhilandy Group of cupriferous sandstones are studied.
At the second stage, laser scanning and UAV (Unmanned Aerial Vehicle) surveying is conducted to perform spatial coordinate referencing to the surveying reference network points of the Vostochny quarry. The study is performed according to the saved SGN coordinates during the intensive mining of the Vostochny quarry using the satellite positioning method. The measurements use GNSS geodetic class satellite navigation equipment: Leica GPS GX-1230 and Leica TS06 plus 5. Measurements are performed statically with a 2-s data recording interval, with receivers located at each of the points delimiting each side of the geodetic network. Based on the obtained coordinate differences in space, changes in line lengths and heights between geodetic network points are calculated. Full vectors of point displacements are then plotted, reflecting the trend movements that occur over this time and the deformations they cause.
Two methods are used to align geodetic measurements. The first method involves centering and orienting the network relative to a “conditionally fixed” point. The second method consists of free network alignment followed by centering and orienting using the least squares method, taking into account minimizing the sum of squares of the displacement vector values.
In the third stage, a 3D quarry with structural characteristic of the quarry slopes by the degree of their disturbance can be obtained by conducting laser scanning of the surface of the quarry slopes placed in a limiting position.
At the fourth stage, a database of actual material on laser scanning and geological-geophysical information of the Vostochny quarry, East Saryoba field of the Zhilandy Group of cupriferous sandstones is created in order to model the natural-technogenic rock mass state to identify the pattern of formation of the near-wall mass destruction zones, taking into account the rock mass SSS and time factor.
At the fifth stage, the rock mass SSS of the Vostochny quarry is modeled.
The sixth stage involves development and patenting a method for strengthening stationary quarry slopes.
The research object is the Vostochny quarry, East Saryoba field of the Zhilandy Group of cupriferous sandstones, which is located in the northern area of the Zhezkazgan ore district (Figure 1).
Figure 1. Location of the study area: Yellow markers–surveyor reference points; pink markers–state geodetic points.
The geological structure of the area involves Precambrian, Poleozoic, Mesozoic and Cenozoic formations. All the Zhilandy Group fields are spatially confined to grey sandstones of the Taskuduk horizon of the Taskuduk Formation and underlying sediments represented by interlayered greenish-grey and grey sandstones, intraformational conglomerates and dark grey or black siltstones and argillites with interlayers of limestone with fauna.
The East Saryoba field, like the rest of the Zhilandy Group fields, is confined to the Zhezkazgan syncline wings. The manifestation of Hercynian tectogenesis has led to the formation of structures here, complicated by longitudinal flexural shear zones and disjunctive faults. The field area is a zone of the highest stresses, which have led to the formation of both folding and discontinuity faults.
The mining-technical conditions of the East Saryoba and West Saryoba fields are characterized by the following indicators.
– ore bodies have a sheet-like shape;
– ores and host rocks are stable and strong;
– by thickness, the ore deposits are low thickness (m<3 m), medium thickness (m = 3–8 m), thick (m = 8–18 m and m>18 m);
– by incidence angle, the deposits are flat-bedding (α<15°), low-inclined (α=15°–20°), inclined (α = 20°–35°) and in flexures represented by steeply inclined occurrence;
– ore is of medium value in terms of content of useful components.
Ore deposits are a sheet-like form of flat-bedding and inclined bedding (90%), as well as steeply inclined bedding and in flexural areas (10%). The thickness of ore bodies varies from 0.6 m to 18.44 m.
Currently, the East Saryoba field is engaged in tunneling and mining operations using both open-pit and underground mining methods.
The ore field of the East Saryoba contains 11 ore deposits with 109 ore bodies explored. The largest deposits are confined to the Taskuduk horizon. They have a north-eastern strike, up to 3,200 m long, 0.5–17 m thick, up to 1,400 m of dip size.
The geological structure is complicated by a series of tectonic faults. The largest of them is the Central Saryoba transpressional fault, which is located between the West and East Saryoba fields. In addition to it, small discontinuities are mapped in the fields.
In this regard, for safe mining of the field, it is necessary to conduct geomechanical research on a permanent basis using various methods, including surveying and geodetic studies. Based on geospatial data obtained from surveying and geodetic studies, geological models of fields can be developed to determine the direction of structures and characteristics of deposits.
To obtain a three-dimensional model of the quarry walls to identify deformed areas, terrestrial laser scanning is applied at the Vostochny quarry. In order to obtain a three-dimensional model of the Vostochny quarry with millimeter accuracy, surveying and geodesic studies are conducted in the area of determining the coordinates and heights of the surveying reference network points at the quarry using Global Navigation Satellite System (GNSS) technologies. Subsequently, the digitized contours of the Vostochny quarry are used to develop a geomechanical model. Satellite measurements are performed in Static mode, and the work in this mode implies conditional subdivision into two stages: field work and office studies.
The following necessary observation conditions are fulfilled during the work: maximum distance between receivers is 5.0 km; minimum number of observed satellites for processing–8; recording interval–5 s; elevation angle mask is 10°; accuracy of obtaining horizontal coordinates is 0.002 m; in height–0.002 m; GDOP (satellite geometry) is less than 5; duration of observations at the point is 80 min.
Triangulation points of the State Geodetic Network provided by the branch of the National Centre of Geodesy and Spatial Information are used as starting points. The Burovaya State Geodetic Network point is used as a base point and for conversion to WGS 84. Initial data are given in Table 1.
Table 1. This is a table. Tables should be placed in the main text near to the first time they are cited.
Point data after balancing and determination of conversion parameters are given in Table 2.
The Burovaya State Geodetic Network point is chosen as the starting point for installing the base reference station. All static measurements are made using the baseline to a given point. The scheme for conducting observations is shown in Figure 2.
Field data from points of International Ground Station (IGS) world network are included in post-processing to obtain precise coordinates and altitudes (Orynbassarova et al., 2022). Processing and equalization of this network is performed at the points on this network on a permanent basis. Referencing of points to the above-mentioned network provides high accuracy and consistency of the obtained coordinates in the WGS84 system. Also, to improve the accuracy of the final results before processing, the project includes such data as accurate satellite ephemerides, ionospheric maps, tropospheric state maps and updated satellite clocks for the period of field work. Parameters for conversion from WGS-84 to the local coordinate and height system are given in Figure 3.
Reference bases are established at the Poselkovyiy and Burovaya State Geodetic Network points. The balanced coordinates of points are taken as the initial data, according to certain parameters for conversion from WGS-84 to the local system of coordinates and heights, which are the geodetic basis for performing terrestrial laser scanning, the results of which form the basis for SSS modeling of the Vostochny quarry slopes, East Saryoba field of the Zhilandy Group of cupriferous sandstones.
Scanning is conducted in safe areas of shedding walls, as well as in areas that the STONEX300 terrestrial scanner is capable of capturing. The quarry is also surveyed using a UAV. In this case, the MATRICE 300 RTK, designed on the basis of an updated hardware and software platform and equipped with a wide range of functions based on artificial intelligence, is used. The drone is equipped with a built-in RTK module that provides the most accurate course data for positioning. In addition, more accurate positioning data is also provided by using the high-precision mobile DJI D-RTK two satellite positioning systems in conjunction with UAV.
The UAV imagery is processed in Agisoft Metashape. The scanner imagery is processed in Reconstructor. Both images are merged into one by the Cloud Compare software. The point cloud and 3D model are created based on two measuring tools (scanner + UAV) and are presented in Figure 4.
Figure 4. 3D model of the Vostochny quarry, East Saryoba field of the Zhilandy Group of cupriferous sandstones.
A 3D model of the Vostochny quarry has been obtained based on the instrumental survey of the quarry using a Stonex 300 laser scanner and MATRICE 300 RTK industrial flight platform.
Digitized contours of the Vostochny quarry, East Saryoba field of the Zhilandy Group of cupriferous sandstones are used to construct the geomechanical model (Figure 4). A homogeneous 3D finite-element model of the field has been created by combining these contours in reference to the coordinate system. When modeling a three-dimensional mass area, the boundary conditions are determined by fixing the displacements of the model.
– the XY plane (model bottom) is fixed in the X, Y and Z-axes;
– the YZ plane is fixed in the X and Y-axes;
– the XZ plane is fixed in the Y-axis.
The finite element mesh of the model (Figure 5) has been obtained by uniformly partitioning the domain into 10-node tetrahedra. The number of elements for the model below is 767,552.
To determine the rock mass SSS and the main characteristic determining the stability of the quarry walls–the safety factor (SF), a well-tested numerical finite element method is used, implemented in the licensed RS3 software (Rocscience, Canada).
The solution is performed in the elastic-plastic formulation using The Mohr–Coulomb (MC) failure criterion (Eq. 1):
where τ–tangential stress (shear stress), MPa; σn–normal stress, MPa; φ–internal friction angle, deg; с–cohesion, MPa.
SF is the most significant numerical modeling result, indicating that the wall can be operated at specified geometric parameters (Eq. 2):
The “strength reduction” procedure, used in the RS3 code, is well adapted for determining the SF value. If the slope is in a limiting state, the Mohr–Coulomb failure criterion can be represented as follows (Eq. 3):
Thus, the slope safety factor is the value of F by which the initial rock shear resistance parameters should be divided in order for the slope to fail. This interpretation makes it possible to determine the safety factor by consistently reducing the real strength characteristics of the rocks composing the slope by F times to such “reduced” (factorized) cf and ϕf values, at which the slope stability with a given geometry becomes impossible, that is, the slope failure occurs. Mathematically, this means a divergence of the iteration process in the numerical solution. When the algorithm fails to converge within the maximum number of iterations set by the user, no stress distribution can be obtained that simultaneously satisfies both the Mohr–Coulomb failure criterion and the global equilibrium condition.
During a visual inspection of the Vostochny quarry slopes, East Saryoba field of the Zhilandy Group of cupriferous sandstones, weakened areas have been noted (Figure 6). Physical-mechanical properties of the weakened Vostochny quarry slope area with a height of 15 m according to geological exploration data are given in Table 3.
Figure 6. Weakened area of the Vostochny quarry, East Saryoba field of the Zhilandy Group of cupriferous sandstones.
Physical-mechanical properties of the Vostochny quarry slope weakened area (Table 3) are used to develop a 2D model (Figure 7).
Figure 7. 2D model geometry with indication of rock types: 1–limestone; 2–limestone mass; 3–sandstone; 4–red limestone; five–ore.
The imported section from the RS3 program into RS2 and the drawing of material lines to separate rock layers are shown in Figure 7 with the indication of rock types. The calculation scheme of the model with boundary conditions and partitioning into triangular finite elements is presented in Figure 8.
Thus, the divergence of the iteration process in the numerical solution physically means slope failure. The value of the strength reduction factor F, at which collapse in the solution occurs, is taken as the slope safety factor.
Performed calculations show that the south-western area is the most potentially hazardous area of the Vostochny quarry, East Saryoba field of the Zhilandy Group of cupriferous sandstones (Figure 9A). However, in this case, it is possible to speak only about a “potential” hazard since the SF exceeds 3 (SF ≥ 3). To identify a potentially hazardous slip surface, a 3D model section is made along the most hazardous area (Figure 9B). The potential slip surface is presented in Figure 10A,B.
Figure 9. Displacement distribution in the Vostochny quarry model (A) and creation of a plane section to determine the slip surface (B).
Figure 10. Slip surface modeling results: (A)–expressed in displacements; (B)–expressed in maximum shear deformations.
Research conducted on a homogeneous macro-level model has shown that, taking into account only the quarry geometry factor and physical-mechanical properties (Table 3) of the rock mass at the current stage of mining operations, the south-western area of the Vostochny quarry is potentially hazardous. In this case, it is possible to speak only of a “potential” hazard, as the safety factor is 3.53.
The results of finite element modeling are given in Figure 11 and Figure 12. The safety factor decreases to the value of SF = 3.39 when the problem is solved in two-dimensional formulation and physical-mechanical properties of rock layers are taken into account (Table 3). Taking this value as a safety factor, it is this value that should be considered when developing methods for quarry wall protection. This result correlates well with studies conducted in (Wang et al., 2023), which show that the transition to a two-dimensional layered model leads to a decrease in SF by no more than 10%.
Figure 11 and Figure 12 show the total displacements and maximum shear deformations for the section of the most potentially hazardous area. As with the three-dimensional problem, the largest potential displacements are to be expected in the western (left) area of the slope, where the resulting angle is 390° (300° in the right wall). The safety factor (SF) of the quarry wall, calculated based on the Strength Reduction procedure described above, is 3.39. This means that the displacements shown in Figure 11 as well as the potential slip line (Figure 12), will occur if the strength characteristics are reduced by 3.39 times. In the real situation, the quarry wall is quite stable.
The pattern of displacements and maximum shear deformations is caused by the area geometry and by the fact that the slope angle exceeds the internal friction angle of the rocks. Zones of increased shear deformations and displacements within the mass are explained by the presence of contacts of rock layers having different physical-mechanical properties.
After identifying the Vostochny quarry weakened areas by means of SSS modeling, a method for strengthening stationary quarry slopes has been developed and patented (Rysbekov et al., 2023). The essence of the method is to create a crushing zone by drilling shielding inclined wells parallel to the future slope surface and blasting them, with additional drilling of wells, installing reinforcement in them and filling them with strengthening solution. This method differs from existing ones in that additional wells are inclined and drilled between inclined shielding wells at approximately the same angle as the shielding inclined wells. It is important that the filling with strengthening solution is performed after the crushing zone has been created. In this case, the reinforcement of additional inclined wells is installed in such a way that it is adjacent to the well wall from the side of the future slope surface. After removing the blasted mass and cleaning the surface of the slope, rods or hooks are welded to the reinforcement of additional inclined wells, on which a metal mesh is fixed over the entire slope surface. Then a layer of strengthening solution is applied to it.
To verify this method for strengthening stationary quarry slopes, a 3D geomechanical model of the slope located at an angle of 60° to the horizontal surface is constructed. The calculation scheme for the corresponding finite element model with the indication of boundary conditions and finite element partitioning is given in Figure 13.
Physical-mechanical rock mass properties are used for modeling (Table 3). The results of modeling the slope without strengthening are shown in Figure 14.
Figure 14. Results of modeling the slope without strengthening: (A) potential displacements; (B) maximum shear deformations.
The Strength Reduction procedure for the area presented in Figure 14A results in a strength reduction factor of 3.83. It is this value that is considered as a safety factor. Thus, for the slope without strengthening, SF = 3.83 at the resulting slope angle of 60°. The area of maximum shear deformations determining the potential slip surface, which is hypothetical to occur at this stage of mining due to a 3.83-fold decrease in the mechanical properties of the rocks, is shown in Figure 14B.
Standard support tools implemented in the RS3 software package are used for modeling the strengthening of stationary quarry slopes according to the developed and patented method. Drilling additional wells, installing reinforcement in them and filling them with strengthening solution are simulated using the Bolt tool according to the method described in (Tereshchuk et al., 2018).
Figure 15 shows the scheme after this modeling stage has been performed. When blasting shielding wells, when the quarry slope is placed in a limiting position, with a mesh of 3 m between inclined wells, half of the well remains on the quarry slope. The reinforcement is placed in this recess. Then, according to the strengthening method, a metal mesh is attached to the reinforcement of inclined wells over the entire slope surface and then a layer of strengthening solution is applied to it. This stage is simulated using the Lining tool. The use of this tool is shown in Figure 15A. The calculation scheme of the finite element model simulating a new method for strengthening slopes with indication of boundary conditions and finite element partitioning is given in Figure 15B.
Figure 15. Scheme for reinforcement installation and application of strengthening solution (A) and calculation scheme of the model simulating the new slope strengthening method (B).
The modeling results of the new slope strengthening method are shown in Figure 16.
Figure 16. Strengthened slope modeling results: (A) potential displacements; (B) maximum shear deformations.
The safety factor after slope strengthening increases by 21% to a value of 4.61, indicating the significant effectiveness of the proposed slope strengthening method.
To study the rock cohesion influence on the effectiveness of the proposed method of slope strengthening, seven options for calculating of this slope stability have been performed. The cohesion varies in steps of 100 KPa from a value of 150–750 KPa. The multi-variant modeling results are shown in Table 4. The interpretation of the multi-variant modeling results is graphically summarized in Figure 17.
When generalizing the modeling results, a regression dependence of the new method effectiveness on cohesion of the rocks composing the slope has been obtained (Eq. 4):
where K–rock cohesion (КPа).
Apply this technology to a quarry-scale model. Model the strengthened area in the south-western wall of the quarry, as shown in Figure 18A. Modeling results are given in Figure 18B.
Figure 18. Strengthened slope modeling: (A)–calculation scheme of finite element analysis on a quarry-scale with a strengthened area; (B)–potential displacements.
The analysis of the modeling results of the new slope strengthening method on a quarry-scale shows a significant increase in the safety factor of the quarry wall up to the value of SF = 5.19.
The methodology is unique in that it combines social and economic significance. Social significance is primarily related to the prevention of technogenic disasters associated with the development of negative geomechanical processes. Strengthening unstable quarry slopes, placed in a limiting position, will prevent large deformations, thereby saving the lives of workers, engineers and technicians mining minerals at great depths. Economic significance–timely issued prediction on unfavorable geomechanical situation of quarry slopes and its timely strengthening will save enormous material costs for handling the consequences of technogenic disasters in subsoil use.
Taking into account the stress state of rocks in the near-wall mass, it is possible to obtain more objective information on the formation of a possible sliding wedge in different periods of quarry operation. This approach allows, even at the design stage, to model geomechanical processes and their possible negative manifestations in the form of landslides and to determine the stability parameters of quarry slopes, taking into account its service life.
The main principal difference of the performed scientific research is the modeling of rock mass SSS to assess and predict the deformation processes of quarry slopes, based on geospatial data, namely, high-precision measurements, terrestrial laser scanning, unmanned aerial vehicle for making managerial decisions to ensure industrial reliability in subsoil mining.
As a result of the research performed, in the future it is planned to study the dynamics of change in geotechnical processes on the basis of geospatial data obtained during subsoil mining with the development of a methodology for observing geotechnical and geodynamic processes, taking into account the patterns of manifestation of the measured values. This will improve the accuracy, reliability and efficiency of determining the rate of development of the rock mass deformation process. It is planned to develop an integrated method that will make it possible to monitor the stability of mine workings and improve subsoil mining safety and mining efficiency.
To determine the rock mass SSS and the main characteristic determining the stability of the quarry walls–the safety factor (SF), a well-tested numerical finite element method is used, implemented in the licensed RS3 software (Rocscience, Canada).
Research conducted on a homogeneous macro-level model has shown that, taking into account only the quarry geometry factor and the averaged physical-mechanical properties of the rock mass at the current stage of mining operations, the south-western area of the Vostochny quarry is potentially hazardous. In this case, it is possible to speak only of a “potential” hazard, as the safety factor is 3.53.
The safety factor decreases to the value of SF = 3.39 when the problem is solved in two-dimensional formulation and physical-mechanical properties of rock layers are taken into account. It is this value that should be taken into account when developing methods for quarry wall protection.
After identifying weakened Vostochny quarry areas by means of SSS modeling, a method for strengthening stationary quarry slopes has been developed and patented. On the one hand, it can provide the necessary bench slope stability for the non-working quarry walls and, in some cases, prevent possible rock failures in weakened areas. On the other hand, it can significantly reduce the volume of stripping operations. The analysis of modeling results has shown that the overall effectiveness of the new slope strengthening method is 20%–25% of the increase in SF, while the lower the rock cohesion, the higher the proposed method effectiveness.
Our study not only enhances the understanding of quarry slope stability, but also provides actionable insights to improve the safety and efficiency of mining operations. By integrating advanced geodetic, geological, and geophysical technologies, our methodology offers a systematic approach to identifying and mitigating risks associated with weakened quarry areas. Moreover, the development and patenting of a novel methodology for strengthening stationary quarry slopes underscore the significance of the research. Ultimately, our research contributes to the advancement of sustainable mining practices, minimizing environmental impact while ensuring the long-term viability of mining operations.
The original contributions presented in the study are included in the article/supplementary material, further inquiries can be directed to the corresponding authors.
PS: Conceptualization, Funding acquisition, Investigation, Project administration, Writing–original draft. KR: Conceptualization, Funding acquisition, Investigation, Methodology, Supervision, Writing–original draft. K-KK: Conceptualization, Funding acquisition, Investigation, Methodology, Project administration, Writing–original draft. VL: Funding acquisition, Investigation, Validation, Writing–original draft, Writing–review and editing. GK: Software, Validation, Visualization, Writing–review and editing. SM: Formal Analysis, Resources, Validation, Writing–review and editing. DB: Data curation, Software, Visualization, Writing–review and editing. AS: Data curation, Formal Analysis, Resources, Writing–review and editing.
The author(s) declare that financial support was received for the research, authorship, and/or publication of this article. This study was carried out as part of the project “Belt and Road Initiative Center for Chinese-European studies (BRICCES)” and was funded by the Guangdong University of Petrochemical Technology. The research was conducted with the financial support of Committee of Science of the Ministry of Science and Higher Education of the Republic of Kazakhstan within the framework of program-target financing BR21881939 “Development of resource-saving energy-generating technologies for mining-metallurgical complex and creation of innovative engineering center” and the No. AP14871828 grant “Research and development of highly effective methodology for monitoring the geotechnical rock mass condition for assessment and prediction of deformation processes during field mining”.
We extend our gratitude to all researchers and scholars whose work has influenced and inspired this research, as well as the reviewers whose constructive feedback has helped improve the quality of this paper.
Author AS was employed by LLP NPK “Algeorithm”.
The remaining authors declare that the research was conducted in the absence of any commercial or financial relationships that could be construed as a potential conflict of interest.
All claims expressed in this article are solely those of the authors and do not necessarily represent those of their affiliated organizations, or those of the publisher, the editors and the reviewers. Any product that may be evaluated in this article, or claim that may be made by its manufacturer, is not guaranteed or endorsed by the publisher.
Adebiyet, B., Iliuf, F. A., Orynbassarova, E., Chernov, A., and Idrissov, K. (2022). 3D Modeling of Satbayev University based on laser scanning and UAV data. Int. Archives Photogrammetry, Remote Sens. Spatial Inf. Sci. 46, 1–6. doi:10.5194/isprs-archives-XLVI-5-W1-2022-1-2022
Aidarbekov, Z. K., and Istekova, S. A. (2022). Classification of geophysical fields in the study of geological and structural features of the zhezkazgan ore district. News Natl. Acad. Sci. Repub. Kazakhstan, Ser. Geol. Tech. Sci. 2 (452), 33–48. doi:10.32014/2022.2518-170x.158
Aidarbekov, Z. K., Istekova, S. A., and Glass, H. (2021). Complex of geophysical research for studying geological structure of Zhezkazgan ore region in Kazakhstan. Eng. Min. Geophys. 2021 (1), 1–9. doi:10.3997/2214-4609.202152070
Akpanbayeva, A., and Issabek, T. (2023). Assessing a natural field of rock mass stress by means of in-situ measurements within Vostochnaya Sary-Oba deposit in Kazakhstan. Min. Mineral Deposits 17 (3), 56–66. doi:10.33271/mining17.03.056
Apollo, M., Jakubiak, M., Nistor, S., Lewińska, P., Krawczyk, A., Borowski, Ł., et al. (2023). Geodata in science-a review of selected scientific fields. Acta Sci. Pol. Form. Circumiectus 22 (2), 17–40. doi:10.15576/ASP.FC/2023.22.2.02
Aukazhieva, Z. M., and Darkenbayeva, A. B. (2021). Definition and theoretical basis of laser scanning. Eng. J. Satbayev Univ. 143 (2), 52–57. doi:10.51301/vest.su.2021.i2.07
Baltiyeva, A., Orynbassarova, E., Zharaspaev, M., and Akhmetov, R. (2023). Studying sinkholes of the earth’s surface involving radar satellite interferometry in terms of Zhezkazgan field, Kazakhstan. Min. Mineral Deposits 17 (4), 61–74. doi:10.33271/mining17.04.061
Bazaluk, O., Anisimov, O., Saik, P., Lozynskyi, V., Akimov, O., and Hrytsenko, L. (2023). Determining the safe distance for mining equipment operation when forming an internal dump in a deep open pit. Sustainability 15 (7), 5912. doi:10.3390/su15075912
Bazaluk, O., Ashcheulova, O., Mamaikin, O., Khorolskyi, A., Lozynskyi, V., and Saik, P. (2022b). Innovative activities in the sphere of mining process management. Front. Environ. Sci. 10 (10), 878977. doi:10.3389/fenvs.2022.878977
Bazaluk, O., Rysbekov, K., Nurpeisova, M., Lozynskyi, V., Kyrgisbayeva, G., and Turumbetov, T. (2022a). Integrated monitoring for the rock mass state during large-scale subsoil development. Front. Environ. Sci. 10, 329. doi:10.3389/fenvs.2022.852591
Cao, D., Zhang, B., Zhang, X., Yin, L., and Man, X. (2023). Optimization methods on dynamic monitoring of mineral reserves for open pit mine based on UAV oblique photogrammetry. Measurement 207, 112364. doi:10.1016/j.measurement.2022.112364
Castillón, M., Forest, J., and Ridao, P. (2022). Underwater 3D scanner to counteract refraction: calibration and experimental results. IEEE/ASME Trans. Mechatronics 27 (6), 4974–4982. doi:10.1109/TMECH.2022.3170504
Coccia, S., Al Heib, M., and Klein, E. (2022). “Combination of UAV-borne lidar and UAV-borne photogrammetry to assess slope stability,” in Proceedings of the 7 th World Congress on Civil, Structural, and Environmental Engineering, Lisbon, Portugal, April 10 - 12, 2022, 1–8. doi:10.11159/icgre22.107
Fandy, P. M., Hutagalung, M. J., Tambing, F. S., Sahetapy, G. B., and Sanggamele, H. (2024). Analysis of limestone quarry slope stability, nimbokrang district, jayapura regency, papua, Indonesia. J. Sains Dan. Teknol. 5 (3), 763–768. doi:10.55338/saintek.v5i3.2375
Fang, K., Tang, H., Li, C., Su, X., An, P., and Sun, S. (2023). Centrifuge modelling of landslides and landslide hazard mitigation: a review. Geosci. Front. 14 (1), 101493. doi:10.1016/j.gsf.2022.101493
Issatayeva, F. M., Aubakirova, G. M., Maussymbayeva, A. D., Togaibayeva, L. I., Biryukov, V. V., and Vechkinzova, E. (2023). Fuel and energy complex of Kazakhstan: geological and economic assessment of enterprises in the context of digital transformation. Energies 16 (16), 6002. doi:10.3390/en16166002
Kalybekov, T., Rysbekov, K., Nаuryzbayeva, D., Toktarov, A., and Zhakypbek, Y. (2020). Substantiation of averaging the content of mined ores with account of their readiness for mining. E3S Web Conf. 201, 01039. doi:10.1051/e3sconf/202020101039
Kalybekov, T., Rysbekov, K., and Zhakypbek, Y. (2015). Efficient land use in open-cut mining. New Dev. Min. Eng. 2015 Theor. Pract. Solutions Mineral Resour. Min., 287–291. doi:10.1201/b19901-51
Kassymkanova, K. K., Istekova, S., Rysbekov, K., Amralinova, B., Kyrgizbayeva, G., Soltabayeva, S., et al. (2023a). Improving a geophysical method to determine the boundaries of ore-bearing rocks considering certain tectonic disturbances. Min. Mineral Deposits 17 (1), 17–27. doi:10.33271/mining17.01.017
Kassymkanova, K. K., Jangulova, G., Issanova, G., Turekhanova, V., and Zhalgasbekov, Y. (2020). Geomechanical processes and their assessment in the rock massifs in Central Kazakhstan. United States: Springer. doi:10.1007/978-3-030-33993-7
Kassymkanova, K. K., Rysbekov, K. B., Nurpeissova, M. B., Kyrgizbayeva, G. M., Amralinova, B. B., Soltabaeva, S. T., et al. (2023b). Geophysical studies of rock distortion in mining operations in complex geological conditions. Int. Archives Photogrammetry, Remote Sens. Spatial Inf. Sci. (48), 57–62. doi:10.5194/isprs-archives-XLVIII-5-W2-2023-57-2023
Kuttykadamov, M. E., Rysbekov, K. B., Milev, I., Ystykul, K. A., and Bektur, B. K. (2016). Geodetic monitoring methods of high-rise constructions deformations with modern technologies application. J. Theor. Appl. Inf. Technol. 93 (1), 24–31.
Li, S., Zhao, Z., Hu, B., Yin, T., Chen, G., and Chen, G. (2023). Three-dimensional simulation stability analysis of slopes from underground to open-pit mining. Minerals 13 (3), 402. doi:10.3390/min13030402
Liu, Y., Ma, C., Zhan, L., Fan, Z., Song, S., and Zeng, Y. (2024). Monitoring and evaluation method for cross border mining in open-pit mines based on drone technology. China Min. Mag. 33 (1), 105–113. doi:10.12075/j.issn.1004-4051.20230676
Malanchuk, Y., Moshynskyi, V., Khrystyuk, A., Malanchuk, Z., Korniienko, V., and Abdiev, A. (2022). Analysis of the regularities of basalt open-pit fissility for energy efficiency of ore preparation. Min. Mineral Deposits 16 (1), 68–76. doi:10.33271/mining16.01.068
Medic, T., Bömer, J., and Paulus, S. (2023). Challenges and recommendations for 3d plant phenotyping in agriculture using terrestrial lasers scanners. ISPRS annals of the photogrammetry. Remote Sens. Spatial Inf. Sci. 10, 1007–1014. doi:10.5194/isprs-annals-X-1-W1-2023-1007-2023
Moldabayev, S. K., Sdvyzhkova, O. O., Babets, D. V., Kovrov, O. S., and Adil, T. K. (2021). Numerical simulation of the open pit stability based on probabilistic approach. Nauk. Visnyk Natsionalnoho Hirnychoho Universytetu (6), 29–34. doi:10.33271/nvngu/2021-6/029
Nurpeisova, M. B., Bitimbayev, M.Zh., Rysbekov, K. B., and Bekbasarov, Sh.Sh. (2021). Forecast changes in the geodynamic regime of geological environment during large-scale subsoil development. Nauk. Visnyk Natsionalnoho Hirnychoho Universytetu (6), 5–10. doi:10.33271/NVNGU/2021-6/005
Nurpeissova, M., Bitimbayev, M. Z., Derbisov, K., Тurumbetov, Т., and Shults, R. (2020). Geodetic substantiation of the Saryarka copper ore region. News Natl. Acad. Sci. Repub. Kazakhstan, Ser. Geol. Tech. Sci. 6 (444), 194–202. doi:10.32014/2020.2518-170x.147
Nurpeissova, M., Rysbekov, К., Levin, Е., Derbisov, K., and Nukarbekova, Z. (2021). Study of slow motions of the earth surface. Eng. J. Satbayev Univ. 143 (5), 3–9. doi:10.51301/vest.su.2021.i5.01
Orynbassarova, E., Yerzhankyzy, A., Shults, R., Roberts, K., and Togaibekov, A. (2022). Strategies of GNSS processing and measuring under various operational conditions. Nauk. Visnyk Natsionalnoho Hirnychoho Universytetu (3), 146–150. doi:10.33271/nvngu/2022-3/146
Pasierb, B., Gajek, G., Urban, J., and Nawrocki, W. (2024). Integrated geophysical and geomorphological studies of caves in calcarenite limestones (jaskinia pod świecami cave, Poland). Surv. Geophys. 45, 663–694. doi:10.1007/s10712-023-09821-9
Petlovanyi, M., Saik, P., Lozynskyi, V., Sai, K., and Cherniaiev, O. (2023). Substantiating and assessing the stability of the underground system parameters for the Sawn Limestone Mining: case study of the Nova Odesa Deposit, Ukraine. Inżynieria Miner. 1 (1), 79–89. doi:10.29227/IM-2023-01-10
Pipatpongsa, T., Fang, K., Leelasukseree, C., Chaiwan, A., and Chanwiset, N. (2024). Reverse toe sliding criteria of laterally confined low wall slope subjected to counterweight fill. Int. J. Rock Mech. Min. Sci. 175, 105683. doi:10.1016/j.ijrmms.2024.105683
Pivnyak, G., Bondarenko, V., Kovalevs’ka, I., and Illiashov, M. (2012). Geomechanical processes during underground mining. Abingdon, Oxfordshire: Routledge.
Pysmennyi, S., Fedko, M., Chukharev, S., Rysbekov, K., Kyelgyenbai, K., and Anastasov, D. (2022). Technology for mining of complex-structured bodies of stable and unstable ores. IOP Conf. Ser. Earth Environ. Sci. 970 (1), 012040. doi:10.1088/1755-1315/970/1/012040
Pysmennyi, S., Fedko, M., Shvaher, N., and Chukharev, S. (2020). Mining of rich iron ore deposits of complex structure under the conditions of rock pressure development. E3S Web Conf. 201, 01022. doi:10.1051/e3sconf/202020101022
Rysbekov, K., Toktarov, A., Kalybekov, T., Moldabayev, S., Yessezhulov, T., and Bakhmagambetova, G. (2020). Mine planning subject to prepared ore reserves rationing. E3S Web Conf. 168, 00016. doi:10.1051/e3sconf/202016800016
Rysbekov, K. B., Nurpeisova, M. B., Kasymkanova, Kh.M., Kyrgizbaeva, G. M., Soltabaeva, S. T., Amralinova, B. B., et al. (2023). RGP «Natsionalnyi institut intellektualnoi sobstvennosti» Ministerstva yustitsii Respubliki Kazakhstan.
Saik, P., Cherniaiev, O., Anisimov, O., and Rysbekov, K. (2023). Substantiation of the direction for mining operations that develop under conditions of shear processes caused by hydrostatic pressure. Sustainability 15 (22), 15690. doi:10.3390/su152215690
Sdvyzhkova, O., Dmytro, B., Moldabayev, S., Rysbekov, K., and Sarybayev, M. (2020). Mathematical modeling a stochastic variation of rock properties at an excavation design. Int. Multidiscip. Sci. GeoConference Surv. Geol. Min. Ecol. Manag. SGEM (1.2), 165–172. doi:10.5593/sgem2020/1.2/s03.021
Sdvyzhkova, O., Moldabayev, S., Bascetin, A., Babets, D., Kuldeyev, E., Sultanbekova, Z., et al. (2022). Probabilistic assessment of slope stability at ore mining with steep layers in deep open pits. Min. Mineral Deposits 16 (4), 11–18. doi:10.33271/mining16.04.011
Sedina, S., Altayeva, A., Shamganova, L., and Abdykarimova, G. (2022). Rock mass management to ensure safe deposit development based on comprehensive research within the framework of the geomechanical model development. Min. Min. Depos. 16 (2), 103–109. doi:10.33271/mining16.02.103
Skrinnik, L., Gadeev, R., Umarbekova, Z., and Tsretyakov, A. (2020). Collisional and orogenic granitoids of Kazakhstan. Int. Multidiscip. Sci. GeoConference SGEM 20 (1.1), 51–66. doi:10.5593/sgem2020/1.1/s01.006
Taiwo, B. O., Yewuhalashet, F., Ogunyemi, O. B., Babatuyi, V. A., Okobe, E. I., and Orhu, E. A. (2023). Quarry slope stability assessment methods with blast induced effect monitoring in akoko edo, Nigeria. Geotechnical Geol. Eng. 41 (4), 2553–2571. doi:10.1007/s10706-023-02414-8
Tereshchuk, R. M., Khoziaikina, N. V., and Babets, D. V. (2018). Substantiation of rational roof-bolting parameters. Nauk. Visnyk Natsionalnoho Hirnychoho Universytetu 1 (1), 19–26. doi:10.29202/nvngu/2018-1/18
Tolovkhan, B., Demin, V., Amanzholov, Zh., Smagulova, A., Tanekeyeva, G., Zairov, Sh., et al. (2022). Substantiating the rock mass control parameters based on the geomechanical model of the Severny Katpar deposit, Kazakhstan. Min. Mineral Deposits 16 (3), 123–133. doi:10.33271/mining16.03.123
Tolovkhan, B., Smagulova, A., Khuangan, N., Asainov, S., Issagulov, S., Kaumetova, D., et al. (2023). Studying rock mass jointing to provide bench stability while Northern Katpar deposit developing in Kazakhstan. Min. Mineral Deposits 17 (2), 99–111. doi:10.33271/mining17.02.099
Tyo, S., and Zeitinova, S. (2023). Optimizing the contours of open pit mining with the use of mining and geological information systems and technologies. Kompleks. Ispolzovanie Miner. Syra 327 (4), 50–56. doi:10.31643/2023/6445.39
Wang, F., Ren, Q., Jiang, X., Niu, J., and Chen, B. (2023). Engineering geology and mechanism of multiple landslides in a large open-pit mine: the case of the Copper Mine in Qinghai Province, China. Bull. Eng. Geol. Environ. 82 (4), 147. doi:10.1007/s10064-023-03186-4
Zerradi, Y., Souissi, M., and Larabi, A. (2023). Application of the deterministic block theory to the slope stability design of an open-pit mine in Morocco. Min. Mineral Deposits 17 (2), 53–60. doi:10.33271/mining17.02.053
Zeylik, B., Arshamov, Y., Baratov, R., and Bekbotayeva, A. (2021). New technology for mineral deposits prediction to identify prospective areas in the Zhezkazgan ore region. Rozrobka Rodovyshch 15 (2), 134–142. doi:10.33271/mining15.02.134
Zhang, S., Chen, W., and Guo, Z. (2023). Study on the spatial regularity of rammed pits of the Ming Great Wall using 3D scanning technique and Random Forest algorithm. J. Cult. Herit. 62, 230–241. doi:10.1016/j.culher.2023.05.033
Keywords: slope, modeling, stress-strain state, rock mass, quarry, geomechanics, laser scanning
Citation: Saik P, Rysbekov K, Kassymkanova K-K, Lozynskyi V, Kyrgizbayeva G, Moldabayev S, Babets D and Salkynov A (2024) Investigation of the rock mass state in the near-wall part of the quarry and its stability management. Front. Earth Sci. 12:1395418. doi: 10.3389/feart.2024.1395418
Received: 03 March 2024; Accepted: 04 June 2024;
Published: 02 July 2024.
Edited by:
Wen Nie, Jiangxi University of Science and Technology, ChinaReviewed by:
Xuexue Su, Anhui University of Science and Technology, ChinaCopyright © 2024 Saik, Rysbekov, Kassymkanova, Lozynskyi, Kyrgizbayeva, Moldabayev, Babets and Salkynov. This is an open-access article distributed under the terms of the Creative Commons Attribution License (CC BY). The use, distribution or reproduction in other forums is permitted, provided the original author(s) and the copyright owner(s) are credited and that the original publication in this journal is cited, in accordance with accepted academic practice. No use, distribution or reproduction is permitted which does not comply with these terms.
*Correspondence: Kanay Rysbekov, Sy5SeXNiZWtvdkBzYXRiYXlldi51bml2ZXJzaXR5; Vasyl Lozynskyi, bHZnLm5tdUBnbWFpbC5jb20=
Disclaimer: All claims expressed in this article are solely those of the authors and do not necessarily represent those of their affiliated organizations, or those of the publisher, the editors and the reviewers. Any product that may be evaluated in this article or claim that may be made by its manufacturer is not guaranteed or endorsed by the publisher.
Research integrity at Frontiers
Learn more about the work of our research integrity team to safeguard the quality of each article we publish.