- 1Istituto Nazionale di Geofisica e Vulcanologia, Sezione di Napoli, Osservatorio Vesuviano, Napoli, Italy
- 2Ministero della Cultura, Parco Archeologico di Pompei, Pompei, Italy
- 3Istituto Nazionale di Geofisica e Vulcanologia, Sezione di Roma 1, Roma, Italy
- 4Independent Researcher, Pompei, Italy
The ancient city of Pompeii, destroyed by the 79 CE Plinian eruption of Vesuvius, is one of the most famous archaeological sites worldwide and an open-air laboratory for many disciplines. The destruction of Pompeii has so far been reconstructed in terms of a succession of volcanic phenomena and related effects, identified as the accumulation of pumice lapilli on roofs and dynamic pressure exerted by pyroclastic currents on buildings, and neglecting the potential effects of the syn-eruptive seismicity, the occurrence of which is beautifully described by an erudite eyewitness to the catastrophe, Pliny the Younger. During a recent excavation in the Insula dei Casti Amanti, in the central part of Pompeii, the peculiar evidence of building collapses, that overwhelmed two individuals, has been uncovered. The multidisciplinary investigation, involving archaeology, volcanology, and anthropology, gathered information on the construction technique of the masonry structures, the volcanological stratigraphy, the traumatic pattern of bone fractures of the skeletons, along with the detection of the wall displacements, that led to archaeoseismological considerations. The merging of the data has highlighted the need of an updated perspective in the assessment of the damage at Pompeii during the 79 CE eruption, by considering the syn-eruptive seismicity as a factor contributing to the destruction of the city and death of the inhabitants. By comparing the attitude and characteristics of different types of damage, and after ruling out any other possible damaging event, our conclusions point to the occurrence of syn-eruptive earthquake-induced failures of masonry structures. The structural collapses, based on our stratigraphic and volcanological data, are chronologically consistent with the beginning of the caldera-forming phase of the eruption which was accompanied by strong seismic shocks. The crush injuries of the skeletons of the two individuals are consistent with severe compression traumas and analogous to those shown by individuals involved in modern earthquakes testifying that, apart from other volcanic phenomena, the effects of syn-eruptive seismicity may be relevant. These outcomes lay the foundation for a more extensive study concerning the assessment of the contribution of the syn-eruptive seismic destruction at Pompeii and open new perspectives for volcanological, archaeoseismological and paleopathological studies.
1 Introduction
Large explosive eruptions are characterized by the high-speed discharge into the atmosphere of a mixture of gas, liquid and solid particles that can be transported upward, forming sustained eruption columns up to tens of kilometers high, or can collapse and spread laterally, forming pyroclastic currents (e.g., Woods, 1995; 2001; Cioni and Pistolesi, 2015). Large eruptions are often preceded and accompanied by a significant seismic activity (Williams and Self, 1983; Cioni et al., 2000a; Yokoyama, 2001; Scandone and Giacomelli, 2008; McNutt and Roman, 2015; Arzilli et al., 2019). Pre- or syn-eruptive seismicity represents an additional threat for settlements in volcanic areas and a cause of damage to buildings, and possibly loss of life, making the assessment of the seismic hazard necessary in addition to the volcanic hazards associated mainly with tephra fallout accumulation and impact of pyroclastic currents (e.g., Pomonis et al., 1999; Zobin, 2001; Zuccaro et al., 2008; Zuccaro and De Gregorio, 2019). Going back in time, evidence of seismicity associated with volcanic activity, and related effects, can be found in historical accounts (e.g., the 1631 sub-Plinian eruption of Vesuvius; Braccini, 1632; Oliva 1632a; 1632b) or can be detected in ancient settlements struck by volcanic catastrophes. Two outstanding examples are the Akrotiri settlement, located on the island of Thera (Santorini, Greece), destroyed by the 3.6 ka Minoan Plinian eruption (Cioni et al., 2000a; 2000b), and the ancient city of Pompeii, located 9.5 km south-east of Vesuvius (Naples, Italy; Figure 1), ravaged during the 79 CE Plinian eruption (VEI 6; Cioni et al., 2003), also known as “Pompeii eruption”. In about 2 days, the ancient Pompeii was buried under a thick pyroclastic blanket consisting of a ∼3 m thick pumice fall deposit overlaid by a sequence of ash deposits emplaced by successive pyroclastic currents. Following the first excavations started in 1748 (Maiuri, 1958), systematic archaeological investigations have unearthed most of the city that has become a landmark in the cultural landscape of Vesuvius (Di Vito et al., 2023) and one of the most important archaeological sites worldwide. The interplay among the succession of volcanic phenomena, the eruptive and emplacement mechanisms, and the urban structure of Pompeii resulted in a well-documented sequence of damage to buildings and death of the inhabitants (Sigurdsson et al., 1982; 1985; Cioni et al., 1990; 1992; 2000a; 2000b; Yokoyama and Marturano, 1997; Varone and Marturano, 1997; Luongo et al., 2003a; 2003b; Giacomelli et al., 2003; 2021; De Carolis and Patricelli, 2003; Gurioli et al., 2005; 2007; Marturano and Varone, 2005; Zanella et al., 2007; Mastrolorenzo et al., 2010; Scandone et al., 2019; Ruggieri et al., 2020; Scarpati et al., 2020; Amoretti et al., 2021; Dellino et al., 2021; Toniolo et al., 2021; Doronzo et al., 2022 and references therein). Before the eruption, Pompeii was struck by historically and archaeologically documented earthquakes, the strongest of which occurred on 5 February 62–63 CE (the exact date is uncertain) resulting in severe damage to buildings throughout the city (e.g., Maiuri, 1942; Adam, 1989; Dessales, 2022). Moreover, volcanic tremors and strong seismic shocks also accompanied the eruption, between the first and second day of the event, as reported by Pliny the Younger in his letters (Epistulae VI, 16–20) to Tacitus. Despite the extensive literature concerning the destruction caused by volcanic phenomena and damage due to the seismic events that occurred before the eruption, notably no evidence has been reported so far of syn-eruptive earthquake-induced damage at Pompeii. The lack of this information likely arises from the difficulties in identifying such type of evidence during the archaeological excavation, in a context where devastation has been primarily caused by, and always exclusively attributed to, primary volcanic phenomena (pumice lapilli fallout accumulation and pyroclastic currents flowage) whose impact may have disguised or obliterated the effects generated by syn-eruptive seismicity. This possibly resulted in an incomplete history of destruction. In fact, unlike the cases described in literature dealing with the archaeological traces of past earthquakes, the study of the co-seismic effects in Pompeii and in the other Roman cities struck by the 79 CE eruption is complicated by the intertwining between seismicity and volcanic phenomena. The rapid succession of volcanic phenomena and earthquakes, or their coincidence, is a pivotal issue for determining their combined effects on buildings, based on different eruption scenarios at Vesuvius, which lead to a progressive deterioration of the building’s resistance (Zuccaro et al., 2008). For example, earthquakes may trigger the collapse of buildings already conditioned by a vertical load of a fall deposit. Alternatively, the dynamic pressure (i.e., the lateral load exerted by the flow process over buildings, e.g., Doronzo et al., 2011; Doronzo and Dellino, 2011) of a pyroclastic current may affect buildings already weakened by seismic shakings. The positive feature is that pyroclastic deposits, engulfing the buildings, freeze the exact moment of destruction and related evidence, protecting them from further break-up due to any type of decay (not considering the breaching of walls caused by the excavation of exploratory tunnels in both past centuries and more recent times, which are extensively documented in Pompeii, e.g., Luongo et al., 2003a; Toniolo et al., 2021; Argento et al., 2024; Ghedini et al., 2024). In addition, some damage and related repairs in Pompeii have been associated with seismicity occurred prior to the 79 CE eruption, in some cases shortly (days) before the catastrophe (e.g., Varone, 1995; 2000; 2005a). Based on this evidence, the seismic swarm, precursor of the impending eruption, may have provoked structural weaknesses in the buildings, increasing their vulnerability and reducing their strength against the following volcanic/seismic processes. Furthermore, co-seismic site effects due to the local geological and geomorphological features, regarded as aspects conditioning the damage in ancient towns (Hinojosa, 2023), probably also played a role in the distribution of the damage in Pompeii (e.g., Amato et al., 2022).
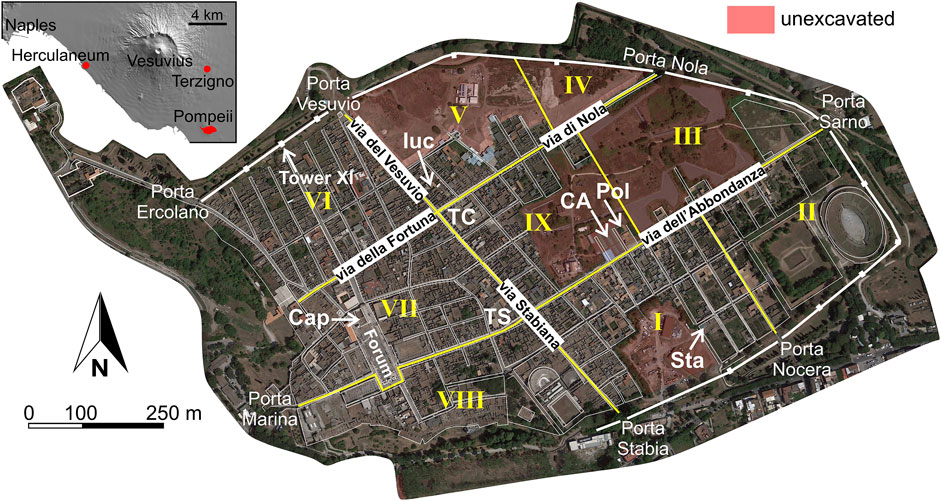
Figure 1. Satellite map of Pompeii archaeological site with subdivision in regiones, indicated by Roman numerals (I-IX), and insulae (white blocks). The locations cited in the text are reported: CA= Insula dei Casti Amanti (IX, 12); Pol= Iulius Polybius’ house (IX, 13); Iuc= Caecilius Iucundus’ house (V, 1); Forum= Foro Civile; Cap= Capitolium; TC= Terme Centrali (IX, 4); TS= Terme Stabiane (VII, 1); Sta= Stabianus’ house (I, 22). Inset: location of Pompeii, Herculaneum and Terzigno with respect to Vesuvius.
Here, we report the peculiar evidence of sudden structural damage and building collapses which involved two individuals, whose skeletons were recovered in the sequence of pyroclastic material and wall debris. The archaeological discovery was unearthed during the ongoing excavation campaign in the so-called Insula dei Casti Amanti (Insula of the Chaste Lovers), in the central part of Pompeii (Figure 1). The excavation was carried out by a multidisciplinary team that involves experts in the field of archaeology, volcanology, anthropology, and archaeoseismology in order to thoroughly assess all the fundamental aspects: the identification of the construction techniques of the Roman building, the detailed reconstruction of the 79 CE stratigraphy and its relation to the structure, the attitude and orientation of the wall failures and their stratigraphic position and, finally, the traumatic pattern of bone fractures of the skeletons of the two individuals. The stratigraphic, archaeological, and volcanological data, integrated with the severity of the crush injuries of the skeletons and the characteristics of the building collapses, have provided information for archaeoseismological considerations pointing to the occurrence of earthquake-induced damage, previously unrecognized, associated with the beginning of caldera-forming phase of the eruption.
2 Outlines of the 79 CE eruption
The 79 CE eruption is well known to have been the first Plinian event described by an eyewitness, Pliny the Younger, and one of the most studied Vesuvius eruptions (see Doronzo et al., 2022 for a review of the eruption studies). Overall, the eruption was characterized by three main phases: a transient, phreatomagmatic, opening phase followed by a paroxysmal phase, with the onset of a sustained to collapsing Plinian eruption column, and a final caldera-forming to phreatomagmatic phase during which successive pyroclastic currents spread around the volcano (e.g., Sigurdsson et al., 1985; Macedonio et al., 1988; Cioni et al., 1990; 1992; 1996; 1999; 2020; Lirer et al., 1993; Doronzo et al., 2022). The 79 CE pyroclastic sequence, with a particular focus on Pompeii, has been studied by many authors who provided detailed stratigraphic frameworks (Sigurdsson et al., 1985; Cioni et al., 1990; 1992; Varone and Marturano, 1997; Marturano and Varone, 2005; Scarpati et al., 2020). Here, the 79 CE stratigraphic framework is borrowed from Cioni et al. (1990; 1992) who arrange the stratigraphy in eight Eruption Units (EU1-EU8), and minor sub-units, having different dispersal, areal distribution, and emplacement mechanisms. Due to the distance from Vesuvius and the different areal distribution of the products, the 79 CE stratigraphy at Pompeii (Figure 2) is composed only of five (EU2f, EU3f, EU4, EU7, and EU8) out of eight EUs. The opening phreatomagmatic phase produced an easterly-dispersed accretionary lapilli-bearing ash fall deposit (EU1) which did not affect Pompeii. A stratified fall deposit made up of white (EU2f) to grey (EU3f) pumice lapilli and blocks, dispersed toward south-east, represents the product of the Plinian phase. The white to grey colour transition reflects a compositional variation of magma from phonolite to tephri-phonolite (Cioni et al., 1995; Doronzo et al., 2022; Melluso et al., 2022). The pumice fall deposit in Pompeii attains a maximum total thickness of 2.8–2.9 m (Lirer et al., 1973; Sigurdsson et al., 1985; Cioni et al., 1990; 1992; Doronzo et al., 2022). At least four partial collapses of the Plinian eruption column generated pyroclastic currents whose ash deposits are interspersed in the Plinian fall deposit. Only the last of these pyroclastic currents affected Pompeii, emplacing a thin ash layer (EU3pfi sub-unit) a few centimeters below the top of EU3f, just outside the north-western city walls (Sigurdsson et al., 1985; Cioni et al., 1990; 1992) and in the northernmost part of Pompeii (Scarpati et al., 2020). The grey pumice deposit is capped by two ash layers separated by a thin horizon of pumice lapilli (EU3pftot sub-unit). This unit records the total collapse of the Plinian eruption column. The transition to the caldera-forming and final phreatomagmatic phase was marked by an abrupt variation of the eruptive and depositional mechanisms of the products, emplaced mainly by multiple pyroclastic currents alternated with brief “lithic-rich” fall phases (Chiominto et al., 2023). EU4 is formed by a basal, centimeter-thick, lithic lapilli-rich fall deposit (EU4bl), overlaid by a decimeter-to meter-thick, grey ash deposit containing pumice lapilli, accretionary lapilli and deep-seated lithic fragments (EU4pf), capped by an accretionary lapilli-bearing ash layer. EU4pf resulted from the most powerful pyroclastic current that overran Pompeii. EU4 is associated with the initial destabilization of the shallow plumbing system and fracturing of the magma chamber roof. This marks the beginning of the caldera collapse accompanied by significant seismicity (Cioni et al., 1999; Cioni et al., 2000a). EU5 and EU6 are matrix-supported lithic-enriched deposits and coarse lithic breccias emplaced by pyroclastic currents, confined in paleovalleys on the slopes of Vesuvius, that mark the definitive caldera collapse (Cioni et al., 1999). EU7 consists of two lithic lapilli-rich fall layers separated by an ash layer and overlaid by an accretionary lapilli-bearing ash layer (EU7pf; Gurioli et al., 2007). EU8 consists mainly of a stratified sequence, up to 1 m thick, of accretionary lapilli-bearing ash layers emplaced by successive phreatomagmatic pyroclastic current pulses during the waning phase of the eruption.
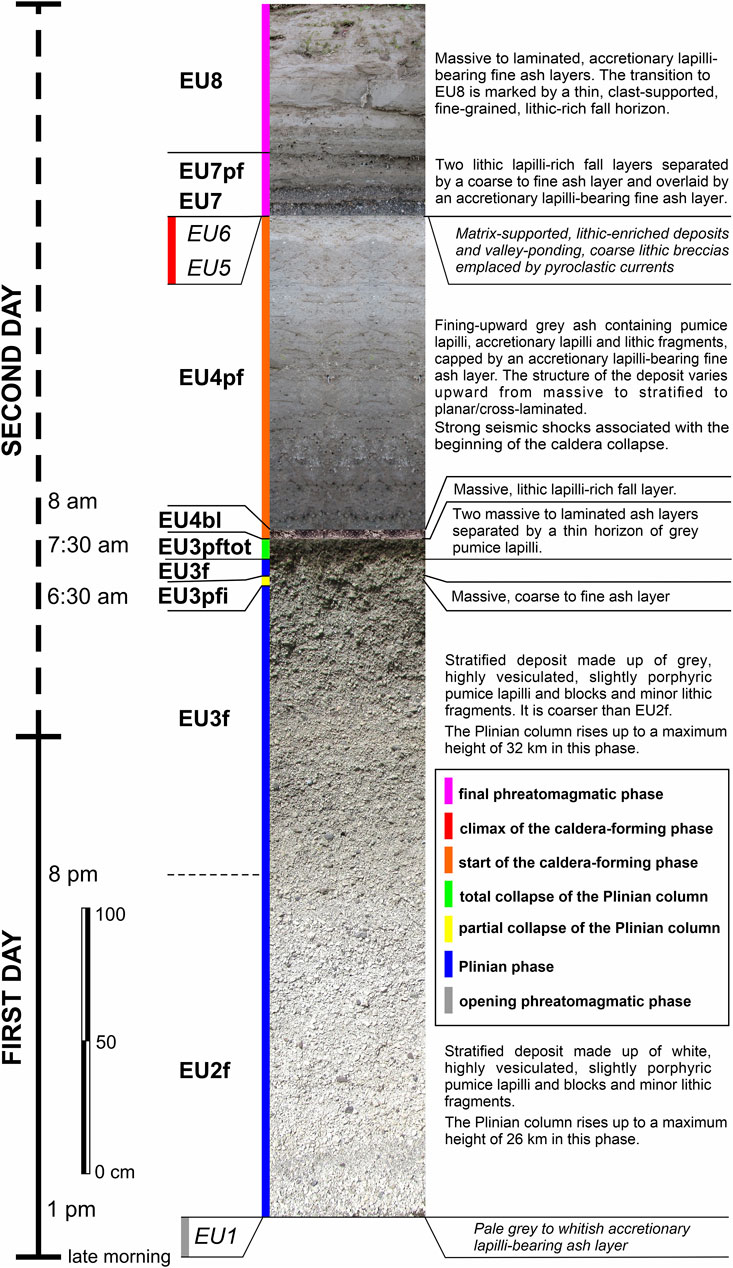
Figure 2. Stratigraphic sequence of the 79 CE eruption with a focus on Pompeii archaeological site. Eruption Units (EU) are from Cioni et al. (1990; 1992) and Gurioli et al. (2007). Units lacking in Pompeii are reported in italics. The pumice fall deposit is interrupted by several ash layers (at least four) emplaced by pyroclastic currents generated by partial collapses of the Plinian column; only the last of these events (EU3pfi sub-unit) reached Pompeii. The maximum height reached by the Plinian column is from Carey and Sigurdsson (1987). The synchronization of the events is from Sigurdsson et al. (1985) and Cioni et al. (2000a). Vertical coloured lines mark deposits associated with the various phases (and sub-phases) of the eruption.
3 Seismicity prior to the 79 CE eruption
Seismicity occurred during the first century CE, prior to the 79 CE eruption, and related effects in Pompeii have been debated for many decades. Information arises by integrating historical sources and archaeological evidence. A first earthquake, which occurred in 37 CE, is reported by Suetonius in his book (Tiberius 74, 2) devoted to the emperor Tiberius. Its estimated magnitude (M) is 4–4.5 (Cubellis and Marturano, 2013). The major seismic event before the 79 CE eruption occurred in 62–63 CE. Two different estimates of the magnitude have been proposed: 5.1 (Cubellis and Marturano, 2002; 2013; Cubellis et al., 2007) and 5.8 (CFTI5med catalogue, Guidoboni et al., 2018; 2019). The damage associated with this earthquake has been estimated, according to the Mercalli-Cancani-Sieberg (MCS) scale, with Intensity (I) 9 at Pompeii, 8–9 at Herculaneum and 7–8 at Naples (Imbò, 1974; Adam, 1989; Guidoboni et al., 2018; 2019). The occurrence of this earthquake is documented by different Latin authors although the exact date is being debated (e.g., Hine, 1984; Savino, 2009; Dessales, 2022). Tacitus (Annales XV, 22) refers to 5 February 62 CE while Seneca (Naturales Questiones VI, 1) gives 63 CE as the year of the event. The 62–63 CE earthquake resulted in such extensive damage, still visible in Pompeii buildings, as repaired fractures and deformations (e.g., Ruggieri et al., 2017; Amato et al., 2022; Dessales, 2022), that it is believed that repair works were still under way 17 years later, making the city a large construction site (Maiuri, 1942) when Vesuvius awoke. Two bas-reliefs, found in the in the house of Caecilius Iucundus (Adam, 1989; Varone, 2000; 2005a), are evocative of the effects of this earthquake in two locations: the Capitolium, in the northern part of the Foro Civile, and Porta Vesuvio (Figure 1). Aftershocks were felt for several days after the major event. The 62–63 CE earthquake was followed by another seismic event in 64 CE (M= 3.5–4; Cubellis and Marturano, 2013) documented by Suetonius and Tacitus who reported the shaking of a theatre in Naples during an artistic performance of the emperor Nero (De Simone, 1995; Pappalardo, 1995; Renna, 1995; Cubellis et al., 2007; La Greca, 2007; Marturano, 2008). The effects of the seismicity following the 62–63 CE major event have often been underestimated. According to Varone (2000; 2005a), the reports concerning the 62–63 CE earthquake provided scholars with the most valid cause-effect relationship to justify the extensive repair works throughout Pompeii at the time of the eruption. The international meeting “Archäologie und Seismologie: la regione vesuviana dal 62 al 79 d.C.: problemi archeologici e sismologici” held in Boscoreale (near Pompeii), in November 1993, convened by the Deutsches Archäologisches Institut, the Osservatorio Vesuviano and the Archaeological Superintendence of Pompeii (currently Pompeii Archaeological Park), gathered scientific contributions reporting evidence of different phases of repair works related to a prolonged seismicity. Examples include repairs and reinforcement interventions at the Terme Stabiane (Figure 1), attributed to the effects of the 64 CE earthquake besides that of 62–63 CE (Ruggieri et al., 2018), and consolidation works of edifices built after 62–63 CE (Jacobelli, 1995; Pappalardo, 1995) such as at the Terme Centrali (Figure 1). Other archaeological clues that point to ongoing repair works are represented by the discovery, in many houses, of goods and furniture set aside, piles of lime, building materials neatly stacked, wall plaster refurbishment, incomplete wall decorations and frescoes (e.g., Allison, 1995; De Simone, 1995; Ling, 1995; Nappo, 1995; Varone, 1995; 2000). Some of the events responsible for the damage to buildings have been attributed to the time interval between 72 and 78 CE (Marturano, 2006) testifying to a period of persistent seismicity that plagued the city for many years prior to the 79 CE eruption (Allison et al., 2003; Scandone and Giacomelli, 2008; Scandone et al., 2019). In some cases, evidence points to the effects of seismicity shortly before the catastrophe, during the year before the eruption (Marturano, 2006) and up to a few days prior to it (Varone, 1995; 2000; 2005a), in the form of warped mosaic floors in the Insula dei Casti Amanti (Figure 1) and repair works to the non-functioning sewers along the eastern alley of the same insula, where septic tanks were found filled with pumice lapilli. According to the cited authors, it appears unlikely that sewers that served an entire block, including rich dwellings, were left unrepaired for a long time before the eruption. The seismicity, precursor of the impending eruption, is also accounted by Pliny the Younger (Epistolae VI, 20) who reported that tremors were felt for several days before the eruption, but they caused little alarm as people in Campania region were accomplished to such phenomena (Praecesserat per multos dies tremor minus formidolosus, quia Campaniae solitus). Ultimately, the picture that emerges is that of a city that, between 62 and 79 CE, suffered the effects of recurring earthquakes and volcanic tremors that forced the inhabitants to undertake continuous reconstruction, renovation and restoration works until the eruption.
4 Syn-eruptive seismicity
No clear evidence of damage related to syn-eruptive earthquakes has been reported in Pompeii so far. Varone (1995) and Marturano and Varone (2005) reported the finding of masonry blocks horizontally displaced from their original positions, suggesting that seismic solicitations related to the eruption (or soon after) should be considered in assessing the damage. However, the occurrence of syn-eruptive seismicity is documented by Pliny the Younger who reported seismic shakings felt in Misenum (∼29 km west of Vesuvius) during the night of the first day of the eruption, and violent shocks at the daybreak of the second day. The scenario depicted by Pliny the Younger’s second letter (Epistulae VI, 20) to Tacitus is a vivid picture of the seismic upheavals:
“tremor (terrae)…illa vero nocte ita invaluit, ut non moveri omnia, sed everti crederentur.”
“earthquakes…that night became so intense that everything seemed not only to be shaken but overturning.”
“Iam hora diei prima, et adhuc dubius et quasi languidus dies…vehicla quae produci iusseramus, quamquam in planissimo campo, in contrarias partes agebantur, ac ne lapidibus quidem fulta in eodem vestigio quiescebant.”
“It was the first hour of the day, but the light was still faint and weak.…the chariots we had ordered to be brought out, though on a level ground, were shaken back and forth and did not remain steady in their places even wedged with stones.”
The latter has been associated with the beginning of the caldera collapse phase with the emplacement of EU4 (Cioni et al., 1999; Cioni et al., 2000a; 2000b). According to the CFTI5med catalogue (Guidoboni et al., 2018; 2019), the estimated M is 6 whilst the MCS Intensity is 8. Scandone et al. (2019) drew an isoseismal map, representing the cumulative effects of the syn-eruptive seismic swarm, through the estimation of the intensity at different localities based on epigraphs documenting repair works made after the eruption. In this map, Pompeii is well within the isoseism defining Intensity 9. According to these authors, such an intensity must have caused severe damage and widespread collapse of buildings, possibly resulting from earthquakes even stronger than the main one of the 62–63 CE sequence.
5 Materials and methods
5.1 The excavation in the Insula dei Casti Amanti
The urban planning of Pompeii, conceived in the mid-19th century by the archaeologist Giuseppe Fiorelli (Varone, 2000), is divided in nine large districts, called “regiones” (singular regio) and indicated by Roman numerals (I-IX), bounded by thoroughfares oriented east-west (Decumani) and north-south (Cardi). Each regio is further subdivided into numbered blocks, called “insulae”, bounded by alleys. The Insula dei Casti Amanti is numbered as insula 12 in Regio IX and faces south onto the via dell’Abbondanza (Figure 1). Its name originates from a fresco, found in a domus (house), depicting two lovers chastely kissing. The first excavations of the façades of the buildings fronting onto the via dell’Abbondanza were undertaken in 1911 by the archaeologist Vittorio Spinazzola (Varone, 2000). A more recent, systematic excavation campaign began in 1987, continued in various phases (Varone, 2000; 2005b; 2005c), and is still ongoing (Calvanese et al., 2024). As a result, the southern part of the insula has been unearthed, as well as the alleys that flank it to east and west. The insula includes different domus (Figure 3): the “casa del Cenacolo Colonnato I and II” in the south-western sector, the “casa dei Casti Amanti” in the south-eastern sector, where the fresco after which the insula was named was found, and the “casa dei Pittori al Lavoro” (house of the Painters at Work) in the northern part, which is still partly buried under the pyroclastic blanket. The latter is articulated around an internal garden (viridarium) surrounded by a portico (peristilium) on three sides (north, west, and east) where piles of lime were found, evidence of ongoing restoration works (Varone, 1995; 2000). The current excavation campaign involves rooms located in the north-eastern part of the house of the Painters at Work, close to the east alley of the insula. In two newly excavated, adjoining rooms (room A and 22 in Figure 3), we found evidence of wall failures and damage whose characteristics (stratigraphic heights, orientation, and attitude) are compared in order to reconstruct the timing of the collapses and triggering mechanisms. Furthermore, in room A, the structural collapses overwhelmed two individuals, whose skeletons have been recovered, providing important clues that have contributed, along with volcanological data and archaeoseismological considerations, to demonstrate that an updated perspective of the destruction of Pompeii during the 79 CE eruption should be considered.
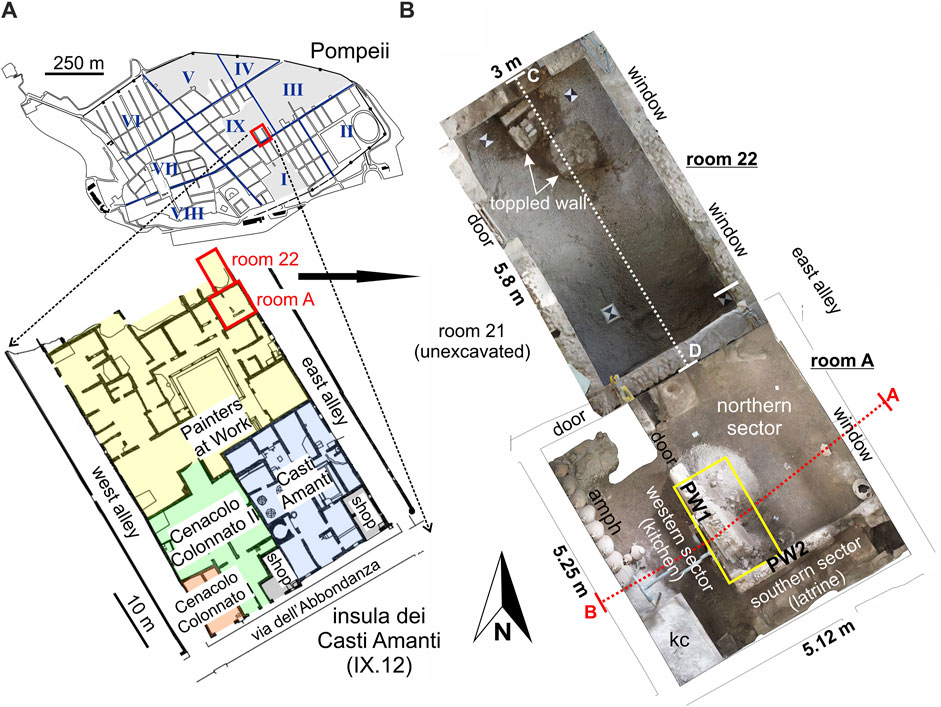
Figure 3. (A) Plan view of the Insula dei Casti Amanti (IX. 12) in the central part of the Pompeii archaeological site. The domus that compose the insula are highlighted with different colours. Rooms A and 22, in the north-eastern sector of the house of the Painters at Work, are outlined in red. (B) Plan view (orthophoto) of rooms A and 22. Room A is subdivided in the three sectors (southern, western, and northern) marked by two, orthogonal, partition walls (PW1 and PW2); kc = kitchen counter; amph = amphorae. The yellow rectangle encloses the main collapse unit in room A. The A-B and C-D transects refer to the cross sections showed in Figure 8.
6 Results
6.1 The archaeological perspective: the context in the house of the Painters at Work
The two adjoining rooms (rooms A and 22 in Figure 3) are located on the ground floor, bounded to the east by the alley oriented approximately NW-SE, and are each accessed through doors opening onto room 21 (Figure 3) which, though still unexcavated and currently filled with pumice lapilli, had the function of an open courtyard. The two rooms are not interconnected; there is no door that allows direct access from one room to the other. Both rooms are delimited by load-bearing, 45 cm thick, perimeter walls built in opus africanum, a building technique consisting of small stone blocks, bonded with different types of binder, inserted between chains of large blocks in which vertical blocks alternate with horizontal ones (Pesando and Guidobaldi, 2018).
Room A has been partly excavated in 1988–1989. It is surrounded to south and west by rooms previously excavated, while it is bordered by room 22 to north, sharing a perimeter wall. Room A has a nearly square shape (5.25×5.12 m). It is accessed through a door, located at the western end of the north-western perimeter wall, which currently opens onto the still buried area (room 21 in Figure 3). The interior of the room is marked by two, 30 cm thick, partition walls with no load-bearing function, perpendicular to each other (Figure 3) being oriented approximately NW-SE (hereafter PW1) and NE-SW (hereafter PW2). The partition walls are built in opus incertum, a building technique consisting of irregular stones of different size bonded with mortar (e.g., Pesando and Guidobaldi, 2018). The partition walls allow to subdivide the area of room A into three rectangular-shaped sectors. The southern sector had the function of a latrine and had already been investigated down to the ground level during the previous excavation. The western sector had the function of a service kitchen, as testified by the finding of a kitchen counter located in the south-western corner. The kitchen was decommissioned at the time of the eruption as indicated by the presence of two piles of lime, one resting directly on the counter and the other one leaning against it. The presence of piles of lime is evidence that restoration and/or renovation works were also under way in this part of the house. The northern sector occupies the largest part of the room, having dimensions of 4×2.91 m. This area is accessed from the western sector through a door located at the northern end of the PW1. Here, the north-eastern perimeter wall comprises a window facing the alley, whose base is 1.70 m above the ground level of the alley itself. In this part of room A, the skeletons of two individuals were found close to the partition walls.
Room 22 is rectangular-shaped (5.8×3 m) with the longest side parallel to the alley. It is accessed through a door, located along the south-western perimeter wall, which opens onto the unexcavated area (room 21 in Figure 3). Two windows, having the same characteristics as described for Room A, are present in the north-eastern perimeter wall.
6.2 The volcanological perspective: Stratigraphy, stratigraphic height of damage, and victims
The stratigraphy of the 79 CE pyroclastic deposits in the recently excavated rooms is described below. The stratigraphic context of each room is described separately to better highlight the different attitude and stratigraphic height of the structural damage. The stratigraphic height and the stance of the skeletons of two individuals found in room A, as well as their relationship with the wall collapses, are also described in detail.
6.2.1 Room A
As previously reported, the southern sector (latrine) had already been completely excavated; therefore, our stratigraphic description is limited to the western and northern sectors.
In the western sector (kitchen), a deposit consisting of coarse, angular, white pumice lapilli (EU2f) was resting directly on the ground, with an uniform thickness of ∼75 cm (Figure 4A). EU2f was capped by anthropically reworked material left by the past excavations. No evidence of roof/attic collapse has been found on the ground or in the pumice lapilli deposit. Eight intact amphorae were found in a row on the ground, propped upright against the south-western perimeter wall and embedded within the pumice lapilli deposit. It is worth noting that the door from which this sector (and the room) is accessed was completely blocked by a pumice lapilli fall deposit, consisting mainly of white pumice.
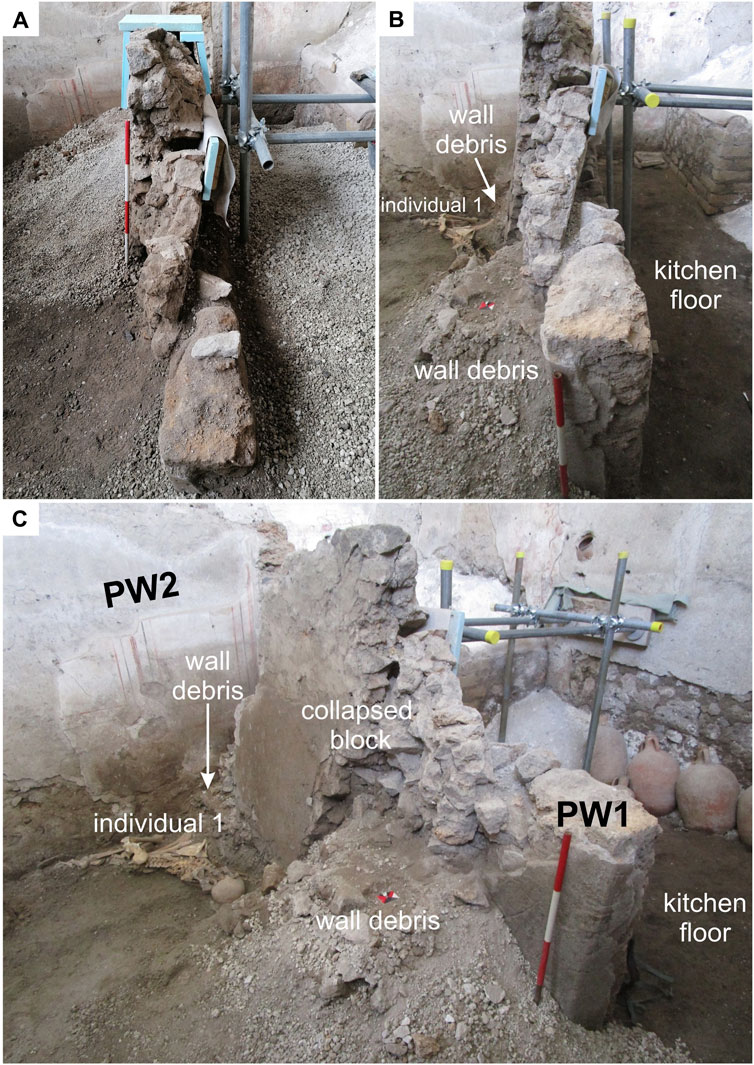
Figure 4. Photographs of the major collapse unit along the PW1 in Room A: (A, B) cross section view (approximately from the NW), in various phases during the excavation, of the collapsed masonry fragment leaning against the residue in situ of the same wall; note the white pumice lapilli filling the western sector (kitchen; right side of the wall) and partly covering the collapsed block in the northern sector (left side of the wall); once the lapilli deposit had been removed, two accumulations of wall debris were found to the side of the collapsed masonry fragment; (C) panoramic view (from the north) of the collapse unit. In (B, C), the skeleton of the individual 1 is visible.
In the northern sector, the ground was covered by an accumulation of grey pumice lapilli (EU3f) forming a heap, whose apex (∼1.3 m) corresponded with the window opening in the north-eastern perimeter wall. The heap of grey pumice lapilli thinned out in all directions, reaching the walls. Plaster fragments, forming thin accumulations, were found interspersed within the lapilli heap close to the walls. A major collapse unit, resulting from the failure and break-up of the upper part of PW1, lay directly on a few centimeters of grey lapilli. The collapse unit consists of two features (Figures 4B,C): a large masonry fragment, measuring 200×140×30 cm, and two heap-shaped accumulations of wall debris to the sides of the masonry fragment and partly overlying it. The large masonry fragment is positioned with its long side upright and leaning laterally against the remaining, in situ, portion of the same wall. The position of the collapsed wall fragment is the result of the horizontal displacement towards the north-east, after sliding over a sub-horizontal shear plane located between 60 and 70 cm above the ground, and downward collapse without breakage, toppling or overturning. The heap-shaped accumulations of wall debris, consisting of centimeter-sized masonry rubble, pieces of mortar, and plaster fragments, were in turn covered by coarse, angular, white pumice lapilli. The deposition of white lapilli was fed from breaches in the PW1. A skeleton (individual 1; Figure 5), lying on its right side, facing the PW1, was found in the south-western corner of this sector, resting on a few centimeters of grey pumice lapilli and directly overlaid by the collapse unit. One of the heaps of wall debris covered the skull, the torso, the upper limbs and part of the lower limbs, leaving the back exposed which was facing the center of the room (Figure 5). The right hand was lying directly under the collapsed masonry fragment. A second skeleton (individual 2; Figure 5), lying on its left side, facing the north-eastern perimeter wall, was found at the same stratigraphic position in the south-eastern corner of this sector. The skull, torso and the lower limbs lay on the lapilli heap while the right foot was directly on the ground. A masonry fragment, 80 cm long, fallen from the upper part of the PW2, lay directly on the right lower limb and pelvis (Figure 5). The skeleton was covered by a heterogeneous deposit consisting of pumice lapilli, plaster fragments detached from the adjacent walls, and subordinate masonry blocks deriving from the collapse of the PW2. At the time of discovery, only the upper part of the skull and the left knee emerged from the accumulation of wall fragments and pumice. In both cases, the internal volume of the skeleton was filled with loose material (wall debris for individual 1; wall debris and pumice clasts for individual 2) that re-settled after the decomposition of the corpses. The presence of the heap of grey lapilli to the north-east and the collapse unit to the south-west resulted in a concave profile which was filled with a grey ash deposit containing pumice lapilli (EU4pf). The ash deposit covered individual 1’s back and partly enveloped the skull penetrating through the voids of the wall debris. Likewise, the ash engulfed individual 2’s skull, which emerged from the lapilli, and partly penetrated the lapilli deposit, filling the space between the grains and touching the individual 2’s back. Close to individual 2, the limit of the ash deposit showed a circular shape which was apparent, although ephemeral, in the accumulation of lapilli. It could represent the trace of a rotted (wooden) object.
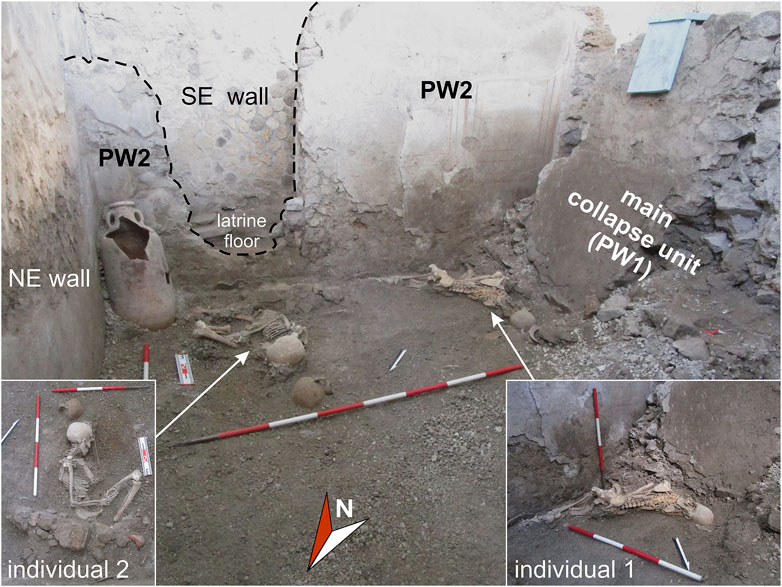
Figure 5. View from the north of the northern sector of room A during the excavation. The skeletons of two individuals lay in the south-western (individual 1) and south-eastern corner (individual 2). Insets: close-up view of the skeletons; note the accumulation of wall debris close to the individual 1 (it also covered the skeleton) and the large wall fragment on the pelvis and right lower limb of the individual 2.
6.2.2 Room 22
The stratigraphy in this room (Figure 6) is characterized by the same three stratigraphic units (EU2f, EU3f and EU4pf) arranged in a less complicated framework. The ground was covered by accumulations of pumice lapilli (EU2f and EU3f) whose maximum thickness corresponded with the door opening in the south-western wall and the two windows in the north-eastern wall. The pumice lapilli were mainly grey. White lapilli were found only close to the door. Similar to room A, these accumulations of lapilli resulted in a longitudinally elongated, concave profile capped by a grey ash deposit containing pumice lapilli (EU4pf), burying the rest of the room. Also in this room, no evidence of roof/attic collapse has been found. A collapsed wall, fragmented in two large blocks having total dimensions of 128×70×45 cm, coming from the south-eastward toppling of the north-western perimeter wall, was found in the pyroclastic sequence (Figure 6). The masonry blocks lay horizontally on the grey ash and were engulfed by the same ash deposit (EU4pf). The thickness of the ash deposit underlying the collapsed wall was at its maximum (22 cm) along the longitudinal axis of the room while it thinned out, moving laterally, towards the north-eastern and south-western walls due to the concave profile of the underlying lapilli deposit. The collapse resulted in a channel-shaped breach in the north-western wall having a maximum depth of 1.3 m (Figure 6).
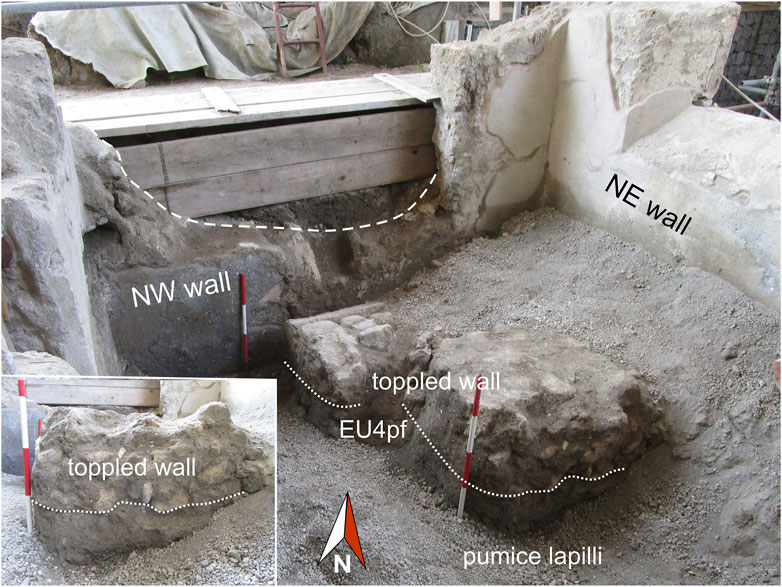
Figure 6. View from the south of the Room 22. In the foreground the topppled wall, fragmented into two blocks, laying on a grey ash deposit (EU4pf) in turn resting on the concave profile of the pumice lapilli deposit; note the thinning of the ash deposit towards the north-eastern perimeter wall while it keeps a constant thickness along the longitudinal axis of the room. In the background, the north-western perimeter wall showing a channel-shaped breach (dashed line). Inset: detail of the collapsed wall and the underlying stratigraphy (view from the SE).
6.3 The anthropological perspective: Condition of the skeletons
The biological profile, based on the macroscopic characteristics of the skull and pelvic girdle (Bertoldi, 2009), indicates that both individuals were male. The age determination, based on the Suchey-Brooks pubic symphysis scoring system (Brooks and Suchey, 1990) when possible, the modifications of the auricular surface (Lovejoy et al., 1985), and the occlusal surface of the maxillary and mandibular teeth (Lovejoy, 1985), suggests a mature-senile age (≥55 years) for both. This age is further suggested by the complete ossification of the manubriosternal joint as well as the degree of obliteration of cranial sutures.
The individual 1 shows a high number of rib fractures (more than 20 per hemithorax) resulting in a complex injury pattern typical of blunt chest trauma (Figure 7A). In general, rib fractures represent the most frequent type of bone fractures. In about fifty percent (50%) of all rib fracture cases, three or more consecutive ribs are broken being also referred to as serial rib fractures or flail chest (Liebsch et al., 2019). Combinations of multiple rib fractures can result in instability and loss of integrity of ribs and chest walls causing the harming of the inner organs, often with fatal consequences (Dogrul et al., 2020). In addition to the very severe pattern of rib fractures, there are also significant fractures of the facial skull and skull base as well as pelvic fractures. The latter, in compression damage, tends to adhere to a certain typology, usually located at the level of the pubic bone (the most fragile portion of the pelvic girdle). Furthermore, the combination of the avulsions of the vertebral spinous processes and additional fractures of variable severity in the upper and lower limbs enhances the traumatic scenario.
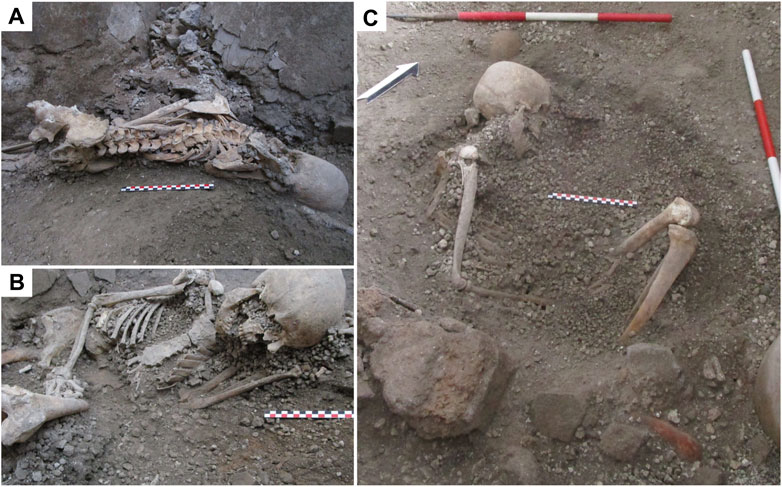
Figure 7. Details of the skeletons found in the northern sector of Room A: (A) individual 1’s back showing rib and shoulder fractures; (B) and (C) individual 2 huddled on his left side with his left hand protecting his head. Note the circular shape of the ash deposit likely representing the trace left by a rotted (wooden) object.
Individual 2 shows a relatively similar pattern of fractures, although some differences are established (such as the presence of a higher number of fractures on the right hemithorax, the one directly exposed to the trauma, while the left one must be sunk into the underlying lapilli deposit). Unlike the individual 1, found directly under the main collapse unit of PW1, individual 2 was mainly buried at the level of the lower limbs by a large wall fragment detached from the PW2. Plaster fragments were in direct contact with the right femur and released red pigments that coloured its surface. At the site of greatest impact, the right iliac wing showed an extremely important cracking scenario resulting in very extensive and comminuted fractures. The same applies to the right radius and ulna characterized by a fracture defined in the literature as “Both Bone Forearm Fractures” (Trionfo and Arcader, 2019), and to the right tibia and fibula directly crushed by the large masonry fragment. Again, costal traumas, combined with facial and skull base traumas, point to a hypothesis of immediate or semi-immediate death. Interestingly, the individual 2 had a series of previous well-repaired fractures with bone remodelling which afflicted both rib cage and wrists. Importantly, the position of the skeleton, huddled on his left side with his left hand protecting his head (Figure 7B), and the faint traces of a circular object directly above the skeleton (Figure 7C) suggest that the individual was aware of the danger and attempted to take cover.
7 Discussion
7.1 The complex picture of the destruction
The detection of past seismic effects is a complex task as the traces of past earthquakes in archaeological stratigraphic successions and within monuments, the object of the so-called “archaeoseismology”, are seldom univocal. The detection of syn-eruptive seismic effects is even more complex due to the intertwining between seismicity and volcanic phenomena as previously described. The literature indicates that the cause-effect relationship between archaeological evidence of destruction and seismic shaking is not straightforward and often questionable (e.g., Karcz and Kafri, 1978; Guidoboni and Santoro, 1995; Stiros, 1996; Galadini et al., 2006; Marco, 2008; Hinzen et al., 2010; Jusseret et al., 2013; Albrecht and Döring-Williams, 2023). For this reason, a normal procedure adopted in archaeoseismology is the exclusion of possible causes of destruction other than those associated with seismic solicitation (e.g., Stiros, 1996; Galadini et al., 2006; Marco, 2008; Hinzen et al., 2010; Stiros and Pytharouli, 2014). Looking for maximum consistency with earthquake damage/destruction, most archaeoseismological works are based on: 1) the evidence of geological co-seismic effects in archaeological sites, mainly surface faulting and sudden areal vertical motion (e.g., Pirazzoli et al., 1992; 1996; Hancock and Altunel, 1997; Galadini and Galli, 1999; Akyüz and Altunel, 2001; Stiros and Papageorgiou, 2001; Marco et al., 2003; Galli and Naso, 2009; Altunel et al., 2009; Schweppe et al., 2021); 2) the areal approach based on the correlation of destruction layers, i.e., horizons in the archaeological stratigraphy showing evidence of sudden destruction caused by human and/or natural agents (Galadini et al., 2006; Sintubin, 2013), throughout a territory or an archaeological area (e.g., Guidoboni et al., 2000; Stiros and Papageorgiou, 2001; Galli and Bosi, 2002; Galadini and Galli, 2004; Ceccaroni et al., 2009; Galadini et al., 2022); 3) the estimation of the consistency of the damage by seismic actions through structural evaluations or modelling ground motion and structural response (e.g., Korjenkov and Mazor, 1999a; 1999b; 2003; 2014; Hinzen, 2005; 2009; Rodrìguez-Pascua et al., 2011; Hinzen et al., 2013; 2021; Kázmér and Major, 2015). In some cases, the different approaches have also benefitted from attempts to quantify the reliability of the seismic hypothesis by using a plausibility matrix or summarizing the consistency of alternative causes of destruction (e.g., Hinzen et al., 2013; 2021; Galadini et al., 2018; Albrecht and Döring-Williams, 2023).
Considering the issues here summarized, we interpret the 79 CE stratigraphy and the associated archaeological destruction layers, along with the condition of the skeletons of the two individuals, to infer the triggering mechanisms of the wall collapses and the timing in the course of the eruption. The merging of the volcanological, archaeological, and anthropological data, along with the analysis of the wall failures and displacements, led to archaeoseismological considerations that suggest an additional syn-eruptive cause of destruction of Pompeii and death of the inhabitants beyond volcanic phenomena. In this light, our discussions are presented according to the following rationale: 1) we interpret the stratigraphic features of the deposits in terms of the sequence of events and timing of the destruction of the two adjacent rooms, 2) we compare the typical damage caused by primary volcanic phenomena, as addressed in the volcanological literature, with that found in the recently excavated rooms in order to highlight the similarities (room 22) and differences (room A) in the characteristics and attitude of the failures, in turn reflecting different dynamics and triggering mechanisms, 3) we propose an archaeoseismological interpretation of the mechanism which triggered the wall collapses in room A, after ruling out any other possible cause, 4) the traumatic pattern of the skeletons is discussed to infer the causes of death.
7.2 Succession of events and timing of destruction
The 79 CE pyroclastic sequence, that is easily recognizable in open space, exhibits anomalous characteristics and thicknesses when the volcanic phenomena interact with buildings (Luongo et al., 2003a; 2003b; Gurioli et al., 2005). Here, the sequence of destruction is reconstructed for the investigated rooms of the house of the Painters at Work and illustrated in Figure 8. Although the stratigraphic record is incomplete in room A due to past excavations, the available data still permit to suggest a sequence of destruction. Since the exact date of the eruption is being debated (late August to November, e.g., Di Giuseppe, 2021; Doronzo et al., 2022; 2023; Altamura, 2022; Foss, 2022), we simply refer to the first and second day of the eruption. The proposed scenario is described in chronological order, following the chronology of the events as reconstructed by Sigurdsson et al. (1985) and Cioni et al. (2000a).
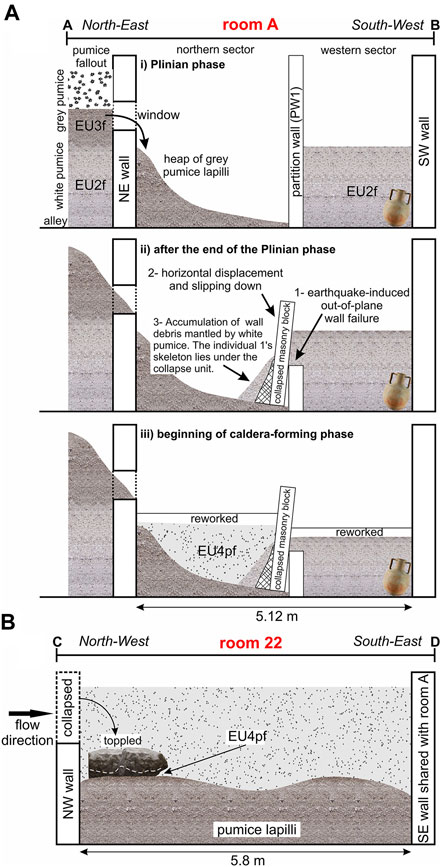
Figure 8. Stratigraphic relationship between pyroclastic deposits and structural collapses and sequence of the events in the investigated rooms; (A) NE-SW cross section (view from the NW) in Room A whose profile (A-B transect) is showed in Figure 3: i) during the Plinian phase, white pumice lapilli (EU2f) penetrate the western sector (kitchen) through a door in the north-western perimeter wall and fill most of the space (see also Figure 9); grey pumice lapilli (EU3f) penetrate the northern sector through the window in the north-eastern perimeter wall, facing the alley; ii) after the end of the Plinian phase, the out-of-plane failure of the PW1 occurs. A large masonry fragment is horizontally (north-easterly) displaced and slipped down as a result of a seismic solicitation. Accumulations of wall debris result from the break-up of the same wall. White pumice lapilli penetrate from the western sector, through breaches in the PW1, covering the collapse unit; iii) at beginning of the caldera-forming phase, the EU4pf parental current penetrates the room and emplaces an ash deposit that entombs the underlying pumice accumulations and the collapse units (and the skeletons). When the new excavations began, the primary pyroclastic deposits were covered by a layer of reworked material left by past excavations. (B) NW-SE cross section (view from the SW) in Rom 22 whose profile (C-D transect) is showed in Figure 3. Pumice lapilli penetrate the space through the openings (door and windows) during the Plinian phase. During the early phase of caldera formation, the EU4pf parental current, coming from the north-west, hit the north-western wall causing the upper part to collapse being pulled down south-eastward. The collapsed wall blocks rest on, and are capped by, the ash deposit emplaced by the same pyroclastic current. The south-eastern perimeter wall, shared with room A (Figure 3), is not damaged at all.
At the beginning of the Plinian phase, around 1 p.m., white pumice lapilli fell on Pompeii. After 7 h, the lapilli fallout switched to grey pumice. The resulting deposit (EU2f and EU3f) was found in both rooms as a consequence of the penetration through openings (doors and windows). In room A, the white pumice deposit (EU2f) has only been recognized in the western sector (kitchen), where it shows an almost uniform thickness (∼75 cm). We are aware that the top of this deposit was affected by past excavations due to: 1) the uniformness of the thickness, 3) the truncated horizontal surface and 3) the presence of anthropically reworked material on top. Evidence comes from a photo (Figure 9), found in the photo archive of the Pompeii Archaeological Park, dating to the early excavations of the Room A in 1988–1989. The photo was taken from the southern sector (latrine) looking at the western sector which is filled with a thick pumice lapilli deposit. Just behind the PW2, the sloping surface of the pumice deposit is visible. We cannot completely exclude that the sloping surface of the pumice deposit may have been partly accentuated by the excavation itself. However, this is further evidence in favour of the conclusion that the thickness uniformness and the horizontal surface resulted from the past archaeological excavations. Consequently, we suggest that the EU2f deposit is the residue of an accumulation of pumice lapilli that penetrated through the door, along the north-western perimeter wall, which is completely blocked by a lapilli deposit (filling the still buried room 21; Figure 3). The grey phase (EU3f) was recorded in the northern sector of room A by the presence of a grey pumice lapilli heap, whose apex corresponded with the window facing the east alley of the insula. The 79 CE pyroclastic sequence in the east alley (close to rooms A and 22) was about 5 m thick, of which 3.1–3.3 m of pumice lapilli (Patti, 2003; de Sanctis et al., 2019; 2020), although this thickness was not uniform and could rapidly vary along the alley due to the sliding/rolling of pumice off sloping roofs (thickening) and/or erosion (thinning) exerted by the successive pyroclastic currents. By taking into account an almost equal proportion between white and grey pumice at Pompeii (Cioni et al., 1990; 1992), a thickness of ∼1.6 m is calculated, respectively, for EU2f and EU3f. Considering that the base of the window is 1.7 m above the ground level of the alley and that the base of EU3f was not higher than 1.6 m, we can conclude that the grey pumice progressively buried the window, penetrated the room through it and extended towards the sides of the room (Figure 8A). The same dynamics can be suggested for room 22 with pumice lapilli penetrating that space through the door along the south-western wall (opening on the unexcavated room 21; Figure 3) and through the windows along the north-eastern wall facing the alley. After the end of the grey pumice fallout (around 7 a.m. on the second day), the collapse and break-up of the partition walls in room A occurred (whose causes are investigated in the following sections) likely causing, in turn, the crumbling of a wooden attic and the death of the two individuals who tried to seek shelter in this room. The wall failure caused the white pumice lapilli from the western sector to slide and partly penetrate the northern sector, through breaches in the PW1, covering the collapse units (Figure 8A). The absence of ash coating or matrix suggests that the sliding of the pumice lapilli occurred before the arrival of the pyroclastic currents. Following the ultimate collapse of the Plinian column and the beginning of the caldera-forming to phreatomagmatic phase of the eruption, a succession of pyroclastic currents overran the city. The pyroclastic currents that emplaced the EU3pftot unit were unable to penetrate the rooms. The successive EU4pf parental current arrived from the north-west and collided against the north-western wall of room 22 that happened to be perpendicular to the flow direction (Figure 8B). The dynamic pressure associated with the flow process caused the wall to be pulled down south-eastward (further evidence that led us to this conclusion is discussed in the following section). The capability of pyroclastic currents to damage buildings is testified by experimental studies (e.g., Valentine, 1998; Nunziante et al., 2003; Doronzo and Dellino, 2011; Zuccaro and Ianniello, 2004; Spence et al., 2004a; 2004b; Baxter et al., 2005), specific field-based studies in Pompeii (Varone and Marturano, 1997; Cioni et al., 2000a; 2000b; Luongo et al., 2003a; Gurioli et al., 2005; Marturano and Varone, 2005), as well as the estimation of the flow dynamic pressure based on external fragments found in EU4pf and entrained by the pyroclastic current (Doronzo et al., 2022) or on the structural analysis of the damage to walls (Ruggieri et al., 2020). The presence of an ash layer, up to 22 cm thick, underlying the collapsed wall fragments suggest that the toppling was instantaneous but did not happen at the first arrival of the pyroclastic current (probably due to the partial protection of buildings located in the northern sector of the Insula dei Casti Amanti which is still buried). The pyroclastic current had time to penetrate the room, likely from openings not completely buried by the pumice lapilli, emplacing the ash on top of the lapilli deposit. The dynamic pressure exerted by the pyroclastic current, then, caused the north-western wall to rapidly collapse, crumbling on the ash deposit and breaking into two fragments upon landing, and then being entombed by the same ash. The pyroclastic current travelled south-eastward across room 22 then, after a few meters, collided against the successive perimeter wall (separating rooms 22 and A; Figure 3; Figure 8B). The pyroclastic current was unable to damage such wall likely as a consequence of the temporary loss of kinetic energy caused by interaction with the previous wall (Luongo et al., 2003a; Gurioli et al., 2005), after which it had mainly a depositional behaviour. The current penetrated the room A (Figure 8A), progressively emplacing an ash deposit that capped the skeletons of the two individuals and the collapse units. The stratigraphic position of the main collapse unit in room A suggests that the wall failure occurred after the end of the Plinian phase (that lasted about 18 h) and before the first arrival of the EU4pf parental current in the early morning of the second day (from 8 a.m.). The death of the two individuals, whose skeletons are strictly related to the collapse of the partition walls, falls within the same chronostratigraphic interval.
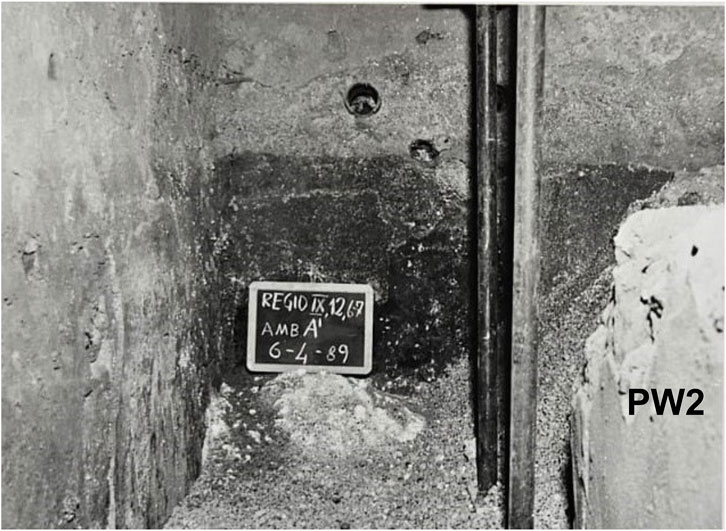
Figure 9. Photo showing the early excavations of the Room A in 1988–1989 (photo archive of the Pompeii Archaeological Park). The photo was taken from the southern sector (latrine) looking at the western sector (kitchen) that appears filled with pumice lapilli showing a sloping surface (behind the PW2). The blackboard, reporting the date 6 April 1989, is placed on a pile of lime resting on the kitchen counter (still buried in the photo).
7.3 Comparison between different types of structural damage
The accumulation of pumice lapilli during the Plinian phase of the eruption, with an average sedimentation rate of 15 cm/h (Sigurdsson et al., 1982; 1985) in open space, caused several roofs to collapse under the vertical load of the accumulating deposit on flat or low-sloping surfaces (Cioni et al., 2000a; 2000b; Luongo et al., 2003a; Giacomelli et al., 2003). Typically, the damage is represented by abundant roof/attic debris and tiles, intact or in fragments, smashed on the ground and covered by the pumice lapilli or embedded within it (Figure 10A). Most of roof collapses occurred a few hours after the beginning of the Plinian phase, under a deposit thickness of 40–60 cm (Sigurdsson et al., 1985; Cioni et al., 1990; 1992; Luongo et al., 2003a) during the emplacement of EU2f. A vertical load may also result in the failure of vertical structures (e.g., Martini et al., 1998; Sandoval et al., 2011), such as walls or columns, which could be a secondary consequence of the roof collapse (Spence et al., 2005). This mechanism has been invoked to explain the crumbling of walls locally associated with roof collapses at Pompeii (Luongo et al., 2003b; Giacomelli et al., 2003). In room A of the house of the Painters at Work, the lack of any evidence of roof collapse on the ground, and the presence of an accumulation of grey pumice lapilli penetrated through a window, suggest that the roof was still in place by the end of the Plinian phase. This is likely due to the presence of a sloping roof that drained most of pumice lapilli into the east alley and, consequently, reduced the progressive increase of the vertical load. In addition, the collapsed partition walls had no load-bearing function so that the vertical forces were mainly discharged on the perimeter (load-bearing) walls. A direct cause-effect relationship between the accumulation of pumice lapilli and the collapse unit in room A can be confidently excluded. Similar considerations lead to rule out the effects of the weight of the pumice lapilli deposit as triggering mechanism for the wall collapse in room 22: 1) no evidence of roof collapse has been found on the ground or embedded in the pyroclastic sequence; 2) pumice lapilli penetrated through the openings; 3) the room is bounded to east by the alley and to west by an open space (courtyard) suggesting the presence of a sloping roof (or a canopy towards the courtyard) that drained most of pumice lapilli.
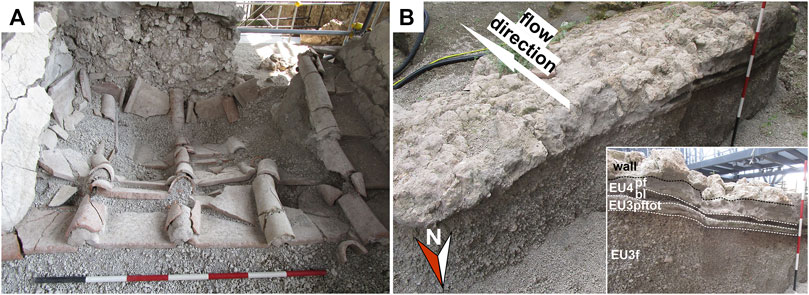
Figure 10. Examples of damage caused by primary volcanic phenomena in the Insula dei Casti Amanti (IX.12): (A) collapsed roof, unearthed during a recent (2023) excavation in the “Casa del Cenacolo Colonnato II”, resulting from a vertical load, found embedded in the pumice lapilli fall deposit; (B) wall pulled down (south-eastward) in the south-western sector of the house of the Painters at Work (close to the west alley); this finding dates to the mid-1990s. Literature sources (Varone and Marturano, 1997; Luongo et al., 2003a; Marturano and Varone, 2005) attribute the wall failure to the dynamic pressure exerted by the EU4pf parental current. This wall lies at the same stratigraphic height and shares the same attitude and orientation as the toppled wall found in room 22 of the same house. Inset: detail of the 79 CE stratigraphy underlying the wall.
After the end of the Plinian phase, a series of pyroclastic currents invaded Pompeii. Among these, the EU4pf parental current is regarded to have triggered building collapses and wall damage (Sigurdsson et al., 1985; Cioni et al., 1992; 2000a; 2000b; Luongo et al., 2003a; Gurioli et al., 2005). As evidenced by Luongo et al. (2003a), through the evaluation of the destruction pattern in the house of Iulius Polybius (Figure 1), the EU4pf parental current (their unit E) was highly mobile and density-stratified (i.e., particle concentration increases toward the base of the current). The concentrated basal part was channelled, partially deflected by the buildings, while the upper diluted part was unaffected by the obstacles moving undisturbed above the buildings (Gurioli et al., 2005; 2007). Luongo et al. (2003a) suggested that the orientation of the walls with respect to the flow direction appears to be a fundamental parameter for the assessment of the damage, as also reported by Spence et al. (2004a) by modelling the building vulnerability to pyroclastic currents. Walls parallel (NW-SE oriented) to the current direction were minimally or not damaged, while walls perpendicular (NE-SW oriented) to the current direction suffered severe damage being toppled or knocked down, resting on a few centimeters of ash emplaced by the pyroclastic current. A similar conclusion is provided by Varone and Marturano (1997) and Marturano and Varone (2005) interpreting a large collapsed wall in the south-western sector of the house of the Painters at Work (Figure 10B). Where successive walls (two or more) are closely spaced, the depth and the amount of the damage appears lower downcurrent (Luongo et al., 2003a). Dynamically, these observations suggest that repeated and closely spaced wall-pyroclastic current interactions forced particles to settle from the depositional system, with a consequent loss of mass resulting, in turn, in a decrease of kinetic energy. These characteristics are fully consistent with those of the damaged wall in room 22 whose demolition was reliably the result of the impact of the EU4pf parental current. In detail, the evidence of a pyroclastic current-induced failure is: 1) The wall happens to be perpendicular to the flow direction; 2) the wall collapsed south-eastward (in the direction of the flow) resulting in toppling; 3) the north-western wall shows a channel-shaped breach; 4) the wall fragments rest on a few centimetres of ash emplaced by the EU4pf parental current.
Nevertheless, we report that not all the scientific community agrees that Pompeii suffered the destructive effects of the pyroclastic currents. Dellino et al. (2021) modelled the impact of the EU4pf parental current on buildings and humans at Pompeii through the estimation of flow physical parameters, among which the dynamic pressure. According to these authors, the EU4pf flow dynamic pressure had a maximum in the first few decimeters and decreased higher in the current to values lower than 1 kPa, suggesting that no severe mechanical damage to walls should be expected with such a flow strength. The model is based on laboratory analyses on samples extracted from the deposit and a field survey carried out in the house of Stabianus (Figure 1), in the south-eastern part of Pompeii, where human corpses as well as tiles and wooden poles (Luongo et al., 2003b; Scandone et al., 2019; Giacomelli et al., 2021) have been embedded in the EU4pf ash deposit, with no details about the relationship between the structure (and the characteristics of the structural damage) and the 79 CE stratigraphy that, in our opinion, is an aspect as decisive as the estimation of the flow dynamic pressure when assessing the damage. Views that differ from that of Dellino et al. (2021) are provided by other authors (Nunziante et al., 2003; Ruggieri et al., 2020; Doronzo et al., 2022) through different estimations of the flow dynamic pressure. Based on the stratigraphic survey and structural analysis of a Roman villa at Terzigno (5 km E-SE of Vesuvius; Figure 1), Nunziante et al. (2003) suggested that values of dynamic pressure up to 5 kPa (range 1–5 kPa) were sufficient to cause the failure of masonry buildings. Doronzo et al. (2022) suggested that the presence in Pompeii of decimeter-sized building fragments locally incorporated in the EU4pf ash deposit, as reported by Gurioli et al. (2007), and possibly entrained and transported by the pyroclastic current, is evidence of dynamic pressures up to 5–10 kPa. Finally, Ruggieri et al. (2020) estimated dynamic pressures up to 30 kPa through the analysis of the damage to the northern Pompeii city walls, near the Tower XI (Figure 1) also referred to as Torre di Mercurio (Mercury’s Tower). In this site, these authors detected the sliding of large blocks, on both the inner and outer leaves of the city walls, that they attributed to the impact of a pyroclastic current (likely the EU4pf parental current). In addition, other aspects should be considered as they affect the strength of the buildings and, therefore, the response to external forces: 1) the construction technique and the quality of masonry structures (e.g., Spence et al., 2004a; Zuccaro et al., 2008; Autiero et al., 2020) and 2) the potential structural preconditioning caused by pre- or syn-eruptive seismicity that would reduce the resistance of the buildings and increase their vulnerability (e.g., Zuccaro et al., 2008), possibly resulting in structural collapses triggered even by relatively low values of dynamic pressure. Given these discrepancies concerning the dynamic pressure values and limit behaviour of the ancient Roman masonry, we rely our interpretation on the characteristics, attitude, and stratigraphic height of the damage, engulfed in the 79 CE pyroclastic sequence, that match those reported in the available field-based studies in Pompeii. Nonetheless, we have attempted a rough estimate of the flow dynamic pressure, which possibly triggered the wall collapse in room 22, by combining geometrical parameters of the wall, measured in the field, and mechanical parameters of Roman masonry structures derived from literature. Nunziante et al. (2003) provided a correlation between the limit tensile strength of Roman masonry walls, having thickness in the range 40–60 cm, and values of dynamic pressure that make the walls unstable and prone to collapse. By means of experimental tests, the authors estimated values of tensile strength in the order of 102 kPa. Assuming an average tensile strength of 1.5×102 kPa (Nunziante et al., 2003), a wall thickness (45 cm) in the range 40–60 cm, and considering the reduction of the wall surface exposed to the passage of the pyroclastic current (due to the partial burying in the pumice lapilli deposit during the Plinian phase), a flow dynamic pressure of 3–4 kPa is roughly estimated. For the sake of clarity, we stress that our interpretation of the collapsed wall in room 22 is based on observations and data collected in a limited area with respect to the areal extent of Pompeii. The same applies to the literature studies concerning the impact of the EU4pf parental current, based on both the modelling of the pyroclastic current behaviour and field-based analysis of the relationship between the pyroclastic deposits and damage. This implies that the results do not necessarily represent the physical behaviour of the pyroclastic current at the scale of the city, which may vary in space due to the disturbance caused by the urban environment (Gurioli et al., 2005; 2007).
Regarding the main collapse unit in room A, based on the stratigraphic data, we can clearly rule out a contribution of the EU4pf parental current to the wall failures. However, we provide other evidence to further constrain our conclusion: 1) the PW1, striking approximately NW-SE, happens to be parallel to the general flow direction, 2) the north-western perimeter wall (shared with the room 22, Figure 3), oriented approximately NE-SW (perpendicular to the flow direction), does not show any type of damage likely due to the protection of the adjoining room 22 to the north, 3) the large masonry fragment, behaving as a monolithic block, was horizontally displaced towards the north-east and then slipped down implying a hypothetical flow dynamic pressure coming from the south-west, 4) the wall was originally confined to the west by a pumice lapilli deposit, which filled most of the western sector (Figure 9), that protected the wall itself from the impact of the pyroclastic current; 5) no overturning or toppling occurred as a consequence of the dynamic solicitation. Ultimately, no cause-effect relationship can be invoked between the EU4pf parental current and the collapse unit in room A.
7.4 The archaeoseismological perspective: exclusion of other damaging events
After ruling out primary volcanic phenomena as the cause of the wall failures in room A, we can look for other possible damaging events like the effects of structural decay due to aging and gravity (e.g., Marco, 2008; Galadini, 2009). As the collapse units in room A have been engulfed by primary volcanic deposits during a geologically instantaneous event (volcanic eruption) that has frozen the exact moment of the destruction, the contribution of aging or gravity for the structural decay, as well as the action of roots and soil flow, cannot be invoked. We can also exclude the contribution of spoliation or exploratory tunnels as they usually appear as roughly circular breaches through the walls filled with anthropically reworked material.
Damage to archaeological structures caused by transient seismic shakings, defined as Earthquake Archaeological Effects (EAEs, Rodrìguez-Pascua et al., 2011; Rodrìguez-Pascua et al., 2023) or Potential Earthquake Archaeological Effects (PEAEs, Jusseret et al., 2013) are diverse, ranging from penetrative fractures to rotated, displaced or collapsed masonry blocks, walls or columns. Seismic destruction usually involves the rapid collapse of large wall fragments, locally associated with accumulations of coarse debris having maximum thickness close to the collapsed wall (e.g., Galadini, 2009). Lateral failure and displacement along a horizontal plane and subsequent collapse of unreinforced masonry walls, whose capability to accommodate the deformation is low (Martini, 1998; Fonti, 2016), require horizontal forces that are consistent with earthquake motion (Martini, 1998; Marco, 2008; Martìn-Gonzáles, 2018). This is the described case of room A, where the PW1 was characterized by a sub-horizontal shear plane above which the large masonry fragment experienced a monolithic behaviour, being displaced (Figure 4; Figure 8A) and then slipped down. The shear plane and displacement likely occurred along the weakest mortar joints through the stone blocks of the opus incertum as demonstrated by Autiero et al. (2020) through experimental tests on reproduced full-scale masonry panels complying with this building technique. This type of failure is also referred to as “out-of-plane” because it results from a load operating perpendicular to the wall surface as opposed to the “in-plane” load acting parallel to the wall surface and, usually, resulting in diagonal cracking (e.g., Martini, 1998; Vanin et al., 2020). Furthermore, as usually observed after modern earthquakes which damaged historical buildings, the collapse of the large masonry fragment was accompanied by the break-up of the adjacent parts of the wall, forming accumulations of debris composed of stones within a matrix of mortar and plaster fragments, giving a picture which has already been sketched as consistent with archaeoseismological evidence (e.g., Galadini, 2009). The seismic hypothesis is also supported by volcanological data that chronologically place the occurrence of the wall failures in room A at the beginning of caldera-forming phase of the eruption, which was accompanied by intense seismicity (see the paragraph about the syn-eruptive seismicity). Furthermore, other evidence suggests the occurrence of shakings also during the Plinian phase. Indeed, the presence of a number of plaster fragments interspersed in the grey pumice lapilli heap, detached from the surrounding walls, may be consistent with low-amplitude volcanic tremors. Similarly, Cioni et al. (2000a; 2000b) reported pieces of mortar lying directly on the ground at Villa dei Papiri, Herculaneum (∼7 km south-west of Vesuvius; Figure 1), resulting from precursory or syn-eruptive (Plinian phase) shakings.
Regarding the toppled wall in room 22, our data point to a structural failure triggered by the EU4pf parental current. However, the hypothetical contribution of an earthquake-induced (both pre- or syn-eruptive) structural preconditioning cannot be completely excluded, even though it cannot be traced. The Pompeian geoarchaeological stratigraphy often records only the last or the most significant damaging event of a sequence. This means that it might not be possible to trace an early earthquake-induced weakening of a masonry structure (e.g., small fractures with no displacement can form) because the effects of the subsequent damaging event (pyroclastic current) can completely obliterate the (minor) seismic damage, recording only the effects of the last event as in the described case in room 22.
The whole picture can also benefit from taking into account the local geological characteristics possibly conditioning the site effects (e.g., Amato et al., 2022; Hinojosa, 2023). From a lithostratigraphic perspective, the ancient Pompeii was built on a small hill serving as lithoid substrate, made up of lavas and welded pyroclastic deposits, covered by loose to compacted sediments consisting of pyroclastic deposits (thin layers of ash and lapilli resulting from pre-79 CE eruptions), buried soils, reworked volcaniclastic deposits and anthropogenic cuts and infills, sealed by the 79 CE eruption (Amato, 2021; Amato et al., 2022). From a geomorphological perspective, the surface of the lithoid substrate is characterized by different landforms as small crater rims, isolated peaks, elongated ridges, and relatively flat areas. This uneven superficial morphology of the lithoid substrate involves a variable thickness of the cover terrains, from absent to a few meters (Amato, 2021; Amato et al., 2022). By merging these geological and geomorphological factors, along with geophysical data (seismic tomography), Amato et al. (2022) proposed a seismic microzonation of the entire Pompeii archaeological site. Regarding the Insula dei Casti Amanti, subsoil profiles along the alleys, drawn through borehole data, suggest the presence of a small morphological depression (lithoid substrate) in which the pre-79 CE sequence of cover terrains thickens, attaining a total thickness of at least 4–5 m (Patti, 2003; de Sanctis et al., 2019; 2020; Amato et al., 2022). Such characteristics, according to the investigation by Amato et al. (2022), make the area of the Insula dei Casti Amanti prone to site effects, possibly associated with Vp and Vs wave velocity between 300 and 600 m/s. The possibility that the local amplification of seismic motion contributed to the observed damage will be investigated in detail during a future-planned campaign of ambient vibration measurements.
7.5 Causes of death
Causes of death in Pompeii during the 79 CE eruption are being debated since long time. Great attention has been given to the modes of death caused by the arrival of the pyroclastic currents and referred to as lethal thermal effects due to heat, asphyxiation due to inhalation of fine ash particles or, very rarely, mechanical impact (Baxter, 1990; Luongo et al., 2003b; Giacomelli et al., 2003; Mastrolorenzo et al., 2010; Dellino et al., 2021; Mastrolorenzo, 2021; Alapont et al., 2023). In addition, the duration of the pyroclastic current, and consequently the exposure time to an ash-rich environment, has been identified as a life-threatening factor contributing to asphyxia (Dellino et al., 2021). Conversely, much less attention has been given to the human remains of individuals who perished during the Plinian phase of the eruption. They are generally regarded as victims of the collapse of the roofs resulting from the overload of the pumice lapilli deposit, if found inside buildings, or killed by the crumbling roof tiles or by large lithic fragments falling from the eruptive column or emplaced as ballistics, if found outdoors (Luongo et al., 2003b; Giacomelli et al., 2003). Moreover, Zanella et al. (2007), based on thermal remanent magnetization data, suggested that the pumice fall deposit was emplaced hot enough to heat up cold material to 120–140 °C, speculating that it would have been able to cause skin burn and carbonization of wood. Luongo et al. (2003b) reject this hypothesis suggesting that the Plinian phase resulted in a cold deposit.
The direct stratigraphic relationship between the two skeletons and the large collapsed masonry fragments (Figure 5) suggests that the death of both individuals is concurrent with the wall failures, whose timing during the eruption has been volcanologically well-constrained. This information allows us to rule out any lethal effect associated with the pyroclastic current phase as cause of death. Their stratigraphic position above the grey pumice lapilli suggests that they were still alive by the end of the Plinian phase, allowing the exclusion of any hypothetical thermal effect of the lapilli deposit. Moreover, taking into account that the grey pumice lapilli progressively penetrated the room through the window facing the eastern alley, the lack of charred wood fragments suggests that the resulting deposit was nearly cold (only the faint trace of a rotted object has been observed above the individual 2’s skeletal remains).
The perimortal traumatic pattern of fractures (thoracic cage, pelvis, skull, limbs) is consistent with extremely severe compression traumas, likely having fatal consequences, caused by the collapse of the partition walls which was triggered, as previously discussed, by a seismic solicitation. For the individual 2, a contribution of asphyxia in a confined space cannot be totally excluded. It is worth noting that such traumas are analogous to those of individuals involved in modern earthquakes (e.g., Naghi et al., 2005; Dong et al., 2010; Tian-wu et al., 2011) providing a further constrain to our interpretation pointing to the contribution of the seismic destruction.
Our conclusions suggest that the effects of the collapse of buildings triggered by syn-eruptive seismicity should be regarded as an additional cause of death in the ancient Pompeii other than those reported in literature. In this light, in a broader view that takes into account the whole city, we consider, as a working hypothesis, that the casualties caused by seismically triggered building failures may not be limited to the two individuals described in this study. According to literature sources (Luongo et al., 2003b; Giacomelli et al., 2003; Scandone et al., 2019), a total of 394 bodies were recovered in the pumice lapilli deposit resulting from the Plinian phase. Of these, 345 were found inside the houses and are thought to have been killed by roof collapses resulting from the weight of the lapilli deposit (Luongo et al., 2003b; Giacomelli et al., 2003). We hypothesize that a number of roofs, which were able to withstand the accumulation of pumice lapilli, may have crumbled shortly after the end of the Plinian phase as a consequence of the strong earthquakes that accompanied the caldera formation. This hypothesis would imply that a number of people who survived to the Plinian phase, and did not attempt to flee their houses after the cessation of the lapilli fallout, may have been overwhelmed by earthquake-induced collapses of roofs loaded by pumice lapilli. The same applies to the roof collapses and deaths that occurred during the Plinian phase. Some of them may have been triggered by earthquakes occurred during the night between the first and second day of the eruption and reported by Pliny the Younger. During modern archaeological excavations, the systematic attribution of the roof failures to the overload of the pumice lapilli possibly resulted in a misinterpretation of the actual triggering mechanism of some of them. The syn-eruptive seismicity probably also played a role in the choices made by the victims of the second phase of the eruption as inferred from their areal distribution (indoor vs. outdoor areas). A total of 650 bodies were recovered in the pyroclastic current deposits (Luongo et al., 2003b; Giacomelli et al., 2003; Scandone et al., 2019); the vast majority were in the EU4pf ash deposits (Luongo, 2003b) while a few victims have been associated with the EU3pftot unit (e.g., Cioni et al., 2000a; Marturano and Varone, 2005). The victims of the pyroclastic current phase are almost equally distributed between indoor (334) and outdoor (316) areas (Luongo et al., 2003b; Giacomelli et al., 2003; Scandone et al., 2019). According to Scarpati et al. (2020), the surviving inhabitants were encouraged to leave their houses or shelters by brief breaks in the succession of volcanic events at Pompeii, represented by stratigraphic hiatuses in the 79 CE pyroclastic sequence consisting of erosion surfaces filled with reworked sediments. Such brief breaks preceded and followed the emplacement of the EU4bl fall layer (their unit D), resulting from a short-lived (about half an hour) eruption column (Scarpati et al., 2020; Chiominto et al., 2023), which anticipated the most devastating and lethal pyroclastic current. It has also been speculated that some survivors who had left their houses at the first signs of unrest, or during the initial phase of the eruption, attempted to return, likely to recover their possessions, by taking advantage of the temporary decline of the volcanic phenomena (e.g., Lirer et al., 1997). However, the evidence of severe, syn-eruptive (caldera collapse phase) seismic damage, provided in this study, suggests that the significant seismicity possibly contributed to push some survivors, still in Pompeii, to flee their refuges (and discouraging the return of most of those who had left), seeking safety outside, then being caught by the arrival of the EU4pf pyroclastic current along roads/alleys and in open spaces, as already speculated by Scandone et al. (2019).
8 Conclusion
Modern volcanology has greatly benefitted from studies concerning the destructive effects of the 79 CE Plinian eruption in Pompeii. This study provides new observations and data on underrated/neglected effects of the eruption through different investigative approaches. Our results represent a starting point for an updated view of the destruction of Pompeii, by considering the syn-eruptive seismicity as a contributing cause of building collapse and death of the inhabitants, and open new perspectives of research, e.g., the interplay between volcanic and seismic processes during the 79 CE eruption, the investigation of ancient seismic effects in volcanic areas, and paleopathological studies on individuals who suffered the consequence of building collapses.
Previous studies in Pompeii have shed light on of how volcanic phenomena interacted with buildings but new information can be gained. The available data indicate that the effects of the seismicity related to the 79 CE eruption cannot be neglected and that a volcanic eruption may imply damaging effects even in absence of destruction directly related to primary volcanic phenomena. The recognition of the effects of any volcanic/seismic event and the spatial distribution of the associated damage can lead to a cumulative map of destruction layers and related timing during the 79 CE eruption. The response and resistance of buildings to seismic actions is fundamental to assess the damage, particularly when combined with vertical loads due to tephra accumulation and dynamic pressure owing to lateral flow processes (Baxter et al., 2005; Zuccaro et al., 2008). Therefore, our next initiatives related to other excavation sites in Pompeii, where evidence consistent with past seismicity is being uncovered, will also involve widespread analysis of site effects, modelling of the dynamic solicitation and structural response of the ancient buildings bearing traces of damage/destruction, in addition to detailed stratigraphic reconstructions. This can also have implications for the mitigation of seismic vulnerability of the archaeological structures in Pompeii (Fonti, 2016; Calvanese and Zambrano, 2021; Satta et al., 2021). In this light, a glance to the Seismic Hazard Map of Italy (Stucchi et al., 2004) seems opportune. The Vesuvius and Pompeii area, along with other peri-Tyrrhenian volcanic areas of peninsular Italy, is included in the Zone 2 that comprises territories that may be affected by quite strong earthquakes. Based on a seismic source zone model, that in the investigated area is derived from the regional historical seismicity (Meletti et al., 2008), the hazard map and the related classification clearly claim for building techniques and interventions that should prevent seismic damage comparable to that associated with the syn-eruptive seismicity. Therefore, the described archaeoseismological case in the Insula dei Casti Amanti, in Pompeii, provides further evidence of the seismic characteristics summarized in the Hazard Map and represents a new incentive to keep attention focused on the importance of the defence against earthquakes in the Vesuvius volcanic area.
The death of two individuals, caused by seismically triggered wall failures, has implications for a lesser-known branch of paleopathological studies of victims of ancient earthquakes, for which very few cases exist in Italy (e.g., Galadini et al., 2010). Also in this case, the multidisciplinary approach is a fundamental tool. The traumatic pattern of bone fractures alone, resulting from compression traumas associated with building collapses, is not indicative of a specific triggering mechanism of the collapse (e.g., Guglielmi et al., 2011). The recognition of multiple bone fractures and the evaluation of the severity of the skeletal crush injuries, compatible with compression traumas having fatal consequences, of human and/or animal remains recovered in archaeological areas may provide important clues that, associated with the local geological and archaeoseismological picture, can contribute to the assessment of the collapse dynamics possibly pointing to the seismic destruction triggered by past and poorly known earthquakes. The comparison of the traumatic pattern with that showed by casualties (or survivors showing severe traumas) of modern earthquakes can further constrain the seismic hypothesis.
Finally, our work emphasizes that a multidisciplinary approach is necessary, even decisive, in the assessment of the damage and the cause-effect relationship during future excavations in Pompeii. This is a challenging task, due to the interplay between volcanic and seismic phenomena, that can be achieved through a synergistic work between archaeologists and earth scientists (and anthropologists and/or archaeozoologists if human/animal remains are recovered), devoid of any preconceived archaeological and/or volcanological scheme in the wake of what has been done in this work.
Data availability statement
The original contributions presented in the study are included in the article/Supplementary material, further inquiries can be directed to the corresponding author.
Ethics statement
Ethical approval was not required for the studies involving humans because the anthropological part of this multidisciplinary study deals with skeletal remains of individuals who died as a consequence of the 79 CE eruption. No living human being has been involved. The guidelines of the Italian Ministry of Culture have been applied. The studies were conducted in accordance with the local legislation and institutional requirements. The human samples used in this study were acquired from skeletal remains recovered during an archaeological excavation. Written informed consent to participate in this study was not required from the participants or the participants᾽ legal guardians/next of kin in accordance with the national legislation and the institutional requirements.
Author contributions
DS: Conceptualization, Data curation, Formal Analysis, Investigation, Methodology, Validation, Visualization, Writing–original draft, Writing–review and editing. VA: Conceptualization, Data curation, Formal Analysis, Investigation, Methodology, Validation, Visualization, Writing–original draft, Writing–review and editing. FG: Conceptualization, Data curation, Formal Analysis, Investigation, Methodology, Validation, Visualization, Writing–original draft, Writing–review and editing. MADV: Writing–original draft, Writing–review and editing, Validation, Visualization. AT: Writing–original draft, Writing–review and editing, Data curation, Investigation. GS: Writing–original draft, Writing–review and editing. GZ: Writing–original draft, Writing–review and editing, Project administration.
Funding
The author(s) declare that no financial support was received for the research, authorship, and/or publication of this article.
Acknowledgments
We are grateful to Saverio De Rosa, Giovanni Ricci and Alessandro Russo for precious discussions about archaeological issues. Marina Loddo is acknowledged for her help in the literature search. Sophie Hay is thanked for the critical reading of the manuscript. Comments and suggestions provided by Salvatore Passaro and Fabio Dioguardi, and the editorial work by Roberto Sulpizio and Valerio Acocella greatly contributed to improve the manuscript.
Conflict of interest
The authors declare that the research was conducted in the absence of any commercial or financial relationships that could be construed as a potential conflict of interest.
Publisher’s note
All claims expressed in this article are solely those of the authors and do not necessarily represent those of their affiliated organizations, or those of the publisher, the editors and the reviewers. Any product that may be evaluated in this article, or claim that may be made by its manufacturer, is not guaranteed or endorsed by the publisher.
References
Adam, J. P. (1989). “Osservazioni tecniche sugli effetti del terremoto di Pompei del 62 d,” in C. I terremoti prima del Mille in Italia e nell'area Mediterranea. Editor E. Guidoboni (Bologna: ING), 460–474.
Akyüz, H. S., and Altunel, E. (2001). Geological and archaeological evidence for post– Roman earthquake surface faulting at Cibyra, SW Turkey. Geodin. Acta 14 (1-3), 95–101. doi:10.1080/09853111.2001.11432437
Alapont, L., Gallello, G., Martinón-Torres, M., Osanna, M., Amoretti, V., Chenery, S., et al. (2023). The casts of Pompeii: post-depositional methodological insights. Plos one 18 (8), e0289378. doi:10.1371/journal.pone.0289378
Albrecht, L., and Döring-Williams, M. (2023). The partial collapse of the basilica of maxentius in late antiquity: an evaluation of possible destruction causes. In: ed. Pecchioli, L. Archaeoseismology. Methodologies and case studies. Natural science in archaeology, Springer Nature, 39–59. doi:10.1007/978-3-031-28303-1_3
Allison, P., 2003. Recurring tremors: the continuing impact of the AD 79 eruption of Mt Vesuvius. In: Natural disasters and cultural change, e.d. Grattan, J., and Torrence, R. London, 107–125.
Allison, P. M. (1995). “On-going seismic activity and its effects on the living conditions in Pompeii in the last decades. In Archäologie und Seismologie,” in Archäologie und Seismologie. La Regione Vesuviana dal 62 al 79 d. C. Problemi archeologici e sismologici. Editor D. A. I. Römische Abteilung, 183–190. Soprintendenza Archeologica di Pompei, Osservatorio vesuviano, Biering and Brinkmann, München, Germany, Colloquium di Boscoreale, 26–27 novembre 1993.
Altamura, F. (2022). Comment on “The 79 CE eruption of Vesuvius: A lesson from the past and the need of a multidisciplinary approach for developments in volcanology” by Doronzo et al., 2022 (Earth-Science Reviews 231, 104072). Earth Sci. Rev. 236, 104266. 2022 (Earth-Science Reviews 231, 104072. doi:10.1016/j.earscirev.2022.104266
Altunel, E., Meghraoui, M., Karabacak, V., Akyüz, H. S., Ferry, M., Yalçıner, Ç., et al. (2009). Archaeological sites (tell and road) offset by the dead sea fault in the amik basin, southern Turkey. Geophys. J. Int. 179 (3), 1313–1329. doi:10.1111/j.1365-246X.2009.04388.x
Amato, V. (2021). “L’approccio geoarcheologico per la ricostruzione crono-stratigrafica del sottosuolo di Pompei: L’esempio dei saggi di scavo all’Insula dei Casti Amanti,” in Ricerche e Scoperte a Pompei: In Ricordo di Enzo Lippolis. Editor M. Osanna (Rome: L’Erma di Bretschneider), 45, 117–130.
Amato, V., Covolan, M., Dessales, H., and Santoriello, A. (2022). Seismic microzonation of the Pompeii archaeological Park (southern Italy): local seismic amplification factors. Geosciences 12 (7), 275. doi:10.3390/geosciences12070275
Ambraseys, N. N. (2006). Earthquakes and archaeology. J. Archaeol. Sci. 33 (7), 1008–1016. doi:10.1016/j.jas.2005.11.006
Amoretti, V., Martellone, A., Perrotta, A., Scarpati, C., and Virtuoso, T. (2021). Nuovi dati stratigrafici, tafonomici e vulcanologici dalla Regio V: il calco mancato dell'Ultimo fuggiasco. In: I calchi di Pompei da Giuseppe Fiorelli a oggi, e.d. Osanna, M., Capurso, A., and Masseroli, S. M., L'Erma di Bretschneider, Rome: Studi e Ricerche del Parco Archeologico di Pompei, 19–28.
Argento, T., Falanga, M. F., Ferrara, D., Scarpa, M. G., Spinosa, A., Tartari, M., et al. (2024). Interventi conservativi e nuovi scavi nella Casa di Leda. Dalla tutela alla conoscenza di un’abitazione del ceto medio di Pompei. E-Journal degli Scavi Pompei 1. Pompei: Ed. Parco Archeologico di Pompei. Available at: https://pompeiisites.org/wp-content/uploads/01_E-Journal-Casa-di-Leda-1-1.pdf.
Arzilli, F., Morgavi, D., Petrelli, M., Polacci, M., Burton, M., Di Genova, D., et al. (2019). The unexpected explosive sub-Plinian eruption of Calbuco volcano (22–23 April 2015; southern Chile): triggering mechanism implications. J. Volcanol. Geotherm. Res. 378, 35–50. doi:10.1016/j.jvolgeores.2019.04.006
Autiero, F., Martino, G., Di Ludovico, M., and Prota, A. (2020). Mechanical performance of full-scale Pompeii-like masonry panels. Constr. Build. Mat. 251, 118964. doi:10.1016/j.conbuildmat.2020.118964
Baxter, P. J. (1990). Medical effects of volcanic eruptions: I. Main causes of death and injury. Bull. Volcanol. 52, 532–544. doi:10.1007/BF00301534
Baxter, P. J., Boyle, R., Cole, P., Neri, A., Spence, R., and Zuccaro, G. (2005). The impacts of pyroclastic surges on buildings at the eruption of the Soufrière Hills volcano, Montserrat. Bull. Volcanol. 67, 292–313. doi:10.1007/s00445-004-0365-7
Bertoldi, F. (2009). “Determinazione del sesso e dell’età alla morte,” in Non omnis moriar. Editors F. Mallegni, and B. Lippi, 31–42.
Braccini, G. C. (1632). Dall’incendio fattosi sul Vesuvio a XVI Dicembre MDCXXXI e delle sue cause ed effetti, con la narrazione di quanto è seguito in esso per tutto marzo 1632 e con la storia di tutti gli altri incendi, nel medesimo monte avvenuti. Napoli: Roncagliolo Secondino, 224.
Brooks, S., and Suchey, J. M. (1990). Skeletal age determination based on the os pubi.s: a comparison of the Acsadi-Nemeskeri and Suchey-Brooks methods. Hum. Evol. 5, 227–238. doi:10.1007/bf02437238
Calvanese, V., Mighetto, P., and Spinosa, A. (2024). Il progetto per l’Insula dei Casti Amanti tra tutela, valorizzazione e fruizione. Pompei: E-Journal degli Scavi di Pompei, 12. Ed. Parco Archeologico di Pompei. Available at: https://pompeiisites.org/wp-content/uploads/12_E-Journal-Insula-dei-Casti-Amanti.pdf.
Calvanese, V., and Zambrano, A. (2021). A conceptual design approach for archaeological structures, a challenging issue between innovation and conservation: a studied case in ancient Pompeii. Buildings 11 (4), 167. doi:10.3390/buildings11040167
Caputo, R., Hinzen, K. G., Liberatore, D., Schreiber, S., Helly, B., and Tziafalias, A. (2011). Quantitative archaeoseismological investigation of the great theatre of larissa, Greece. Bull. Earthq. Eng. 9, 347–366. doi:10.1007/s10518-010-9206-6
Carey, S., and Sigurdsson, H. (1987). Temporal variations in column height and magma discharge rate during the 79 AD eruption of Vesuvius. Geol. Soc. Am. Bull. 99 (2), 303–314. doi:10.1130/0016-7606(1987)99<303:TVICHA>2.0.CO;2
Ceccaroni, E., Ameri, G., Gomez Capera, A. A., and Galadini, F. (2009). The 2nd century AD earthquake in central Italy: archaeoseismological data and seismotectonic implications. Nat. Hazards 50, 335–359. doi:10.1007/s11069-009-9343-x
Chiominto, G., Scarpati, C., Perrotta, A., Fedele, L., and Santangelo, I. (2023). “Fallout events during the post-plinian phase of the AD 79 vesuvius eruption,” in Recent research on sedimentology, stratigraphy, paleontology, tectonics, geochemistry, volcanology and petroleum geology. MedGU 2021. Advances in science, technology and innovation. Editor A. Çiner (Cham: Springer). doi:10.1007/978-3-031-43222-4_32
Cioni, R., Civetta, L., Marianelli, P., Metrich, N., Santacroce, R., and Sbrana, A. (1995). Compositional layering and syn-eruptive mixing of a periodically refilled shallow magma chamber: the AD 79 Plinian eruption of Vesuvius. J. Pet. 36 (3), 739–776. doi:10.1093/petrology/36.3.739
Cioni, R., Gurioli, L., Sbrana, A., and Vougioukalakis, G. (2000a). Precursory phenomena and destructive events related to the Late Bronze Age Minoan (Thera, Greece) and AD 79 (Vesuvius, Italy) Plinian eruptions; inferences from the stratigraphy in the archaeological areas. Geol. Soc. Lon. 171 (1), 123–141. doi:10.1144/gsl.sp.2000.171.01.11
Cioni, R., Gurioli, L., Sbrana, A., and Vougioukalakis, G. (2000b). Precursors to the plinian eruptions of Thera (late bronze age) and vesuvius (AD 79): data from archaeological areas. Phys. Chem. Earth, Part A Solid Earth Geodesy 25 (9-11), 719–724. doi:10.1016/s1464-1895(00)00111-3
Cioni, R., Longo, A., Macedonio, G., Santacroce, R., Sbrana, A., Sulpizio, R., et al. (2003). Assessing pyroclastic fall hazard through field data and numerical simulations: example from Vesuvius. J. Geophys. Res. Solid Earth 108 (B2). doi:10.1029/2001jb000642
Cioni, R., Marianelli, P., and Sbrana, A. (1990). L'eruzione del 79 d.C.: stratigrafia dei depositi ed impatto sugli insediamenti romani nel settore orientale e meridionale del Somma-Vesuvio. Riv. studi Pompeiani 4, 179–198.
Cioni, R., Marianelli, P., and Sbrana, A. (1992). Dynamics of the AD 79 eruption: stratigraphic, sedimentologic and geochemical data on the successions of the Somma-Vesuvius southern sector. Acta Vulcanol. 2, 109–123.
Cioni, R., and Pistolesi, M. (2015). “Plinian and subplinian eruptions,” in The encyclopedia of volcanoes. Editor H. Sigurdsson (Academic Press), 519–535.
Cioni, R., Santacroce, R., and Sbrana, A. (1999). Pyroclastic deposits as a guide for reconstructing the multi-stage evolution of the Somma-Vesuvius Caldera. Bull. Volcanol. 61, 207–222. doi:10.1007/s004450050272
Cioni, R., Sbrana, A., and Gurioli, L. (1996). “The deposits of AD 79 eruption,” in Vesuvius decade volcano workshop handbook. Editor R. Santacroce (Rome, Italy: C.N.R), E1–E31.
Cioni, R., Tadini, A., Gurioli, L., Bertagnini, A., Mulas, M., Bevilacqua, A., et al. (2020). Estimating eruptive parameters and related uncertainties for pyroclastic density currents deposits: worked examples from Somma-Vesuvius (Italy). Bull. Volcanol. 82 (9), 65. doi:10.1007/s00445-020-01402-7
Cubellis, E., Luongo, G., and Marturano, A. (2007). Seismic hazard assessment at Mt. Vesuvius: maximum expected magnitude. J. Volcanol. Geotherm. Res. 162 (3-4), 139–148. doi:10.1016/j.jvolgeores.2007.03.003
Cubellis, E., and Marturano, A. (2002). Mt. Vesuvius: a macroseismic study of the earthquake of 9 October 1999. J. Volcanol. Geotherm. Res. 118 (3-4), 339–351. doi:10.1016/s0377-0273(02)00301-3
Cubellis, E., and Marturano, A. (2013). Felt index, source parameters and ground motion evaluation for earthquakes at Mt. Vesuvius. Ann. Geophys. 56 (4), S0439. doi:10.4401/ag-6445
De Carolis, E., and Patricelli, G. (2003). Vesuvius, AD 79: the destruction of Pompeii and Herculaneum. L'Erma di Bretschneider, Rome: Getty P. J. Publications, 1–124.
Dellino, P., Dioguardi, F., Isaia, R., Sulpizio, R., and Mele, D. (2021). The impact of pyroclastic density currents duration on humans: the case of the AD 79 eruption of Vesuvius. Sci. Rep. 11 (1), 4959. doi:10.1038/s41598-021-84456-7
de Sanctis, L., Iovino, M., Maiorano, R. M. S., and Aversa, S. (2020). Seismic stability of the excavation fronts in the ancient Roman city of Pompeii. Soils Found. 60 (4), 856–870. doi:10.1016/j.sandf.2020.05.009
de Sanctis, L., Maiorano, R. M. S., Brancaccio, U., and Aversa, S. (2019). Geotechnical aspects in the restoration of Insula dei Casti Amanti in Pompeii. Proc. Inst. Civ. Eng. Geotech. Eng. 172 (2), 121–130. doi:10.1680/jgeen.17.00221
De Simone, A. (1995). “I terremoti precedenti l’eruzione. Nuove attestazioni da recenti scavi,” in Archäologie und Seismologie. La Regione Vesuviana dal 62 al 79 d. C. Problemi archeologici e sismologici, 37–44. DAI. Römische Abteilung, Soprintendenza Archeologica di Pompei, Osservatorio vesuviano, Biering and Brinkmann, München, Germany, Colloquium di Boscoreale, 26–27 novembre 1993.
Dessales, H. (2022) “Ricostruire dopo un terremoto,” in Riparazioni antiche a pompei. Naples, Italy: Centre Jean Bérard, 1–331.
Di Giuseppe, H. (2021). L’iscrizione a carboncino che non data l’eruzione del Vesuvio. Oebalus Studi sulla Camp. nell’Antichità 16, 41–62.
Di Vito, M. A., Sparice, D., de Vita, S., Doronzo, D. M., Ricciardi, G. P., and Uzzo, T. (2023). The Museum of the Osservatorio Vesuviano: inviting the public to explore the geoheritage of the world’s first volcano observatory. Bull. Volcanol. 85 (8), 45. doi:10.1007/s00445-023-01658-9
Dogrul, B. N., Kiliccalan, I., Asci, E. S., and Peker, S. C. (2020). Blunt trauma related chest wall and pulmonary injuries: an overview. Chin. J. Traumatol. 23 (3), 125–138. doi:10.1016/j.cjtee.2020.04.003
Dong, Z. H., Yang, Z. G., Chen, T. W., Feng, Y. C., Chu, Z. G., Yu, J. Q., et al. (2010). Crush thoracic trauma in the massive Sichuan earthquake: evaluation with multidetector CT of 215 cases. Radiology 254 (1), 285–291. doi:10.1148/radiol.09090685
Doronzo, D. M., and Dellino, P. (2011). Interaction between pyroclastic density currents and buildings: numerical simulation and first experiments. Earth Planet. Sci. Lett. 310 (3-4), 286–292. doi:10.1016/j.epsl.2011.08.017
Doronzo, D. M., De Tullio, M. D., Dellino, P., and Pascazio, G. (2011). Numerical simulation of pyroclastic density currents using locally refined Cartesian grids. Comput. fluids 44 (1), 56–67. doi:10.1016/j.compfluid.2010.12.006
Doronzo, D. M., Di Vito, M. A., Arienzo, I., Bini, M., Calusi, B., Cerminara, M., et al. (2022). The 79 CE eruption of Vesuvius: a lesson from the past and the need of a multidisciplinary approach for developments in volcanology. Earth. Sci. Rev. 231, 104072. doi:10.1016/j.earscirev.2022.104072
Doronzo, D. M., Di Vito, M. A., de Vita, S., Ricciardi, G. P., and Sparice, D. (2023). Reply to the comment on “The 79 CE eruption of Vesuvius: A lesson from the past and the need of a multidisciplinary approach for developments in volcanology” by Doronzo et al., 2022 (Earth-Science Reviews 231, 104072). Earth Sci. Rev. 236, 104267. 2022 (Earth-Science Reviews 231, 104072. doi:10.1016/j.earscirev.2022.104267
Ferreira, T. M., Costa, A. A., and Costa, A. (2015). Analysis of the out-of-plane seismic behavior of unreinforced masonry: a literature review. Int. J. Archit. Herit. 9 (8), 949–972. doi:10.1080/15583058.2014.885996
Fonti, R. (2016). On the reading of the structural behavior of old masonry: the issue of the seismic assessment of archeological ruins. AIP Conf. Proc. 1790 (1), 130011. doi:10.1063/1.4968729
Foss, P. W. (2022). Pliny and the eruption of Vesuvius. Taylor and Frencis Group, London: Routledge, 1–352.
Galadini, F. (2009). Defining the causes of ancient building collapse (structural decaying vs. seismic shaking) in archaeological deposits of central Italy. It. J. Quat. Sci. 22 (1), 73–82.
Galadini, F., Ceccaroni, E., Dixit Dominus, G., Falcucci, E., Gori, S., Maceroni, D., et al. (2022). Combining Earth Sciences with Archaeology to investigate natural risks related to the cultural heritage of the Marsica region (central Apennines, Italy). Mediterr. Geosci. Rev. 4, 287–318. doi:10.1007/s42990-022-00078-9
Galadini, F., Ceccaroni, E., and Falcucci, E. (2010). Archaeoseismological evidence of a disruptive late antique earthquake at alba fucens (central Italy). Boll. Geofis. Teor. Appl. 51 (2-3), 143–161.
Galadini, F., and Galli, P. (1999). Palaeoseismology related to the displaced Roman archaeological remains at Egna (Adige Valley, northern Italy). Tectonophysics 308 (1-2), 171–191. doi:10.1016/s0040-1951(99)00080-3
Galadini, F., and Galli, P. (2004). The 346 AD earthquake (Central-Southern Italy): an archaeoseismological approach. Ann. Geophys. 47, 885–905. doi:10.4401/ag-3341
Galadini, F., Hinzen, K. G., and Stiros, S. (2006). Archaeoseismology: methodological issues and procedure. J. Seismol. 10, 395–414. doi:10.1007/s10950-006-9027-x
Galadini, F., Ricci, G., Falcucci, E., and Panzieri, C. (2018). Archaeoseismological evidence of past earthquakes in Rome (fifth to ninth century A.D.) used to quantify dating uncertainties and coseismic damage. Nat. Hazards 94 (1), 319–348. doi:10.1007/s11069-018-3390-0
Galli, P., and Bosi, V. (2002). Paleoseismology along the Cittanova fault: implications for seismotectonics and earthquake recurrence in Calabria (southern Italy). J. Geophys. Res. Solid Earth 107 (B3), ETG 1-1-ETG 1–19. doi:10.1029/2001JB000234
Galli, P., and Naso, J. A. (2009). Unmasking the 1349 earthquake source (southern Italy): paleoseismological and archaeoseismological indications from the Aquae Iuliae fault. J. Struct. Geol. 31, 128–149. doi:10.1016/j.jsg.2008.09.007
Galli, P., Ruga, A., Scionti, V., and Spadea, R. (2006). Archaeoseismic evidence for a late roman earthquake in the crotone area (ionian calabria, southern Italy): seismotectonic implications. J. Seismol. 10, 443–458. doi:10.1007/s10950-006-9030-2
Ghedini, F., Salvadori, M., Amoretti, V., Sparice, D., and Terracciano, A. (2024). E-Journal degli Scavi di Pompei. Pompei: 14. Ed. Parco Archeologico di Pompei. Available at: https://pompeiisites.org/wp-content/uploads/14-def_E-Journal-Casa-dei-Pittori-1.pdf.
Giacomelli, L., Perrotta, A., Scandone, R., and Scarpati, C. (2003). The eruption of Vesuvius of 79 AD and its impact on human environment in Pompeii. Episodes J. Int. Geosci. 26 (3), 235–238. doi:10.18814/epiiugs/2003/v26i3/014
Giacomelli, L., Scandone, R., and Rosi, M. (2021). The loss of geological memory of past catastrophes: the case of Pompeii. Ann. Geophys. 64 (5), VO547. doi:10.4401/ag-8631
Guglielmi, G., Sica, G., Palumbo, L., D’Errico, S., Pomara, C., Fineschi, V., et al. (2011). Lethal injuries following building collapse: comparison between autopsy and radiographic findings. La Radiol. Medica 116 (6), 969–981. doi:10.1007/s11547-011-0673-x
Guidoboni, E., Ferrari, G., Mariotti, D., Comastri, A., Tarabusi, G., Sgattoni, G., et al. (2018). CFTI5Med, Catalogo dei Forti Terremoti in Italia (461 a.C.-1997) e nell’area Mediterranea (760 a.C.-1500). Rome: Istituto Nazionale di Geofisica e Vulcanologia. doi:10.6092/ingv.it-cfti5
Guidoboni, E., Ferrari, G., Tarabusi, G., Sgattoni, G., Comastri, A., Mariotti, D., et al. (2019). CFTI5Med, the new release of the catalogue of strong earthquakes in Italy and in the Mediterranean area. Sci. Data 6 (1), 80. doi:10.1038/s41597-019-0091-9
Guidoboni, E., Muggia, A., and Valensise, G. (2000). “Aims and methods in territorial archaeology: possible clues to a strong fourth-century AD earthquake in the Straits of Messina (southern Italy),”in The archaeology of geological catastrophes. Editors W. J. McGuire, D. R. Griffiths, P. L. Hancock, and I. S. Stewart (Lyell Collection, London: Special Publication), 171, 45–70. doi:10.1144/gsl.sp.2000.171.01.06
Guidoboni, E., and Santoro Bianchi, S. (1995). Collapses and seismic collapses in archaeology: proposal for a thematic atlas. Ann. Geophys. 5-6, 1013–1017. doi:10.4401/ag-4102
Gurioli, L., Pareschi, M. T., Zanella, E., Lanza, R., Deluca, E., and Bisson, M. (2005). Interaction of pyroclastic density currents with human settlements: evidence from ancient Pompeii. Geology 33 (6), 441–444. doi:10.1130/g21294.1
Gurioli, L., Zanella, E., Pareschi, M. T., and Lanza, R. (2007). Influences of urban fabric on pyroclastic density currents at Pompeii (Italy): 1. Flow direction and deposition. J. Geophys. Res. Solid Earth 112 (B5). doi:10.1029/2006jb004444
Hancock, P. L., and Altunel, E. (1997). Faulted archaeological relics at hierapolis (pamukkale), Turkey. J. Geodyn. 24 (1–4), 21–36. doi:10.1016/S0264-3707(97)00003-3
Hine, H. M. (1984). The date of the Campanian earthquake AD 62 or AD 63, or both? L'Antiquité Classique, 266–269.
Hinojosa, H. R. (2023). The importance of assessing the geological site effects of ancient earthquakes from the archaeoseismological point of view. Eng 4 (1), 719–737. doi:10.3390/eng4010043
Hinzen, K. G. (2005). The use of engineering seismological models to interpret archaeoseismological findings in tolbiacum, Germany: a case study. Bull. Seismol. Soc. Am. 95 (2), 521–539. doi:10.1785/0120040068
Hinzen, K. G. (2009). Simulation of toppling columns in archaeoseismology. Bull. Seismol. Soc. Am. 99 (5), 2855–2875. doi:10.1785/0120080241
Hinzen, K. G., Meghraoui, M., Bahrouni, N., Houla, Y., and Reamer, S. K. (2021). Archaeoseismological study of the Cherichira aqueduct bridge, Kairouan, Tunisia. Mediterr. Geosci. Rev. 3, 403–430. doi:10.1007/s42990-021-00062-9
Hinzen, K. G., Schreiber, S., Fleischer, C., Reamer, S. K., and Wiosna, I. (2013). Archeoseismic study of damage in Roman and Medieval structures in the center of Cologne, Germany. J. Seismol. 17, 399–424. doi:10.1007/s10950-012-9327-2
Hinzen, K. G., Schreiber, S., and Yerli, B. (2010). The lycian sarcophagus of arttumpara, pinara, Turkey: testing seismogenic and anthropogenic damage scenarios. Bull. Seismol. Soc. Am. 100 (6), 3148–3164. doi:10.1785/0120100079
Imbò, G. (1974). Investigations regarding the crustal base of somma vesuvius and the magmatic characteristics of the volcano, together with the earthquakes between eruptions, particularly those of the 4th September 1968 and the 5th February AD. Atti dell’Accademia di Napoli, classe di Scienze. Fis. Mat. 8 (4), 53–154.
Jacobelli, L. (1995). “I terremoti fra il 62 e il 79 d.C. nell’area Vesuviana: le ragioni di un convegno,” in Archäologie und Seismologie. La Regione Vesuviana dal 62 al 79 d. C. Problemi archeologici e sismologici. Soprintendenza Archeologica di Pompei, Osservatorio vesuviano, Biering and Brinkmann, München, Germany, Colloquium di Boscoreale. Editor D. A. I. Römische Abteilung, 17–21. 26–27 novembre 1993.
Jusseret, S., Langohr, C., and Sintubin, M. (2013). Tracking earthquake archaeological evidence in late minoan IIIB (∼ 1300–1200 BC) crete (Greece): a proof of concept. Bull. Seismol. Soc. Am. 103 (6), 3026–3043. doi:10.1785/0120130070
Karcz, I., and Kafri, U. (1978). Evaluation of supposed archaeoseismic damage in Israel. J. Archaeol. Sci. 5, 237–253. doi:10.1016/0305-4403(78)90042-0
Kázmér, M., and Major, B. (2015). Sāfitā castle and rockfalls in the ‘dead villages’ of coastal Syria – an archaeoseismological study. Comptes Rendus Geosci. 347 (4), 181–190. doi:10.1016/j.crte.2015.06.011
Korjenkov, A. M., and Mazor, E. (1999a). Seismogenic origin of the ancient avdat ruins, negev desert, Israel. Nat. Hazards 18, 193–226. doi:10.1023/A:1026488932377
Korjenkov, A. M., and Mazor, E. (1999b). Earthquake characteristics reconstructed from archeological damage patterns: shivta, the Negev Desert, Israel. Isr. J. Earth Sci. 48, 265–282.
Korjenkov, A. M., and Mazor, E. (2003). Archeoseismology in mamshit (southern Israel): cracking a millennia-old code of earthquakes preserved in ancient ruins. Archäologischer Anz. 2, 51–82.
Korjenkov, A. M., and Mazor, E. (2014). Archaeoseismological damage pattern at the ancient ruins of Rehovot-ba-Negev, Israel. Archäologischer Anz. 1, 75–92. doi:10.34780/0at4-6147
La Greca, F. (2007). I terremoti in Campania in età romana e medioevale. Sismologia e sismografia storica. Ann. Stor. Principato Citra 1, 5–34.
Liebsch, C., Seiffert, T., Vlcek, M., Beer, M., Huber-Lang, M., and Wilke, H. (2019). Patterns of serial rib fractures after blunt chest trauma: an analysis of 380 cases. PLoS One 14 (12), e0224105. doi:10.1371/journal.pone.0224105
Ling, R. (1995). “Earthquake damage in Pompeii I 10: one earthquake or two?,” in Archäologie und Seismologie. La Regione Vesuviana dal 62 al 79 d. C. Problemi archeologici e sismologici. Soprintendenza Archeologica di Pompei, Osservatorio vesuviano, Biering and Brinkmann. Editor D. A. I. Römische Abteilung (München, Germany: Colloquium di Boscoreale), 201–209.
Lirer, L., Munno, R., Petrosino, P., and Vinci, A. (1993). Tephrostratigraphy of the AD 79 pyroclastic deposits in perivolcanic areas of Mt. Vesuvio (Italy). J. Volcanol. Geoth. Res. 58 (1-4), 133–149. doi:10.1016/0377-0273(93)90105-Z
Lirer, L., Munno, R., Postiglione, I., Vinci, A., and Vitelli, L. (1997). The AD 79 eruption as a future explosive scenario in the Vesuvian area: evaluation of associated risk. Bull. Volcanol. 59, 112–124. doi:10.1007/s004450050179
Lirer, L., Pescatore, T., Booth, B., and Walker, G. P. (1973). Two plinian pumice-fall deposits from Somma-Vesuvius, Italy. Geol. Soc. Am. Bull. 84 (3), 759–772. doi:10.1130/0016-7606(1973)84<759:tppdfs>2.0.co;2
Lovejoy, C. O. (1985). Dental wear in the Libben population: its functional pattern and role in the determination of adult skeletal age at death. Am. J. Phys. Anthropol. 68, 47–56. doi:10.1002/ajpa.1330680105
Lovejoy, C. O., Meindl, R. S., Pryzbeck, T. R., and Mensforth, R. P. (1985). Chronological metamorphosis of the auricular surface of the ilium: a new method for the determination of adult skeletal age at death. Am. J. Phys. Anthropol. 68, 15–28. Chronological metamorphosis of the auricular surface of the ilium: a new method for the determination of adult skeletal age at death. doi:10.1002/ajpa.1330680103
Luongo, G., Perrotta, A., and Scarpati, C. (2003a). Impact of the AD 79 explosive eruption on Pompeii, I. Relations amongst the depositional mechanisms of the pyroclastic products, the framework of the buildings and the associated destructive events. J. Volcanol. Geoth. Res. 126 (3-4), 201–223. doi:10.1016/s0377-0273(03)00146-x
Luongo, G., Perrotta, A., Scarpati, C., De Carolis, E., Patricelli, G., and Ciarallo, A. (2003b). Impact of the AD 79 explosive eruption on Pompeii, II. Causes of death of the inhabitants inferred by stratigraphic analysis and areal distribution of the human casualties. J. Volcanol. Geoth. Res. 126 (3-4), 169–200. doi:10.1016/s0377-0273(03)00147-1
Macedonio, G., Pareschi, M. T., and Santacroce, R. (1988). A numerical simulation of the Plinian fall phase of 79 AD eruption of Vesuvius. J. Geophys. Res. Solid Earth 93 (B12), 14817–14827. doi:10.1029/JB093iB12p14817
Marco, S. (2008). Recognition of earthquake-related damage in archaeological sites: examples from the Dead Sea fault zone. Tectonophysics 453 (1-4), 148–156. doi:10.1016/j.tecto.2007.04.011
Marco, S., Hartal, M., Hazan, N., Lev, L., and Stein, M. (2003). Archaeology, history, and geology of the A.D. 749 earthquake, Dead Sea transform. Geology 31, 665–668. doi:10.1130/G19516.1
Martín-González, F. (2018). Earthquake damage orientation to infer seismic parameters in archaeological sites and historical earthquakes. Tectonophysics 724, 137–145. doi:10.1016/j.tecto.2018.01.013
Martini, K. (1998). Ancient structures and modern analysis: investigating damage and reconstruction at Pompeii. Automation Constr. 8 (1), 125–137. doi:10.1016/s0926-5805(98)00070-3
Marturano, A. (2006). “Chapter 4 Geophysical precursors at vesuvius from historical and archeological sources,” in Vesuvius. Developments in volcanology. Editor F. Dobran (Elsevier), 8, 249–263. doi:10.1016/s1871-644x(06)80008-2
Marturano, A. (2008). Sources of ground movement at Vesuvius before the AD 79 eruption: evidence from contemporary accounts and archaeological studies. J. Volcanol. Geoth. Res. 177 (4), 959–970. doi:10.1016/j.jvolgeores.2008.07.017
Marturano, A., and Rinaldis, V. (1995). “Il terremoto vesuviano del 62 d.c.: un evento carico di responsabilità,” in Archäologie und Seismologie. La Regione Vesuviana dal 62 al 79 d. C. Problemi archeologici e sismologici. Editor D. A. I. Römische Abteilung (Germany: Colloquium di Boscoreale), 131–135. 26–27 novembre 1993.
Marturano, A., and Varone, A. (2005). “The AD 79 eruption: seismic activity and effects of the eruption on Pompeii,” in Cultural responses to the volcanic landscape: the Mediterranean and beyond (Archaeological Institute of America, AIA colloquia and conference papers), 241–260.
Mastrolorenzo, G. (2021). L'eruzione pliniana del Vesuvio del 79 dC: un'ipotesi interpretativa in merito alla morte dei pompeiani. In: I calchi di Pompei da Giuseppe Fiorelli a oggi, e.d. Osanna, M., Capurso, A., and Masseroli, S. M., L'Erma di Bretschneider, Rome.: Studi e Ricerche del Parco Archeologico di Pompei 46: 3–18.
Mastrolorenzo, G., Petrone, P., Pappalardo, L., and Guarino, F. M. (2010). Lethal thermal impact at periphery of pyroclastic surges: evidences at Pompeii. PloS one 5 (6), e11127. doi:10.1371/journal.pone.0011127
McNutt, S. R., and Roman, D. C. (2015). “Volcanic seismicity,” in The encyclopedia of volcanoes. Editor H. Sigurdsson (Academic Press), 1011–1034.
Meletti, C., Galadini, F., Valensise, G., Stucchi, M., Basili, R., Barba, S., et al. (2008). A seismic source zone model for the seismic hazard assessment of the Italian territory. Tectonophysics 450, 85–108. doi:10.1016/j.tecto.2008.01.003
Melluso, L., Scarpati, C., Zanetti, A., Sparice, D., and de' Gennaro, R. (2022). The petrogenesis of chemically zoned, phonolitic, Plinian and sub-Plinian eruptions of Somma-Vesuvius, Italy: role of accessory phase removal, independently filled magma reservoirs with time, and transition from slightly to highly silica undersaturated magmatic series in an ultrapotassic stratovolcano. Lithos 430, 106854. doi:10.1016/j.lithos.2022.106854
Naghi, T. M., Kambiz, K., Shahriar, J. M., Afshin, T., Reza, S. K., Behnam, P., et al. (2005). Musculoskeletal injuries associated with earthquake: a report of injuries of Iran's December 26, 2003 Bam earthquake casualties managed in tertiary referral centers. Injury 36 (1), 27–32. doi:10.1016/j.injury.2004.06.021
Nappo, S. C. (1995). “Evidenze di danni strutturali, restauri e rifacimenti nelle insulae gravitanti su Via Nocera a Pompei,” in Archäologie und Seismologie. La Regione Vesuviana dal 62 al 79 d. C. Problemi archeologici e sismologici. Editor D. A. I. Römische Abteilung, 45–55. Soprintendenza Archeologica di Pompei, Osservatorio vesuviano, Biering and Brinkmann, München, Germany, Colloquium di Boscoreale, 26–27 novembre 1993.
Nunziante, L., Fraldi, M., Lirer, L., Petrosino, P., Scotellaro, S., and Cicirelli, C. (2003). Risk assessment of the impact of pyroclastic currents on the towns located around Vesuvio: a non-linear structural inverse analysis. Bull. Volcanol. 65, 547–561. doi:10.1007/s00445-003-0282-1
Oliva, N. M. (1632a) “Lettera del Sig. Nicolo' Maria Oliva nella quale da' relatione delli segni,” in terremoti et incendii del Monte Vesuvio, cominciando dalli 16 del mese di dicembre 1631 alli 5 di gennaio 1632. Napoli: Scoriggio Lazzaro, 7.
Oliva, N. M. (1632b). La ristampata lettera con aggiunta di molte cose notabili del Signor Nicolo' Maria Oliva scritta all'illustriss. Signor, Abbate D. Flavio Ruffo. Nella quale da' vera, et minuta relatione delli segni, terremoti, el incendij del Monte Vesuvio, cominciando dalli 10 del mese di dicembre 1631. Napoli: Scoriggio Lazzaro, 8.
Pappalardo, U. (1995). “Osservazioni su un secondo grande terremoto a Pompei,” in Archäologie und Seismologie. La Regione Vesuviana dal 62 al 79 d. C. Problemi archeologici e sismologici. Editor D. A. I. Römische Abteilung, 191–194. Soprintendenza Archeologica di Pompei, Osservatorio vesuviano, Biering and Brinkmann, München, Germany, Colloquium di Boscoreale, 26–27 novembre 1993.
Patti, O. (2003). Nuovi dati per la ricerca geo-archeologica dall'Insula dei Casti Amanti (IX, 12). Riv. Studi Pompeiani 14, 320–328.
Pesando, F., and Guidobaldi, M. P. (2018). in Le tecniche edilizie. Editors M. P. Guidobaldi, F. Pesando, O. Pompei, S. Ercolano, and B. Guide archeologiche Laterza (Laterza and Figli Spa).
Pirazzoli, P. A., Ausseil-Badie, J., Giresse, P., Hadjidaki, E., and Arnold, M. (1992). Historical environmental changes at phalasarna harbor, west crete. Geoarchaeology 7 (4), 371–392. doi:10.1002/gea.3340070406
Pirazzoli, P. A., Laborel, J., and Stiros, S. (1996). Earthquake clustering in the Eastern Mediterranean during historical times. J. Geophys. Res. 101, 6083–6097. doi:10.1029/95JB00914
Pomonis, A., Spence, R., and Baxter, P. (1999). Risk assessment of residential buildings for an eruption of furnas volcano, sao miguel, the azores. J. Volcanol. Geotherm. Res. 92 (1-2), 107–131. doi:10.1016/s0377-0273(99)00071-2
Renna, E. (1995). “La realtà sismologica dell’area vesuviana prima e dopo il 79 d.C. attraverso l’analisi delle fonti antiche,” in Archäologie und Seismologie. La Regione Vesuviana dal 62 al 79 d. C. Problemi archeologici e sismologici (München, Germany: Colloquium di Boscoreale), 26–27.
Rodríguez-Pascua, M. A., Pérez-López, R., Giner-Robles, J. L., Silva, P. G., Garduño-Monroy, V. H., and Reicherter, K. (2011). A comprehensive classification of Earthquake Archaeological Effects (EAE) in archaeoseismology: application to ancient remains of Roman and Mesoamerican cultures. Quat. Int. 242 (1), 20–30. doi:10.1016/j.quaint.2011.04.044
Rodríguez-Pascua, M. Á., Perucha, M. Á., Silva, P. G., Montejo Córdoba, A. J., Giner-Robles, J. L., Élez, J., et al. (2023). Archaeoseismological evidence of seismic damage at medina azahara (córdoba, Spain) from the early 11th century. Appl. Sci. 13 (3), 1601. doi:10.3390/app13031601
Ruggieri, N. (2017). Seismic vulnerability of the ancient Pompeii through the evaluation of the 62 AD earthquake effects. Int. J. Archit. Herit. 11 (4), 490–500. doi:10.1080/15583058.2016.1263690
Ruggieri, N., Galassi, S., and Tempesta, G. (2018). I terremoti del I secolo d.C. a Pompei. Osservazioni intorno ai danni e consolidamenti nelle Terme Stabiane con particolare riguardo ad uno sperone nel Destrictarium. Restauro Archeol. 26 (2), 72–91. doi:10.13128/RA-24194
Ruggieri, N., Galassi, S., and Tempesta, G. (2020). The effect of pyroclastic flows of the 79 AD eruption of Mount Vesuvius on the Pompeii's city walls. The case study of the sector near the Tower XI. J. Cult. Herit. 43, 235–241. doi:10.1016/j.culher.2019.10.008
Sandoval, C., Roca, P., Bernat, E., and Gil, L. (2011). Testing and numerical modelling of buckling failure of masonry walls. Constr. Build. Mat. 25 (12), 4394–4402. doi:10.1016/j.conbuildmat.2011.01.007
Satta, M. L., Ruggieri, N., Tempesta, G., and Galassi, S. (2021). Remains of the ancient colonnade in the archaeological site of Pompeii, Italy: vulnerability analysis and strengthening proposal. J. Cult. Herit. 52, 93–106. doi:10.1016/j.culher.2021.09.006
Savino, E. (2009). “Nerone, Pompei e il terremoto del 63 d.C,” in Interventi imperiali in campo economico e sociale da Augusto al tardoantico. Editors M. Storchi, and D. Merola, 225–244.
Scandone, R., and Giacomelli, L. (2008). Precursors of eruptions at vesuvius (Italy). J. Volcanol. Geoth. Res. 171 (3-4), 191–200. doi:10.1016/j.jvolgeores.2007.11.018
Scandone, R., Giacomelli, L., and Rosi, M. (2019). Death, survival and damage during the 79 AD eruption of vesuvius which destroyed Pompeii and Herculaneum. J. Res. Didact. Geogr. 2, 5–30.
Scarpati, C., Perrotta, A., Martellone, A., and Osanna, M. (2020). Pompeian hiatuses: new stratigraphic data highlight pauses in the course of the AD 79 eruption at Pompeii. Geol. Mag. 157 (4), 695–700. doi:10.1017/s0016756819001560
Schweppe, G., Hinzen, K. G., Reamer, S. K., and Marco, S. (2021). Reconstructing the slip velocities of the 1202 and 1759 CE earthquakes based on faulted archaeological structures at Tell Ateret, Dead Sea Fault. J. Seismol. 25, 1021–1042. doi:10.1007/s10950-021-10009-0
Sigurdsson, H., Carey, S., Cornell, W., and Pescatore, T. (1985). The eruption of Vesuvius in A.D. 79. Nat. Geogr. Res. 3, 332–397.
Sigurdsson, H., Cashdollar, S., and Sparks, S. R. (1982). The eruption of Vesuvius in AD 79: reconstruction from historical and volcanological evidence. Am. J. Archaeol. 86 (1), 39–51. doi:10.2307/504292
Sintubin, M. (2013). “Archaeoseismology,” in Encyclopedia of earthquake engineering. Editors M. Beer, I. Kougioumtzoglou, E. Patelli, and I. K. Au (Berlin, Heidelberg: Springer). doi:10.1007/978-3-642-36197-5_29-2
Spence, R. J., Baxter, P. J., and Zuccaro, G. (2004a). Building vulnerability and human casualty estimation for a pyroclastic flow: a model and its application to Vesuvius. J. Volcanol. Geotherm. Res. 133 (1-4), 321–343. doi:10.1016/S0377-0273(03)00405-0
Spence, R. J., Zuccaro, G., Petrazzuoli, S., and Baxter, P. J. (2004b). Resistance of buildings to pyroclastic flows: analytical and experimental studies and their application to Vesuvius. Nat. Haz. Rev. 5 (1), 48–59. doi:10.1061/(ASCE)1527-6988(2004)5:1(48)
Spence, R. J. S., Kelman, I., Baxter, P. J., Zuccaro, G., and Petrazzuoli, S. (2005). Residential building and occupant vulnerability to tephra fall. Nat. Haz. Earth Syst. Sci. 5 (4), 477–494. doi:10.5194/nhess-5-477-2005
Stiros, S. C. (1996). Identification of earthquakes from archaeological data: methodology, criteria and limitations. Archaeoseismology 7, 129–152.
Stiros, S. C., and Papageorgiou, S. (2001). Seismicity of western crete and the destruction of the town of kisamos at AD 365: archaeological evidence. J. Seismol. 5, 381–397. doi:10.1023/A:1011475610236
Stiros, S. C., and Pytharouli, S. I. (2014). Archaeological evidence for a destructive earthquake in Patras, Greece. J. Seismol. 18, 687–693. doi:10.1007/s10950-014-9437-0
Stucchi, M., Meletti, C., Montaldo, V., Akinci, A., Faccioli, E., Gasperini, P., et al. (2004). Pericolosità sismica di riferimento per il territorio nazionale MPS04 [Data set]. Rome: Istituto Nazionale di Geofisica e Vulcanologia. doi:10.13127/sh/mps04/ag
Tian-wu, C., Zhi-gang, Y., Zhi-hui, D., Si-shi, T., Zhi-gang, C., and Heng, S. (2011). Earthquake-related pelvic crush fracture vs. non-earthquake fracture on digital radiography and MDCT: a comparative study. Clinics 66 (4), 629–634. doi:10.1590/S1807-59322011000400018
Toniolo, L., Amoretti, V., Gravina, E., Martinelli, R., Scala, S., and Sparice, D. (2021). Da Mumia alle ultime vittime di Pompei: nuove ricerche nella villa di Civita Giuliana. Riv. Studi Pompeiani 31, 123–130. doi:10.48255/2240-9653.RSP.32.2021.09
Trionfo, A., and Arkader, A. (2019). Both bone Forearm fractures. Oper. Tech. Orthop. 29 (1), 49–54. doi:10.1053/j.oto.2018.12.009
Valentine, G. A. (1998). Damage to structures by pyroclastic flows and surges, inferred from nuclear weapons effects. J. Volcanol. Geotherm. Res. 87 (1-4), 117–140. doi:10.1016/s0377-0273(98)00094-8
Vanin, F., Penna, A., and Beyer, K. (2020). A three-dimensional macroelement for modelling the in-plane and out-of-plane response of masonry walls. Earthq. Eng. Struct. Dyn. 49 (14), 1365–1387. doi:10.1002/eqe.3277
Varone, A. (1995). “Più terremoti a Pompei? I nuovi dati degli scavi di Via dell’Abbondanza,” in Archäologie und Seismologie. La Regione Vesuviana dal 62 al 79 d. C. Problemi archeologici e sismologici. Editor D. A. I. Römische Abteilung, 29–35. Soprintendenza Archeologica di Pompei, Osservatorio vesuviano, Biering and Brinkmann, München, Germany, Colloquium di Boscoreale, 26–27 novembre 1993.
Varone, A. (2000). Pompei, i misteri di una città sepolta: storia e segreti di un luogo in cui la vita si è fermata duemila anni fa. Rome: Newton and Compton editori, 1–364.
Varone, A. (2005a). Convivere con i terremoti. La travagliata ricostruzione di Pompei dopo il terremoto del 62 dC alla luce delle nuove scoperte. Omni pede stare. Saggi architettonici e circumvesuviani in memoriam Jos de Waele. Studi della Soprintend. archeol. Pompei 9, 315–324.
Varone, A. (2005b). “Il progetto di scavo e pubblica fruizione dell’insula pompeiana dei Casti Amanti (insula IX 12),” in Nuove ricerche archeologiche a Pompei ed Ercolano. Editors P. Guzzo, and M. P. Guidobaldi, 191–199.
Varone, A. (2005c). “L’Insula pompeiana dei Casti Amanti (IX 12): dallo scavo alla valorizzazione,” in Domus romane: dallo scavo alla valorizzazione. Editors F. Morandini, and F. Rossi, 297–306.
Varone, A., and Marturano, A. (1997). L'eruzione vesuviana del 24 agosto del 79 dC attraverso le lettere di Plinio ii Giovane e le nuove evidenze archeologiche. Riv. Studi Pompeiani 8, 57–72. doi:10.1400/258534
Williams, S. N., and Self, S. (1983). The october 1902 plinian eruption of Santa María volcano, Guatemala. J. Volcanol. Geotherm. Res. 16 (1-2), 33–56. doi:10.1016/0377-0273(83)90083-5
Woods, A. W. (1995). The dynamics of explosive volcanic eruptions. Rev. Geophys. 33, 495–530. doi:10.1029/95rg02096
Woods, A. W. (2001). Explosive volcanic eruptions. “Geomorphological fluid mechanics”. Lect. Notes Phys. 582, 188–208. doi:10.1029/95RG02096
Yokoyama, I. (2001). The largest magnitudes of earthquakes associated with some historical volcanic eruptions and their volcanological significance. Ann. Geofis. 44 (5-6), 1021–1029.
Yokoyama, T., and Marturano, A. (1997). Volcanic products of the vesuvius eruption in AD 79 at Pompeii, Italy. Opusc. Pompeiana VII, 1–32.
Zanella, E., Gurioli, L., Pareschi, M. T., and Lanza, R. (2007). Influences of urban fabric on pyroclastic density currents at Pompeii (Italy): 2. Temperature of the deposits and hazard implications. J. Geophys. Res. Solid Earth 112 (B5). doi:10.1029/2006jb004775
Zobin, V. M. (2001). Seismic hazard of volcanic activity. J. Volcanol. Geotherm. Res. 112 (1-4), 1–14. doi:10.1016/S0377-0273(01)00230-X
Zuccaro, G., Cacace, F., Spence, R. J. S., and Baxter, P. J. (2008). Impact of explosive eruption scenarios at Vesuvius. J. Volcanol. Geotherm. Res. 178 (3), 416–453. doi:10.1016/j.jvolgeores.2008.01.005
Zuccaro, G., and De Gregorio, D. (2019). Impact assessments in volcanic areas-The Vesuvius and Campi Flegrei cases studies. Ann. Geophys. 62 (1), VO02. doi:10.4401/ag-7827
Keywords: 79 CE Plinian eruption, Pompeii archaeological site, syn-eruptive earthquakes, volcano seismicity, damage assessment, masonry building collapse, compression traumas, archaeoseismology
Citation: Sparice D, Amoretti V, Galadini F, Di Vito MA, Terracciano A, Scarpati G and Zuchtriegel G (2024) A novel view of the destruction of Pompeii during the 79 CE eruption of Vesuvius (Italy): syn-eruptive earthquakes as an additional cause of building collapse and deaths. Front. Earth Sci. 12:1386960. doi: 10.3389/feart.2024.1386960
Received: 16 February 2024; Accepted: 10 June 2024;
Published: 18 July 2024.
Edited by:
Roberto Sulpizio, University of Bari Aldo Moro, ItalyReviewed by:
Salvatore Passaro, National Research Council (CNR), ItalyFabio Dioguardi, University of Bari Aldo Moro, Italy
Copyright © 2024 Sparice, Amoretti, Galadini, Di Vito, Terracciano, Scarpati and Zuchtriegel. This is an open-access article distributed under the terms of the Creative Commons Attribution License (CC BY). The use, distribution or reproduction in other forums is permitted, provided the original author(s) and the copyright owner(s) are credited and that the original publication in this journal is cited, in accordance with accepted academic practice. No use, distribution or reproduction is permitted which does not comply with these terms.
*Correspondence: Domenico Sparice, ZG9tZW5pY28uc3BhcmljZUBpbmd2Lml0