- 1School of Energy Resources, China University of Geosciences (Beijing), Beijing, China
- 2Key Laboratory for Marine Reservoir Evolution and Hydrocarbon Abundance Mechanism, Beijing, China
- 3Institute of Earth Sciences, China University of Geosciences, Beijing, China
Introduction: China is a large country of REE resources and production, supplying more than 90% of the world’s rare earth consumption market, China’s traditional REE resources, although rich, but reserves are also declining, in recent years, scholars have shown that the REEs in the specific geological conditions in the coals can be enriched, and even reach the industrial grade, the search for more REE mineral resources will be of great strategic significance.
Methods: The article has selected the Zibo, Taozao, Huainan, Datong, Huozhou, Jungar, and Weibei coalfields in North China as the research objects. Based on inductively coupled plasma mass spectrometry (ICP-MS) analyses, the study provides a detailed analysis of the enrichment rules and distribution patterns of rare earth elements (REEs) in coal under different geological conditions.
Results: The REE distribution patterns in Late Palaeozoic coals in North China are characterized by enrichment of LREEs, deficit of HREEs with gentle changes, and obvious negative anomalies of Eu, the enrichment of REE in coal is controlled by provenance and volcanism, and is related to distance of transport terrigenous material and the sedimentary environment. The REEs concentrations in North China indicates that Late Palaeozoic coals in the mining areas of the north, northwest, and western parts of North China are significantly enriched in REEs. In addition, REEs in Late Palaeozoic coals in the southeastern mining areas are relatively enriched, and the central and eastern regions are significantly depleted.
Discussion: Large coal-type rare earth deposits are expected to be developed in North China, particularly the Datong coalfield, Jungar coal mine and the Weibei coal mine in the eastern margin of the Ordos Basin, and the Zibo coalfield.
1 Introduction
Given the rapid development of high-tech industries and the rapidly increasing demand for rare earths and other critical metals (Commission, 2017) (e.g., lithium, gallium, etc.), the search for new critical metals resources has become very urgent. Certain critical metals, including lithium, aluminium, gallium, germanium, uranium, and rare earth elements (REEs), can be enriched in coal under specific geological conditions. These elements may then form mineral resources with mining value (Seredin et al., 2013; Schulz, 2017; Dai et al., 2020). According to the 2023 reports from China’s Ministry of Land and Resources and BP World Energy Statistics, China’s coal resources amount to 207.012 billion tons (Ministry of Natural Resources, 2023). China’s coal production accounts for 50.5% of the world’s production and 53.8% of the energy consumption structure (BP, 2023). The critical metallic inorganic elements in coal, if studied and exploited, will be of great strategic significance in ensuring China’s national security and resource security.
The world’s REE resources are mainly derived from large and super-large REE deposits (Weng et al., 2015; Survey, 2023), as shown in Figure 1. For example, the Bayunebo deposit in China, the Mountain Pass and Bear Lodge deposits in the United States, and the Strange Lake deposit in Canada are the major sources of rare earth resources worldwide. Based on data from the U.S. Geological Survey in 2022, China’s proven reserves of rare earth minerals are estimated to be 44 million tons (Survey, 2023), representing 35% of the world’s total. In the same year, China produced over 210,000 tons of rare earth minerals, accounting for 69.8% of global production. As a large rare earth country, China is rich in rare earth mineral resources, but the demand for rare earths is increasing and the reserves are decreasing (Wang et al., 2020; Guo and You, 2023). It is of great strategic importance to find more rare earth mineral resources.
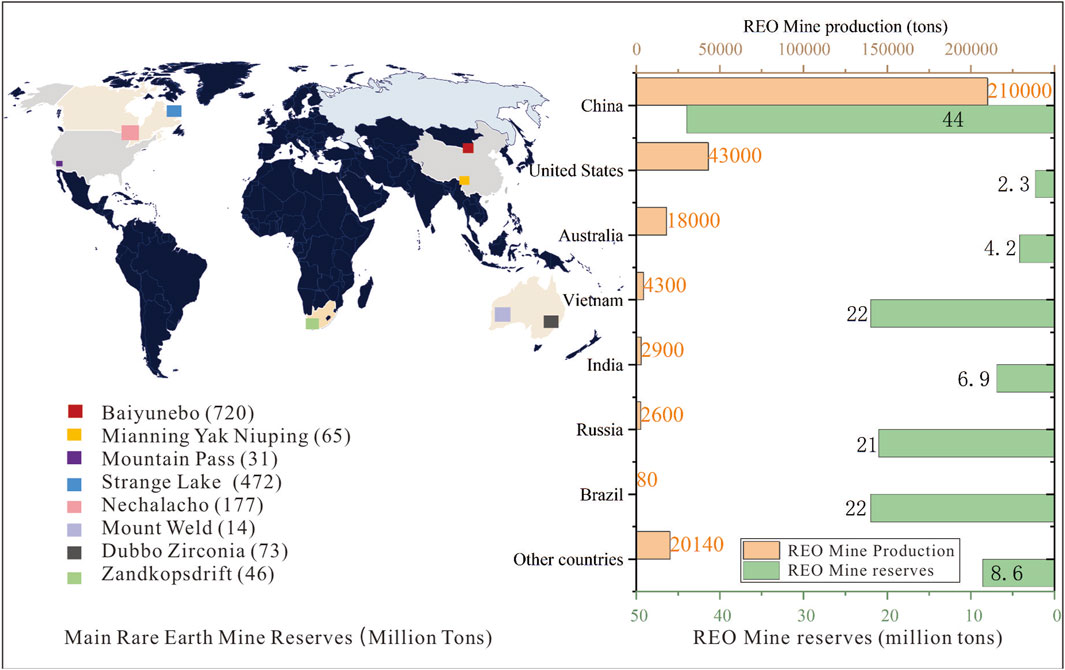
Figure 1. Distribution of major rare earth deposits in the world (left), global annual REO mine production and global REO mine reserves (right); data from U.S. Geological Survey (2023).
In recent years, several countries including China, the United States, Russia, and the United Kingdom have conducted extensive research on critical metallic inorganic elements in coal, attempted to extract critical elements from coal ash, and achieved many important results (Blissett, Smalley, and Rowson, 2014; Hower and Dai, 2016; Jane and Arnold, 2018; Arbuzov, Chekryzhov, et al., 2019; Dai et al., 2020). Currently, germanium deposits in coal have been mined and utilized in Lincang, Yunnan Province and Wulantuga coalfield, Inner Mongolia, resulting in significant benefits and demonstration of their potential (Dai, Ren, et al., 2012). Additionally, many coalfields in northern China, including some coal seams in Jungar coalfield, exhibit critical metal enrichment, such as rare earth elements, aluminium, gallium, and lithium, which are concomitantly enriched (Kang, Kang, and Shan, 2014; Jiu et al., 2023). Furthermore, Ziemkiewicz (2020) and his team have elaborated a unique technique for REE recovery from what was initially thought to be REE-low/moderately-enriched post-coal-mining waste. The industrial recycling of rare earth elements found in fly ash can provide significant economic and environmental benefits (Chowdhury, Deng, and Jin, 2021; Rybak and Rybak, 2021). Despite the challenges associated with utilizing key factors such as economic mining technology and addressing environmental concerns during the recycling process, the potential for industrial utilization of coal beds is extensive (Franus, Wiatros-Motyka, and Wdowin, 2015; Dai and Finkelman, 2018; Pan et al., 2018; Taggart, Hower, and Heileen, 2018). In recent years, scholars from Russia, the United States, and China, including Seredin (2012), Hower and Dai (2016), Finkelman, Palmer, and Wang (2018), and Dai, Zou, et al. (2012), have conducted in depth studies on the concentration, state of occurrence, and sources of critical metallic inorganic elements in coal and fly ash. Finkelman (1994) and Arbuzov, Finkelman, et al. (2019) found that rare earth elements in coal primarily occur in phosphate minerals and are derived from terrigenous clasts, solutions, or colloids. The concentration of rare earth elements in coal is primarily dependent on the concentration of inorganic components. REEs in the coals of the Pavlovskoe coal deposit in Russia are mineral-forming, i.e., they exist as dominant cations in minerals, e.g., lanthanite-(Nd), kimuraite-(Y), Seredin (1996) discovered the coexistence of REEs and platinum group elements in the coal of Cenozoic coal-bearing basins in the Far East of Russia, He and his team also reported unknown REE minerals, such as those composed of individual REE (Gd, Dy) or their compounds with some other metals (Sm-Fe-Co, etc.). This suggests that the enrichment of rare earth elements in coal may have some internal relationship with other trace elements. Dai et al. (2006) concluded that rare earth elements in coal or coal measures can be enriched through sediment source, volcanic ash sedimentation, hydrothermal fluid intrusion, groundwater dripping, and sedimentary environment influenced by seawater. Based on regional geological conditions, Dai et al. (Dai et al., 2006; Dai, Graham, and Ward, 2016) proposed that the northern Ordos Basin in North China has the potential for large coal rare earth deposits. According to his research, the enrichment of rare earth elements in coal in North China is influenced by provenance and volcanism. The research conducted by the authors on the No. 2 and No. 14 coals of the Taozao Shanxi Formation in North China also supports this point. The provenance properties during the sedimentary period control the input of rare earth elements, while the enrichment degree of rare earth elements in local coal seams is affected by volcanic ash deposition.
The average concentration of flat rare earth elements in world coal is only 68 μg/g (Ketris M. P. and Yudovich YaE. 2009), which is lower than the average concentration in the upper continental crust (168.4 μg/g) (Taylor and McLennan, 1995). However, the concentration of rare earth oxides in coal ash can reach 485 μg/g (Seredin and Finkelman, 2008; Ketris MáP. and Yudovich YaE. 2009; Dai, Graham, and Ward, 2016). It is important to note that rare earth elements are found in Chinese coal at an average concentration of 137.9 μg/g (Dai, Graham, and Ward, 2016). Seredin and Dai (2012) proposed an industrial utilization standard for rare earth elements in coal and coal ash, stating that the concentration of rare earth element oxides in coal ash should be greater than 1,000 μg/g. Additionally, a “prospect coefficient” was introduced to evaluate the development and utilization potential of rare earth (Dai et al., 2017). In recent years, it has been discovered that the coal in the Jungar and the Daqingshan coalfields of the Ordos Basin in North China is relatively enriched in rare earth elements. The average concentration of rare earth elements in the coal of the Jungar Heidaigou coal deposit can reach 255 μg/g (Dai, Zou, et al., 2012), the concentration of rare earth elements in the coal of the Jungar Haerwusu coal deposit is 172 μg/g (Dai et al., 2008), and the concentration of rare earth elements in the coal of the Daqingshan Hailiushu coal deposit is 194 μg/g (Dai, Li, et al., 2015). However, the previous studies only cover the shallow parts of individual coal mines. Research on the geochemical characteristics of REEs in coal seams and their geological influences is relatively weak or inconsistent. There is a lack of systematic research on the concentration and distribution patterns of REEs in coal in North China, which hinders an overall and regular understanding of their enrichment characteristics and geological significance. Limited research has been conducted on the REEs in coal as the main content to reflect the material sources and past geological conditions; regarding the distribution pattern of REEs, different scholars have different views on whether seawater can influence the concentration and distribution pattern of REEs in coal; the study of the geochemical characteristics of REEs in coals with different degrees of metamorphism in North China is still at the exploratory stage, and the influence of REEs in coals with different degrees of metamorphism needs to be studied in depth.
This article focuses on the Carboniferous-Permian coal seams in North China to determine the levels of rare earth elements in coal and their distribution patterns in the region. Our research and analysis involved increased sampling of coal seams that have been reported to exhibit abnormal enrichment of these elements. Our objective was to explore the distribution and enrichment patterns of REEs in the Carboniferous-Permian coal of North China.
2 Geological tectonic setting
The North China Plate is a stable region within the Paleo-Tarim-North China Plate, spanning China from west to east and playing a key role in China’s tectonic framework. The tectonic evolution of the North China Plate involved the formation of the Archean-Proterozoic continental core, the splitting and reassembling of the Paleozoic continents, and the intraplate deformation of the Mesozoic and Cenozoic, resulting in the present tectonic pattern (Ge et al., 2009; Dong, Shao, and Mingpei, 2017) (Figure 2). Coal-bearing clastic deposits of alternating marine and land facies of Carboniferous- Permian strata in North China are one of the most important coal-bearing strata in China, with a total thickness of 326–1,146 m (Li, Yu, and Guo, 2002). Table 1 shows the Carboniferous-Permian strata and their distribution in North China.
The Zibo mining area is situated in the eastern margin of the North China Platform and the middle part of the Zibo Syncline in Shandong Province (Figure 3). The coal seams consist mainly of early Paleozoic, Middle and Upper Carboniferous marine-continental coal-bearing strata, as well as Permian and Triassic continental clastic rock coal-bearing strata (Chen et al., 2011).
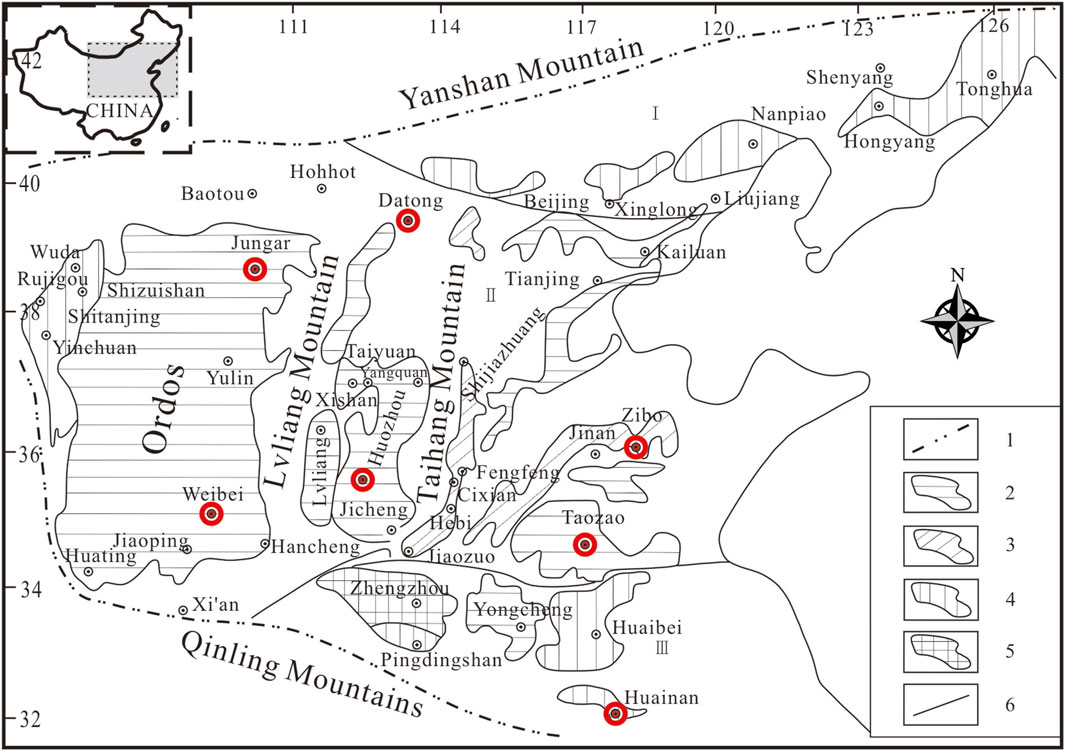
Figure 3. Location of mining areas in North China. (1 North China Plate Boundary; 2 I-II Primary Structural Coal; 3 II-III Structural Coal; 4 III-IV Structural Coal; 5 IV-V Structural Coal; 6 Coal Body Structural Strip Boundary).
The Taozao coalfield is situated in the southern part of Southwest North China and the southern region of Shandong Province. It spans approximately 30 km from east to west and 10 km from north to south. The coalfield is located in a plain area between the northern and southern mountains, with the mountains stretching from east to west (Huang et al., 2004). The coal-bearing strata in this area consist of the Carboniferous-Permian Shanxi Formation and the Taiyuan Formation, with a thickness of around 280 m (Huang et al., 2004).
The Huainan coalfield is situated in the southeastern margin of the North China Platform, in the northern part of Anhui Province and the middle reaches of the Huaihe River. It extends in a NW-W direction from the Tanlu fault zone in the east to the Hefei Depression in the south. The coal measure strata contain the Permian Shihezi Formation, Shanxi Formation, and Carboniferous Taiyuan Formation (Huang et al., 2001; Tanfu et al., 2015).
The Huozhou mining area is situated in the central and southern regions of North China. It spans from the Huoshan in the east to the Luliang Mountain in the west, and from the Hongdong in the south to the Fenxi in the north (Wang, 2012). The coal-bearing strata in the Huozhou coalfield are positioned in the Taiyuan Formation of the Late Carboniferous, the Shanxi Formation of the Early Permian, and the Shihezi Formation. The Taiyuan Formation is a deposit that contains coal-bearing shallow-sea carbonate and clastic rocks. However, it hardly develops mineable coal seams (Huang et al., 2000). During the early Permian period, the sedimentary environment changed from marine facies to terrestrial facies, resulting in the development of thicker mineable coal seams. The late Permian Lower the Shihezi Formation is a delta plain deposit that is favorable to coal formation and forms the main coal-accumulating seam in the Huozhou coalfield (Huang et al., 2000; Song et al., 2018).
The Datong coalfield is situated in the central part of North China and the northern region of Shanxi Province, with a NE trend. Structurally, it is an asymmetric syncline with a steep and narrow eastern wing and a wide and gentle western wing. The east-west width is approximately 30 km from north to northeast of the Yungang and south to the Yujing, and the length of the syncline axis is about 50 km. The Datong coalfield contains coal-bearing strata, including the Benxi Formation of the Middle Carboniferous, the Taiyuan Formation of the Upper Carboniferous, and the Lower Permian Shanxi Formation (Yuan et al., 2011).
The Jungar coalfield is situated in the northeastern part of the Ordos Basin, spanning the northern slope of Shaanxi and the flexure fold belt of Western Shanxi. The internal structure is relatively simple, with only a few small normal faults developed. The coal-bearing rock series comprises the Benxi Formation of the Upper Carboniferous, Taiyuan Formation, and the Shanxi Formation of Lower Permian (Dai et al., 2008). The Weibei coalfield is situated in the southeast of the Ordos Basin and the eastern part of the Weibei uplift. It is oriented in a northeast-southwest direction, with high-angle normal faults and large folds in its internal structure (Cai et al., 2014). The Shanxi Formation and the Taiyuan Formation are the main mineable coal seams (Yao et al., 2009).
3 Samples and method
3.1 Samples
Five mines were selected for sampling work according to the national standard GB482-2008. These include No. 6 coal of Heidaigou mine in Jungar Coalfield, No. 5 coal of Haurusu mine, No. 4 and No. 6 coal of Huangyuchuan mine, and No. 3 and No. 11 coals of Sangshuping mine in Weibei Coalfield, as well as No. 2 and No. 11 coals of Xiayukou mine. A total of 59 samples were taken. Furthermore, according to the data of Huang et al. (2000) on coal mine samples in North China, coal No. 9 of Zibo mine, coal No. 2, 14, 17, 18 of Taozao mine, coal No. 11 of Huainan mine, Anhui province, coal No. 9 and 10 of Datong, Shanxi province, and coal No. 9 and 10 of Huozhou mine, Shanxi province, a total of 59 samples were selected as a supplement. The study included a total of 117 samples (Table 2; Figure 4).
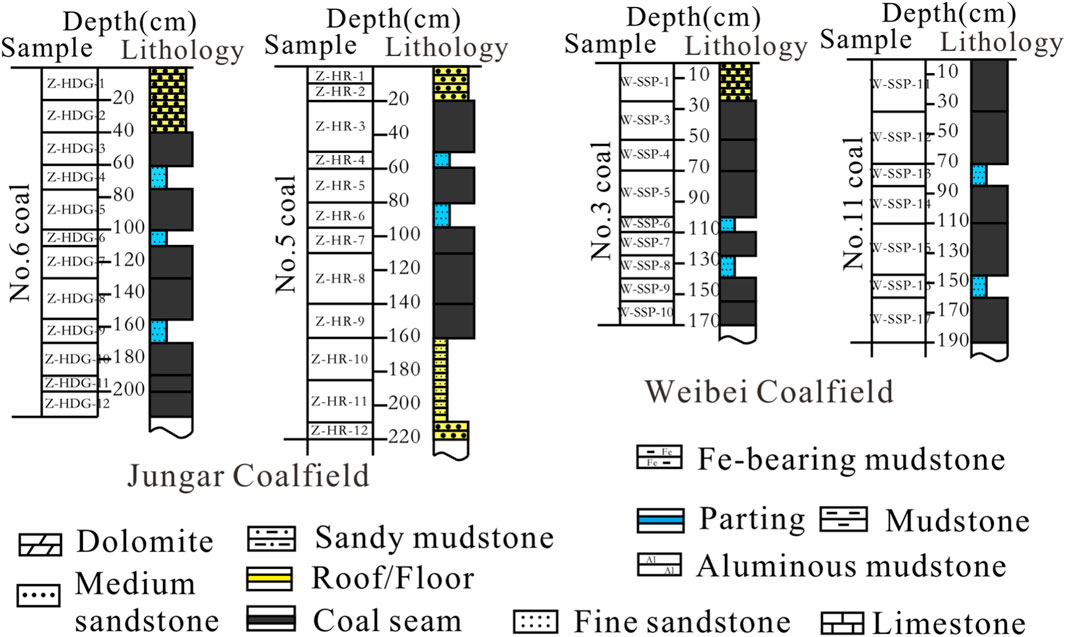
Figure 4. The distribution of Carboniferous-Permian sedimentary sequence, coal seams and sampling positions in North China.
3.2 Analytical methods
Inductively Coupled Plasma Mass Spectrometry (ICP-MS, ICAP Q series): The ELEMENT XR plasma mass spectrometer produced by Thermo Science in Germany, was used to identify 44 elements; including trace elements and rare earth elements (Y, Th, and Sc were not included in this sample test) in coal from parting, roof, and floor. The specific steps are as follows: We weighed 0.05 g of the sample ground to 200 mesh (75 μm) or less and place it in a sample dissolving bottle filled with polytetrafluoroethylene. Next, we added 6 mL of analytically pure HNO3, 0.5 mL HClO4 (also analytically pure), and 2 mL HF (electronically pure). Seal and place on a 200°C hot plate to heat for 48 h. After opening, evaporate the sample in the bottle until a gel is formed, add 2 mL of aqua regia, seal it, and place it on a 200°C hot plate to heat for 24 h before digesting with HNO3 (5%) to a sample solution, without precipitation. Finally, dilute to 50 mL for testing. The trace element concentrations of the samples were measured by inductively coupled plasma mass spectrometry (ICP-MS, ICAP Q series). The ICP-MS analyses are based on the procedures outlined by Dai, Yang, et al. (2015) and were carried out at the State Key Laboratory of Coal Resources and Safe Mining, China University of Mining and Technology (Beijing). For ICP-MS analysis, samples underwent digestion using an UltraClave Microwave High Pressure Reactor (Milestone). The digestion tank contained 330 mL of distilled H2O, 30 mL of 30% H2O2, and 2 mL of 98% H2SO4. The initial nitrogen pressure was set at 50 bars, and the highest temperature was set at 240°C for 75 min. The reagents used for digesting a 50-mg sample were 5 mL of 40% HF, 2 mL of 65% HNO3, and 1 mL of 30% H2O2. Trace element concentrations were calibrated using Multi-element standards, including Inorganic Ventures CCS-1, CCS-4, CCS-5, and CCS-6, NIST 2685b, and the Chinese standard reference GBW 07114 (Dai et al., 2011).
4 Results
Table 3 shows the results of trace element concentrations measured by inductively coupled plasma mass spectrometry (ICP-MS, ICAP Q series). Geochemical parameters of rare earth elements can reflect the characteristics of rare earth elements. Some parameters are derived from the normalized values of rare earth elements. Different reference standards can reflect different characteristics. Usually, the average values of rare earth elements in chondrites, North American shales or upper continental crust are used to standardize rare earth elements in research samples (Huang et al., 2000; Lin et al., 2017; Bau et al., 2018). In order to visually show the variation characteristics of REE in different coal samples, the average abundance of UCC (Taylor and McLennan, 1985) and the Leedy chondrite concentration (Masuda, Nakamura, and Tanaka, 1973) were used to normalize REE in coal.
In order to show the characteristics of REE in different coal samples, this study has adopted a “dichotomy” classification scheme for REE. Rare earth elements are divided into light rare earth elements (LREEs) and heavy rare earth elements (HREEs). The specific methods of classification are as follows: LREE includes La, Ce, Pr, Nd, Pm, Sm, Eu, HREE includes Gd, Tb, Dy, Ho, Er, Tm, Yb, Lu, and Y. The total concentration of each element is ∑REE. L/H represents the ratio of ∑LREE and ∑HREE, which can reflect the distribution difference between Light and Heavy Rare Earth Elements. The larger the L/H value, the more enriched the light rare earth element (LREE) type centration; on the contrary, the more concentrated the rare earth element (HREE) type concentration.
δEu reflects the degree of Eu anomaly, which is useful for identifying provenance, verifying diagenetic conditions, and classifying rocks (Dai et al., 2014). Sedimentary rocks inherit rare earth elements from their parent rocks, and positive and negative Eu anomalies are often determined by the parent rock. Consequently, δEu can effectively identify likely sources of constituents.
Where EuN, SmN, GdN, are the values of Eu, Sm, Gd normalized to the UCC. Research shows that when Ba/Eu > 1,000, the Eu value of the ICP-MS laboratory will be interfered by BaO and BaOH, and the Eu value will be higher (Loges et al., 2012; Dai, Graham, and Ward, 2016). The Ba/Eu distribution in this study was between 10.7 and 460, with an average of 105.1, so the results of Eu anomalies were credible. Most of the δEu values Eq. 1 show negative anomaly characteristics (δEu < 1). These patterns are similar to those found in the upper continental crust. Therefore, it can be inferred that the rare earth elements in Carboniferous-Permian coals in North China mainly originate from the upper continental crust. Coal seams No. 2 and No. 14 of the Shanxi Formation in the Taozao coalfield (samples: ZT2-4, 5, 11; ZT14-1, 2, 6, 7) showed significant positive anomaly characteristics (δEu > 1). There are slight negative Eu anomalies and even some samples have positive Eu anomalies, suggesting that the source of the material has changed significantly during different epochs. It is possible that deep material from the lower crust or material from the Mantle source was added during the coal formation process in the Zibo, Taozao, and Jungar, resulting in a significant change in provenance.
δCe indicates the degree of Ce anomaly. Ce3+ in oxygen-rich sedimentary water can be oxidised to Ce4+ and separated from other REE. If the sedimentary water is anoxic, Ce3+ retains its original valence state (Hower, Ruppert, and Eble, 1999; Dai et al., 2013). The Ce negative anomaly is an important symbol of the marine sedimentary environment of sedimentary rocks.
where CeN, LaN, PrN are the values of Ce, La, Pr normalized to the UCC. Most of the δCe values Eq. 2 range from 0.8 to 1.03, and the δCe values of No. 5 Shanxi Formation coal (sample: Z-HR- 3, 7, 8, 9) in the Jungar coalfield and No. 14 and 17 Taiyuan formation coal (ZZ14-4, ZZ17-4) in the Taozao Mining Area are obviously negative anomalies (<0.7).
5 Discussion
5.1 Distribution pattern of rare earth elements
The normalised distribution patterns of REE to chondrites (Masuda, Nakamura, and Tanaka, 1973) show the characteristics of light REE enrichment, heavy REE depletion, but a slight variation in the heavy REE range, and Eu is mostly characterised by negative anomalies and most samples show a clear lanthanide prevailence in the whole REE profile (Figures 5A1, B1, C1 and Figures 6A1, B1, C1), indicating that there is a relatively stable supply of terrestrial materials during the Late Paleozoic Carboniferous-Permian coal formation period in North China. The L/H of Late Paleozoic Carboniferous-Permian coal in North China is generally between 2 and 12, which is more than 12 in roof and floor, and the highest in pyrite nodules, reaching 21.03 (sample: ZT14-6); the peat swamps affected by marine facies is relatively rich in HREE (Wang et al., 2008). Except that the L/H value of No. 5 coal in the Jungar Shanxi Formation is 1.86 (sample: Z-HR-7), the L/H ratio of the Taiyuan Formation coal is generally lower than that of the Shanxi Formation coal. After testing the concentrations of rare earth elements in 58 samples of Carboniferous-Permian coal samples, roof and floor and parting in North China(Huang, Jiu, and Li, 2019), Huang found that the L/H ratio of high-sulphur and low-ash coal in the Huozhou and Zaozhuang mining areas reached the lowest value (samples: H9-2, H9-2, H10-2, and H10-3), but the L/H of calcareous pyrite nodules in high-sulphur coal of Taiyuan formation reached the maximum value in all samples.
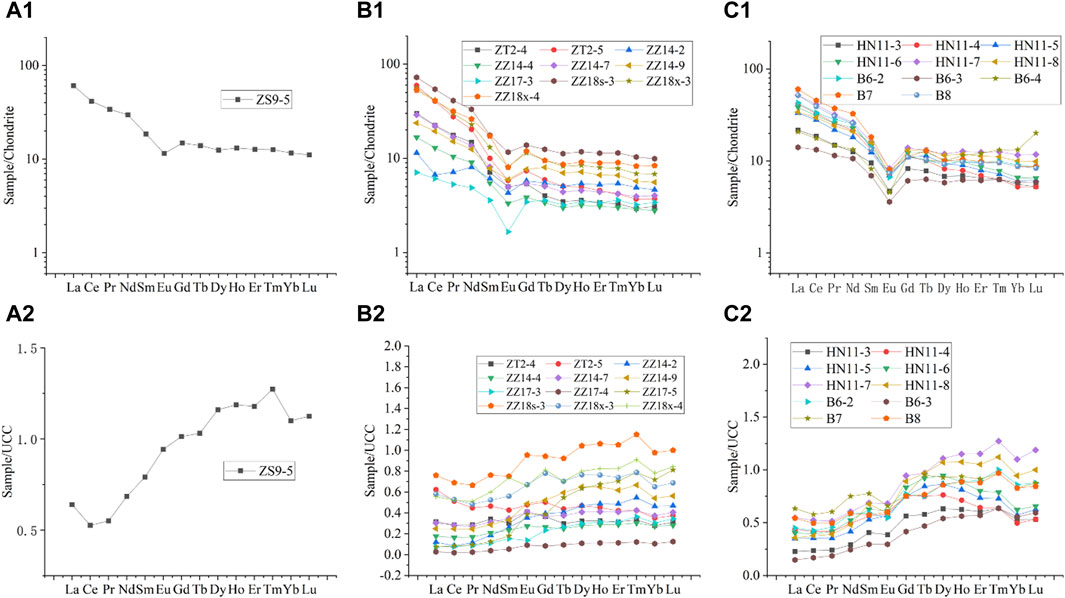
Figure 5. The REE distribution pattern of Carboniferous-Permian coals in North China, normalized to UCC (Taylor and McLennan, 1995) and Chondrite (Masuda, Nakamura, and Tanaka, 1973): (A1) The Zibo coal, normalized to Chondrite; (A2) The Zibo coal, normalized to UCC; (B1) The Taozao coal, normalized to Chondrite; (B2) The Taozao coal, normalized to UCC; (C1) The Huainan coal, normalized to Chondrite; (C2) The Huainan coal, normalized to UCC.
Upon analysing the data presented in Table 3, it is evident that the average values of L/H in the Zibo, Taozao, Datong, Jungar and Weibei coalfields in Shandong Province are higher than those in other areas of China. Conversely, in the Huainan mining area of Anhui Province, the average value of L/H in the Huozhou coalfield is significantly lower than that in other areas of China and slightly lower than the global average. The distribution patterns of rare earth elements vary even within different layers of the same coal seam. In the Taozao, Huainan, Datong and Jungar Heidaigou coal seams (Figures 5B, C, 6B, C), the graphic curves tend to form a “V” shape, but the concentration of rare earth elements differs significantly, as shown in the distribution pattern diagram. The distribution curve is not coincident, but rather exhibits a certain interval arrangement. This is consistent with the work done by many academics (Dai, Graham, and Ward, 2016; Dai et al., 2020), which shows that the distribution pattern of rare earth elements in coal indicates the inhomogeneity of these elements within the coal seam. It also reflects significant changes in the microenvironment of the same coal seam during coal formation.
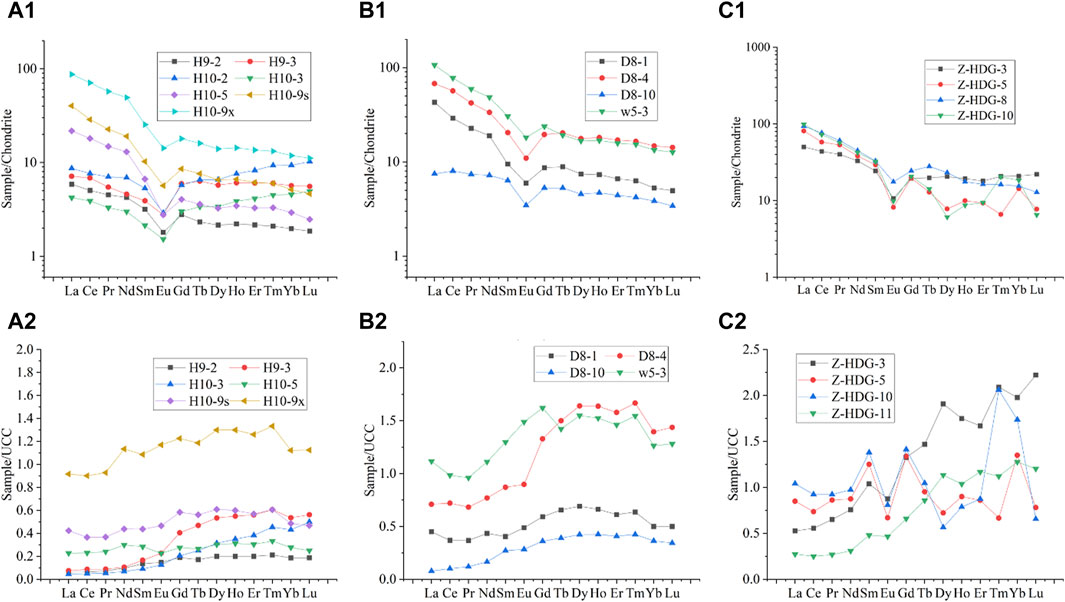
Figure 6. The REE distribution pattern of Carboniferous-Permian coals in North China, normalized to UCC (Taylor and McLennan, 1995) and Chondrite (Masuda, Nakamura, and Tanaka, 1973): (A1) The Huozhou coal, normalized to Chondrite; (A2) The Huozhou coal, normalized to UCC; (B1) The Daotong coal, normalized to Chondrite; (B2) The Datong coal, normalized to UCC; (C1) The Jungar coal, normalized to Chondrite; (C2) The Jungar coal, normalized to UCC.
The distribution patterns of rare earth elements in Carboniferous-Permian coals in North China (as shown in Figures 5, 6) are characterized by enrichment of LREE, deficit of HREE with gentle changes, and obvious negative anomalies of Eu. These patterns are similar to those found in the upper continental crust. Therefore, it can be inferred that the rare earth elements in Carboniferous-Permian coals in North China mainly originate from the upper continental crust. The upper continental crust contains a significantly higher concentration of LILE (Large Ion Lithophile Elements) compared to the primitive mantle. This leads to an enrichment of LREEs and a deficiency of HREE (Tatsumi, Hamilton, and Nesbitt, 1986; Shu et al., 2023). The deficiency of HREEs fractionation in the upper continental crust results in a deficiency of HREEs differentiation (Zhao, Wang, and Zhao, 2021; Teng et al., 2023), and the Eu negative anomalies are attributed to the deficiencies of Eu in the upper continental crust due to elemental differentiation (Rudnick, 1992).
When the distribution pattern of REE normalized to chondrites is similar, the REE normalized to Upper Continental Crust diagram can be used to distinguish different forms of differentiation and further identify the characteristics of the source rocks (Gallet et al., 1998; Awadh et al., 2023). The UCC standardized diagram of rare earth elements in Carboniferous-Permian coals in North China (Figures 5, 6) shows that the Zibo (Figure 5A2), Taozao (Figure 5B2), and Jungar (Figure 6C2) coals differ from the other groups. They exhibit deficits in light rare earths and relatively enriched heavy rare earths. Additionally, there are slight negative Eu anomalies and even some samples have positive Eu anomalies, suggesting that the source of the material has changed significantly during different epochs. It is possible that deep material from the lower crust or material from the Mantle source was added during the coal formation process in the Zibo, Taozao and Jungar, resulting in a significant change in provenance.
The distribution pattern of rare earth elements in coal seams affected by magmatic contact metamorphism has significantly changed (Li et al., 2018; Sutcu et al., 2021; Moroeng, Murathi, and Wagner, 2024). For instance, taking No. 2 and No. 14 coal of Shanxi Formation in the Taozao coalfield (Figure 7), the influence of seawater during the formation of coal seam is not apparent, and the trace elements in coal are mainly controlled by terrigenous debris (Huang et al., 2001; Zhang et al., 2022). The concentration of associated elements in nodular pyrite in mudstone of the coal seam roof is significantly higher than that in layered pyrite in the coal seam. This difference may be due to the original concentration of trace elements in the metallogenic material and the different coal-forming and mudstone swamp microenvironments (Liu, Yang, and Tang, 2000; Hao et al., 2023). Additionally, the coal seam is affected by magmatic contact metamorphism. In the contact zone between the magmatic intrusion and the coal seam, and in the coal seam close to the magmatic intrusion, there is no significant loss of europium, and the distribution pattern of rare earth elements tends to follow that of the magmatic parent rock. As a result, the typical right-leaning “V” shape of the rare earth distribution pattern is no longer present, and instead, it has an approximate linear shape (Figure 7A). However, in coal seams distant from magmatic intrusions, the distribution pattern of rare earth elements exhibits a V-shaped feature that leans to the right (Figure 5B1).
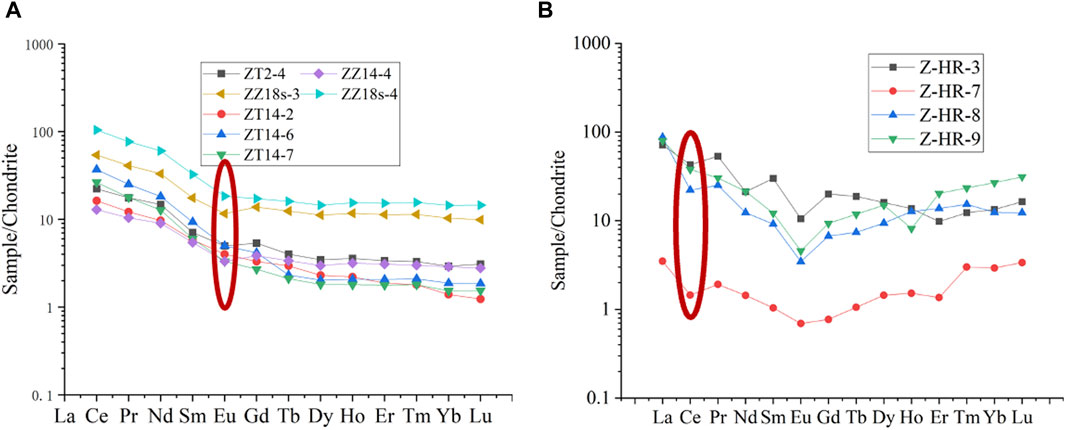
Figure 7. The REE distribution pattern of Carboniferous-Permian coals in North China, normalized to Chondrite (Masuda, Nakamura, and Tanaka, 1973): (A) The Taozao coal and pyrite nodules (ZT14-2∼7), No obvious Eu anomalies and no typical right-skewed “V” shape of the REEs distribution patterns, it has an approximately linear shape; (B) The Jungar coal of Shanxi Formation, significant negative Ce anomalies and Eu deficiency.
Some elements—particularly the HREE group—are characterised by behaving somewhat differently in terms of maximum content. For example, the maximum contents of Lu and Yb occur in the 2-HR-9 (coal) sample, while the maximum contents of Ho, Er and Yb occur in the ZZ18x-5 (floor) sample; and the maximum contents of Gd and Tb occur in the ZS9-b (reef) sample. This may be related to the well-known tetrad effect. REE geochemical studies conducted by scholars on various natural materials have shown that the tetrad effect of REEs generally exists (Masuda et al., 1987; Jolliff et al., 1989; Sami et al., 2020), which is helpful in understanding the genesis of mineral deposits and revealing rock-specific genesis mechanisms (Monecke et al., 2002; Zaharescu et al., 2017; Abedini, Azizi, and Dill, 2020). Meanwhile, the (La/Yb) N ratio as a geochemical factor can be used to evaluate the acidic and alkaline conditions of the depositional environment, and Abedini, Calagari, and Azizi (2018) found that the (La/Yb) N values are lower under acidic conditions and higher under alkaline conditions. The (La/Yb) N of samples Z-HR-9, ZZ18x-5, and ZS9-b are 3.04, 4.86 and 23.68, respectively, from which it can be concluded that samples Z-HR-9 from the Jungar coalfield and ZZ18x-5 from the Taozao coalfield under acidic conditions and sample ZS9-b from the Zibo coalfield under alkaline conditions have higher (La/Yb) N ratios. It can be inferred that as the stability of these complexes increases with atomic number, the HREEs are preferentially associated with some minerals in coal in solution, such as in the form of carbonate complexes (Cantrell and Byrne, 1987; Abedini, Calagari, and Azizi, 2018). Furthermore, the occurrence of the tetrad effect in the normalised REE distribution pattern may be due to the presence of various ligands in the aqueous system and the stability of the REE complexes (Feng et al., 2014; Abedini, Azizi, and Dill, 2020). This implies that acid mine drainage promotes the precipitation of REE-bearing minerals, especially the HREE group. This may explain the increase in HREEs in these samples.
Seawater is enriched in both LREE and HREE. Additionally, rare earth element distribution in seawater exhibits a significant deficiency of Ce, i.e., La > Ce, and Ce is a negative anomaly(Fleet, 1984; Floback and Moffett, 2021; Liu et al., 2022), which is obviously different from the distribution pattern of trace elements in river water and other geological bodies (Wang et al., 2000). The deficiency of Ce may be due to oxidation of Ce3+ to Ce4+ and precipitation from solution as CeO2 under marine conditions, while other rare earth elements still retain the valence state of +3 (Liu, Yang, and Tang, 2000; Huang et al., 2001; Li et al., 2008). Huang et al. (2000) studied the REE characteristics of Late Palaeozoic coals in the Huozhou Coalfield, concluded that the REE content of seawater-influenced high-sulphur coals was lower than that of terrestrial facies low-sulphur coals; Zhao, Tang, and Li (2000) studied the REE characteristics of coal from the Huainan Coalfield and found that the REE concentrations of coals from the Huainan Coalfield increased when the seawater influence was weakened; Wang et al. (2008) analysed the REE characteristics of coals from Jinbei. He concluded that the enrichment and loss of REEs in seawater-influenced coals were related to the paleogeography of coal-forming swamps. Dai, Ren, and Li (2003) concluded that seawater exhibits significant δCe-negative anomalies and very low REE concentrations. The distribution pattern diagram of No. 5 coal in Shanxi Formation of the Jungar coalfield exhibits a negative Ce anomaly and significant Eu deficiency (Figure 7B), while the distribution of rare earth elements in seawater shows significant Ce deficiency. This indicates that the No. 5 coal of Shanxi Formation in the Jungar coalfield was also influenced by seawater during coal formation. The REE concentrations in the seawater-affected coals are generally low (Z-HR-3, 7, 8, and 9). The negative δCe anomalies of the No. 5 Shanxi Formation in Jungar are more pronounced, indicating a deepening of the peat swamp water body after the seawater intrusion, increased reducibility and depletion of Ce sources. The distribution pattern of the coals in the No. 5 Shanxi Formation shows no significant differentiation between the LREE and HREE sections (Figure 7B), indicating a stable source of provenance supply. The intrusion of seawater does not increase the concentration of REEs. However, it can deepen the water body of peat swamps and create a more reducing depositional environment, which affects the distribution pattern of REEs. This is evident in the negative anomaly at Ce.
5.2 Enrichment characteristics of rare earth elements
5.2.1 Enrichment characteristics of HREE in North China
Upon analysing the data presented in Table 3, it is evident that the average values of ∑LREE/∑HREE in the Zibo, Taozao, Datong, Jungar and Weibei coalfields in Shandong Province are higher than those in other areas of China. Conversely, in the Huainan mining area of Anhui Province, the average value of ∑LREE/∑HREE in the Huozhou coalfield is significantly lower than that in other areas of China and slightly lower than the global average (refer to Figure 8A).
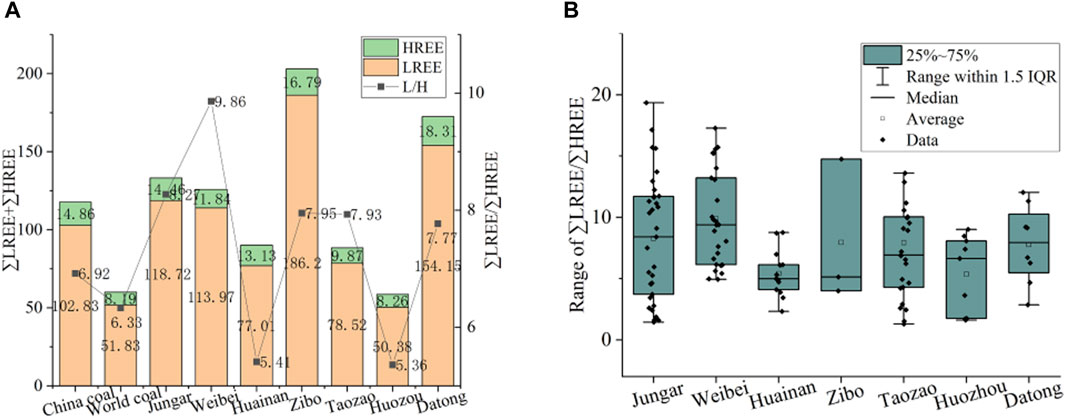
Figure 8. Characteristics of rare earth element average concentrations in Carboniferous-Permian coals from North China: (A) Bar-graphs of light and heavy rare earth elements average concentrations in Carboniferous-Permian coals from North China; (B) Box-plots of ∑LREE/∑HREE in Carboniferous-Permian coals in North China.
As can be seen from Figure 8B, the average L/H value of coal seam, roof, floor and parting in the Zibo mining area of Shandong Province is about 3.99–14.75, and the average value is 7.95, which indicates in the Zibo mining area in Shandong province, light rare earth elements are enriched more than heavy rare earth elements; The L/H distribution in the coal seam, roof, floor and parting of the Taozao mining area ranged from 1.29 to 21.03, with an average value of 7.93, indicating the light rare earth elements are more enriched than heavy rare earth elements in the Taozao mining area; The L/H distribution in coal seam, roof, floor and parting of the Huainan mining area in Anhui Province ranges from 2.32 to 8.76, with an average value of 5.41, indicating that light rare earth elements are enriched in heavy rare earth elements in the Huainan mining area of Anhui; The L/H distribution in coal seam, roof, floor and parting of the Huozhou coalfield is 1.61–9.01, with an average value of 5.36, indicating that light rare earth elements are enriched in the Huozhou coalfield compared to heavy rare earth elements; The distribution of L/H in coal seam, roof, floor and parting in the Datong mining area is about 4.67–12.03, with an average value of 7.77, which indicates that light rare earth elements are more abundant than heavy rare earth elements in the Datong mining area; The distribution of L/H in coal seam, roof, floor and parting in the Jungar coalfield is about 1.64–19.34, with an average of 8.27, which indicates that light rare earth elements are enriched in the Jungar coalfield compared to heavy rare earth elements; The distribution of L/H in the coal seam, roof, floor and parting of the Weibei coalfield ranges from 4.93 to 17.28, with an average of 9.86, indicating that light rare earth elements are more enriched than heavy rare earth elements in the Weibei coalfield.
Among them, the average ΣHREE in Datong coalfield in Shanxi, Zibo coalfield in Shandong and Jungar coalfield in Ordos are all greater than 14 μg/g and HREE is highly enriched; The average ΣHREE in Anhui Huainan coalfield, Ordos Weibei coalfield and Shandong Taozao coalfield are all greater than 10 μg/g and HREE is relatively enriched; The average value of ΣHREE in the Huozhou coalfield in Shanxi is relatively low (<8 μg/g), and HREE is relatively deficient. In summary, the northern part of North China is gener-ally rich in heavy rare earth elements; The southern part of North China is relatively enriched in heavy rare earth elements; Although the heavy rare earth elements in the central part of North China are lower than the average of China coal, they are still higher than that of world coal. The heavy rare earth elements show a significant deficit compared to other regions in China (Figure 8A).
5.2.2 Enrichment characteristics of rare earth elements in coal in North China
China’s large-scale coal production bases are mainly located in the north, especially in the northern Ordos Basin, and some coal seams with unusually high REE concentrations have been found. For example, the rare element concentration of carboniferous coal seams in Daqingshan, Inner Mongolia, reached 721 μg/g, and the rare element concentration of No. 6 coal seams in Heidaigou and Haerwusu, Inner Mongolia, reached 1,031 μg/g and 1,347 μg/g, respectively (Huang, Jiu, and Li, 2019). The world REE concentration in coal calculated by Valcovic is 46.3 μg/g (Valkovic, 1983), the total REE concentration in US coal calculated by Finkleman is 62.1 μg/g (Finkelman, 1993), and the REE concentration in Chinese coal calculated by Ren is 105.57 μg/g (Ren et al., 1999). Ketris M. P. and Yudovich YaE. (2009) give an average of 68.5 μg/g in coal, which is much lower than 168.4 μg/g in the upper continental crust (Taylor and McLennan, 1995). It is evident that the average concentration of rare earth elements in Chinese coal is higher than in the US. In 2012, Dai et al. (2014) reported that the concentration of rare earth elements in Chinese coal was 137.9 μg/g. Rare earth elements in raw coal are typically enriched to a certain degree (300 μg/g or more) before they can be enriched to close to or exceed industrial grade in coal ash (Huang, Jiu, and Li, 2019). Generally, the concentration of rare earth elements in coal is low and difficult to use directly. However, after combustion, rare earth elements in coal are enriched again, resulting in a much higher concentration of rare earth elements in coal ash compared to coal. Seredin, Dai, and Chekryzhov (2012) report that the average REE concentration in coal ash in the world is 404 μg/g, and in the US. it is 513 μg/g (Seredin, Dai, and Chekryzhov, 2012; Huang, Jiu, and Li, 2019). These data show that the REE concentrations in coal ash can be 6 to 9 times higher than in raw coal.
Finkelman (1993) calculated the concentrations of REE in coal and coal ash in the United States and found that if the REE in coal could be extracted from existing coal production, more than half of the domestic demand for REE could be met. Dai et al. also found that the coal seam enriched with rare earth elements is significantly enriched with rare earth elements in coal ash. For instance, the coal ash from Hedaigou coal mine has an average REO (i.e., REE oxides) of 0.15%, Harwusu coal mine has an average REO of 0.14%, Guanbanusu coal mine has an average REO of 0.11%, and Adaohai coal mine has an average REO of 0.98% (Dai et al., 2006). This indicates that rare earth elements are significantly enriched in coal ash and can reach or exceed industrial grade, thus having potential for industrial development. Seredin, Dai, and Chekryzhov (2012) suggested that the industrial grade of REE in coal ash is REO >0.1%.
North China is abundant in coal resources, with a focus on developing Carboniferous and Permian coal seams. Studies have shown that the rare earth concentrations in coal seams in North China are generally high, and the average value can be as high as 3 times that of US coal (Huang et al., 2000). By analysing the data in Table 3, it is evident that the total concentration of rare earth elements in the Taiyuan Formation coal in the Zibo Mining Area varies widely, ranging from 16.68 μg/g to 497.98 μg/g, as shown in Figure 9. The average value of ∑REE is 56 μg/g, slightly lower than that of world coal of 68.42 μg/g, which is about one third of the average value of China coal of 135.89 μg/g, and compared with most China coals, the concentration of rare earth elements shows a significant deficit. However, the coke mixed sample (sample: ZS9-b) on the floor had a concentration of 497.9 μg/g, showing obvious enrichment characteristics. The total REE concentrations in coal from the Shanxi and Taiyuan formations in the Taozao mining area tend to be stable, ranging from 4.90 μg/g to 243.06 μg/g. Most of the coal samples have ∑REE ranging from 30 μg/g to 70 μg/g, with an average value of 88.44 μg/g. Most coal samples have ∑REE values ranging from 30 μg/g to 70 μg/g, with an average value of 88.44 μg/g. This value is higher than the world coal’s ∑REE value of 68.42 μg/g, but only about half of the average value of Chinese coal, which is 135.89 μg/g. Compared to most coal in China, this coal has a significant deficit in rare earth element concentration.
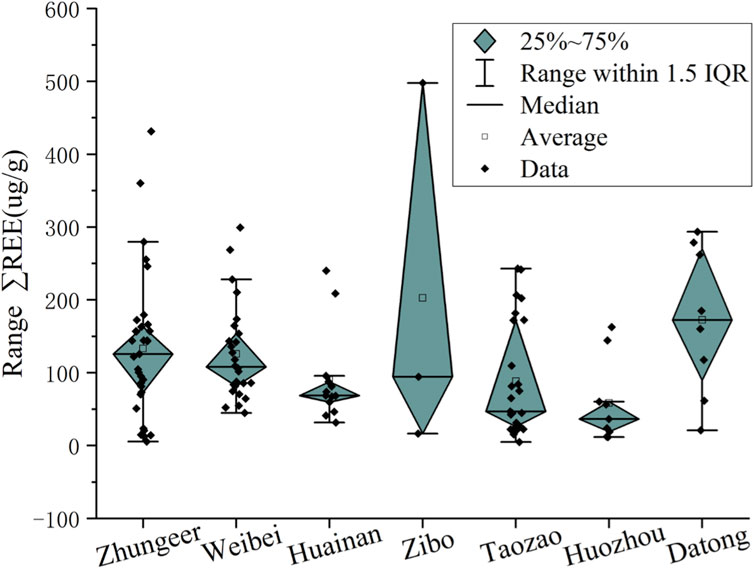
Figure 9. Concentration characteristics of rare earth elements in late Paleozoic coal samples from various mining areas in North China.
As can be seen in Figure 9, the ∑REE concentration of coal samples from the Taiyuan Formation in the Huainan mining area is the lowest, averaging 18 μg/g. The average REE concentration of coal samples from the Shanxi Formation is also not high, averaging 63 μg/g (Huang et al., 2000). The Xiashihezi Formation coal in the Huainan mining area of Anhui Province exhibits a wide variation in total rare earth element concentrations, ranging from 31.66 μg/g to 240.27 μg/g. The total rare earth element concentrations of coal samples from the Xiashihezi Formation and Shangshihezi Formation are significantly higher, with the former averaging 160.361 μg/g and the latter 191.243 μg/g (Huang et al., 2000). The coal’s REE concentration increases in the order of Taiyuan Formation, Shanxi Formation and Xiashihezi Formation. This characteristic is related to the transportation distance of terrigenous materials and the control of the sedimentary environment on rare earth elements. The concentration of rare earth elements in coal is higher when closer to the terrigenous source due to the greater influence of terrigenous supply. Conversely, the concentration of rare earth elements in coal is lower when further away from the terrestrial source due to the greater influence of seawater and marine biological debris. The Taiyuan Formation coal in the Zaozhuang mining area was formed in a swamp environment that was influenced by an epicontinental sea. In contrast, the Taiyuan Formation coal in Datong, Shanxi Province was formed in a delta plain environment, with the coal-forming swamp located near the terrigenous area. This resulted in less seawater influence and no leaching. The mudstone parting of Datong mining area (samples w5-10, w5-15, mean: 286.2 μg/g) contains a significantly higher concentration of rare earth elements than the coal. The total rare earth element concentrations in the coal and parting of the Datong mining area in Shanxi Province vary widely, ranging from 20.92 μg/g to 293.62 μg/g, The majority of coal samples have a ∑REE value ranging from 118 μg/g to 228 μg/g, with an average value of 172.46 μg/g. The concentration of rare earth elements (ZZ14-2, 4, 7, 9) in low-medium ash high-sulfur coal of Taiyuan Formation in Zaozhuang coalfield of Shandong Province is lower than that of Taiyuan Formation coal of Datong in Shanxi Province (D8-4, w5-2) and Shihezi Formation coal of Huainan coalfield in Anhui Province (HN11-3 ∼ B8), with a difference of 2–15 times. From Figure 9, it can be seen that the concentrations of REE in coals from the Huozhou coalfield in Shanxi Province vary widely, ranging from 11.64 μg/g to 162.83 μg/g. These values are significantly lower than the average concentration of rare earth elements in coal found in China. The majority of coal samples have a ∑REE value ranging from 20 μg/g to 50 μg/g, with an average value of 58.64 μg/g.
The total REE concentration in the coal of the Junge coalfield varies widely, ranging from 13.99 μg/g to 473.74 μg/g. The ∑REE value of most coal samples ranges from 118 μg/g to 228 μg/g, and the average value is 155.71 μg/g (Figure 9), which shows obvious enrichment compared to most coals in China. The average REE concentration of coal from the Shanxi Formation on the eastern margin of the Ordos Basin is 120. 90 μg/g, which is very close to the average REE concentration of 120. 72 μg/g in the Taiyuan Formation coal, and the value of ∑REY in the Shanxi Formation coal first decreases and then increases, while the value of ∑REY in the Taiyuan Formation coal gradually decreases from north to south. Crowley, Stanton, and Ryer (1989); Hower, Ruppert, and Eble (1999) study the geochemical characteristics of REEs in coal containing volcanic ash and its alteration minerals in the United States, found that the coal seam located in the lower part of the parting of volcanic ash causes, its REE concentrations than the overlying parting several times to tens of times higher, The analysis shows that it is the enrichment of rare earth elements in the coal seam caused by groundwater leachate. In the No. 6 coal of the Jungar Coal Field in the north of the eastern edge of the Ordos Basin, the REE concentrations in the partings are much lower than those in the underlying coal seams, and the cause is also considered to be leached by groundwater (Dai et al., 2008; Shi et al., 2014). The REE-rich leachate precipitated as authigenic mineral phases in the underlying coal (Hower, Ruppert, and Eble, 1999). As shown in Figure 10, the concentration of REE in the roof of the No. 6 coal seam in the Hedaiigou mining area is higher than that in the coal, reaching 360.2 μg/g, with an average value of 277.37 μg/g, while the concentration of REE in the parting is lower, with an average value of only 25.39 μg/g. It is speculated that the low REE concentration in the parting is due to groundwater circulation, which brings rare earth elements in parting into the underlying coal seam only in the form of leachate (Kang et al., 2014; Sun et al., 2014). In addition, in the No. 6 coal seam sample of the Hedaigou mining area, the concentration of rare earth elements in the coal sample is significantly higher than that in the parting sample, and the δCe in the coal seam overlying the parting is generally less than 1 (Figure 10). It is speculated that the formation of the partings is related to the influence of seawater, and the rare earth elements are not enriched in seawater (Piepgras and Jacobsen, 1992; Dai, Ren, and Li, 2003), which is one of the reasons for the low concentration of rare earth elements in partings.
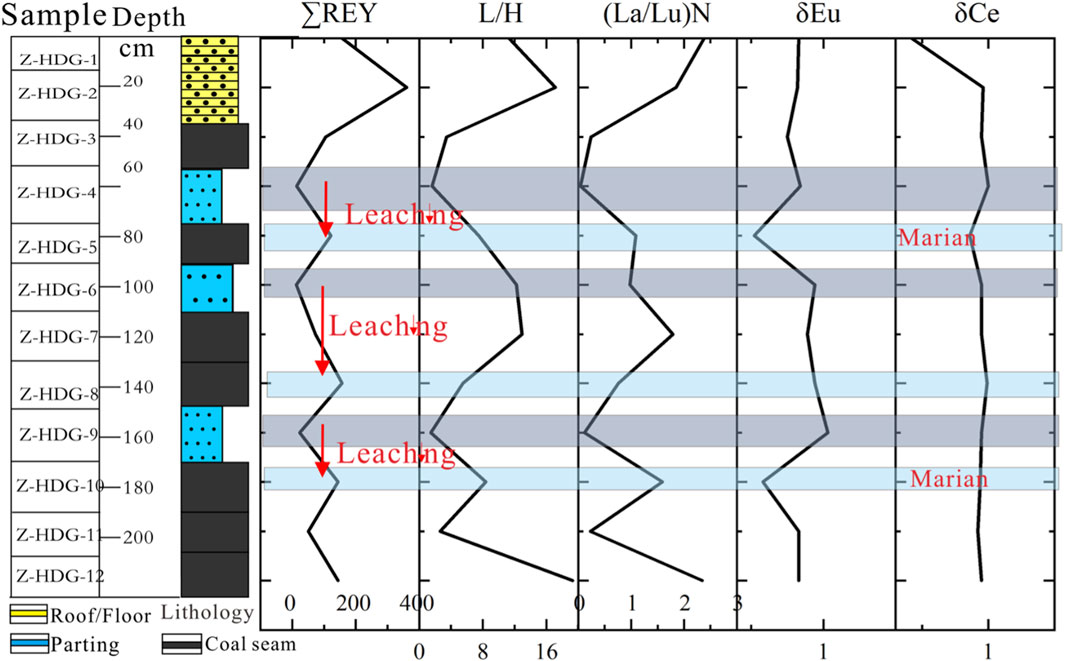
Figure 10. Vertical distribution of geochemical parameters of rare earth elements. (La/Lu) N, (La/Sm) N, (Gd/Lu) N, (La/Yb) N are the normalized ratios of rare earth elements to the UCC [67]. 2EuN/(SmN + GdN), δCe = Ce/Ce∗ = 2CeN/ (LaN × PrN), where EuN, SmN, GdN, CeN, LaN, and PrN are the values of Eu, Sm, Gd, Ce, La, Pr normalized to the UCC.
The concentration of REE in the Weibei coalfield varies widely (Figure 9), ranging from 55.44 μg/g to 340.87 μg/g. The ∑REE value of most coal samples ranges from 55 μg/g to 140 μg/g, and the average value is 145.20 μg/g, which shows obvious enrichment compared to most coals in China.
From a macro perspective (Figures 9, 11), the average concentration of rare earth elements in the coal of the Datong mining area in Shanxi Province, North China is 172.46 μg/g, and the concentration in parting is as high as 293.6 μg/g. The average concentration of rare earth elements in the coal of the Jungar mining area in Ordos Basin is 155.71 μg/g, and the concentration of rare earth elements in floor is 431.5 μg/g. The average concentration of rare earth elements in the Weibei coalfield is 145.20 μg/g, and the concentration in parting is as high as 299.5 μg/g. These three mining areas show obvious characteristics of REE enrichment and have certain industrial exploitation prospects. The average concentration of rare earth elements in the coal of Taozao mining area is 88.44 μg/g, and the highest value is 243.1 μg/g in floor. The average concentration of rare earth elements in the coal of the Huainan mine in Anhui Province is 80.27 μg/g, and the highest value is 240.08 μg/g in roof. These two mining areas are characterised by a slight enrichment. The average concentration of rare earth elements in coal in the Huozhou mining area of Shanxi Province was 58.64 μg/g, and the highest value was 162.8 μg/g in parting. The average concentration of REE in coal in the Zibo mining area is 56.00 μg/g, which shows obvious depletion and has no significant mining value. However, the concentration in the mixed sample of coking rock and coal in floor (sample: ZS9-b) was as high as 497.9 μg/g. It is worthy of further investigation and exploitation.
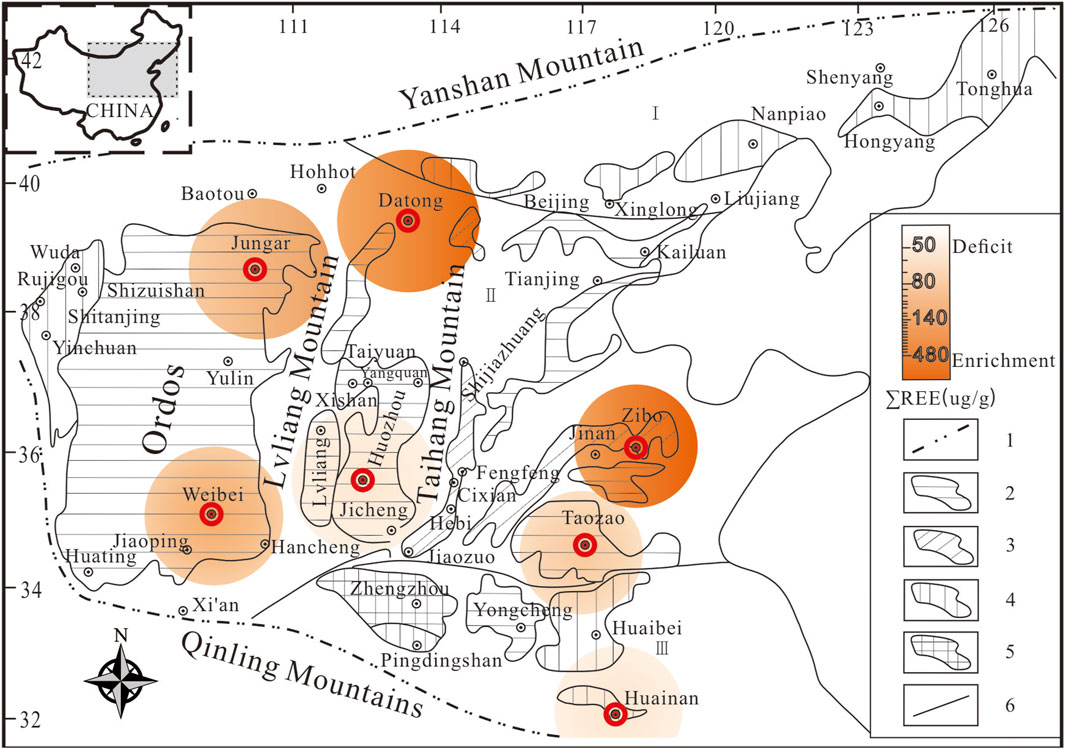
Figure 11. Rare earth enrichment zone of late palaeozoic coals and its partings, roofs and floors in North China. (1 North China Plate Boundary; 2 I-II Primary Structural Coal; 3 II-III Structural Coal; 4 III-IV Structural Coal; 5 IV-V Structural Coal; 6 Coal Body Structural Strip Boundary).
In general, as shown in Figure 11, the concentrations of REE in the carboniferous Permian coals in the northern, northwestern and western mining areas of North China are high and show obvious enrichment characteristics. The southeastern mining area of North China also has a relatively high concentration of REE in the carboniferous Permian coal, which is slightly lower than the average REE concentration of coal in China, showing a slight enrichment. The concentration of rare earth elements in Carboniferous coals in the central and eastern parts of North China is low, showing an obvious deficit.
6 Conclusion
The present study examined the REE-enriched coal samples from seven coal mines in North China. The concentration, distribution pattern and geological influences of REEs in coals, roofs, floors and partings were investigated, such informations are crucial for future mining planning. Further study of the occurrence mode of REE in coal in North China, as well as the provenance characteristics and sedimentation rates, will deepen and improve the understanding of REEs enrichment and mineralisation in coals in North China, and at the same time provide a reliable geological basis for the metallogenic mechanism of a variety of critical metal minerals (REEs-rich, Li-rich, Ga-rich minerals, etc.,) in coal seams.
(1) The REE distribution patterns in Late Palaeozoic coals in North China show that the HREEs are very rich in the north, relatively rich in the south and obviously deficient in the central part of North China. It can be concluded that with favourable HREEs in Late Palaeozoic coals in North China are distributed in the Datong, Jungar, Weibei and the Huainan Coalfields.
(2) The REEs concentration in Late Paleozoic coals in North China generally showed high enrichment characteristics in the northern, northwestern and western mining areas, far exceeding the average REEs concentration in most other coals in China. Some samples, including coals, roofs, floors, and partings, have concentrations above 300 μg/g, which is close to or exceeds the industrial grade. The REEs concentration in Late Paleozoic coal in the southeastern coalfields of North China is slightly lower than the average of the China coal, but higher than the average of the World coal. In the central and eastern parts of North China, the REEs concentration in Late Paleozoic coals is low and shows obvious depletion. However, the REEs concentration in the partings of the Zibo Coalfield is higher (more than 400 μg/g). It can be concluded that the favourable areas for REEs in Late Paleozoic coals in North China are the Datong Coalfield, the Jungar and Weibei coal mines in the eastern margin of the Ordos Basin, and the Zibo Coalfield.
(3) North China, especially the northern part of the Ordos Basin, has the conditions for the development of large coal-type REE deposits. The REEs enrichment in coals is controlled by provenance and volcanism, and is related to the distance of transport terrigenous material and the sedimentary environment. The closer to the terrestrial source, the greater the influence of terrestrial supply, the higher the REE concentration in the coal; the further from the terrestrial source, the greater the influence of seawater and marine biological debris, the lower the REE concentration in the coal. Magmatic contact metamorphism and volcanic ash deposition influence the degree of rare earth element distribution patterns and enrichment in local coal seams. Additionally, due to groundwater circulation, only the REEs in partings are transported into the underlying coal seam in the form of leachate, resulting in a low concentration of REEs in partings.
Data availability statement
The original contributions presented in the study are included in the article/Supplementary material, further inquiries can be directed to the corresponding authors.
Author contributions
LW: Conceptualization, Data curation, Methodology, Writing–original draft, Writing–review and editing. WH: Funding acquisition, Supervision, Writing–review and editing. YZ: Funding acquisition, Supervision, Writing–review and editing. BJ: Data curation, Investigation, Writing–review and editing.
Funding
The author(s) declare that financial support was received for the research, authorship, and/or publication of this article. This work was financially supported by the “National Natural Science Foundation of China” (Grant No. 42272201), “National key research and development program” (Grant No. 2021YFC2902003) and the “National Science and Technology Major Project” (Grant No. 2017ZX05009-002).
Conflict of interest
The authors declare that the research was conducted in the absence of any commercial or financial relationships that could be construed as a potential conflict of interest.
Publisher’s note
All claims expressed in this article are solely those of the authors and do not necessarily represent those of their affiliated organizations, or those of the publisher, the editors and the reviewers. Any product that may be evaluated in this article, or claim that may be made by its manufacturer, is not guaranteed or endorsed by the publisher.
References
Abedini, A., Ali, A. C., and Rezaei Azizi, M. (2018). The tetrad-effect in rare earth elements distribution patterns of titanium-rich bauxites: evidence from the Kanigorgeh deposit, NW Iran. J. Geochem. Explor. 186, 129–142. doi:10.1016/j.gexplo.2017.12.007
Abedini, A., Rezaei Azizi, M., and Dill, H. G. (2020). The tetrad effect in REE distribution patterns: a quantitative approach to genetic issues of argillic and propylitic alteration zones of epithermal Cu-Pb-Fe deposits related to andesitic magmatism (Khan Kandi District, NW Iran). J. Geochem. Explor. 212, 106516. doi:10.1016/j.gexplo.2020.106516
Arbuzov, S. I., Finkelman, R. B., Il’enok, S. S., Maslov, S. G., Mezhibor, A. M., and Blokhin, M. G. (2019b). Modes of occurrence of rare-earth elements (La, Ce, Sm, Eu, Tb, Yb, Lu) in coals of northern asia (review). Solid Fuel Chem. 53, 1–21. doi:10.3103/S0361521919010026
Arbuzov, S. I., Yu Chekryzhov, I., Finkelman, R. B., Sun, Y. Z., Zhao, C. L., Il'enok, S. S., et al. (2019a). Comments on the geochemistry of rare-earth elements (LA, CE, SM, EU, TB, YB, LU) with examples from coals of north Asia (Siberia, Russian far East, North China, Mongolia, and Kazakhstan). Int. J. Coal Geol. 206, 106–120. doi:10.1016/j.coal.2018.10.013
Awadh, S. M., Al-Ankaz, Z. S., Al-Owaidi, M. R. A., and Armstrong-Altrin, J. S. (2023). Provenance and depositional setting of the Late Miocene- Pleistocene clastic sediments in the eastern Arabian Peninsula and western Iraq using rare earth elements geochemistry. Acta Geochim. 12 (09), 399–410. doi:10.1007/s11631-023-00658-y
Bau, M., Schmidt, K., Pack, A., Bendel, V., and Kraemer, D. (2018). The European Shale: an improved data set for normalisation of rare earth element and yttrium concentrations in environmental and biological samples from Europe. Appl. Geochem. 90, 142–149. doi:10.1016/j.apgeochem.2018.01.008
Blissett, R. S., Smalley, N., and Rowson, N. A. (2014). An investigation into six coal fly ashes from the United Kingdom and Poland to evaluate rare earth element content. Fuel 119 (), 236–239. doi:10.1016/j.fuel.2013.11.053
Bp, Company, (2023). BP statistical review of world energy. https://www.bp.com/en/global.
Cai, Y., Liu, D., Zhang, K., Elsworth, D., Yao, Y., and Tang, D. (2014). Preliminary evaluation of gas content of the No. 2 coal seam in the Yanchuannan area, southeast Ordos basin, China. J. Petroleum Sci. Eng. 122, 675–689. doi:10.1016/j.petrol.2014.09.010
Cantrell, K. J., and Robert, H. B. (1987). Rare earth element complexation by carbonate and oxalate ions. Geochimica Cosmochimica Acta 51 (3), 597–605. doi:10.1016/0016-7037(87)90072-X
Chen, J., Liu, G., Jiang, M., Chou, C.-L., Li, H., Wu, B., et al. (2011). Geochemistry of environmentally sensitive trace elements in Permian coals from the Huainan coalfield, Anhui, China. Int. J. Coal Geol. 88 (1), 41–54. doi:10.1016/j.coal.2011.08.002
Chowdhury, N. A., Deng, S., Jin, H., Prodius, D., Sutherland, J. W., and Nlebedim, I. C. (2021). Sustainable recycling of rare-earth elements from NdFeB magnet swarf: techno-economic and environmental perspectives. ACS Sustain. Chem. Eng. 9 (47), 15915–15924. doi:10.1021/acssuschemeng.1c05965
Commission, E. (2017). Committee and the committee of the regions on the 2017 list of critical raw materials for the EU. Brussels, Belgium: European Commission.
Crowley, S. S., Stanton, R. W., and Ryer, T. A. (1989). The effects of volcanic ash on the maceral and chemical composition of the C coal bed, Emery Coal Field, Utah. Org. Geochem. 14 (3), 315–331. doi:10.1016/0146-6380(89)90059-4
Dai, S., and Finkelman, R. B. (2018). Coal as a promising source of critical elements: progress and future prospects. Int. J. Coal Geol. 186, 155–164. doi:10.1016/j.coal.2017.06.005
Dai, S., Graham, I. T., and Ward, C. R. (2016). A review of anomalous rare earth elements and yttrium in coal. Int. J. Coal Geol. 159, 82–95. doi:10.1016/j.coal.2016.04.005
Dai, S., Li, D., Chou, C.-L., Zhao, L., Zhang, Y., Ren, D., et al. (2008). Mineralogy and geochemistry of boehmite-rich coals: new insights from the Haerwusu surface mine, jungar coalfield, inner Mongolia, China. Int. J. Coal Geol. 74 (3), 185–202. doi:10.1016/j.coal.2008.01.001
Dai, S., Li, T., Jiang, Y., Ward, C. R., Hower, J. C., Sun, J., et al. (2015). Mineralogical and geochemical compositions of the Pennsylvanian coal in the Hailiushu Mine, Daqingshan Coalfield, Inner Mongolia, China: implications of sediment-source region and acid hydrothermal solutions. Int. J. Coal Geol. 137, 92–110. doi:10.1016/j.coal.2014.11.010
Dai, S., Luo, Y., Seredin, V. V., Ward, C. R., Hower, J. C., Zhao, L., et al. (2014). Revisiting the late Permian coal from the Huayingshan, Sichuan, southwestern China: enrichment and occurrence modes of minerals and trace elements. Int. J. Coal Geol. 122, 110–128. doi:10.1016/j.coal.2013.12.016
Dai, S., Ren, D., Chou, C. L., Finkelman, R. B., Seredin, V. V., and Zhou, Y. (2012a). Geochemistry of trace elements in Chinese coals: a review of abundances, genetic types, impacts on human health, and industrial utilization. Int. J. Coal Geol. 94, 3–21. doi:10.1016/j.coal.2011.02.003
Dai, S., Ren, D., Chou, C. L., Li, S., and Jiang, Y. (2006). Mineralogy and geochemistry of the No. 6 coal (pennsylvanian) in the junger coalfield, Ordos Basin, China. Int. J. Coal Geol. 66 (4), 253–270. doi:10.1016/j.coal.2005.08.003
Dai, S., Ren, D., and Li, S. (2003). Modes of occurrence of rare earth elements in some late paleozoic coals of NorthNorth China. Acta Geosci. Sin. (3), 273–278. doi:10.3321/j.issn:1006-3021.2003.03.013
Dai, S., Wang, X., Zhou, Y., Hower, J. C., Li, D., Chen, W., et al. (2011). Chemical and mineralogical compositions of silicic, mafic, and alkali tonsteins in the late Permian coals from the Songzao Coalfield, Chongqing, Southwest China. Chem. Geol. 282 (1), 29–44. doi:10.1016/j.chemgeo.2011.01.006
Dai, S., Xie, P., Ward, C. R., Yan, X., Guo, W., French, D., et al. (2017). Anomalies of rare metals in lopingian super-high-organic-sulfur coals from the yishan coalfield, guangxi, China. Ore Geol. Rev. 88, 235–250. doi:10.1016/j.oregeorev.2017.05.007
Dai, S., Yang, J., Ward, C. R., Hower, J. C., Liu, H., Garrison, T. M., et al. (2015). Geochemical and mineralogical evidence for a coal-hosted uranium deposit in the Yili Basin, Xinjiang, northwestern China. Ore Geol. Rev. 70, 1–30. doi:10.1016/j.oregeorev.2015.03.010
Dai, S., Zhang, W., Ward, C. R., Seredin, V. V., Hower, J. C., Li, X., et al. (2013). Mineralogical and geochemical anomalies of late Permian coals from the Fusui Coalfield, Guangxi Province, southern China: influences of terrigenous materials and hydrothermal fluids. Int. J. Coal Geol. 105, 60–84. doi:10.1016/j.coal.2012.12.003
Dai, S., Zhao, L., Wei, Q., Song, X., Wang, W., Liu, J., et al. (2020). Resources of critical metals in coal-bearing sequences in China: enrichment types and distribution. Chin. Sci. Bull. 65 (33), 3715–3729. doi:10.1360/tb-2020-0112
Dai, S., Zou, J., Jiang, Y., Ward, C. R., Wang, X., Li, T., et al. (2012b). Mineralogical and geochemical compositions of the Pennsylvanian coal in the Adaohai Mine, Daqingshan Coalfield, Inner Mongolia, China: modes of occurrence and origin of diaspore, gorceixite, and ammonian illite. Int. J. Coal Geol. 94, 250–270. doi:10.1016/j.coal.2011.06.010
Dong, D., Shao, L., and Mingpei, L. I. (2017). Study on coal accumulating law of late Carboniferous-early Permian coal-bearing strata in North China Platform. Coal Sci. Technol. 52 (9), 175–181. doi:10.13199/j.cnki.cst.2017.09.029
Finkelman, R. B. (1993). “Trace and minor elements in coal,” in Organic geochemistry: principles and applications. Editors M. H. Engel, and S. A. Macko (Boston, MA, USA: Springer US).
Finkelman, R. B. (1994). Modes of occurrence of potentially hazardous elements in coal: levels of confidence. Fuel Process. Technol. 39 (1-3), 21–34. doi:10.1016/0378-3820(94)90169-4
Finkelman, R. B., Palmer, C. A., and Wang, P. (2018). Quantification of the modes of occurrence of 42 elements in coal. Int. J. Coal Geol. 185, 138–160. doi:10.1016/j.coal.2017.09.005
Fleet, A. J. (1984). “Aqueous and sedimentary geochemistry of the rare earth elements,” in Developments in geochemistry (Elsevier). Amsterdam, Netherlands.
Floback, A. E., and Moffett, J. W. (2021). Rare earth element distributions in the Arabian Sea reveal the influence of redox processes within the oxygen deficient zone. Chem. Geol. 577, 120214. doi:10.1016/j.chemgeo.2021.120214
Franus, W., Wiatros-Motyka, M. M., and Wdowin, M. (2015). Coal fly ash as a resource for rare earth elements. Environ. Sci. Pollut. Res. 22, 9464–9474. doi:10.1007/s11356-015-4111-9
Gallet, S., Jahn, B.-ming, Lanoë, B. V. V., Dia, A., and Rossello, E. (1998). Loess geochemistry and its implications for particle origin and composition of the upper continental crust. Earth Planet. Sci. Lett. 156 (3-4), 157–172. doi:10.1016/s0012-821x(97)00218-5
Ge, X. H., Ma, W. P., Liu, J., Ren, S. M., Liu, Y., Yuan, S., et al. (2009). A discussion on the tectonic framework of Chinese mainland. Geol. China 36, 949–965. doi:10.3969/j.issn.1000-3657.2009.05.001
Guo, Q., and You, W. (2023). A comprehensive evaluation of the international competitiveness of strategic minerals in China, Australia, Russia and India: the case of rare earths. Resour. Policy 85, 103821. doi:10.1016/j.resourpol.2023.103821
Hao, H., Huang, W., Hao, R., Mu, N., Wen, L., and Yu, C. (2023). Mineralogy and elemental geochemistry of pyrite coal-balls, Datong Coalfield, Shanxi Province, China: implications for differentiation mechanism. Ore Geol. Rev. 159, 105538. doi:10.1016/j.oregeorev.2023.105538
Hower, J. C., and Dai, S. (2016). Petrology and chemistry of sized Pennsylvania anthracite, with emphasis on the distribution of rare earth elements. Fuel 185 (1), 305–315. doi:10.1016/j.fuel.2016.07.055
Hower, J. C., Ruppert, L. F., and Eble, C. F. (1999). Lanthanide, yttrium, and zirconium anomalies in the Fire Clay coal bed, Eastern Kentucky. Int. J. Coal Geol. 39 (1), 141–153. doi:10.1016/S0166-5162(98)00043-3
Huang, W., Che, Y., Qi, Y., Tang, D., Zhao, Z., and Tang, X. (2004). Geochemistry of sulfur and hazardous elements in late paleozoic coals and partings from Taozao coalfield, Shandong province, China. J. China Univ. Geosciences 15 (01), 15–22.
Huang, W., Jiu, Bo, and Yuan, Li (2019). Distribution characteristics of rare earth elements in coal and its prospects on development and exploitation. J. China Coal Soc. 44 (1), 287–294. doi:10.13225/j.cnki.jccs.2018.1708
Huang, W., Qi, Y., Peng, S., and Zhao, Z. (2001). Geochemistry of permian coal and its combustion residues in huainan coalfield, China. J. China Univ. Geosciences (4), 283–290.
Huang, W., Qi, Y., Tang, D., Tang, X., and Zhao, Z. (2000). Rare earth element geochemistry of Late Palaeozoic coals in North China. Acta Geol. Sinica-English Ed. 74 (1), 74–83. doi:10.1111/j.1755-6724.2000.tb00433.x
Jane, W. N., and Arnold, M. (2018). Rare earth elements in select Main Karoo Basin (South Africa) coal and coal ash samples. Int. J. Coal Geol. 196, 82–92. doi:10.1016/j.coal.2018.06.020
Feng, J., Zhao, Z., Chen, F., and Hu, H. (2014). Rare earth elements in sinters from the geothermal waters (hot springs) on the Tibetan Plateau, China. J. Volcanol. Geotherm. Res. 287, 1–11. doi:10.1016/j.jvolgeores.2014.09.009
Jiu, B., Huang, W., Baruch, S., Ruilin, H., Mu, N., Wen, L., et al. (2023). Distribution of Li, Ga, Nb, and REEs in coal as determined by LA-ICP-MS imaging: a case study from Jungar coalfield, Ordos Basin, China. Int. J. Coal Geol. 267, 104184. doi:10.1016/j.coal.2023.104184
Jolliff, B. L., Papike, J. J., Shearer, C. K., and Shimizu, N. (1989). Inter- and intra-crystal REE variations in apatite from the bob ingersoll pegmatite, black hills, south Dakota. Geochimica Cosmochimica Acta 53 (2), 429–441. doi:10.1016/0016-7037(89)90394-3
Kang, J., Kang, Z., and Shan, H. (2014a). Abundance of rare earth elements and yttrium in coals from the guanbanwusu mine, jungar coalfield, northern China, and their geological implication. Adv. Mater. Res. 962-965, 152–155. doi:10.4028/www.scientific.net/AMR.962-965.152
Kang, J., Zhao, L., Wang, X., Song, W., Wang, P., Wang, R., et al. (2014b). Abundance and geological implication of rare earth elements and yttrium in coals from the Suhaitu Mine, Wuda Coalfield, northern China. Energy Explor. exploitation 32 (5), 873–889. doi:10.1260/0144-5987.32.5.873
Ketris, M. áP., and Yudovich, Ya E. (2009a). Estimations of Clarkes for Carbonaceous biolithes: world averages for trace element contents in black shales and coals. Int. J. coal Geol. 78 (2), 135–148. doi:10.1016/j.coal.2009.01.002
Ketris, M. P., and Yudovich, Ya E. (2009b). Estimations of Clarkes for Carbonaceous biolithes: world averages for trace element contents in black shales and coals. Int. J. Coal Geol. 78 (2), 135–148. doi:10.1016/j.coal.2009.01.002
Li, C., Liang, H., Wang, S., and Liu, J. (2018). Study of harmful trace elements and rare earth elements in the Permian tectonically deformed coals from Lugou Mine, North China Coal Basin, China. J. Geochem. Explor. 190, 10–25. doi:10.1016/j.gexplo.2018.02.016
Li, H., ying, H., Yang, L., and Liu, Y. (2008). Characteristics of bitumen trace elements and rare earth elements of coal in southeastern margin of Ordos Basin. Xinjiang Pet. Geol. (2), 159–162.
Li, Z. X., Yu, J. F., and Guo, J. B. (2002). Study on coal accumulation under marine transgression events in the epicontinental basin of North China. Coal Geol. Explor. 30 (5), 1–4.
Lin, R., Howard, B. H., Roth, E. A., Bank, T. L., Granite, E. J., and Soong, Y. (2017). Enrichment of rare earth elements from coal and coal by-products by physical separations. Fuel 200, 506–520. doi:10.1016/j.fuel.2017.03.096
Liu, D., Qi, Y., and Tang, D. (2000). Occurrence and geological genesis of pyritesin late paleozoic coals in NorthNorth China. Chin. J. Geochem. (4), 301–311.
Liu, X.-F., Zhai, S., Wang, X.-K., Liu, X., and Liu, X.-M. (2022). Rare earth element geochemistry of late cenozoic island carbonates in the south China sea. Minerals 12 (5), 578. doi:10.3390/min12050578
Loges, A., Wagner, T., Barth, M., Bau, M., Göb, S., and Markl, G. (2012). Negative Ce anomalies in Mn oxides: the role of Ce4+ mobility during water–mineral interaction. Geochimica Cosmochimica Acta 86, 296–317. doi:10.1016/j.gca.2012.03.017
Masuda, A., Kawakami, O., Dohmoto, Y., and Takenaka, T. (1987). Lanthanide tetrad effects in nature: two mutually opposite types, W and M. Geochem. J. 21 (3), 119–124. doi:10.2343/geochemj.21.119
Masuda, A., Nakamura, N., and Tanaka, T. (1973). Fine structures of mutually normalized rare-earth patterns of chondrites. Geochimica Cosmochimica Acta 37 (2), 239–248. doi:10.1016/0016-7037(73)90131-2
Ministry of Natural Resources, PRC (2023). China mineral resources: Ministry of Natural Resources of the People’s Republic of China. https://www.mnr.gov.cn/.
Monecke, T., Kempe, U., Monecke, J., Sala, M., and Wolf, D. (2002). Tetrad effect in rare earth element distribution patterns: a method of quantification with application to rock and mineral samples from granite-related rare metal deposits. Geochimica Cosmochimica Acta 66 (7), 1185–1196. doi:10.1016/S0016-7037(01)00849-3
Moroeng, O. M., Murathi, B., and Wagner, N. J. (2024). Enrichment of rare earth elements in epigenetic dolomite occurring in contact metamorphosed Witbank coals (South Africa). Int. J. Coal Geol. 282, 104405. doi:10.1016/j.coal.2023.104405
Pan, J., Zhou, C., Liu, C., Tang, M., Cao, S., Hu, T., et al. (2018). Modes of occurrence of rare earth elements in coal fly ash: a case study. Energy & Fuels 32 (9), 9738–9743. doi:10.1021/acs.energyfuels.8b02052
Piepgras, D. J., and Jacobsen, S. B. (1992). The behavior of rare earth elements in seawater: precise determination of variations in the North Pacific water column. Geochimica Cosmochimica Acta 56 (5), 1851–1862. doi:10.1016/0016-7037(92)90315-A
Ren, D., Zhao, F., Wang, Y., and Yang, S. (1999). Distributions of minor and trace elements in Chinese coals. Int. J. Coal Geol. 40 (2), 109–118. doi:10.1016/S0166-5162(98)00063-9
Rudnick, R. L. (1992). Restites, Eu anomalies and the lower continental crust. Geochimica Cosmochimica Acta 56 (3), 963–970. doi:10.1016/0016-7037(92)90040-p
Rybak, A., and Rybak, A. (2021). Characteristics of some selected methods of rare earth elements recovery from coal fly ashes. Metals 11 (1), 142. doi:10.3390/met11010142
Sami, M., Mahdy, N. M., Ntaflos, T., and Fathy, D. (2020). Composition and origin of Ti–Nb–Ta–Zr bearing minerals in the Abu Diab highly evolved granite from the Central Eastern Desert of Egypt. J. Afr. Earth Sci. 165, 103808. doi:10.1016/j.jafrearsci.2020.103808
Schulz, K. J. (2017). Critical mineral resources of the United States: economic and environmental geology and prospects for future supply. Department of the Interior: Geological Survey (U.S.). Geological Survey. United States. doi:10.3133/pp1802
Seredin, V., Dai, S., and Chekryzhov, I. (2012). “Rare metal mineralization in tuffaceous strata of the Russian and Chinese coal basins,” in Diagnostics of volcanogenic rocks in sedimentary strata. Editor Ya.E. Yudovich (Edmonton, Canada: Geoprint).
Seredin, V. V. (1996). Rare earth element-bearing coals from the Russian Far East deposits. Int. J. Coal Geol. 30 (1), 101–129. doi:10.1016/0166-5162(95)00039-9
Seredin, V. V. (2012). From coal science to metal production and environmental protection: a new story of success. Int. J. Coal Geol. 90, 1–3. doi:10.1016/j.coal.2011.11.006
Seredin, V. V., and Dai, S. (2012). Coal deposits as potential alternative sources for lanthanides and yttrium. Int. J. Coal Geol. 94, 67–93. doi:10.1016/j.coal.2011.11.001
Seredin, V. V., Dai, S., Sun, Y., and Chekryzhov, I.Yu. (2013). Coal deposits as promising sources of rare metals for alternative power and energy-efficient technologies. Appl. Geochem. 31, 1–11. doi:10.1016/j.apgeochem.2013.01.009
Seredin, V. V., and Finkelman, R. B. (2008). Metalliferous coals: a review of the main genetic and geochemical types. Int. J. Coal Geol. 76 (4), 253–289. doi:10.1016/j.coal.2008.07.016
Shi, S., Liu, Q., Sun, J., Wu, Z., and Sun, B. (2014). Enrichment features and causes of boehmite in high-alumina partings of Junger coalfield. Coal Eng. 46 (5), 116–118. doi:10.11799/ce201405038
Shu, X., Meng, F., Tao, R., and Wang, K. (2023). Mantle metasomatism and REE enrichment in the genetic source of the dalucao carbonatite complex (sichuan, China): insights from elemental geochemistry and in-situ Sr isotopes of two calcite types. Lithosphere 2023 (1). doi:10.2113/2023/lithosphere_2023_122
Song, Y., Ma, X., Liu, S., Jiang, L., Hong, F., and Yi, Q. (2018). Accumulation conditions and key technologies for exploration and development of Qinshui coalbed methane field. Petroleum Res. 3 (4), 320–335. doi:10.1016/j.ptlrs.2018.11.002
Sun, Y., Zhao, C., Li, Y., and Wang, J. (2014). Minimum mining grade of the selected trace elements in Chinese coal. J. China Coal Soc. 39 (04), 744–748. doi:10.13225/j.cnki.jccs.2013.1718
Sutcu, E. C., Şentürk, S., Kapıcı, K., and Gökçe, N. (2021). Mineral and rare earth element distribution in the Tunçbilek coal seam, Kütahya, Turkey. Int. J. Coal Geol. 245, 103820. doi:10.1016/j.coal.2021.103820
Taggart, R. K., Hower, J. C., and Heileen, H. K. (2018). Effects of roasting additives and leaching parameters on the extraction of rare earth elements from coal fly ash. Int. J. Coal Geol. 196, 106–114. doi:10.1016/j.coal.2018.06.021
Tanfu, Li, Jiao, W., Zhou, C., Liu, G., Luo, J., and Liu, M. (2015). Gangue in main mineable coal seams and resources utilization research in huainan mining area. Coal Geol. China 27 (08), 53–56.
Tatsumi, Y., Hamilton, D. L., and Nesbitt, R. W. (1986). Chemical characteristics of fluid phase released from a subducted lithosphere and origin of arc magmas: evidence from high-pressure experiments and natural rocks. J. Volcanol. Geotherm. Res. 29 (1-4), 293–309. doi:10.1016/0377-0273(86)90049-1
Taylor, S. R., and McLennan, S. M. (1985). The continental crust: its composition and evolution. An examination of the geochemical record preserved in sedimentary rocks. Blackwell Scientific Pub. Hoboken, NY, USA
Taylor, S. R., and McLennan, S. M. (1995). The geochemical evolution of the continental crust. Rev. Geophys. 33 (2), 241–265. doi:10.1029/95rg00262
Teng, J., Qiu, L., Ma, C., Liu, H., Fang, Z., and Yu, J. (2023). Rare earth partition characteristics and sedimentary diagenetic response in layered argillaceous limestone: taking the shale of upper Es4 in the Nx55 well area as an example. Geofluids 2023, 1–15. doi:10.1155/2023/2248940
Wang, J., Guo, M., Liu, M., and Xinqiang, W. (2020). Long-term outlook for global rare earth production. Resour. Policy 65, 101569. doi:10.1016/j.resourpol.2019.101569
Wang, W., Qin, Y., Sang, S., Zhu, Y., Wang, C., and Weiss, D. J. (2008). Geochemistry of rare earth elements in a marine influenced coal and its organic solvent extracts from the Antaibao mining district, Shanxi, China. Int. J. Coal Geol. 76 (4), 309–317. doi:10.1016/j.coal.2008.08.012
Wang, Y. (2012). Water bursting mechanism analysis and water disaster control in liyazhuang Coalmine,Huozhou mining area. Coal Geol. China 24 (01), 29–31+40.
Wang, Z., Liu, C., Xu, Z., Han, G., and Zhu, J. (2000). Advances in research on geochemistry of rare earth elements in rivers. Adv. Earth Sci. 15 (5), 553. doi:10.11867/j.issn.1001-8166.2000.05.0553
Weng, Z., Jowitt, S. M., Mudd, G. M., and Haque, N. (2015). A detailed assessment of global rare earth element resources: opportunities and challenges. Econ. Geol. 110 (8), 1925–1952. doi:10.2113/econgeo.110.8.1925
Yao, Y., Liu, D., Tang, D., Tang, S., Che, Y., and Huang, W. (2009). Preliminary evaluation of the coalbed methane production potential and its geological controls in the Weibei Coalfield, Southeastern Ordos Basin, China. Int. J. Coal Geol. 78 (1), 1–15. doi:10.1016/j.coal.2008.09.011
Yuan, Y., Cao, D., Lin, Z., and Liu, K. (2011). Study on structural coal-control characteristics in Datong coalfield. Coal Geol. China 23 (08), 63–65+77. doi:10.3969/j.issn.1674-1803.2011.08.15
Zaharescu, D. G., Burghelea, C. I., Dontsova, K., Presler, J. K., Maier, R. M., Huxman, T., et al. (2017). Ecosystem composition controls the fate of rare earth elements during incipient soil genesis. Sci. Rep. 7 (1), 43208. doi:10.1038/srep43208
Zhang, B., Cheng, W., Zhang, Q., Li, Y., Sun, P., and Fathy, D. (2022). Occurrence patterns and enrichment influencing factors of trace elements in paleogene coal in the fushun basin, China. ACS Earth Space Chem. 6 (12), 3031–3042. doi:10.1021/acsearthspacechem.2c00263
Zhao, Z., Tang, X., and Li, B. (2000). Geochemistry of rare-earth elements of coal in Huainan mining area. Acta Sedimentol. Sin. 18 (3), 453–459. doi:10.14027/j.cnki.cjxb.2000.03.022
Zhao, Z., Wang, H., and Zhao, Z. (2021). Fractionation and provenances of rare earth elements in coastal sediments in tropical China. J. Coast. Conservation 25 (1), 13. doi:10.1007/s11852-021-00803-w
Keywords: North China, coal, rare earths, enrichment, characteristics
Citation: Wen L, Huang W, Zhang Y and Jiu B (2024) Geochemical characteristics of rare earth elements in late Palaeozoic coals from North China. Front. Earth Sci. 12:1374780. doi: 10.3389/feart.2024.1374780
Received: 22 January 2024; Accepted: 18 March 2024;
Published: 03 April 2024.
Edited by:
Liliana Lefticariu, Southern Illinois University Carbondale, United StatesReviewed by:
Mabrouk Sami, United Arab Emirates University, United Arab EmiratesŁukasz Kruszewski, Polish Academy of Sciences, Poland
Copyright © 2024 Wen, Huang, Zhang and Jiu. This is an open-access article distributed under the terms of the Creative Commons Attribution License (CC BY). The use, distribution or reproduction in other forums is permitted, provided the original author(s) and the copyright owner(s) are credited and that the original publication in this journal is cited, in accordance with accepted academic practice. No use, distribution or reproduction is permitted which does not comply with these terms.
*Correspondence: Yuanfu Zhang, zhangyuanfu@vip.163.com; Wenhui Huang, huangwh@cugb.edu.cn