- School of Geographic Sciences, Institute of Disaster Risk Management, Nanjing University of Information Science and Technology, Nanjing, China
Drought, being one of the most devastating natural disasters, has a far-reaching impact. In the context of global warming, it becomes crucial to quantitatively and scientifically assess the effects of anthropogenic climate change on meteorological drought in China. This assessment not only enhances our understanding of anthropogenic climate change but also aids in formulating more effective strategies for managing the risks associated with meteorological drought. This study employs the standardized precipitation evapotranspiration index (SPEI) to compute drought events by utilizing both observational data and counterfactual climate data (i.e., detrended observations). Subsequently, it analyzes the impact of anthropogenic climate change on the characteristics of drought, including frequency, intensity, duration, and affected area, in China as well as nine major river basins from 1960 to 2019. The outcomes of the analysis reveal that based on observational data, there is a discernible upward trend in the frequency, intensity, duration, and affected area of meteorological droughts in China. Notably, the regions experiencing an increase in frequency, intensity, and duration are primarily situated in the northeastern part of the Northwestern Rivers basin, the central and western parts of the Yellow River basin, the central and northern parts of the Yangtze River basin, the western part of the Southeastern River basins, and the eastern part of the Pearl River basin. Conversely, when considering a counterfactual climate scenario, the frequency and intensity of meteorological droughts in China demonstrate an upward trend, while the duration and affected area exhibit a downward trend. The impact of anthropogenic climate change on China has been evident in the increased frequency, intensity, duration, and affected area of droughts. Specifically, regions located in the northeastern parts of Northwest River basins, the southern part of the Songliao River basin, the northern part of the Haihe River basin, the central-northern part of the Yangtze River basin, the eastern part of the Pearl River basin, and the western part of the Southwest River basins have experienced amplified levels of drought. Anthropogenic climate change is highlighted as the primary factor influencing the observed drought characteristics changes in China, with contribution rates of 84.67%, 75.25%, 190.32%, and 133.99% for changes in the increased drought frequency, intensity, duration, and affected area, respectively. These changes have significant implications for water resource management and agricultural practices in the affected regions.
1 Introduction
In 2023, the average global temperature has increased by 1.45°C ± 0.12°C compared to pre-industrial levels (1850–1900) (WMO, 2021). This rise in temperature has resulted in a surge in extreme events, particularly droughts, which are now more frequent and severe. Droughts have wide-ranging impacts on industrial production, the ecological environment, and socioeconomic aspects, drawing significant attention. Despite only constituting 6% of extreme events between 1970 and 2019, droughts were responsible for 34% of related deaths and 7% of economic losses (WMO, 2021). As global temperatures continue to climb, regions in Africa, Oceania, Asia, Europe, and North America are projected to experience an increase in the frequency and severity of droughts, with more areas seeing rises rather than declines (IPCC, 2012; Chen et al., 2022; Jiang and Wang, 2021; Zhao et al., 2023b). Anthropogenic (e.g., human-induced) activities have been identified as the main driver behind the increase in global temperatures, as acknowledged by the Intergovernmental Panel on Climate Change (IPCC, 2012) and Su et al. (2022). Consequently, it becomes crucial to investigate the repercussions of anthropogenic climate change on the development of regional drought patterns. Such exploration can enhance our comprehension of the influence exerted by human actions on drought characteristics, thereby furnishing a scientific foundation for implementing adaptive strategies in response to climate change.
The research conducted on drought primarily concentrates on examining the spatiotemporal distribution patterns, causes, impact mechanisms, risks, and other relevant aspects (Zhai et al., 2010; Liu and Chen, 2021; Su et al., 2021). However, there has been a relatively lesser emphasis on conducting attribution studies of drought. The current research focus is centered around differentiating between natural forcing and anthropogenic forcing, with the primary utilization of data provided by the Coupled Model Intercomparison Project (CMIP). CMIP data, which is derived from Global Climate Models (GCMs), involves inputting initial states of various climate variables into physical models to obtain climate data under both natural and all-forcing conditions. The utilization of CMIP5 or CMIP6 data in attribution studies of drought has allowed scholars to detect and quantify changes caused by external forcings. Through this approach, researchers have been able to identify and quantify the impacts of external forcings, thereby discerning the relative contributions of anthropogenic factors. Previous studies conducted by Gudmundsson and Seneviratne (2016) and Zhang et al. (2022) have already employed these datasets to investigate the attribution of drought. Their findings indicate that increased human emissions have led to a higher frequency of drought and intensified evapotranspiration, resulting in a more pronounced trend towards aridification. This trend is particularly evident in regions such as northeastern South America and West Africa, as highlighted by the IPCC (2012). The studies mentioned, including those by Eyring et al. (2016) and Gillett et al. (2016), have contributed to our understanding of the impacts of anthropogenic factors on drought patterns. Anthropogenic warming has resulted in more frequent heat droughts in China, as highlighted by Chen and Sun (2017). This phenomenon is believed to be a significant contributing factor to the rise in summer heat drought events in northeastern China, as indicated by Li et al. (2020). However, the data obtained from GCMs have limited resolution and are insufficient for accurately assessing the impacts of climate change on regional-scale droughts. To overcome this limitation, downscaling and bias correction techniques are necessary to obtain localized climate change information. It is important to note that the process of bias correction may introduce additional uncertainties to the models, as discussed by Huang et al. (2018), Su et al. (2016), and Wu et al. (2022). In this regard, the Inter-Sectoral Impact Model Intercomparison Project (ISIMIP) refrains from utilizing GCM data in its attribution studies. Instead, it employs statistical models that are constructed using long time series observational data to generate counterfactual climate data resembling natural forcing conditions through detrending techniques (Gelfan et al., 2023; Yang and Huang, 2023; Wen et al., 2024). Counterfactual climate data by detrending observational data is known for its straightforward computation, which accurately reflects the climate system’s response, and is not bound by the simulation time of climate models. Nonetheless, there has been a scarcity of research that leverages this data to investigate the effects of anthropogenic climate change on meteorological drought (Mengel et al., 2021).
This study is grounded on observational data spanning from 1960 to 2019 and employs detrending techniques to derive counterfactual climate data. By utilizing the Standardized Precipitation Evapotranspiration Index (SPEI), it examines the attributes of drought frequency, intensity, duration, and affected area. Through approaches like contribution analysis, it delves into the degree to which human-induced climate change influences the observed alterations in drought characteristics. The primary objective of this investigation is to establish a scientific foundation for comprehending the repercussions of human-induced climate change and suggesting adaptive strategies to alleviate disaster risks. The article is structured into three key sections: observed changes in drought characteristics, changes in drought characteristics in counterfactual scenario, and the influence of human-induced climate change.
2 Methods
2.1 Study area
China, situated in eastern Asia, occupies an expansive region between 3°52′ and 53°33′N latitude and 73°40′ to 135°2′E longitude (Figure 1). With a total land area of approximately 9.6 million square kilometers, the country showcases a diverse topography encompassing plateaus, plains, mountains, hills, basins, and various other geographical formations. Notably, there exists a significant variation in winter temperatures from north to south, with the southern regions experiencing relatively warmer climates while temperatures gradually decrease towards the northern regions. Conversely, summers are generally characterized by hot weather, although the Qinghai-Tibet Plateau region maintains lower temperatures throughout the year (Liu et al., 2020). The distribution of precipitation exhibits variations in both space and time, with a general decrease observed from the southeast to the northwest (Du et al., 2023). This pattern is particularly evident in China, where different climate types, including temperate monsoon climate, tropical monsoon climate, and plateau mountain climate, contribute to the overall precipitation patterns. To analyze these patterns, the researchers subdivided China into nine hydrological basins: the Songliao River basin, the Haihe River basin, the Yellow River basin, the Huaihe River basin, the Yangtze River basin, the Pearl River basin, the Southeastern River basins, the Southwestern Rivers basin, and the Northwest Rivers River basin. It is important to note that the latter three basins are composed of several smaller river basins.
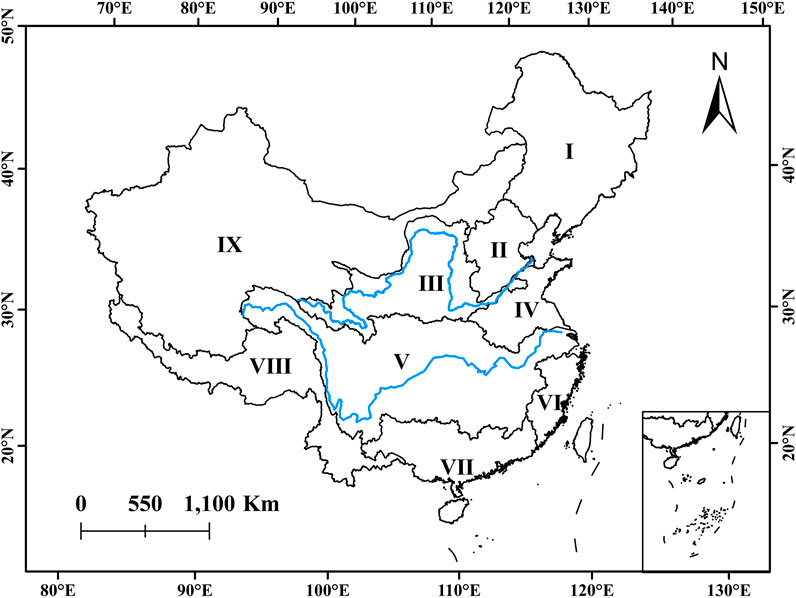
Figure 1. Spatial distribution of the nine major river basins in China (Ⅰ Songliao River basin, Ⅱ Haihe River basin, Ⅲ Yellow River basin, Ⅳ Huaihe River basin, Ⅴ Yangtze River basin, Ⅵ Southeast River basins, Ⅶ Pearl River basin, Ⅷ Southwest River basins, Ⅸ Northwest River basins).
2.2 Methodological approach
2.2.1 Observed meteorological data
The GSWP3 (Global Soil Wetness Comparison Project)—W5E5 dataset (Kim, 2017; Lange et al., 2021) provides observations on various climatic variables such as maximum temperature, minimum temperature, precipitation, 2 m (m) wind speed, relative humidity, and solar radiation. These observations cover a time period from 1901 to 2019, with a temporal resolution of days and a spatial resolution of 0.5°. It is important to note that these observations are influenced by both anthropogenic and natural factors, indicating a combination of human activities and natural processes shaping the climate.
The GSWP3-W5E5 dataset encompasses the third phase of the GSWP3 and W5E5, comprising a diverse array of meteorological elements. GSWP3 is an enhanced and corrected version of the 20th Century Reanalysis (20CR) data, which has been downscaled and adjusted for biases (Compo et al., 2011). This downscaled and bias-corrected iteration of the 20CR is further described by Compo et al. (2011). The spatial resolution of GSWP3 is 0.5°, and it spans from 1901 to 2010, as indicated by Kim (2017). On the other hand, the W5E5 dataset is derived by interpolating the (fifth generation ECMWF atmospheric reanalysis of the global climate) ERA5 dataset to a resolution of 0.5° and undergoing bias correction, covering the time period from 1979 to 2019.
When comparing GSWP3 and W5E5, it becomes evident that W5E5 incorporates a greater number of observational datasets for bias correction, resulting in a more accurate reflection of reality starting from 1979. However, it is important to acknowledge that W5E5 is limited to the time frame of 1979–2019. To address this constraint, the GSWP3-W5E5 climate dataset was created by merging the two datasets using quantile mapping (Lange, 2019).
2.2.2 Counterfactual meteorological data
Counterfactual climate data is a hypothetical climate data, which removes the influence of temperature rises on climate variables by detrending method. It consists of several variables including maximum temperature, minimum temperature, precipitation, 2 m wind speed, relative humidity, and solar radiation. These data were obtained by detrending the GSWP3-W5E5 dataset, which covers the time period from 1901 to 2019 and has a spatial resolution of 0.5°. For the purpose of this paper, the study period selected is from 1960 to 2019.
Diverging from conventional methods that solely eliminate linear trends, the detrending technique employed in this dataset incorporates the connection between global mean temperature (GMT) changes and other climate variables (such as precipitation, wind speed, etc.), acknowledging their potential functional relationship. The evaluation factor utilized in this approach is the alteration in global mean temperature (T). Initially, Singular Spectrum Analysis (SSA) is implemented to smoothen the GMT, utilizing a 10-year smoothing window, resulting in the predictor variable (T). This step is crucial in preserving the natural climate variability on shorter time scales, including those influenced by El Niño-Southern Oscillation (ENSO). Subsequently, appropriate parameter values are selected by considering the attributes of diverse climate variables. A probability density function
The overwhelming majority of global warming experienced from 1850 to the present can be attributed to anthropogenic climate change, leaving natural factors and internal climate variability with a negligible role in driving climate change over the past century. In other words, the impact of volcanic eruptions and solar activity on warming since the industrialization era has been almost insignificant (IPCC, 2012). Consequently, this paper defines the disparity between observed and counterfactual climate data as the impact of anthropogenic climate change.
2.3 Data analytical strategy
2.3.1 SPEI
The severity of drought can be effectively assessed and compared using the drought index, which serves as a characteristic measure. The SPEI takes into account both precipitation and temperature variables, acknowledging the significant influence of temperature on drought development. Furthermore, SPEI exhibits the ability to analyze multiple time scales, enabling the characterization of the probability of the disparity between precipitation (P) and evapotranspiration (PET) within a specific time frame.
SPEI encompasses various time scales, with the commonly utilized ones being SPEI-1, 3, and 12, representing monthly, seasonal, and annual droughts, respectively. Previous research has demonstrated that the 12-month time scale SPEI is more effective in capturing the patterns of drought and flooding over a longer duration (Huang et al., 2018; Su et al., 2021). Consequently, this study opts for the 12-month time scale SPEI to depict the characteristics of annual-scale drought changes.
The calculation procedure is outlined as follows:
1) PET can be determined by applying the Penman-Monteith equation;
2) The time series D(t) can be computed by subtracting the precipitation P from the potential evapotranspiration PET;
3) Assuming that D(t) follows a log-logistic probability distribution, the parameters can be estimated to identify the most suitable fit. The probability distribution function of D(t) is then calculated based on the fitted distribution;
4) To obtain the Standardized Precipitation Evapotranspiration Index (SPEI), the probability values obtained in the previous step are transformed to the standard normal distribution through standard normalization.
2.3.2 Identification of drought characteristics
A drought occurrence was detected when the SPEI indicated a value lower than −1 and persisted for a minimum of 2 months. The primary aspects of drought investigated encompass frequency, duration, intensity, and area ratio, which are expounded upon as follows:
1) Drought frequency refers to the overall count of drought events that satisfy the criteria for being classified as a drought event;
2) Drought duration signifies the number of months between the commencement and conclusion of a drought event, during which the SPEI remains below a specified threshold value (∂);
3) Drought intensity is determined by calculating the ratio between the absolute value of the cumulative SPEI value throughout a drought episode and the duration of the drought in the calendar time. This measure provides insight into the average water scarcity conditions experienced in the study area during a drought episode;
4) Drought-affected area is quantified as the proportion of the total study area that experiences drought at a given time. It reflects the extent to which the drought impacts the geographical coverage of the study area.
In order to investigate the potential influence of human-induced climate change on the characteristics of drought, the study period from 1960 to 2019 (a total of 60 years) is divided into two equal climate states: one before 1990 and the other after 1990. Each of these periods spans 30 years. The researchers calculate the drought frequency, intensity, duration, and affected area separately for the periods 1960–1989 and 1990–2019 in China, considering both the observed and counterfactual climate backgrounds. By comparing the drought characteristics of these two time periods, the study aims to identify the observed changes in drought characteristics as well as the changes that would have occurred under a counterfactual climate scenario.
2.3.3 Contribution rate formula
The impact of anthropogenic climate change on the observed changes in drought characteristics, as measured by the Contribution Rate (CR). The formula for calculating the CR is as follows (Eq. 1):
where CR quantifies the extent to observed changes in drought characteristics can be ascribed to human-induced climate change. AObs represents the observed changes in drought characteristics, while ACounter signifies the changes in drought characteristics that would occur under counterfactual climate conditions. A CR exceeding 50% signifies the predominant influence of anthropogenic climate change.
3 Results
3.1 Observed spatial and temporal characteristics of meteorological drought in China
China witnessed a frequency of meteorological droughts of approximately 6.50 between 1960 and 1989. However, from 1990 to 2019, there was a notable increase in the frequency of droughts in China, reaching around 6.64. This rise in drought frequency amounted to 0.14 when compared to the period of 1960–1989, as indicated in Figure 2A.
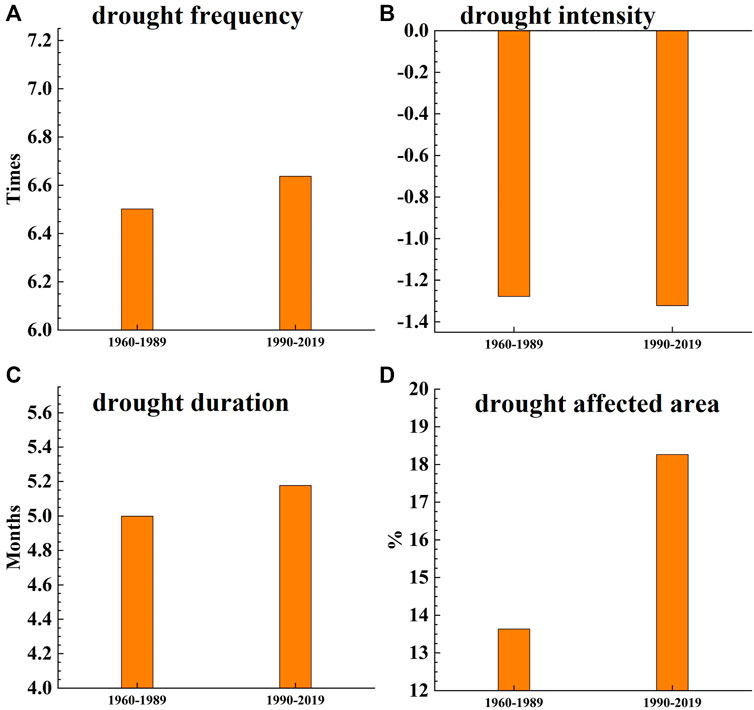
Figure 2. Frequency (A), intensity (B), duration (C) and affected area (D) of drought in China from 1960–1989 and 1990–2019 based on the observatory meteorological data.
Spatially, there has been an increase in the frequency of droughts in several river basins in China. These include the Northwest River basins, Yangtze River basin, Southeast River basins, and Pearl River basin, as well as the east-central part of the Northwest River basins. These areas collectively cover approximately 54.87% of the country’s total area. On average, the frequency of droughts has increased by 4.85 occurrences per 30 years (or 1.62 occurrences per 10 years).
Conversely, there has been a decrease in drought frequency in certain regions. This includes the northern, central, and parts of the southern areas of the Northwest River basins, the central part of the Southwest River basins, the western part of the Yangtze River basin, and the northern part of the Huaihe River basin, eastern Yellow River basin, southern Haihe River basin, and part of the western Songliao River basin. In these regions, the frequency of droughts has decreased on average by 4.58 occurrences per 30 years (or 1.53 occurrences per 10 years) (Figure 3A).
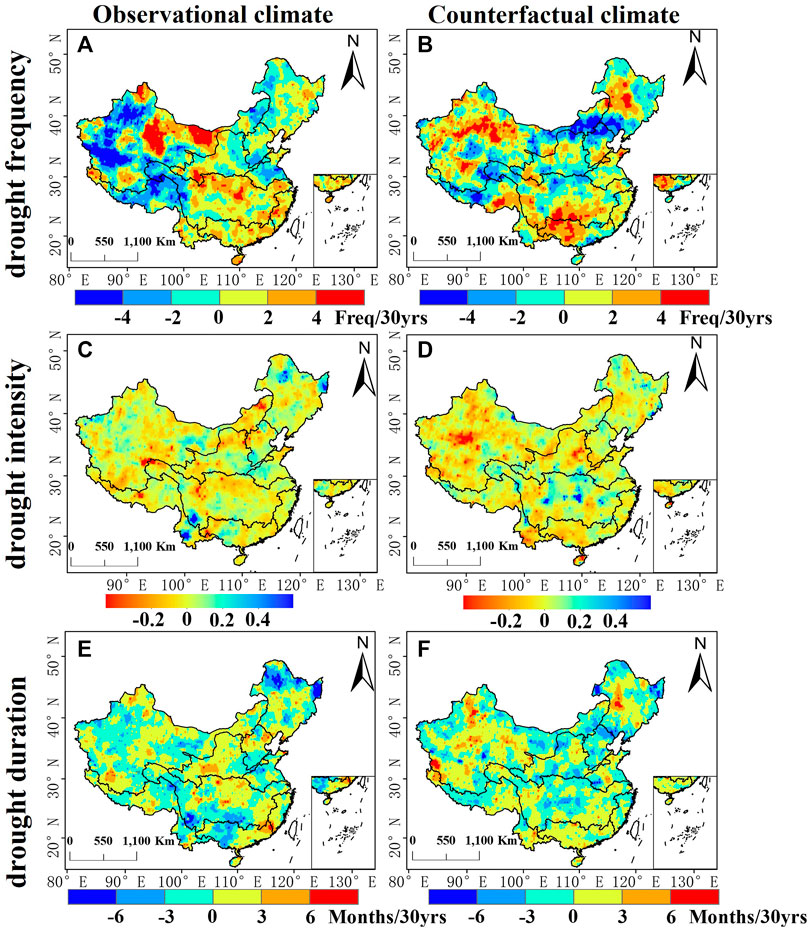
Figure 3. Relative changes in drought frequency (A,B), intensity (C,D), and duration (E,F) for 1990–2019 versus 1960–1989.
Furthermore, the intensity of meteorological droughts in China was approximately −1.27 (SPEI value) from 1960 to 1989. However, in the subsequent period of 1990–2019, the intensity of droughts increased to about −1.32. This indicates a slight enhancement in the intensity of droughts by 0.05 compared to the earlier period of 1960–1989 (Figure 2B).
Spatial differentiation is evident in the changes observed in drought intensity. Specifically, there are regions within the Songliao River basin, Haihe River basin, Yellow River basin, Huaihe River basin, Yangtze River basin, Southeast River basins, and Northwest River basins that experience a decrease in drought intensity. These regions cover approximately 43.54% of the country’s area and exhibit an average decrease of 0.09/30 years (0.03/10 years). However, the Northwest River basins shows an increase in drought intensity.
Increased drought intensity is predominantly observed in specific regions of China. These regions include the northeast of the Northwest River basins, the junction of the north-central Yellow River basin and the northwest of the Haihe River basin, the northern part of the Yangtze River basin, the central and southern parts of the Northwest River basins, and the northwest of the Southwest River basins. These areas account for approximately 56.46% of the country’s total land area. Over a span of 30 years, there has been an average increase of 0.24/30 years (0.08/10 years) in drought intensity in these regions (Figure 3C).
From 1960 to 1989, China experienced a drought that lasted approximately 4.99 months. However, in the period between 1990 and 2019, the duration of drought increased to around 5.17 months, indicating an average rise of 0.17 months compared to the previous period (Figure 2C). This increase in drought duration was observed in an area that accounted for 53.62% of the national territory. Moreover, the average increase in drought duration over a 30-year period was 2.67 months, or 0.89 months over a 10-year period. The majority of this increase was concentrated in the eastern region of China. Conversely, the western region experienced a decrease in drought duration, particularly in the northwestern and southwestern river basins. Notably, the most significant decrease in drought duration occurred in the northeastern part of the Songliao River basin (Figure 3E).
During the period spanning 1960 to 1989, the average annual area affected by meteorological drought in China was recorded at 13.63%. However, in the subsequent years from 1990 to 2019, this value increased to 18.27%. Notably, there was a reduction of 4.64% in the average area impacted by drought during the period of 1990–2019 when compared to 1960–1989, as indicated in Figure 2D.
3.2 Spatiotemporal characteristics of meteorological drought in China under counterfactual climate
During the period spanning from 1990 to 2019, China experienced a notable rise in the occurrence of meteorological droughts. Specifically, the frequency of these droughts increased from 6.35 (observed during 1960–1989) to 6.38. On average, this frequency witnessed a slight increment of 0.03 throughout this time frame, as depicted in Figure 4A.
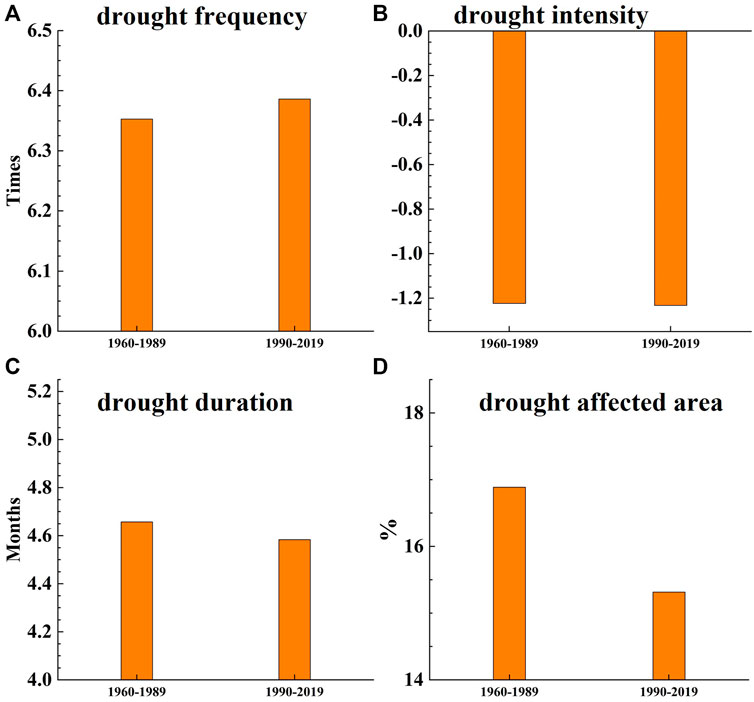
Figure 4. Frequency (A), intensity (B), duration (C) and affected area (D) of drought in China in 1960–1989 and 1990–2019 based on the counterfactual climate.
The central and southern parts of the Northwest River basins, the central and western parts of the Songliao River basin, the southern part of the Yangtze River basin, and the western part of the Pearl River basin have witnessed the most significant increase in drought frequency. On average, there has been a rise of 1.62 occurrences per 30 years (or 0.54 occurrences per 10 years) in these regions, constituting approximately 53.85% of the entire country. Conversely, there are also areas where the frequency of droughts has decreased. These regions are primarily located in the southern part of the Songliao River basin, the northeastern part of the Northwest River basins, and the northern part of the Haihe River basin at their intersection (Figure 3B).
The study revealed that there was not a significant change in drought intensity, with values ranging from −1.22 to −1.23. On average, there was a marginal increase of 0.01 (Figure 4B). However, when examining the spatial distribution of drought intensity changes, notable heterogeneity was observed. The central and northern regions of the Northwest River basins, the north-central part of the Yellow River basin, and the southern area of the Southeast River basins experienced an intensified drought, with an average increase of 0.11/30 years (0.04/10 years). These regions accounted for a substantial portion, 55.68%, of the entire country. Conversely, certain areas exhibited a decrease in drought intensity, particularly in the western, north-central, and eastern parts of the Yangtze River basin, the northern section of the Songliao River basin, the Haihe River basin, and the eastern part of the Northwest River basins (Figure 3D).
In the period of 1990–2019, the duration of droughts experienced a slight decrease compared to the years between 1960 and 1989. Specifically, the drought calendar time decreased from an average of 4.65 months–4.58 months, resulting in a decrease of 0.07 months on average (Figure 4C). The regions where the duration of droughts increased were primarily situated in the central part of the Songliao River basin, as well as the central, northern, and southwestern areas of the Northwest River basins. Conversely, the regions where the duration of droughts decreased were mainly found in the northern and southern parts of the Songliao River basin, the Haihe River basin, the northwestern part of the Northwest River basins, the south-central part of the Yellow River basin, the central part of the Yangtze River basin, and the western part of the Southeast River basins. On average, these regions experienced a decrease of 3.24 months over a 30-year period (equivalent to a decrease of 1.08 months per decade), accounting for approximately 56.56% of the country (Figure 3F). Furthermore, the area affected by droughts witnessed a decline from 16.89% during the period of 1960–1989 to 15.32%, indicating a reduction of approximately 1.57% (Figure 4D).
3.3 Impact of anthropogenic climate change on meteorological drought in China
Based on the results of observed and counterfactual meteorological drought, the impact of anthropogenic climate on droughts in China are explored. Compared to the time span spanning from 1960 to 1989, the occurrence of droughts in China has witnessed a rise of 0.10 during the period from 1990 to 2019, primarily due to anthropogenic climate change (Figure 5A). In terms of spatial distribution, this phenomenon has led to an increase in drought frequency in approximately 52.85% of China’s regions. These regions are predominantly situated in the northern sections of various river basins in the northwest, central, and eastern parts of the country. Additionally, the eastern and southern regions of the Songliao River basin, the northern part of the Haihe River basin, and the central and northern parts of the Yangtze River basin have also experienced an escalation in drought occurrences (Figure 6A).
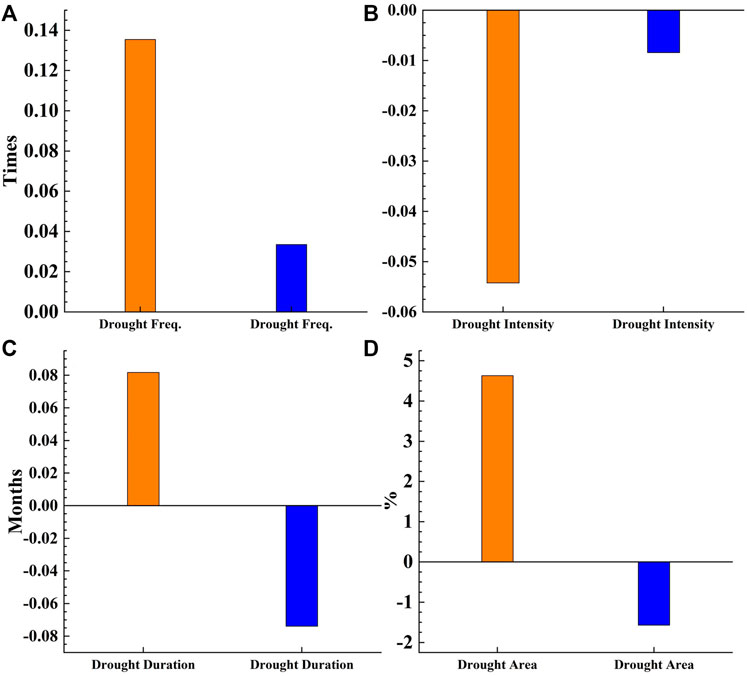
Figure 5. Compared to 1960–1989, relative changes in drought frequency (A), intensity (B), duration (C), and affected area (D) in China under observed (orange) and counterfactual climate (blue) in 1990–2019.
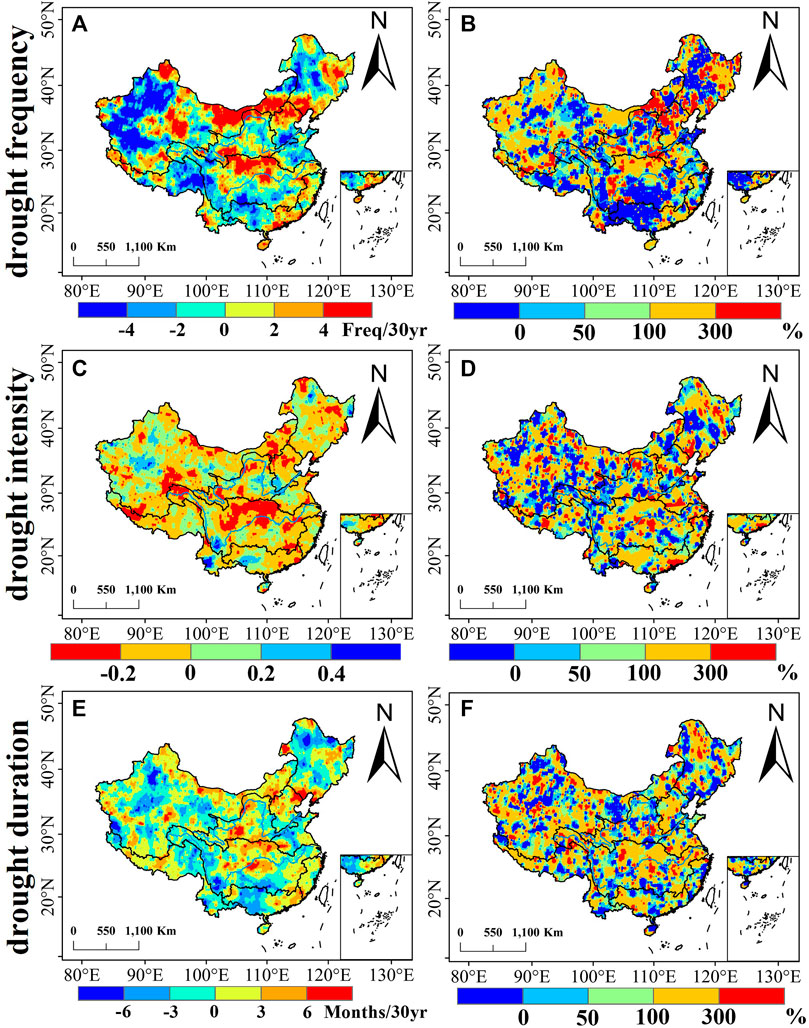
Figure 6. The relative changes in drought frequency (A), intensity (C), and duration (E) under anthropogenic climate change, as well as the CR of anthropogenic climate change to the observed changes in drought frequency (B), intensity (D), and duration (F) in China.
The impact of anthropogenic climate change has led to a 0.05 increase in observed drought intensity from 1990 to 2019 (Figure 5B). Changes in drought intensity are indicative of negative alterations, with an increase signifying heightened intensity and a decrease suggesting the opposite. Geographically, regions affected by increased drought intensity due to anthropogenic climate change are primarily concentrated in the southern, central, and northeastern parts of Northwest River basins, the central portion of the Songliao River basin, the northern area of the Haihe River basin, the central and northern sectors of the Yellow River basin, most parts of the Yangtze River basin, the southern regions of Southeast River basins, and the western areas of Southwest River basins. These specific locations encompass around 61.87% of China’s total land area (Figure 6C).
The increase in observed drought duration by 0.15 months from 1990 to 2019, as compared to the period from 1960 to 2019, is attributed to anthropogenic climate change (Figure 5C). The regions affected by anthropogenic climate change, resulting in prolonged drought duration, encompass roughly 52.90% of China’s total land area. Among these regions, those experiencing a rise in drought duration ranging from 0 to 3 months are more prevalent, while areas with a drought duration increase exceeding 6 months are scattered sporadically in the northeastern parts of various river basins in the northwest, the southern part of the Songliao River basin, the northern part of the Haihe River basin, the southwestern part of the Yellow River basin, and the Yangtze River basin (Figure 6E). Anthropogenic climate change plays a substantial role in the expansion of drought-affected areas, accounting for a 6.20% increase (Figure 5D).
Figures 6B, D, F depict the impact of anthropogenic climate change on drought characteristics. These visual representations suggest that anthropogenic climate change has a positive influence on the frequency, intensity, and duration of droughts in most regions of China, with human-induced factors being the primary drivers of these changes. However, there are variations in spatial patterns. In terms of drought frequency, it can be inferred that human-induced climate change accounts for 84.67% of the observed changes, with more than half (64.43%) of the country’s regions experiencing a CR exceeding 50%. Notable regions where anthropogenic climate change plays a dominant role include the southwestern, western, northern, and northeastern parts of the Northwest River basins, the eastern and southern parts of the Songliao River basin, the Haihe River basin, the southwestern part of the Huaihe River basin, the central-northern part of the Yangtze River basin, and the eastern part of the Pearl River basin, among others.
The impact of human-induced climate change on drought intensity in China is significant, accounting for 75.25% of the observed changes. A substantial majority of the country’s regions, approximately 70.05%, have experienced a drought severity exceeding a critical ratio of 50%. However, it is important to highlight that in specific areas such as the Northwest River basin, Songliao River basin, Yangtze River basin, and Yellow River basin, factors other than anthropogenic climate change play a more prominent role in altering drought intensity. In terms of drought duration, approximately 66.60% of the country’s regions have witnessed an increase in duration surpassing the critical ratio of 50%. Anthropogenic climate change is particularly influential in areas like the Haihe River basin, Huaihe River basin, Yangtze River basin, and Pearl River basin. Notably, the CR of anthropogenic climate change to the observed changes in the affected area of drought is remarkably high, reaching 133.99% (Figure 7).
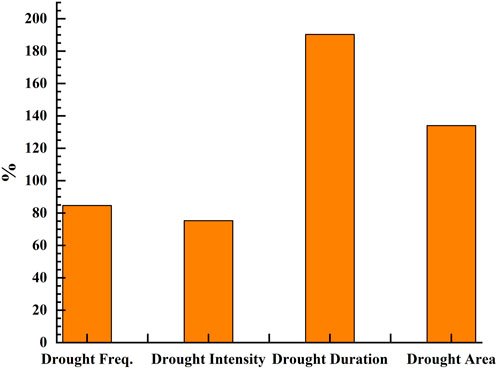
Figure 7. Contribution of anthropogenic climate change to the observed changes in frequency, intensity, duration and affected area for meteorological drought in China.
4 Discussion
This study utilized observational data and counterfactual climate data, in conjunction with the SPEI, to extract the distinctive features of meteorological droughts under both observed and natural climate change conditions. Subsequently, an analysis was conducted to evaluate the impact of anthropogenic climate change on these meteorological drought characteristics. The results demonstrate that, under observed conditions, there is an upward trajectory in the frequency, intensity, duration, and affected area of meteorological droughts in China. It is evident that anthropogenic climate change plays a significant role in driving the amplification of these drought factors in China, occupying a dominant position.
Our findings are aligned with previous research, indicating that the expansion of droughts in China, in terms of their range, severity, and frequency, is consistent with a warming climate (Han et al., 2019). The variability of droughts on an interannual to interdecadal scale is primarily influenced by natural factors, while the long-term trend of droughts is predominantly attributed to human activities. The impact and risk of hot and dry events in various regions of China have been amplified by anthropogenic warming (Trenberth et al., 2013; Chen and Sun, 2017; Gu et al., 2019; Fan et al., 2022). The reasons behind these findings are multifaceted. On one hand, the increase in greenhouse gas concentrations resulting from human activities has contributed to an observed rise in intense precipitation across global land areas, particularly in North America, Europe, and Asia. On the contrary, the rise in global temperatures caused by human activities has resulted in increased evaporation rates, leading to more pronounced drought trends (Seager et al., 2010; Giorgi et al., 2011).
This overall warming trend may also bring about alterations in precipitation patterns and an augmented evaporation rate, thereby leading to regional disparities in the impact of anthropogenic climate change on droughts (Zhang et al., 2019; Treydte et al., 2023). Earlier investigations utilizing CMIP6 data have determined that anthropogenic climate change has heightened the frequency, duration, and severity of droughts in Southeast China, while simultaneously diminishing droughts in the Northwest (Yong et al., 2023). Our study findings reveal that anthropogenic climate change has exacerbated droughts in specific regions, namely the northeastern part of the Northwest River basins, the southwestern and central parts of the Songliao River basin, the northern part of the Haihe River basin, and the north-central part of the Yangtze River basin. Conversely, it has reduced droughts in the central part of the Northwest River basinss.
Our study introduces a fresh outlook on evaluating the influence of anthropogenic climate change on droughts. In contrast to previous studies that primarily assess the impact of anthropogenic climate change by analyzing the difference between natural forcing experiments and all forcing experiments in CMIP6 (Samaniego et al., 2018; Chiang et al., 2021; Zhao et al., 2023a), our research utilizes the distinction between observational data and counterfactual climate data to determine the effects of anthropogenic climate change. Nevertheless, two aspects necessitate consideration and improvement for future research endeavors.
First, the research findings may encompass inherent uncertainties, primarily stemming from the data and detrending techniques employed (Dai and Zhao, 2017). In comparison to using observational station data, this study utilized the GSWP3-W5E5 dataset as its data source, which may introduce biases. Furthermore, different results can be obtained in trend analysis by selecting different statistical methods, fitting models, and predictors. In this study, the predictor chosen was global temperature change, which can effectively capture the changing characteristics of most regional climate variables. However, it is important to acknowledge that changes in regional climate variables may also be influenced by land use changes, aerosol emissions, and the regional climate characteristics of large-scale decadal climate oscillations (Mudelsee, 2019), thereby introducing uncertainties.
Second, the choice of time scale for drought indices is a critical factor to consider. A 12-month time scale may not adequately capture short-term drought events, potentially resulting in an incomplete understanding of the essential dynamics of short-term droughts. This is particularly relevant in regions where precipitation exhibits significant seasonal variations. To address this limitation, the utilization of the SPEI with multiple time scales can provide a more comprehensive analysis of droughts. Several studies (Zhao et al., 2017; Mukherjee et al., 2018; Zhang et al., 2023) have emphasized the advantages of employing SPEI with different time scales.
5 Conclusion
This study employs the SPEI as a drought indicator to analyze the impact of anthropogenic climate change on the characteristics of meteorological droughts in China. The analysis is based on observational data from GSWP3-W5E5 covering the years 1960–2019, in addition to counterfactual climate data. The main findings of this research can be summarized as follows:
1) In 1960–2019, China witnessed an augmentation in drought frequency, intensity, duration, and affected area. The rise in drought occurrences has been particularly evident in regions covering 54.87% of the country’s landmass, namely the Northwest River basins, the Yangtze River basin, the Southeast River basins, and the Pearl River basin. Notably, the central and eastern parts of the Northwest River basins have experienced an average increase of 1.62 occurrences per decade. Moreover, drought intensity has intensified in 56.46% of the regions, primarily concentrated in the northeastern part of the Northwest River basins, the north-central part of the Yellow River basin, the junction of the northwestern part of the Haihe River basin, the northern part of the Yangtze River basin, the central and southern parts of the Northwest River basins, and the northwestern part of the Southwest River basins, with an average increase of 0.08. Additionally, 53.62% of the national area has witnessed an increase in drought duration, predominantly in the central and northern parts of the Yellow River basin, the central and northern parts of the Yangtze River basin, the Southeast River basins, and the eastern part of the Pearl River basin, with an average increase of 0.89 months per decade.
2) In 1960–2019, China has witnessed a rise in the occurrence and severity of droughts, assuming a counterfactual climate scenario. However, there has been a decline in the duration of droughts and the extent of land affected by them. On average, the frequency of droughts has increased by 0.54 per decade, encompassing 53.85% of the country’s landmass. These droughts are primarily concentrated in the central and southern regions of the Northwest River basins, the central-western part of the Songliao River basin, the southern part of the Yangtze River basin, and the western area of the Pearl River basin. Simultaneously, the intensity of droughts has risen by an average of 0.04 per decade, impacting 55.68% of the national territory. The most affected areas are predominantly located in the central and northern regions of the Northwest River basins, the central-northern part of the Yellow River basin, and the southern section of the Southeast River basins. In contrast, the duration of droughts has decreased by an average of 1.08 months per decade, covering 56.56% of China’s territory. This decline is primarily observed in the central part of the Songliao River basin, as well as the central, northern, and southwestern regions of the Northwest River basins.
3) Over the years from 1960 to 2019, the effects of anthropogenic climate change have manifested in an escalation of the occurrence, intensity, duration, and impacted area of meteorological droughts in China. Anthropogenic climate change has had a discernible impact on drought patterns in China between 1990 and 2019 when compared to the period from 1960 to 1989. The frequency of droughts increased by 0.10, their intensity heightened by 0.05, and their duration extended by 0.15 months. Additionally, the affected area expanded by 2.95%. The impact of anthropogenic climate change on drought patterns is evident in several regions of China, such as the northeastern part of the Northwest River basins, southwestern and central-eastern parts of the Songliao River basin, northern part of the Haihe River basin, central-northern part of the Yangtze River basin, eastern part of the Pearl River basin, and northwestern part of Southwestern Rivers basin. Anthropogenic climate change is identified as the key driver behind the observed changes in drought frequency, intensity, duration, and affected area, with CR of 84.67%, 75.25%, 190.32%, and 133.99%, respectively. The regions most impacted by anthropogenic climate change are predominantly located in the Haihe River basin, Huaihe River basin, Yangtze River basin, and Pearl River basin, et al.
Data availability statement
The original contributions presented in the study are included in the article/Supplementary material, further inquiries can be directed to the corresponding author.
Author contributions
RD: Conceptualization, Investigation, Resources, Software, Writing–original draft, Writing–review and editing. JH: Supervision, Writing–review and editing. ZC: Writing–review and editing, Investigation, Resources, Software. JZ: Investigation, Resources, Software, Writing–review and editing. PH: Writing–review and editing, Supervision.
Funding
The author(s) declare that financial support was received for the research, authorship, and/or publication of this article. This research was funded by Guangxi Key Research and Development Program Project (Guike AB22080060) and National Natural Science Foundation of China—United Nations Environment Program (NSFC-UNEP) International Cooperation Project (42261144002).
Acknowledgments
We sincerely acknowledge the reviewers whose kind and valuable comments greatly improved the manuscript.
Conflict of interest
The authors declare that the research was conducted in the absence of any commercial or financial relationships that could be construed as a potential conflict of interest.
Publisher’s note
All claims expressed in this article are solely those of the authors and do not necessarily represent those of their affiliated organizations, or those of the publisher, the editors and the reviewers. Any product that may be evaluated in this article, or claim that may be made by its manufacturer, is not guaranteed or endorsed by the publisher.
References
Chen, H., and Sun, J. (2017). Anthropogenic warming has caused hot droughts more frequently in China. J. Hydrol. 544, 306–318. doi:10.1016/j.jhydrol.2016.11.044
Chen, Y., Li, Y., Li, Z., Liu, Y., Huang, W., Liu, X., et al. (2022). Analysis of the impact of global climate change on arid regions. Adv. Earth Sci. 37, 111–119. (in Chinese with English abstract). doi:10.11867/j.issn.1001-8166.2022.006
Chiang, F., Mazdiyasni, O., and AghaKouchak, A. (2021). Evidence of anthropogenic impacts on global drought frequency, duration, and intensity. Nat. Commun. 12, 2754. doi:10.1038/s41467-021-22314-w
Compo, G. P., Whitaker, J. S., Sardeshmukh, P. D., Matsui, N., Allan, R. J., Yin, X., et al. (2011). The twentieth Century reanalysis Project. Q. J. R. Meteorol. Soc. 137, 1–28. doi:10.1002/qj.776
Dai, A., and Zhao, T. (2017). Uncertainties in historical changes and future projections of drought. Part I: estimates of historical drought changes. Clim. Change 144, 519–533. doi:10.1007/s10584-016-1705-2
Du, J., Qiu, Y., Li, Y., Lu, Q., Hao, C., and Liu, H. (2023). Evolution characteristics of annual precipitation and its intra-annual distribution from 1956 to 2016 in China Adv. Water Sci. 34, 182–196. doi:10.14042/j.cnki.32.1309.2023.02.003
Eyring, V., Bony, S., Meehl, G. A., Senior, C. A., Stevens, B., Stouffer, R. J., et al. (2016). Overview of the coupled model Intercomparison Project phase 6 (CMIP6) experimental design and organization. Geosci. Model. Dev. 9, 1937–1958. doi:10.5194/gmd-9-1937-2016
Fan, K., Zhang, Q., Gu, X., Singh, V. P., Xu, C.-Y., Shen, Z., et al. (2022). Global soil moisture drought identification and responses to natural and anthropogenic forcings. J. Hydrol. 610, 127993. doi:10.1016/j.jhydrol.2022.127993
Frieler, K., Volkholz, J., Lange, S., Schewe, J., Mengel, M., Rivas López, M. R., et al. (2023). Scenario set-up and forcing data for impact model evaluation and impact attribution within the third round of the Inter-Sectoral Model Intercomparison Project (ISIMIP3a). EGUsphere 2023, 1–83. doi:10.5194/egusphere-2023-281
Gelfan, A., Kalugin, A., and Krylenko, I. (2023). Detection, attribution, and specifying mechanisms of hydrological changes in geographically different river basins. Clim. Change 176, 122. doi:10.1007/s10584-023-03557-6
Gillett, N. P., Shiogama, H., Funke, B., Hegerl, G., Knutti, R., Matthes, K., et al. (2016). The detection and attribution model Intercomparison Project (DAMIP v1.0) contribution to CMIP6. Geosci. Model. Dev. 9, 3685–3697. doi:10.5194/gmd-9-3685-2016
Giorgi, F., Im, E. S., Coppola, E., Diffenbaugh, N. S., Gao, X. J., Mariotti, L., et al. (2011). Higher hydroclimatic intensity with global warming. J. Clim. 24, 5309–5324. doi:10.1175/2011jcli3979.1
Gu, L., Chen, J., Xu, C.-Y., Kim, J.-S., Chen, H., Xia, J., et al. (2019). The contribution of internal climate variability to climate change impacts on droughts. Sci. Total Environ. 684, 229–246. doi:10.1016/j.scitotenv.2019.05.345
Gudmundsson, L., and Seneviratne, S. (2016). Anthropogenic climate change affects meteorological drought risk in Europe. Environ. Res. Lett. 11, 044005. doi:10.1088/1748-9326/11/4/044005
Han, L., Zhang, Q., Jia, J., Wang, Y., and Huang, T. (2019). Intensity, frequency, and duration of drought under the background of climate warming in China and its north-south differences. J. Desert Res. 39, 1–10. (in Chinese with English abstract). doi:10.7522/j.issn.1000-694X.2018.00112
Huang, J., Zhai, J., Jiang, T., Wang, Y., Li, X., Wang, R., et al. (2018). Analysis of future drought characteristics in China using the regional climate model CCLM. Clim. Dyn. 50, 507–525. doi:10.1007/s00382-017-3623-z
IPCC (2012). Summary for policymakers. Manag. Risks Extreme Events Disasters Adv. Clim. Change Adapt. Cambridge, United Kingdom: Cambridge University Press
IPCC (2021a). Climate change 2021: the physical science basis. Cambridge, United Kingdom and New York, NY, USA: Cambridge University Press.
IPCC (2021b). Summary for policymakers. In: climate change 2021: the physical science basis. Contribution of working group I to the sixth assessment report of the intergovernmental Panel on climate change. Cambridge, United Kingdom and New York, NY, USA: Cambridge University Press.
Jiang, D., and Wang, N. (2021). Interpretation of IPCC AR6 report: changes in the water cycle. Adv. Clim. Chang. Res. 17, 699–704. doi:10.12006/j.issn.1673-1719.2021.160
Kim, H. (2017). Data from: global soil wetness Project phase 3 atmospheric boundary conditions (experiment 1). Data Integration Analysis Syst. (DIAS). [Data set]. doi:10.20783/DIAS.501
Lange, S. (2019). Trend-preserving bias adjustment and statistical downscaling with ISIMIP3BASD (v1.0). Geosci. Model. Dev. 12, 3055–3070. doi:10.5194/gmd-12-3055-2019
Lange, S., Menz, C., Gleixner, S., Cucchi, M., Weedon, G. P., Amici, A., et al. (2021). Data from: WFDE5 over land merged with ERA5 over the ocean (W5E5 v2.0). ISIMIP Repos. doi:10.48364/ISIMIP.342217
Li, H., Chen, H., Sun, B., Wang, H., and Sun, J. (2020). A detectable anthropogenic shift toward intensified summer hot drought events over northeastern China. Earth Space Sci. 7doi. doi:10.1029/2019ea000836
Liu, K., Nie, G., and Zhang, S. (2020). Spatio-temporal evolution characteristics of temperature and precipitation in China from 1951 to 2018. Adv. Earth Sci. 35, 1113–1126. (in Chinese with English abstract). doi:10.11867/j.issn.1001-8166.2020.102
Liu, Y., and Chen, J. (2021). Future global socioeconomic risk to droughts based on estimates of hazard, exposure, and vulnerability in a changing climate. Sci. Total Environ. 751, 142159. doi:10.1016/j.scitotenv.2020.142159
Mengel, M., Treu, S., Lange, S., and Frieler, K. (2021). ATTRICI v1.1 – counterfactual climate for impact attribution. Geosci. Model. Dev. 14, 5269–5284. doi:10.5194/gmd-14-5269-2021
Mudelsee, M. (2019). Trend analysis of climate time series: a review of methods. Earth Sci. Rev. 190, 310–322. doi:10.1016/j.earscirev.2018.12.005
Mukherjee, S., Mishra, A., and Trenberth, K. E. (2018). Climate change and drought: a perspective on drought indices. Curr. Clim. Chang. Rep. 4, 145–163. doi:10.1007/s40641-018-0098-x
Samaniego, L., Thober, S., Kumar, R., Wanders, N., Rakovec, O., Pan, M., et al. (2018). Anthropogenic warming exacerbates European soil moisture droughts. Nat. Clim. Chang. 8, 421–426. doi:10.1038/s41558-018-0138-5
Seager, R., Henderson, N., and Vecchi, G. (2010). Thermodynamic and dynamic mechanisms for large-scale changes in the hydrological cycle in response to global warming. J. Clim. 23, 4651–4668. doi:10.1175/2010JCLI3655.1
Su, B., Chen, Z., Huang, J., and Jiang, T. (2022). Attribution of climate change: new insights from IPCC AR6 WGⅡ. Acta Meteorol. Sin. 45, 512–519. doi:10.13878/j.cnki.dqkxxb.20220425006
Su, B., Huang, J., Gemmer, M., Jian, D., Tao, H., Jiang, T., et al. (2016). Statistical downscaling of CMIP5 multi-model ensemble for projected changes of climate in the Indus River basin. Atmos. Res. 178-179, 138–149. doi:10.1016/j.atmosres.2016.03.023
Su, B., Huang, J., Mondal, S. K., Zhai, J., Wang, Y., Wen, S., et al. (2021). Insight from CMIP6 SSP-RCP scenarios for future drought characteristics in China. Atmos. Res. 250, 105375. doi:10.1016/j.atmosres.2020.105375
Trenberth, K., Dai, A., Schrier, G., Jones, P., Barichivich, J., Briffa, K., et al. (2013). Global warming and changes in drought. Nat. Clim. Chang. 4, 17–22. doi:10.1038/nclimate2067
Treydte, K., Liu, L., Padrón, R. S., Martínez-Sancho, E., Babst, F., Frank, D. C., et al. (2023). Recent human-induced atmospheric drying across Europe unprecedented in the last 400 years. Nat. Geosci. 17, 58–65. doi:10.1038/s41561-023-01335-8
Wen, S., Su, B., Huang, J., Wang, Y., Treu, S., Jiang, F., et al. (2024). Attribution of streamflow changes during 1961–2019 in the upper Yangtze and the upper Yellow River basins. Clim. Change 177, 60. doi:10.1007/s10584-024-03712-7
WMO (2021). “Atlas of mortality and economic losses from weather, climate and water extremes (1970–2019),” in State of the global climate 2023 (Geneva: WMO).
Wu, Y., Miao, C., Fan, X., Gou, J., Zhang, Q., and Zheng, H. (2022). Quantifying the uncertainty sources of future climate projections and narrowing uncertainties with bias correction techniques. Earth’s Future. 10, e2022EF002963. doi:10.1029/2022EF002963
Yang, X., and Huang, S. (2023). Attribution assessment of hydrological trends and extremes to climate change for Northern high latitude catchments in Norway. Clim. Change 176, 139. doi:10.1007/s10584-023-03615-z
Yong, Y., Zheng, W., Zhao, T., Yang, F., Chen, W., and Chen, X. (2023). Analysis of the impact of human activities on meteorological drought in China. J. Hydrol. 43, 78–85. doi:10.19797/j.cnki.1000-0852.20220038
Zhai, J., Su, B., Krysanova, V., Vetter, T., Gao, C., and Jiang, T. (2010). Spatial variation and trends in PDSI and SPI indices and their relation to streamflow in 10 large regions of China. J. Clim. 23, 649–663. doi:10.1175/2009jcli2968.1
Zhang, J., Sun, F., Lai, W., Lim, W., Wang, T., Wang, P., et al. (2019). Attributing changes in future extreme droughts based on PDSI in China. J. Hydrol. 573, 607–615. doi:10.1016/j.jhydrol.2019.03.060
Zhang, Q., Miao, C., Gou, J., and Zheng, H. (2023). Spatiotemporal characteristics and forecasting of short-term meteorological drought in China. J. Hydrol. 624, 129924. doi:10.1016/j.jhydrol.2023.129924
Zhang, X., Yu, J., Li, L. Z. X., and Li, W. (2022). Role of anthropogenic climate change in autumn drought trend over China from 1961 to 2014. J. Meteorol. Res. 36, 251–260. doi:10.1007/s13351-022-1178-3
Zhao, H., Gao, G., An, W., Zou, X., Li, H., and Hou, M. (2017). Timescale differences between SC-PDSI and SPEI for drought monitoring in China. Phys. Chem. Earth 102, 48–58. doi:10.1016/j.pce.2015.10.022
Zhao, R., Sun, H., Xing, L., Li, R., and Li, M. (2023a). Effects of anthropogenic climate change on the drought characteristics in China: from frequency, duration, intensity, and affected area. J. Hydrol. 617, 129008. doi:10.1016/j.jhydrol.2022.129008
Keywords: anthropogenic climate change, meteorological drought, detrend method, drought characteristics, impact
Citation: Dai R, Huang J, Chen Z, Zhou J and Havea PH (2024) Impacts of anthropogenic climate change on meteorological drought in China. Front. Earth Sci. 12:1369523. doi: 10.3389/feart.2024.1369523
Received: 12 January 2024; Accepted: 14 May 2024;
Published: 31 May 2024.
Edited by:
Jianqing Zhai, China Meteorological Administration, ChinaCopyright © 2024 Dai, Huang, Chen, Zhou and Havea. This is an open-access article distributed under the terms of the Creative Commons Attribution License (CC BY). The use, distribution or reproduction in other forums is permitted, provided the original author(s) and the copyright owner(s) are credited and that the original publication in this journal is cited, in accordance with accepted academic practice. No use, distribution or reproduction is permitted which does not comply with these terms.
*Correspondence: Jinlong Huang, huangjl_2012@163.com