- Chinese Academy of Geological Sciences, Beijing, China
In dealing with the occurrence of earthquakes, urban communities and people within the system should primarily consider the geological background, secondary hazards caused by earthquakes, countermeasures to mitigate earthquake disasters, community connectivity, and the impact of various indicators and criteria on the earthquake-proof capacity of the community. It is important to comprehensively assess these factors to ensure that a community is well-prepared to withstand an earthquake. This study takes Shenzhen (China) as an example to conduct a preliminarily evaluation of a method of earthquake-proof capacity during an earthquake, with the results reflecting the comprehensive capacity of communities. The evaluation framework focuses more on effectively improving the disaster response capacity and resilience of urban communities.
1 Introduction
The study of urban communities’ responses to seismic hazards provides key strategies for the safety of community member at the initial moments of an earthquake, the prevention of secondary hazards, and the resolution of key issues such as post-earthquake rescue. With the rapid construction and development of cities and the active development of deep underground space as a resource (Li et al., 2016), there is a closer connection between geological tectonics, geophysics, and research related to urban systems, and there is an increasing demand for the ability to respond to seismic hazards in urban community planning and design. This requires the continuous improvement of a quantitative seismic hazard risk assessment model from the perspective of seismic hazard prevention to guide decision-making regarding urban seismic hazard evacuation, thus improving community capacity for seismic hazard mitigation (Xie, 2006).
Toward the end of last century, the United Nations International Strategy for Disaster Reduction (UNISDR) secretariat proposed project RADIUS (Risk Assessment Tools for Diagnosis of Urban Areas Against Seismic Disasters) to provide a framework for the level of seismic hazards to which cities may be exposed (Davidson and Shan, 1997; Okazaki et al., 2000). The Earthquake Disaster Risk Index (EDRI) was proposed as the first quantitative evaluation of the risk level of different cities, evaluating the relative severity of potential earthquake hazards. This methodology analyzes cities’ exposure to seismic hazard impacts by combining criteria such as seismic hazard, seismic zone resources, vulnerability and external factors, and emergency response and recovery (Liu, 2009).
Earthquake prevention and mitigation capacity is usually defined as the ability of a city or region to ensure safety in the event of an earthquake (Xie, 2006). Seismic hazard risk assessment is an effective way to measure this capacity; it focuses on urban communities or a city as a whole. Specifically, healthcare, transportation, comprehensive pipeline corridors, and other city systems are treated as units for coping with seismic hazards, and their susceptibility to urban seismic hazard risk is assessed and analyzed in a targeted manner (Zhang et al., 2004; Yu et al., 2008; Lin et al., 2011). Currently, with the introduction of concepts, such as urban resilience (Wang et al., 2018; Xie, 2020), urban system evaluation indicators and systems (Yu and Guo, 2021), and evacuation strategies for earthquake-stricken people (Yang et al., 2022), the assessment of seismic hazard risk has become more systematic and comprehensive. By selecting fuzzy cluster analysis (Feng et al., 2007) and hierarchical analysis methods, the ability of seismic disaster response units to address seismic risk is assessed by categorizing indicators at different levels and scoring them through expert discussions.
The study of seismic risk assessment not only works when assessing post-earthquake rescue and recovery capacity as an important part of establishing emergency rescue capacity system to strengthen the ability of an urban community to prevent and mitigate earthquakes; it also provides important references for the urban construction planning process, urban resilience, and other directions for research. In the methods used for seismic risk assessment, various factors (magnitude, intensity, building characteristics, moment of occurrence, geological type of the epicenter, climate, weather conditions of the occurrence of earthquake, number of aftershocks, population density, etc.) are used as elements of overall disaster assessment, or through construction of a system of indicators for evaluating emergency response capacity to earthquakes, which provides a customized basis for earthquake relief by government and relevant departments (Ma et al., 2004; Sun, 2008).
Although previous seismic capacity evaluation involves each discipline as comprehensively as possible and evaluation methods of urban earthquake prevention and mitigation capacity have been studied from different perspectives, there are still few studies on relatively isolated communities and related systems as a unit system for responding to earthquakes in the event of an earthquake. This study, from the perspective of urban earthquake prevention and mitigation capacity, proposes an earthquake-proof capacity assessment method framework that considers urban communities as disaster-bearing bodies (Ren and Wang, 2021). The scope of the study is thus urban communities’ earthquake-proof capacity at the moment of an earthquake’s early warning or at a certain moment of the co-seismic, and the object of responding to seismic hazards is the urban community, as well as potentially disaster-affected people in that community, by discussing seismic risk-resistance capacity. By narrowing down the study time of a targeted area and considering communities that need to be earthquake-resistant, a new strategy has been identified to study the response to seismic disaster risk. This will provide a reference for urban community planning, the management of earthquake-resistant rescue, and the study of the methods of earthquake-resistant emergency command.
2 Methodology
2.1 Methodological objectives
Analytic hierarchy process (AHP) is a decision evaluation method proposed by Saaty (2004), an American operations researcher, which can deal with qualitative and quantitative factors in decision-making in a unified way and is suitable for use in the decision analysis of complex systems. This method can combine qualitative and quantitative analysis, establish a concise and clear structural model of hierarchical analysis through multiple criteria, calculate weights of influencing impact factors on objectives by constructing a judgment matrix, and participate in final decision-making. This approach has a wide range of applications in the assessment of the earthquake-proof capacity of urban systems, urban geological suitability, and community disaster preparedness.
Rather than evaluating their earthquake-proof capacity, lifelines, and structures, urban communities can focus on the safety and security that neighborhood systems can provide for the population and regional systems in moments of response to seismic hazard. Compared to streets and urban areas, communities have a lower need for specialized assessments of urban geology, seismic risk assessment, lifelines, underground corridors, and connectivity. The results of the assessments are more appropriately integrated with local scientific outreach to improve the ability of potentially affected people to cope with earthquake hazards.
The application of hierarchical analysis for earthquake-proof capacity assessment of urban communities has following characteristics. 1) The earthquake-proof capacity of urban communities is evaluated as a unified system. As a unit of production and life, many factors in the community that can affect earthquake-proof capacity can be broken down into different indicators through hierarchical guidelines. 2) Through a combination of qualitative and quantitative analysis, systems associated with the earthquake-proof capacity of urban communities can be broken down into different components, with no need for a strong logical correlation between each criterion; at same time, it is also possible to integrate problems in different ways into same the quantitative target, with relatively simple calculation. 3) This approach combines quantitative and qualitative research, and, at the methodological level, considers previous work in related fields as well as expert advice, and is suitable for preliminary research on methods for quantitatively assessing the seismic capacity of communities. 4) The quantitative assessment of the earthquake-proof capacity of urban communities lays the foundation for the subsequent introduction of quantitative resilience assessment, network hierarchy analysis, and other methods.
2.2 Selection of criterion and index
2.2.1 Geological background
This criterion mainly investigates the tectonic-geological background of urban communities and the evaluation of regional geohazard risk. However, in the research methodology of regional geohazard risk evaluation, the evaluation of the geological background of the area, the potential danger of geohazards, and so forth are often included, and this evaluation can also result in a calculation of urban disaster resilience (Zhang et al., 2019). In process of geohazard risk evaluation, hazard and vulnerability analyses are conducted mainly from the perspective of loss (population casualties, economic loss, and damage to resources and environment) (Zhang et al., 2003). In this, hazard analysis mainly includes the degree of historical activities and potential formation conditions, and vulnerability analysis includes the assessment of disaster-bearing capacity as well as prevention and control capacity. In the event of an earthquake, evaluating the earthquake-proof capacity of a community from the perspective of expected losses (risk evaluation) as a separate criterion for categorizing different indicators would make the scoring weights of the evaluation process, hazard analysis, and vulnerability analysis relatively balanced; however, this is inappropriate for evaluating crisis response during a disaster. For example, geological background includes local tectonic context, which can be obtained from seismic zoning and hazard analysis evaluation methods, and more importantly, the impact on the understanding of geological results from customized geological and geophysical studies, modeling and simulation results related to the evaluation. Thus, the geological background can be summarized as a combination of seismicity (spatial distribution of fractures and historical activity) and site exposure to earthquakes. The evaluation of geologic hazard susceptibility can be related to the previous research results.
2.2.2 Earthquake secondary hazard
Earthquake secondary hazard mainly includes the secondary hazards in the district and the impacts of seismic geologic hazards and structure vulnerability, which are closely related to the community's disaster response strategy. Earthquakes often lead to secondary hazards (such as fires and tsunami), and this indicator requires a field investigation of the urban community combined with community regional planning and the basic information from the relevant lifeline departments that mark key locations (gas stations and substations) and combine the relevant disaster evaluation methods to identify areas to be avoided as evacuation routes. As distinct from the geological background criterion, which is also based on information obtained from preliminary investigation, the earthquake secondary hazard criterion for community response to earthquakes is based on the evaluation of the impacts of geologic hazards occurring in the community at the time of an earthquake, rather than all hazards. This criterion corresponds to strengthened evaluation of seismic geohazards occurring in the region at moment of an earthquake. The vulnerability of community structures can similarly be determined from design indicators and related research.
2.2.3 Countermeasures to earthquake disaster
This criterion mainly includes emergency disaster relief supplies for communities, emergency shelter, emergency evacuation strategies, and individual emergency relief. Emergency disaster relief supplies for communities mainly include community response materials for earthquake-related disasters and secondary disasters and the ability to quickly respond to the problems faced in disasters on the basis of safety, which mainly includes factors such as emergency shelter, disaster defense facilities, and medical facilities. The establishment and location of emergency shelters are based on the risk of secondary disasters and the availability of specific materials. Emergency evacuation strategies for communities have been systematically studied (Yang et al., 2022). In the preliminary study of individual emergency relief supplies, we can only consider the presence of materials. This indicator is determined according to the situation of the particular community, early popularized in science, can be effective in influencing communities to help people with earthquake disaster materials.
2.2.4 Community connectivity
During an earthquake, mutual aid to the community can be considered on the basis of self-help. This criterion mainly considers the accessibility of the community’s main road to external rescue and good connectivity between communities for efficient self-rescue strategies for people afflicted by disaster.
2.3 Hierarchical modeling
We analyzed the existing evaluation indexes of earthquake emergency response capacity, the geological suitability of urban underground space, seismic disaster risk, emergency response capacity evaluation, and previous research. Based on this analysis, the goal of community resilience can be divided into four guidelines the geological context of a community’s area, the probability of secondary hazard risk, the disaster response of a community, community connectivity. These guidelines aim to ensure that individuals in a community can receive necessary support and assistance during a seismic disaster.
Evaluating the impact of the geological context (B1, criteria B1–4, and indexes C1–10 are illustrated in Figure 1) on the target community consists of two main aspects: regional tectonic background (C1) and geological disaster susceptibility (C2). This criterion mainly includes basic information obtained from regional geologic data before the earthquake. The geological context may include the influence of historical earthquakes on the seismic activity of urban ruptures (Dong et al., 2020) and related research on earthquake early warning to the local area. The frequency, scale, and density of historical earthquakes can directly affect seismic hazard analysis of urban communities (Zhang et al., 2003). Earthquake secondary hazard (B2) mainly contains secondary hazards in a community’s area (C3), seismic and geologic hazards in a community’s region (C4), and the vulnerability of community structures (C5). Among these, the assessment of secondary hazards in community’s area can refer to guidelines for the evaluation of seismic zoning, and remaining indicators can follow corresponding evaluation specifications.
Countermeasures to earthquake disaster (B3) guidelines mainly comprise indicators for individuals and communities, such as emergency shelter (C7), emergency evacuation strategies (C8), and other evaluation indicators that require community advance planning and design, and disaster relief emergency supplies for communities (C6)—relevant materials that a community has stockpiled based on the evaluation of local seismic hazards—and individual emergency relief supplies (C9), which include emergency relief materials prepared by individuals. This indicator can be used as an initial indicator for earthquake disaster evaluation; with wider awareness of earthquake disaster response, the impact of this indicator on overall target can be significantly increased. Community connectivity (B4) is considered a separate criterion and is usually a critical relief route (relief facilities of neighboring communities (C10)) that includes safe evacuation routes and strategies.
In constructing a judgment matrix hierarchy, pairwise comparisons of the importance of all evaluation factors for each level is based on the judgment matrix scale (Table 1). Based on the results of expert scoring, a comparison scale is determined to obtain the hierarchical judgment matrix. The need for hierarchical single-sorting, a consistency test, and a product method to calculate the largest eigenroot and eigenvectors, set judgment matrix for A, where elements
Although consistency is not required in constructing judgment matrix A, it is important not to deviate too far from consistency. Therefore, after calculating the weight values that indicate importance ranking, the consistency of each judgment matrix must be tested.
We utilized the consistency index, random consistency index, and consistency ratio for testing, and formulas are as follows:
where CR is the stochastic consistency ratio, CI is the consistency indicator, and RI is the stochastic consistency indicator, the values of which are shown in Table 2; they are related to matrix order n. When CR is less than 0.1, it can be considered that the constructed judgment matrix passes the consistency test; otherwise, the judgment matrix needs to be reconstructed.
When calculating the earthquake-proof capacity of a whole region by multi-factor comprehensive judgment, the study area can be divided into a number of evaluation cells. We select a number of evaluation indicators that can accurately evaluate the appropriateness of dividing cells, then conduct grid-cell scoring for different levels of evaluation indicators, and superimpose the grid-cell scores according to impact weights to obtain the comprehensive scoring value of the evaluation cells by each factor that affects the urban community. Here, as a preliminary test of the proposed methodological framework, the boundaries of each residential area were used as the evaluation boundaries.
3 Preliminary experiments
We selected a site in Guangming District, Shenzhen, China, as methodological test (Figure 2A) for the following reasons. 1) This study is based on the Urban Geological Survey project, which is executed in Shenzhen among other places. 2) The evaluation method in this paper is proposed in the context of deep underground space development in an urban area, where the study of geological formations is more closely integrated with the evaluation of the city’s geology. Research on the earthquake-proof capacity of communities during actual earthquakes can provide references for urban development, underground space development, and other planning studies. 3) Shenzhen: although there is a north-east oriented Lianhuashan Fracture Zone running through whole territory, the Henggang Fracture is main fracture in Shenzhen. However, activity of the regional fracture zone is not strong, and so is suitable for this preliminary study.
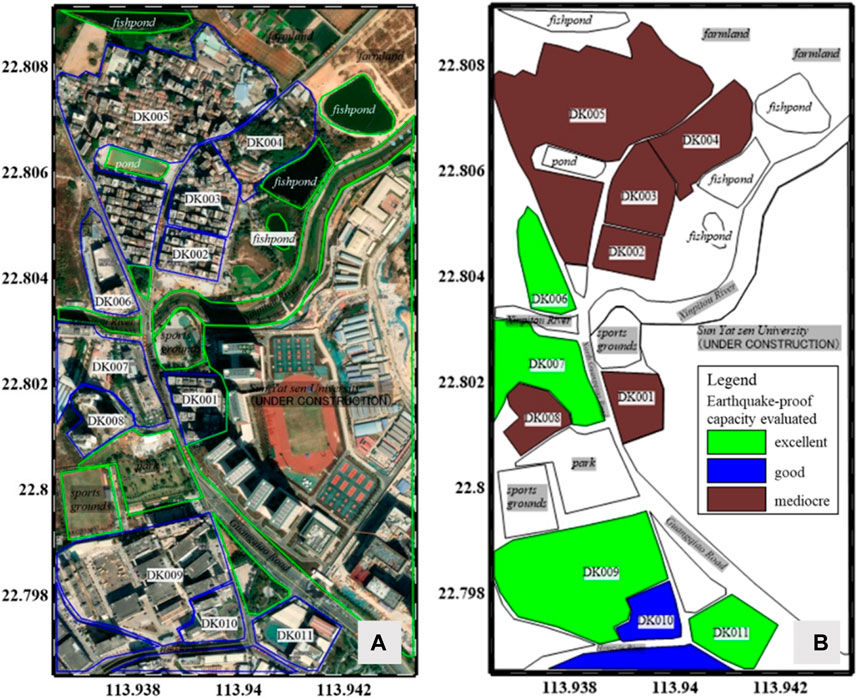
Figure 2. Investigation scope and results of earthquake resistance capacity in Shenzhen Guangming District. (A) Diagram of investigation scope; (B) evaluation results of community.
The test area was selected as an overall survey of easier and relatively independent 12 residential areas, according to the method mentioned in this paper of performing a preliminary evaluation of the earthquake-proof capacity of urban communities.
3.1 Calculating evaluation indicator weights
According to the hierarchical structure given in Figure 1, we evaluated the seismic capacity of 12 residential regions, not neighborhoods, which allowed preliminary results of community earthquake-proof capacity evaluation and provides a basis for improving our methodology.
Combined with the local situation in Shenzhen and evaluation factor scale given by experts, we constructed a judgment matrix from target level to criterion level and from criterion level to indicator level. The weight values of first-level and second-level evaluation indicators were calculated, respectively, and the total order of second-level evaluation indicators’ weights was calculated. Consistency tests of hierarchical single-ranking and total-ranking are shown in Tables 3–6, and the results based on the experts’ scores are given in Table 7.
(1) The target-criteria judgment matrix and consistency test are evaluated according to results given in Table 3.
(2) Judgment matrix and consistency test of criterion layer Bi-indicator layer Cj (4).
We considered this test for verifying the operability of the method, scoring system of evaluation indicators in the study area. The test indicators were set to four reference scoring standards—poor (≤25 points), general (25–50 points), good (50–75 points), and excellent (>75 points) —and each indicator was evaluated through the criteria given by integrated experts to relevant scores (Table 7). The geological context was mainly obtained through the literature (Kang and Li, 1998; Yu, 2010).
The test area (Figure 2A) has been stable for a long time without sudden tectonic movements. The nearest rupture in the region was approximately 30 km away, so 75 points (good) were assigned to the field survey of regional seismic and geologic hazards of the community in the item of geological effects (including geological background and hazard susceptibility) as well as community hazards during earthquakes. The regional secondary hazards field survey process mainly considered gas stations, substations, and other points that might cause secondary disasters during an earthquake. Community scoring was reduced in this field survey, with 75 points (good) as a base score. The community residential area surveyed is closer to a substation but no gas stations, gas pipeline nodes, etc., so there the risk of secondary hazards in community scoring was adjusted downward to 10–20 points, with the remaining community scores unchanged: vulnerability of community structures, field survey based on the age of completion of the community, building structure design, and other comprehensive scoring. Individual and community disaster response guidelines, community disaster relief, and emergency supplies indicator evaluation found that all residential areas in the field survey were equipped with fire hydrants and other infrastructure, so the evaluation area as a whole was assigned 75 points. Emergency evacuation strategies based on the width of streets in neighborhood, connectivity, etc., emergency evacuation sites scoring, plazas in residential area, and the presence of open space, as well as clear signage of evacuation sites affected the overall scoring. Individual emergency relief materials were more difficult to investigate, but in related publicity materials, the survey area as a whole had no anti-earthquake publicity, so the overall score was 60 points. Community connectivity was affected by COVID-19, and the local community was more closed processing, so the community disaster relief connectivity and subsequent foreign aid disaster relief was affected. Connectivity is poor, but part of open residential area or open space area will score more highly, accordingly.
3.2 Test results
Evaluation results are shown in Figure 2B, and we scored 12 relevant residential areas based on the survey area selected as an evaluation unit in the preliminary test. Scoring results were multiplied with weights to obtain the final earthquake-proof capacity of the community during an earthquake. The evaluation results are categorized into three grades: excellent, good, and fair. DK001∼DK005 are older residential areas, with dense housing and mostly brick-concrete buildings, narrow streets and closed access roads except for main roads, and residential areas were close to substations and other facilities, which are prone to secondary hazards (Figure 3C). DK006 is a commercial area, with a number of stores based on supermarkets; supermarket buildings were no more than 40 m and mostly nearly 10 years old. DK007 is a new residential district built in the last 5 years, with better facilities, planning and built structures, higher floors, with multiple access roads and were close to the main road (Figure 3A), while DK008 is a collection of poorly designed factories and districts, with narrower buildings that may affect internal escape routes, and distances between buildings that are too close to each other. DK008 is a collection of poorly designed factories and neighborhoods, where the narrowness of buildings may affect internal escape routes, and the close proximity of buildings leads to insufficient access routes; all are factors that affected the evaluation of their earthquake-proof capacity (Figure 3D). DK009, DK010 (Figure 3B), and DK012 are factories and industrial zones, and the main factors affecting their ratings were their earlier construction time and the impact of relatively independent and closed parks on access routes. DK011 is a newly built school with a low-rise basement shop around it, surrounded by a main road.
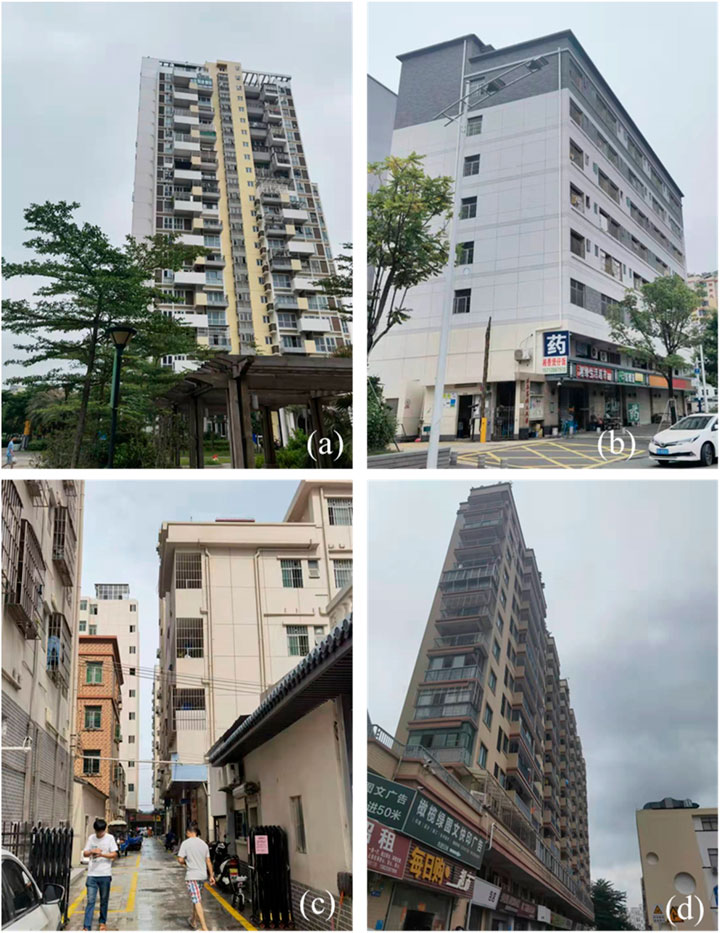
Figure 3. Photographs of surveyed community. (A) DK007 excellent earthquake-proof capacity, (B) DK010 good earthquake-proof capacity, (C) DK002, and (D) DK008 mediocre earthquake-proof capacity.
A total of 12 residential areas within the regional scope of this test were selected for investigation, and the subdivisions were in accordance with the local enclosure. The earthquake-proof capacity obtained also reflects only that capacity of each residential area at the moment of investigation. By evaluating results, it is possible to grade the earthquake-proof capacity of residential areas at the moment of survey. Results show that the earthquake-proof capacity of residential areas in the test area was usually average and was mainly for densely populated residential areas built or more 20 years ago. The results also show that the age of the district may be an important factor in ratings, as the design of seismic capacity is more scarce when a district is built earlier and is a residential area, the design of the earthquake-proof capacity of industrial parks built earlier is better than that of residential areas, and districts and industrial zones built in last 10 years or so have better seismic mitigation and related facilities. In addition, there are no gas stations and other locations prone to secondary disasters in this test area, so relevant scores for this area are more homogeneous, and the actual application of the method of investigation process needs to be evaluated according to relevant design planning for reference. Further investigation through data processing can provide a basis for subsequent larger residential and urban areas for grid-based analysis.
4 Discussion
This study proposes a framework of an earthquake-proof capacity evaluation system for urban communities at the moment of earthquake. During an earthquake, urban communities to be assessed are relatively closed individuals. The selection of the community’s scope has a greater connection with the moment of the earthquake. For example, if an earthquake occurs in the daytime, the affected people may be in office buildings and business districts. If it occurs at night, it may be community in a that is mainly residential. On the way to and from daily work, people may be located in subways, highways, urban arterials, and other open neighborhoods. Therefore, before an earthquake, we should pay attention to our own personal familiar environment as much as possible and its emergency measures. The government may need to organize emergency response training and attentiveness. In addition, lacking in current emergency training is popular awareness of earthquake geology, the environment, and disaster response measures in our community. What follows is a discussion of the methodology for modifying the AHP method mentioned above and the impact of the adjustment of different criteria and corresponding indicators on the evaluation results.
4.1 Impact of adjustments to evaluation methodology on evaluation results
We assessed the earthquake-proof capacity of urban communities during earthquakes using the analytic hierarchy process method (AHP); in the initial selection of impact criteria and indexes, only cases in which indexes independent of each other are taken into account. In order to consider the possible mutual influence of the indicator factors and consider the scalability of the evaluation method, we further adapted AHP into a analytic network process method (ANP) by combining the target and criterion layers in AHP (Figure 1) mentioned above into a control layer. This represents four aspects affecting the results of evaluation, takes criteria as clusters of the network layer in ANP, so that the elements in each clusters are the AHP indexes (Saaty, 2004). A simple network system created for ANP is shown by Figure 4. The criteria in AHP method correspond to clusters of elements in ANP method, and indexes in AHP correspond to elements in ANP. In the ANP method, despite the relative separation of the control layer from the network layer, each criterion in the control layer still represented an element cluster in the network layer, means a relatively well-connected cluster of elements in the network layer. Details of pairwise comparisons in an ANP are shown in the attached list.
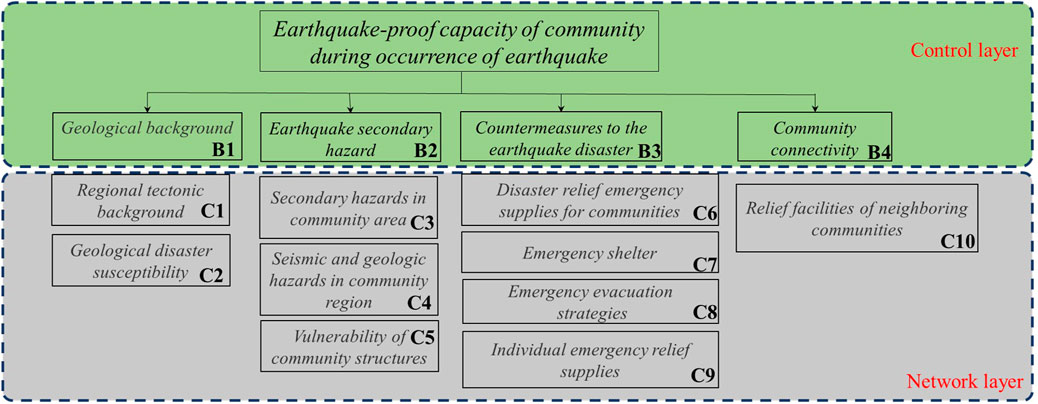
Figure 4. Evaluation of earthquake-proof capacity of urban communities during earthquakes under the ANP method.
It is worth noting that this earthquake-proof evaluation methodology is intended for people or communities affected by an earthquake at a co-seismic moment. Therefore, in the evaluation process, some relatively subjective factors will affect the evaluation of objective factors at the moment of disaster, such as the emergency evacuation strategy formulated for individuals or government departments of an affected community. However, at moment of an earthquake, it will be affected by the specific situation, and disaster-stricken people will ignore the objective facts of the geological background or evaluation of susceptibility to geological hazards, and instead obtaining relief materials or implement evacuation strategies. Therefore, in an evaluation process, although the results of evaluation maybe minimally affected, it is necessary to consider the pairwise comparisons in evaluation.
Compared with the AHP evaluation method, ANP considers the mutual influence of factors from a more refined aspect, and its evaluation results also consider the comprehensive influence among clusters of elements in the network layer in a more integrated way, and whether it is more worthwhile to promote it than AHP with abbreviated algorithms in specific applications. In Table 8, it can be seen that, on the basis of experiments on the AHP method (Table 7), the influence of some indexes on final results produced some changes, requiring further assessment of the indexes’ adjustment.
4.2 Significance tests for changes in criteria and indexes
In order to further analyze the test results, we first calculated whether the difference in evaluation results between AHP and ANP is significant using significance level judgment threshold α = 0.05, and calculating significance level p; if p < α, then there is significant difference between the evaluation results. The calculation results are shown in Figure 4, where the difference between the evaluation results of two methods is not significant. In our ANP methodology of earthquake-proof capacity, indicators such as the C5 and C6 indexes are more affected by method adjustment, and standard deviation caused by two methods reached 0.08802 and 0.03448, respectively. This suggests that, when considering more detailed pairwise comparisons, there will be a greater impact on the two indexes relative to each other.
A comparison of the results can be seen in Figure 5. Blue numbers indicate weighted total weights obtained by AHP, red numbers indicate weighted medium weights obtained by ANP, and black numbers indicate the standard deviation by the difference in calculation of the two methods to calculation of AHP method, which it is possible impact on assessment results due to the difference in choice of methods. The results of significance level calculation show that the significance level of difference between two methods p=0.9995 means that difference between two methods is not significant.
The evaluation of earthquake-proof capacity result based on the AHP and ANP methods is not significant (Figure 5). On the one hand, it may be because we refer to the basic settings of the AHP method in constructing the network model as well as in considering the mutual influence of elements. On the other hand, it also indicates that it is feasible to select the AHP method while making a simple assessment of earthquake-proof capacity. However, in the process of practical application, we have to confront the situation where internal elements have a greater influence, such as geological disaster susceptibility (C2, shown in Figure 4) and secondary hazards in community area (C3) having a greater interplay in some communities. This kind of situation only needs to adjust the weight influence on the basis of ANP, but on the basis of AHP, it needs to reclassify the hierarchical structure. Therefore, when we make a simple assessment of the earthquake-proof capacity of an urban community, the results given in our discussion show that AHP is a simple method, but when it is necessary to comprehensively and systematically assess the seismic capacity of the community and the impact of different indicators and adjustments, ANP is a better method.
We will further discuss the significance of different scoring results for criteria weights and indexes weights separately. First, we discuss impact on results of the guideline criteria that could have been fully evaluated before the earthquake, based on some scenarios that might actually occur.
Case 1. Poor community connectivity, but far from active fracture zones. Weights of B3 and B4 can be increased appropriately.
Case 2. Poor community connectivity and close to active fracture zones. Weight of B2 needs to be strengthened.
Case 3. Good community connectivity, close to active fracture zones. Weight of B2 needs to be further strengthened and weight of B4 needs to be reduced.
The weights of the indicators were adjusted as shown in Table 9. Results obtained for the level of significance are shown in Figure 5. Figure 6 compares different evaluation criteria, and the error bars in it indicate the possible impact of the three cases discussed in the evaluation of the initial case (Table 7, Case0). Black text indicates the value of the standard deviation of the impact of different cases on the evaluation of Case 0. Figure 6 also shows that C4 and C5 are subjected to different scenarios of criteria adjustments that have a greater impact on the overall evaluation results, perhaps due to the fact that criterion B2 is adjusted more in different cases.
The level of significance p of the differences produced by the three cases compared to the case 0 is shown in Table 10.
The results show that the significance levels are all greater than the threshold, indicating that the results are not significantly different. Therefore, adjusting the significance of the criteria with some variations for different situations from actual cases in the pilot area will not have a significant impact on the overall evaluation results.
For the different indexes we can also discuss several cases: in the geological context, we can consider the influence of the differing importance of the geological tectonic context and geological disaster susceptibility on the results by selecting each value of pairwise comparison a11=1/3,1/6,1/9 in Table 4, respectively. We thus get a significance level of p of 0.9539, 0.9333, and 0.9252, meaning that the difference between the evaluation results is not significant. Two cases can be considered for seismic and geologic hazards in the community’s region at the time of earthquake: if the severity of secondary hazards in this community is equal to the importance of seismic and geological hazards in that region, then the importance of the first two items is less the vulnerability of community structures; if the severity of secondary hazards in this community is greater than the importance of seismic and geologic hazards in that region, then the importance of the first two items is less than the vulnerability of the community structures. The significance levels obtained were p=0.2402 and p=0.2254 —again, not significant. Among the countermeasures to the earthquake disaster criterion, emergency evacuation strategies were the most important for the test area, followed by emergency shelters. Several scenarios can be discussed around the importance of disaster relief emergency supplies for communities versus individual emergency relief supplies, which is usually the case when individuals are not adequately prepared to cope with earthquake supplies. When the importance of community relief supplies is the same as that of emergency shelters, p=0.2985, and when the importance of community emergency supplies differs from that of emergency shelters, then it may be that the community lacks sufficient emergency shelters, p=0.3489. Therefore, by reasonably adjusting the importance of different indicators according to the actual situation in the test area, the significance level will not be affected and it will have a greater impact on the evaluation results of earthquake-proof capacity during an earthquake. In fact, adjustments to different indexes are less likely to make a significant difference in the impact of overall evaluation relative to the impact of the criteria on overall evaluation results, and adjustments to the impact of some indexes only affect the assessment of weights, which is no more than four indexes.
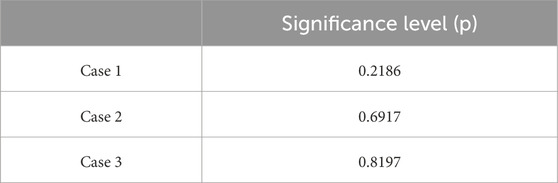
Table 10. Significance of differences between the importance of different criteria and original criteria.
5 Conclusion
Current research on urban earthquake-proof capacity and earthquake vulnerability usually examines the whole of earthquake occurrence-rescue or even the earthquake recovery process, and it examines the ability of a specific system (e.g., lifeline and medical care) or urban community in a city, as a disaster-affected body in a specific tectonic environment or urban environment, to cope with earthquake disasters. In this study, a framework for an earthquake-proof capacity evaluation method for urban communities during an earthquake is proposed, which provides a basis for the seismic response strategy of a community as a relatively closed whole at the moment of an earthquake. This framework is in the context of the growing sophistication of big-data processing and analytics and the development of the Internet of Things (IoT). The following problems may be solved by the method here proposed.
(1) For the first time, a relatively closed disaster-affected community or people in such a community during an earthquake becomes the research object. Through the further study of this method, we provide a customized reference for popularizing earthquake coping strategies for those across different communities.
(2) This evaluation method is an immediate evaluation indicator, which is specific only to the relatively short period between the actual earthquake and time of the external rescue connection, that is crucial for a community and its residents to help themselves. This approach also lays a foundation for subsequent research on the quantitative seismic resilience of urban communities and the effective improvement of urban seismic resilience.
The earthquake-proof capacity during an earthquake in each residential area was evaluated by investigating a test case in Shenzhen, using different residential areas as the dividing line. The results show that, during earthquakes, the earthquake-proof capacity of the test area was mostly general because most of it was built more than 10 years ago as residential areas, mostly self-built 4∼5-story buildings whose safety is not guaranteed. Corresponding industrial parks, on the other hand, have better design and access roads to ensure evacuation and rescue at the moment of an earthquake. Newly built parks and neighborhoods in the survey area had better road connections and open space design, which greatly strengthened earthquake-proof capacity. By discussing the influence of indicator selection and adjustment of evaluation results, the principle of selecting different indicators for each criterion was determined, and no significant difference in evaluation results were produced when the importance of each indicator was reasonably adjusted.
The evaluation of earthquake-proof capacity during an earthquake is a topic of research on seismic capacity and the resilience of cities in research on urban development and geotectonic that is becoming more and more relevant. The research objectives covering such guidelines are closely related to the development of urban deep underground space and the study of regional geological and geophysical tectonics. The methodology of this paper provides important references for earthquake disaster response strategies, seismic planning, and post-disaster reconstruction programs by combining the results of previous research on urban geological surveys as well as seismic resilience assessment.
Data availability statement
The datasets presented in this study can be found in online repositories. The names of the repository/repositories and accession number(s) can be found in the article/Supplementary material.
Author contributions
TR: writing–review and editing, writing–original draft, visualization, validation, supervision, software, resources, project administration, methodology, investigation, funding acquisition, formal analysis, data curation, and conceptualization. ZW: writing–review and editing, supervision, and investigation. YM: writing–review and editing. JY: writing–review and editing. KL: writing–review and editing. HL: writing–review and editing.
Funding
The author(s) declare that financial support was received for the research, authorship, and/or publication of this article. This research was supported by the Project of China Geological Survey (DD20211314 and DD20190287).
Conflict of interest
The authors declare that the research was conducted in the absence of any commercial or financial relationships that could be construed as a potential conflict of interest.
Publisher’s note
All claims expressed in this article are solely those of the authors and do not necessarily represent those of their affiliated organizations, or those of the publisher, the editors, and the reviewers. Any product that may be evaluated in this article, or claim that may be made by its manufacturer, is not guaranteed or endorsed by the publisher.
References
Davidson, R., and Shan, H. (1997). An urban earthquake disaster risk index. Report, 121. Stanford, California: Blume Earthquake Engineering Center.
Dong, P., Ren, T., and Wang, Z. (2019). Numerical study of the impact on fault activity in ji’nan prior zone caused by historical strong earthquakes in north China. J. Geodesy Geodyn. 40 (10), 1055–1061. doi:10.14075/j.jgg.2020.10.012
Feng, Q., Sun, Z., Yan, F., Wu, Y., and Gao, J. (2007). Model of fuzzy synthetic evaluation on seismic resistance capacity of urban communities. World Earthq. Eng. 23 (01), 1–5.
Kang, Z., and Li, Z. (1998). The characteristic and stability studies on shenzhen (Luo hu) -Hong Kong (yuen long) fault belt. Chin. J. Geol. Hazard Control 9 (Suppl. ment), 172–178.
Li, X., Li, C., Aurele, P., Wu, W., Li, H., Sun, L., et al. (2016). Multiple resources and their sustainable development in Urban Underground Space. Tunn. Undergr. Space Technol. 55, 59–66. doi:10.1016/j.tust.2016.02.003
Lin, S., Xie, L., Gong, M., and Li, M. (2011). Methodology for estimating seismic capacity of city building. J. Nat. Disasters 20 (4), 31–37.
Liu, L. (2009). Calibration capability of the urban seismic prevention and disaster mitigation and research on acceptable risk level. Harbin, China: Institute of Engineering Mechanics.
Ma, Y., Zhang, Y., Zhang, C., and Wang, J. (2004). Theory and approaches to the risk evaluation of geological hazards. J. Geomechanics 10 (1), 7–18. doi:10.3969/j.issn.1006-6616.2004.01.002
Okazaki, K., Villacis, C., and Cardona, C. (2000). RADIUS: risk assessment tools for diagnosis of urban areas against seismic disasters. UN. International Strategy for Disaster Reduction (ISDR). https://www.undrr.org/publication/radius-risk-assessment-tools-diagnosis-urban-areas-against-seismic-disasters.
Ren, T., Li, H., Wang, Z., Yan, J., Liu, K., Guo, C., et al. (2021). Method and system for calculating seismic resilience of urban community during occurrence of earthquakes. 2021106733
Ren, T., Wang, Z., Yan, J., Liu, K., Xu, B., Guo, C., et al. (2021). Method and system for determining earthquake-proof capacity of community during occurrence of earthquake. 2021103768
Saaty, T. (2004). Decision making — the analytic hierarchy and network processes (AHP/ANP). Syst. Sci. Syst. Eng. 13, 1–35. doi:10.1007/s11518-006-0151-5
Sun, Z. (2008). Quantitative methodology and application of urban natural disaster assessment. Qindao, China: Ocean University of China.
Wang, Z., Ma, D., and Wang, X. (2018). Research on seismic disaster prevention countermeasures to improve urban resilience. Beijing Plan. Rev. 8 (2), 30–33.
Wang, Z., and Yi, F. (2009). AHP-Based evaluation of occurrence easiness of geological disasters in mianyang city. J. Nat. Disasters 18 (1), 14–23.
Xie, J. (2020). Urban resilience assessment based on earthquake disaster. Chongqing, China: Chongqing University.
Xie, L. (2006). A method for evaluating cities’ ability of reducing earthquake disaster. Earthq. Eng. Eng. Vib. 26 (3), 1–10.
Yang, J., Zhan, H., Chen, L., and Dou, Z. (2022). Planning of emergency evacuation routes in densely populated urban areas during earthquakes. Tsinghua Univ (Sci Technol). 62 (1), 70–76. doi:10.16511/j.cnki.qhdxxb.2021.21.020
Yu, C. (2010). Research on the faults activity and seismic hazard in shenzhen. Hangzhou, China: Zhejiang University.
Yu, T., and Guo, E. (2021). Comprehensive seismic capacity assessment method of regional water supply network. Earthq. Eng. Eng. Dyn. 41 (4), 100–108. doi:10.13197/j.eeev.2021.04.100.yuty.011
Yu, Y., Li, G., Li, P., Zhu, Q., Yuan, D., Wang, C., et al. (2008). Investigation and analysis of electric equipment damage in sichuan power grid caused by wenchuan earthquake. Power Syst. Technol. 32 (11), 5–10. doi:10.13335/j.1000-3673.pst.2008.11.021
Zhang, C., Wu, M., and Zhang, Y. (2002). Method and prospect of geological disaster risk assessment. J. Nat. Disasters 12 (1), 96–102. doi:10.13577/j.jnd.2003.0116
Zhang, F., Xie, L., and Fan, L. (2004). Study on evaluation of cities’ ability reducing earthquake disasters. Acta Seismol. Sin. 26 (3), 318–329+342. doi:10.3321/j.issn:0253-3782.2004.03.011
Keywords: earthquake-proof capacity, Shenzhen—China, earthquake evacuation strategies, analytic hierarchy process method, analytic network process
Citation: Ren T, Wang Z, Ma Y, Yan J, Liu K and Li H (2024) Study of an evaluation method of earthquake-proof capacity of a community during an earthquake. Front. Earth Sci. 12:1366697. doi: 10.3389/feart.2024.1366697
Received: 07 January 2024; Accepted: 05 March 2024;
Published: 11 April 2024.
Edited by:
Karoly Nemeth, Institute of Earth Physics and Space Science (EPSS), HungaryReviewed by:
Mostafa Toni, Helwan University, EgyptAnastasia Nekrasova, Institute of Earthquake Prediction Theory and Mathematical Geophysics (RAS), Russia
Copyright © 2024 Ren, Wang, Ma, Yan, Liu and Li. This is an open-access article distributed under the terms of the Creative Commons Attribution License (CC BY). The use, distribution or reproduction in other forums is permitted, provided the original author(s) and the copyright owner(s) are credited and that the original publication in this journal is cited, in accordance with accepted academic practice. No use, distribution or reproduction is permitted which does not comply with these terms.
*Correspondence: Zhihui Wang, d2FuZzE1ODA2QDE2My5jb20=; Yan Ma, eW1hNjQxMTExQDE2My5jb20=