- 1Observation and Research Station of Crustal Stress and Strain in Beijing, Ministry of Natural Resources, Institute of Geomechanics, Chinese Academy of Geological Sciences, Beijing, China
- 2School of Earth Sciences and Resources, China University of Geosciences, Beijing, China
- 3Key Laboratory of Active Tectonics and Crustal Stability Assessment, Institute of Geomechanics, Chinese Academy of Geological Sciences, Beijing, China
- 4Institute of Geological Survey, China University of Geosciences, Wuhan, China
The Niangmuco region on the east margin of the Eastern Himalayan Syntaxis features a large number of glacial lakes. The development process and stability classification of glacial lakes is of great significance to the study of seasonal glaciers in the eastern Himalayan margin, with implications for economic development and disaster prevention. Based on Landsat remote sensing image data from 2000 to 2021, this study analyzed the development and change characteristics of glacial lakes in the Niangmuco region during the past 21 years, and classified the stability of lakes with areas >0.02 km2 using the fuzzy consistent matrix method. In this area, 126 glacial lakes were identified within an elevation range of 3044–4156 m with a total area of 10.94 km2. These lakes primarily included glacial erosion lakes, valley lakes, tectonic lakes, and landslide dam lakes. Specifically, glacial erosion lakes accounted for 88.9% of the total number of lakes and 60.3% of the total lake area, followed by valley lakes with 6.3% and 23.7%, respectively. From 2000 to 2010, the total area of glacial lakes decreased from 10.53 km2 to 10.09 km2, which may be attributable to climate fluctuations. Subsequently, the area of lakes increased significantly to 10.94 km2 in 2021, an increase of 0.41 km2. Compared with 2000, among the lakes with a growth rate of 0.019 km2/a in 21 years, glacial erosion lakes exhibited the largest change. Among the classified glacial lakes in the study area, 95.7% were stable and relatively stable, mostly comprising glacial erosion lakes at high altitudes between 3468 and 4156 m. Only 4 unstable and extremely unstable glacial lakes were identified, and they were located near a fault zone. The findings show that the development and the change of glacial lakes in the area are primarily controlled by temperature and precipitation, and the topography and fault activity have important influences on the stability of glacial lakes.
1 Introduction
The Niangmuco region on the eastern margin of the Eastern Himalayan Syntaxis is the core of the continental-continental collision between the Indian Plate, the Myanmar Plate, and the Qinghai-Tibet Block, featuring active tectonic movements, extensive distribution of active faults and frequent earthquakes (Dong et al., 2018; Wei et al.,. 2023). At the same time, this region is characterized by wide variations in topographic height and significant differences in rainfall between dry and wet seasons. Furthermore, a large number of glacial lakes are distributed in the region. Under the influence of various processes, such as climate change (including rainfall and snow), fault activity, and earthquakes, these lakes are very likely to be subjected to instability phenomena such as lake contraction, expansion, and outburst (Richardson and Reynolds, 2000; Bajracharya et al., 2007; Nie et al., 2017). Therefore, the analysis of the characteristics and stability of these glacial lakes has important implications for economic development and disaster prevention in the Niangmuco region. It is also of great significance to study seasonal glaciers in the eastern Himalayas.
Glacial lakes are defined as lakes formed by glaciation, and are widely distributed in high-altitude and high-latitude mountain areas. Their main source of recharge is modern glacial meltwater or they may form as a result of water accumulation in glacial moraine depressions (Yao et al., 2017). In recent decades, with global warming, global mountain glaciation zones have seen a significant retreat of mountain glaciers, accompanied by the formation of a large number of glacial lakes as well as their expansion. The rapid expansion of glacial lake area has increased the risk of glacial lake outburst (Wu et al., 2019; Ding et al., 2021; Wang et al., 2022). As the dam break of glacial lakes poses severe threats to downstream areas, the development and stability of glacial lakes have received much attention (Veh et al., 2018; Hu et al., 2022). Previous studies on glacial lakes have mainly focused on changes in the distribution of glacial lakes (Wang et al., 2013; Zhang et al., 2020; Ding et al., 2022), the identification of potentially high-risk glacial lakes (Allen et al., 2019; Zhang et al., 2022), and the mechanism of typical glacial lake outbursts (Nie et al., 2018; Liu et al., 2019b; Li H. et al., 2021; Shi et al., 2021), achieving fruitful results. For example, Yang et al. (2019) established a set of glacial lake inventory standards and completed the Chinese glacial lakes inventory in 2015. Chen et al. (2021) completed a 30 m dataset for glacial lakes in High Mountain Asia from 2008 to 2017 and conducted a corresponding research on glacial lakes. Tao et al. (2021) analyzed the spatial changes of the glacial lakes in the western Nyainqentanglha Range. Liu et al. (2019a) analyzed the temporal and spatial distribution and dynamic changes of glacial lakes in the Parlung Zangbo River Basin, and identified and evaluated potentially dangerous glacial lakes. Sun et al. (2022) studied the spatio-temporal variation and identified high-risk glacial lakes in the Yarlung Zangbo River Basin. Liu et al. (2009) analyzed and summarized the outburst mechanism of an end-moraine lake in Tibet, primarily considering the overflow and piping-break types of outburst. Stuart-Smith et al. (2021) analyzed the influence of anthropogenic factors on glacier retreat and glacial lake outburst flood disasters. Selecting Guangxieco as the research object, Chang et al. (2017) analyzed the meteorological and hydrological conditions, genetic mechanism, and risk of glacial lake outburst.
Studies on the stability of glacial lakes have focused the risk assessment (Dubey and Goyal, 2020; Khadka et al., 2021). In general, previous studies have evaluated the risk of glacial lake outbursts using evaluation indicators, such as topography, the slope of dam, and the impact area of disaster (Bolch et al., 2011; Fan et al., 2019). For example, Aggarwal et al. (2017) developed a comprehensive inventory of glacial lakes in Sikkim, India, based on high-resolution remotely sensed data and evaluated the degree of the susceptibility of glacial lakes to outbursts. Zheng et al. (2021) conducted a comprehensive assessment of past evolution, current status, and modeled future changes of glacial lakes in the Third Pole and determined the risk of glacial lake outburst flooding.
A seasonal glacial area refers to a region where glaciers accumulate in winter and melt in summer. A number of large-scale studies of glacial lakes have been carried out in the high mountains of Asia, including the Himalayas and the Tianshan Mountains, but previous studies on glacial lakes have focused on areas with perennial alpine polar glaciers (Shugar et al., 2020; Wang et al., 2020; Zhang et al., 2021). However, suitable case studies on the stability of glacial lakes in seasonal glacial areas in tectonically active areas are lacking (Haritashya et al., 2018; Li et al., 2020a). Precious studies on glacial areas have emphasized on the influence of glaciers on the formation, development, and outburst mechanisms of glacial lakes (Prakash and Nagarajan, 2017; Zhang et al., 2023). In seasonal glacial lakes, glaciers do not provide a continuous supply of meltwater and thus cannot strongly influence the development process of glacial lakes; instead, tectonic activity should play an important role.
Based on Landsat TM/OLI remote sensing image, this study investigated the development and change of seasonal glacial lakes in the Niangmuco region on the eastern margin of the Eastern Himalayan Syntaxis. Considering the regional characteristics, we selected the influencing factors. The weights of influencing factors was determined using the fuzzy consistent matrix method, which is based on mathematical and statistical methods (Yao and Zhang, 1997; Hu et al., 2022). Also, the stability classification of glacial lakes was achieved by this method.
2 Geological background
The study area is located in the eastern margin of the eastern Himalayas, south of the Zabra Pass (Figure 1), with the highest elevation at 4437 m and the lowest elevation at 600 m. The topography is characterized by significant differences in elevation, and the terrain is high in the southeast and low in the northwest. Under the influence of the Indian Ocean Monsoon, it has a subtropical monsoon climate with relatively mild conditions and dense vegetation (Bai et al., 2004; Wang et al., 2011). In particular, precipitation exhibits large differences between summer and winter, with high temperature and abundant rainfall in summer, and mild temperature and scarce rainfall in winter. Owing to its terrain, transportation is inconvenient.
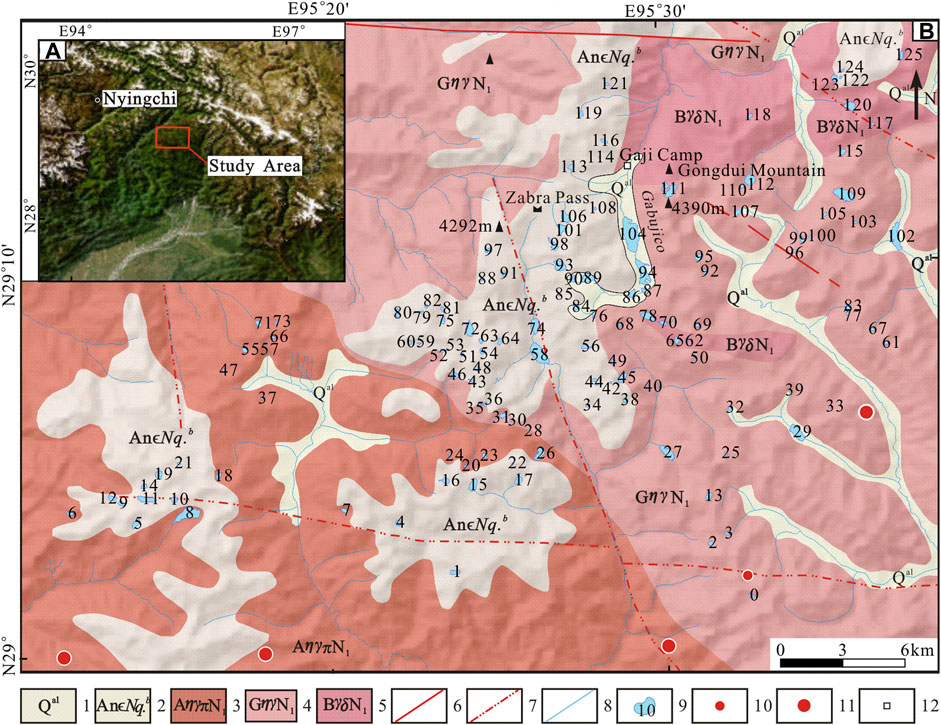
FIGURE 1. (A) Location of the study area (B) Geological map of the study area.1—Holocene series; 2—Nyainqentangglha rock group; 3—Medog supergroup Anzhala unit Porphyry biotite monzogranite; 4—Medog supergroup Gongdui unit Porphyry biotite monzogranite; 5—Jinzhu supergroup Bengbengla unit Biotite granodiorite; 6—Fault; 7—Inferred fault; 8—River; 9—Glacial Lake (The number on the glacial lake is the code name of the glacial); 10—M3∼3.9 (Earthquakes); 11—M4∼4.9 (Earthquakes); 12—Place names.
The lakes in the central part of the study area are mainly developed in the late Proterozoic Nyainqentangglha rock group (AnЄNq.b) and the intrusive rocks of the late Yan Mountains Medog supergroup (Du et al., 2016; Wang et al., 2019). The Nyainqentangglha rock group is a middle-high grade metamorphic rock series dominated by banded migmatite and biotite plagioclase gneiss, with the occurrence of local rock types such as marble, schist, quartzite, granulite, and amphibolite. Some lakes also developed in the alluvial deposits of quaternary gullies at lower elevations. Magmatic activities are mainly represented by intrusive rocks of the Medog supergroup, which can be divided into the porphyry biotite monzogranite of the Anzhala unit and the porphyry biotite monzogranite of the Gongdui unit. Some lakes are also scattered in the biotite granodiorite of the Bengbengla unit of the Jinzhu supergroup in the north. The Niangmuco region, located on the eastern margin of the Eastern Himalayan Syntaxis, is characterized by a tectonically active area, with a wide distribution of faults and frequent earthquakes (Li S. et al., 2021; Huang et al., 2021). Through a comprehensive analysis of the previous 1:250,000 regional geological survey and visual interpretation of remote sensing images, several faults have been identified in the eastern and central parts of the study area, including nearly EW-trending faults and NNW-trending faults (Figure 1).
3 Data and methods
3.1 Data
This study selected Landsat TM/ETM +/OLI images with small cloud cover and snow cover as primary data. Specifically, 34 images with a spatial resolution of 30 m covering the period from 2000 to 2021 were selected (https://earthexplorer.usgs.gov/). Multispectral images of 15 m resolution were obtained through the image fusion of multi-spectral images with a resolution of 30 m and panchromatic band images with resolution of 15 m. This fusion could improve the spatial resolution of multispectral images (Thomas et al., 2008; Li Z. et al., 2021). Some images with poor quality were replaced by those close to the corresponding months, primarily focusing on the months from September to December. We used Google Earth as supplementary means to interpret and classify the glacial lake. The elevation data were obtained from ASTER GDEM with a resolution of 30 m (https://www.gscloud.cn/). Climatic data were collected from temperature and precipitation grid data of the Qinghai Tibet Plateau and its surrounding areas in 1998–2017 (https://data.tpdc.ac.cn/) (Ding, 2019). The geological data were collected from the Mêdog section of the geological map of the People’s Republic of China (1:250,000), using historical earthquake data (Department of China Earthquake Administration protection, 1995; Department of China Earthquake Administration protection, 1999) and monitoring data from the China Earthquake Network (http://www.ceic.ac.cn/history).
3.2 Methods
Two methods were mainly employed to extract glacial lakes: computer automatic extraction and manual visual interpretation. In order to improve the accuracy of glacial lake boundary extraction, the method of artificial visual interpretation was used in ArcGIS to extract the glacial lake and classify the glacial lakes.
3.2.1 Error analysis
The spatial resolution of satellite data is a key factor affecting the uncertainty of glacier lake area measurement. In this study, the uncertainty was evaluated on the basis of the glacial lake extraction error, which is proportional to the resolution of the sensor (Chen et al., 2021).
where
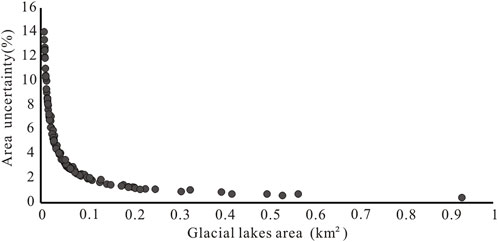
FIGURE 2. Relationship between the uncertainties in area and the areas of all the glacial lakes in the study region.
3.2.2 Interpretation and classification of glacial lakes
Thus far, no uniform standard has been established for the classification of glacial lakes (Li et al., 2020b; Lesi et al., 2022), and different scholars have adopted different classification standards according to different research purposes. For example, Yao et al. (2017) established a classification system of glacial lakes by dividing glacial lakes into their formation mechanism, geomorphology, and spatial distribution. According to Yang et al. (2021), glacial lakes in western Sichuan and eastern Tibet can be divided into three types: glacial erosion lakes, moraine lakes, and barrier lakes. Rinzin et al. (2021) divided glacial lakes into four categories: non-glacier-fed, glacier-unconnected, glacier-connected, and supraglacial lakes. Considering that the region is tectonically active and lacks perennial glaciers, changes in glacial lakes in the region are most affected by the geological and climatic conditions of the region. Therefore, according to the genetic type, the glacial lakes in the region can be divided into four types: glacial erosion lakes, valley lakes formed by glacial meltwater convergence, tectonic lakes, and landslide dam lakes formed by landslide blockage.
(1) Glacial erosion lakes (Figure 3A): Glaciers and the rocks they carry continuously erode the ice bed during movement to form depressions, which are then filled with water as the glaciers recede. Such lakes are usually located near or above the glacier or snow line, and have a typical snow-grain basin and glacial erosion depression landform. In general, the plane shape of the lakes is nearly round, the water outlet is located on the bedrock surface, the lakes are relatively stable, and the area is small.
(2) Valley lakes (Figure 3B): A valley lake refers to a lake formed by continuous water accumulation in valleys through accumulation and erosion, and their shape is mostly elliptical.
(3) Tectonic lakes (Figure 3C): After their formation some glacial lakes are deformed by tectonic activities, resulting in tectonic lakes. In the study area, tectonic lakes are mainly reflected in the linear arrangement of some lakes in the fault zones and the elongated shape of lakes, in sharp contrast to the partially elliptical shape of the other three forms.
(4) Landslide dam lakes (Figure 3D): These lakes refer to lakes formed by a landslide blocking a river valley, and landslide deposits can be clearly observed at the lower mouth of the lake.
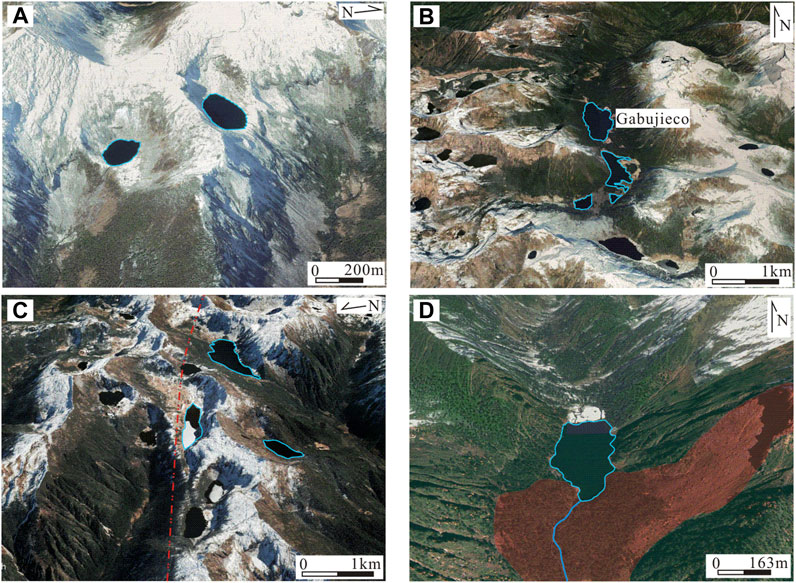
FIGURE 3. Classification of glacial lakes (A) Glacial erosion lakes (Mape Date: Google Earth, © 2024 CNES/Airbus) (B) Valley Lakes (Gabujieco valley) (Mape Date: Google Earth, © 2024 CNES/Airbus) (C) Tectonic Lakes (fault denoted by the red dotted line) (Mape Date: Google Earth, © 2024 CNES/Airbus) (D) Landslide dam lake (red area indicates landslide area) (Mape Date: Omap, 2010-2022 © Beijing Ovital Software Co., Ltd.).
3.2.3 Stability classification of glacial lake based on fuzzy consistent matrix
Regarding the stability classification of the glacial lakes, previous studies primarily evaluated the susceptibility of glacial lakes to outburst mainly using the fuzzy matrix method (Duan et al., 2020), analytic hierarchy process (Muneeb et al., 2021), and catastrophe progression method (Tang et al., 2023). The most widely used method is the analytic hierarchy process. However, limited by the factor intensity of 1–9, the analytic hierarchy process does not provide sufficient consistency in some cases (Huang et al., 2005). The fuzzy consistent matrix can effectively avoid the inconsistency in pairwise comparison, and it offers simple calculation and easy adjustment. Therefore, the weight of each indicator is calculated using the fuzzy consistent matrix method (Hu et al., 2022). Regarding the selection of factors, previous studies did not consider the factors of tectonic activities and sudden geological events. The tectonic factor could lead to geological events, such as landslides. Considering the particularity of the study area, in addition to the commonly used parameters such as glacial lake area and watershed area, the tectonic factor was added to classify the stability of glacial lakes with an area larger than 0.02 km2 in the study area.
In this study, the following influencing factors were selected for the classifying the stability of glacial lakes.
(1) Potential factors of ice and rock avalanches: ice and rock avalanches are among the major factors leading to glacial lake outburst. Any slope of >30° and overall slope trajectory of >14° (tan α = 0.25) is considered to be likely to collapse into a glacial lake. Within each lake watershed, the tan α values for all grid cells fulfilling these two criteria were summed to obtain a quantitative measure of the potential for avalanches to trigger an outburst from that lake (Allen et al., 2019).
(2) Tectonic factors: the Eastern Himalayan Syntaxis experiences tectonic activities frequently, and the strong tectonism will affect the stability of glacial lakes. Combining the geological map with the interpreted fault, the analysis of the buffer zone of the fault implied that the stability of the glacial lake decreases with proximity to the fault.
(3) Glacial lake area: the larger the glacial lake area, the greater the pressure on the dam body in front of the glacial lake, which will affect the stability of the dam body.
(4) Type of glacial lake: glacial erosion lakes are a relatively stable type of glacial lakes, and they have a small area and relatively stable dam body (Yang et al., 2021). Valley lakes are relatively stable because of their low elevation and flat terrain, and the glacial lake dam may be an ancient moraine. In contrast, landslide dam lakes and tectonic lakes are more unstable.
(5) The mean slope value of the dam body in front of a glacial lake: the stability of dams and the potential for self-destruction are key factors affecting the stability of glacial lakes.
(6) Watershed area: A larger upstream watershed is considered to increase the potential for runoff (Allen et al., 2019). During heavy rainfall events or inflow of glacial meltwater into glacial lakes, the watershed area of the upper reaches of lakes will affect lake stability.
The quantification of the stability classification was achieved by grading each impact indicator as shown in Table 1. Lower values of stability index indicate more stable conditions of glacial lakes, while higher values indicate more unstable conditions.
In the fuzzy consistent matrix method, a scale of 0.1 to 0.9 is set for the indices, and two indicators are set for pairwise comparison. Given that the importance of indicator A is greater than that of indicator B when comparing indicators A and B, RAB is set to be greater than 0.5. Given that indicator A is of the same importance as indicator B when comparing indicators A and B, RAB is set to 0.5. According to previous studies on factors affecting glacial lake outburst, Liu et al. (2019b) sorted out glacial lake outburst events and their basic characteristics in Tibet, China, and analyzed six causes, namely, ice collapse/ice landslide, buried ice melting, glacial meltwater, heavy precipitation, debris flow and upstream glacial lake outburst flood. Among them, ice collapse/ice landslide and related combinations accounted for 70% of the total causes. Begam et al. (2018) pointed out that glacial lake outburst is mainly caused by the surge wave generated by falling rocks or avalanches, or it may also be caused by glacial meltwater or sudden heavy precipitation. Byers et al. (2018) conducted a numerical simulation of a glacial lake that had collapsed and found that rock fall was the main factor of glacial lake outburst. These studies emphasize that ice and rock collapse, as a sudden event, is a major cause of glacial lake outburst.
As the study area features seasonal glaciers without perennial glaciers, glacier-related factors cannot be taken as influencing factors. Through data analysis and actual investigation, the potential of rock fall is concluded to be the main cause of glacial lake outburst. At the same time, as the region is a tectonically active area, regional tectonic activity has a significant impact on the stability of the glacial lakes. The consistency of the matrix is tested using Eqs 2, 3, and Rij is the index of comparison between indicator i and j. The constructed fuzzy consistency matrix is shown in Table 2.
After constructing the fuzzy consistent matrix, the weight values of each indicator are calculated by using the following formulas.
where Wi is the weight of the indicator, n is the total number of indicators, and Aik is the value calculated by the fuzzy consistent matrix method in Table 2.
As shown in Table 2, the weights A1 to A6 calculated by the fuzzy consistent matrix are 0.2266, 0.1866, 0.1066, 0.1466, 0.1666, and 0.1666 respectively. After the weights are determined, the stability P of each glacial lake is calculated by the stability index of different indicator grades and the weights of each indicator through Eq. 6.
where P is the stability index, SIi is the stability index value of each indicator to different levels, and Wi is the weight value of each indicator.
The calculated stability index of the glacial lakes was classified into four categories: stable (p < 0.5), relatively stable (P: 0.5–0.65), unstable (P: 0.65–0.75), and extremely unstable (p>0.75).
4 Results and analysis
Remote sensing images on 27 October 2000, 4 November 2010, 28 November 2010, and 30 November 2021 were selected as the basic images, and other images were used as auxiliary images to complete the glacial lake extraction for 2000, 2010, and 2021. The extracted glacial lakes in 2021 were classified and the stability of the glacial lakes was evaluated.
4.1 Characteristics of glacial lake development
4.1.1 Regional distribution and changes of glacial lakes
In this study, 126 glacial lakes were extracted in the study area, covering a total area of 10.94 km2, with the largest glacial lake occupying an area of 0.93 km2. Glacial erosion lakes are mainly distributed in the central and southwest regions of the study area at higher elevations, among which 112 small glacial erosion lakes accounted for 88.9% of the total number of lakes, with an area of 6.6 km2 accounting for 60.3% of the total glacial lake area (Figure 4). Valley lakes are mainly developed in areas with gentle terrain in the central part (glacial lakes No. 94, 102 and 104 in Figure 1), with a total of 8 lakes accounting for 6.3% of the total number, and an area of 2.59 km2 accounting for 23.7% of the total area. Although their number is small, they occupy a large area. Four tectonic lakes (glacial lakes No. 5, 8, 11 and 58 in Figure 1) with a total area of 1.32 km2 were mainly developed in the southwest and central fault zones. Landslide dam lakes are developed in the central valley area, with a total area of 0.43 km2 (glacial lakes No. 72 and 74 in Figure 1).
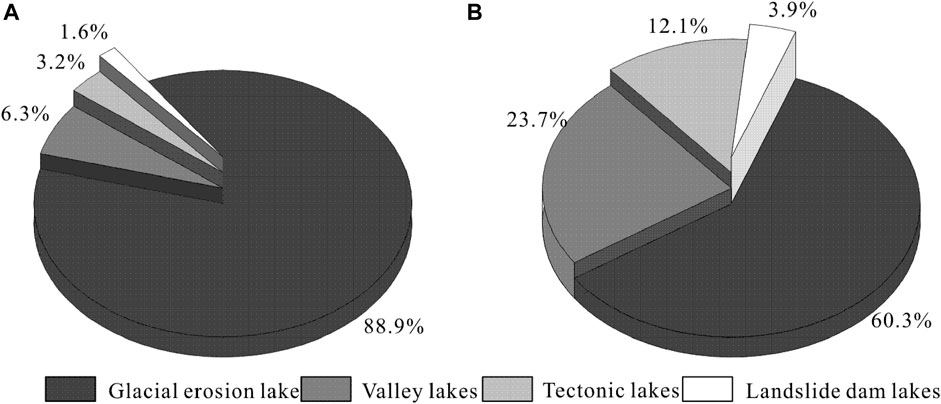
FIGURE 4. Proportion of different types of glacial lakes in the study area. (A) Proportion of the number of different types of glacial lakes (B) Proportion of the area of different types of glacial lakes.
As shown in Figure 5, the total area of glacial lakes in this region generally increased by 0.41 km2, with a growth rate of 0.019 km2/a. Specifically, the glacial lake area decreased significantly from 10.53 km2 in 2000 to 10.09 km2 in 2010. However, the total glacial lake area showed a significant upward trend, increasing from 10.09 km2 in 2010 to 10.94 km2 in 2021, accounting for an increase of 0.85 km2. The area of the largest glacial lake (glacial lake No. 104 in Figure 1) increased from 0.91 km2 to 0.93 km2 from 2000 to 2010, and the overall area further increased from 2010 to 2021. According to the changes of the total glacial lake area and the largest glacial lake area in the region, the changes of the glacial lake area in the past two decades mainly showed an upward trend. Affected by climate fluctuations, the glacial lake area decreased slightly from 2000 to 2010, but increased significantly from 2010 to 2021. The area of each type of glacial lake has increased to varying degrees. The total area of glacial erosion lakes increased from 6.3 km2 to 6.6 km2, with a growth rate of 0.014 km2/a. The total area of the valley lakes increased from 2.51 km2 to 2.59 km2, an increase of 0.08 km2. Glacial erosion lakes exhibited a large increase in area, serving as the largest contributor to the change of the overall area of regional glacial lakes, which could be attributed to the influence of glacial meltwater and precipitation. The total area of tectonic lakes and landslide dam lakes showed little change from 2000 to 2021.
4.1.2 Elevation distribution of glacial lake
In the study area, glacial lakes are distributed within an elevation range from 3044 to 4156 m, with an average elevation of 3790 m. The elevation range of glacial lakes was divided with 200 m as a grading interval. As shown in Figure 6A, the number of glacial lakes in the range of 3800–4000 m was the largest, accounting for 48.4% of the total number, and the area of glacial lakes in the range of 3600–3800 m was the largest, accounting for 32.6% of the total area. The relationship between glacial lake area and elevation showed an obvious negative correlation. The higher the elevation, the smaller the glacial lake area, and the lower the elevation, the larger the glacial lake area. For example, the number of glacial lakes <3600 m accounted for only 10.3% of the total number, while the area accounted for 32% of the total area. The number of glacial lakes within 3600–3800 m was smaller than that within 3800–4000 m, and the total area was larger.
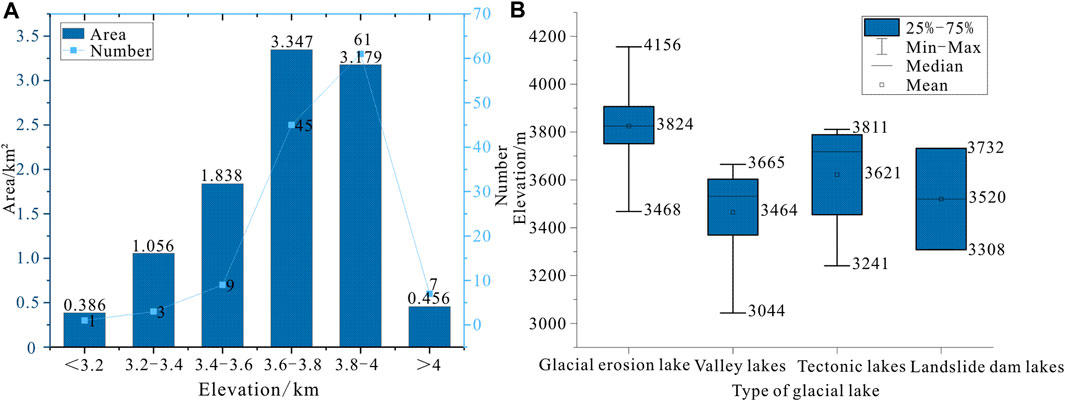
FIGURE 6. Elevation distribution of glacial lakes. (A) Area distribution of glacial lakes in different elevation regions (B) Elevation distribution of different types of glacial lakes.
As shown in Figure 6B, glacial erosion lakes with more regional development are distributed with an elevation range from 3468 to 4156 m. According to the average elevation, they are mainly distributed at higher elevations. Valley lakes are distributed within an elevation range from 3044 to 3665 m, with an average elevation of 3464 m, which is the lowest among the average elevations of different glacial lake types. This further indicates that valley lakes develop in flat terrain with lower elevation. Some tectonic lakes are distributed at relatively high elevations, with an average elevation of 3621 m and the lowest being 3241 m. Their distribution is related to the specific fault distribution. The distribution of landslide dam lakes did not exhibit any unique pattern, with the higher elevation at 3732 m and the lower elevation at 3308 m.
4.2 Stability classification of glacial lakes
The stability classification results of glacial lakes with an area larger than 0.02 km2 revealed two extremely unstable glacial lakes, which are glacial lakes No. 58 and No. 74 in Figure 7, respectively. Among them, glacial lake No. 58, distributed in the central part of the NNW-trending fault, was classified as the most unstable glacial lake. There are 6 relatively stable glacial lakes and 82 stable glacial lakes. The relatively stable glacial lakes and stable glacial lakes account for 95.7% of the total number of assessed glacial lakes, and they are dominated by high altitude glacial erosion lakes (mainly distributed at high altitudes from 3468 to 4156 m) and some low altitude valley lakes without tectonic influence.
5 Discussion
5.1 Development of glacial lakes in the Niangmuco region of the east margin of the Eastern Himalayan syntaxis
5.1.1 Development of glacial lakes and climate change
Glacial lakes primarily receive water supply from glacial melt water and atmospheric precipitation. When the water supply is greater than the outflow and evaporation, the area of glacial lakes increases. Climate change and precipitation are the main factors leading to the change of glacial lakes. The increase in the area of glacial lakes is driven by increases in temperature and precipitation (Zhang et al., 2017; Lei et al., 2022).
As shown in Figure 8A, from the annual mean temperature change from 1998 to 2017 (Ding, 2019), the annual mean temperature increased from 6.5°C to 7.9°C from 2000 to 2010, with a rate of change of 0.14°C/a. An overall fluctuating upward trend was observed after 2000, with a corresponding upward trend of glacial lake area. A comparison of glacial lake changes with temperature changes shows that the glacial lake area decreased from 2000 to 2010, probably due to temperature fluctuations. In 2010, there was a downward trend in temperature, and glacial lake area showed a decrease, which could also be attributed to slight differences in seasonal conditions for the months selected, with significantly more precipitation in October than in November. Rising temperatures can influence changes in glacial lakes and increase the size of glacial lakes by facilitating the flow of glacial meltwater into valleys. However, because the glaciers in the region are not perennial glaciers, they cannot provide a large amount of continuous glacial meltwater. Therefore, the overall slow upward trend of glacial lake area with a slight fluctuation is driven by climate change.
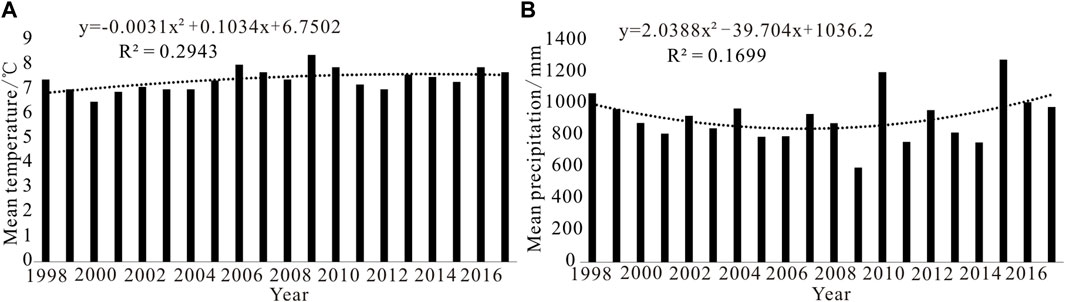
FIGURE 8. Climate change in the study area. (A) Annual mean temperature change (B) Mean precipitation change.
As shown in Figure 8B, the annual precipitation changes in the study area fluctuated considerably, with a general trend of decreasing and then increasing after 2000. Precipitation exhibited a decreasing trend from 1998 to 2010, which is consistent with the precipitation trend reported by Nie et al. (2018) and may have contributed to the decrease in glacial lake area. There was a sudden increase in precipitation in 2010, but the glacial lake did not become larger as a result of the sudden increase in precipitation in 1 year. This may be because the selected images are for fall and winter. Specifically, the region has a subtropical monsoon climate, and the low precipitation in winter is not sufficient to induce significant changes in glacial lake area. In summary, the overall trend of increasing temperature and precipitation from 2000 to 2021 is the main reason for the expansion of glacial lake area. In 2010, the glacial lake area in 2010 was possibly affected by the decreasing trend of temperature in the region between 2010 and 2012, and the decreasing trend of precipitation between 1998 and 2010, as well as the selection of winter images.
Previous studies on glacial lake changes in the Tibetan Plateau (Zhang et al., 2015; Nie et al., 2017; Shugar et al., 2020) suggest that small lakes and those at higher altitudes have experienced more significant changes in area, and global warming, which is accelerating the retreat of glaciers, is believed to be the main cause of the expansion of most glacial lakes. By comparing the changes of glacial lakes in the seasonal glacial area of the tectonically active Niangmuco region with the results of previous studies on glacial lake changes in the Tibetan Plateau region, the overall glacial lake area in this study showed an increasing trend from 2000 to 2021, which is the same as the previous trend of glacial lake area expansion in the last two decades. However, the decline in glacial lake area in 2010 due to climate fluctuations in the region reflects prominent regional variability in the change of glacial lake area, which is strongly influenced by factors such as topography, tectonics, and seasonal precipitation.
5.1.2 Development of glacial lakes and tectonic activity
The Niangmuco region is located in the eastern margin of the Eastern Himalayan Syntaxis. The geological background of this region is characterized by extensive faults and frequent earthquakes. The comprehensive analysis of the previous 1:250,000 regional geologic survey and visual interpretation of remote sensing images revealed a number of the nearly EW-trending fault and NNW-trending fault in the eastern and central parts of the study area (Figure 1). As shown in Figure 3C, combined with the tectonic distribution characteristics and development characteristics of glacial lake morphology, glacial lakes in the southwest of the study area are affected by the faults. Morphologically, the lakes appear in the form of strips, and the linear arrangement of lake distribution and the direction of the fault development is consistent with the EW-trending fault. Therefore, the formation of these lakes is probably related to the historical activities of the fault. The development of glacial lake No. 58 in the central part (Figure 1) was influenced by faults and topography, and its morphology is also consistent with the direction of fault development, which is NNW-trending. Although the area of tectonic lakes has not changed much in the past two decades, it is important to note that tectonic activities can occur suddenly. At the same time, tectonically active areas are prone to earthquakes, and if sudden tectonic deformation, earthquakes, and other geological events occur in the region, the shape of the glacial lakes may be rapidly altered, or the lakes may even be destabilized, leading to disasters, such as glacial lake outbursts.
In summary, the development of faults has an important influence on the morphology and distribution of glacial lakes in the region, and the distribution of some glacial lakes is consistent with the direction of fault development. In the tectonic steady state, faults have little effect on the area of glacial lakes, whereas sudden geologic events, such as tectonic deformation, are an important factor influencing the rapid destabilization of glacial lakes.
5.2 Factors affecting the stability and development mechanism of glacial lakes
5.2.1 Factors influencing regional glacial lake stability
As shown in Figure 7, 95.7% of the glacial lakes in the region are relatively stable, indicating that although the region is a tectonically active area, the stability of small glacial lakes at high altitudes are not significantly affected over a short period of time. The two extremely unstable glacial lakes are No. 58 and No. 74, and the unstable glacial lakes are No. 11 and No. 102. The instability of No. 58 is primarily attributable to the high possibility of rock avalanche, the strong potential influence of tectonic activity and the large watershed area. The instability of No. 74 is primarily attributable to its location in a large watershed area, which increases the likelihood of heavy rainfall events increasing the water catchment area and the probability of rock avalanches leading to the formation of landslide dam lake. No. 102 is a valley lake, which is also unstable due to its watershed area and the potential impact of rock avalanches. No. 11 is unstable mainly because of its location in the fault zone. It is noteworthy that the primary factor controlling the stability of glacial lakes in the study area is the possibility of landslides generated by rock avalanches. The region is a tectonically active area with a large slope around the lake, and receives abundant rainfall, which is likely to promote the instability of the slopes and the dam in front of the glacial lake. In the event of landslides triggered by sudden fault activity, earthquakes, or heavy precipitation, landslides will flow into the glacial lake and form surges, which will result in the decline of the stability of the glacial lake.
In order to better provide information for the early warning of disasters in the Niangmuco region, it is necessary to strengthen the monitoring of unstable glacial lakes at a later stage, further collect glacial lake data through outdoor surveys, and analyze the impact of glacial lake outburst floods on the downstream.
5.2.2 Analysis of regional glacial lake development mechanism
The formation of glacial lakes cannot be separated from the elements of “topography and geomorphology” and “glacial meltwater” in the development area (Emmer et al., 2020; Wang et al., 2022). However, different types of glacial lakes develop under different mechanisms and processes. For example, the development of glacial erosion lakes is mainly controlled by the development and flow of glaciers, and the development of valley lakes is controlled by the amount of glacial meltwater, which is further determined by climatic factors and the valley watershed area. The formation of tectonic lakes is mainly controlled by faults and seismic activities, and the development of landslide dam lakes is also mainly controlled by tectonic activity or climate change.
Considering the type of glacial lake development and the tectonic and climatic background of the study area, glacial erosion lakes in the area are believed to have developed under three main stages: glacial development, formation of depressions through the erosion of ice beds by glaciers during movement, and formation of lakes by the accumulation of water in the eroded depressions after glacial recession (Figure 9A). The formation of valley lakes is mainly controlled by precipitation in the watershed, and their development is also believed to have occurred in three stages: valley development, convergence of precipitation in the watershed, and expansion of accumulated water into lakes (Figure 9B). The formation of tectonic lakes is mainly controlled by the distribution and activities of fault zones, and their development involved three main stages: fault development, formation of linear depressions by fault activities, and formation of lakes by watershed catchment (Figure 9C). The formation mechanism and process of landslide dam lakes have been extensively studied (Wang et al., 2016; Morris et al., 2018; Shi et al., 2021). The formation of landslide dam lakes in the region is also mainly controlled by landslides or tectonic activities. Therefore, topography and tectonics are the controlling factors affecting the development pattern of glacial lakes in the region, and climate change and precipitation are indispensable and important factors in the development process of glacial lakes.
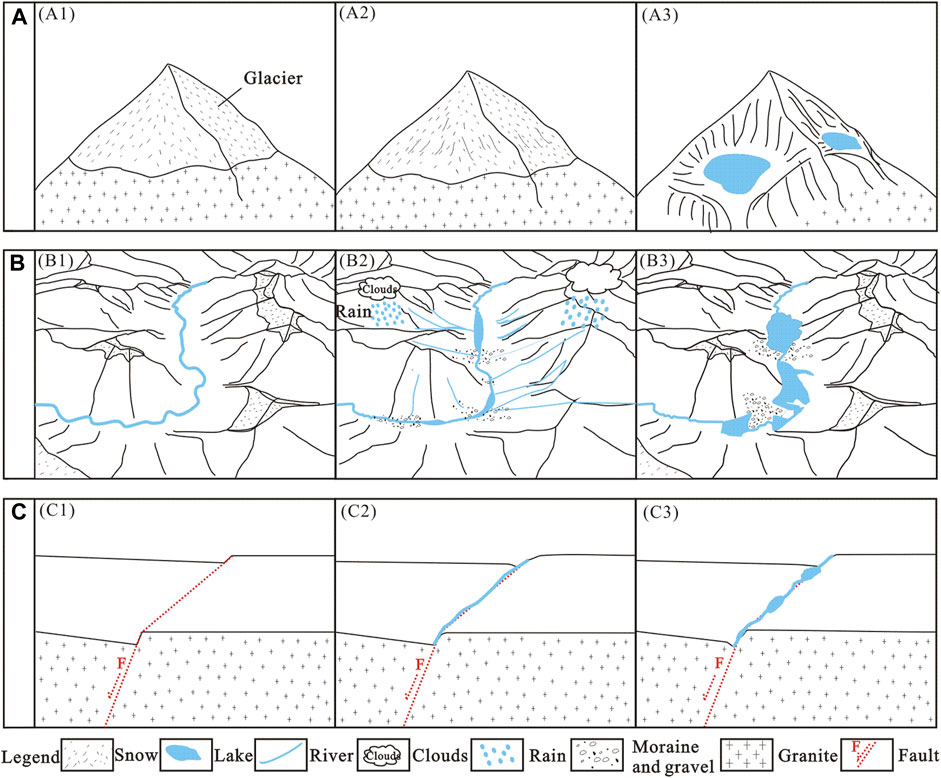
FIGURE 9. Schematic diagram of the development mechanism of glacial lakes. (A) Glacial erosion lake (B) Valley Lake (C) Tectonic Lake.
6 Conclusion
Based on Landsat images, this study extracted glacial lake data of the Niangmuco region on the eastern margin of Himalayan eastern tectonic structure, classified glacial lake by genetic type, and classified the stability of glacial lakes with areas larger than 0.02 km2. From the results, the following conclusions were reached.
(1) The study area has 126 glacial lakes with a total area of approximately 10.94 km2. Four genetic types of glacial lakes were identified in the study area, namely, glacial erosion lakes, valley lakes, tectonic lakes, and landslide dam lakes. Glacial erosion lakes are mainly developed at high altitude, valley lakes are developed at low-lying areas in the middle, and the distribution of tectonic lakes is consistent with the development direction of faults. The analysis of multi-temporal glacial lake change revealed that the total area of glacial lakes in this region increased from 10.53 km2 in 2000 to 10.94 km2 in 2021, an increase of 0.41 km2. Nevertheless, glacial lake area decreased from 2000 to 2010, probably due to climate fluctuations, and increased significantly from 10.09 km2 to 10.94 km2 after 2010. The overall trend of changes in glacial lakes in the study area is consistent with that of the Tibetan Plateau region, but with some regional variability. Meanwhile, glacial erosion lakes are the largest contributor to the change of glacial lake area, and the development and change characteristics of glacial lakes are mainly controlled by temperature and precipitation.
(2) The classification results of the stability of the glacial lakes show that 95.7% of the glacial lakes in the region are relatively stable and stable, and only 2 extremely unstable and 2 unstable glacial lakes were identified. The main factor controlling the stability of glacial lakes is the potential for rock avalanches within the watershed area, while tectonic faulting also increases instability.
(3) According to the comprehensive analysis, different types of glacial lakes in the region have different development mechanisms and processes. The development of glacial erosion lakes is mainly controlled by the process of glacier development and flow, and the development of valley lakes is controlled by the amount of glacial meltwater and the watershed area, which are associated with climatic factors. The formation of tectonic lakes is mainly controlled by faults and seismic activities, and the development of landslide dam lakes is also mainly controlled by tectonic activity and climate change.
Data availability statement
The original contributions presented in the study are included in the article/supplementary material, further inquiries can be directed to the corresponding author.
Author contributions
YW: Conceptualization, Data curation, Formal Analysis, Investigation, Methodology, Visualization, Writing–original draft, Resources, Validation. LJ: Writing–review and editing, Conceptualization, Funding acquisition, Methodology, Project administration, Supervision, Formal Analysis. XM: Writing–review and editing, Conceptualization, Supervision, Funding acquisition. ZL: Writing–review and editing.
Funding
The author(s) declare financial support was received for the research, authorship, and/or publication of this article. This study was supported by the Geological Survey Project of China Geological Survey (Grant No. DD202330249) and the National Natural Science Foundation of China (42272222).
Acknowledgments
The authors would like to thank all the reviewers who participated in the review and MJEditor for its linguistic assistance during the preparation of this manuscript. This paper has benefited from valuable comments and suggestions by Jiarui Mao whose efforts are gratefully acknowledged.
Conflict of interest
The authors declare that the research was conducted in the absence of any commercial or financial relationships that could be construed as a potential conflict of interest.
Publisher’s note
All claims expressed in this article are solely those of the authors and do not necessarily represent those of their affiliated organizations, or those of the publisher, the editors and the reviewers. Any product that may be evaluated in this article, or claim that may be made by its manufacturer, is not guaranteed or endorsed by the publisher.
References
Aggarwal, S., Rai, S. C., Thakur, P. K., and Emmer, A. (2017). Inventory and recently increasing GLOF susceptibility of glacial lakes in Sikkim, Eastern Himalaya. Geomorphology 295, 39–54. doi:10.1016/j.geomorph.2017.06.014
Allen, S. K., Zhang, G., Wang, W., Yao, T., and Bolch, T. (2019). Potentially dangerous glacial lakes across the Tibetan Plateau revealed using a large-scale automated assessment approach. Sci. Bull. 64, 435–445. doi:10.1016/j.scib.2019.03.011
Bai, H., Dong, W., and Ma, Z. (2004). Climatic characteristics of qinghai-xizang Plateau and its surrounding. Plateau Meteorol. 23, 890–897.
Bajracharya, B., Shrestha, A. B., and Rajbhandari, L. (2007). Glacial Lake outburst floods in the sagarmatha region. Mt. Res. Dev. 27, 336–344. doi:10.1659/mrd.0783
Begam, S., Sen, D., and Dey, S. (2018). Moraine dam breach and glacial lake outburst flood generation by physical and numerical models. J. Hydrol. 563, 694–710. doi:10.1016/j.jhydrol.2018.06.038
Bolch, T., Peters, J., Yegorov, A., Pradhan, B., Buchroithner, M., and Blagoveshchensky, V. (2011). Identification of potentially dangerous glacial lakes in the northern Tien Shan. Nat. Hazard. 59, 1691–1714. doi:10.1007/s11069-011-9860-2
Byers, A. C., Rounce, D. R., Shugar, D. H., Lala, J. M., Byers, E. A., and Regmi, D. (2018). A rockfall-induced glacial lake outburst flood, Upper Barun Valley, Nepal. Landslides 16, 533–549. doi:10.1007/s10346-018-1079-9
Chang, M., Tang, C., and Dou, X. (2017). Mechanism and hazards of typical glacier-lake burst in Southeastern Tibet. South-to-North Water Transfers Water Sci. Technol. 15, 115–121. doi:10.13476/j.cnki.nsbdqk.2017.06.017
Chen, F., Zhang, M., Guo, H., Allen, S., Kargel, J. S., Haritashya, U. K., et al. (2021). Annual 30 m dataset for glacial lakes in High Mountain Asia from 2008 to 2017. Earth Syst. Sci. Data. 13, 741–766. doi:10.5194/essd-13-741-2021
Department of China Earthquake Administration protection (1995). Catalogue of historical strong earthquakes in China. Beijing: Earthquake Press.
Department of China Earthquake Administration protection (1999). Catalogue of modern Chinese earthquakes, 1912-1990. Beijing: China Science and Technology Press.
Ding, M. (2019). Data from: temperature and precipitation grid data of the Qinghai Tibet Plateau and its surrounding areas in 1998-2017. A Big Earth Data Platf. Three Poles. doi:10.11888/Meteoro.tpdc.270239
Ding, Y., Liu, R., Zhang, C., Tong, L., and Dong, J. (2022). Remote sensing monitoring of glacier and glacial lake changes in Yairu Zangbo Basin, Himalayas. Arid. Land Geogr. 45, 1870–1880. doi:10.12118/j.issn.1000–6060.2022.110
Ding, Y., Mu, C., Wu, T., Hu, G., Zou, D., Wang, D., et al. (2021). Increasing cryospheric hazards in a warming climate. Earth Sci. Rev. 213, 103500. doi:10.1016/j.earscirev.2020.103500
Dong, H., Xu, Z., Cao, H., Li, Y., Liu, Z., Li, H., et al. (2018). Comparison of eastern and western boundary faults of eastern himalayan syntaxis, and its tectonic evolution. Earth Sci. 43, 933–951. doi:10.3799/dqkx.2018.701
Du, G., Zhang, Y., Lv, W., Zhang, G., Zhou, C., and Guo, C. (2016). Landslide susceptibility assessment based on weighted information value model in southeast Tibet. J. Catastrophology 31, 226–234. doi:10.3969/j.issn.1000-811X.2016.02.042
Duan, H. Y., Yao, X. J., Zhang, D. H., Qi, M. M., and Liu, J. (2020). Glacial Lake changes and identification of potentially dangerous Glacial Lakes in the yi'ong Zangbo River Basin. Water 12, 538. doi:10.3390/w12020538
Dubey, S., and Goyal, M. K. (2020). Glacial Lake outburst flood hazard, downstream impact, and risk over the Indian Himalayas. Water Resour. Res. 56, 26533. doi:10.1029/2019wr026533
Emmer, A., Harrison, S., Mergili, M., Allen, S., Frey, H., and Huggel, C. (2020). 70 years of lake evolution and glacial lake outburst floods in the Cordillera Blanca (Peru) and implications for the future. Geomorphology 365, 107178. doi:10.1016/j.geomorph.2020.107178
Fan, J., An, C., Zhang, X., Li, X., and Tan, J. (2019). Hazard assessment of glacial lake outburst floods in Southeast Tibet based on RS and GIS technologies. Int. J. Remote Sens. 40, 4955–4979. doi:10.1080/01431161.2019.1577578
Haritashya, U. K., Kargel, J. S., Shugar, D. H., Leonard, G. J., Strattman, K., Watson, C. S., et al. (2018). Evolution and controls of large glacial lakes in the Nepal himalaya. Remote Sens. 10, 798. doi:10.3390/rs10050798
Hu, J., Yao, X., Duan, H., Zhang, Y., Wang, Y., and Wu, T. (2022). Temporal and spatial changes and GLOF susceptibility assessment of glacial lakes in Nepal from 2000 to 2020. Remote Sens. 14, 5034. doi:10.3390/rs14195034
Huang, C., Chang, L., and Ding, Z. (2021). Crustal anisotropy in the eastern Himalayan syntaxis and adjacent areas. Chin. J. Geophys. 64, 3970–3982. doi:10.6038/cjg2021P0034
Huang, J., Wang, C., Wang, G., and Zhang, C. (2005). Application of fuzzy comprehensive evaluation method in risk degree determination for ice-lake outburst—an example of luozha county in Tibet. Earth Environ. 33, 109–114. doi:10.14050/j.cnki.1672-9250.2005.s1.025
Khadka, N., Chen, X., Nie, Y., Thakuri, S., Zheng, G., and Zhang, G. (2021). Evaluation of Glacial Lake outburst flood susceptibility using multi-criteria assessment framework in mahalangur himalaya. Front. Earth Sci. 8, 601288. doi:10.3389/feart.2020.601288
Lei, P., Wang, W., and Zhang, T. (2022). Changes in glacial lakes in naqu from 1990 to 2020. J. Beijing Normal Univ. Sci. 58, 936–944. doi:10.12202/j.0476-0301.2021303
Lesi, M., Nie, Y., Shugar, D. H., Wang, J. D., Deng, Q., Chen, H. Y., et al. (2022). Landsat- and sentinel-derived glacial lake dataset in the China-Pakistan economic corridor from 1990 to 2020. Earth Syst. Sci. Data. 14, 5489–5512. doi:10.5194/essd-14-5489-2022
Li, D., Shangguan, D., and Huang, W. (2020a). Research on the area change of lake merzbacher in the tianshan mountains during 1998 - 2017. J. Glaciol. Geocryol. 42, 1126–1134. doi:10.7522/j.issn.1000-0240.2019.0308
Li, D., Shangguan, D. H., and Anjum, M. N. (2020b). Glacial Lake inventory derived from Landsat 8 OLI in 2016-2018 in China-Pakistan economic corridor. ISPRS Int. J. Geo-Inf. 9, 294. doi:10.3390/ijgi9050294
Li, H., Chen, H., Neupane, R., Ruan, H., and Li, X. (2021). Experimental stury on mechanism of moraine dam failure due to surge wave of glacier avalanches. Bull. Soil Water Conservation 41, 25–34+42. doi:10.13961/j.cnki.stbctb.2021.02.004
Li, S., Li, C., and Kang, X. (2021). Development status and future prospects of multi-source remote sensing image fusion. Natl. Remote Sens. Bull. 25, 148–166. doi:10.11834/jrs.20210259
Li, Z., Wang, X., Yu, Y., Zhang, H., Su, Q., Miao, X., et al. (2021). The impacts of base level and lithology on fluvial geomorphic evolution at the tectonically active Laohu and Hasi Mountains, northeastern Tibetan Plateau. Sci. China Earth Sci. 51, 906–919. doi:10.1007/s11430-020-9743-1
Liu, J., Cheng, Z., Li, Y., and Luo, X. (2009). A study of the outburst form of the end-moraine lake in Tibet. Earth Sci. Front. 16, 372–380.
Liu, J., Yao, X., Gao, Y., Qi, M., Duan, H., and Zhang, D. (2019a). Glacial lake variation and hazard assessment of glacial lakes outburst in the Parlung Zangbo River Basin. J. Lake Sci. 31, 1132–1143. doi:10.18307/2019.0420
Liu, J., Zhang, J., Gao, B., Li, Y., Li, M., Wujin, D., et al. (2019b). An overview of glacial lake outburst flood in Tibet, China. J. Glaciol. Geocryol. 41, 1335–1347. doi:10.7522/j.issn.1000-0240.2019.0073
Morris, M., West, M., and Hassan, M. (2018). A guide to breach prediction. Dams Reservoirs 28, 150–152. doi:10.1680/jdare.18.00031
Muneeb, F., Baig, S. U., Khan, J. A., and Khokhar, M. F. (2021). Inventory and GLOF susceptibility of glacial lakes in hunza River Basin, western karakorum. Remote Sens. 13, 1794. doi:10.3390/rs13091794
Nie, Y., Liu, Q., Wang, J., Zhang, Y., Sheng, Y., and Liu, S. (2018). An inventory of historical glacial lake outburst floods in the Himalayas based on remote sensing observations and geomorphological analysis. Geomorphology 308, 91–106. doi:10.1016/j.geomorph.2018.02.002
Nie, Y., Sheng, Y. W., Liu, Q., Liu, L. S., Liu, S. Y., Zhang, Y. L., et al. (2017). A regional-scale assessment of Himalayan glacial lake changes using satellite observations from 1990 to 2015. Remote Sens. Environ. 189, 1–13. doi:10.1016/j.rse.2016.11.008
Prakash, C., and Nagarajan, R. (2017). Outburst susceptibility assessment of moraine-dammed lakes in Western Himalaya using an analytic hierarchy process. Earth Surf. Process. Landforms 42, 2306–2321. doi:10.1002/esp.4185
Richardson, S. D., and Reynolds, J. M. (2000). An overview of glacial hazards in the Himalayas. Quat. Int. 65-6, 31–47. doi:10.1016/s1040-6182(99)00035-x
Rinzin, S., Zhang, G. Q., and Wangchuk, S. (2021). Glacial Lake area change and potential outburst flood hazard assessment in the Bhutan himalaya. Front. Earth Sci. 9, 775195. doi:10.3389/feart.2021.775195
Shi, Z., Zhou, M., Peng, M., Zhou, G., Zhang, L., and Cai, S. (2021). Research progress on overtopping failure mechanisms and breaching flood of landslide dams caused by landslides and avalanches. Chin. J. Rock Mech. Eng. 40, 2173–2188. doi:10.13722/j.cnki.jrme.2020.1188
Shugar, D. H., Burr, A., Haritashya, U. K., Kargel, J. S., Watson, C. S., Kennedy, M. C., et al. (2020). Rapid worldwide growth of glacial lakes since 1990. Nat. Clim. Change. 10, 939–945. doi:10.1038/s41558-020-0855-4
Stuart-Smith, R. F., Roe, G. H., Li, S., and Allen, M. R. (2021). Increased outburst flood hazard from Lake Palcacocha due to human-induced glacier retreat. Nat. Geosci. 14, 85–90. doi:10.1038/s41561-021-00686-4
Sun, X., Cao, B., Pan, B., Li, K., Zhao, X., and Guan, W. (2022). Identification of hazardous glacial lakes in the Yarlung Zangbo River Basin based on lakes changes determined using google Earth engine. Front. Earth Sci. 10, 825482. doi:10.3389/feart.2022.825482
Tang, M., Chen, H., Zhao, H., Yang, W., and Deng, W. (2023). Identification, development law and risk assessment of the hidden dangers of glacial lake oueburst disasters on the Qinghai-Tibet Plateau. Geol. Bull. China 42, 730–742. doi:10.12097/j.issn.1671-2552.2023.05.006
Tao, J., Zhao, W., Wang, X., and Xue, J. (2021). Spatial changes of the glacial lakes in the western Nyainqentanglha Range. Arid Zone Res. 38, 618–628. doi:10.13866/j.azr.2021.03.04
Thomas, C., Ranchin, T., Wald, L., and Chanussot, J. (2008). Synthesis of multispectral images to high spatial resolution: a critical review of fusion methods based on remote sensing physics. IEEE Trans. Geosci. Remote Sens. 46, 1301–1312. doi:10.1109/tgrs.2007.912448
Veh, G., Korup, O., Roessner, S., and Walz, A. (2018). Detecting Himalayan glacial lake outburst floods from Landsat time series. Remote Sens. Environ. 207, 84–97. doi:10.1016/j.rse.2017.12.025
Wang, Q., Wang, X., Lei, D., Yin, Y., Wei, J., and Zhang, Y. (2022). The interaction mechanisms between mountain glacier evolution and glacial lake development. J. Glaciol. Geocryol. 44, 1041–1052. doi:10.7522/j.issn.1000-0240.2022.0097
Wang, W., Yao, T., Gao, Y., Yang, X., and Kattel, D. B. (2011). A first-order method to identify potentially dangerous glacial lakes in a region of the southeastern Tibetan plateau. Mt. Res. Dev. 31, 122–130. doi:10.1659/mrd-journal-d-10-00059.1
Wang, X., Ding, Y., Liu, S., Jiang, L., Wu, K., Jiang, Z., et al. (2013). Changes of glacial lakes and implications in Tian Shan, central Asia, based on remote sensing data from 1990 to 2010. Environ. Res. Lett. 8, 044052. doi:10.1088/1748-9326/8/4/044052
Wang, X., Guo, X. Y., Yang, C. D., Liu, Q. H., Wei, J. F., Zhang, Y., et al. (2020). Glacial lake inventory of high-mountain Asia in 1990 and 2018 derived from Landsat images. Earth Syst. Sci. Data. 12, 2169–2182. doi:10.5194/essd-12-2169-2020
Wang, Y., Jin, J., and Yuan, R. (2019). Analysis on spatial distribution and influencing factors of geological disasters in southeast Tibet. J. Seismol. Res. 42, 428–437+456.
Wang, Y., Ren, G., Xia, M., Zhou, Y., and Qiu, J. (2016). Overview on stability of landslide dam. Chin. Chin. J. Geol. Hazard Control 27, 6–14. doi:10.16031/j.cnki.issn.1003-8035.2016.01.02
Wei, S., Xu, X., Wu, Y., Liang, S., and Luo, J. (2023). The present-day strain characteristics and dynamic mechanism around the Eastern Himalayan Syntaxis revealed by the GPS data. Acta Geol. Sin. 97, 1–15. doi:10.19762/j.cnki.dizhixuebao.2023140
Wu, G., Yao, T., Wang, W., Zhao, H., Yang, W., Zhang, G., et al. (2019). Glacial hazards on Tibetan Plateau and surrounding alpines. Bull. Chin. Acad. Sci. 34, 1285–1292. doi:10.16418/j.issn.1000-3045.2019.11.011
Yang, C., Wang, X., Wei, J., Liu, Q., Lu, A., Zhang, Y., et al. (2019). Chinese glacial lake inventory based on 3S technology method. Acta Geol. Sin. 74, 544–556. doi:10.11821/dlxb201903011
Yang, Z., Dong, W., Liu, J., and You, Y. (2021). Genetic types and distribution of glacial lakes in western Sichuan and eastern Tibet. Geol. Bull. China. 40, 2071–2079.
Yao, M., and Zhang, S. (1997). Fuzzy consistent matrix and its application. J. Syst. Eng. Electron. 8, 57–64.
Yao, X., Liu, S., Han, L., Sun, M., and Zhao, L. (2017). Definition and classification systems of glacial lakefor inventory and hazards study. Acta Geol. Sin. 72, 1173–1183. doi:10.11821/dlxb201707004
Zhang, D., Zhou, G., Li, W., Han, L., Zhang, S., Yao, X., et al. (2023). A robust glacial lake outburst susceptibility assessment approach validated by GLOF event in 2020 in the Nidu Zangbo Basin, Tibetan Plateau. Catena 220, 106734. doi:10.1016/j.catena.2022.106734
Zhang, G., Yao, T., Xie, H., Yang, K., Zhu, L., Shum, C. K., et al. (2020). Response of Tibetan Plateau lakes to climate change: trends, patterns, and mechanisms. Earth Sci. Rev. 208, 103269. doi:10.1016/j.earscirev.2020.103269
Zhang, G. Q., Yao, T. D., Piao, S. L., Bolch, T., Xie, H. J., Chen, D. L., et al. (2017). Extensive and drastically different alpine lake changes on Asia's high plateaus during the past four decades. Geophys. Res. Lett. 44, 252–260. doi:10.1002/2016gl072033
Zhang, G. Q., Yao, T. D., Xie, H. J., Wang, W. C., and Yang, W. (2015). An inventory of glacial lakes in the Third Pole region and their changes in response to global warming. Glob. Planet. Change. 131, 148–157. doi:10.1016/j.gloplacha.2015.05.013
Zhang, M. M., Chen, F., Zhao, H., Wang, J. X., and Wang, N. (2021). Recent changes of glacial lakes in the High Mountain Asia and its potential controlling factors analysis. Remote Sens. 13, 3757. doi:10.3390/rs13183757
Zhang, T., Wang, W., Gao, T., An, B., and Yao, T. (2022). An integrative method for identifying potentially dangerous glacial lakes in the Himalayas. Sci. Total Environ. 806, 150442. doi:10.1016/j.scitotenv.2021.150442
Keywords: glacial lakes, eastern margin of Eastern Himalayan Syntaxis, Landsat remote sensing images, fuzzy consistent matrix method, stability
Citation: Wei Y, Jia L, Ma X and Lei Z (2024) Development genetic and stability classification of seasonal glacial lakes in a tectonically active area—A case study in Niangmuco, east margin of the Eastern Himalayan Syntaxis. Front. Earth Sci. 12:1361889. doi: 10.3389/feart.2024.1361889
Received: 27 December 2023; Accepted: 02 February 2024;
Published: 16 February 2024.
Edited by:
Chong Xu, National Institute of Natural Hazards, ChinaReviewed by:
Ionut Cristi Nicu, Norwegian Institute for Cultural Heritage Research, NorwayHuiran Gao, National Institute of Natural Hazards, China
Copyright © 2024 Wei, Jia, Ma and Lei. This is an open-access article distributed under the terms of the Creative Commons Attribution License (CC BY). The use, distribution or reproduction in other forums is permitted, provided the original author(s) and the copyright owner(s) are credited and that the original publication in this journal is cited, in accordance with accepted academic practice. No use, distribution or reproduction is permitted which does not comply with these terms.
*Correspondence: Liyun Jia, MTU4OTQzNjUzQHFxLmNvbQ==