- 1Chengdu Technological University, Chengdu, China
- 2School of Geoscience and Technology, Southwest Petroleum University, Chengdu, China
- 3Yangtze University, School of Geoscience, Wuhan, China
The early Carboniferous Kushanhe Formation and Hantiereke Formation in the Kushanhe section in the piedmont of the southwestern margin of Tarim Basin are in a medium-deep water sedimentary environment with a stratigraphic thickness of more than 500 m, and the sedimentary facies is slope-shelf facies. Among these deposits, soft-sediment deformation structures (SSDS) are common, dominated by various types of slumps. The appearance of SSDS is related to the geotectonic environment of early Carboniferous in southwest Tarim Basin. In the Early Carboniferous, the Paleo-Tethys ocean dived under the Tarim plate, forming a back-arc structure, and a structural slope break belt easily appeared along the basin edge, which provided topographic conditions for the development of marginal platform marginal belt and slope facies. Under this background, the ultra-thick volcanic rocks of Wulate Formation accumulate along the subduction zone, and earthquakes with M≥ 5.0 are prone to occur. Landslides related to earthquakes and corresponding SSDS are easily triggered at the outer edge of the slope break zone. The large earthquake induced by tectonic environment is an important trigger factor of early Carboniferous SSDS. Therefore, the appearance of early carboniferous SSDS in Kushanhe section is an important sedimentary response to the early carboniferous piedmont tectonic activities in southwestern Tarim.
Introduction
Soft-sediment deformation structures (SSDS) have been a topic of interest in sedimentology in recent years. This term has been generally defined in the geological deformation of sediments as various structures which resulted from deformation before the still soft or plastic sediments were consolidated into rocks during their burial and diagenetic stages. A precise definition was offered by Qiao et al. (2017): SSDS are “deformation structures of the unconsolidated sediments at the top of the depositional sequence, products of synsedimentary or quasi-synsedimentary deformation, manifesting themselves macroscopically as small-thickness (there are documented cases of m-thick SSDS) deformation layers sandwiched between undeformed layers.” (Qiao et al., 2017). The recognition, genetic mechanisms, and related nomenclature of SSDS have been controversial. After G. Shanmugam’s article “The Seismite Problem” was translated into Chinese and published in the Journal of Paleogeography (Chinese version) in 2017, scholars such as Ji Shaocheng, Feng Zengzhao, Jiang Hanchao, Su Dechen and Qiao Xiufu made responses by bringing out related papers in the sixth issue of China Technology in 2018, which have greatly promoted the research on SSDS in China (Shanmugam, 2016; Dechen and Xiufu, 2018; Hanchao, 2018; Shaocheng, 2018; Zengzhao, 2018). Tarim basin has experienced multi-stage geological tectonic movement and evolution, and SSDS are widely developed in the basin, which is an ideal area for studying SSDS. This paper selects the Kushanhe section in the southwest of Tarim basin for research.
Late Devonian-Early Carboniferous continuous deposits were found in Kushanhe section in the southwest margin of Tarim Basin, and there were a large number of SSDS in Kushanhe Formation and Hantiereke Formation. During the early exploration in Tarim Basin, some scholars studied the early Carboniferous stratigraphic paleontology of this section (Tuci, 1986; Tang et al., 2022). After that, except Zhang et al. made some analysis on the genesis of the breccia limestone of Hantiereke Formation in Kushanhe section from the angle of sedimentary facies, there was little research on this section in the Early Carboniferous, especially on it. (Zhang et al., 2021). The inducement of SSDS is related to many endogenous and exogenous geological processes, such as earthquake, storm wave and volcanic activity. Studying this profile is helpful to restore and rebuild the early Carboniferous paleoenvironment in southwest Tarim. SSDS is a sedimentary structure, which has many causes. “Seismic rock” is a genetic term, which refers to strata with soft sediment deformation structure caused by earthquake. Earthquake is only a cause of soft sediment deformation structure. Not all rocks including ssds are necessarily caused by earthquakes. The origin of soft sedimentary structure in Kushanhe section selected in this paper is caused by earthquake. For this reason, this study describes in detail the types and development characteristics of SSDS in the Early Carboniferous Kushanhe Formation and Hantiereke Formation, and makes an attempt to analyze, in combination with the regional geological background, the Late Devonian–Early Carboniferous paleoenvironment in southwest Tarim Basin. We hope that this research can inspire further studies on SSDS and on Early Carboniferous tectonic–sedimentary evolution in this region.
Regional geology
Tarim basin is located in the northwest of China, surrounded by Tianshan-Beishan orogenic belt, Altun orogenic belt and Kunlun orogenic belt. It is a large marine-continental superimposed basin developed on Precambrian metamorphic basement and the largest oil-bearing sedimentary basin in China. In the south, Tarnum Uplift has accumulated about 0.6 km of Altun strike-slip system. (Laborde et al., 2019; Tang et al., 2022). The southwest depression of the Tarim Basin is a first-order structural unit, with an area of about 12×104 km2. It is bounded by the Serikbuya-Mazartag fault to the northeast and by the South Tianshan orogenic belt to the north, and adjoined by the Tekilik uplift on the north margin of the Kunlun structural belt to the southwest. The depression can be structurally zoned into such second-order units as piedmont thrust nappe, Hotan–Kashi sag, and Makit Slope (Figure 1). The Kushanhe section in question, located on the piedmont thrust nappe structural belt, has been strongly affected by tectonic movement.
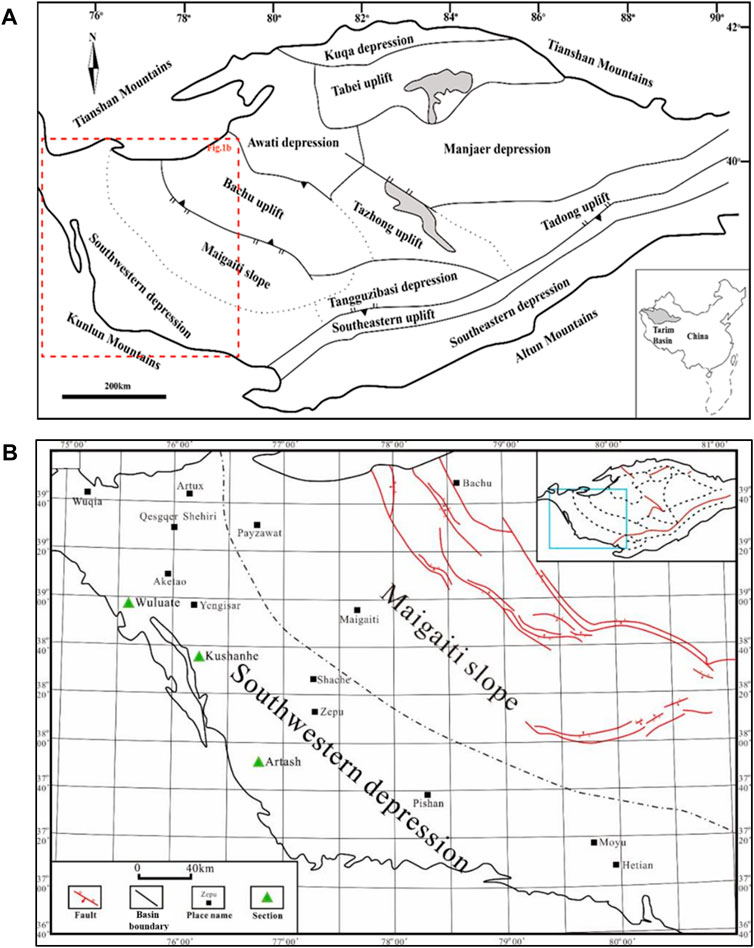
FIGURE 1. Geographical location map of Tarim Basin and location map of research area. (A) The map of tectonic divisions in Tarim Basin; (B) Location plan of the southwest margin of the Tarim Basin.
Characteristics of the carboniferous horizon in the Kushanhe section
The total thickness of the Early Carboniferous Kushanhe Formation is 1,500 m, and that of the Hantiereke Formation is 4,000 m, as shown in Figure 2 and Figure 3. Field observation indicates that in the lower zone of the Kushanhe Formation, grayish-green to dark-gray sandstone is alternated in varying thickness with silty mudstone, with glutenite visible at the bottom. In the middle zone, ash-black mudstone is alternated in varying thickness with yellowish-brown sandstone. In the upper zone, “mixed deposits” of sandshale and carbonatite lavas occur, with several, 1–2 m thick layers of limestone breccia, with several sets of breccia limestone visible, each 1–2 m thick. These sets of breccia limestone contain a lot of coral breccia such as Beichuanophyllum, Kueichoupora, Syringopora and Favositida, and brachiopods such as Cyrtospirifer and Tenticospirifer. The lithology of the Hantiereke Formation is mainly charcoal-gray micritic–microlitic limestone and micritc bioclastic limestone, which are generally alternated in varying thickness with thin layers of carbonaceous shale, siltstone and other clastic rocks. Sandwiched between the alternating layers are multiple sets of breccia limestone (altogether >200 m thick). The breccia is mostly composed of various coral fossils and brachiopods; the coral genus is much the same as that of the Kushanhe Formation, and the brachiopods are mainly Spirifer and Neospirifer. The Hantiereke Formation is in tectonic contact with the Jurassic sequence above. Overall, the Kushanhe section shows the sedimentary characteristics of the platform margin slope–continental shelf facies.
Types of soft-sediment deformation structures (SSDS)
The SSDS discussed in this paper belong to two main types: slides and slumps. Slides and slumps occurred when contemporaneous or penecontemporaneous semi-consolidated sediments moved downhill under gravity along rupture planes. Some scholars also refer to them as “mass-transport complexes” (Grimm and Orange, 1997; Khudoley and Guriev, 2003). Specifically, “slides” denote the downward movement of sediments as a whole, with a relatively planar shear surface at the bottom. From Figure 4C, there are traces of sliding, which are parallel to the direction of the current layer. During the process, the primary sedimentological features remained unaltered, thus they fall into the category of elastic deformation. “Slumps” signify the tumbling, crumpling, and crushing that occur within the mass of sediments during the downward movement, and thus are generally characterized by plastic deformation.
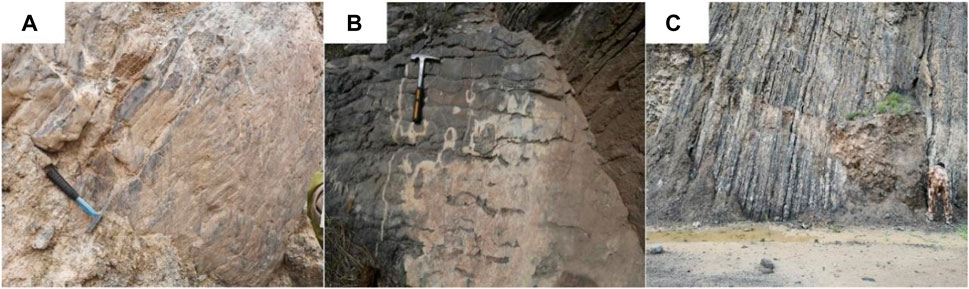
FIGURE 4. Carboniferous slide deformation sediments of the Kushanhe section (A) Gray micrite, with slight crumples across the entirety of the layer. Kushanhe Formation. (B) Gray micrite contains mudstone sandwiching, with layers slightly deformed, and small ruptures Locally visible. Hantiereke Formation. (C) Gray micrite, with slight crumples in separate layers. Kushanhe Formation.
Some slides occurred mainly in laminar micrite or marlstone in the middle zone of the Kushanhe Formation and the upper zone of Hantiereke Formation in the piedmonts of the West Kunlun Mountains. Overall, the layering of rocks is preserved, except for slight crumples (Figures 4A, B), local thinning, pinching out, interruption, cross-layer slight deformation, or small ruptures in some layers (Figure 4C).
There are extensively distributed slump deposits, ranging from <10 cm to 5–10 m in thickness, in the Kushanhe Formation–Hantiereke Formation. They often take various forms of SSDS, and tend to be layered and sandwiched between normal fine-grained layers (Figures 5A–D). In this paper, normal formation refers to the formation without SSDS. They have different appearance in different lithologic associations, which can be classified as follows.
(1) Alternating layers of sandstone with mudstone, which often occur in the lower zone of the Kushanhe Formation. In some cases, sand “ball-and-pillow structures” can be seen in silty mudstone; strong convolution and folding can be found along the bedding within the silty–fine-grained sandstone, thus forming “convolute bedding; ” and injectite dish structures are also common (Figure 5E). On the contact surface of sandstone and mudstone, load cast structures often occur on the basal surface of sandstone (Figure 5F). In the interior of mudstone can be found a plethora of irregular and plastically deformed “fluidized breccia” which are arranged along the bedding. Around the fluidized breccia, a large number of silty-sandy veins developed, some of which even “cut off” the breccia—an indicator that sand sediments exerted “flow emplacement” or “penetration” into silty mudstone when they had been liquefied from their still soft or semi-solid state during their post-depositiona stage. It can be seen in thin sections that some siltstone contains plastically-deformed syn-sedimentary irregular mudstone blobs or “rip-up clasts”. Moreover, there are plastically-deformed concentric ring structures within the mudstone rip-up clasts (Figures 6A, B). The bedding without slump structures is an “undisturbed” bed.
(2) Alternating layers of dark-colored marlstone or mudstone with breccia limestone, which commonly developed in the Kushanhe Formation upper zone–Hantiereke Formation lower zone (Figure 4B). The breccia limestone in section is >200 m thick, and the breccia is composed of a plethora of reef breccia (colonial and solitary corals) and various types of grainstones (Figures 5G, H). The breccia of colonial corals is relatively large in size, 30–40 cm in thickness, while solitary coral breccia is relatively small, about 5 cm in diameter and about 15–20 cm in length—an indicator that breccias are characterized by heterogeneous clast (fragment) sizes. And some of them have distinct independent or nearly independent lumps—pudding structures—resulting from plastic extensional deformation.
(3) Alternating thin layers of medium-grained sandstone with bioclastic micrite, which are locally distributed in the middle zone of the Hantiereke Formation. Scour–fill structures often developed in the interface between sandstone and carbonate rock, and a huge number of shells typically gathered near the scour surface. A myriad of liquefaction injectite vertically occurred in the sandstone layer, cut through the bedding (Figures 5I, J). In addition, some sandstone layers are rich in breccia and bands of micrite; the bands feature plastic tension deformation and assume an approximate linear shape (Figures 5K–L), similar to “plunged sediment mixture” structures (Qiao et al., 2017). In the thin sections of bioclastic micrite, there are usually a large number of terrigenous quartz particles, with an apparent characteristic of “mixed sediments” (Figures 6C, D). Also, a certain amount of lithic grains-clasts (Figure 5E) can be seen in sandstone, and the quartz particles in sandstone are often in directional arrangement (Figure 5F), indicating the directional scouring of water flow.
(4) Thin-bedded micrite and marl developed in the upper part of Hantiereke Formation. The original stratigraphic structure is generally intact, but the layers show plastic deformation to some extent, The breccia is poorly sorted, the gravel content is 30%–50%, and the whole breccia clasts display angular to sub-circular shape. The interstitial materials between the gravels include sand limestone and bioclastic limestone from high-energy sedimentary facies belt, mudstone and marl from low-energy facies belt, and shear sliding and curling deformation are found locally, and some of them have been extended or “ripped up” into breccia, lumps or strips which, in varying shapes and sizes, are intermittently distributed along their original layers (Figures 5M, N).
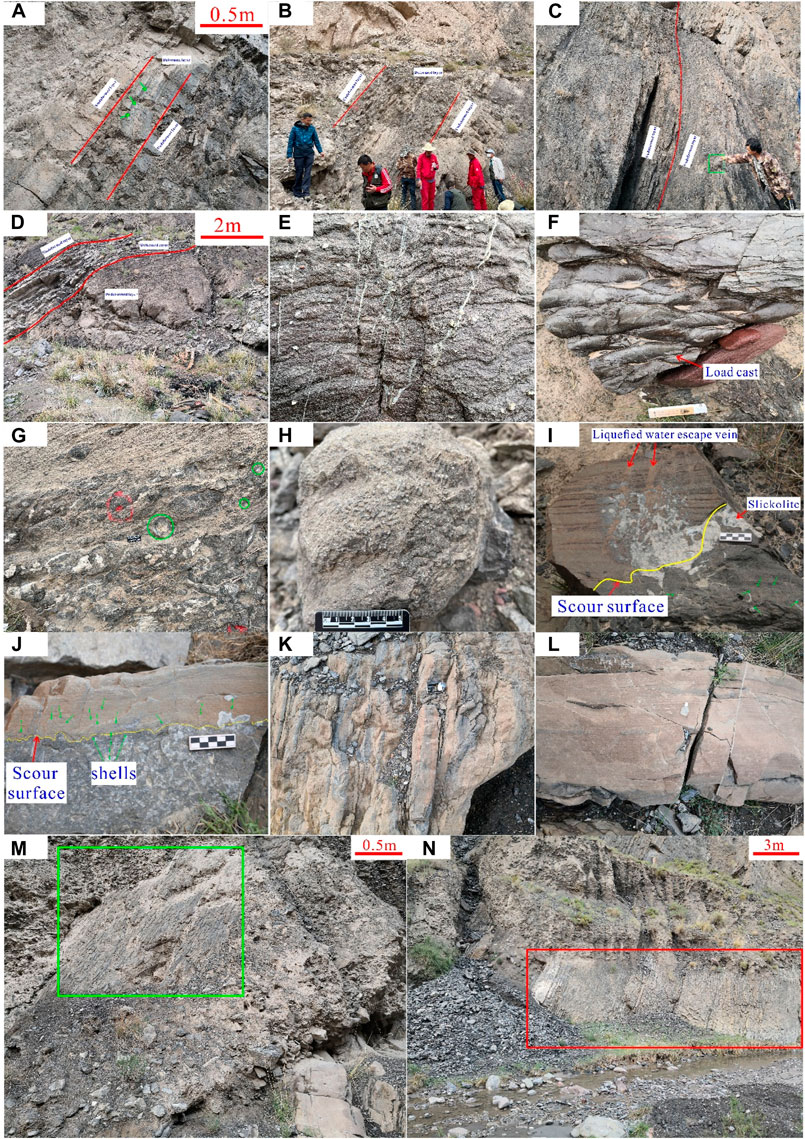
FIGURE 5. Carboniferous slump deposits in the Kushanhe section (A) Brownish-gray and gray mudstone contain thin–medium-thick sandwiching of silty–fine-grained sandstone, with a deformed layer of tens of centimeters thick and convolute bedding (indicated by the green arrow) visible. Kushanhe Formation. (B) Ash-black marlstone and micrite contain breccia limestone sandwiching, with breccia in the form of “lumps” and plastically deformed. Kushanhe Formation. (C) Ash-black marlstone contain breccia limestone sandwiching, with colonial coral breccia visible (shown in the green box). Kushanhe Formation. (D) Micrite contains breccia limestone sandwiching. Hantiereke Formation. (E) Gritstone containing breccia, with “dish structures” of liquefaction njectite origin. Kushanhe Formation. (F) Load cast structures of sandstone basal surface. Kushanhe Formation. (G) Breccia limestone, with breccia in directional arrangement, and “imbricate” structures. Hantiereke Formation. (H) Breccia of colonial corals. Hantiereke Formation. (I–L) Silty–fine-grained sandstone and bioclastic micrite form thin alternating layers, commonly in scour-surface contact, with detailed “plunged sediment mixture” structures visible. A myriad of shells gather near the contact surface. Liquefaction njectite occur in the sandstone. Hantiereke Formation. (M, N) Thin layers of marlstone and micrite, with plastically deformed and “rip-up” breccia. Hantiereke Formation.
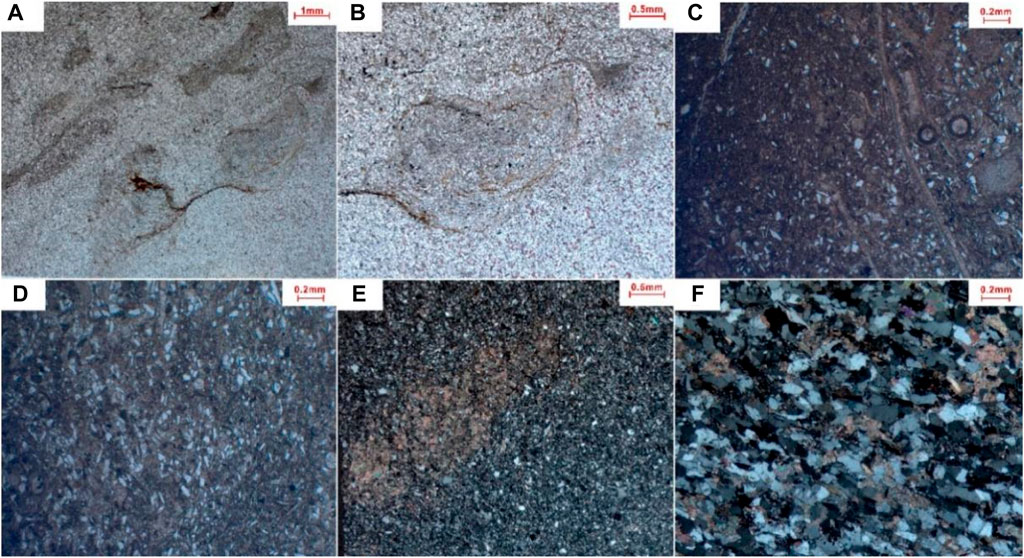
FIGURE 6. Micro characteristics of Early Carboniferous slump deposits in the Kushanhe section (A) Argillaceous siltstone, with argillaceous bands plastically deformed and broken into “breccia.” Kushanhe Formation. Plane polarized light. (B) A detailed photo of (A), with argillaceous “breccia” plastically contorted. Kushanhe Formation. Plane polarized light. (C, D) Bioclastic micrite, with a plethora of terrigenous quartz grains visible. Hantiereke Formation. Plane polarized light. (E) Siltstone, with carbonate clasts visible. Hantiereke Formation. Crossed polarized light. (F) Packstone, with quartz particles in distinct directional arrangement, and calcareous cement between particles. Hantiereke Formation. Crossed polarized light.
Discussion
Conventionally, SSDS have been believed to have the characteristics of “seismite” triggered by earthquake—a viewpoint still dominant in Chinese and international academic communities. However, as noted earlier, scholars such as Shanmugam proposed that there are at least 21 triggering mechanisms (volcanic eruptions, earthquakes, storm waves, cyclones, depositional loading, etc.) for SSDS (Shanmugam, 2016). In other words, taking SSDS as an indicator of paleo-earthquake is problematic. To decide whether the Early Carboniferous SSDS was seismogenic, we have to consider both the characteristics of the SSDS and the regional geotectonic background against which they occurred.
Depositional characteristics of soft-sediment deformation structures
Based on the above description of the Early Carboniferous SSDS of the Kushanhe section, typical SSDS are drawn (Figure 7).
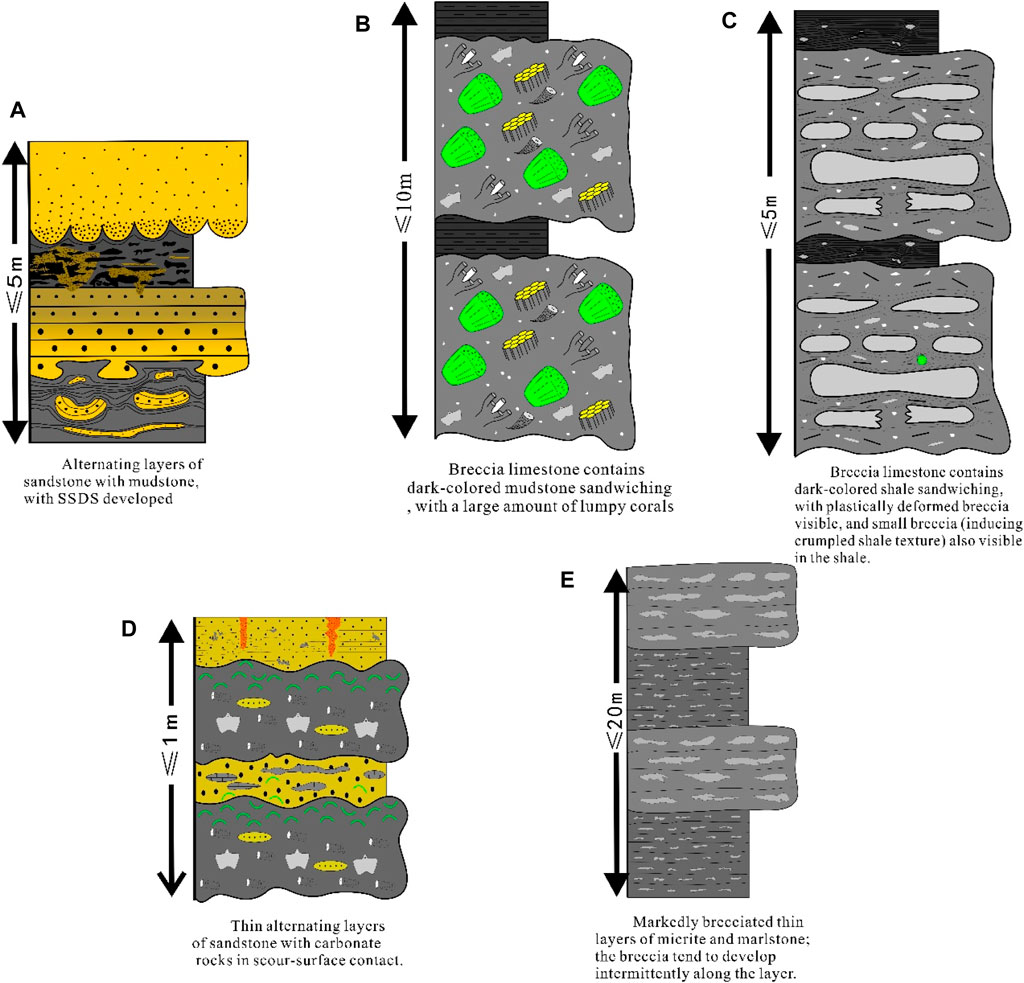
FIGURE 7. Typical facies associations of Carboniferous soft-sediment deformation structures (SSDS) in the Kushanhe section. (A) Alternating layers of sandstone with mudstone,with SSDS developed; (B) Breccia limestone contains dark-colored mudstone sandwiching, with a large amount of lumpy corals; (C) Breccia limestone contains dark-colored shale sandwiching, with plastically deformed breccia visible, and small breccia (inducing crumpled shale texture) also visible in the shale; (D) Thin alternating layers of sandstone with carbonate rocks in scour-surface contact; (E) Markedly brecciated thin layers of micrite and marlstone; the breccia tend to develop intermittently along the layer.
As mentioned earlier, there are a large number of poorly sorted corals and solitary coral breccias in carbonate rocks of the Early Carboniferous. Carbonate rocks are usually mixed with terrigenous quartz, sand and interlayer, which shows the characteristics of gravity flow deposition. Macroscopically, breccias are usually arranged in imbricate shape (Figure 7), indicating that traction current played a role in the development of breccia limestone. All these signs point to slope facies deposition. The appearance of colony coral shows that turbulent shallow water is warm, clean and light-transmitting. Yang et al. studied the Heshilafu Formation reef in Altas section at the southwest foot of Tarim Basin (Yang et al., 2014). They found that in the mid–late Viséan period of the Early Carboniferous, when the west–east transgression occurred in piedmonts, a platform margin facies belt began to develop, and discontinuous clusters of point reefs emerged along the belt—a phenomenon consistent with the recovery of the metazoan skeleton reefs in the mid–late Visean period across the globe. Also, “slope skirt” deposits appeared parallel to the belt. It follows that the breccia limestone rich in coral fragments was the product of the process in which the platform margin reefs were broken and then slumped along the slopes of the front reefs under external forces (Gong et al., 2021).
Probable genetic background
According to previous studies, in the Carboniferous period, when the piedmonts of southwest Tarim Basin used to be a passive continental margin, the Paleo-Tethys Ocean emerged; then in the Early Permian, when the ocean began its subduction beneath the Tarim block, the piedmonts evolved into an active continental margin (Jia, 1999; Jia and Wei, 2002). But it is worth noting that super-thick volcanic rocks (including pillow basalt and rhyolite) developed in the Early Carboniferous Wuluate Formation in the northwest piedmonts. Zircon dating in recent years suggests that their age is 343 Ma, roughly occurring in the early–middle period of the Early Carboniferous Viséan. The basalt shows the geochemistry of island are volcanics, and thus has been speculated to be formed in the back-arc basin whose emergence was related to the process of subduction. The forming of acidic lava is likely to be related to the detachment of subducting slabs after collision, or to the lithospheric mantle delamination and asthenospheric upwelling (Yun et al., 2015). It then follows that, in the Early Carboniferous, the Paleo-Tethys Ocean crust had begun its subduction beneath the Tarim plate, and a back-arc rift basin settled in the piedmonts. Kang Lei et al., who explored the Late Paleozoic magmatism in the north section of the West Kunlun Mountains, also suggested that, in 339–291 Ma (Carboniferous Viséan–Early Permian Asselian), a back-arc basin came into existence in the north part of the piedmonts due to the northward subduction of the Branch I of south Tethys Ocean (Kang et al., 2015). It follows that since the Early Carboniferous Viséan, an active continental margin began to form in the piedmonts because the Paleo-Tethys Ocean crust was being subducted beneath the Tarim plate. In addition, according to the studies made by Ma Qing et al., under the impact of the back-arc rift basin, the piedmonts began to subside and underwent drowning since the Early Carboniferous, forming a series of stepped normal faults and “east–west inclining” paleotopography (Qing et al., 2019). Against this tectonic background, slope break belts were prone to develop in the transitional zone between sea and land in the piedmonts, with rimmed carbonate platforms on the inner side and slope–shelf facies sediments on the outer side.
However, according to current seismological research, subduction zones are often where M≥5.0 earthquakes occur (Ruff and Kanamori, 1980; Fan et al., 2019; Zhao and Wu Zh, 2020; Wirth et al., 2022), and the earthquakes are likely to trigger submarine slope failure and slides, leading to gravity flow deposits (Shan et al., 2022). Therefore, based on the realistic principle of “the present is the key of the past”, the Early Carboniferous subduction zone from the Paleo-Tethys Ocean to the Tarim plate was prone to host M≥5.0 earthquakes. Taking into account the super-thick volcanic rocks in the Wuluate area, it is very likely that a “volcano–earthquake zone” was extensively stretched along the piedmonts of the southwest Tarim Basin. In this tectonic scenario, submarine slides were easily triggered in the slope break zones in the piedmonts. Moreover, based on previous studies which have documented that only SSDS induced by M≥ 5.0 earthquakes can be preserved, the Early Carboniferous SSDS in the Kushanhe section have probably been triggered by major earthquakes (Galli, 2000; Qiao et al., 2017). According to the characteristics of soft sedimentary structure and the evolution characteristics of plate tectonics in this region, these large earthquakes are easy to cause submarine earthquake landslides along the slope break zone, and further demonstrate the appearance of early Carboniferous SSDS in Kushanhe section in the southwest foothills of Tarim Basin, the product of the combined action of east-west inclined paleotopography induced by the tectonic background at that time and large earthquakes Figure 8.
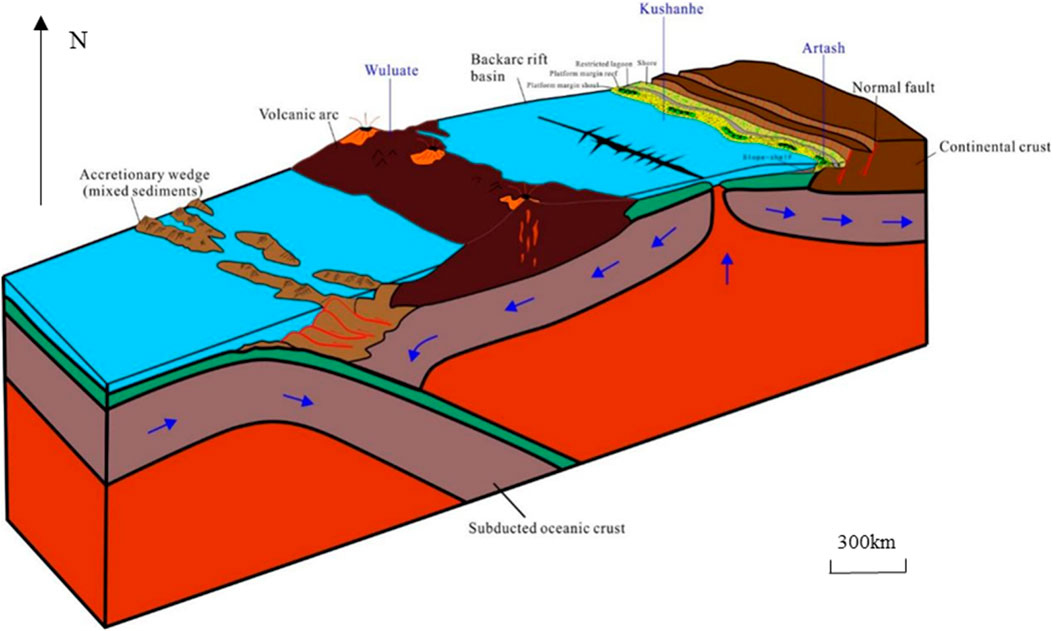
FIGURE 8. Early Carboniferous tectonic–sedimentary response model in the piedmonts of the southwest Tarim Basin (adapted from Stanley and Donna (Chernicoff and Whitney, 1995)).
Seizure rock is seizure rock is the key rock record to record to record paleoseismic events in strata. The identification and development sequence of seismites are helpful to understand the tectonic background and regional geotectonic process of basin formation. Soft sediment deformation structure caused by paleoearthquakes, such as molar structure, microfossil fracture and liquefaction curl deformation, was identified in Kushanhe section in the southwest of Tarim Basin, and typical seismites, seismic turbidites, moraines and thin calcareous mudstones were developed, and two periods of seismicity sequences, deep-water turbidity current deposition-ice-water mixing-earthquake activity and background deposition-earthquake activity, were formed in the upper part of Nanhua system (Maltman, 1994; Morley, 2003; Shi et al., 2021).
The genetic mechanism of soft sediment deformation structure is mainly based on other factors. Soft sediment deformation structure is divided into load structure, convolute bedding, deformation staggered layer, slump structure, drainage and sediment crowding structure, collapse structure and deformation structure formed by biological and chemical actions. The triggering factors of soft sediment deformation structure are various, but the deformation caused by the liquefaction of sediments caused by earthquakes is the main one. Therefore, the causes of soft sediment deformation structure can be identified in three steps: sedimentary facies analysis, trigger factor evaluation and standard evaluation.
Conclusion
(1) In the Early Carboniferous Kushanhe and Hantiereke Formations in the piedmonts of the southwest Tarim Basin, there developed semi-deep to deep water deposits, among which SSDS extensively occurred. Field observations and microscopic examinations reveal that they have the dual appearance of gravity flow and traction flow, which are actually the characteristics of platform margin slope facies deposits. Moreover, they manifest different appearance in different lithologic associations.
(2) The Paleo-Tethys Ocean plate began its subduction, plate began its northward subduction, beneath the Tarim plate, which led to the shaping of a back-arc rift basin against the extensional background where the piedmonts of the present southwest Tarim Basin are located. In this scenario, a series of stepped normal faults arose along the margin of the rift basin. Consequently, the piedmonts began to sink, and a transgression occurred from west to east. The tectonic setting was favorable for structural slope break zones to take shape. Those zones, have dual emplacement processes of gravity flow and traction flow, which are typical of platform margin, slope facies deposits.
(3) At the same time, in the Early Carboniferous, a “volcanic earthquake zone” was formed in the southwest margin of Tarim plate. It is very likely that a large earthquake with M≥5.0 will occur, which will easily lead to submarine earthquake landslides along the slope break zone. When determining the origin of early Carboniferous SSDS in Kushanhe section, we cannot rule out the factors of seismic activity. The east-west inclined paleotopography produced by geotectonics formed landslides or collapses under the action of major earthquakes, and finally formed early Carboniferous SSDS.
Data availability statement
The original contributions presented in the study are included in the article/Supplementary material, further inquiries can be directed to the corresponding author.
Author contributions
YW: Conceptualization, Data curation, Investigation, Methodology, Project administration, Software, Supervision, Writing–original draft, Writing–review and editing. GY: Conceptualization, Data curation, Investigation, Methodology, Software, Supervision, Writing–original draft, Writing–review and editing. ZW: Data curation, Methodology, Supervision, Validation, Visualization, Writing–original draft, Writing–review and editing. SL: Formal Analysis, Investigation, Resources, Software, Writing–original draft, Writing–review and editing.
Funding
The author(s) declare that no financial support was received for the research, authorship, and/or publication of this article.
Acknowledgments
The authors would like to show sincere thanks to those techniques who have contributed to this research.
Conflict of interest
The authors declare that the research was conducted in the absence of any commercial or financial relationships that could be construed as a potential conflict of interest.
Publisher’s note
All claims expressed in this article are solely those of the authors and do not necessarily represent those of their affiliated organizations, or those of the publisher, the editors and the reviewers. Any product that may be evaluated in this article, or claim that may be made by its manufacturer, is not guaranteed or endorsed by the publisher.
References
Chernicoff, S., and Whitney, D. (1995). Geology: an introduction to physical geology. Available at: https://cir.nii.ac.jp/crid/1130000798383525632.
Dechen, S., and Xiufu, Q. (2018). Discussion on the term of seismite and its Chinese translations. China Termin. 20 (6), 39. doi:10.3969/j.issn.1673-8578.2018.06.007
Fan, X., Scaringi, G., Korup, O., West, A. J., van Westen, C. J., Tanyas, H., et al. (2019). Earthquake-induced chains of geologic hazards: patterns, mechanisms, and impacts. Rev. Geophys. 57 (2), 421–503. doi:10.1029/2018rg000626
Galli, P. (2000). New empirical relationships between magnitude and distance for liquefaction. Tectonophysics 324 (3), 169–187. doi:10.1016/s0040-1951(00)00118-9
Gong, E. P., Huang, W. T., Guan, C. Q., Zhang, Y. L., Wang, L. F., Yang, Z. Y., et al. (2021). The coupled relationship between Carboniferous reefs and the Late Paleozoic ice age. Acta Geol. Sin. 95 (6), 1671–1692.
Grimm, K. A., and Orange, D. L. (1997). Synsedimentary fracturing, fluid migration, and subaqueous mass wasting; intrastratal microfractured zones in laminated diatomaceous sediments, miocene monterey formation, California, u.s.a. J. Sediment. Res. 67. doi:10.1306/d42685e5-2b26-11d7-8648000102c1865d
Hanchao, J. (2018). Whether Should the Term Seismites Be Abandoned: Discussions Based on the Recent Advance of Limnic Seismology in the Eastern Tibetan Plateau. China Termin. 20 (6), 44.
Jia, C., and Wei, G. (2002). Structural characteristics and petroliferous features of Tarim Basin. Chin. Sci. Bull. 47, 1–11. doi:10.1007/bf02902812
Jia, C. Z. (1999). Structural characteristics and oil/gas accumulative regularity in Tarim Basin. Xinjiang Pet. Geol. 20 (3), 177–183.
Kang, L., Xiao, P., and Gao, X. (2015). Neopaleozoic and Mesozoic granitoid magmatism and tectonic evolution of the western West Kunlun Mountains. Geol. China 42 (3), 533–552.
Khudoley, A. K., and Guriev, G. A. (2003). Influence of syn-sedimentary faults on orogenic structure: examples from the neoproterozoic-mesozoic east siberian passive margin. Tectonophysics 365 (1–4), 23–43. doi:10.1016/s0040-1951(03)00016-7
Laborde, A., Barrier, L., Simoes, M., Li, H., Coudroy, T., Van der Woerd, J., et al. (2019). Cenozoic deformation of the Tarim Basin and surrounding ranges (Xinjiang, China): A regional overview. Earth-Science Rev. 197, 102891. ISSN 0012-8252. doi:10.1016/j.earscirev.2019.102891
Morley, C. K. (2003). Subsurface sediment mobilization. Bangalore, India: Geological Society Special Publication.
Qiao, X. F., Li, H. B., Su, D. C., He, B. Z., Tian, H. S., Guo, X. P., et al. (2017). Soft sediment deformation structures-earthquake and ancient earthquake records.
Qing, M. A., Tao, M. A., Haijun, Y. A. N. G., Xueqin, Z. H. A. O., and Yiqing, Z. H. U. (2019). Development characteristics of the third-order sequence of Upper Devonian–Lower Carboniferous shore-mixed shelf in Tarim Basin, NW China. Petroleum Explor. Dev. 46 (4), 701–710. doi:10.1016/s1876-3804(19)60227-2
Ruff, L., and Kanamori, H. (1980). Seismicity and the subduction process. Phys. Earth Planet. interiors 23 (3), 240–252. doi:10.1016/0031-9201(80)90117-x
Shan, Z., Wu, H., Ni, W., Sun, M., Wang, K., Zhao, L., et al. (2022). Recent technological and methodological advances for the investigation of submarine landslides. J. Mar. Sci. Eng. 10 (11), 1728. doi:10.3390/jmse10111728
Shanmugam, G. (2016). The seismite problem. J. Palaeogeogr. 5 (4), 318–362. doi:10.1016/j.jop.2016.06.002
Shi, J., Huang, W., and Shang, J. (2021). Discovery of Neoproterozoic seismite in the northwest margin of Tarim Basin and its geological significance. Geol. Sci. 56 (3), 758–769.
Tang, Y., Ye, X., Wang, X., Huang, X., Hou, Z., Hu, K., et al. (2022). A synthesis of Carboniferous stratigraphy in Xinjiang, Northwest China with emphasis on regional and global correlations. J. Asian Earth Sci. 241, 105476. doi:10.1016/j.jseaes.2022.105476
Tuci, C. (1986). The Strata of the Early Stage of Early Carboniferous Hebukehe Formation and Kushanhe Formation in Xinjiang. Xinjiang Geol. 4 (4), 60–66.
Wirth, E. A., Sahakian, V. J., Wallace, L. M., and Melnick, D. (2022). The occurrence and hazards of great subduction zone earthquakes. Nat. Rev. Earth Environ. 3 (2), 125–140. doi:10.1038/s43017-021-00245-w
Yang, H. Q., Shen, J. W., Yang, H. J., Zhang, L. J., Li, M., and Wang, J. P. (2014). Mixed carbonate-clastic facies in the Eocene Kalatar Formation of the southwest Tarim Basin (NW China): tectonic and climatic controls. Facies 60, 111–131. doi:10.1007/s10347-013-0373-1
Yun, J., Gao, X., and Xiao, P. (2015). Geochemical characteristics of the Lower Carboniferous volcanic rocks of the Wuluate Formation in the Western Kunlun Mountains and their geological significance. Geol. China 42 (3), 587–600.
Zengzhao, F. (2018). Discussions on Seismites, “dizhenyan”, “zhenjiyan”, Soft-sediment Deformation Structures, etc. China Termin. 20 (6), 28.
Zhang, Z., Yang, X., Zhang, L., Wu, C., Luo, T., Zhu, W., et al. (2021). Sedimentation and mineralization of the Late Paleozoic extensional basin in the western Kunlun Mountains, China. Solid Earth Sci. 6 (2), 142–177. doi:10.1016/j.sesci.2021.02.001
Keywords: Kushanhe section, early carboniferous, soft-sediment deformation structures (SSDS), geodynamic setting, sedimentary response, southwest Tarim Basin
Citation: Wang Y, Yang G, Wang Z and Luo S (2024) Characteristics and geological significance of early carboniferous soft-sediment deformation structures in the Kushanhe section, southwest Tarim Basin, Northwest China. Front. Earth Sci. 12:1331539. doi: 10.3389/feart.2024.1331539
Received: 01 November 2023; Accepted: 22 January 2024;
Published: 23 February 2024.
Edited by:
Luca Aldega, Sapienza University of Rome, ItalyReviewed by:
Mattia Pizzati, University of Parma, ItalyMahmoud Leila, Mansoura University, Egypt
Alessio Lucca, University of Parma, Italy
Copyright © 2024 Wang, Yang, Wang and Luo. This is an open-access article distributed under the terms of the Creative Commons Attribution License (CC BY). The use, distribution or reproduction in other forums is permitted, provided the original author(s) and the copyright owner(s) are credited and that the original publication in this journal is cited, in accordance with accepted academic practice. No use, distribution or reproduction is permitted which does not comply with these terms.
*Correspondence: Yijun Wang, eHVqaW5Ac3dwdS5lZHUuY24=