- 1Department of Anatomy and Anthropology, Faculty of Medicine, Tel Aviv University, Tel Aviv, Israel
- 2The Shmunis Family Anthropology Institute, The Dan David Center for Human Evolution and Biohistory Research, Faculty of Medicine, Tel Aviv University, Tel Aviv, Israel
- 3The Department of Oral Biology, The Maurice and Gabriela Goldchleger School of Dental Medicine, Tel Aviv University, Tel Aviv, Israel
- 4Faculty of Health Sciences, Ben-Gurion University, Beer Sheva, Israel
- 5Clalit Research Institute, Innovation Division, Clalit Health Services, Ramat Gan, Israel
- 6Department of Physical Therapy, Zefat Academic College, Zefat, Israel
The calcaneal morphology changed considerably during human evolution to enable efficient bipedal locomotion. However, little information exists regarding its adaptation to changes in habitual activities following the transition to a sedentary lifestyle. We aimed to examine changes in calcaneal morphology during the Pleistocene-Holocene Levant in light of sexual dimorphism. We studied three archaic Homo sapiens calcanei dated to the Middle and Upper Paleolithic, 23 Natufian hunter-gatherers, 12 Pre-Pottery Neolithic early farmers, and 31 Chalcolithic farmers. The calcanei were scanned via a surface scanner and measured, and bone proportions were calculated. Measurements included the height, length, and width of various calcaneal elements. The sex of each individual was determined using methods based on calcaneal morphology. The validity of these methods was tested in those individuals who had the pelvis (92.3% agreement rate). Accordingly, the sample included 59.4% males and 40.6% females. Most calcaneal indices were sex-independent, except for the relative width, relative anterior length, and the cuboid index. Temporal trends between the Natufian and Chalcolithic periods were more pronounced among males than females. While in the proximal calcaneus, the temporal trend was similar between males and females, it differed in the distal part and articular facets. The calcanei of archaic H. sapiens exceeded the average of the Natufian hunter-gatherer for most variables, though the trend varied. To conclude, males and females were affected differently by the changing environment. The calcanei of archaic H. sapiens were better adapted for activity involving high mobility, independent of sex. During the transition to a sedentary way of life, different factors probably designed the male and female calcaneus. These could include factors related to the sexual division of labor, adaptation to lengthy standing, and changes in footwear.
1 Introduction
The study of skeletal remains enables the reconstruction of past populations’ daily life and physical loading since bones respond to mechanical loadings applied to them (Lieberman, 1997; Püschel and Benítez, 2014) and change their morphology to sustain these loads (Ruff et al., 2006; Macintosh et al., 2014). Accordingly, cross-sectional geometry analysis of femoral and tibial midshaft from human skeletal remains is among the most common methods for reconstructing physical loadings and mobility of past populations (Holt, 2003; Marchi et al., 2011; Macintosh et al., 2014; Macintosh et al., 2015; Ruff et al., 2015; May and Ruff, 2016). These studies suggested that bones’ cortical area and thickness, strength and rigidity, and shape expressed the temporal changes in physical loadings and mobility. Consequently, the transition to a sedentary way of life is considered one of the most significant socioeconomic revolutions that affected bone morphology due to major changes in daily life activities (Holt, 2003; Marchi et al., 2011; Macintosh et al., 2014; Ruff et al., 2015). Previous studies have suggested that adaptation to a sedentary lifestyle also affected morphology of the foot bones and their trabecular characteristics (Saers et al., 2019; Sorrentino et al., 2020; Harper, 2023).
The calcaneus offers a good candidate for studying changes in physical loading and mobility throughout time due to its major role in locomotion and weight-bearing, as it carries approximately 60% of body weight in static stance and 80% during the heel strike phase of normal gait (Donatelli, 1985; Vyce et al., 2010). Therefore, the adaptations of the calcaneus to the forces applied to it during human evolution and the transition to bipedal locomotion and upright stance are well established (Weidenreich, 1923; Aiello and Dean, 1990; Harcourt-Smith and Aiello, 2004; McNutt et al., 2018; DeSilva et al., 2019). Furthermore, the human calcaneus had to adapt to higher ground reaction force applied to it at heel strike (Latimer and Lovejoy, 1989). These adaptations included an asymmetric and convex cuboid facet, a broader and shorter calcaneal tuberosity, and a larger lateral plantar process, which was more plantarly positioned compared to apes (Bojsen-Møller, 1979; Latimer and Lovejoy, 1989; Boyle et al., 2018; Harper et al., 2021; Harper et al., 2022a). Furthermore, it has been suggested that human calcaneal morphology of shorter calcaneal tuber (body) improves running economy (Raichlen et al., 2011).
Previous studies have also demonstrated differences in calcaneal morphology between populations having different lifestyles. Accordingly, hunter-gatherers or non-sedentary populations had taller and wider posterior calcaneus, wider anterior and middle talar facets, a protuberance on the dorsal aspect of the bone, and their internal structure included higher bone volume fraction than sedentary groups (Raichlen et al., 2011; Weiss, 2012; Hoover and Colette Berbesque, 2018; DeMars et al., 2021; Harper et al., 2022b; Harper, 2023). Furthermore, it has been suggested that additional factors affected calcaneal morphology. For example, a decrease in calcaneal length between Medieval and post-Medieval populations was related to changes in footwear (Albee, 2022). Nevertheless, while the impact of human evolution and the transition to bipedal locomotion is well established (Weidenreich, 1923; Susman, 1983; Langdon, 1985; Latimer and Lovejoy, 1989; Harcourt-Smith and Aiello, 2004; Zipfel et al., 2011; Prang, 2015; McNutt et al., 2018; Oishi et al., 2018; DeSilva et al., 2019), data on modifications in calcaneal morphology within the Homo sapiens species over time and with economic behavior changes is scarce (Albee, 2022; Harper, 2023).
Among the known factors affecting calcaneal morphology is the sex of the individuals. Males have a significantly larger calcaneus than females, except for the angle between the anterior and middle talar articular surfaces (Steele, 1976; Riepert et al., 1996; Peckmann et al., 2015). These differences enabled the development of discriminant functions to estimate the sex based on calcaneal measures. The correct estimation rates achieved based on these equations varied between 64% and 95.7% (Steele, 1976; Riepert et al., 1996; Bidmos and Asala, 2003; Bidmos and Asala, 2004; Gualdi-Russo, 2007; DiMichele and Spradley, 2012; Kim et al., 2013; Alonso-Llamazares and Pablos, 2019). Nevertheless, the criteria for sex estimation based on calcaneal morphology is population-specific and should be carried out with caution (Gualdi-Russo, 2007; Alonso-Llamazares and Pablos, 2019).
The southern Levant is a geographical region rich in human fossils from the earliest expansion of Homo sapiens out of Africa (Hershkovitz et al., 2018) until recent times. This enables studying temporal changes in human skeletal remains within a well-documented and limited geographical region. It also reduces confounders related to landscape topography and environment. In addition, this region was among the first to experience major socioeconomic transitions that considerably changed the social organization and behavior of the people inhabiting the region, such as the “Agricultural (Neolithic) Revolution” (Belfer- Cohen and Goring-Morris, 2020; Shavit and Sharon, 2023). This transition occurred during the terminal Pleistocene-early Holocene period. Until the terminal Pleistocene, the populations inhabiting the Levant, i.e., during the Paleolithic period, were hunter-gatherers (Lieberman et al., 1993). Afterward, the subsistence strategy changed from relying on hunting and gathering to food production, facilitating the transition to a sedentary way of life. Subsequently, this transition resulted in significant changes in human lifestyle and behavior, which affected their daily physical activity patterns and loadings (Hershkovitz and Gopher, 1990; Bar-Yosef and Belfer-Cohen, 1991; May and Ruff, 2016). The Neolithization process was followed by the “Secondary Products Revolution” (e.g., exploitation of animals for dairy products, transportation, and power, i.e., their usage for plowing). While the exact timing of this revolution is debated (Vigne and Helmer, 2007), it is agreed that it occurred after the “Agricultural Revolution,” in the late Pottery Neolithic or Chalcolithic period (Gilead, 1988; Hershkovitz and Gopher, 1990) and resulted in an intensification of the sedentary lifestyle.
The “Agricultural Revolution” was initiated by the Natufians (15,000–11,600 cal. BP (Belfer-Cohen and Goring-Morris, 2011). Thus, the Natufians are considered the last hunter-gatherers of the Levant, they were nomadic or semi-sedentary, and characterized by living in small groups (Lieberman, 1991; Belfer-Cohen and Goring-Morris, 2011). Their hunting methods required high physical demands and necessitated the occupation of large hunting areas, similar to Paleolithic populations that preceded them (Bar-Yosef and Belfer-Cohen, 1989; Trinkaus and Ruff, 1999; Valla et al., 2002; Yeshurun et al., 2007). However, they were also engaged in cereal harvesting in the wild, food processing (pounding), and small-scale construction (e.g., rounded, low-walled structures) (Valla et al., 2002; Eshed et al., 2004; Belfer-Cohen and Goring-Morris, 2011; Yeshurun et al., 2014). A full sedentary lifestyle was achieved a few thousands of years later during the Pre-Pottery Neolithic (PPN) B period (10,500–8,250 cal. BP), though they still continued to hunt (Belfer-Cohen and Goring-Morris, 2011). The transition to a sedentary way of life was evident in the increased settlements’ size, construction of permanent houses with plastered floors, and installations for storage (Kuijt and Goring-Morris, 2002; Belfer-Cohen and Goring-Morris, 2011). Mortuary practices also changed, reflecting conceptual changes regarding territoriality and land ownership (Bar-Yosef and Belfer-Cohen, 1991). By the Chalcolithic period (6,500–5,500 cal. BP), the lifestyle was fully sedentary (Segal et al., 1998). The innovations in the early Holocene impacted personal and social life, triggering rapid population growth, territorial expansion, and the need for technological developments, most prominently metallurgy (Rowan and Golden, 2009). The occupation at this time was characterized by specialization and craftsmanship (e.g., farmers, miners, potters, and metalworkers) (Rowan and Golden, 2009). Despite the major cultural transformations during that time, the PPN populations are considered continuous with the Natufian ones (Lazaridis et al., 2016). Nevertheless, the late Chalcolithic population was an admixture of Anatolian Neolithic, Iran Chalcolithic, and Levantine groups, though their major component was Levantine (Harney et al., 2018). This ensures, to some level, a higher similarity between these groups compared to individuals originating from distant regions.
The major aim of this study was to reveal changes in calcaneal morphology in humans throughout time, focusing on the transition from a subsistence strategy based on hunting and gathering to farming. Since the morphology of the calcaneus is sexually dimorphic, we also aimed to reveal the differences between males and females in response to the changes in lifestyle. We hypothesized that the morphology of the calcaneus would change throughout time in both males and females, responding to the decrease in mobility and changes in the pattern of loadings (Harper, 2023). In addition, we hypothesized that the variance in morphology between males and females will decrease over time, i.e., reduced sexual dimorphism.
2 Materials and methods
2.1 Materials
The study population included 69 calcanei of archaic Homo sapiens (Middle Pleistocene, MP: Qafzeh H9) and modern humans from the Early Upper Paleolithic (EUP, Manot Cave), Late Upper Paleolithic (LUP, Ohalo H2), Natufians hunter-gatherers, PPN early farmers, and Chalcolithic farmers (Table 1). The study consisted of only adult calcanei according to the complete fusion of the calcaneal posterior tuberosity (Scheuer and Black, 2004; Schaefer et al., 2009; Ekizoglu et al., 2015) with no signs of trauma or pathologies. The sex of the individuals included in the study was estimated using previously published methods (see the “Methods” section).
All human skeletal remains included in this study are housed at the Dan David Center for Human Evolution and Biohistory Research, Faculty of Medicine, Tel Aviv University (TAU).
2.2 Methods
All calcanei were surface scanned via a Space Spider (Artec Europe, Luxembourg) high-resolution portable 3D scanner and aligned using Artec Studio software (v. 15 Professional, Artec Europe, Luxembourg).
2.2.1 Calcaneus measurements
Linear measurements of the calcaneus were calculated as the distance between two landmarks placed on the bone surface (Figure 1; Supplementary Table S1). Definitions of the measurements appear in Table 2 and visualized in Figure 1.
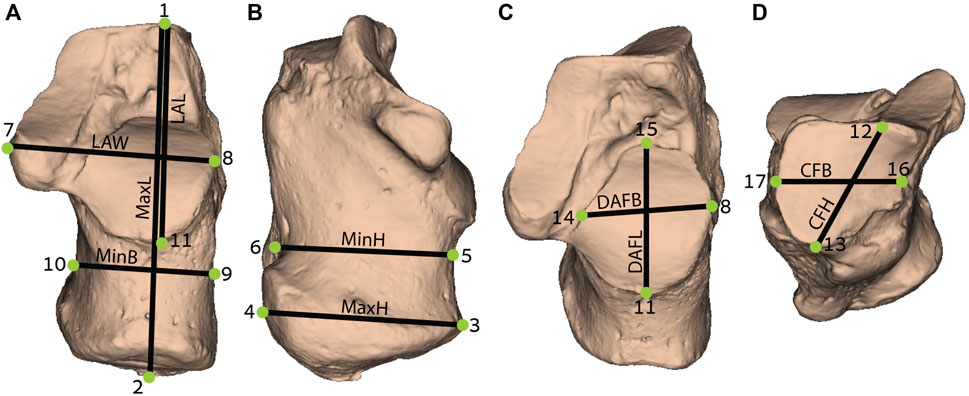
FIGURE 1. Measurements obtained from the calcaneus (definition of measurements are provided in Table 2): (A) Dorsal (superior) view; (B) Medial view; (C) Superoanterior view; and (D) Anterior view. MaxL, Maximum length; LAW, Load arm width; LAL, Load arm length; MinB, Minimum breadth; MaxH, Maximum height; MinH, Minimum height; DAFB, Dorsal articular facet breadth; DAFL, Dorsal articular facet length; CFB, Cuboid facet breadth; CFH, Cuboid facet height. For detailed landmarks’ position, refer to Supplementary Table S1.
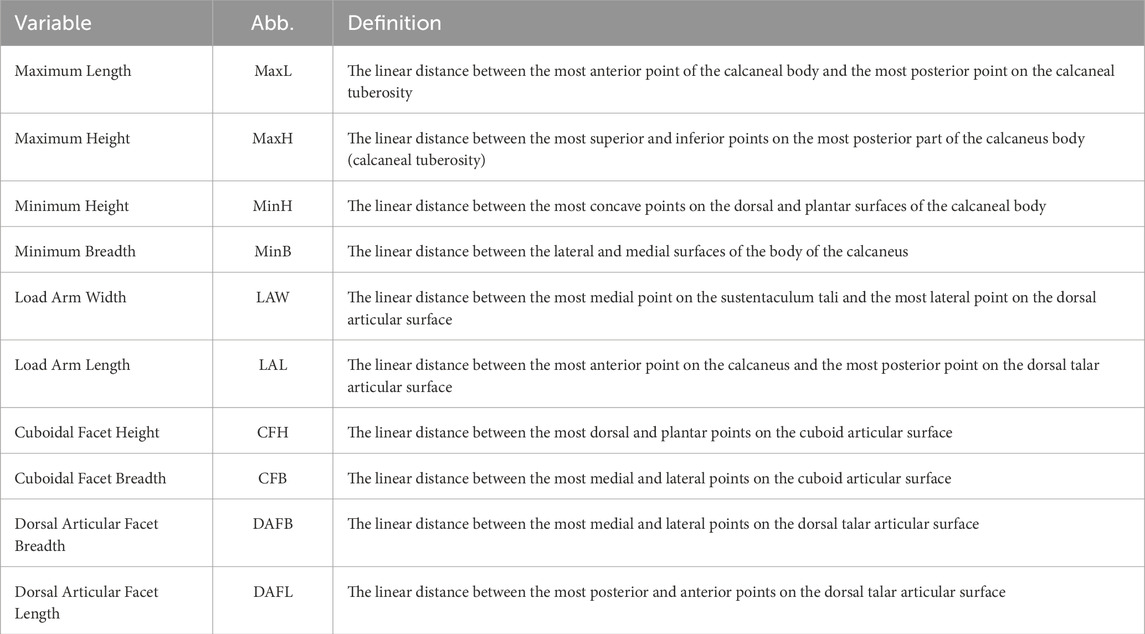
TABLE 2. Definition of calcaneal measurements following Murphy (2002), and Kim et al. (2013), which are adapted from Martin and Saller (1957) and Bräuer (1988).
2.2.2 Calcaneus proportions
To analyze the characteristics of the calcaneus independent of its size, we calculated ratios between various measurements (maximum height (MaxH); minimum height (MinH) and breadth (MinB); load arm length (LAL); and load arm width (LAW)) that were divided by the maximum length (MaxL) (Table 2). Additional indices were calculated between the measurements of the articular facets: dorsal articular facet breadth (DAFB)-to-dorsal articular facet length (DAFL) and cuboid facet breadth (CFB)-to-cuboid facet height (CFH), as these ratios are related to functionality in bipedal walking and running (Raichlen et al., 2011; Nozaki et al., 2020).
2.2.3 Sex estimation
Sex determination was carried out using four discriminant functions (Supplementary Table S2) (Gualdi-Russo, 2007; Zakaria et al., 2010; Spradley and Jantz, 2011; Alonso-Llamazares and Pablos, 2019), which are based on linear measurements of the calcaneus (Table 2). The final sex determination for each individual was based on the most frequent sex estimated per individual.
The four sex estimation functions included in our sample (out of 11 functions) had a high success estimation rate in the original study (>75%) and a high validity (>80%) in our sample. The latter was tested in a subsample of individuals having both the pelvis and the calcaneus (N=13). Hence, we examined the agreement rate of sex estimation between methods based on the pelvis and the calcaneus [(number of compatible results/total number of cases)*100] (Supplementary Table S2).
2.2.4 Statistical analysis
Statistical analyses were carried out via IBM SPSS (v. 22), Past software (V. 4.03), and R (v. 4.2.1). Significance level was determined at p<0.05. Reliability analyses were carried out to examine the reproducibility of the measurements by the same researcher as well as by a different one. Therefore, for intra-observer variation, the same researcher measured 14 calcanei twice with a week-long interval between measuring sessions. For inter-observer variation, an additional researcher measured the bones. Then, Intraclass correlation coefficient (ICC) analyses were carried out. The level of agreement was categorized according to Cicchetti (1994). Independent sample t-test or Mann-Whitney test (according to parameter distribution) were carried out to examine differences between males and females, and Kruskal-Wallis tests with multiple comparisons using Bonferroni correction to reveal differences between groups (only when N≥5).
3 Results
3.1 Reliability
Intra- and inter-observer variation of the measurements yielded excellent results (0.879≤ICC≤0.999) (Supplementary Table S3). Accordingly, data reproducibility is reliable either by the same researcher or by a different one.
3.2 Demography
The agreement rate of sex estimation using calcaneal morphometry yielded an excellent result of 92.3%. Accordingly, the study included 59.4% males and 40.6% females, with a varying male-to-female ratio between the periods (Table 3). The Natufian and PPN periods demonstrated a higher ratio of males to females (2 males: 1 female), whereas, in the Chalcolithic period, the ratio was approximately 1:1. Furthermore, Qafzeh H9 and Ohalo H2 were identified as males and Manot as female.
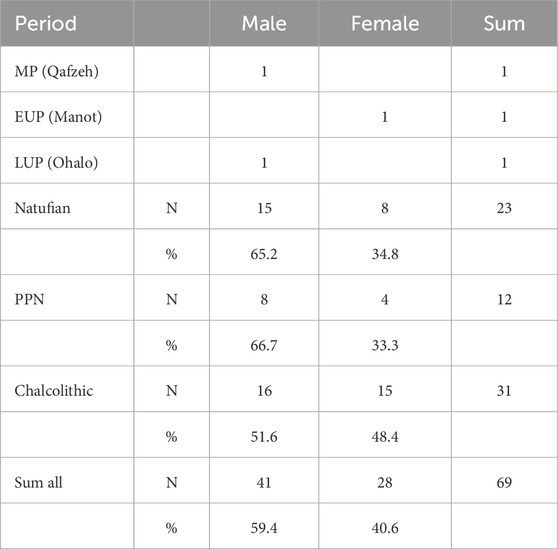
TABLE 3. Sex estimation based on calcaneal characteristics by period. See Supplementary Table S2 for discriminant functions used.
3.3 Sexual dimorphism in calcaneal morphology
Sex was estimated based on the sexual dimorphism of the calcaneus. Therefore, significant differences in calcaneal size existed for all measurements between males and females, with males having larger values than females (Table 4). However, for the indices, only several variables yielded significant results, with females manifesting larger values (Figure 2; Supplementary Table S4). Accordingly, the relative width (LAW/MaxL) and relative anterior length (LAL/MaxL) were larger in females than in males. The cuboid facet index (CFB/CFH) indicated that it was wider than taller in females and the opposite in males.
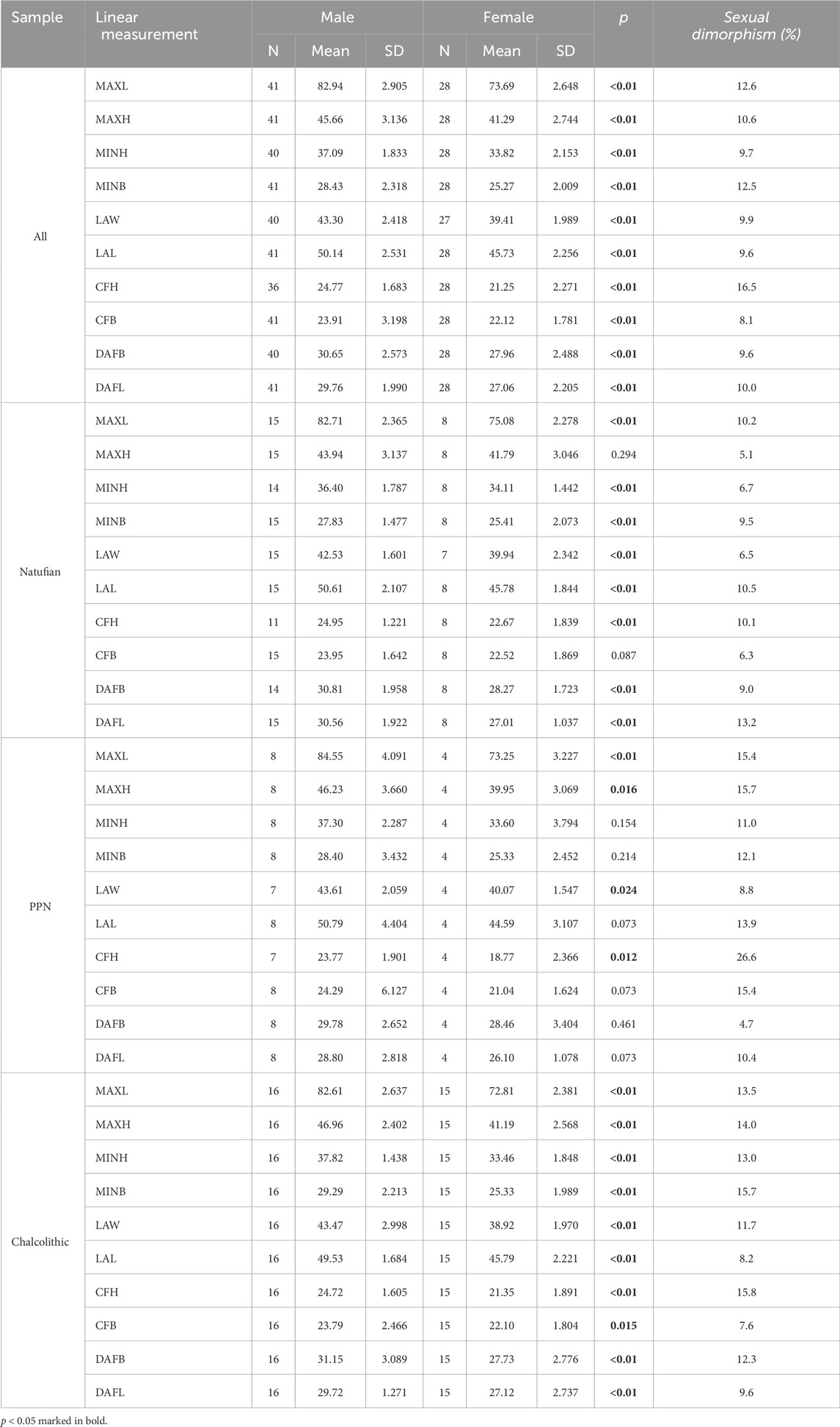
TABLE 4. Descriptive statistics and Mann-Whitney test to examine differences between males and females in calcaneal measurements. Sexual dimorphism ratea (%) was calculated for the entire sample and by period.
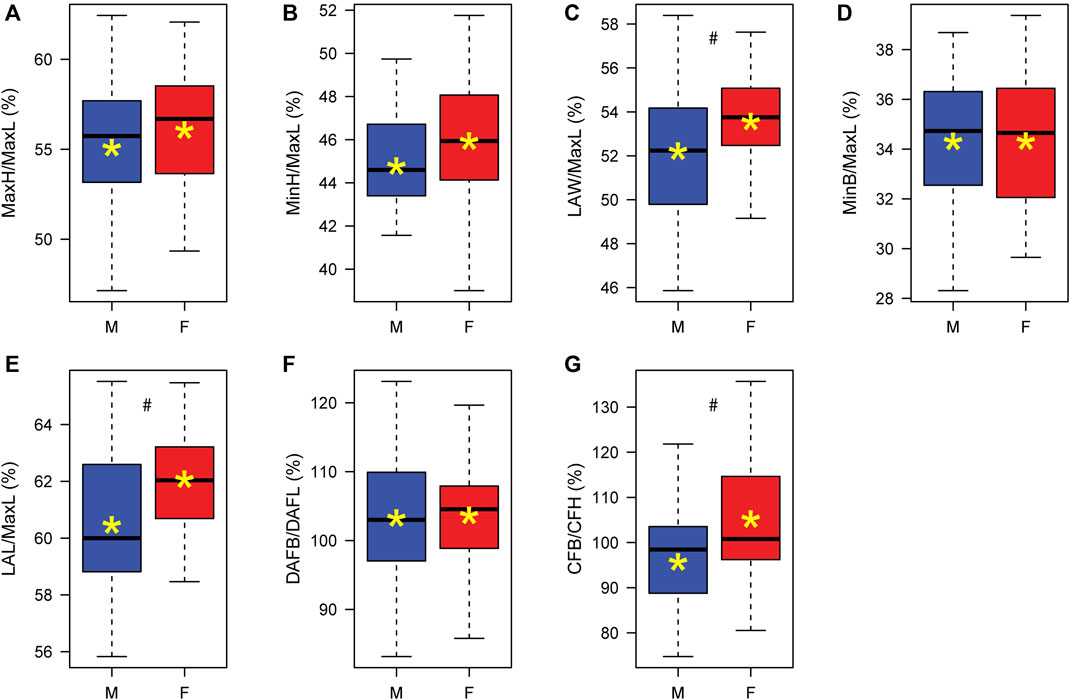
FIGURE 2. Boxplot of calcaneal indices in males (M) and females (F): (A) Maximum height divided by maximum length (MaxH/MaxL); (B) Minimum height divided by maximum length (MinH/MaxL); (C) Load arm width divided by maximum length (LAW/MaxL); (D) Minimum breadth divided by maximum length (MinB/MaxL); (E) Load arm length divided by maximum length (LAL/MaxL); (F) Dorsal articular facet breadth divided by Dorsal articular facet length (DAFB/DAFL); and (G) Cuboid facet breadth divided by cuboid facet height (CFB/CFH). *, Mean value; #, p < 0.05.
3.4 Changes in calcaneal size throughout time
Among males, no significant differences were found between the populations of the terminal Pleistocene-early Holocene (Table 5). However, on average, a tendency of increase was observed for most variables, including the MaxH and MinH, MinB, and LAW. However, at that time span, the LAL was reduced (Figure 3).
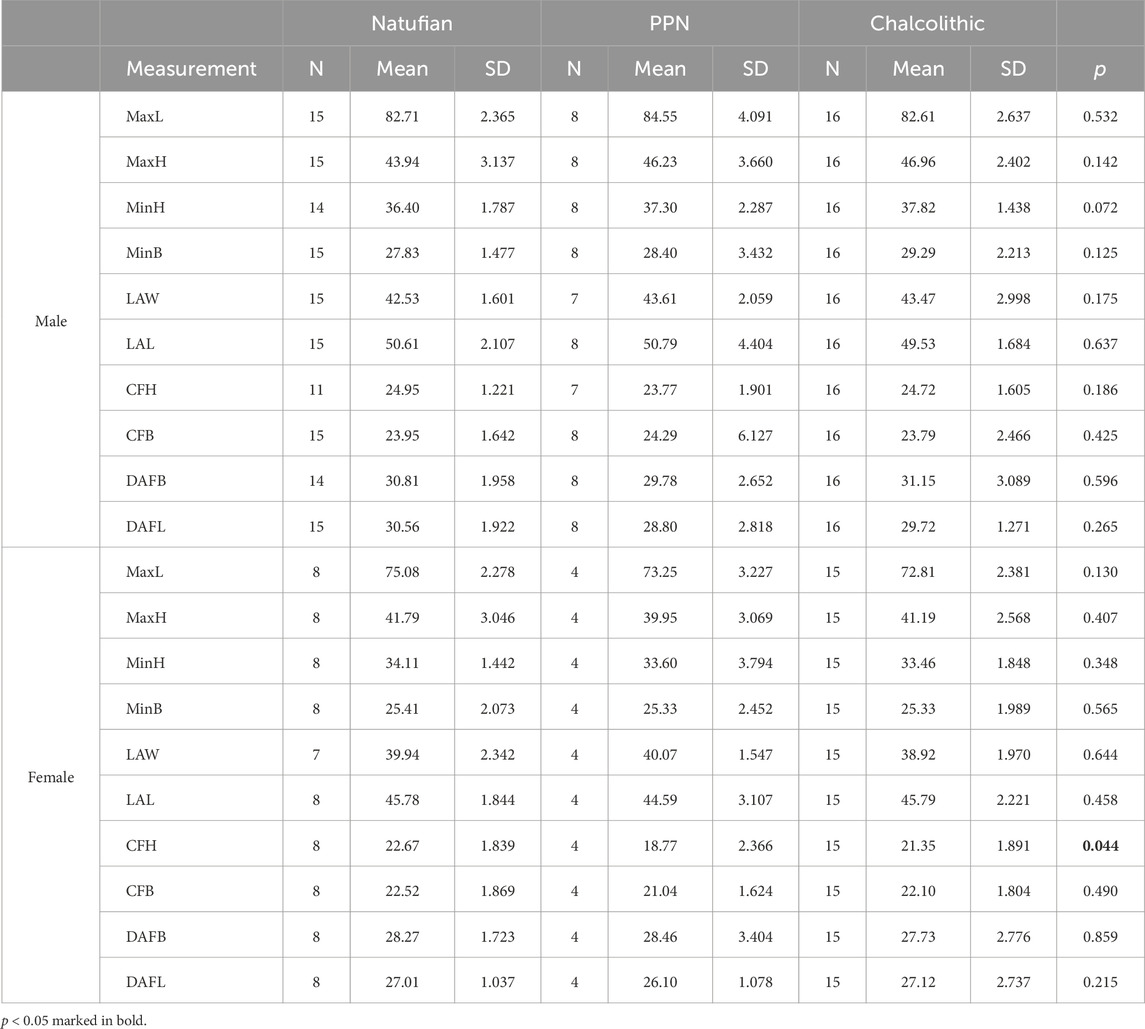
TABLE 5. Descriptive Statistics and Kruskal-Wallis test for examining differences in calcaneal size between periods by sex.
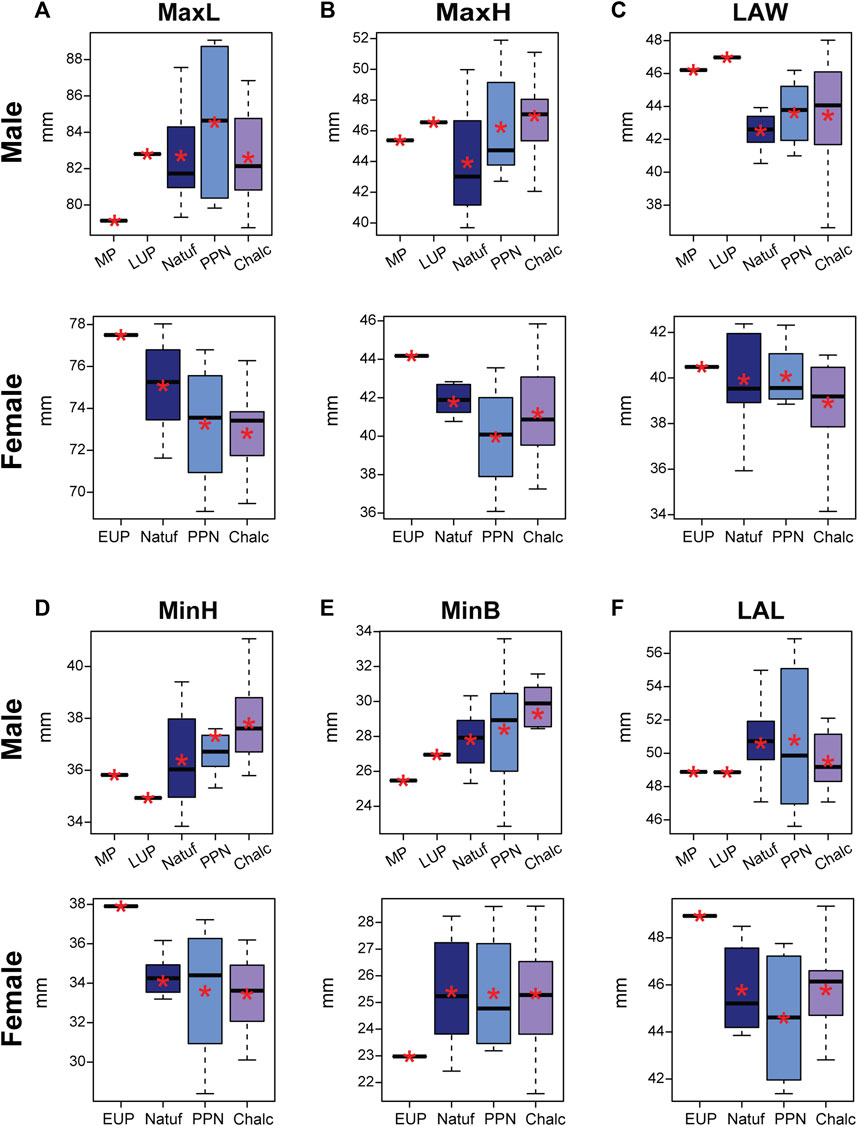
FIGURE 3. Boxplot of calcaneal linear measurements in males and females, by periods: Middle Paleolithic (MP); Early Upper Paleolithic (EUP); Late Upper Paleolithic (LUP); Natufian (Natuf); Pre-Pottery Neolithic (PPN) and Chalcolithic (Chalc). (A) Maximum length (MaxL); (B) Maximum height (MaxH); (C) Load arm width (LAW); (D) Minimum height (MinH); (E) Minimum breadth (MinB); and (F) Load arm length (LAL). *, Mean value.
Generally, the values obtained for the calcanei of Paleolithic H. sapiens were at the edges of the variance of the later samples. While Qafzeh H9 and Ohalo H2 were aligned with the temporal trend observed for the MinH, minB, and MaxL variables (i.e., smaller than the average value of the Natufians), they deviated from it for the LAW and LAL variables. Although their MaxH was not aligned with the trend observed between the later periods, it fell at the upper edge of the Natufian variance (Figure 3; Table 5). Accordingly, the Qafzeh calcaneus was shorter in total length, calcaneal body height (MinB), and LAL. Yet, its LAW was larger. The Ohalo H2 calcaneus for most variables demonstrated the same characteristics as those observed for Qafzeh H9, though for MaxL and MinB, it fell closer to the Natufian’s mean (Figure 3).
For most variables, except calcaneal MaxL, females demonstrated less clear trends from the terminal Pleistocene to the early Holocene, with no significant differences between the groups (except for CFH) (Figure 3; Table 5). The MaxL reduced throughout time, with the Manot calcaneus having the longest calcaneal body and the Chalcolithic females the shortest on average (Figure 3). Furthermore, Manot calcaneus exceeded the values of the later samples for most variables, demonstrating a calcaneus with a taller (MaxH and MinH) and narrower body (MinB) and longer LAL (Figure 3; Table 5). Notably, the sexual dimorphism of calcaneal size measures increased throughout time, especially between the Natufian and the two later groups (Figure 4; Table 4).
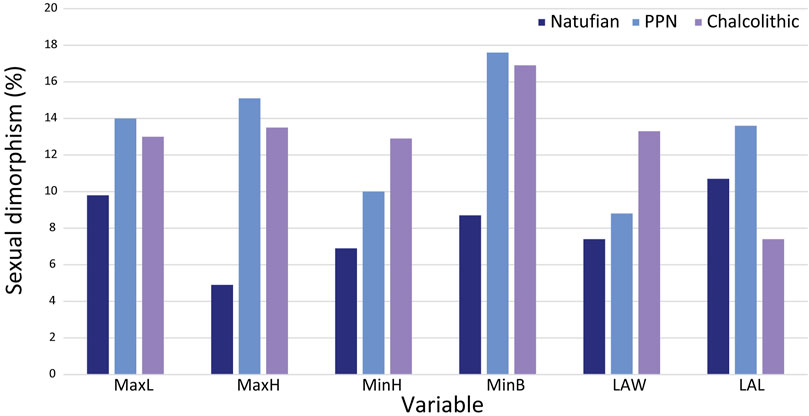
FIGURE 4. Sexual dimorphism (%) by calcaneal measurement and period. MaxL, Maximum length; MaxH, Maximum height; MinH, Minimum height; MinB, Minimum breadth; LAW, Load arm width; LAL, Load arm length.
In both sexes, no clear trend occurred throughout time in the size variables of the calcaneal articular surfaces (talar and cuboid facets). Nevertheless, the calcanei of Paleolithic H. sapiens fell outside the variation of Natufians. Their dorsal articular facet was narrower, yet while the DAFL of Qafzeh H9 and Ohalo H2 was shorter (29.1 mm and 26.7 mm, respectively), in Manot it was longer (30.5 mm) (Figure 5). The cuboid facet height, on the contrary, was longer in Qafzeh H9 and Ohalo H2 (27.2 mm and 28.1 mm, respectively) than that of the later samples, whereas the Manot one was shorter (18.4 mm), similar to the PPN average (Figure 5; Table 5). Moreover, relative to the later samples’ variation, the cuboid facet was narrower in the Qafzeh fossil yet wider in the Ohalo H2 and Manot specimens (20.3 mm, 25.6 mm, and 23.4 mm, respectively) (Figure 5; Table 5).
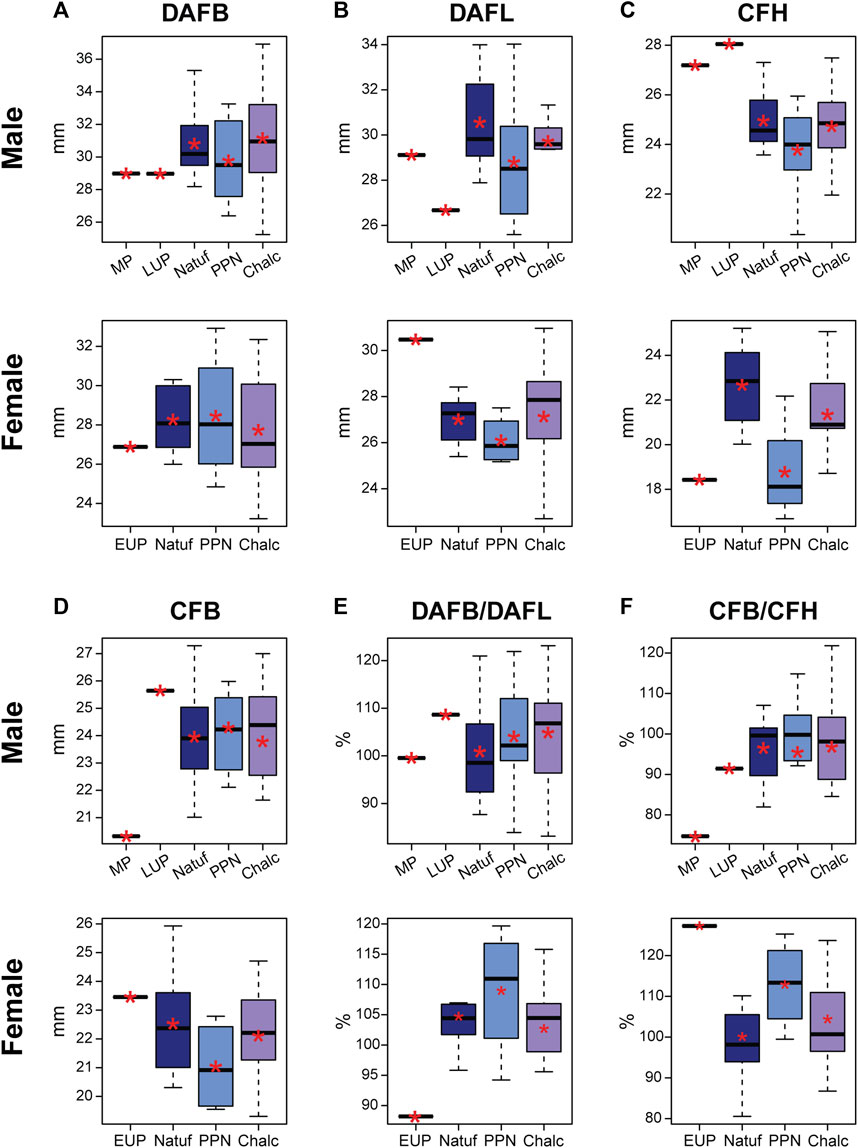
FIGURE 5. Boxplot of linear measurements and indices of the calcaneal articular surfaces in males and females by period: Middle Paleolithic (MP); Early Upper Paleolithic (EUP); Late Upper Paleolithic (LUP); Natufian (Natuf); Pre-Pottery Neolithic (PPN) and Chalcolithic (Chalc). (A) Dorsal articular facet breadth (DAFB); (B) Dorsal articular facet length (DAFL); (C) Cuboid facet height (CFH); (D) Cuboid facet breadth (CFB); (E) Ratio between the dorsal articular facet breadth and dorsal articular facet length (DAFB/DAFL); and (F) Ratio between breadth and height of the cuboid facet (CFB/CFH). *, Mean value.
3.5 Changes in calcaneal proportions throughout time
Throughout the terminal Pleistocene-early Holocene, a temporal increase in calcaneal proportions was observed in males, specifically in the proportions of MaxH, MinH, and MinB of the calcaneus. However, the LAL-to-MaxL ratio was reduced during this period (Figure 6). This implies that the calcaneal body became relatively taller, wider, and longer over time with a relatively taller calcaneal tuber.
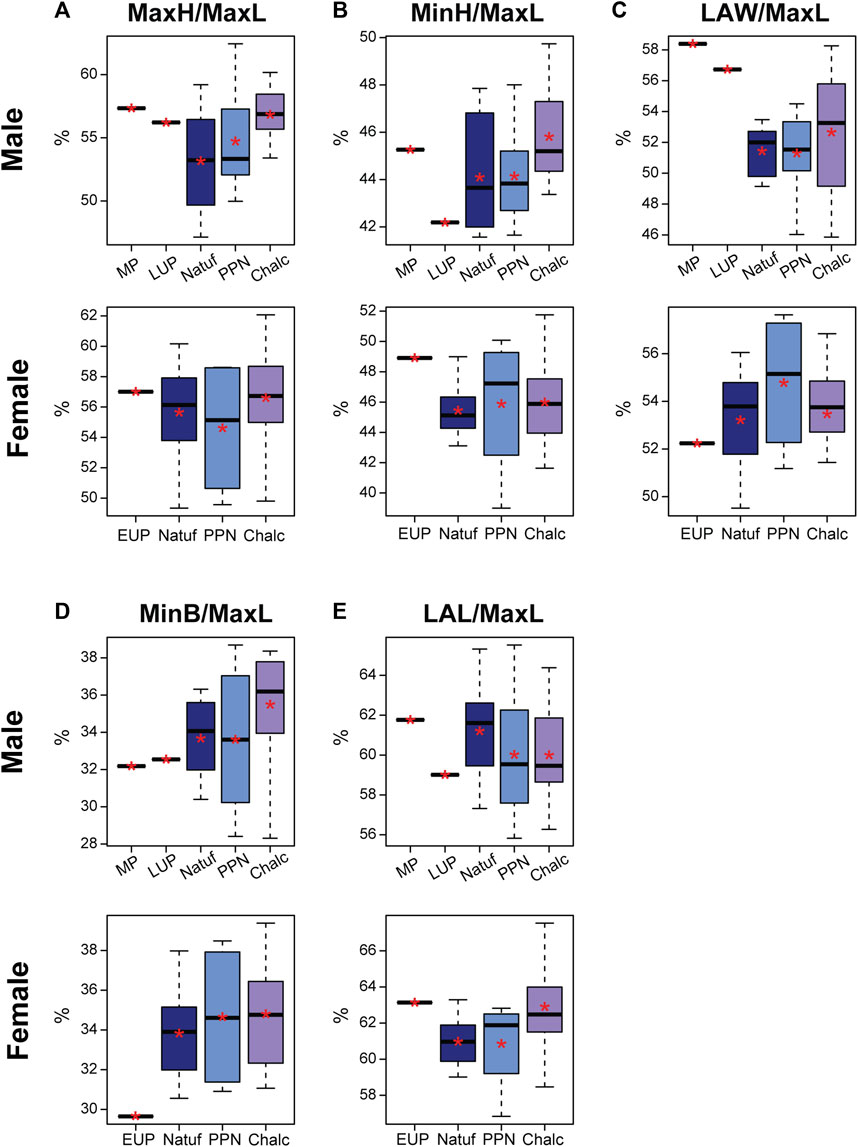
FIGURE 6. Boxplot of calcaneal indices in males and females by period: Middle Paleolithic (MP); Early Upper Paleolithic (EUP); Late Upper Paleolithic (LUP); Natufian (Natuf); Pre-Pottery Neolithic (PPN) and Chalcolithic (Chalc). (A) Maximum height divided by maximum length (MaxH/MaxL); (B) Minimum height divided by maximum length (MinH/MaxL); (C) Load arm width divided by maximum length (LAW/MaxL); (D) Minimum breadth divided by maximum length (MinB/MaxL); and (E) Load arm length divided by maximum length (LAL/MaxL). *, Mean value.
The MP calcaneus (Qafzeh H9) was aligned with these tendencies only for the MinB-to-MaxL and LAL-to-MaxL ratios (Figure 6), meaning that from a dorsal view, Qafzeh H9 calcaneal body was relatively narrower and shorter. Nevertheless, for the other measures, it exceeded the noted tendencies (Figure 6). Hence, the calcaneal body and tuber were relatively taller, and the distal calcaneus was relatively wider. However, the dorsal subtalar articular surface had proportions close to the Natufian’s mean. The cuboid articular facet exceeded later calcanei variance, demonstrating a vertical rectangular shape, while the Natufians and later calcanei were more quadrangular (Figure 5).
The LUP calcaneus was aligned with the observed tendencies for the MinH-to-MaxL and MinB-to-MaxL (Figure 6). Hence, the Ohalo H2 calcaneal body was relatively shorter and narrower than the average Natufian one. However, the other measurements exceeded these tendencies, suggesting a relatively wider distal calcaneus, a relatively taller calcaneal tuber, and relatively longer body (Figure 6). The dorsal subtalar articular surface was relatively wide (Figure 5). Nevertheless, the cuboid articular surface fell within the later periods’ variation, demonstrating an almost perfect quadrangular proportion (CFB/CFH=0.91) (Figure 5).
In females, trends in calcaneal proportions were more subtle from the terminal Pleistocene to the Holocene (Figure 6). These included a slight increase in the relative calcaneal body height and width and the relative distal calcaneal width. A more pronounced tendency was observed for the increase in the LAL-to-MaxL ratio (i.e., implying a decrease in calcaneal body length) (Figure 6; Supplementary Table S5). Manot calcaneus fell at the edge of the Natufian variance in most measurements, except for the relative MinB, which was smaller (Figure 6). However, the articular facets deviated from later periods variance, with the Manot DAFB being narrower relative to its length and the cuboid articular facets rectangular horizontally (Figure 5).
4 Discussion
This study followed temporal changes in calcaneal morphology during the terminal Pleistocene-early Holocene Levant. It also examined the morphology of earlier Paleolithic calcanei relative to the variation in these later populations. These analyses were carried out in light of changes in calcaneal sexual dimorphism. Notably, the sex of the Paleolithic specimens (i.e., Qafzeh H9 and Ohalo H2) was in accordance with some previous studies (Hershkovitz et al., 1995; Coutinho-Nogueira et al., 2021). While there is a consensus regarding the male sex of Ohalo H2, the sex of Qafzeh H9 is debated. We identified it as a male like many others (Rosenberg et al., 1988; Rak, 1990; Coqueugniot et al., 2000; Coutinho-Nogueira et al., 2021). However, few researchers suggested Qafzeh H9 was a female or probably female based on several specific characteristics of the pelvis and skull (Vandermeersch, 1981; Trinkaus, 1984). Following our results and others (Rosenberg et al., 1988; Rak, 1990; Coqueugniot et al., 2000; Coutinho-Nogueira et al., 2021), we relate to Qafzeh H9 as a male as his calcaneus fell within the male variance, similar to Ohalo H2, outside the female variation, and different from the Manot female calcaneus.
While a clear tendency was observed in calcaneal morphology throughout the terminal Pleistotcene-early Holocene among males, the modifications in females were more subtle. Nevertheless, the calcanei of Paleolithic H. sapiens for most measures did not follow the trend observed between the Natufian and Chalcolithic periods. The Paleolithic H. sapiens males had extreme values for most measures, yet they fell within the male variations (except for LAW/MaxL and cuboid facet measures and index). The Paleolithic H. sapiens female calcaneus from Manot fell outside the female variation in size. However, its proportions fell within their variation (except for the MinB/MaxL and the indices of the articular facets). For most measurements, Manot calcaneus was larger (e.g., MaxL, MaxH, MinH, and LAL) than later females, yet smaller than the Paleolithic H. sapiens male calcanei. Differences between samples can be attributed to factors such as body size, physical activity, genetic composition, and variability in sexual dimorphism (Alonso-Llamazares and Pablos, 2019).
A decrease in sexual dimorphism with the transition to farming was previously suggested to be associated with reduced sexual division of labor (Ruff, 1987). Nevertheless, sexual dimorphism of the calcaneus size increased with the transition to a sedentary lifestyle. This might be related to the positive association between body mass and calcaneus size (Harper et al., 2022a). The overlap in variance between males and females in calcaneal indices with only a few significant differences (LAW/MaxL, LAL/MaxL, and CFB/CFH) corresponded with previous findings demonstrating no significant differences in calcaneal shape between the sexes (Harper, 2023). However, others suggested that sexual dimorphism also exists in calcaneal shape (Nozaki et al., 2020). Furthermore, our results corresponded to prior studies suggesting that females have less pronounced skeletal modifications with the transition from subsistence based on hunting and gathering to farming (Sparacello et al., 2011; Macintosh et al., 2014; Macintosh et al., 2015). Accordingly, the changes in the loading intensity or its pattern during the Neolithization process were probably lower in females.
As others (Harper, 2023), we found an increase in the absolute size of the calcaneus in males throughout time, i.e., PPN and Chalcolithic farmers had larger calcaneus than Natufian hunter-gatherers. Nevertheless, for the Paleolithic H. sapiens inconsistency was observed. Some measurements (e.g., MinH and MinB) followed the trend, while others (e.g., MaxH and LAW) demonstrated larger values than the Natufians. Females had an opposite temporal trend for some variables, with the Natufians demonstrating larger mean values than the preceding populations. Yet, the Manot calcaneus exhibited an exceptionally large calcaneus (except for the MinB) compared to later female populations.
Previous researchers suggested that an increase in calcaneus size can be related to an increase in body size over time, as a larger calcaneus is required to sustain the loadings applied in bipedal locomotion (Harper et al., 2021; Harper, 2023). In a recent paper, Stock et al. (2023) showed that in the Levant from the UP to the early Holocene, the stature of males slightly decreased while their body mass remained relatively constant. In females, an opposite trend was found, with the stature remaining relatively constant and the body mass reducing and then remaining constant. This might explain the inconsistency in trends observed in calcaneus variables throughout time and between the sexes. Furthermore, the female calcaneal morphology, especially its length, corresponded with the reduction in robusticity previously noted for various skeletal elements (Marchi et al., 2011; Ruff et al., 2015; Holt et al., 2017). The decrease in calcaneal length was previously also related to changes in footwear (Albee, 2022). Since the earliest use of footwear was in the middle UP period (Trinkaus, 2005; Trinkaus and Shang, 2008), it might indicate footwear had some effect on calcaneal length, though, in our study, we observed this only in females. However, since no prominent tendency was observed in MaxL in males (e.g., the MP calcaneus of Qafzeh H9 was shorter than others), footwear cannot be the exclusive factor for explaining the reduction in calcaneal length. An alternative explanation might include sex-based division of labor and genetic factors.
Considering the dependence of calcaneal size on body weight (Harper et al., 2021) and environment (Albee, 2022), and based on our results that calcaneal proportions are less sexually dimorphic, we suggest that calcaneal proportions, which are also proxies for shape, are better indicators for examining the effect of habitual activity and mobility on calcaneal morphology (Nozaki et al., 2020; Harper et al., 2021). Changes in physical loadings and reduced mobility were previously reported for the populations inhabiting the Levant throughout the “Agriculture Revolution” (Eshed et al., 2004; May and Ruff, 2016). Hence, we hypothesized that calcaneal morphology would react to the changes in physical loadings applied to the bone, i.e., hunter-gatherers will have taller (MaxH) calcaneal tuber, taller (MinH) and wider (MinB) calcaneal body, wider distal calcaneus (LAW), and wider and longer dorsal articular surface (DAFB and DAFL) than later sedentary populations (Gierse, 1976; Su et al., 1999; Pablos et al., 2014; Harper et al., 2022b). However, the results only partially corresponded with our hypothesis (Figure 7). Accordingly, opposed to our expectations, Natufian hunter-gatherer males had relatively shorter calcaneal tuber (MaxH), a shorter (MinH) and narrower calcaneal body, and narrower distal calcaneus than the Chalcolithic farmers. Nevertheless, as we expected, they had a relatively longer distal calcaneus (i.e., a relatively shorter body).
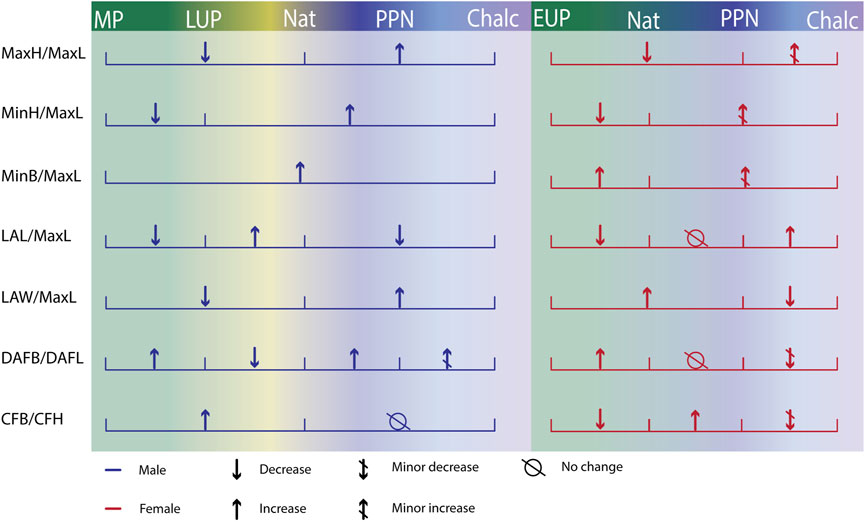
FIGURE 7. Summary of the trends observed in calcaneal proportions over time by sex. MaxL, Maximum length; MaxH, Maximum height; MinH, Minimum height; MinB, Minimum breadth; LAW, Load arm width; LAL, Load arm length; CFH, Cuboid facet height; CFB, Cuboid facet breadth. Middle Paleolithic (MP); Early Upper Paleolithic (EUP); Late Upper Paleolithic (LUP); Natufian (Nat); Pre-Pottery Neolithic (PPN), and Chalcolithic (Chalc).
The calcanei of Paleolithic H. sapiens corresponded better with our hypothesis. Both male calcanei had relatively taller calcaneal tuber, though only Qafzeh H9 had a relatively shorter calcaneal body, and both had relatively wider distal calcaneus. Furthermore, they had a relatively taller cuboid facet and wider dorsal articular facet. In females, the transition to farming seemed to affect mainly the distal calcaneus, though opposed to our expectations, with an increase in its relative width and length. Nevertheless, the EUP calcaneal morphology corresponded well with our expectations for some features, demonstrating a relatively tall calcaneal tuber and short body. However, it had a relatively narrower distal calcaneus, the cuboid articular facet was relatively wider, and the dorsal articular facet was longer than its width.
The morphology observed in the MP and EUP calcanei, such as a relatively short body (implied by the relative distal calcaneal length proportion, i.e., LAL/MaxL), suggests that they were better adapted for running as it relates to a shorter moment arm of the Achilles tendon (Raichlen et al., 2011). Furthermore, the MP, EUP, and LUP calcanei had a relatively taller calcaneal tuber (ca. 57% of calcaneal length) than the later Natufian hunter-gatherers, suggesting better resistance to higher bending stresses, which is also essential for efficient running (Raichlen et al., 2011; Harper et al., 2022a). A relatively wide dorsal articular facet is considered to contribute to a better contact area with the talus and a larger area for distributing the forces applied to the joint. Hence, it is better for sustaining larger forces (Jungers, 1988; Ruff, 1988). Accordingly, higher mobility is related to a wider calcaneal subtalar facet relative to its length (Sorrentino et al., 2020). This corresponded with the relatively wide facet Ohalo H2 had but not with the even width-to-length or relatively narrower facet Qafzeh H9 and Manot had, respectively. Hence, while some of the traits observed in Qafzeh H9 and Manot calcanei can be related to loadings, others could be related to their antiqueness, as they presented some Neanderthal-like traits (Pablos et al., 2014; Pablos et al., 2019; Borgel et al., 2021).
The consistent result of a temporal increase in the relative width of the calcaneal body and the increase in calcaneal body height from the Natufians to the Chalcolithic in both sexes contradicted our hypothesis, as they are considered archaic features (Pablos et al., 2014). There are several possible explanations for these trends. Among these, changes in body mass or proportions may have affected bone morphology (Ruff, 2000; Shaw and Stock, 2011). Additionally, changes in daily physical activity may have also affected the observed trends, as farmers were more involved in local activities in standing positions (Eshed et al., 2004). Thus, the morphology of the calcaneus was adapted to sustain the loading applied by the triceps surae muscle while standing (Pablos et al., 2014).
The cuboid facet of the calcanei of Paleolithic H. sapiens differed in proportions from later groups. While the Paleolithic H. sapiens males had a vertically elongated facet, Manot had a horizontally elongated facet. Furthermore, in males, it remained quadrangular in shape (i.e., a ratio of 1) between the Natufian hunter-gatherers and Chalcolithic farmers, and in females, it demonstrated mixed results (quadrangular in the Natufian and Chalcolithic samples and elongated horizontally in the PPN). It is well established that the calcaneocuboid joint plays a crucial role in restricting the range of motion and increasing the stability of the human foot, which is necessary for bipedal locomotion (Kidd et al., 1996; Meldrum and Hilton, 2004; Ginot et al., 2016). Furthermore, based on a study on rodents, it was reported that a wider facet on the horizontal plane enables a broader range of horizontal movement, and a taller facet on the sagittal plane facilitates a wider range of sagittal movement (Ginot et al., 2016). However, the inconsistent results obtained here require further investigation to understand how the morphology of the cuboid articular surface was affected, if at all, by the transition to a sedentary way of life. Inconsistency in the trend was also observed for the dorsal articular surface. In males, the relative width increased during the terminal Pleistocene-early Holocene, whereas in females, as expected, it decreased, i.e., becoming more oval. Noteworthy is that a more oval facet was also related to footwear, suggesting females had more restrictive footwear (Sorrentino et al., 2020).
To conclude, our results imply that the timing of change differed between the traits, as well as the factors causing it (Figure 7). The calcanei of Paleolithic H. sapiens were better adapted for high mobility, independent of sex. Thus, changes related to the decrease in mobility occurred before the Natufian period. During the transition to a sedentary lifestyle, the changing environment affected males and females differently. The decrease in calcaneal length in females, the increase in the relative dorsal articular facet in males, and the increase in calcaneal body relative height and width in both sexes may suggest that factors related to sexual division of labor, adaptation to lengthy standing, and changes in footwear designed the sedentary human calcaneus.
This study faced some limitations inherited in anthropological research, mainly those related to the sample size, especially of Paleolithic samples, which are scarce. Furthermore, misclassification of sex based on the calcaneus only could confound the results. These reasons could also explain different patterns throughout time between males and females. Further investigation with a large sample size per site is required to understand whether the differences between males and females are population-specific or depend on the period studied. Furthermore, this study relied on linear measurements and indices that cannot capture the complicated shape of the calcaneus and, therefore, are restricted to gross changes.
Data availability statement
The datasets presented in this article are not readily available because The raw data supporting the conclusion of this article will be made available by the author, dependent on collections policies. Requests to access the datasets should be directed to HM, mayhila@tauex.tau.ac.il.
Ethics statement
Ethical approval was not required for the studies involving humans because The sample included in this study are of ancient material, older than 6,000 years. The studies were conducted in accordance with the local legislation and institutional requirements. Written informed consent for participation was not required from the participants or the participants’ legal guardians/next of kin in accordance with the national legislation and institutional requirements because the sample included in this study are of ancient material, older than 6,000 years.
Author contributions
AD: Data curation, Investigation, Writing–original draft, Formal Analysis, Visualization. AP: Methodology, Writing–review and editing. MA: Data curation, Writing–review and editing, Formal Analysis. GY: Data curation, Validation, Writing–review and editing. RK-P: Formal Analysis, Writing–review and editing. HM: Conceptualization, Formal Analysis, Supervision, Funding acquisition, Validation, Writing–original draft.
Funding
The author(s) declare financial support was received for the research, authorship, and/or publication of this article. We would like to acknowledge the Israel Science Foundation (#1005/22), the United States-Israel Binational Science Foundation (#2019041), the Gerda Henkel Foundation (#AZ 06/F/22), the Israel Antiquities Authority, and the Dan David Foundation.
Acknowledgments
This study is part of AD MSc thesis from the Faculty of Medicine, Tel Aviv University, which was carried out under the supervision of HM. We would like to thank Prof. Ofer Marder, Ben Gurion University of the Negev, Israel, and Dr. Omry Barzilai, the Israeli Antiquities Authority (IAA), for their permission to study Manot calcaneus, Prof. Daniel Nadel, Haifa University, Israel for the permission to study Ohalo H2, and Prof. Israel Hershkovitz, Tel Aviv University for the permission to study both Manot and Ohalo H2 calcanei. In addition, we would like to thank the archeologists who excavated the Natufian, PPN, and Chalcolithic archeological sites included in this study: Prof. Daniel Nadel, Haifa University; Dr. Hamudi Khalila, Dr. Jacob Vardi, Dr. Ianir Milevski, and Dr. Dina Shalem from the IAA; and Dr. Ehud Galili. We thank Ruth Peleg-Kallevag for the English Editing the manuscript.
Conflict of interest
The authors declare that the research was conducted in the absence of any commercial or financial relationships that could be construed as a potential conflict of interest.
Publisher’s note
All claims expressed in this article are solely those of the authors and do not necessarily represent those of their affiliated organizations, or those of the publisher, the editors and the reviewers. Any product that may be evaluated in this article, or claim that may be made by its manufacturer, is not guaranteed or endorsed by the publisher.
Supplementary material
The Supplementary Material for this article can be found online at: https://www.frontiersin.org/articles/10.3389/feart.2024.1294350/full#supplementary-material
References
Albee, M. E. (2022). Tarsal metric trends over the Medieval-Post-Medieval transition in London. HOMO 73 (1), 33–47. doi:10.1127/homo/2022/1551
Alonso-Llamazares, C., and Pablos, A. (2019). Sex estimation from the calcaneus and talus using discriminant function analysis and its possible application in fossil remains. Archaeol. Anthropol. Sci. 11 (9), 4927–4946. doi:10.1007/s12520-019-00855-y
Bar-Yosef, O., and Belfer-Cohen, A. (1989). The origins of sedentism and farming communities in the levant. J. World Prehistory 3 (4), 447–498. doi:10.1007/BF00975111
Bar-Yosef, O., and Belfer-Cohen, A. (1991). From sedentary hunter-gatherers to territorial farmers in the levant. Between Bands States, 181–202.
Belfer-Cohen, A., and Goring-Morris, A. N. (2011). Becoming farmers: the inside story. Curr. Anthropol. 52 (4), S209–S220. doi:10.1086/658861
Belfer-Cohen, A., and Goring-Morris, N. (2020). From the epipalaeolithic into the earliest neolithic (PPNA) in the South levant. Doc. Praehist. 47, 36–52. doi:10.4312/dp.47.3
Bidmos, M. A., and Asala, S. A. (2003). Discriminant function sexing of the calcaneus of the South African whites. J. Forensic Sci. 48 (6), JFS2003104. doi:10.1520/JFS2003104
Bidmos, M. A., and Asala, S. A. (2004). Sexual dimorphism of the calcaneus of South African blacks. J. Forensic Sci. 49 (3), 1–5. doi:10.1520/JFS2003254
Bojsen-Møller, F. (1979). Calcaneocuboid joint and stability of the longitudinal arch of the foot at high and low gear push off. J. Anat. 129 (1), 165–176.
Borgel, S., Latimer, B., McDermott, Y., Sarig, R., Pokhojaev, A., Abulafia, T., et al. (2021). Early upper paleolithic human foot bones from Manot cave, Israel. J. Hum. Evol. 160, 102668. doi:10.1016/j.jhevol.2019.102668
Boyle, E. K., McNutt, E. J., Sasaki, T., Suwa, G., Zipfel, B., and DeSilva, J. M. (2018). A quantification of calcaneal lateral plantar process position with implications for bipedal locomotion in australopithecus. J. Hum. Evol. 123, 24–34. doi:10.1016/j.jhevol.2018.05.008
Bräuer, G. (1988). “Osteometry,” in Anthropologie. Editors R. Martin, and R. Knuβman (Stuttgart: Fisher), 160–232.
Cicchetti, D. V. (1994). Guidelines, criteria, and rules of thumb for evaluating normed and standardized assessment instruments in psychology. Psychol. Assess. 6 (4), 284–290. doi:10.1037/1040-3590.6.4.284
Coqueugniot, H., Tillier, A. M., and Bruzek, J. (2000). Mandibular ramus posterior flexure: a sex indicator in Homo sapiens fossil hominids? Int. J. Osteoarchaeol. 10 (6), 426–431. doi:10.1002/1099-1212(200011/12)10:6<426::aid-oa533>3.0.co;2-#
Coutinho-Nogueira, D., Coqueugniot, H., and Tillier, A. M. (2021). Qafzeh 9 early modern human from southwest Asia: age at death and sex estimation re-assessed. HOMO 72 (4), 293–305. doi:10.1127/homo/2021/1513
DeMars, L. J. D., Stephens, N. B., Saers, J. P. P., Gordon, A., Stock, J. T., and Ryan, T. M. (2021). Using point clouds to investigate the relationship between trabecular bone phenotype and behavior: an example utilizing the human calcaneus. Am. J. Hum. Biol. 33 (2), e23468. doi:10.1002/ajhb.23468
DeSilva, J., McNutt, E., Benoit, J., and Zipfel, B. (2019). One small step: a review of plio-pleistocene hominin foot evolution. Am. J. Phys. Anthropol. 168, 63–140. doi:10.1002/ajpa.23750
DiMichele, D. L., and Spradley, M. K. (2012). Sex estimation in a modern American osteological sample using a discriminant function analysis from the calcaneus. Forensic Sci. Int. 221 (1-3), 152.e1–152.e5. doi:10.1016/j.forsciint.2012.03.026
Donatelli, R. (1985). Normal biomechanics of the foot and ankle. J. Orthop. Sports Phys. Ther. 7 (3), 91–95. doi:10.2519/jospt.1985.7.3.91
Ekizoglu, O., Hocaoglu, E., Can, I. O., Inci, E., Aksoy, S., and Bilgili, M. G. (2015). Magnetic resonance imaging of distal tibia and calcaneus for forensic age estimation in living individuals. Int. J. Leg. Med. 129, 825–831. doi:10.1007/s00414-015-1187-1
Eshed, V., Gopher, A., Galili, E., and Hershkovitz, I. (2004). Musculoskeletal stress markers in natufian hunter-gatherers and neolithic farmers in the levant: the upper limb. Am. J. Phys. Anthropol. 123 (4), 303–315. doi:10.1002/ajpa.10312
Gierse, H. (1976). The cancellous structure in the calcaneus and its relation to mechanical stressing. Anat. Embryology 150 (1), 63–83. doi:10.1007/BF00346287
Gilead, I. (1988). The Chalcolithic period in the levant. J. World Prehistory 2 (4), 397–443. doi:10.1007/BF00976197
Ginot, S., Hautier, L., Marivaux, L., and Vianey-Liaud, M. (2016). Ecomorphological analysis of the astragalo-calcaneal complex in rodents and inferences of locomotor behaviours in extinct rodent species. PeerJ 4, e2393. doi:10.7717/peerj.2393
Gualdi-Russo, E. (2007). Sex determination from the talus and calcaneus measurements. Forensic Sci. Int. 171 (2-3), 151–156. doi:10.1016/j.forsciint.2006.10.014
Harcourt-Smith, W. E. H., and Aiello, L. C. (2004). Fossils, feet and the evolution of human bipedal locomotion. J. Anat. 204 (5), 403–416. doi:10.1111/j.0021-8782.2004.00296.x
Harney, É., May, H., Shalem, D., Rohland, N., Mallick, S., Lazaridis, I., et al. (2018). Ancient DNA from Chalcolithic Israel reveals the role of population mixture in cultural transformation. Nat. Commun. 9 (1), 3336. doi:10.1038/s41467-018-05649-9
Harper, C. M. (2023). Human calcaneal variation relative to subsistence strategy, activity level, and footwear. Front. Earth Sci. 11. doi:10.3389/feart.2023.1213374
Harper, C. M., Ruff, C. B., and Sylvester, A. D. (2021). Calcaneal shape variation in humans, nonhuman primates, and early hominins. J. Hum. Evol. 159, 103050. doi:10.1016/j.jhevol.2021.103050
Harper, C. M., Ruff, C. B., and Sylvester, A. D. (2022a). Scaling and relative size of the human, nonhuman ape, and baboon calcaneus. Anat. Rec. 305 (1), 100–122. doi:10.1002/ar.24642
Harper, C. M., Zipfel, B., DeSilva, J. M., McNutt, E. J., Thackeray, F., and Braga, J. (2022b). A new early hominin calcaneus from Kromdraai (South Africa). J. Anat. 241, 500–517. (March). doi:10.1111/joa.13660
Hershkovitz, I., and Gopher, A. (1990). Paleodemography, burial customs, and food-producing economy at the beginning of the Holocene: a perspective from the southern levant. Mitekufat Haeven, 9–47.
Hershkovitz, I., and Gopher, A. (2008). “Demographic, biological and cultural aspects of the neolithic revolution: a view from the southern levant,” in The neolithic demographic transition and its consequences. Editors J. P. Bocquet-Appel, and O. Bar-Yosef (Dordrecht: Springer), 441–479. doi:10.1007/978-1-4020-8539-0_17
Hershkovitz, I., Speirs, M. S., Frayer, D., Nadel, D., Wish-Baratz, S., and Arensburg, B. (1995). Ohalo II H2: a 19,000-year-old skeleton from a water-logged site at the Sea of Galilee, Israel. Am. J. Phys. Anthropol. 96 (3), 215–234. doi:10.1002/ajpa.1330960302
Hershkovitz, I., Weber, G. W., Quam, R., Duval, M., Grün, R., Kinsley, L., et al. (2018). The earliest modern humans outside Africa. Science 359 (6374), 456–459. doi:10.1126/science.aap8369
Holt, B., Whittey, E., Niskanen, M., Sládek, V., Berner, M., and Ruff, C. B. (2017). “Temporal and geographic variation in robusticity,” in Skeletal variation and adaptation in Europeans: upper paleolithic to the twentieth century. Editor C. B. Ruff (Incorporated: John Wiley & Sons), 91–132. doi:10.1002/9781118628430.ch5
Holt, B. M. (2003). Mobility in upper paleolithic and mesolithic Europe: evidence from the lower limb. Am. J. Phys. Anthropol. 122 (3), 200–215. doi:10.1002/ajpa.10256
Hoover, K. C., and Colette Berbesque, J. (2018). Early Holocene morphological variation in hunter-gatherer hands and feet. PeerJ 6, e5564. doi:10.7717/peerj.5564
Jungers, W. L. (1988). Relative joint size and hominoid locomotor adaptations with implications for the evolution of hominid bipedalism. J. Hum. Evol. 17 (1–2), 247–265. doi:10.1016/0047-2484(88)90056-5
Kidd, R. S., O’Higgins, P., and Oxnard, C. E. (1996). The OH8 foot: a reappraisal of the functional morphology of the hindfoot utilizing a multivariate analysis. J. Hum. Evol. 31 (3), 269–291. doi:10.1006/jhev.1996.0061
Kim, D. I., Kim, Y. S., Lee, U. Y., and Han, S. H. (2013). Sex determination from calcaneus in Korean using discriminant analysis. Forensic Sci. Int. 228 (1-3), 177.e1–177.e7. doi:10.1016/j.forsciint.2013.03.012
Kuijt, I., and Goring-Morris, N. (2002). Foraging, farming, and social complexity in the pre-pottery neolithic of the southern levant: a review and synthesis. J. World Prehistory 16 (4), 361–440. doi:10.1023/A:1022973114090
Langdon, J. H. (1985). Fossils and the origin of bipedalism. J. Hum. Evol. 14 (7), 615–635. doi:10.1016/S0047-2484(85)80071-3
Latimer, B., and Lovejoy, C. O. (1989). The calcaneus of australopithecus afarensis and its implications for the evolution of bipedality. Am. J. Phys. Anthropol. 78 (3), 369–386. doi:10.1002/ajpa.1330780306
Lazaridis, I., Nadel, D., Rollefson, G., Merrett, D. C., Rohland, N., Mallick, S., et al. (2016). Genomic insights into the origin of farming in the ancient Near East. Nature 536 (7617), 419–424. doi:10.1038/nature19310
Lieberman, D. E. (1991). Seasonality and gazelle hunting at hayonim cave: new evidence for “sedentism” during the natufian. Paléorient 17 (1), 47–57. doi:10.3406/paleo.1991.4538
Lieberman, D. E. (1997). Making behavioral and phylogenetic inferences from hominid fossils: considering the developmental influence of mechanical forces. Annu. Rev. Anthropol. 26, 185–210. doi:10.1146/annurev.anthro.26.1.185
Lieberman, D. E., Belfer-Cohen, A., Henry, D. O., Kaufman, D., Mackie, Q., Olszewski, D. I., et al. (1993). The rise and fall of seasonal mobility among hunter-gatherers: the case of the southern Levant [and comments and replies]. Curr. Anthropol. 34 (5), 599–631. doi:10.1086/204209
Macintosh, A. A., Davies, T. G., Pinhasi, R., and Stock, J. T. (2015). Declining tibial curvature parallels ∼6150 years of decreasing mobility in central European agriculturalists. Am. J. Phys. Anthropol. 157 (2), 260–275. doi:10.1002/ajpa.22710
Macintosh, A. A., Pinhasi, R., and Stock, J. T. (2014). Lower limb skeletal biomechanics track long-term decline in mobility across ∼6150 years of agriculture in Central Europe. J. Archaeol. Sci. 52, 376–390. doi:10.1016/j.jas.2014.09.001
Marchi, D., Sparacello, V., and Shaw, C. (2011). “Mobility and lower limb robusticity of a pastoralist Neolithic population from northwestern Italy,” in Human bioarchaeology of the transition to agriculture. Editors R. Pinhasi, and J. Stock (New York: Wiley-Blackwell), 317–346. doi:10.1002/9780470670170.ch13
May, H., and Ruff, C. (2016). Physical burden and lower limb bone structure at the origin of agriculture in the levant. Am. J. Phys. Anthropol. 161 (1), 26–36. doi:10.1002/ajpa.23003
McNutt, E. J., Zipfel, B., and DeSilva, J. M. (2018). The evolution of the human foot. Evol. Anthropol. 27 (5), 197–217. doi:10.1002/evan.21713
Meldrum, J., and Hilton, C. E. (2004). From biped to strider: the emergence of modern human walking, running, and resource transport. Springer Science and Business Media.
Murphy, A. M. C. (2002). The calcaneus: sex assessment of prehistoric New Zealand Polynesian skeletal remains. Forensic Sci. Int. 129 (3), 205–208. doi:10.1016/S0379-0738(02)00301-8
Nozaki, S., Watanabe, K., Kamiya, T., Katayose, M., and Ogihara, N. (2020). Three-dimensional morphological variations of the human calcaneus investigated using geometric morphometrics. Clin. Anat. 33, 751–758. doi:10.1002/ca.23501
Oishi, M., Ogihara, N., Shimizu, D., Kikuchi, Y., Endo, H., Une, Y., et al. (2018). Multivariate analysis of variations in intrinsic foot musculature among hominoids. J. Anat. 232 (5), 812–823. doi:10.1111/joa.12780
Pablos, A., Martínez, I., Lorenzo, C., Sala, N., Gracia-Téllez, A., and Arsuaga, J. L. (2014). Human calcanei from the middle Pleistocene site of sima de los huesos (sierra de atapuerca, burgos, Spain). J. Hum. Evol. 76, 63–76. doi:10.1016/j.jhevol.2014.05.005
Peckmann, T. R., Orr, K., Meek, S., and Manolis, S. K. (2015). Sex determination from the calcaneus in a 20th century Greek population using discriminant function analysis. Sci. Justice 55 (6), 377–382. doi:10.1016/j.scijus.2015.04.009
Prang, T. C. (2015). Calcaneal robusticity in plio-pleistocene hominins: implications for locomotor diversity and phylogeny. J. Hum. Evol. 80, 135–146. doi:10.1016/j.jhevol.2014.09.001
Püschel, T. A., and Benítez, H. A. (2014). Femoral functional adaptation: a comparison between hunter gatherers and farmers using geometric morphometrics. Int. J. Morphol. 32 (2), 627–633. doi:10.4067/s0717-95022014000200041
Raichlen, D. A., Armstrong, H., and Lieberman, D. E. (2011). Calcaneus length determines running economy: implications for endurance running performance in modern humans and neandertals. J. Hum. Evol. 60 (3), 299–308. doi:10.1016/j.jhevol.2010.11.002
Rak, Y. (1990). On the differences between two pelvises of Mousterian context from the Qafzeh and Kebara caves, Israel. Am. J. Phys. Anthropol. 81 (3), 323–332. doi:10.1002/ajpa.1330810302
Riepert, T., Drechsler, T., Schild, H., Nafe, B., and Mattern, R. (1996). Estimation of sex on the basis of radiographs of the calcaneus. Forensic Sci. Int. 77 (3), 133–140. doi:10.1016/0379-0738(95)01832-8
Rosenberg, K. R., Brace, C. L., Frayer, D. W., Geise, M. C., Greene, D. L., Tague, R. G., et al. (1988). The functional significance of neandertal pubic length [and comments and reply]. Curr. Anthropol. 29 (4), 595–617. doi:10.1086/203678
Rowan, Y. M., and Golden, J. (2009). The Chalcolithic period of the southern levant: a synthetic review. J. World Prehistory 22, 1–92. doi:10.1007/s10963-009-9016-4
Ruff, C. (1987). Sexual dimorphism in human lower limb bone structure: relationship to subsistence strategy and sexual division of labor. J. Hum. Evol. 16 (5), 391–416. doi:10.1016/0047-2484(87)90069-8
Ruff, C. (1988). Hindlimb articular surface allometry in hominoidea and Macaca, with comparisons to diaphyseal scaling. J. Hum. Evol. 17 (7), 687–714. doi:10.1016/0047-2484(88)90025-5
Ruff, C., Holt, B., and Trinkaus, E. (2006). Who’s afraid of the big bad wolff? ‘wolff’s law’ and bone functional adaptation. Am. J. Phys. Anthropol. 129 (4), 484–498. doi:10.1002/ajpa.20371
Ruff, C. B. (2000). Body size, body shape, and long bone strength in modern humans. J. Hum. Evol. 38 (2), 269–290. doi:10.1006/jhev.1999.0322
Ruff, C. B., Holt, B., Niskanen, M., Sladek, V., Berner, M., Garofalo, E., et al. (2015). Gradual decline in mobility with the adoption of food production in Europe. Proc. Natl. Acad. Sci. U. S. A. 112 (23), 7147–7152. doi:10.1073/pnas.1502932112
Saers, J. P. P., Ryan, T. M., and Stock, J. T. (2019). Trabecular bone functional adaptation and sexual dimorphism in the human foot. Am. J. Phys. Anthropol. 168 (1), 154–169. doi:10.1002/ajpa.23732
Schwarcz, H. P., Grün, R., Vandermeersch, B., Bar-Yosef, O., Valladas, H., and Tchernov, E. (1988). ESR dates for the hominid burial site of Qafzeh in Israel. J. Hum. Evol. 17 (8), 733–737. doi:10.1016/0047-2484(88)90063-2
Segal, D., Carmi, I., Gal, Z., Smithline, H., and Shalem, D. (1998). Dating a chalcolithic burial cave in peqi’in, upper galilee, Israel. Radiocarbon 40 (2), 707–712. doi:10.1017/S0033822200018658
Shavit, A., and Sharon, G. (2023). Can models of evolutionary transition clarify the debates over the Neolithic Revolution? Philosophical Trans. R. Soc. B Biol. Sci. 378 (1872), 20210413. doi:10.1098/rstb.2021.0413
Shaw, C. N., and Stock, J. T. (2011). The influence of body proportions on femoral and tibial midshaft shape in hunter-gatherers. Am. J. Phys. Anthropol. 144 (1), 22–29. doi:10.1002/ajpa.21363
Sorrentino, R., Stephens, N. B., Carlson, K. J., Figus, C., Fiorenza, L., Frost, S., et al. (2020). The influence of mobility strategy on the modern human talus. Am. J. Phys. Anthropol. 171 (3), 456–469. doi:10.1002/ajpa.23976
Sparacello, V. S., Pearson, O. M., Coppa, A., and Marchi, D. (2011). Changes in skeletal robusticity in an iron age agropastoral group: the samnites from the Alfedena necropolis (Abruzzo, Central Italy). Am. J. Phys. Anthropol. 144 (1), 119–130. doi:10.1002/ajpa.21377
Spradley, M. K., and Jantz, R. L. (2011). Sex estimation in forensic anthropology: skull versus postcranial elements. J. Forensic Sci. 56 (2), 289–296. doi:10.1111/j.1556-4029.2010.01635.x
Steele, D. G. (1976). The estimation of sex on the basis of the talus and calcaneus. Am. J. Phys. Anthropol. 45 (3), 581–588. doi:10.1002/ajpa.1330450323
Stock, J. T., Pomeroy, E., Ruff, C. B., Brown, M., Gasperetti, M. A., Li, F. J., et al. (2023). Long-term trends in human body size track regional variation in subsistence transitions and growth acceleration linked to dairying. Proc. Natl. Acad. Sci. U. S. A. 120 (4), e2209482119. doi:10.1073/pnas.2209482119
Su, S. C., Skedros, J. G., Bachus, K. N., and Bloebaum, R. D. (1999). Loading conditions and cortical bone construction of an artiodactyl calcaneus. J. Exp. Biol. 202 (22), 3239–3254. doi:10.1242/jeb.202.22.3239
Susman, R. L. (1983). Evolution of the human foot: evidence from plio-pleistocene hominids. Foot Ankle 3 (6), 365–376. doi:10.1177/107110078300300605
Trinkaus, E. (1984). Neandertal pubic morphology and gestation length. Curr. Anthropol. 25 (4), 509–514. doi:10.1086/203173
Trinkaus, E. (2005). Anatomical evidence for the antiquity of human footwear use. J. Archaeol. Sci. 32 (10), 1515–1526. doi:10.1016/j.jas.2005.04.006
Trinkaus, E., and Ruff, C. B. (1999). Diaphyseal cross-sectional geometry of near eastern middle palaeolithic humans: the femur. J. Archaeol. Sci. 26 (4), 409–424. doi:10.1006/jasc.1998.0343
Trinkaus, E., and Shang, H. (2008). Anatomical evidence for the antiquity of human footwear: tianyuan and Sunghir. J. Archaeol. Sci. 35 (7), 1928–1933. doi:10.1016/j.jas.2007.12.002
Valla, F. R., Khalaily, H., Samuelian, N., and Bocquentin, F. (2002). From foraging to farming. The contribution of the mallaha (eynan) excavations, 1996–2001. Bull. Cent. Rech. français à Jérusalem 10, 71–90.
Vandermeersch, B. (1981) Les hommes fossiles de Qafzeh, Israël, Editions du Centre national de la recherche scientifique.
Vigne, J., and Helmer, D. (2007). Was milk a “secondary product” in the old world neolithisation process? Its role in the domestication of cattle, sheep, and goats. Anthropozoologica 42 (2), 9–40.
Vyce, S. D., Addis-Thomas, E., Mathews, E. E., and Perez, S. L. (2010). Painful prominences of the heel. Clin. Podiatric Med. Surg. 27 (3), 443–462. doi:10.1016/j.cpm.2010.04.005
Weidenreich, F. (1923). Evolution of the human foot. Am. J. Phys. Anthropol. 6 (1), 1–10. doi:10.1002/ajpa.1330060102
Weiss, E. (2012). Calcaneal spurs: examining etiology using prehistoric skeletal remains to understand present day heel pain. Foot 22 (3), 125–129. doi:10.1016/j.foot.2012.04.003
Yeshurun, R., Bar-Oz, G., and Weinstein-Evron, M. (2007). Modern hunting behavior in the early middle paleolithic: faunal remains from misliya cave, mount carmel, Israel. J. Hum. Evol. 53 (6), 656–677. doi:10.1016/j.jhevol.2007.05.008
Yeshurun, R., Bar-Oz, G., and Weinstein-Evron, M. (2014). Intensification and sedentism in the terminal Pleistocene natufian sequence of el-wad terrace (Israel). J. Hum. Evol. 70, 16–35. doi:10.1016/j.jhevol.2014.02.011
Zakaria, M. S., Mohammed, A. H., Habib, S. R., Hanna, M. M., and Fahiem, A. L. (2010). Calcaneus radiograph as a diagnostic tool for sexual dimorphism in Egyptians. J. Forensic Leg. 17 (7), 378–382. doi:10.1016/j.jflm.2010.05.009
Keywords: calcaneus, mobility, Southern Levant, “Agricultural Revolution”, load distribution, subsistence strategy
Citation: Dann A, Pokhojaev A, Anton M, Yalovitsky G, Kallevag-Pelleg R and May H (2024) Changes in human calcaneal morphology throughout the Pleistocene-Holocene Levant. Front. Earth Sci. 12:1294350. doi: 10.3389/feart.2024.1294350
Received: 14 September 2023; Accepted: 16 January 2024;
Published: 15 February 2024.
Edited by:
Rita Sorrentino, University of Bologna, ItalyReviewed by:
Annalisa Pietrobelli, University of Bologna, ItalyMarta Pina, London South Bank University, United Kingdom
Adrián Pablos, Complutense University of Madrid, Spain
Copyright © 2024 Dann, Pokhojaev, Anton, Yalovitsky, Kallevag-Pelleg and May. This is an open-access article distributed under the terms of the Creative Commons Attribution License (CC BY). The use, distribution or reproduction in other forums is permitted, provided the original author(s) and the copyright owner(s) are credited and that the original publication in this journal is cited, in accordance with accepted academic practice. No use, distribution or reproduction is permitted which does not comply with these terms.
*Correspondence: Hila May, mayhila@tauex.tau.ac.il