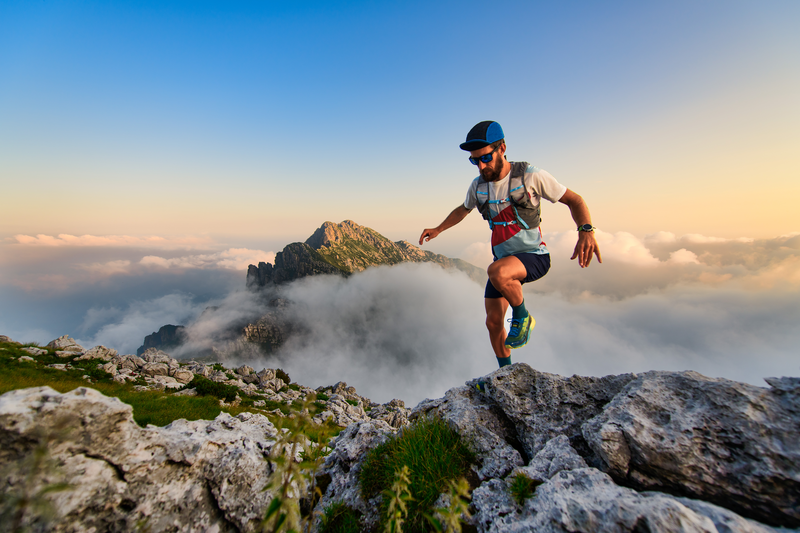
95% of researchers rate our articles as excellent or good
Learn more about the work of our research integrity team to safeguard the quality of each article we publish.
Find out more
ORIGINAL RESEARCH article
Front. Earth Sci. , 15 February 2024
Sec. Petrology
Volume 12 - 2024 | https://doi.org/10.3389/feart.2024.1287221
In order to identify and evaluate reservoir wettability, so as to select suitable mining methods to improve oil recovery, wettability evaluation through one-dimensional (1D) and two-dimensional (2D) nuclear magnetic resonance (NMR) technology combined with physical simulation experiments was carried out in this study, and the feasibility of the experimental method was verified by contact Angle method. According to the principle of hydrophilic inorganic content and hydrophobic organic content of shale, the wettability can be evaluated through vacuum self-imbibition oil and self-imbibition water physical simulation experiments combined with 1D NMR technology, that is, total water absorption can be considered as inorganic content, organic content can be obtained by subtracting total water absorption from total oil absorption, and adsorbed oil content can be obtained by subtracting pore volume from total oil absorption. In addition to the wettability measurement by contact Angle method to verify the experimental method, the occurrence ratio of adsorbed oil can also be verified twice by 2D NMR spectrum. The results show that the error between the proportion of adsorbed oil measured by 2D NMR spectrum and that measured by self-imbibition method is within 4%. In the saturated oil-bound water state, the bound water is within the relaxation interval of 1<T1<10 ms and 1<T2<10 ms, and the oil signal is within the relaxation interval of T1>10 ms and T2>10 ms. And the relaxation time of aqueous phase moved to the right compared with that in the saturated water state, the interaction force between water and the pore wall was weakened, showing the characteristics of free fluid, and the rock sample became oil-wet, which was consistent with the wettability results measured by contact Angle. Therefore, the wettability of rock samples can be evaluated by 1D NMR technique combined with vacuum imbibition method, or by analyzing the changes of NMR spectra of aqueous phase in completely saturated water and saturated oil-bound water.
Wettability is one of the important parameters to evaluate reservoir characteristics, and the evaluation of rock wettability is very important for the selection of reservoir development mode, the degree of recovery, the distribution of remaining oil and the enhancement of oil recovery (Anderson William et al., 1986; Zhuang et al., 2017; Lin et al., 2020; Gu et al., 2021). The development of micropore throat and mineral composition of shale oil reservoir are complicated, so the research on the new method of reservoir wettability identification is of great significance for the exploitation of shale oil reservoir (Guo et al., 2020; Huang et al., 2021; Xiao et al., 2021). Since the 1930s, domestic and foreign scholars have proposed a variety of methods to analyze wettability, among which qualitative analysis methods include low temperature electron scanning method, Wilhelmy dynamic plate method, relative permeability method, capillary pressure measurement with microporous membrane technology and wettability method (Wu et al., 2001). Quantitative analysis methods include contact Angle measurement, Amott method, USBM method, automatic imbibition method, NMR relaxation method, etc (Donaldson et al., 1969; Anderson, 1986; Yan, 2001; Liang et al., 2019). The current research is mainly based on quantitative method. Among them, the surface roughness, surface heterogeneity and molecular surface imbibition of rock sample during contact Angle measurement will cause hysteresis in the measurement process. The Amott method is suitable for measuring the mean wettability of the core, and the measured results are close to the actual condition of the reservoir, but not sensitive when it is close to neutral wettability. USBM method and imbibition method are the most commonly used methods to evaluate wettability in laboratory experiments. USBM method cannot determine whether the rock sample belongs to fractional wettability and mixed wettability. The automatic imbibition method not only has a long experimental period, but also cannot be used in logging technology. Nuclear magnetic resonance (NMR), as an efficient, non-destructive and rapid technology for measuring fluid and its distribution, is not only widely used in laboratory core analysis, but also an important geophysical logging method. Some scholars also use this technology to evaluate the wettability of reservoir rocks (Looyestijn and Hofman, 2006; Sun et al., 2012; Li et al., 2015). Howard (1998) established the relationship between the relaxation movement of saturated water, water saturation and wettability based on the 1D NMR T2 map under different water saturation. Fleury and Deflandre, (2003) defined 1D NMR wettability index to evaluate the wettability of rock samples based on the surface area of oil and water philicity. However, 1D NMR evaluation method needs to completely separate the relaxation information of oil phase and aqueous phase. With the development of 2D NMR technology, diffusion-relaxation (D-T2) and T1-T2 2D maps have become important tools to interpret and evaluate the type and distribution of reservoir fluids. Minh et al. (2015) established a wettability characterization method based on D-T2 atlas, but this method is difficult to apply to shale oil reservoirs with very short relaxation component information. At present, there are few studies on the evaluation of wettability of shale oil reservoirs using T1-T2 2D atlas. Therefore, the representative cores in Qinghai and Jilin blocks were took as the research object in this study, and 1D and 2D NMR evaluation methods were combined. Firstly, physical simulation experiments of vacuum self-imbibition oil and vacuum self-imbibition water of rock samples were carried out based on hydrophilic inorganic pores and hydrophobic organic pores of shale, and the experimental results were analyzed by 1D NMR technology. 2D NMR and contact Angle method were used to verify the experimental results. Secondly, the 2D NMR spectra and changes of aqueous phase in fully saturated water and saturated oil-bound water state were analyzed, and the experimental results were also verified by contact Angle method. In this way, 1D and 2D NMR analysis methods for evaluating reservoir wettability can be established.
This study always follows the standards of experimental testing of shale oil (Li et al., 2019). The two different methods for evaluating the wettability of rock reservoirs established in this study are based on different principles. Firstly, the principle of evaluating the wettability of rock reservoir by vacuum imbibition method is that inorganic pores are hydrophilic and organic pores are hydrophobic. Therefore, the experiment was carried out under the condition of vacuum self-imbibition, mainly aiming at the oil content of inorganic pores and organic pores under the condition of vacuum self-imbibition to judge the wettability of rocks. Therefore, the vacuum pressure used in this experiment was not large, which was 0.1 MPa pressure. If the pressure was too large, it is not possible to determine whether the oil or water entering the core is immersed under pressure or because of wettability, which affects the analysis of the experimental results.
Secondly, the principle of judging the wettability of rock reservoirs by 2D NMR is that the NMR spectra of water phase in saturated oil state move to the right compared with that in fully saturated water state, and the wettability of rock samples can be rapidly evaluated by analyzing the NMR spectra and changes of water phase in fully saturated water and saturated oil bound water state. Therefore, the experiment was carried out under the condition of pressure saturation, and the wettability of the rock was qualitatively and quickly judged by the nuclear magnetic resonance T1 maps of the water phase in the two states of completely saturated water and saturated oil-bound water in the core.
Ten shale samples were selected to carry out experimental research, rock samples No. 1 to No. 6 were carried out vacuum self-imbibition oil and self-imbibition water physical simulation experiments, and rock samples No. 7 to No. 10 were carried out oil flooding physical simulation experiments after saturated water. Samples No.1 to No. 6 were selected from the Qinghai shale oil cores in Qaidam Basin, and the reservoir mainly developed in the paleogene saline lacustrine shale reservoir. The “two-stage” continuous and efficient hydrocarbon generation of the low-abundance shale in the saline lacustrine basin provided the hydrocarbon generation basis for oil and gas enrichment. The high carbonate mineral content and low clay mineral content, as well as the development degree of the striated structure laid the foundation for the reservoir transformation of the shale reservoir (Liu et al., 2018; Zhang et al., 2021; Song et al., 2022; Li et al., 2023). Rock samples No. 7 to No. 10 were selected from Jilin shale oil cores in Songliao Basin. Shale oil in Songliao Basin is significantly different from shale oil in saline lake basin in terms of mineral composition, physical characteristics, oil content and mobilization, especially its stratified structure and bedding structure are very developed, so the occurrence mechanism and transportation mechanism of shale oil in pore and fracture system are also relatively complicated (Zeng et al., 2022; Feng et al., 2023; Zhou, 2023). The core physical property parameters are shown in Table 1. For the pore structure, wettability and other physical parameters of cores 1–6 will be affected after the completion of the first saturated water and saturated oil experiment, so it is not suitable to continue the second experiment. Therefore, cores in two blocks were selected to carry out two different experiments.
Nuclear magnetic resonance (NMR) is a phenomenon in which some nuclei with spin magnetic moments absorb electromagnetic waves of a specific frequency under the action of an external magnetic field, thereby changing the energy state. When NMR technology is applied in petroleum exploration and development, physical properties and fluid parameters such as reservoir permeability, porosity, oil saturation and percentage of movable fluid can be quickly obtained through NMR testing of rock samples. As a non-destructive and reliable technology, NMR can characterize the type of fluid in tight reservoirs, and the whole testing process has almost no damage to the cores. Compared with 1D NMR technology, 2D NMR technology can also separate the water and oil signals and quantitatively and intuitively analyze the reservoir occurrence state. When measuring core NMR spectra, whether 1D or 2D, the core sample needs to be placed in a magnetic field and the nuclear spin resonance is excited by applying a radio frequency pulse. The signal strength at different T2 times is then obtained by measuring the decay of the nuclear spin signal. In this study, 1D and 2D NMR analysis methods are combined with physical simulation experiments to establish two analytical methods for evaluating reservoir wettability.
Passey et al. (2011) believe that organic pores are hydrophobic, while inorganic pores are hydrophilic. Sang et al. (2018) and Gong et al. (2020) believe that when water or oil is sucked into the hydrophilic pipe, the capillary pressure is consistent with the direction of fluid flow. Under the action of capillary pressure, both water and oil can be adsorbed into the hydrophilic pipe. The higher the capillary pressure, the greater the imbibition power. When the oil is sucked into the hydrophobic pipe, the capillary pressure is still the driving force. However, when the water touches the hydrophobic pipe, the capillary pressure becomes the resistance, and the higher the capillary pressure, the greater the resistance. Therefore, even under vacuum conditions, water cannot be sucked into the organic pores. When calculating, the vacuum self-imbibition water volume can be regarded as inorganic content, the difference between the vacuum self-imbibition oil volume and the vacuum self-imbibition water volume is the organic content, and the difference between the vacuum self-imbibition oil volume and the pore volume is the absorbed oil volume. The pore volume can be calculated according to the calculation of the pores measured by helium gas. Based on the research of Sang et al. (2018), the following innovations are made in this paper. Firstly, the calibration equation of the signal strength and volume of the fluid NMR is established by 1D NMR, which is more accurate than the experimental results of oil absorption/water absorption obtained by the burette method adopted by Sang. Secondly, the feasibility of this experimental method was verified by 2D NMR and wetting Angle measurement. The calculation method is shown in Eqs 1–3.
Where,
Where,
Where,
According to the experiment of vacuum self-imbibition oil and vacuum self-imbibition water, it mainly includes vacuum device and NMR instrument. The 1D NMR instrument used is Recore-04 core NMR analyzer. The main test parameters are: echo interval time is 300 μm, echo number is 1,024, scan times are 64, wait time is 3,000 ms, gain is 50. The density and surface tension of the simulated oil were 0.80 g/cm3 and 25.98 mN/m at room temperature. The configuration of formation water is in accordance with laboratory standards: 10,000 salinity brine should be combined with 1L water, 4.49 g Nacl2, 4.49 g Kcl, 0.77 g Cacl2, and 0.51 g Mgcl2.
(1) The rock samples No.1 to No. 6 were placed in a drying oven and dried at 110°C for 24 h.
(2) Core porosity was measured by helium, core permeability was measured by nitrogen, and water drop Angle measuring instrument was used to measure the contact Angle between core and water.
(3) By roughly calculating the capillary resistance of shale core through the capillary force formula, it can be found that the core can be saturated under the pressure of negative pressure of 0.1 MPa, and the nuclear magnetic resonance curve of the core hardly changes after saturation to 24 h. So the rock samples No.1 to No. 6 were vacuumed and saturated with 140,000 ppm of formation water for 24 h (the formation water with 140,000 salinity was selected to simulate the salinity of formation water in Qinghai shale field), and 1D NMR T2 spectrum of the saturated water samples were measured.
(4) The rock samples after saturated water were replaced in a drying oven and dried at 110°C for 24 h. The dried rock sample was vacuumed with saturated oil for 24 h, and the 1D and 2D NMR T2 spectra of the rock samples after saturated oil were measured.
The bound water will be distributed on the surface of fine pore throat or large pore throat of the rock samples with strong hydrophilicity after saturated oil, while the non-wetting oil phase is distributed in the middle of pore throat, and the interaction force between the oil phase and the pore throat surface is very weak. According to the NMR surface relaxation principle, the stronger the interaction force between hydrogen-containing fluid and the pore throat surface, the shorter the relaxation time, and vice versa (Yang et al., 2019; Xiao et al., 2020). Therefore, the non-wetting oil phase is distributed in the part with a longer relaxation time, while the wetting aqueous phase is distributed in the part with a shorter relaxation time. When the sample is oil-wet, the wettable oil phase is distributed on the pore throat surface and the force with the larger pore throat surface is stronger, which inevitably leads to the relaxation time of oil phase is shortened and the relaxation time of aqueous phase is longer. Macroscopically, the NMR spectra of aqueous phase in saturated oil state shifted to the right compared with that in completely saturated water state. Based on the above principles, the wettability of rock samples can be rapidly evaluated by analyzing the NMR spectra and changes of the aqueous phase in the state of completely saturated water and saturated oil bound water. In addition, T1 and T2 can reflect the interaction force between the fluid and the pore throat surface. The T1 and T2 correlation could be represented by Eqs 4, 5.
By comparing formula (6), (7), it can be found that when the relaxation time of the fluid is ignored, the transverse relaxation time is still affected by the diffusion relaxation time, and the surface relaxation characteristics between the fluid and the pore throat surface cannot be accurately reflected, while the longitudinal relaxation time is not affected by the diffusion relaxation time. Therefore, T1 relaxation time is more suitable for the study of surface relaxation to characterize the strength of the force between the fluid and the pore throat surface. Based on the research of Xiao et al. (2021), this paper verifies the feasibility of the experimental method by measuring the contact Angle.
The bound water experiment was established based on vacuum pressurized saturated water and oil flooding, which mainly included vacuum pressurized device, ISCO displacement pump, manual pump, core holder and 2D NMR instrument, as shown in Figure 1. The 2D NMR analyzer used in the experiment is Geospec produced by Oxford, United Kingdom. The selected measurement parameters are consistent with 1D NMR.
(1) The rock samples No.7 to No. 10 were placed in a drying oven and dried at 110°C for 24 h.
(2) Core porosity was measured by helium, core permeability was measured by nitrogen, and water drop Angle measuring instrument was used to measure the contact Angle between core and water.
(3) The rock samples No.7 to No. 10 were vacuumed and saturated with 30,000 ppm of formation water for 24 h (the formation water with 30,000 salinity was selected to simulate the salinity of formation water in Jilin shale field). After that, the cores were saturated under pressure of 16 MPa for 24 h, and 2D NMR T2 spectrum of the saturated water samples were measured.
(4) The rock samples after saturated water were placed in the core holder to carry out the physical simulation experiment of oil flooding until the water was no longer produced, and the cores after flooding were carried out the pressurized saturated oil experiment.
(5) The cores in saturated water and saturated oil bound water state were analyzed in contrast by 2D NMR spectra.
Figures 2A–F are 1D NMR T2 spectra of vacuum self-imbibition oil and vacuum self-imbibition of rock samples No. 1, 2, 3, 4, 5 and 6, respectively. The calibration equation of fluid NMR signal amplitude and volume was established. The correlation between signal and volume is shown in formula 6, 7.
Among them,
According to formula (1)–(3) and (6), (7), quantitative analysis of the occurrence content of rock samples No. 1 to No. 6 was conducted, as shown in Table 2. The inorganic content of No. 1 to No. 2 and No. 5 to No. 6 is greater than the organic content, with the average inorganic content accounting for 79.98% and the average organic content accounting for 20.05%. Due to the nature of hydrophilic inorganic content and hydrophobic organic content of shale, samples No. 1 to No. 2 and No. 5 to No. 6 are hydrophilic. According to the proportion of inorganic and organic content, the hydrophilicity of samples No. 1 to No. 2 and No. 5 to No. 6 is ranked as No. 1, No. 2, No. 6 and No. 5 in descending order. The organic content of rock samples No. 3 to No. 4 is higher than the inorganic content, with the average organic content accounting for 56.45% and the average inorganic content accounting for 43.55%. The samples tend to be weakly oil-wet. The total adsorbed oil content of rock samples is higher than that of free oil, the average proportion of adsorbed oil is 68.02%, and the average proportion of free oil is 31.98%.
In order to verify the feasibility of the experimental method, the contact Angle between rock samples No. 1 to No. 6 and water was measured, as shown in Figure 3. Figure 3A–F shows water contact Angle of rock samples No. 1, 2, 3, 4, 5, and 6, respectively. Generally defined as water wetting when Ø<75°. When 75°<Ø<105°, it is neutral wetting. When Ø>105°, it is oil wet.
As can be seen from Table 3, the contact angles of rock samples No. 1, No. 2, No. 5 and No.6 are 46.4°, 59.5°, 90.0° and 66.6° respectively, and the hydrophilicity is ranked as No. 1, No. 2, No.6 and No. 5 in descending order. The contact Angle of rock samples No. 3 to No. 4 is about 110°, which tends to be weak oil wet. The above results are consistent with those of 1D NMR wettability analysis.
In addition, the oil saturated state of samples No. 1 to No. 6 was measured by 2D NMR. Figures 4A–F show the 2D NMR spectra of saturated oil in rock samples No. 1, 2, 3, 4, 5, and 6, respectively. Quantitative analysis of 2D NMR results was conducted, and the results were shown in Table 4.
As can be seen from Table 4, the average proportion of free oil of samples No. 1 to No. 6 is 28%, that of adsorbed oil is 72%. The error between the data measured by 2D NMR and that measured by vacuum imbibition method of Table 2 is within 4%. Therefore, the new experimental method is feasible.
According to the 2D NMR spectra of rock samples No. 7 to No. 10 in saturated water and saturated oil-bound water in Figure 5. Figures 5A,C,E,G are 2D NMR spectra of saturated water in rock samples No. 7, 8, 9, and 10, respectively; Figures 5B,D,F,H are 2D NMR spectra of saturated oil-bound water in rock samples No. 7, 8, 9, and 10, respectively. It can be seen that in saturated water state, the longitudinal relaxation time T1 of rock sample is mainly distributed between 1 and 250 ms, and the transverse relaxation time T2 is mainly distributed between 1 and 250 ms, with T1/T2 values ranging from 1 to 100. Some T1/T2 values exceed 100. After oil flooding, due to the larger pore throat radius, the smaller the capillary resistance. So the oil preferentially occurs in the large pore throat during oil flooding. Therefore, the long relaxation in the upper right corner of T1-T2 2D spectrum corresponds to the oil peak (T2>10 ms, T1>10 ms). The relaxation time of 1< T2<10 ms and 1< T1<10 ms corresponds to bound water peak. The T1/T2 values of the two peaks are different, the value of the oil peak ranges from 0.01 to 70, and the value of the bound water ranges from 0.1 to 7.
Based on the principles of section 2.3.2, the NMR T1 spectra of the aqueous phase in the state of completely saturated water and saturated oil-bound water in rock sample No. 7 to No. 10 were compared and analyzed, as shown in Figure 6. Figures 6A–D show the T1 NMR spectra of saturated water and saturated oil-bound water in rock samples No. 7, 8, 9, and 10, respectively. It can be seen from the figure that the signal amplitude of the short relaxation part of the four rock samples after oil flooding was significantly reduced (1–10 ms), and the overall signal of the NMR T1 spectra shifted to the right. This phenomenon shows that after oil flooding, the strength of the force between water and the pore throat wall is obviously weakened, reflecting the relaxation characteristics of free fluid. Therefore, the four rock samples show hydrophilic characteristics before oil flooding, and the overall oil-wet characteristics after oil flooding.
In order to further verify this result, the contact Angle between four rock samples and water before and after oil flooding was measured. Cores No. 8 and No. 9 were taken as representative cores, as shown in Figure 7. Figures 7A,C respectively show the water contact angle between of rock samples No. 8 and No. 9 before oil flooding. Figures 7B,D are the water contact angle of rock samples No. 8 and No. 9 after oil flooding. The contact angles between rock samples No. 7 to No. 10 and water before oil flooding are 40.4°, 66.6°, 22.3° and 30.4°, respectively, while the contact angles between rock samples No. 7 to No. 10 and water after oil flooding are 120.4°, 128.9°, 122.0° and 128.0°, respectively. The rock samples were water-wet before oil flooding and turned to oil-wet after oil flooding, which is consistent with the results obtained by T1-T2 NMR evaluation. Therefore, the wettability of reservoir rock samples can be evaluated effectively by measuring T1-T2 NMR spectra in completely saturated water and saturated oil-bound water, and analyzing the changes of the NMR spectra in the two states of water phase.
In this study, two kinds of physical simulation experimental methods for evaluating reservoir wettability were established by using vacuum self-imbibition oil and self-imbibition water combined with 1D NMR technology, or by analyzing the NMR spectra and changes of aqueous phase in the state of completely saturated water and saturated oil bound water. The following conclusions were obtained.
(1) By vacuum self-imbibition analysis, it is found that the inorganic content of samples No. 1 to No. 2 and No. 5 to No. 6 in Qinghai is higher than the organic content, with the average inorganic content accounting for 79.98% and the average organic content accounting for 20.05%. The samples are hydrophilic and the hydrophilic properties are ranked as No. 1, No. 2, No. 6 and No. 5 from strong to weak. The organic content of rock samples No. 3 to No. 4 is higher than the inorganic content, with the average organic content accounting for 56.45% and the average inorganic content accounting for 43.55%. The rock samples tend to be weakly oil-wet, and the results of wettability determination by contact Angle method are consistent with those by self-imbibition method.
(2) The average proportion of adsorbed oil is 68.02% and the average proportion of free oil is 31.98%. After the self-imbibition oil was measured by 2D NMR technology, the average proportion of free oil was 28%, and the average proportion of adsorbed oil was 72%. The error between the data measured by 2D spectrum and that measured by self-imbibition method was about 4%. Therefore, it is feasible to evaluate the reservoir wettability by self-imbibition method after two verification.
(3) After oil flooding, the signal amplitude of the short relaxation part of the four Jilin rock samples decreased significantly (that is, the water signal of 1–10 ms), and the overall signal of the NMR T1 spectrum shifted to the right. It shows that the strength of the force between water and the pore throat wall is obviously weakened after oil flooding, which reflects the relaxation characteristic of free fluid. Therefore, the four rock samples showed hydrophilic properties before oil flooding and oil-wet properties after oil flooding, which was consistent with the wettability results determined by contact Angle method. Therefore, the wettability of rock samples can be rapidly evaluated by analyzing the NMR spectra and changes of the aqueous phase in the state of completely saturated water and saturated oil bound water.
The original contributions presented in the study are included in the article/Supplementary Material, further inquiries can be directed to the corresponding author.
LY: Methodology, Software, Writing–original draft, Writing–review and editing. ZY: Methodology, Software, Supervision, Writing–review and editing. HL: Investigation, Methodology, Software, Supervision, Writing–review and editing. TZ: Methodology, Software, Supervision, Writing–review and editing. YZ: Methodology, Software, Supervision, Writing–review and editing. NW: Conceptualization, Investigation, Resources, Writing–review and editing. MD: Data curation, Investigation, Software, Writing–review and editing. QH: Conceptualization, Investigation, Software, Writing–review and editing. XC: Conceptualization, Formal Analysis, Investigation, Writing–review and editing. HM: Conceptualization, Methodology, Software, Writing–review and editing.
The author(s) declare financial support was received for the research, authorship, and/or publication of this article. This research is funded by the following projects. Research on Shale Oil Development Mechanism and Technology (2022kt1001). Research on Flow Law of Typical Low-Grade Reservoirs and New Methods of Enhanced Oil Recovery (2021DJ1102). Tight Oil Reproducibility Evaluation and Enhanced Recovery Mechanism Research (2021DJ2202). Fluid Occurrence Mechanism, Flow Mechanism and Enhanced Oil Recovery Technology in Shale Oil Reservoir (2021DJ 1804). Single Well EUR Laboratory Experimental Study on Multi-media Combination of Shale Oil (JI2021-117). Ministry of science and technology of PetroChina (2021ZZ01-03). Research on New Methods and Technologies of Enhanced Oil Recovery Experiment (2023ZZ04-01). The funder was not involved in the study design, collection, analysis, interpretation of data, the writing of this article or the decision to submit it for publication.
Author NW was employed by Baiyin Nonferrous Group Co., Ltd.
The remaining authors declare that the research was conducted in the absence of any commercial or financial relationships that could be construed as a potential conflict of interest.
All claims expressed in this article are solely those of the authors and do not necessarily represent those of their affiliated organizations, or those of the publisher, the editors and the reviewers. Any product that may be evaluated in this article, or claim that may be made by its manufacturer, is not guaranteed or endorsed by the publisher.
Anderson, W. G. (1986). Wettability literature survey- Part 2: wettability measurement. J. Petrol. Technol. 38 (11), 1246–1262. doi:10.2118/13933-pa
Anderson William, G. (1986). Wettability literature survey- Part 1: rock/oil/brine interactions and the effects of core handling on wettability. J. Petrol. Technol. 38 (10), 1125–1144. doi:10.2118/13932-pa
Donaldson, E. C., Thomas, R. D., and Lorenz, P. B. (1969). Wettability determination and its effect on recovery efficiency. Soc. Pet. Eng. J. 9 (1), 13–20. doi:10.2118/2338-pa
Feng, L., Zhang, J., Jiang, Z., Li, C., and Bai, Y. (2023). High-precision sedimentary cycle framework and organic matter enrichment response of Qingshankou Formation in Songliao Basin. Acta Petrol. Sin. 44 (2), 299–311.
Fleury, M., and Deflandre, F. (2003). Quantitative evaluation of porous media wettability using NMR relaxometry. Magn. Reson. Imaging 21 (3-4), 385–387. doi:10.1016/s0730-725x(03)00145-0
Gong, H., Li, Z., Lyu, W., Zhu, C., Sang, Q., Li, Y., et al. (2020). Evaluation methods of oil recovery in inorganic and organic matter of shale oil. Exp. Technol. Manage. 37 (10), 30–34+37. doi:10.16791/j.cnki.sjg.2020.10.009
Gu, Z., Liu, E., Wang, X., Li, R., Xu, J., and Zhou, B. (2021). Development characteristics and exploration potential of shale in Chang7 Member in southeast of Ordos Basin. Petrol. Geol. Recovery Effic. 28 (1), 95–105. doi:10.13673/j.cnki.cn37-1359/te.2021.01.012
Guo, J., Tao, L., Chen, C., Li, M., and Zhao, Z. (2020). A new method for evaluating the mixed wettability of shale in Longmaxi Formation in the southern Sichuan. Acta Petrol. Sin. 41 (2), 216–225.
Howard, J. J. (1998). Quantitative estimates of porous media wettability from proton NMR measurements. Magn. Reson. Imaging 16 (5-6), 529–533. doi:10.1016/s0730-725x(98)00060-5
Huang, X., Dou, L., Zuo, X., Gao, H., and Li, T. (2021). Dynamic imbibition and drainage laws of factures in tight reservoirs. Acta Petrol. Sin. 42 (7), 924–935.
Li, G., Wu, K., Zhu, R., Zhang, Y., Wu, S., Chen, Y., et al. (2023). Enrichment model and high efficiency production of thick plateau mountainous shale oil reservoir:a case study of the Yingxiongling shale oil reservoir in Qaidam Basin. Acta Petrol. Sin. 44 (1), 144–157.
Li, H., Guo, H., Wang, X., He, J., Sun, Y., and Xu, F. (2015). Influence of core wettability on NMR T_2 cutoff value of movable fluid. J. Xi'an Shiyou Univ. Nat. Sci. Edn.) 30 (5), 43–478.
Li, L., Xue, Y., Chen, X., Su, J., and Niu, J. (2019). Research and deevelopment of shale oil standard system. Technol. Superv. Petrol. Ind. 35 (12), 15–19. doi:10.3969/j.issn.1004-1346.2019.12.006
Liang, C., Xiao, L., Zhou, C., Guo, L., Hu, F., Liao, G., et al. (2019). Nuclear magnetic resonance characterizes rock wettability:preliminary experimental results. Chin. J. Geophys. 62 (11), 4472–4481. doi:10.6038/cjg2019M0266
Lin, H., Wang, S., Yang, Y., and Liu, X. (2020). Shale oil reservoir characteristics of middle permian lucaogou formation in bogda area. Fault-Block Oil Gas. Field 27 (4), 418–423. doi:10.6056/dkyqt202004003
Liu, D., Zhu, K., Bao, Y., Liu, T., and Chen, L. (2018). Organic geochemical characteristics of lower Jurassic shale in Xishan——jinhongshan area. J. Qinghai Univ. 36 (5), 89–96. doi:10.13901/j.cnki.qhwxxbzk.2018.05.014
Looyestijn, W., and Hofman, J. (2006). Wettability-index determination by nuclear magnetic resonance. SPE Reserv. Eval. Eng. 9 (2), 146–153. doi:10.2118/93624-pa
Minh, C. C., Crary, S., Singer, P. M., Valori, A., Bachman, N., Hursan, G., et al. (2015). “Determination of wettability from magnetic resonance relaxation and diffusion measurements on fresh-state cores,” in International Conference and Exhibition, Barcelona, Barcelona, Spain, 3-6 April, 2015.
Passey, Q. R., Bohacs, K. M., Esch, W. L., Klimentidis, R., and Sinha, S. (2011). “Geologic and petrophysical characterization of unconventional shale-gas reservoirs,” in International Oil and Gas Conference and Exhibition, Beijing, China, 8–10 June 2011.
Sang, Q., Zhang, S., Li, Y., Dong, M., and Bryant, S. (2018). Determination of organic and inorganic hydrocarbon saturations and effective porosities in shale using vacuum-imbibition method. Int. J. Coal Geol. 200, 123–134. doi:10.1016/j.coal.2018.10.010
Song, R., Liu, J., Yang, C., and Sun, S. (2022). Study on the multiphase heat and mass transfer mechanism in the dissociation of methane hydrate in reconstructed real-shape porous sediments. Energy 254, 124421. doi:10.1016/j.energy.2022.124421
Sun, J., Yang, Z., Liu, X., and Xiong, S. (2012). Reservoir wettability evaluation using nuclear magnetic resonance technology. Sci. Technol. Rev. 30 (27), 65–71. doi:10.3981/j.issn.1000-7857.2012.27.011
Wu, Z., Mou, B., Wang, X., Zhou, J., and Ni, F. (2001). Reservoir wettability and its measurement. Oilfield Chem. 18 (1), 90–96. doi:10.3969/j.issn.1000-4092.2001.01.028
Xiao, Q., Yang, Z., Wang, Z., Qi, Z., Wang, X., and Xiong, S. (2020). A full-scale characterization method and application for pore-throat radius distribution in tight oil reservoirs. J. Petrol. Sci. Eng. 187, 106857. doi:10.1016/j.petrol.2019.106857
Xiao, W., Yang, Y., Li, M., You, J., Zhao, J., Zheng, L., et al. (2021). Experimental study on the oil production characteristics during the waterflooding of different types of reservoirs in Ordos Basin, NW China. Petrol. explor. Dev. 48 (4), 935–945. doi:10.1016/s1876-3804(21)60078-2
Yan, J. (2001). A new method for determination of wettability of reservoir rocks. Petrol. explor. Dev. 28 (2), 83–86. doi:10.3321/j.issn:1000-0747.2001.02.025
Yang, Z., Liu, X., Li, H., Lei, Q., Luo, Y., and Wang, X. (2019). Analysis on the influencing factors of imbibition and the effect evaluation of imbibition in tight reservoirs. Petrol. explor. Dev. 46 (4), 779–785. doi:10.1016/s1876-3804(19)60235-1
Zeng, H., Huo, Q., Zhang, X., Fan, Q., Wang, Y., Lu, R., et al. (2022). Quantitative analysis on occurrence evolution of Gulong shale oil in Songliao Basin. Petrol. Geol. Oilfield Dev. Daqing 41 (3), 80–90.
Zhang, Y., Li, Y., Guo, W., Han, W., Li, Y., and Wang, W. (2021). Characteristics and main controlling factors of pore structures in low thermal maturity continental shale of Jurassic, northern Qaidam basin. Acta Geol. Sin. 95 (2), 565–577. doi:10.19762/j.cnki.dizhixuebao.2021103
Zhou, Z. (2023). Co-evolution characteristics of organic matter and reservoir in continental shale:a case study of Shahezi Formation in Changling Faulted Depression,Songliao Basin. Petrol. Geol. Exp. 45 (2), 243–251.
Keywords: shale oil, wettability, nuclear magnetic resonance, physical simulation experiment, pore fluid
Citation: Yao L, Yang Z, Li H, Zhou T, Zhang Y, Wang N, Du M, Huang Q, Chen X and Meng H (2024) Nuclear magnetic resonance study on wettability of shale oil reservoir. Front. Earth Sci. 12:1287221. doi: 10.3389/feart.2024.1287221
Received: 01 September 2023; Accepted: 16 January 2024;
Published: 15 February 2024.
Edited by:
Mehdi Ostadhassan, Northeast Petroleum University, ChinaReviewed by:
Rui Yang, China University of Geosciences Wuhan, ChinaCopyright © 2024 Yao, Yang, Li, Zhou, Zhang, Wang, Du, Huang, Chen and Meng. This is an open-access article distributed under the terms of the Creative Commons Attribution License (CC BY). The use, distribution or reproduction in other forums is permitted, provided the original author(s) and the copyright owner(s) are credited and that the original publication in this journal is cited, in accordance with accepted academic practice. No use, distribution or reproduction is permitted which does not comply with these terms.
*Correspondence: Lanlan Yao, MjM2MjI5NDEyNkBxcS5jb20=; Zhengming Yang, eXpobTY5QHBldHJvY2hpbmEuY29tLmNu; Haibo Li, bGloYWlibzA1QHBldHJvY2hpbmEuY29tLmNu
Disclaimer: All claims expressed in this article are solely those of the authors and do not necessarily represent those of their affiliated organizations, or those of the publisher, the editors and the reviewers. Any product that may be evaluated in this article or claim that may be made by its manufacturer is not guaranteed or endorsed by the publisher.
Research integrity at Frontiers
Learn more about the work of our research integrity team to safeguard the quality of each article we publish.