- 1Earth Observatory of Singapore, Nanyang Technological University, Singapore, Singapore
- 2Institut de Physique du Globe de Paris, Université Paris Cité, Paris, France
- 3Asian School of the Environment, Nanyang Technological University, Singapore, Singapore
Monitoring volcanoes is of the most importance in volcano risk mitigation to safeguard lives and economies. Thanks to recent technological advances, both on-ground and in space, our understanding of volcanic processes has improved significantly. Though there is no one-system-fits-all, optimizing infrastructure for efficient monitoring stands as key objective. The impacts of volcanic hazards can span from local to global scales, affecting us both in the short and long term. This highlights the worldwide significance of improving volcano monitoring. Previously reliant on local ground-based instruments, today’s monitoring approach is enhanced by remote and space-based techniques such as satellite remote sensing, scanning-Differential Optical Absorption Spectroscopy (DOAS), and infrasound. Designing an effective monitoring infrastructure for volcano observatories involves careful consideration of various factors such as network coverage, type of sensors, data transmission, and power supply to ensure that the targeted parameters meet the specific needs of each volcano (e.g., type of activities, early warning systems). Additionally, fostering collaboration and information sharing within the global scientific community is essential for addressing the current challenges in volcanology. In line with this, we’ve established the Global Volcano Monitoring Infrastructure Database (GVMID) to compile data from volcano monitoring across the globe. Global Volcano Monitoring Infrastructure Database serves as an integral component of WOVOdat, the global volcano unrest database, aiming to enhance our understanding of eruptive processes and improve eruption forecasts. The database incorporates monitoring metadata comprising networks, stations, and instruments, all standardized and managed using a MySQL relational database management system. Accessed through a web-based interface (https://wovodat.org/gvmid/home.php), GVMID offers an informative snapshot and foundational overview of the techniques and instruments in place at diverse volcanoes. This interactive platform allows for queries, visualizations, and downloads, serving as a valuable resource for the volcano community. GVMID can assist observatories in various ways, by: (a) Facilitating the setup or enhancement of monitoring systems for specific volcanoes. (b) Providing insights into the latest monitoring technologies and instrumentation. (c) Identifying existing monitoring gaps that could be addressed through remote sensing infrastructure and future instrument deployments. We extend an invitation to the global volcano community to actively participate in the development and enrichment of GVMID. Our aim is for it to become a continually updated and indispensable resource that caters to diverse needs within the volcanology community.
1 Introduction
Volcano monitoring encompasses a range of methods and measurement techniques tailored to specific volcanoes and monitoring objectives, and it is often a balance between institutional priorities and available resources (Ewert et al., 2005; Ewart et al., 2018; Carvelli et al., 2021). Rapid advances in monitoring instrumentations and techniques offers opportunities to improve detection, forecasting, and response to volcanic activities and hazards (National Academies of Sciences Engineering and Medicine, 2017; Pallister et al., 2019; Power et al., 2020; Lowenstern et al., 2022). Integration of multi-parameter datasets and the use of novel techniques (e.g., aerial imaging and gas sampling by drone, UV SO2 camera), along with enhanced spatio-temporal resolution and coverage through the combination of ground- and space-based monitoring capabilities, have improved unrest detection, evolution of eruptions, changes in eruption styles, mapping of eruption products and impacts, and enhanced eruption forecasts and hazard as3sessment (e.g., Scarpa, 2001; Sparks, 2003; Marzocchi et al., 2008; Tilling, 2008; Segall, 2013; Winson et al., 2014; Acocella et al., 2023). Significant eruptions such as Mount St. Helens in 1980 (Lipman and Mullineaux, 1981; Dzurisin, 2018), Mount Pinatubo in 1991 (Newhall and Punongbayan, 1996), Merapi in 2010 (Surono et al., 2012; Ratdomopurbo et al., 2013), Bardarbunga in 2014 (Sigmundsson et al., 2015), and Kilauea in 2018 (Neal et al., 2019; Neal and Anderson, 2020) have demonstrated the value of monitoring infrastructure and monitoring data in anticipating these events and their hazards. However, optimizing monitoring infrastructure is not straightforward as it involves rationalizing its efficiency, operational aspects, resource allocation, and capability (Ewart et al., 2018; Carvelli et al., 2021). These requires careful consideration of factors like network coverage, sensor types, data transmission, and power supply. This ensures that the parameters being monitored align with the specific requirements of each volcano, including the nature of activities and the implementation of early warning systems for risk mitigation. To optimize monitoring infrastructure capabilities for real-time data analysis, which is critical for timely and robust decision-making, observatories must prioritize fundamental research efforts aimed at finding the optimal design, upgrading instrumentation, and adopting innovative monitoring methods (Moran et al., 2008; Newhall and Pallister, 2015; National Academies of Sciences Engineering and Medicine, 2017). This also includes the use of state-of-the-art instrumental technology, extending network coverage, improving measurement sampling rates, and implementing automatic data processing (Lowenstern et al., 2022; Mandeville et al., 2022). Moreover, a well-defined data management strategy for volcano monitoring infrastructure is essential for its long-term usability and accessibility. This involves organizing, storing, and archiving data in standardized formats, along with appropriate key metadata elements, to ensure its integrity and enable future analysis and research (Newhall et al., 2017; Pallister et al., 2019; Ueda et al., 2019; Beauducel et al., 2020; Andrews et al., 2022). Different institutions may employ various techniques and methods based on their monitoring objectives, expertise, history of development, and available resources (Sparks et al., 2012; Lowenstern et al., 2022). The use of standardized metadata across different monitoring systems and institutions is crucial for easy data sharing, integration, and comparison. This standardization enhances data usability and accessibility (Newhall et al., 2017; Fujita et al., 2020; Andrews et al., 2022).
The Global Volcano Monitoring Infrastructure Database (GVMID; https://wovodat.org/gvmid/home.php) is aimed at documenting capabilities of volcano monitoring from the ground and space. It provides a snapshot and baseline view of the techniques and instrumentation that are in place at various volcanoes, which can be used by volcano observatories as reference to setup new monitoring systems or enhancing existing networks at a specific volcano. These data will allow the identification of monitoring gaps, which can be then targeted by remote sensing infrastructure and future instrument deployments (Biggs et al., 2014; Pritchard et al., 2018; Valade et al., 2019). GVMID is an integral component of the global volcano unrest database, WOVOdat, which stored the processed monitoring datasets is aimed to better understand the eruptive processes and improve eruption forecasts (Newhall et al., 2017; Costa et al., 2019). This resource is currently hosted at the Earth Observatory of Singapore and is intended for use by the global volcano community. It is important to note that the metadata stored and displayed in GVMID do not reflect real-time operational volcano monitoring infrastructure, and the ownership of the data remains with the data contributors. It does not aim to duplicate the databases of individual observatories or data centers but instead aims to bring together key information on volcano monitoring infrastructure as a community database.
In this paper we discuss various aspects related to GVMID, including the backend database structure and standard format, data contribution, web interface and tools for querying and visualizing data, discussion about potential use of the metadata. We emphasize that a comprehensive and well-designed monitoring infrastructure is crucial for improving volcano hazard forecasts. GVMID designed to provide an overview of the global volcano monitoring infrastructure, intended for use by scientific community. The database’s comprehensiveness depends on the active involvement of data contributors, encouraging them to consistently input and update their data. The more complete the records, the more useful the database becomes. Hence, we extend an invitation to volcano observatories and the scientific community to actively participate by contributing data, thereby enhancing the utility of the database.
2 Methods
2.1 Data policy and ownership
The data in WOVOdat are contributed by member observatories, universities, research agencies, and others in the interest of free and open, non-commercial uses such as education, scientific research, and responses to volcanic crises. We welcome all interested data contributors and users. Data contributors must register so that proper credit can be given for their data contributions. As a data repository, GVMID is not the proprietary of the datasets hosted in its database; the contributors maintain full control of the data ownership for all datasets that they contributed to the database. The Data Policy (https://wovodat.org/populate/dataPolicy.php) plays a crucial role in defining the principles and guidelines for data contribution and usage that supports community benefit as an open access database. Open access to data encourages the contribution, integration, and reuse of datasets from various sources, foster collaboration and facilitate interdisciplinary studies, and provides opportunities for new insights.
2.2 Volcano monitoring metadata
Volcano monitoring encompasses a range of methods and measurement techniques tailored to specific volcanoes and monitoring objectives (Lowenstern et al., 2022). Ground-based methods, such as earthquake monitoring using seismometers, ground deformation measurements using Global Positioning System (GPS), tiltmeters, and/or gas sampling, provide detailed insights into the internal processes of volcanoes. The optimization of volcano monitoring infrastructure involves improving its efficiency, operational capabilities, resource utilization, and the ability to detect changes in monitoring signals that reflect volcanic processes. In the past decades, volcano monitoring has transitioned to digital recording, real-time data transmission, higher sampling rates, and increased accuracy in various parameters. These advancements in monitoring techniques and instrumentation have significantly contributed to mitigating volcanic hazards. Moreover, techniques such as infrasound monitoring, and optical and thermal web cameras have been developed and are now integrated into volcano monitoring operations in some cases (Dietterich and Neal, 2022). On the other hand, remote sensing techniques, including satellite-based observations and aerial surveys, offer broader spatial coverage and enable the detection of changes in volcanic activity over larger areas (Reath et al., 2019; Poland et al., 2020; Poland and Zebker, 2022). The rapid growth in remote sensing technology and the adoption of unmanned aircraft systems (UAS) have significantly enhanced volcano monitoring capabilities (Darmawan et al., 2018; Román et al., 2022). Satellites equipped with optical, thermal, and synthetic aperture radar (SAR) sensors have improved our ability to detect ground deformation, rapidly measure topographic changes, map eruptive products, and detect and characterize thermal signals and ash clouds at high temporal and spatial resolutions (Biggs et al., 2016; Ebmeier et al., 2018; Giudicepietro, et al., 2019; Coppola et al., 2020; Schneider et al., 2020; Bemelmans et al., 2023). These remote-based and space-based technological advancements have helped bridge regional monitoring gaps, widening spatial coverage, especially for under-monitored and remote volcanoes.
The GVMID serves as a platform to showcase the current state-of-the-art capabilities, in spatio-temporal coverage, and various methods of volcano monitoring. Proper management of volcano monitoring data is crucial for its long-term usability and accessibility. This involves organizing, storing, and archiving data in standardized formats, along with appropriate metadata, to ensure its integrity and enable future analysis and research. However, different institutions may employ different techniques and methods based on their expertise and available resources. Hence, collaboration through data sharing and exchange expertise and experiences in managing and designing volcano monitoring network among institutions are necessary.
The metadata for volcano monitoring data needs to include information about the following key elements:
- Time range: This refers to the operational period of the monitoring infrastructure, indicating the period during which instrument had been installed and operational to collect and record data.
- Data source: It includes the data contributor(s) or owner(s) of the monitoring system, specifying the institutions or observatories responsible for installation, operation, and maintenance of the monitoring system and/or collecting, managing, and processed the data. It should also mention the catalog owner if the data are part of a larger data repository or catalog.
- Type of measurement: This indicates whether the metadata represents permanent (continuous instrumental measurement or records) or non-permanent (periodic survey or observation) monitoring efforts. Permanent measurements are typically collected through ground-based instruments or sensors that continuously monitor various parameters, while non-permanent measurements are often conducted during specific survey campaigns or observation periods and may revisit the same location (benchmark).
- Type of installation: This describes the type of monitoring infrastructure used for data collection. It can include in situ ground-based installations, remote ground-based installations, or airborne/space-based platforms. In situ ground-based installations involve instruments or sensors placed directly on or near the volcano, while remote ground-based installations may use remote sensing techniques or equipment located at a distance from the volcano. Airborne/space-based installations refer to measurements conducted using aircraft or satellite platforms.
- Monitoring methods: This section specifies the specific techniques or methods such as seismic monitoring (recording and analyzing seismic waves), deformation monitoring (measuring ground deformation using GPS or other techniques), gas monitoring (sampling and measuring volcanic gases), hydrology monitoring (monitoring water-related parameters), potential fields monitoring (measuring gravity, electrical or magnetic field variations), thermal monitoring (detecting and characterizing thermal signals), and meteorology monitoring (monitoring atmospheric conditions). It may also include other relevant methods employed in the monitoring efforts e.g., visual observation using closed circuit television (CCTV) or digital single-lens reflex (DSLR) camera.
The instrumental and data acquisition information, along with the above key elements of metadata, play a vital role in understanding the data and maximizing its usefulness. Standardizing metadata across different monitoring systems and institutions ensures that the data can be easily shared, integrated, and compared, facilitating collaboration, and enabling comprehensive volcano monitoring for risk mitigation purposes.
2.3 Database schema and structure
The GVMID database serves as a repository for volcano monitoring infrastructure information, encompassing networks, stations, and instruments. The database is designed to interlink observation systems and adhere to common standards for architecture and data sharing. The structure of the database allows for capturing detailed information about the monitoring infrastructure, from the hierarchical details of the monitoring network to the station site information and instrumentation specifics.
GVMID database schema and structure has evolved to follow and adjust the ever-growing development of volcano monitoring technique both in ground and space, adopting and adapting the new techniques and methods. It dynamically adopts new techniques and methods, such as the utilization of remote sensing approaches. This includes the deployment of instruments on mobile vehicles, enabling spatial observation coverage of volcanoes through drones and satellites (e.g., Valade et al., 2019; Ganci et al., 2020; Calvari et al., 2022; Aoki, 2023). Adaptations may involve structural modifications to the database to facilitate the integration of data from multiple stations within a shared network. As an example, data from permanent Global Navigation Satellite System (GNSS) stations in a deformation network at an active volcano can be integrated. This integration is demonstrated in monitoring magmatic intrusions, as illustrated by pressure source models (e.g., Beauducel et al., 2020; Bruno et al., 2022).
In February 2021, we co-organised a 4-day online workshop focusing on volcano monitoring infrastructure on the ground and in space in collaboration with the remote sensing community. This workshop, which brought together representatives from volcano observatories worldwide, aimed to enhance the understanding of the current volcano monitoring capabilities and constraints. During this workshop, the GVMID was introduced as a new initiative, a platform that serves to improve knowledge within the volcano community regarding the diverse aspects of volcano monitoring from both ground-based and space-based perspectives. Detailed materials of the workshop can be accessed at https://wovodat.org/about/cov_timeline.php. Subsequent to this event, we gathered valuable feedback on the optimal method for incorporating satellite remote sensing infrastructure into GVMID alongside ground-based infrastructure. Given that satellites are mobile and not fixed to specific points like ground stations, orbiting the globe and monitoring all volcanoes, practical considerations preclude marking every volcano with a satellite icon. Instead, we designate a volcano as having satellite monitoring coverage when data from satellite remote sensing, including InSAR, thermal imaging, and SO2 emissions, is stored in WOVOdat (the database of monitoring data from volcano unrest, wovodat.org). This designation also serves as metadata for the associated time series data. Additionally, GVMID is capable of storing campaign data. For instance, monitoring metadata for airborne UV DOAS gas measurements at Popocatépetl collected by airplane surveys is included. Popocatépetl volcano features SO2 gas emission data from an airplane survey, represented by an icon at the volcanic vent (https://wovodat.org/gvmid/index.php?type=single&vd_num=341090). This icon becomes visible when “Selected methods (Airplane/Satellite)” is chosen, and “Gas” is selected. Similarly, a satellite icon will appear when “Selected methods (Airplane/Satellite)” is chosen, and “Thermal” is selected.
While the metadata are stored in GVMID tables, the time series data or processed data recorded by a monitoring infrastructure of a volcano, using sensors located at a station belonging to the network of this volcano, will be archived in WOVOdat. It's important to note that WOVOdat does not archive raw waveform data or seismograms; it only stores time series processed data, such as RSAM (Real-time Seismic Amplitude Measurement), seismic event hypocenters, and earthquake counts. GVMID will visualize only the data stored in the database server. The data owner/source will be acknowledged on the webpage, including a URL link to the respective observatory/institution.
To ensure successful operation, the database follows a standardized structure and format. Each key component of the metadata is included in the GVMID registry and configured to establish communication with other tables in the database system. This standardized approach facilitates effective data management and interoperability.
Given the diverse nature of infrastructure monitoring data, including different data types, spatial variability, and time variability, the database is designed to handle and archive such data appropriately.
The structure allows for the monitoring of a volcano by multiple networks (seismic, deformation, gas, hydrology, thermal, potential field, and meteorology), and a network can monitor multiple volcanoes (Figure 1). Within a network, there are multiple stations, each hosting different instruments located at specific geographic positions. Metadata about instrument specifications, installation setups, data acquisition operations, and data owners can also be archived. Instrumentation metadata is crucial for understanding the process variables being measured, tracking original source of the data, and information on how the data is acquired or measured.
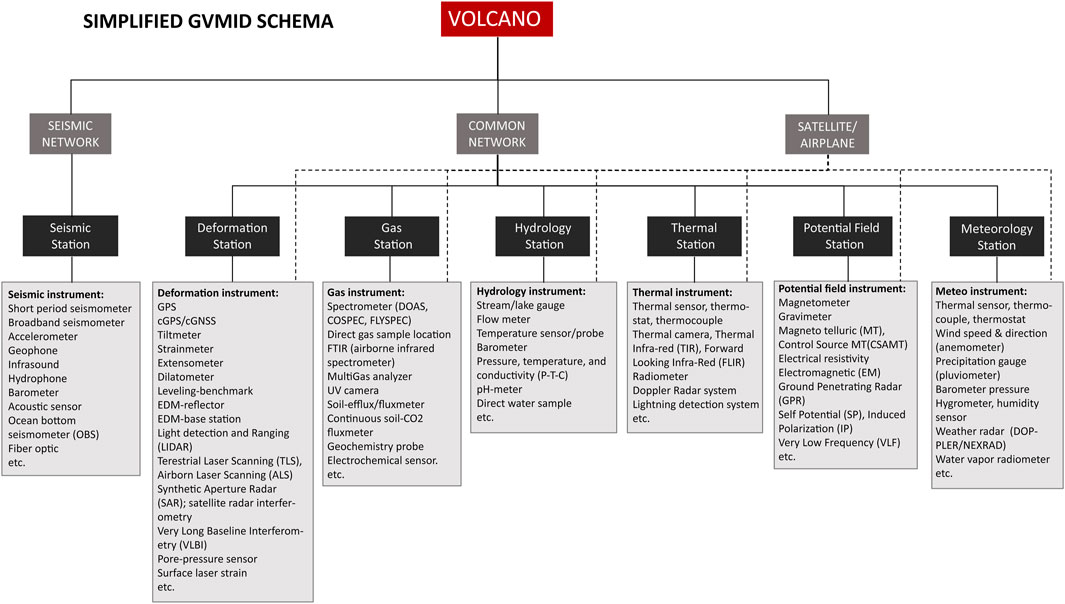
FIGURE 1. The GVMID database utilizes a hierarchical relational database management system, specifically MySQL. The database structure links all available instruments in a specific volcano through stations and networks, with the “volcano” serving as the parent table. Detail explanation of the schema can be found in the text.
The GVMID contains the following main tables:
- Volcano table: serves as the parent table and contains information about the volcanoes being monitored. It includes attributes such as volcano ID, volcano name, location coordinates, and other relevant descriptive details.
- Network table: contains the different monitoring networks involved in volcano monitoring. It includes attributes such as network ID, network name, and additional network-specific details.
- Station table: stores information about the individual monitoring stations within each network. It includes attributes such as station ID, station name, geographic location coordinates, and any other relevant station details.
- Instrument table: contains information about the instruments used for monitoring volcanic activity. It includes attributes such as instrument ID, instrument type, instrument specifications, installation setup details, data acquisition and other operational information.
- Satellite/airborne table: stores information about satellites and airplanes that are used for collecting data from above the surface of the earth. It includes attributes such as satellite/airplane ID, code, and name, satellite orbital type, spatial resolution, return time, angle of view, owners ID.
The GVMID metadata tables are linked hierarchically to the monitoring data collected from the instruments that stored in WOVOdat tables. Monitoring data include attributes such as data timestamp, data values, associated volcano, network, station, and instrument IDs for easy linkage and retrieval. The metadata associated with the monitoring data, including information about the data source, data owner(s), and other relevant details are also hosted in this table.
The relationships between these tables can be established using “primary key”- “foreign key” relations. For example, the volcano ID can serve as the primary key in the Volcano table and as a foreign key in the Network, Station, and Data tables. Similarly, the network ID and station ID can serve as foreign keys in the Station and Data tables, linking them to their respective networks. Indexing and constraints are defined to optimize query performance and ensure data integrity within the database.
The database structure is designed in MySQL, a hierarchical relational database management system, with interlinked tables capturing information about monitoring infrastructure components with Volcano as parent table. The metadata and standardization of the structure and format are important for the successful operation of the GVMID, ensuring effective communication between tables and proper archiving of diverse data types, spatial information, and time variability. Each table in the database should include the necessary metadata fields, such as creation date, last update date, and any other relevant information to track the history and changes in the metadata. The metadata in the GVMID are timestamp and georeferenced to enable the study of volcanic activity in both space and time. The timestamp field can be added to relevant tables to indicate when the data was recorded.
The database schema and structure for the GVMID (Figure 1) consist of main tables, with the following hierarchical relationship from Volcano as parent table, to Network, Station, Instrument and Component (only for seismic):
- Volcano-Network: Defines a many-to-many relationship between volcanoes and monitoring networks, as a volcano can be monitored by multiple networks, and a network can monitor multiple volcanoes.
- Network-Station: Establishes a one-to-many relationship between monitoring networks and stations, as a network can consist of multiple stations, but a station belongs to only one network.
- Station-Instrument: Is a one-to-many relationship between stations and instruments, as a station can host multiple instruments, but an instrument is associated with only one station.
- Satellite-Instrument: A satellite can host various instruments; therefore, it is also a one-to-many relationship between satellite and instruments, but an instrument only associated with one satellite.
The GVMID schema for the ground-based monitoring are shown by the continuous lines (Figure 1), which illustrate the hierarchical relations between Network-to-Station and Station-to-Instrument. This explains that each ground-based instrument table will need to be attached to a station table, in which is defined the geographic location where the instrument was installed. The instrument located at a specific station can be removed or replaced by another instrument. While for airborne/space-based monitoring, which in Figure 1 is shown by the dashed lines, the satellite/airplane table directly linked to instrument table. This explains that satellite or airplane are a mobile device, therefore no attachment to a specific station needed and that satellite or airplane can monitor many observation point locations simultaneously. Note that seismic network is separated to the ground-based common network (deformation, gas, hydrology, thermal, potential fields, and meteorology) to allow an earthquake data located by a seismic network that consist of more than one station, while other type of data belong to an instrument located at a specific station.
It is important to note that this is a simplified representation of the database schema. Technical insights and a more detailed description of the database can be found on the Documentation (https://wovodat.org/doc/database/1.1/index_gvmid.php) menu, providing a reference for those interested in understanding the database structure. It also provides descriptions of each table, including fields, indexes, links, and constraints.
2.4 Interactive web interface
2.4.1 Data contribution
To initiate the metadata submission, the user must create a login account by completing the registration form, which requires an email and institutional affiliation. This step ensures proper acknowledgment and record-keeping of the data uploaders, and their institutional affiliation will be recorded as the data owner of the uploaded datasets. The following link (https://wovodat.org/doc/system/CreateNewWOVOdatAccount.pdf) offers comprehensive guidance on creating a new user account. Once users have successfully registered and logged in, they can access the interactive tools within the GVMID system to submit their metadata (as shown in Figure 2). To accommodate the diverse metadata formats from different observatories, GVMID provides an Excel spreadsheet template format that conforms to the standard metadata format and structure of WOVOdat. This template facilitates the organization and structuring of metadata before uploading it to GVMID. The template includes several spreadsheets that cover various aspects of monitoring infrastructure metadata, such as contact information, monitoring network details, station information, instrument specifications, satellite/airplane information, satellite/airplane instrument details, and data format constraints. Users can download the spreadsheet template using the following link: https://wovodat.org/populate/submitDataDoc/GVMID_Monitoring%20metadata_template.xlsx.
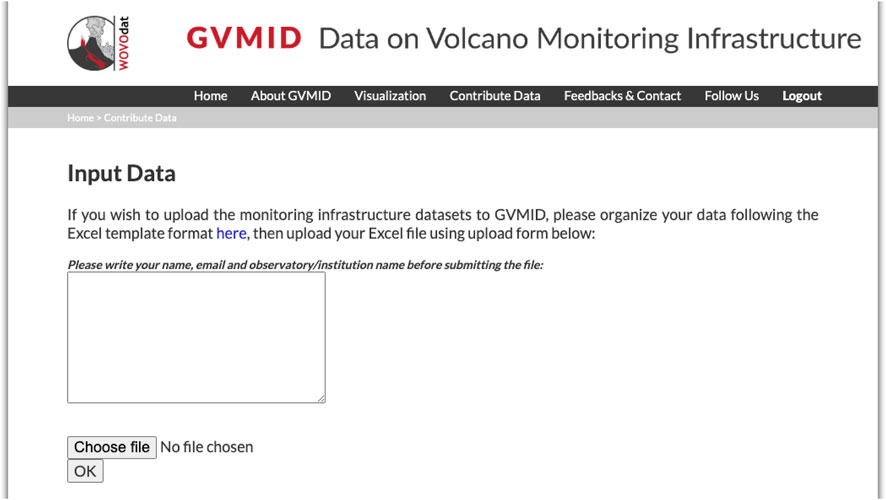
FIGURE 2. Screenshot of the GVMID online interface for contributing metadata. The user needs to provide contact details to ensure proper acknowledgment and record-keeping of the metadata uploaders. The user’s institutional affiliation becomes the owner of the datasets they upload.
Each spreadsheet within the template provides a structured format for users to input their metadata, ensuring consistency and coherence with the WOVOdat data format requirements (Venezky and Newhall, 2007; Newhall, et al., 2017). Users can fill in the relevant information based on their specific monitoring setup and observations. After completing the metadata template in Excel, users can submit their metadata through the online interface provided by GVMID. The system will convert the submitted metadata into WOVOdat-XML common formats (WOVOml) to ensure standardization. The converted metadata will then be uploaded and stored within the GVMID database system, making it easily accessible and ready for analysis. For a more detailed description of the GVMID database structure, including information on tables, fields, indexes, links, and constraints, which can refer to the provided link (https://wovodat.org/doc/database/1.1/index_gvmid.php). This documentation provides in-depth insights into the underlying structure and organization of the GVMID database. The data submission process, metadata template, and database structure of GVMID are designed to streamline the submission and storage of volcano monitoring infrastructure metadata, ensuring standardization, and facilitating data access and usage.
2.4.2 Metadata visualization
The GVMID visualization tools allow users to query and view monitoring infrastructure datasets for volcanoes worldwide. It is important to note that for accessing, visualizing, and downloading metadata through the GVMID visualization tools, login is not required. Login is only necessary to upload datasets. We provide a user manual and video tutorial on using the GVMID that can be found in the user manual page of the website, link: https://wovodat.org/about/manual_gvmid.php.
There are two types of visualization available:
a. Worldwide Volcano Map: Users can access the worldwide volcano map using the following link: https://wovodat.org/gvmid/index.php?type=world). The map displays circles of different colors, representing the number of monitoring stations installed within a 30 km radius of each volcanic vent. These circles are overlaid on a Google map. Users can interact with the map by selecting different monitoring metadata types and applying specific criteria in the filter panel, such as the time range, metadata source, and selected area Figure 3. When users click on a volcano icon on the map, they will be directed to the single volcano visualization.
b. Single Volcano Map: Users can access the single volcano map using the following link: (https://wovodat.org/gvmid/index.php?type=single&vd_num=282080 (example link for Sakura-jima volcano, japan). The map provides detailed information about the monitoring stations and available instruments installed within a 30 km radius of the volcanic vent. The station and instrument locations are displayed with different icon shapes and colors overlaid on a Google map. Figure 4 Users can refine their search and apply various criteria in the filter panel, such as the time range, selected area of interest, type of installation, metadata source, and type of instrument.
When users click on a station’s or instrument’s icon on the map, a popup box will appear. Detailed information about the selected station or instrument will be displayed below the Google map. Users can also search and select volcano monitoring metadata by applying criteria in the filter panel or by clicking on specific station/instrument icons on the single volcano visualization menu. The detailed information about the stations and instruments can be individually downloaded in CSV format s (Figure 5).
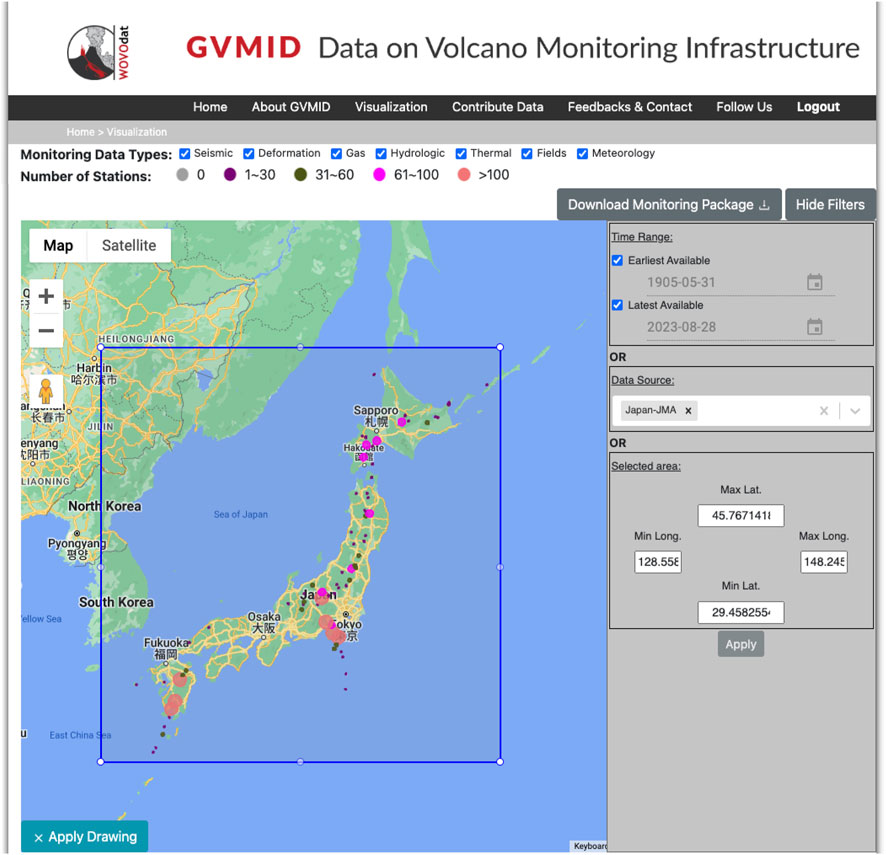
FIGURE 3. Screenshot of the Worldwide volcano map visualization displaying number of monitoring stations installed within 30 km from each volcano vent (colored circlesThe user can interactively select different monitoring metadata types a filter panel allows to select time range, metadata source, and specify rectangle bound or type in latitude/longitude coordinates in the filter box to create area of selection.
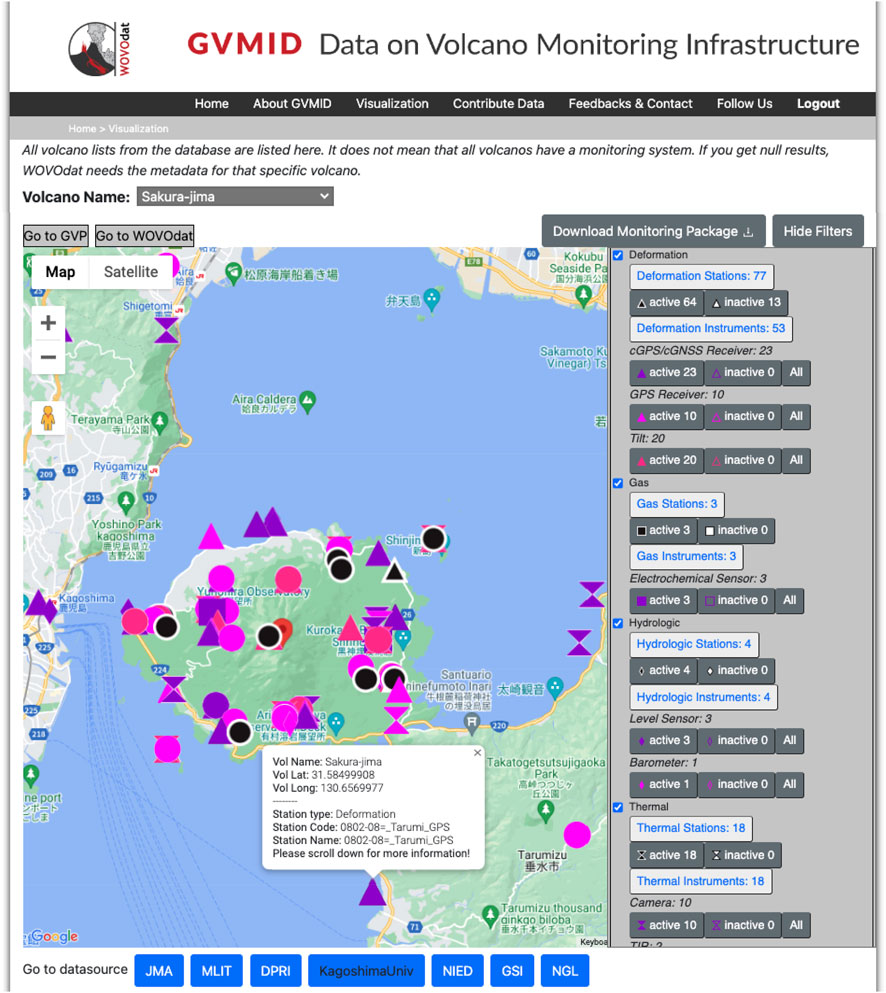
FIGURE 4. Screenshot of single volcano visualization tool for Sakura-Jima displaying map location of monitoring stations installed within 30 km from the vent (colour icons). Users can select criteria with options in the filter panel, e.g., time range, selected area of interest, type of installation, metadata source, type of instrument, etc.
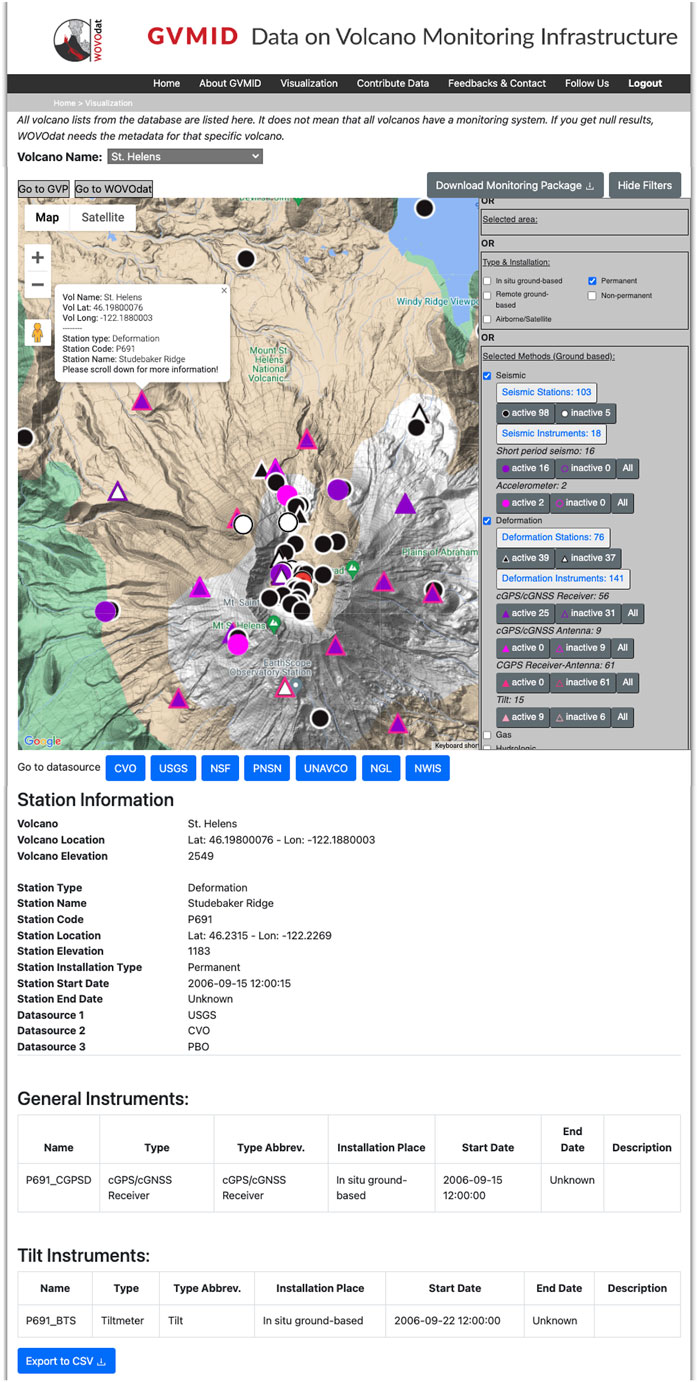
FIGURE 5. Screenshot of single volcano visualization tool for Mount St. Helens displaying popup box of deformation station P691, where cGPS/cGNSS and tiltmeter instruments were installed. Users can download the detail information on the selected station and instrument with the button “Export to CSV”, or download the entire monitoring infrastructure metadata of the volcano with the corresponding button in grey.
2.4.3 Download data
The GVMID platform offers a comprehensive and customizable experience for users to explore and download volcano monitoring metadata based on their specific requirements. The user-friendly interface provides various filtering options and the ability to download metadata packages at various levels of detail, providing flexibility and granularity in the metadata packages according to the specific needs. Users can utilize the filtering panel in the GVMID platform to search and select volcano monitoring metadata based on specific criteria. These criteria include the time range, metadata source, selected area, type of installation, installation status, monitoring method, and instrument type. The platform also includes a single volcano visualization menu where users can visualize the specific station/instrument detailed information.
To download metadata, users have options:
- They can individually download the detailed station/instrument information in CSV format file.
- Users can also download a monitoring package for a specific volcano or the worldwide volcano map. The downloaded package contains all the metadata for the selected volcano, including sets of spreadsheets with the different monitoring techniques such as Deformation, Potential field, Gas, Hydrologic, Meteorology, Seismic, and Thermal.
- Users can also download a monitoring package by clicking on the worldwide volcano map (Figure 4). In this case, the downloaded package will include sets of spreadsheets with detailed information about stations within the selected area and time range. However, please note that when selecting the worldwide package, only station information is stored in the downloaded package. To obtain instrument information, users will need to navigate to a specific volcano.
The downloaded monitoring packages are stored in zip files named “Monitoring Worldwide System package from wovodat.zip” for the worldwide package or “wovodat.zip” for a single volcano. These zip files contain Excel spreadsheet files representing different monitoring techniques, providing users with comprehensive and organized metadata for their analysis and research purposes.
2.5 Metadata in GVMID
The GVMID database can be a resource for the volcano community by incorporating metadata obtained from various sources, with major contributors from World Organisation of Volcano Observatory (WOVO), Japan Meteorological Agency (JMA), GNS Science, United State geological Survey (USGS), Instituto Geográfico Nacional (IGN), Philippine Institute of Volcanology and Seimology (PHIVOLCS), Centre for Volcanology and Geological Hazard Mitigation (CVGHM), open data centers University NAVSTAR Consortium (UNAVCO), Nevada geodetic Laboratory (NGL), Incorporated Research Institutions for Seismology (IRIS), universities as well as metadata compiled from published references.
Currently, the GVMID database archives metadata from more than 13,000 monitoring stations, comprising over 16,000 instruments. These stations and instruments cover a wide range of monitoring parameters and are associated with more than 550 volcanoes worldwide (Table 1; Table 2; Figure 6). Most of the metadata in the database pertain to deformation monitoring (63%), followed by seismic monitoring (19%) and gas monitoring (6%). Additionally, metadata related to thermal monitoring, hydrology, potential fields, and meteorology are also available. The total number of instruments is much larger than the number of stations (Table 1); this reflects frequent update on the instrumentations throughout the operation of a specific station. For now, there is still a low percentage of the actual monitoring infrastructure data represented in GVMID, as we expect there are many more instruments and stations that have not been shared by volcano observatories and monitoring agencies worldwide. The current data available in GVMID shows that most volcanoes are still not monitored or under-monitored, only ∼600 out of 1,302 Holocene volcanoes (VOTW; GVP, 2023; Sparks et al., 2012) have monitoring station(s) with ∼300 volcanoes at least equipped with 2 monitoring methods such as seismic, deformation or gas. The capability of monitoring infrastructure to detect unrest or an impending eruption also relies on factors, for example, instrument sensitivity, station location, spatial coverage of the network (e.g., Pallister and McNutt, 2015; Authors Anonymous, 2021; Rosi et al., 2022), but also includes considerations of data transmission, power supply, and accessibility for maintenance. Although these latter three aspects are not discussed in the current paper, they are key factors to consider in ensuring the sustainability of monitoring operations to meet the specific goals of monitoring, such as monitoring hazards like lahars or detecting precursory signals of eruptions for early warning.
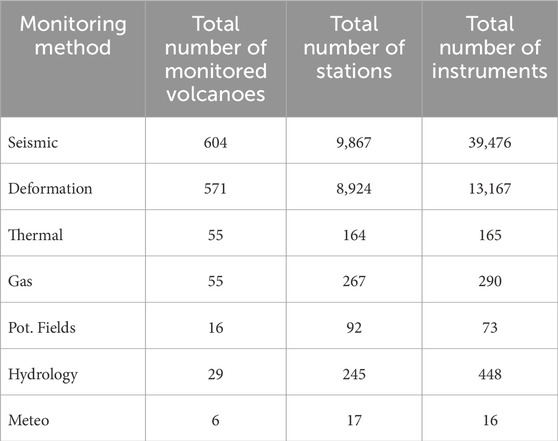
TABLE 1. Statistical representation of ground-based volcano monitoring infrastructure metadata currently archived in GVMID, as of August 2023.
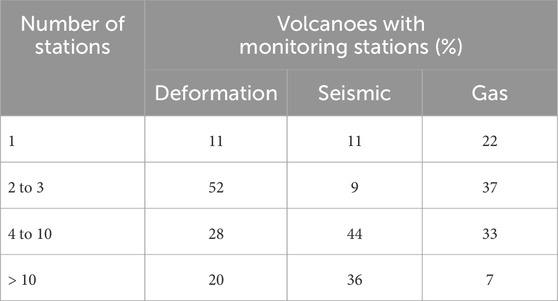
TABLE 2. Statistical representation of volcanoes (in percentage) covered by seismic, deformation and gas monitoring stations based on metadata currently archived in GVMID. Refer to Table 1 for the total number of monitored volcanoes.
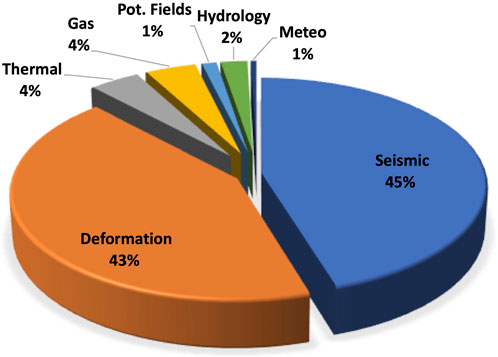
FIGURE 6. Pie chart of volcano monitoring infrastructure metadata currently archived in GVMID, color shown different monitoring method.
The global overview of monitoring infrastructure can be interactively explored through the worldwide map visualization tool of GVMID (https://wovodat.org/gvmid/index.php?type=world), and for more detail infrastructure at single volcano through https://wovodat.org/gvmid/index.php?type=single. We plan to continuously grow the GVMID, adding metadata mostly from the open access global database such as Earthscope consortium (https://www.earthscope.org/), International GNSS Service (IGS, https://igs.org/), and International Federation of Digital Seismograph Network (FDSN, https://www.fdsn.org/) for those stations operated around volcanoes. We also invite volcano observatories and the scientific community worldwide to actively participating in data contribution and updates, to help build a comprehensive and up-to-date volcano monitoring infrastructure database. The participation of volcano community reflects the importance of their expertise and data in shaping a comprehensive and valuable resource for building the global volcano monitoring database. This GVMID will be able to capture the continuity of evolving capabilities in volcano monitoring infrastructure, providing a valuable resource for observatories, scientists, and decision-makers worldwide.
3 Discussion
3.1 GVMID potential use
GVMID is an open access library of resources provided by volcano monitoring agencies within the volcano community and it aims at enhancing the understanding of current capabilities and limitations of volcano monitoring infrastructure. The provided datasets could serve as a valuable resource of reference for volcano community in multiple ways:
(a) Setting up new monitoring systems or improving network’s operational capability: Observatories can use the database to gather information about existing monitoring systems deployed at specific volcanoes. By studying the instrumentation and infrastructure used at other analogue volcanoes (Tierz et al., 2019), observatories can gain insights that help in designing, justifying, and make informed decisions when setting up new monitoring systems or improving their existing networks. This helps ensure that the monitoring infrastructure is optimized for the specific needs and characteristics of the volcano in question. In the case example presented by James et al. (2023), the assessment of the potential to detect vertical magma migration is conducted by analyzing the seismic network design. The study investigates how the arrangement and characteristics of seismic stations contribute to the ability to identify and track vertical movements of magma beneath the Earth’s surface. Such analysis could include considerations of station distribution, sensor sensitivity, and network coverage, providing valuable insights into the seismic network’s effectiveness in monitoring subsurface volcanic activity. The exchange of information among observatories through the database allows for knowledge sharing and learning from best practices. Even though the dataset does not reflect the active monitoring infrastructure currently operated at the volcanoes, it provides a snapshot view of the existing capabilities, to assess progress and identify areas for improvement. This information helps in strategic planning and decision-making regarding the allocation of resources for volcano monitoring infrastructure. Hence, to optimize the use of GVMID, the datasets in database should be populated through time and updated periodically.
(b) Exploring current instrumentation systems and trends: Accessing the database allows observatories to stay well-informed about the current instrumentation systems and trends, capturing the evolving capabilities in volcano monitoring infrastructure worldwide. Information on diverse monitoring techniques used at other volcanoes helps observatories assess the relevance of these systems to their specific monitoring networks, tailored to their needs and monitoring goals, whether in risk mitigation or research. Implementing suitable instrumentation systems enables observatories to enhance their capabilities in detecting unrest, monitoring volcanic activity, and assessing hazards.
(c) Identifying monitoring gaps: The database provides a comprehensive overview of volcano monitoring infrastructure worldwide. Observatories can use this information to identify gaps in monitoring coverage, particularly at volcanoes where the monitoring systems may be limited or insufficient. By recognizing these gaps, observatories can prioritize the deployment of remote sensing infrastructure and plan future instrument deployments to fill these monitoring gaps effectively. This ensures a more comprehensive and balanced spatial and temporal coverage of volcano monitoring. This also promotes collaboration and facilitates connections among users and providers of satellite data, fostering the integration of space-based observations into volcano monitoring practices.
3.2 Priorities in volcano monitoring infrastructure
The reliability and comprehensiveness of a monitoring infrastructure is essential for accurate eruption forecasting and hazard assessment such as ability in earliest unrest detection, efficiently monitor the evolving volcanic activity, and capable in quantifying the potential hazards (Marzocchi et al., 2008; Moran et al., 2008; Phillipson et al., 2013; Gottsmann et al., 2019). We highlighted some key factors and metrics to consider as diagnostic evaluation for establishing best practices in volcano monitoring:
a. Monitoring methods: Assessment of the range and diversity of monitoring methods in the infrastructure. A comprehensive monitoring system should cover key parameters such as seismicity, ground deformation, gas emissions, thermal anomalies, hydrological changes, and other relevant indicators specific to the volcano. The inclusion of multiple parameters provides a more holistic understanding of volcanic processes and enhances the ability to detect and monitor volcanic activity.
b. Instrument performance: Evaluating the quality and reliability of the monitoring instruments deployed within the infrastructure. Consideration of factors such as instrument sensitivity, accuracy, precision, and durability. Robust and well-maintained instruments are crucial for capturing accurate and reliable data, especially during critical periods of volcanic activity. Regular calibration and maintenance procedures should be in place to ensure instrument performance.
c. Network coverage and density: Consideration of the spatial coverage and density of monitoring instruments within the infrastructure. A well-designed network should provide sufficient coverage of the volcano and its surroundings to capture signals from various areas of interest. The density of instruments should be optimized to ensure the detection of subtle changes in volcanic activity and provide reliable data for hazard assessment.
d. Data quality and metadata: Assessment of the quality of the monitoring data and the availability of metadata. Robust quality control procedures should be in place to ensure data accuracy, consistency, and reliability. Metadata, including instrument specifications, calibration details, and processing procedures, are crucial for interpreting and validating the data. Well-documented metadata enhance the comprehensiveness and reliability of the monitoring infrastructure.
By evaluating these factors and metrics, it is possible to measure the reliability and comprehensiveness of a monitoring infrastructure. This process helps to identify strengths, weaknesses, and areas for improvement, leading to the optimization of capabilities in detecting volcanic unrest and monitoring its potential hazards.
4 Conclusion
The GVMID is aimed at documenting and improving capabilities of volcano monitoring from the ground and space, as it provides a snapshot and baseline of the techniques and instrumentation that are in place at various volcanoes. This can be use as source reference by volcano observatories and the volcano community in general. These metadata could be used for (a) designing the setup of new monitoring system or improving networks at a specific volcano, help design and justify their current system (b) exploring the up-to-date and state-of-the-art monitoring technology and instrumentation, (c) identifying monitoring gaps, which can be then targeted by remote sensing infrastructure and future instrument deployments.
It is important to maintain a comprehensive and up-to-date database that allow capturing the continuity of the evolving capability in volcano monitoring infrastructure, which is readily accessible to observatories, scientists, and decision-makers worldwide. For this, the GVMID focuses on promoting open data sharing, including establishing a consistent data format and standards, ensuring data quality for future usage of volcano monitoring infrastructure metadata.
The optimal monitoring infrastructure will cater to the need in each volcano, which varies according to volcanic activity and hazards related to the specific volcano; hence, the advancement of the monitoring infrastructure capability requires a good strategy and plan for optimal capability in monitoring, with key priorities such as diversity of monitoring methods, instrument performance, network design (coverage and density), and the availability of metadata.
Through the active participation of volcano observatories and the scientific community, the comprehensive volcano monitoring infrastructure database can grow and evolve. It facilitates data-driven analyses, fosters collaboration, and supports efforts to improve eruption forecasts, hazard evaluation, and mitigation actions.
Data availability statement
The datasets presented in this study can be found in online repositories. The names of the repository/repositories and accession number(s) can be found below: https://wovodat.org/gvmid/home.php.
Author contributions
CW: Conceptualization, Data curation, Writing–original draft, Methodology, Supervision, Validation, Visualization. NT: Conceptualization, Data curation, Software, Visualization, Writing–original draft, Methodology, Validation. TE: Conceptualization, Data curation, Writing–original draft. FC: Conceptualization, Project administration, Supervision, Writing–original draft, Methodology. BT: Project administration, Supervision, Writing–original draft, Conceptualization.
Funding
The author(s) declare financial support was received for the research, authorship, and/or publication of this article. This research/project is supported by the National Research Foundation, Singapore under its 8th NRF Investigatorship (NRFI) (Award NRF-NRFI08-2022-0015). This work comprises EOS contribution number 545.
Acknowledgments
The Authors wish to thanks the contributors of data, including the Smithsonian Institutions, USGS, JMA, NIED, GNS, PHIVOLCS, CVGHM, IGN, Earthscope Consortium, Cornell University, NGL, ISC, and other WOVO-IAVCEI’s observatories. Their invaluable contributions significantly enriched the database. Special appreciation is extended to Matt Pritchard for coordinating the workshop on volcano monitoring infrastructure on the ground and in space. We also thankful to Edwin Tan Seng Tat (Sysadmin) and Nguyen Duong Quynh Chi (IT programmer) for their dedicated efforts in maintaining the server and developing the GVMID web interface. The authors are grateful to the reviewers for their insightful comments and suggestions, which significantly contributed to the improvement of the manuscript.
Conflict of interest
The authors declare that the research was conducted in the absence of any commercial or financial relationships that could be construed as a potential conflict of interest.
Publisher’s note
All claims expressed in this article are solely those of the authors and do not necessarily represent those of their affiliated organizations, or those of the publisher, the editors and the reviewers. Any product that may be evaluated in this article, or claim that may be made by its manufacturer, is not guaranteed or endorsed by the publisher.
References
Acocella, V., Ripepe, M., Rivalta, E., Peltier, A., Galetto, F., and Joseph, E. (2023). Towards scientific forecasting of magmatic eruptions. Nat. Rev. Earth Environ. 5, 5–22. doi:10.1038/s43017-023-00492-z
Andrews, B. J., Costa, F., Venzke, E., and Widiwijayanti, C. (2022). Databases in volcanology. Bull. Volcanol. 84, 92. doi:10.1007/s00445-022-01597-x
Aoki, Y. (2023). Editorial: remote sensing in volcanology. Front. Earth Sci. 11, 40675. doi:10.3389/feart.2023.1140675
Authors Anonymous (2021). Overcoming financial limitations in global volcano monitoring. Nat. Commun. 12, 1976. doi:10.1038/s41467-021-22247-4
Beauducel, F., Lafon, D., Béguin, X., Saurel, J.-M., Bosson, A., Mallarino, D., et al. (2020). WebObs: the Volcano observatories missing link between research and real-time monitoring. Front. Earth Sci. 8. doi:10.3389/feart.2020.00048
Bemelmans, M. J. W., Biggs, J., Poland, M., Wookey, J., Ebmeier, S. K., Diefenbach, A. K., et al. (2023). High-resolution InSAR reveals localized pre-eruptive deformation inside the crater of agung volcano, Indonesia. J. Geophys. Res. Solid Earth 128, e2022JB025669. doi:10.1029/2022JB025669
Biggs, J., Ebmeier, S. K., Aspinall, W. P., Lu, Z., Pritchard, M. E., Sparks, R. S. J., et al. (2014). Global link between deformation and volcanic eruption quantified by satellite imagery. Nat. Commun. 5, 3471. doi:10.1038/ncomms4471
Biggs, J., Robertson, E., and Cashman, K. (2016). The lateral extent of volcanic interactions during unrest and eruption. Nat. Geosci. 9, 308–311. doi:10.1038/ngeo2658
Bruno, V., Aloisi, M., Gambino, S., Mattia, M., Ferlito, C., and Rossi, M. (2022). The most intense deflation of the last two decades at Mt. Etna: the 2019–2021 evolution of ground deformation and modeled pressure sources. Geophys. Res. Lett. 49, e2021GL095195. doi:10.1029/2021GL095195
Calvari, S., Bonaccorso, A., Cappello, A., Giudicepietro, F., and Sansosti, E. (2022). Volcanic processes monitoring and hazard assessment using integration of remote sensing and ground-based techniques. RemoteSens 14, 3626. doi:10.3390/rs14153626
Cervelli, P. F., Mandeville, C., Avery, V. F., and Wilkins, A. (2021). Five-year management plan for establishing and operating NVEWS—the national volcano early warning system. U.S. Geological Survey Open-File Report. doi:10.3133/ofr20211092
Coppola, D., Laiolo, M., Cigolini, C., Massimetti, F., Delle Donne, D., Ripepe, M., et al. (2020). Thermal remote sensing for Global Volcano Monitoring: experiences from the MIROVA system. Front. Earth Sci. 7, 362. doi:10.3389/feart.2019.00362
Costa, F., Widiwijayanti, C., Nang, T. Z. W., Fajiculay, E., Espinosa-Ortega, T., and Newhall, C. (2019). WOVOdat – the global volcano unrest database aimed at improving eruption forecasts. Disaster Prev. Manag. Int. J. 28, 738–751. doi:10.1108/DPM-09-2019-0301
Darmawan, H., Walter, T. R., Brotopuspito, K. S., Subandriyo, M., and Nandaka, I. G. M. A. (2018). Morphological and structural changes at the Merapi lava dome monitored in 2012–15 using unmanned aerial vehicles (UAVs). J. Volcanol. Geotherm. Res. 349, 256–267. doi:10.1016/j.jvolgeores.2017.11.006
Dietterich, H. R., and Neal, C. A. (2022). A look ahead to the next decade at US volcano observatories. Bull. Volcanol. 84, 63. doi:10.1007/s00445-022-01567-3
Dzurisin, D. (2018). Mount st. Helens retrospective: lessons learned since 1980 and remaining challenges. Front. Earth Sci. 6. doi:10.3389/feart.2018.00142
Ebmeier, S. K., Andrews, B. J., Araya, M. C., Arnold, D. W. D., Biggs, J., Cooper, C., et al. (2018). Synthesis of global satellite observations of magmatic and volcanic deformation: implications for volcano monitoring and the lateral extent of magmatic domains. J. Appl. Volcanol. 7, 2. doi:10.1186/s13617-018-0071-3
Ewert, J. W., Diefenbach, A. K., and Ramsey, D. W. (2018). 2018 update to the U.S. Reston, VA: Geological Survey national volcanic threat assessment. doi:10.3133/sir20185140
Ewert, J. W., Guffanti, M., and Murray, T. L. (2005). An assessment of volcanic threat and monitoring capabilities in the United States: framework for a national volcano early warning system (NVEWS). USGS Open-File Rep.
Fujita, E., Ueda, H., and Nakada, S. (2020). A new Japan volcanological database. Front. Earth Sci. 8, 205. doi:10.3389/feart.2020.00205
Ganci, G., Cappello, A., Bilotta, G., and Del Negro, C. (2020). How the variety of satellite remote sensing data over volcanoes can assist hazard monitoring efforts: the 2011 eruption of Nabro volcano. Remote Sens. Environ. 236, 111426. doi:10.1016/j.rse.2019.111426
Giudicepietro, F., Calvari, S., Alparone, S., Bianco, F., Bonaccorso, A., Bruno, V., et al. (2019). Integration of ground-based remote-sensing and in situ multidisciplinary monitoring data to analyze the eruptive activity of stromboli volcano in 2017-2018. Remote Sens. 11, 1813. doi:10.3390/rs11151813
Global Volcanism Program (GVP) (2021). Volcanoes of the World (v. 4.9.4; 17 mar 2021). Distributed by smithsonian institution, compiled by venzke, E. Washington, DC: GVP. doi:10.5479/si.GVP.VOTW5-2023.5.1
Gottsmann, J., Komorowski, J.-C., and Barclay, J. (2019). “Volcanic unrest and pre-eruptive processes: a hazard and risk perspective,” in Volcanic unrest: from science to society. Editors J. Gottsmann, J. Neuberg, and B. Scheu (Cham: Springer International Publishing), 1–21. doi:10.1007/11157_2017_19
James, K. E., Espinosa-Ortega, T., Tan, C. T., and Taisne, B. (2023). Assessing the potential to detect vertical magma migration using seismicity through the analysis of the seismic network design. J. Volcanol. Geotherm. Res. 435, 107769. doi:10.1016/j.jvolgeores.2023.107769
Lipman, P. W., and Mullineaux, D. R. (1981). The 1980 eruptions of Mount St. Helens. Washington: USGS. doi:10.3133/pp1250
Lowenstern, J. B., Wallace, K., Barsotti, S., Sandri, L., Stovall, W., Bernard, B., et al. (2022). Guidelines for volcano-observatory operations during crises: recommendations from the 2019 volcano observatory best practices meeting. J. Appl. Volcanol. 11, 3. doi:10.1186/s13617-021-00112-9
Mandeville, C., Cervelli, P. F., Avery, V. F., and Wilkins, A. (2022). The Volcano hazards program — strategic science plan for 2022–2026. Reston, VA: USGS. doi:10.3133/cir1492
Marzocchi, W., Sandri, L., and Selva, J. (2008). BET_EF: a probabilistic tool for long- and short-term eruption forecasting. Bull. Volcanol. 70, 623–632. doi:10.1007/s00445-007-0157-y
Moran, S. C., Freymueller, J. T., LaHusen, R. G., McGee, K. A., Poland, M. P., Power, J. A., et al. (2008). Instrumentation recommendations for volcano monitoring at US volcanoes under the national volcano early warning system. Sci. Investig. Rep. 47, 2008–5114. doi:10.3133/sir20085114
National Academies of Sciences Engineering and Medicine (2017). Volcanic eruptions and their repose, unrest, precursors, and timing. Washington, D.C.: National Academies Press. doi:10.17226/24650
Neal, C. A., and Anderson, K. R. (2020). Preliminary analyses of volcanic hazards at Kīlauea Volcano. Reston, VA: Hawai. doi:10.3133/ofr20201002
Neal, C. A., Brantley, S. R., Antolik, L., Babb, J. L., Burgess, M., Calles, K., et al. (2019). The 2018 rift eruption and summit collapse of Kīlauea Volcano. Science 363, 367–374. doi:10.1126/science.aav7046
Newhall, C. G., Costa, F., Ratdomopurbo, A., Venezky, D. Y., Widiwijayanti, C., Win, N. T. Z., et al. (2017). WOVOdat – an online, growing library of worldwide volcanic unrest. J. Volcanol. Geotherm. Res. 345, 184–199. doi:10.1016/j.jvolgeores.2017.08.003
Newhall, C. G., and Pallister, J. S. (2015). “Using multiple data sets to populate probabilistic volcanic event trees,” in Volcanic hazards, risks and disasters. Editors J. F. Shroder, and P. Papale (Boston: Elsevier), 203–232. doi:10.1016/B978-0-12-396453-3.00008-3
Newhall, C. G., and Punongbayan, R. S. (1996). Fire and mud: eruptions and lahars of Mount Pinatubo. Seattle and London: University of Washington Press. Available at: https://pubs.usgs.gov/pinatubo/index.html.
Pallister, J., and McNutt, S. R. (2015). “Chapter 66 - synthesis of Volcano Monitoring,” in The encyclopedia of volcanoes. Editor H. Sigurdsson (Amsterdam: Academic Press), 1151–1171. doi:10.1016/B978-0-12-385938-9.00066-3
Pallister, J., Papale, P., Eichelberger, J., Newhall, C., Mandeville, C., Nakada, S., et al. (2019). Volcano observatory best practices (VOBP) workshops - a summary of findings and best-practice recommendations. J. Appl. Volcanol. 8, 2. doi:10.1186/s13617-019-0082-8
Phillipson, G., Sobradelo, R., and Gottsmann, J. (2013). Global volcanic unrest in the 21st century: an analysis of the first decade. J. Volcanol. Geotherm. Res. 264, 183–196. doi:10.1016/j.jvolgeores.2013.08.004
Poland, M. P., Lopez, T., Wright, R., and Pavolonis, M. J. (2020). Forecasting, detecting, and tracking volcanic eruptions from space. Remote Sens. Earth Syst. Sci. 3, 55–94. doi:10.1007/s41976-020-00034-x
Poland, M. P., and Zebker, H. A. (2022). Volcano geodesy using InSAR in 2020: the past and next decades. Bull. Volcanol. 84, 27. doi:10.1007/s00445-022-01531-1
Power, J. A., Haney, M. M., Botnick, S. M., Dixon, J. P., Fee, D., Kaufman, A. M., et al. (2020). Goals and development of the Alaska Volcano Observatory seismic network and application to forecasting and detecting volcanic eruptions. Seismol. Res. Lett. 91, 647–659. doi:10.1785/0220190216
Pritchard, M. E., Biggs, J., Wauthier, C., Sansosti, E., Arnold, D. W. D., Delgado, F., et al. (2018). Towards coordinated regional multi-satellite InSAR volcano observations: results from the Latin America pilot project. J. Appl. Volcanol. 7, 5. doi:10.1186/s13617-018-0074-0
Ratdomopurbo, A., Beauducel, F., Subandriyo, J., Nandaka, I. G. M. A., Newhall, C. G., Suharna, G., et al. (2013). Overview of the 2006 eruption of Mt. Merapi. J. Volcanol. Geotherm. Res. 261, 87–97. doi:10.1016/j.jvolgeores.2013.03.019
Reath, K., Pritchard, M., Poland, M., Delgado, F., Carn, S., Coppola, D., et al. (2019). Thermal, deformation, and degassing remote sensing time series (CE 2000–2017) at the 47 most active volcanoes in Latin America: implications for volcanic systems. J. Geophys. Res. Solid Earth 124, 195–218. doi:10.1029/2018JB016199
Román, A., Tovar-Sánchez, A., Roque-Atienza, D., Huertas, I. E., Caballero, I., Fraile-Nuez, E., et al. (2022). Unmanned aerial vehicles (UAVs) as a tool for hazard assessment: the 2021 eruption of Cumbre Vieja volcano, La Palma Island (Spain). Sci. Total Environ. 843, 157092. doi:10.1016/j.scitotenv.2022.157092
Rosi, M., Acocella, V., Cioni, R., Bianco, F., Costa, A., De Martino, P., et al. (2022). Defining the pre-eruptive states of active volcanoes for improving eruption forecasting. Front. Earth Sci. 10, 795700. doi:10.3389/feart.2022.795700
Schneider, D. J., Van Eaton, A. R., and Wallace, K. L. (2020). Satellite observations of the 2016–2017 eruption of Bogoslof volcano: aviation and ash fallout hazard implications from a water-rich eruption. Bull. Volcanol. 82, 29. doi:10.1007/s00445-020-1361-2
Segall, P. (2013). Volcano deformation and eruption forecasting. Geol. Soc. Lond. Spec. Publ. 380, 85–106. doi:10.1144/SP380.4
Sigmundsson, F., Hooper, A., Hreinsdóttir, S., Vogfjörd, K. S., Ófeigsson, B. G., Heimisson, E. R., et al. (2015). Segmented lateral dyke growth in a rifting event at Bárðarbunga volcanic system, Iceland. Nature 517, 191–195. doi:10.1038/nature14111
Sparks, R. S. J. (2003). Forecasting volcanic eruptions. Earth Planet. Sci. Lett. 210, 1–15. doi:10.1016/S0012-821X(03)00124-9
Sparks, R. S. J., Biggs, J., and Neuberg, J. W. (2012). Monitoring volcanoes. Science 335, 1310–1311. doi:10.1126/science.1219485
Surono, S., Jousset, P., Pallister, J., Boichu, M., Buongiorno, M. F., Budisantoso, A., et al. (2012). The 2010 explosive eruption of Java’s Merapi volcano—a ‘100-year’ event. J. Volcanol. Geotherm. Res. 241–242, 121–135. doi:10.1016/j.jvolgeores.2012.06.018
Tierz, P., Loughlin, S. C., and Calder, E. S. (2019). VOLCANS: an objective, structured and reproducible method for identifying sets of analogue volcanoes. Bull. Volcanol. 81, 76. doi:10.1007/s00445-019-1336-3
Tilling, R. ∼I. (2008). The critical role of volcano monitoring in risk reduction. Adv. Geosci. 14, 3–11. doi:10.5194/adgeo-14-3-2008
Ueda, H., Yamada, T., Miwa, T., Nagai, M., and Matsuzawa, T. (2019). Development of a data sharing system for Japan volcanological data network. J. Disaster Res. 14, 571–579. doi:10.20965/jdr.2019.p0571
Valade, S., Ley, A., Massimetti, F., D’Hondt, O., Laiolo, M., Coppola, D., et al. (2019). Towards Global Volcano Monitoring using multisensor sentinel missions and artificial intelligence: the MOUNTS monitoring system. Remote Sens. 11, 1528. doi:10.3390/rs11131528
Venezky, D. Y., and Newhall, C. G. (2007). WOVOdat design document: the schema, table descriptions, and create table statements for the database of worldwide volcanic unrest (WOVOdat Version 1.0). Version 1. doi:10.3133/ofr20071117
Keywords: GVMID, WOVOdat, volcano monitoring, infrastructure, database, web interface
Citation: Widiwijayanti C, Thin Zar Win N, Espinosa-Ortega T, Costa F and Taisne B (2024) The global volcano monitoring infrastructure database (GVMID). Front. Earth Sci. 12:1284889. doi: 10.3389/feart.2024.1284889
Received: 29 August 2023; Accepted: 22 January 2024;
Published: 07 February 2024.
Edited by:
Severine Moune, UMR6524 Laboratoire Magmas et Volcans (LMV), FranceReviewed by:
Lauren N. Schaefer, United States Geological Survey, United StatesSilvia Massaro, Università degli Studi Aldo Moro, Italy
Mauro Rosi, University of Pisa, Italy
Masato Iguchi, Kyoto University, Japan
Copyright © 2024 Widiwijayanti, Thin Zar Win, Espinosa-Ortega, Costa and Taisne. This is an open-access article distributed under the terms of the Creative Commons Attribution License (CC BY). The use, distribution or reproduction in other forums is permitted, provided the original author(s) and the copyright owner(s) are credited and that the original publication in this journal is cited, in accordance with accepted academic practice. No use, distribution or reproduction is permitted which does not comply with these terms.
*Correspondence: Christina Widiwijayanti, Y3dpZGl3aWpheWFudGlAbnR1LmVkdS5zZw==