- Department of Biology, Cape Breton University, Sydney, NS, Canada
Amniota is a tetrapod group that was recognized originally in the 19th century on the basis of developmental features (extra-embryonic membranes), but since the widespread adoption of phylogenetic systematics in the late 20th century, it has been recast as a crown clade. The oldest amniotes are Late Carboniferous in age (ca. 318 million years ago), and they are preserved in coal beds and lycopod tree stumps that have yielded rich faunas of temnospondyls, anthracosaurs, and other early tetrapods. Numerous phylogenetic studies of the past three decades have focused on the relationships of amniotes to other early tetrapods, resulting in the prevailing picture that Amniota is the crown of a total group with many extinct Paleozoic clades comprising its stem group. The content and the sequence of branching among stem amniotes are vigorously debated topics in early tetrapod paleontology. The ‘traditional’ stem amniote group Diadectomorpha has been proposed as the sister group of synapsid amniotes in a series of papers by one research group. If correct, the placement of Diadectomorpha within Amniota implies that high-fiber herbivory, a key component of terrestrial vertebrate ecosystems of modern aspect, is an entirely amniote phenomenon. Another stem group, Recumbirostra, has been recovered by other researchers within Amniota as reptiles. Recumbirostrans exhibit cranial features that are correlated with a fossorial lifestyle, and most species for which we have postcrania reveal the phenomena of body elongation and many of these exhibit limb reduction. If correct, the identification of recumbirostrans as early reptiles not only greatly augments the content of Paleozoic Amniota but also marks the evolution of skeletal features indicative of a burrowing lifestyle that predates the appearance of unequivocal fossorial amniotes (cistecephalid dicynodonts) by 40 million years. However, it is premature to accept either diadectomorphs or recumbirostrans as amniotes, given issues with scorings for amniote taxa, character argumentation, and poor homologization of braincase features between amniote and anamniote taxa.
Introduction
Mammals, birds, crocodiles, tuataras, lizards, snakes, and turtles were recognized to form a natural group by Haeckel (1866) because they shared a reproductive strategy that involved extra-embryonic membranes that, in turn, allowed them to forgo the larval stages and the metamorphosis characteristic of amphibian and fish development. Vertebrate paleontologists of the late 19th and much of the 20th centuries acknowledged this classification but parsed their research on in an evolutionary systematic (non-cladistic) context, which accepted a paraphyletic class Reptilia that gave rise to classes Mammalia and Aves. During this time, some tetrapods of Permo-Carboniferous age (ca. 323.2 to 273.01 Ma) as reptiles (i.e., amniotes) that were generally regarded to be amphibians (here, anamniotes) were proposed by some vertebrate paleontologists as reptiles.
One example concerns amniote-like tetrapods known as diadectomorphs. E. D. Cope (1878) described the first diadectomorph, Diadectes sideropelicus, and descriptions of other diadectomorphs (Diasparactus zenos, Desmatodon hollandi, and Limnoscelis paludis) appeared in the following decades, and these were regarded as reptiles (e.g., Romer, 1946; Watson, 1957; Parrington, 1959) until the 1960s, when diadectomorphs were reinterpreted to be amphibians (Romer, 1967; Carroll, 1969). The first phylogenetic analyses to include diadectomorphs as ingroup taxa recovered them as a clade that was the sister group of Amniota (Gauthier et al., 1988; Laurin and Reisz, 1995).
Another assemblage of Permo-Carboniferous tetrapods that were mooted as reptiles is microsaurs. The name Microsauria was created by Dawson (1863) for the oldest known amniote Hylonomus lyelli, a diminutive reptile from the Bashkirian of Canada. Following Dawson (1863), subsequent workers described numerous small, lizard-like tetrapods from Permo-Carboniferous localities across North America and Europe as microsaurs. By the middle of the 20th century, H. lyelli had been ousted from Microsauria, and the group was envisioned as an assemblage of anamniotes with close affinities to nectrideans. Species of microsaurs and reptiles were confused with one another, and some researchers, such as Westoll (1942) and Vaughn (1962), argued that reptiles had evolved from microsaurs. Romer (1967) demurred and proposed it was more likely that microsaurs had evolved from reptiles. By the time Carroll and Gaskill (1978) published their monographic review of microsaurs, the consensus among early tetrapod workers viewed microsaurs as lepospondyl amphibians. The first major phylogenetic studies to include microsaurs recovered them outside of a clade of amniotes and diadectomorphs, thereby reinforcing the consensus view (Carroll, 1995; Laurin and Reisz, 1997; Ruta et al., 2003).
Following the widespread adoption of phylogenetic systematics over the past four decades, the interrelationships of amniotes and their closest relatives have been enthusiastically investigated and galvanized by computed tomography, which has greatly aided the discovery and the documentation of the distribution of characteristic states in both new and previously described early tetrapod fossils. On the contrary, the overall picture generated by the early-tetrapod research community agrees that Amniota is a clade (monophyletic group) that is divided into Synapsida on one hand and Reptilia on the other hand; slight disagreements on the exact placement of certain early amniote groups continue (e.g., varanopids: Ford and Benson, 2019; Benoit et al., 2021), but they do not greatly alter the content or the reconstruction of the general scope of early amniote evolution (Figure 1). However, a series of papers by two separate research groups have proposed that either diadectomorphs or (a subset of) microsaurs can be placed in Amniota, each with slightly different implications for interpretations of early amniote history.
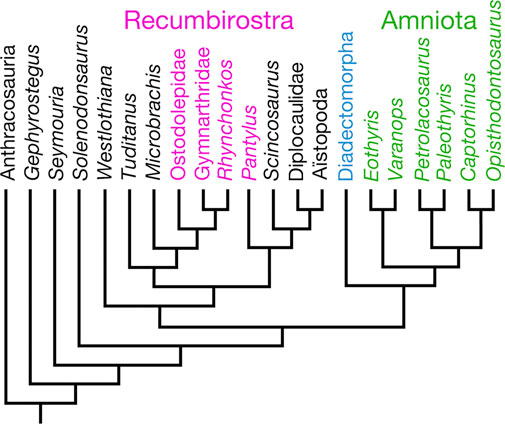
Figure 1. Amniota and their closest relatives, mostly after figure 9 in Marjanović and Laurin (2019). Some “microsaur” taxa that appear in Marjanović and Laurin (2019), i.e., Asaphestera, Saxonerpeton, Hapsidopareion, and “modern amphibians,” have been excluded for clarity.
Systematic overview
Diadectomorphs as amniotes
In contrast to previous cladistic work identifying Diadectomorpha as the sister group of Amniota (Gauthier et al., 1988; Laurin and Reisz, 1995, 1997), Berman (2000, 2013) recovered the former group within the latter as the sister group of Synapsida. Berman’s (2000) initial proposal that diadectomorphs were amniotes was based on results from the analysis of a small data matrix (9 taxa × 8 characters), followed by the results of the analysis of a larger data matrix (9 taxa × 40 characters: Berman, 2013). However, in the former analysis, the ingroup included higher taxa (Lepospondyli, Temnospondyli, etc.), for which ancestral states were reconstructed by the author, six diadectomorph genera were included in the ingroup (although apparently at the expense of the higher anamniote ingroups used in Berman, 2000) in the latter to test diadectomorph monophyly, and scorings were determined for the terminal taxa Synapsida and Reptilia, as before. The second study recovered a monophyletic Diadectomorpha in a sister group relationship with Synapsida (Berman, 2013).
Later, Klembara et al. (2019) revisited the conclusions of Berman (2000) and Berman (2013), following the description of new otic morphology informed from computed tomography on the diadectomorphs Diadectes absitus and Orobates pabsti, as well as CT data from the seymouriamorph Seymouria baylorensis and the captorhinid reptile Labidosaurus hamatus. On a much larger scale than Berman (2000) and Berman (2013), Klembara et al. (2019) examined the relationships of seven diadectomorphs in a data matrix that included 8 amniotes, 10 seymouriamorphs, 12 anthracosaurs, several other reptiliomorph species, and a small selection of non-reptiliomorphs. Their parsimony analysis found 421 optimal trees, with Diadectomorpha forming a sister group relationship with Synapsida in 60% of these trees (Klembara et al., 2019). Following re-weighting of characters, the authors re-ran their analysis and found a single optimal tree, which recovered Diadectomorpha and Synapsida as sister taxa within Amniota (Klembara et al., 2019). Marjanović and Laurin (2019) recovered a diadectomorph–synapsid sister group relationship, following their analysis of the corrected and augmented data matrix of Ruta and Coates (2007).
Most recently, Klembara et al. (2021) revisited their diadectomorphs-as-synapsids hypothesis, following a CT study of the skull of the diadectomorph Limnoscelis dynatis. Parsimony analysis of their augmented data matrix found 165 optimal trees, in 89 trees of which (54% of trees) Diadectomorpha and Synapsida were recovered in a sister group relationship within Amniota (Klembara et al., 2021). Following character reweighting and implied weighting, Klembara et al. (2021) recovered a single optimal tree, in which Diadectomorpha is nested within Amniota as the sister group of Synapsida (Figure 2). Klembara et al. (2021) listed 22 apomorphies in support of the sister group relationship of Diadectomorpha and Synapsida.
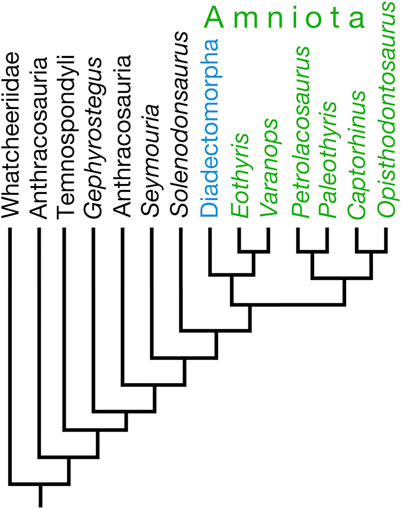
Figure 2. Tree illustrating Klembara et al.’s (2021) hypothesis placing Diadectomorpha within Amniota as the sister taxon of Synapsida (Eothyris and Varanops).
Recumbirostrans as amniotes
Recumbirostra is a clade name that was formulated by Anderson (2007) for a group of Permo-Carboniferous tetrapods that traditionally were regarded to be “microsaurs,” within the larger clade Lepospondyli. Up until the work of Carroll and Baird (1968); Carroll and Gaskill (1978), “microsaurs” had been, at times, confused with amniotes (Romer, 1950) or vice versa (Moodie, 1912), or touted to be amniotes (Gregory, 1948; Vaughn, 1962). The first large-scale phylogenetic analyses that included numerous “microsaur” and other lepospondyl terminal taxa found “microsaurs” to be a paraphyletic assemblage within either an amphibian (Laurin and Reisz, 1997, 1999; Vallin and Laurin, 2004) or a gymnophonian stem (Anderson, 2001). Following the conception of Recumbirostra by Anderson (2007), most studies on recumbirostran “microsaurs,” apart from Huttenlocker et al. (2013), focused on their anatomy (Maddin et al., 2011; Pardo et al., 2015; Szostakiwskyj et al., 2015) until Pardo et al. (2017) published a new phylogeny of Paleozoic tetrapods, which placed Recumbirostra within Amniota (Figure 3).
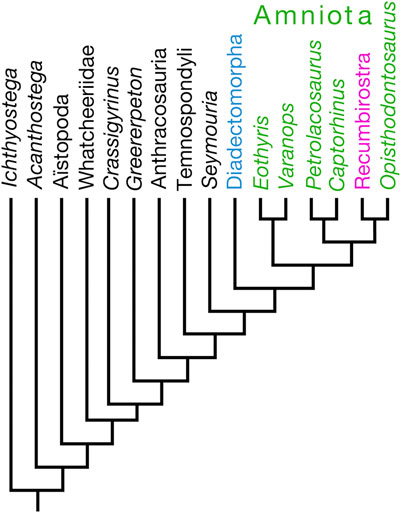
Figure 3. Tree illustrating Pardo et al.’s (2017) hypothesis placing Recumbirostra within Amniota as the sister taxon of the captorhinid reptile Opisthodontosaurus carrolli.
Ostensibly, the primary matter of Pardo et al.’s (2017) phylogenetic results was the more rootward placement of the tetrapod group Aïstopoda, traditionally interpreted to be lepospondyls, as the sister taxon of the clade that includes whatcheeriids, anthracosaurs, temnospondyls, and amniotes; the recovery of recumbirostrans within Amniota was noted as little more than an incidental remark by Pardo et al. (2017). Specifically and interestingly, Pardo et al. (2017) recovered recumbirostrans in a sister group relationship with the captorhinid eureptile Opisthodontosaurus carrolli. Those authors, however, neither discussed nor listed the synapomorphies that diagnosed the Recumbirostra-O. carrolli clade. The Pardo et al. (2017) data matrix was adopted subsequently by Mann and Maddin (2019) and Mann et al. (2019, 2022a), who described new species of recumbirostrans as amniotes. As a consequence of Pardo et al. (2017) using only two captorhinid species as terminal taxa, their results suggest that O. carrolli is not a captorhinid; hence, Mann et al. (2022b) described O. carrolli as a “putative captorhinid.” Marjanović and Laurin (2019) noted that, although Pardo et al. (2017) introduced dozens of new braincase characters, postcranial characters were underrepresented in the Pardo et al. (2017) study with respect to other large-scale analyses of early tetrapods. From this author’s perspective, as one who has published several anatomical and systematic studies on captorhinid reptiles (Dodick and Modesto, 1995; Modesto, 1996, 1998; Modesto and Smith, 2001; Modesto et al., 2007, 2014, 2016, 2018, 2019; Sidor et al., 2022), the use of only two captorhinid terminal taxa is not an adequate test of captorhinid monophyly. I am aware that Pardo et al. (2017) did not set out to examine the monophyly of Captorhinidae, but if their intention was to use fossil amniotes, for which there is a sufficient breadth of knowledge of the braincase, there are more informative captorhinid species than O. carrolli and Captorhinus laticeps for this purpose (e.g., Captorhinus aguti and Labidosaurus hamatus).
Discussion
Diadectomorphs as amniotes
If Klembara et al.’s (2021) hypothesis that diadectomorphs are crown amniotes is correct, it has two major implications for amniote evolutionary history. The most obvious of these is the taxonomic augmentation of the early Amniota at the expense of stem amniote diversity. What is more impactful, however, is the identification of diadectomorphs as bona fide amniotes would finally cement high-fiber herbivory as a uniquely amniote adaptation: as anamniotes, diadectid diadectomorphs have been an evolutionary puzzle because they have been regarded as the only non-amniote herbivores in all of tetrapod evolutionary history (Sues and Reisz, 1998; but see Das, 1996; Hill et al., 2015). From a research point of view, a broad acceptance of the idea that diadectomorphs are amniotes would inspire wider research on this poorly studied group of early tetrapods (versus the tacit understanding that diadectomorphs are an evolutionary “dead end”).
However, Klembara et al.’s (2021) hypothesis that diadectomorphs are amniotes by way of a sister group relationship with synapsids, while attractive, is weakened by faulty character argumentation and flawed character scoring. One of the most curious faults of many of their characters states is that they are formatted strictly as absent or present states that do not adequately cover the observed range of morphologies for each character, and I was left with the conclusion that the authors either tried to pigeonhole a character state for a particular taxon or were forced to code that taxon as “?”. For example, their characters 157 and 158 concerned the morphology of the ventral surface of the parasphenoid. The former character was constructed such that a parasphenoid with a single median depression on its ventral surface represents the derived state, and any parasphenoid in which this morphology was absent was the primitive state. The latter character was constructed such that a parasphenoid with paired lateral depressions on its ventral surface represents the derived state and any parasphenoid in which this morphology was absent exhibits the primitive state. From my understanding of parasphenoid morphology of early tetrapods, the derived states of these two characters could not both manifest in the same taxon, and they can be combined together to form a multiple-state character. In addition, the primitive morphology for neither character is actually described, and this seems to me to be a curious, if unintentional, means to circumvent the outgroup comparison. This confusing type of character construction may also have led to patent character duplication with regard to Klembara et al.’s (2021) characters 251 (L-shaped proximal tarsal element absent or present) and 274 (tibiale, intermedium, and proximal centrale not fused to form an astragalus or fused to form an astragalus).
Striking problems I found with Klembara et al.’s (2021) study are that their data matrix is, at least with regard to the amniote taxa, riddled with scoring errors, and a seeming lack of awareness of the evolving literature on early amniotes. For example, Klembara et al. (2021) used Paleothyris acadiana as a representative reptile, and I presume (because they do not provide their sources for their scoring choices), they based their scores solely from Carroll (1969) description. However, Modesto (2006) concluded that one of Carroll (1969) referred specimens, one that provided most of the information for Carroll’s reconstruction of the skull of this reptile in the occipital view, is incorrectly assigned to P. acadiana and probably belongs to another taxon. Removal of this questionable specimen from the hypodigm of P. acadiana affects the scoring of 22 characters (i.e., 7% of the total characters) for this reptile. I would recode an additional 17 characters for P. acadiana, resulting in a total of 13% of Klembara et al.’s (2021) 294 phylogenetic characters incorrectly coded for this taxon (see Supplementary Appendix SA1 in online Supplementary Material for my comments on Klembara et al., ’s 2021 scorings for P. acadiana). In another example, Klembara et al. (2021) seem to have relied on Romer and Price (1940) to code the synapsids Eothyris parkeyi and Varanops brevirostris: for the former synapsid, I was able to score 21 out of the 24 characters that I rescored using Reisz et al. (2009), and for the latter, I was able to score 24 out of 26 characters that I re-scored using Williston (1911) and Campione and Reisz (2010, 2011); see Supplementary Appendices SA4, SA5 in the online Supplementary Material. In addition, I would rescore 24 and 26 characters for the reptiles Captorhinus aguti and Labidosaurus hamatus, respectively; these figures represent 8%–9% of Klembara et al.’s (2021) 294 phylogenetic characters.
Perhaps the greatest weakness in Klembara et al.’s (2021) phylogenetic conclusions is that these authors neglected to include any “microsaurs,” which is surprising in light of numerous previous studies that recover “microsaurs” (with other lepospondyls) closer to Amniota than to either seymouriamorphs, gephyrostegids, or chroniosuchids (Carroll, 1995; Laurin and Reisz, 1997; Ahlberg and Clack, 1998; Anderson, 2001; Ruta et al., 2003; Huttenlocker et al., 2013). For instance, many, if not most, “microsaurs” exhibit elliptical and elongate nostrils (Klembara et al.’s (2021) character 1), the presence of an oblique orientation of the anterior surface of the premaxilla (Klembara et al.’s (2021) character 5), a lacrimal with a total length that is less than two and a quarter times its maximum pre-orbital length (their character 27), the presence of parietal lateral lappets (Klembara et al.’s (2021) character 40), and the absence of a single median depression on the parasphenoid (Klembara et al.’s (2021) character 157). The inclusion of “microsaurs” might also help resolve the mis-homologizing of certain anatomical features, such as the identity of the anterior and posterior Meckelian foramina in amniotes, because “microsaurs” have only the latter (Carroll and Gaskill, 1978).
To conclude this part of the Discussion, my examination of Klembara et al.’s (2021) scorings for the amniote terminal taxa that appear in their analysis reveals a startingly incomplete phylogenetic survey of early amniotes. The most puzzling weakness of Klembara et al.’s (2021) phylogenetic analysis is the total omission of lepospondyls, particularly “microsaurs,” which have been recovered as close relatives of amniotes in several major phylogenetic evaluations of the interrelationships of early amniotes and their relationships to other Paleozoic tetrapods (e.g., Laurin and Reisz, 1995, 1997, 1999; Anderson, 2001; Vallin and Laurin, 2004). Given these problems, it is premature to accept Klembara et al.’s (2021) hypothesis that diadectomorphs are amniotes; however, I hope that it fosters increased interest in this group of Permo-Carboniferous tetrapods because the more detailed knowledge we have about this group, which is the sister taxon of traditional Amniota, the more confident we will be in elucidating the origins of amniote synapomorphies and the evidence they hold for reconstructing macroevolutionary advances made by the earliest amniotes.
Recumbirostrans as amniotes
Pardo et al.’s (2017) hypothesis that a cohesive group of “microsaurs,” recently identified and named Recumbirostra by Anderson (2007), is nested within Amniota as reptiles is the first cladistic support for mid-20th century taxonomic proposals that “microsaurs” are amniotes and closely allied with captorhinids (as “captorhinomorphs;” Westoll, 1942; Gregory, 1948; Vaughn, 1962).
If Pardo et al. (2017) are correct in identifying recumbirostrans (including lysorophians) as amniotes, their hypothesis not only implies that Permo-Carboniferous amniote taxonomic diversity was greater than generally appreciated but also that Amniota of this time was more ecologically diverse than conventional reconstructions of early amniote adaptations (and that anamniotes were less so). Early amniote ways of life include insectivory (Modesto et al., 2009), carnivory (Brink and Reisz, 2014), high-fiber herbivory (Sues and Reisz, 1998), amphibious (Romer and Price, 1940; but see Laurin and de Buffrénil, 2016), aquatic (Modesto, 2010), and facultatively bipedal (Berman et al., 2000, 2021) adaptations. However, evidence of a fossorial way of life in unequivocal amniotes does not appear until the Late Permian (Smith, 1987). Most recumbirostrans are fossorial forms (Anderson, 2007; Maddin et al., 2011); if they are amniotes, as envisioned by Pardo et al. (2017), this implies that fossoriality evolved in amniotes as early as, and in concert with, the evolution of high-fiber herbivory in amniotes, at the end of the Carboniferous (approximately 300 million years ago).
Recumbirostra includes “microsaurs” such as ostodolepids, gymnarthrids, Pantylus cordatus, Rhynchonkos stovalli, and their close relatives (Anderson, 2007; Szostakiwskyj et al., 2015). Most recumbirostrans exhibit stoutly constructed skulls with upturned snouts that are analogous with those of recent head-digging fossorial amniotes (Maddin et al., 2011), and most recumbirostrans for which we have postcrania exhibit elongate bodies and limb reduction (similar to fossorial squamates). Among unequivocal fossil amniotes, the earliest possible evidence for head-digging appears in the Late Permian with cistecephalid therapsids (synapsids; Kammerer, 2021). Recumbirostrans, as reptiles, would accelerate the acquisition of head-digging fossorial ways of life in amniotes by roughly 40 million years.
There is, however, some doubt regarding the phylogenetic evidence for placing Recumbirostra within Amniota. As noted above, Marjanović and Laurin (2019) remarked that whereas Pardo et al. (2017) introduced dozens of new braincase characters of phylogenetic utility, their analysis is relatively poor in postcranial characters. Marjanović and Laurin (2019) also mused whether the placement of Recumbirostra within Amniota might be an artifact of taxon and character sampling, noting that the sample of “microsaurs” was not representative of their traditional diversity (i.e., microbrachids were not included) and that nectridean lepospondyls were absent altogether.
In the course of this review, I examined Pardo et al.’s (2017) scorings for the animals with which I am most familiar: captorhinid reptiles. Interestingly, Pardo et al. (2017) used only two captorhinid species (Captorhinus laticeps and Opisthodontosaurus carrolli) as terminal taxa in their work, ostensibly those of “well-preserved and completely described neurocranial anatomy” (Pardo et al., 2017, Methods). I am somewhat puzzled by this statement with regard to the inclusion of Opisthodontosaurus carrolli because actually, very little of the braincase of this captorhinid is available in the literature (Reisz et al., 2015). I am also concerned with Pardo et al.’s (2017) conclusion that the braincase of Captorhinus laticeps is well-preserved and completely described because the anatomy of this captorhinid has a checkered history of which those authors appear to be unaware.
With regard to O. carrolli, the parabasisphenoid is the only braincase element that was described and illustrated by Reisz et al. (2015), and it is exposed only in ventral view in those specimens in which it is preserved. Although technically not a braincase element, the stapes is known from a single specimen and only in the ventral view. The preservation of these two elements in a single view each belies Pardo et al.’s (2017) statement of a “well-preserved and completely described neurocranial anatomy” for O. carrolli, and as a result, Pardo et al. (2017) were able to code this captorhinid for only 3 of their 58 new braincase characters. All characters considered, Pardo et al. (2017) were able to code O. carrolli for 124 (33.5%) of their 370 phylogenetic characters. I would recode 64 of these characters (see Supplementary Appendix SA6 in the online Supplementary Material).
Pardo et al.’s (2017) use of Captorhinus laticeps as a terminal taxon, particularly one that purportedly has a well-preserved and well-documented braincase material, is problematic in as much these authors appear to be unaware of the background concerning Heaton’s (1979) work on the cranial anatomy of this captorhinid. Heaton’s (1979) work was carried out in a pre-cladistic context, in which he regarded C. laticeps (then “Eocaptorhinus” laticeps) as morphologically indistinguishable from C. aguti, apart from the presence of multiple rows of marginal teeth in the latter. As a result, he freely used the material of small captorhinids from the Richards Spur locality to supplement his description of C. laticeps. However, C. laticeps is not listed in faunal accounts for the locality (e.g., Evans et al., 2009), and the Supplementary Material probably (as remarked by Heaton, 1979) belongs to C. aguti. Accordingly, some of the cranial anatomy attributed to C. laticeps is the suspect: the prootic, the dorsal surface of the parabasisphenoid, the cavum cranii, the anterodorsal portion of the supraoccipital, the membranous labyrinth of the ear, and the ventral surface of the parietal are among the cranial regions of C. laticeps that were described and reconstructed by Heaton (1979) but should not be used to code this captorhinid for phylogenetic characters concerning such areas. I have noted scorings concerning these cranial regions in the online Supplementary Material. Looking through Pardo et al.’s (2017) line of scoring for C. laticeps, I would correct 64 (17%) of their 370 characters (see Supplementary Appendix SA7 in the online Supplementary Material).
Further investigating Pardo et al.’s (2017) scoring of C. laticeps, they appear to have relied entirely on Heaton (1979) for information on C. laticeps but were not critical of his usage of Richards Spur specimens to supplement his description. They also appear to have misinterpreted certain aspects of the cranial anatomy of C. laticeps, leading, in particular, to mis-homologizing the dorsal anatomy of the supraoccipital of this captorhinid. This area of the braincase is not fully accessible in material of C. laticeps that was available to Heaton (1979) because it comprised partial skeletons and articulated skulls. One skull exhibited a transverse break that afforded Heaton (1979) fortuitous access to the inner cranial morphology, such as the ventral surface of the frontals and some of the surrounding skull roof bones, and access to the dorsal surface to the palate and its interaction with the antorbital buttress. However, the dorsal region of the braincase and the cranial surface were not accessible, and as a result, the braincase reconstruction in figure 30 of Heaton (1979) was supplemented with information from Richards Spur specimens. The braincase of C. laticeps in the occipital view is largely based on that published by Price (1935); Heaton (1979) even reconstructed the posterior profile of the supraoccipital of C. laticeps as relatively low, although the opisthotic–supraoccipital specimen that Price (1935) used was formerly broken and then poorly repositioned when repaired. As a result, Price’s (1935) reconstruction of the supraoccipital presents a dorsoventrally shorter element than he would have had to work with if he had repositioned the opisthotic and the supraoccipital together prior to joining them (figure 7 in Modesto, 1998). A problem with Pardo et al.’s (2017) examination of Heaton’s (1979) C. laticeps braincase is that they (their characters 218 and 219; see online Supplementary Material) have misinterpreted the median and lateral ascending processes of the supraoccipital, extensive processes that rise directly from the dorsal surface of the supraoccipital: the median process extends upward as a somewhat V-shaped extension (see figure 8 in Modesto, 1998) that makes contact with the posteroventral surface of the skull table; the lateral ascending processes extend dorsolaterally from the dorsolateral corners of the supraoccipital plate to contact the posterior margin of the skull roof (figure 8 in Modesto, 1998). Richards Spur specimens show this morphology much more completely than the material available to Heaton (1979). However, Szostakiwskyj et al. (2015) equated the median ascending process of the supraoccipital in C. laticeps with a feature that they identified as median ascending processes in Rhynchonkos stovalli, Aletrimyti gaskillae, and Dvellecanus carrolli. However, the structure in these recumbirostrans and that reconstructed for C. laticeps look nothing alike; the median ascending process of these microsaurs appears to be a low peak of the bone, judging from figures 6a, 9a, and 15f, g in Szostakiwskyj et al. (2015), rather than the conspicuous process seen in captorhinids (Heaton, 1979: fig. 27; Modesto, 1998: fig. 8). Furthermore, Szostakiwskyj et al. (2015) mistakenly identified a median ascending process on the supraoccipital of the diapsid reptile Petrolacosaurus kansensis, but this is merely the medial portion of the dorsal margin of the bone (figure 7b in Reisz, 1981) that looks nothing like the dorsomedial process in captorhinids (figure 8 in Modesto, 1998). Notably, Szostakiwskyj et al. (2015) identified a region on the supraoccipital that extends anteriorly and horizontally as the lateral ascending process and homologized it with the lateral ascending process in C. laticeps, which extends dorsolaterally from the main body of the supraoccipital in this captorhinid to underlie the ventral portion of the postparietal and form the dorsomedial border of the post-temporal fenestra. The “lateral ascending process” appears to form the posterolateral wall of the braincase in these recumbirostrans (figure 12 in Szostakiwskyj et al., 2015) and thus differs greatly in shape and in its contacts with neighboring elements. Given the structural differences in these two features in recumbirostrans and C. laticeps, I believe that the “lateral ascending process” in the latter taxon should not have been homologized with that attributed to recumbirostrans by Szostakiwskyj et al. (2015).
To conclude this part of the discussion, I infer that Pardo et al.’s (2017) phylogenetic recovery of recumbirostrans within Amniota is partly attributable to narrow taxon selection among both “microsaurs” and amniotes, particularly captorhinids, misinterpretation of captorhinid anatomy, and haphazard scoring of the captorhinid terminal taxa. It is striking that Pardo et al. (2017, page 5) stated that their taxon selection prioritized tetrapods with “well-preserved and completely described neurocranial anatomy,” but one of the captorhinid species they included in their analysis (Opisthodontosaurus carrolli) is one of the most poorly known early reptiles in this respect. As discussed above, I believe that Pardo et al. (2017) also appear to have mis-homologized the median and lateral ascending processes of captorhinids with quite different structures on the supraoccipitals of the recumbirostrans with which they were familiar. A more rigorous examination of the recumbirostrans-as-amniotes hypothesis will require the inclusion of additional captorhinid terminal taxa, particularly species such as Captorhinus aguti and Labidosaurus hamatus, for which we have a better preserved and documented braincase material than the poorly known O. carrolli and the problematic C. laticeps, as well as a selection of non-recumbirostran “microsaurs,” notably Microbrachis and Tuditanus, for a fair assessment of the amniote affinities of the Recumbirostra.
Conclusion
Two separate research groups have been following different paths while investigating the origin and relationships of a group of tetrapods known as amniotes. One group has pursued the possibility that a group of tetrapods that most workers have recovered as close relatives of traditional amniotes have recently presented phylogenetic evidence that the group, called Diadectomorpha, can at last be placed with the amniotes. The other group has reported the results of their research that proposes that relatively small, mostly fossorial forms known as recumbirostrans may be ancient and previously unappreciated members of Amniota. Their inclusion as Amniota suggests that the diversification of early reptiles included an early adoption of a fossorial lifestyle and diversification of several lines of fossorial reptiles. Following my review of the character scorings of amniote terminal taxa from both published data matrices, I infer that the researchers were unfamiliar with the literature on early amniotes, resulting in the use of obsolete anatomical information for certain amniote terminal taxa. In one case, mis-homologization of particular braincase characters may have misled one group of researchers to recover one stem amniote group, called recumbirostrans, as amniotes. For the other research group, the exclusion of an entire clade of stem amniotes (Lepospondyli, which includes Recumbirostra), may have facilitated the recovery of a synapsid–diadectomorph sister group relationship, but there remains no reason any longer to omit “microsaurs” and other lepospondyls, given the growing database of digital scans that are being increasingly published on these putative amniotes.
Author contributions
The author confirms being the sole contributor of this work and has approved it for publication.
Funding
This work was supported by a New Opportunities Fund award from the Canadian Foundation for Innovation and a grant from the Nova Scotia Research and Innovation Trust.
Acknowledgments
The author thanks reviewers for their comments and suggestions.
Conflict of interest
The author declares that the research was conducted in the absence of any commercial or financial relationships that could be construed as a potential conflict of interest.
Publisher’s note
All claims expressed in this article are solely those of the authors and do not necessarily represent those of their affiliated organizations, or those of the publisher, the editors, and the reviewers. Any product that may be evaluated in this article, or claim that may be made by its manufacturer, is not guaranteed or endorsed by the publisher.
Supplementary material
The Supplementary Material for this article can be found online at: https://www.frontiersin.org/articles/10.3389/feart.2024.1155806/full#supplementary-material
References
Ahlberg, P. E., and Clack, J. A. (1998). Lower jaws, lower tetrapods – a review based on the Devonian genus Acanthostega. Trans. R. Soc. Edinb. Earth Sci. 89, 11–46. doi:10.1017/s0263593300002340
Anderson, J. S. (2001). The phylogenetic trunk: maximal inclusion of taxa with missing data in an analysis of the Lepospondyli (Vertebrata, Tetrapoda). Syst. Biol. 50, 170–193. doi:10.1080/10635150151125833
Anderson, J. S. (2007). “Incorporating ontogeny into the matrix: a phylogenetic evaluation of developmental evidence for the origin of modern amphibians,” in Major transitions in vertebrate evolution. Editors J. S. Anderson, and H.-D. Sues (Indiana: Indiana University Press), 182–227.
Benoit, J., Ford, D. P., Miyamae, J. A., and Ruf, I. (2021). Can maxillary canal morphology inform varanopid phylogenetic affinities? Acta Palaeontol. Pol. 66, 389–393. doi:10.4202/app.00816.2020
Berman, D. S. (2000). Origin and early evolution of the amniote occiput. J. Paleontol. 74, 938–956. doi:10.1017/s0022336000033114
Berman, D. S. (2013). “Diadectomorphs, amniotes or not?,” in The Carboniferous–Permian transition. Editors S. G. Lucas, W. J. Nelson, W. A. DiMichele, J. A. Spielmann, K. Krainer, J. E. Barricket al. (Albuquerque: New Mexico Museum of Natural History & Science), 22–35.
Berman, D. S., Reisz, R. R., Scott, D., Henrici, A. C., Sumida, S. S., and Martens, T. (2000). Early Permian bipedal reptile. Science 290, 969–972. doi:10.1126/science.290.5493.969
Berman, D. S., Sumida, S. S., Henrici, A. C., Scott, D., Reisz, R. R., and Martens, T. (2021). The early Permian bolosaurid Eudibamus cursoris: earliest reptile to combine parasagittal stride and digitigrade posture during quadrupedal and bipedal locomotion. Front. Ecol. Evol. 9. doi:10.3389/fevo.2021.674173
Brink, K., and Reisz, R. R. (2014). Hidden dental diversity in the oldest terrestrial apex predator Dimetrodon. Nat. Comm. 5, 3269. doi:10.1038/ncomms4269
Campione, N. E., and Reisz, R. R. (2010). Varanops brevirostris (Eupelycosauria: varanopidae) from the Lower Permian of Texas, with discussion of varanopid morphology and interrelationships. J. Vertebr. Paleontol. 30, 724–746. doi:10.1080/02724631003762914
Campione, N. E., and Reisz, R. R. (2011). Morphology and evolutionary significance of the atlas-axis complex in varanopid synapsids. Acta Palaeontol. Pol. 56, 739–748. doi:10.4202/app.2010.0071
Carroll, R. L. (1995). Problems of the phylogenetic analysis of Paleozoic choanates. Bull. Mus. Nat. D’hist. Nat. Paris 4ème sér 17, 389–445.
Carroll, R. L. (1969). A Middle Pennsylvanian captorhinomorph, and the interrelationships of primitive reptiles. J. Paleontol. 43, 151–170.
Carroll, R. L., and Baird, D. (1968). The Carboniferous amphibian Tuditanus [Eosauravus] and the distinction between microsaurs and reptiles. Am. Mus. Novit. 2337, 1–50.
Carroll, R. L., and Gaskill, P. (1978). The order Microsauria. Memoirs Am. Philosophical Soc. 126, 1–211.
Cope, E. D. (1878). Descriptions of extinct Batrachia and Reptilia from the Permian formation of Texas. Proc. Amer. Phil. Soc. 17, 505–530.
Das, I. (1996). Folivory and seasonal changes in diet in Rana hexadactyla (Anura: ranidae). J. Zool. 238, 785–794. doi:10.1111/j.1469-7998.1996.tb05430.x
Dodick, J. T., and Modesto, S. P. (1995). The cranial anatomy of the captorhinid reptile Labidosaurikos meachami from the Lower Permian of Oklahoma. Palaeontology 38, 687–711.
Evans, D. C., Maddin, H. C., and Reisz, R. R. (2009). A re-evaluation of sphenacodontid synapsid material from the Lower Permian fissure fills near Richards Spur, Oklahoma. Palaeontology 52, 219–227. doi:10.1111/j.1475-4983.2008.00837.x
Ford, D. P., and Benson, R. B. J. (2019). The phylogeny of early amniotes and the affinities of Parareptilia and Varanopidae. Nat. Ecol. Evol. 4, 57–65. doi:10.1038/s41559-019-1047-3
Gauthier, J., Kluge, A. G., and Rowe, T. (1988). “The early evolution of the Amniota,” in The phylogeny and classification of the tetrapods. Editor M. J. Benton (Oxford: Clarendon Press), 103–155.
Gregory, J. T. (1948). The structure of Cephalerpeton and affinities of the Microsauria. Am. J. Sci. 246, 550–568. doi:10.2475/ajs.246.9.550
Heaton, M. (1979). Cranial anatomy of primitive captorhinid reptiles from the Late Pennsylvanian and Early Permian Oklahoma and Texas. Okla. Geol. Surv. 127, 1–84.
Hill, R. L., Mendelson, J. R., and Stabile, J. L. (2015). Direct observation and review of herbivory in Sirenidae (Amphibia: Caudata). South. Nat. 14, N5–N9. doi:10.1656/058.014.0104
Huttenlocker, A. K., Pardo, J. D., Small, B. J., and Anderson, J. S. (2013). Cranial morphology of recumbirostrans (Lepospondyli) from the Permian of Kansas and Nebraska, and early morphological evolution inferred by micro-computed tomography. J. Vertebr. Paleontol. 33, 540–552. doi:10.1080/02724634.2013.728998
Kammerer, C. F. (2021). Elevated cranial sutural complexity in burrowing dicynodonts. Front. Ecol. Evol. 9, 674151. doi:10.3389/fevo.2021.674151
Klembara, J., Hain, M., Ruta, M., Berman, D. S., Pierce, S. E., and Henrici, A. C. (2019). Inner ear morphology of diadectomorphs and seymouriamorphs (Tetrapoda) uncovered by high-resolution x-ray microcomputed tomography, and the origin of the amniote crown group. Palaeontology 63, 131–154. doi:10.1111/pala.12448
Klembara, J., Ruta, M., Hain, M., and Berman, D. S. (2021). Braincase and inner ear anatomy of the Late Carboniferous tetrapod Limnoscelis dynatis (Diadectomorpha) revealed by high-resolution X-ray microcomputed tomography. Front. Ecol. Evol. 9, 709766. doi:10.3389/fevo.2021.709766
Laurin, M., and de Buffrénil, V. (2016). Microstructural features of the femur in early ophiacodontids: a reappraisal of ancestral habitat use and lifestyle of amniotes. Comptes Rendus Palevol 15, 115–127. doi:10.1016/j.crpv.2015.01.001
Laurin, M., and Reisz, R. R. (1995). A reevaluation of early amniote phylogeny. Zool. J. Linn. Soc. 113, 165–223. doi:10.1006/zjls.1995.0007
Laurin, M., and Reisz, R. R. (1997). “A new perspective on tetrapod phylogeny,” in Amniote origins: completing the transition to land. Editors S. S. Sumida, and K. L. M. Martin (San Diego: Academic Press), 9–59.
Laurin, M., and Reisz, R. R. (1999). A new study of Solenodonsaurus janenschi, and a reconsideration of amniote origins and stegocephalian evolution. Can. J. Earth Sci. 36, 1239–1255. doi:10.1139/e99-036
Maddin, H. C., Olori, J. C., and Anderson, J. S. (2011). A redescription of Carrolla craddocki (Lepospondyli: Brachystelechidae) based on high-resolution CT, and the impacts of miniaturization and fossoriality on morphology. J. Morph. 272, 722–743. doi:10.1002/jmor.10946
Mann, A., and Maddin, H. C. (2019). Diabloroter bolti, a short-bodied recumbirostran ‘microsaur’ from the Francis Creek Shale, Mazon Creek, Illinois. Zool. J. Linn. Soc. 187, 494–505. doi:10.1093/zoolinnean/zlz025
Mann, A., Pardo, J. D., and Maddin, H. C. (2019). Infernovenator steenae, a new serpentine recumbirostran from the ‘Mazon Creek’ Lagerstätte further clarifies lysorophian origins. Zool. J. Linn. Soc. 187, 506–517. doi:10.1093/zoolinnean/zlz026
Mann, A., Pardo, J. D., and Maddin, H. C. (2022a). Snake-like limb loss in a Carboniferous amniote. Nat. Ecol. Evol. 6, 614–621. doi:10.1038/s41559-022-01698-y
Mann, A., Pardo, J. D., and Sues, H.-D. (2022b). Osteology and phylogenetic position of the diminutive ‘microsaur’ Odonterpeton triangulare from the Pennsylvanian of Linton, Ohio, and major features of recumbirostran phylogeny. Zool. J. Linn. Soc. 197, 641–655. doi:10.1093/zoolinnean/zlac043
Marjanović, D., and Laurin, M. (2019). Phylogeny of Paleozoic limbed vertebrates reassessed through revision and expansion of the largest published relevant data matrix. PeerJ 6, e5565. doi:10.7717/peerj.5565
Modesto, S. P. (1996). A basal captorhinid reptile from the Fort Sill fissures, Lower Permian of Oklahoma. Okla. Geol. Notes 56, 4–14.
Modesto, S. P. (1998). New information on the skull of the Early Permian reptile Captorhinus aguti. PaleoBios 18, 21–35.
Modesto, S. P. (2010). The postcranial skeleton of the aquatic parareptile Mesosaurus tenuidens from the Gondwanan Permian. J. Vertebr. Paleontol. 30, 1378–1395. doi:10.1080/02724634.2010.501443
Modesto, S. P. (2006). The cranial skeleton of the Early Permian aquatic reptile Mesosaurus tenuidens: implications for relationships and palaeobiology. Zool. J. Linnean Soc. 146, 345–368.
Modesto, S. P., Flear, V. J., Dilney, M. M., and Reisz, R. R. (2016). A large moradisaurine tooth plate from the Lower Permian of Texas and its biostratigraphic implications. J. Vertebr. Paleontol. 36, e1221832. doi:10.1080/02724634.2016.1221832
Modesto, S. P., Lamb, A. J., and Reisz, R. R. (2014). The captorhinid reptile Captorhinikos valensis from the Lower Permian Vale Formation of Texas, and the evolution of herbivory in eureptiles. J. Vertebr. Paleontol. 34, 291–302. doi:10.1080/02724634.2013.809358
Modesto, S. P., Richards, C. D., Ide, O., and Sidor, C. A. (2019). The vertebrate fauna of the upper Permian of Niger—X. The mandible of the captorhinid reptile Moradisaurus grandis. J. Vertebr. Paleontol. 38, e1531877. doi:10.1080/02724634.2018.1531877
Modesto, S. P., Scott, D., and Reisz, R. R. (2018). A new small captorhinid reptile from the lower Permian of Oklahoma and resource partitioning among small captorhinids in the Richards Spur fauna. Pap. Palaeontol. 4, 293–307. doi:10.1002/spp2.1109
Modesto, S. P., Scott, D. M., Berman, D. S., Müller, J., and Reisz, R. R. (2007). The skull and the palaeoecological significance of Labidosaurus hamatus, a captorhinid reptile from the Lower Permian of Texas. Zool. J. Linn. Soc. 149, 237–262. doi:10.1111/j.1096-3642.2007.00242.x
Modesto, S. P., Scott, D. M., and Reisz, R. R. (2009). Arthropod remains in the oral cavities of fossil reptiles support inference of early insectivory. Biol. Lett. 5, 838–840. doi:10.1098/rsbl.2009.0326
Modesto, S. P., and Smith, R. M. H. (2001). A new Late Permian captorhinid reptile: a first record from the South African Karoo. J. Vertebr. Paleontol. 21, 405–409. doi:10.1671/0272-4634(2001)021[0405:anlpcr]2.0.co;2
Moodie, R. L. (1912). The Pennsylvanic Amphibia of the Mazon Creek, Illinois, shales. Kans. Univ. Sci. Bull. 6, 323–359.
Pardo, J., Szostakiwskyj, M., and Anderson, J. S. (2015). Cranial morphology of the brachystelechid ‘microsaur’ Quasicaecilia texana Carroll provides new insights into the diversity and evolution of braincase morphology in recumbirostran ‘microsaurs’. PLOS ONE 10 (6), e0130359. doi:10.1371/journal.pone.0130359
Pardo, J. D., Szostakiwskyj, M., Ahlberg, P. E., and Anderson, J. S. (2017). Hidden morphological diversity among early tetrapods. Nature 546, 642–645. doi:10.1038/nature22966
Parrington, F. R. (1959). The problem of the classification of reptiles. Zool. J. Linn. Soc. 19, 99–115. doi:10.1111/j.1096-3642.1958.tb01712.x
Reisz, R. R. (1981). A diapsid reptile from the Pennsylvanian of Kansas. Spec. Publ. Mus. Nat. Hist. Univ. Kans. 7, 1–74.
Reisz, R. R., Godfrey, S. J., and Scott, D. (2009). Eothyris and Oedaleops: do these Early Permian synapsids from Texas and New Mexico form a clade? J. Vertebr. Paleontol. 29, 39–47. doi:10.1671/039.029.0112
Reisz, R. R., LeBlanc, A. R. H., Sidor, C. A., Scott, D., and May, W. (2015). A new captorhinid from the Lower Permian of Oklahoma showing remarkable dental and mandibular convergence with microsaurian tetrapods. Sci. Nat. 102, 50. doi:10.1007/s00114-015-1299-y
Romer, A. S. (1950). The nature and relationships of the Paleozoic microsaurs. Am. J. Sci. 248, 628–654. doi:10.2475/ajs.248.9.628
Ruta, M., and Coates, M. I. (2007). Dates, nodes and character conflict: addressing the lissamphibian origin problem. J. Syst. Palaeontol. 5, 69–122. doi:10.1017/s1477201906002008
Ruta, M., Coates, M. I., and Quicke, D. L. J. (2003). Early tetrapod relationships revisited. Biol. Rev. Camb. Phil. Soc. 78, 251–345. doi:10.1017/s1464793102006103
Sidor, C. A., Ide, O., Larsson, H. C. E., O’Keefe, F. R., Smith, R. M. H., Steyer, J.-S., et al. (2022). The vertebrate fauna of the upper Permian of Niger—XI. Cranial material of a juvenile Moradisaurus grandis (Reptilia: Captorhinidae). J. Vertebr. Paleontol. 41, e2030345. doi:10.1080/02724634.2021.2030345
Smith, R. M. H. (1987). Helical burrow casts of therapsid origin from the Beaufort Group (Permian) of South Africa. Palaeogeog. Palaeoclimatol. Palaeoecol. 60, 155–169. doi:10.1016/0031-0182(87)90030-7
Sues, H.-D., and Reisz, R. R. (1998). Origins and early evolution of herbivory in tetrapods. Trends Ecol. Evol. 13, 141–145. doi:10.1016/s0169-5347(97)01257-3
Szostakiwskyj, M., Pardo, J., and Anderson, J. S. (2015). Micro-CT study of Rhynchonkos stovalli (Lepospondyli, Recumbirostra), with description of two new genera. PLOS ONE 10 (6), e0127307. doi:10.1371/journal.pone.0127307
Vallin, G., and Laurin, M. (2004). Cranial morphology and affinities of Microbrachis, and a reappraisal of the phylogeny and lifestyle of the first amphibians. J. Vertebr. Paleontol. 24, 56–72. doi:10.1671/5.1
Vaughn, P. P. (1962). The Paleozoic microsaurs as close relatives of reptiles, again. Amer. Midl. Nat. 67, 79–84. doi:10.2307/2422819
Watson, D. M. S. (1957). On Millerosaurus and the early history of the sauropsid reptiles. Phil. Trans. R. Soc. Lond. Ser. B 240, 325–400.
Westoll, T. S. (1942). Ancestry of captorhinomorph reptiles. Nature 149, 667–668. doi:10.1038/149667a0
Keywords: Amniota, Diadectomorpha, evolution, phylogeny, Recumbirostra
Citation: Modesto SP (2024) Problems of the interrelationships of crown and stem amniotes. Front. Earth Sci. 12:1155806. doi: 10.3389/feart.2024.1155806
Received: 31 January 2023; Accepted: 12 March 2024;
Published: 02 April 2024.
Edited by:
Xing Xu, Yunnan University, ChinaReviewed by:
Michel Laurin, UMR7207 Centre de recherche sur la paléobiodiversité et les paléoenvironnements (CR2P), FranceBruce S. Lieberman, University of Kansas, United States
Copyright © 2024 Modesto. This is an open-access article distributed under the terms of the Creative Commons Attribution License (CC BY). The use, distribution or reproduction in other forums is permitted, provided the original author(s) and the copyright owner(s) are credited and that the original publication in this journal is cited, in accordance with accepted academic practice. No use, distribution or reproduction is permitted which does not comply with these terms.
*Correspondence: Sean P. Modesto, c2Vhbm1vZGVzdG9AeWFob28uY2E=