- 1State Key Laboratory of Lake Science and Environment Research, Nanjing Institute of Geography and Limnology, Chinese Academy of Sciences, Nanjing, China
- 2University of Chinese Academy of Sciences, Beijing, China
- 3Department of Chemistry, College of Science, King Saud University, Riyadh, Saudi Arabia
The Amu Darya River, as one of the important flows of the Aral Sea located in the semi-arid area of Central Asia, also performed as the primary water supply for Uzbekistan. Therefore, the residues and risks of anthropogenic-related persistent organic pollutants (POPs) in waters of the Amu Darya River were conducted in the present study to elucidate their possible effects on the water safety in such a specific area as well as on the Aral Sea Crisis from a new perspective. Thirty-nine water samples distributed along the Amu Darya River to the coastal of the Aral Sea were analyzed for both polycyclic aromatic hydrocarbons (PAHs) and organochlorine pesticides (OCPs) occurrence, showing the total concentrations of ΣOCPs and ΣPAHs in the range of 1.16–22.75 ng/L and 3.18–506.26 ng/L, respectively. Spatial differences showed higher levels for both OCPs and PAHs along the lower reaches of the Amu Darya River due to intense human activities. Source identification performed by isomer ratios indicated that dichlorodiphenyltrichloroethanes (DDTs) probably originated from recent use, while hexachlorocyclohexanes (HCHs), chlordanes, and endosulfans originated mainly from historical usage. Furthermore, the principal component analysis showed PAHs were from coal and petroleum combustion (65.2%), biomass combustion (27.2%), and industrial chemical combustion (7.64%). The human health risk assessment demonstrated no carcinogenic or non-carcinogenic risks at present. However, moderate to high ecological risks to aquatic organisms especially were observed along the lower reaches, especially the delta area. The results obtained would not only provide important basic data for such a semi-arid area but also show us the possible toxic effects induced by such pollutants, which should attract more attention in the shrinking case of the Aral Sea.
1 Introduction
Organochlorine pesticides (OCPs) and polycyclic aromatic hydrocarbons (PAHs) are recognized as a group of persistent organic pollutants (POPs) that have received extensive attention in the world since the 1980s (Kwach and Lalah, 2009; Bourgeault and Gourlay-france, 2013; Mojiri et al., 2019). The unique properties of these substances, such as their toxicity, persistence, and long-range transport in the environment, pose potential risks to human health and the ecological environment, making them significant global environmental concerns (Nagy et al., 2013; Aziz et al., 2014). The arid inland region of Central Asia is susceptible to climate change and human impacts. Water quality degradation has become increasingly severe in recent decades due to increased industrial, agricultural, and domestic water consumption. (Tao et al., 2019; Yang et al., 2020). The contradiction between the supply and demand of water resources has become more prominent, while the irrational utilization of water resources poses a significant risk to Central Asia. The Aral Sea, Once the fourth largest lake in the world, has been rapidly shrinking from 67,000 km2 in 1960 to 9,374 km2 in 2017 (Tornqvist et al., 2011; Unger-Shayesteh et al., 2013). The decline in the water level of the Aral Sea and the substantial evaporation of its waters have consequently led to severe regional ecological issues, including agricultural land salinization within the watershed, degradation of the local climate, and heightened pollution levels in both water and the atmosphere, becoming one of the most significant ecological disasters in modern times (Nezlin et al., 2005). One of the two primary water sources for the Aral Sea, the Amu Darya, is recognized as the largest inland river in Central Asia. It serves as the principal water supply for Uzbekistan, catering to local industries, agriculture, and domestic consumption. During the Soviet era, extensive industrial and agricultural development in the Aral Sea basin significantly diminished water resources and strengthened this region’s substantial decline in water quality (Jin et al., 2017; Karthe et al., 2017). Pollutants have been spreading heavily, especially in the Amu Darya delta area, where large-scale agricultural activities occur. In the same period, to maintain a stable and high yield, the use of pesticides and fertilizers has increased yearly, leading to the deterioration of the ecological environment and seriously threatening the health of its inhabitants in this area (Matlyuba et al., 2019). Studies have found elevated levels of arsenic and OCPs in individuals' blood, hair, and urine near the Aral Sea Basin. Additionally, surface and groundwater in the region contain copper, lead, chromium, and uranium levels that exceed World Health Organization limits (WHO) (Schlüter et al., 2005; Crosa et al., 2006; Herbst et al., 2008). Although OCPs have been banned for over 3 decades, residual concentrations of OCPs detected in local topsoil and soil profiles may still threaten the environment and public health (Artikov et al., 2018). However, limited studies on POPs in the downstream environment of the Amu Darya River—the most crucial water resource in Uzbekistan and the Aral Sea basin—have yet to be reported in the delta region, the region of most significant concern.
In the present study, we conducted a preliminary study of surface water in the Amu Darya River basin in Uzbekistan to 1) analyze the concentration levels and distribution characteristics of POPs in surface water, 2) discuss potential sources of PAHs and OCPs in waters, and 3) assess the suitability of surface water for different applications and the potential threat to human health. The findings from this research provide a scientific foundation for the comprehensive evaluation of water resources and human ecosystems in the Aral Sea region.
2 Materials and methods
2.1 Study area and sampling
The Amu Darya River, which rises 2,550 km from the Pamir Plateau, is one of the primary water resources in Uzbekistan. The river can be divided into three sections: Upstream, along the Afghanistan-Tajikistan border, is the source of most of the water flow; The middle reaches first border Uzbekistan and Afghanistan, then into Turkmenistan; The downstream flows entirely through Uzbekistan, and finally merges into the Aral Sea in Uzbekistan, while forming a large-scale wetland delta at the mouth of the river (Schlüter et al., 2013; Burr et al., 2019). The river is mainly supplied by the melting water of snow and ice in the mountains and the winter and spring rainfall in the upper mountains, and it reaches its highest during the summer, from June to August. The Aral Sea, a Salt Lake straddling the border between Kazakhstan and Uzbekistan, has seen its surface area decrease since 1960 due to reduced runoff and split into two separate bodies of water in 1986, the southern Aral Sea supplied by the Amu Darya River and the northern Syr River. In 2003, the South Aral Sea was further split east and west (Groll et al., 2013). The Aral Sea region is classified as a “temperate continental climate” zone, with potential evaporation 10–15 times that of precipitation due to hot and dry summers (Shibuo et al., 2006; Tornqvist et al., 2011).
The study area covers the territory of Uzbekistan, bordering the Aral Sea, including Khorezm Oblast and Karakalpak Republic. The former is the central rice-producing region of Uzbekistan. It is the highest cotton production region in the country. At the same time, the latter has cotton cultivation, animal husbandry, and fishing along the coast of the Aral Sea, as well as power stations, natural gas fields, and agricultural processing industries. The capital, Nukus, is the economic and administrative center of the Republic of Karakalpak, and the surrounding cities are densely populated and have excellent transportation conditions.
The water samples were collected in August 2019, including river water, the Aral Sea, drainage water, well water, and ponds, for 39 samples to assess OCPs and PAHs concentrations (Figure 1). Sampling was performed using a global positioning system. Water samples were collected using a surface sampler at a controlled depth of about 0.50 m per sample point, with a volume greater than 1 L collected in clean brown glass bottles. The samples were filtered through a 0.45 μm Millipore nitrocellulose filter (Merck-Millipore) and transported under refrigeration (4°C) to the laboratory, where they were stored at −20°C and analyzed within 1 week after collection.
2.2 Sample analysis
2.2.1 Chemicals and reagents
20 OCPs including four HCH isomers (α-, β, γ-, and δ-HCH), four DDT isomers (pˏpʹ -DDE, oˏpʹ -DDT, pˏpʹ -DDD, pˏpʹ -DDT), chlordanes (CC: cis-chlordane and TC: trans-chlordane), endosulfans (α-endosulfan, β-endosulfan and endosulfan sulfate), heptachlor, heptachlor epoxide, aldrin, endrins (endrin, endrin aldehyde and endrin ketone) and methoxychlor. 16 PAHs, listed by the USEPA (United States Environmental Protection Agency) as priority PAHs, including naphthalene (Nap), acenaphthene (Acy), acenaphthene (Ace), Fluorene (Flu), phenanthrene (Phe), anthracene (Ant), fluorothracene (Fla), Pyrene (Pyr), Benzoanthracene (BaA), chrysene (Chr), benzo [b] fluoranthene (BbF), benzo [k] fluoranthene (BkF), benz [a] pyrene (BaP), dibenzo [a,h] anthracene (DahA), indeno [1, 2, 3-cd] pyrene (IcdP) and benzo [g, h, i] perylene (BghiP). All the solvents are high-performance liquid chromatography grade, and the standards are purchased from Dr. Ehrenstorfer.
2.2.2 Sample treatment
The extraction of OCPs and PAHs in water was performed using the same method of solid-phase extraction (SPE). 1 L filtered water sample was enriched by C18 solid-phase extraction cartridges (Supelco, 500 mg/6 mL) at a flow rate of 5 mL/min. The cartridges were immersed and rinsed with 5 mL methanol and 5 mL ultrapure water for preconditioning. After extraction, 20 mL dichloromethane and n-hexane (V: V=7:3) were used for elution, and Na2SO4 (Sodium sulfate) was added to remove residual water. Each eluate was concentrated to 1 mL under a gentle, purified nitrogen stream for analysis.
2.2.3 Instrumental analysis
High-performance liquid chromatography (HPLC, Agilent 1200) with diode array detector (DAD) and fluorescence detector (FLD) was used to analyze PAHs. Gas Chromatograph-Mass Spectrometer (GC-MS, Agilent 7890-5975C) and gas chromatograph with an electron capture detector (GC-ECD, Agilent 7890A) were used for the qualification and quantification of OCPs, respectively. Previous studies described PAHs and OCPs analysis methods well (Shen et al., 2021). Specific parameters for OCPs and PAHs analysis were shown in Supplementary Material S1.
2.3 Risk assessment
To obtain a comprehensive understanding of the possible ecological risks of POPs in the Aral Sea basin, the aquatic biota was assessed by calculating risk quotients (RQ), and carcinogenic and non-carcinogenic risks were used to assess the potential hazards to human health from exposure to surface water. Generally, the non-carcinogenic risk is generally measured by the hazard index (HI) (Yadav et al., 2019).
The calculation of RQ involves relating the concentration of pollutants in water to their corresponding quality values. These quality values include negligible concentrations (NCs) and maximum permissible concentrations (MPCs) of PAHs (Wu et al., 2014; Bai et al., 2018), continuous criteria concentrations (CCCs), and maximum criteria concentrations (CMCs) of OCPs.
Regarding human health, dermal contact and water ingestion were the primary modes of exposure being considered. Preliminary calculations indicated that the risk values due to the dermal exposure route were much smaller than those due to ingestion, so this study assessed the health risks for adults and children primarily based on the water ingestion route (Zhang et al., 2011; Ai et al., 2021). The calculations and details of variables were presented in Supplementary Tables S2, S3.
2.4 Quality assurance and quality control
A single standard determined the retention time of PAHs and OCPs, and the quantitative detection in samples was carried out by an external standard curve method with multi-point correction. The HPLC-FLD had no response to Acy but was sensitive to the other 15 PAHs. Therefore, the DAD was used to detect the concentration of Acy and correspond with the FLD in sequence to avoid mistakes in the sample testing.
Quality control and quality assurance in the sample analysis were implemented according to laboratory blank, a spiked standard recovery, and replicate samples. The spiked standard recovery was a complete pre-treatment operation by adding PAHs / OCPs standard with fixed concentration into blank samples. Quantitative analysis of the concentration of standard samples in blank samples could indicate the entire pre-treatment operation process and determine the applicability and stability of the method. In addition, in the sample analysis process, the finished samples were randomly selected, and the samples with doubtful test results were retested by adding standard substances to ensure the accuracy of the qualitative analysis. No target compounds were detected in the solvent blanks, and the relative standard deviations (RSDs) were within 20% for the parallel samples. The spiked recoveries of PAHs / OCPs were in the range of 75.0%–120%, with the limit of detection (LOD, S/N=3) of OCPs and PAHs in the water were 0.01–0.06 ng L-1 and 0.05–0.21 ng L-1, respectively. The ranges of the linearity and linearity correlation coefficients (R2) of the calibration curves and the detailed method performance parameters are listed in Supplementary Table S1.
2.5 Statistical analysis
The contribution rate of PAHs to the source in surface water was identified by principal component analysis (PCA), which was performed using SPSS 25.0. ArcGIS 10.2 software was used to display the spatial distribution of POPs concentration.
3 Results and discussion
3.1 Concentration and distribution characteristics
PAH compounds were ubiquitous in the water of the Amu Darya River Basin. 16 PAHs were detected with a total concentration range of 3.18–506.26 ng/L and a mean value of 65.83 ± 93.73 ng/L (Figure 2A). Flu, Ant, Fla, Pyr, Chr, and BaP were detected in 100% of all the water, while DahA, BghiP, and IcdP were detected at a lower rate (Supplementary Table S4). Concerning aromatic ring numbers, concentrations of PAHs detected in the waters were ND-20.034 ng/L for the 2-ring (Nap), ND-333.2 ng/L for the 3-ring (Acy, Ace, Flu, Ant), ND-24.38 ng/L for the 4-ring (Fla, Pyr, BaA, Chr) range, ND- 69.19 ng/L for the 5-ring (BbF, BkF BaP, DahA) range, and 6-ring (BghiP, IcdP) concentrations ranged from ND-48.24 ng/L.
The water samples in the study area were divided into five categories for further discussion, namely, Amu Darya River water, pond water, Aral Sea water, irrigation drainage water, and well water (Figure 1). The mean levels of ∑PAHs in each water body were 42.68 ± 39.74 ng/L, 290.57 ± 228.69 ng/L, 22.36 ± 17.55 ng/L; 71.41 ± 61.31 ng/L and 29.87 ± 20.08 ng/L as shown in Table1. The highest total concentration was found in pond water samples, mainly due to oil leakage pollution from fishing boats and other vessels docked around the ponds and the low mobility of pond water. This was followed by the irrigation drainage water samples, which were mainly return water from the irrigation of the Amu Darya River. Hence, the mean and median values were higher than the river water samples. In comparison, Aral Sea and well water samples have relatively low concentrations. Concentrations of ∑PAHs in the Amu Darya samples varied widely (CV=93.1%), especially for the high-molecular-weight PAHs (CV=141.8% for high-molecular-weight PAHs, HMW-PAHs, and 62.7% for low-molecular-weight LMW-PAHs), indicating that the variation of HMW PAHs concentrations had more influence on the ∑PAHs variation. The lowest concentration was found in the main river water of Amu Darya River, probably due to the large water discharge at this point. At the same time, the concentration of river samples showed an increasing trend from the upper to the lower reaches, and the concentration was generally increased in the area close to the Aral Sea, indicating that water flow was an important carrier for the transport of PAHs. The most abundant substances with the highest mean concentrations in the five types of water samples were mainly LMW PAHs, including Acy (114.96 and 6.27 ng/L) in pond and river samples and Nap (7.42 ng/L) in well water, which was in line with the characteristics of high-water solubility of LMW PAHs. In contrast, the highest mean concentrations of the substances in the samples of irrigation drainage and the Aral Sea were both BbF (10.4 ng/L and 2.65 ng/L), which may be related to pollution from fossil burning in the region. Acy existed at an abnormally high point (333.2 ng/L) in the pond water, and Acy was caused by the leakage and volatilizing of petroleum products, which was consistent with the situation of obvious oil pollution in the pond (Tongo et al., 2017). In contrast, the relative variability of 3-ring PAHs such as Ace, Flu, Phe, Ant, and Fla was small (CV<90%), which were mainly derived from biomass burning (Shi et al., 2005).
In water samples from the basin, 17 OCPs were detected. Water concentrations of ∑OCPs were 1.16–22.75 ng/L, with a mean value of 7.59 ng/L and a median value of 6.6 ng/L (Figure 2B). ∑HCHs (α-HCH, β-HCH, γ-HCH, δ-HCH) ranged from 0.48 to 14.71 ng/L, with a mean value of 2.72 ± 2.66 ng/L. ∑DDTs (pˏpʹ -DDE, oˏpʹ -DDT, pˏpʹ -DDD, pˏpʹ -DDT) concentrations ranged from 0.39–10.16 ng/L, with an average of 2.48 ± 2.20 ng/L. ∑Sulfan (α-Endosulfan, β-Endosulfan, Endosulfan sulfate) concentration range was ND-1.97 ng/L, mean 0.12 ± 0.36 ng/L, the concentration of ∑Chlordanes (TC and CC) was 0.06–3.28 ng/L, with a mean of 0.61 ± 0.59 ng/L. The detection rates of α-HCH, β-HCH, γ-HCH, δ-HCH, cis-Chlordane, and pˏpʹ -DDT were 100%, while Heptachlor, Endrin ketone, and Methoxychlor were not detected in the water. HCHs and DDTs were the main OCPs in water, accounting for 35.75% and 32.62%, respectively. The highest concentrations of α-HCH, Endrin aldehyde, and pˏpʹ -DDT were detected at 0.20–8.34 ng/L, 0.12–4.01 ng/L, and 0.39–10.35 ng/L, accounting for 19.6%, 15.2% and 22.2% of ∑OCPs, respectively. Compared with PAHs, the distribution of OCPs concentration in water bodies was relatively less variable, and the mean values of ∑OCPs in five different waters were 7.98 ± 5.82 ng/L in rivers, 11.98 ± 6.54 ng/L in ponds, 5.65 ± 2.53 ng/L in the Aral Sea, 6.85 ± 4.05 ng/L in irrigation drainage, and 4.93 ± 1.65 ng/L in well. The highest mean concentration of ∑OCPs in the river water of Amu Darya was accompanied by a relatively high coefficient of variation (CV=72.9%). In contrast, the lowest mean concentration and coefficient of variation of ∑OCPs in the well water samples (CV=33.4%), pˏpʹ-DDT (10.35 ng/L), and Endrin aldehyde (1.47 ng/L) were the most abundant compounds in the Amu Darya and well samples. In contrast, α-HCH (1.67, 8.34, and 4.56 ng/L) was the most prevalent compound in the Aral Sea, pond, and irrigation drainage samples. It has been reported that banned pesticides may continue to be used in these remote areas of intensive agriculture and that low levels of legacy pesticides may be the result of extensive use or may have been left behind through atmospheric deposition or recycling of irrigation runoff in the area (Ali et al., 2014; Wegerich et al., 2015).
3.2 Composition characteristics and source analysis
3.2.1 Composition characteristics and potential source analysis of PAHs
The composition of PAHs in the study area was dominated by 3-ring PAHs (Acy, Ace, Flu, Phe, Ant) (mean concentration 21.14 ± 53.14 ng, Figure 3A), and the compound with the highest detection rate, Phe, was usually associated with tar production. In contrast, the output of Flu was associated with biomass combustion, suggesting that combustion pollution was mixed in the whole basin area (Zhang et al., 2012; Han et al., 2019). LMW-PAHs were usually dominant in water due to their high solubility (Sarria-Villa et al., 2016). However, the five to six ring PAHs accounted for a relatively high proportion of the pond and the water samples in the lower reaches of the Amu Darya River near the tidal flats of the Aral Sea (Figure 3B). HMW PAHs dominated the water samples, such as the shallow inland lakes in Spain, the Nile water bodies in Egypt, and the Yellow River estuary basin, whose pollution sources were mainly from high-temperature combustion of fossil fuels (Badawy and Emababy, 2010; Hijosa-Valsero et al., 2016; Li and Li, 2017). The samples in the above areas were also at a high level of PAHs pollution, which may be contaminated by traffic, ship leakage, and coal. In addition, the lower Amu Darya River area might also affect the overall regional water environment in terms of PAHs pollution level due to the reduction of perennial runoff, high total evaporation, lack of adequate circulation in most areas, and return flow of agricultural irrigation water (Leng et al., 2021).
The ratios between the characteristic compounds of PAHs can be applied to identify potential source patterns. The ratios of Ant/(Phe+Ant) and Fla/(Fla+Pyr) in most samples in the study area were between 0.1–0.4 and 0.4–0.6 (Figure 4A), respectively, which were mainly concentrated in the burning area (Aziz et al., 2014; Montuori et al., 2016). It can be seen that PAHs in the samples mostly come from the combustion of coal, vegetation, and other biomass. The Fla/(Fla+Pyr) ratio was less than 0.4 at some sites, including the downstream locations of the Amu Darya River and the pond samples, indicating the presence of petroleum pollution in this area, and combined with the Ant/(Phe+Ant) ratio. It can be judged that there was a mixed source of petroleum and combustion pollution of PAHs in the study area.
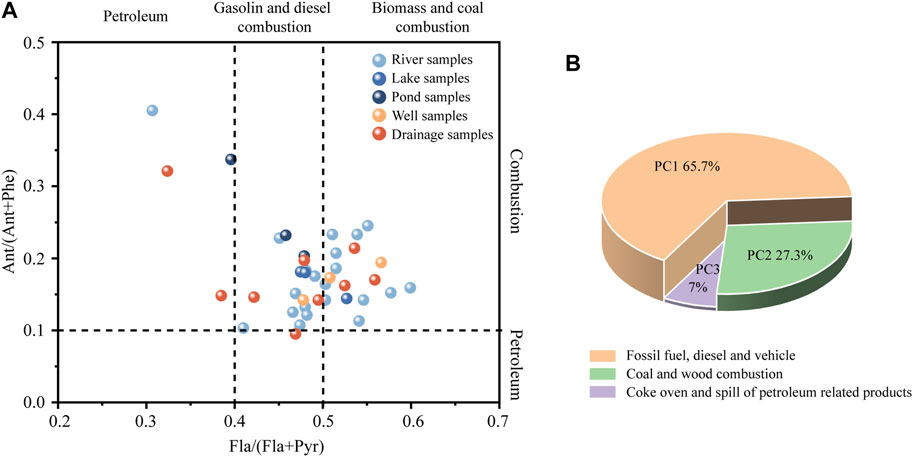
FIGURE 4. The PAH cross-plots for the ratio Ant/(Ant+Phe) versus Fla/(Fla+Pyr) (A); Source composition profiles based on the PCA analysis (B) in waters in the study area.
In addition to qualitatively distinguishing pollutant sources by diagnostic ratios, PCA was also used to identify the pollution contributions of PAHs in this study (Figure 4B) (Gupta et al., 2021). Three principal components were determined with average contributions of 65.2%, 27.2%, and 7.64%, respectively. PC1 was mainly loaded by the HWM PAHs BkF and BghiP, followed by BaA, Chr, BaP, IcdP, Pyr, and DahA, which indicated the combustion source of fossil fuels such as coal and oil. Also, it included exhaust emission sources of gasoline and diesel burning from vehicles (Guo et al., 2009). The LWM PAHs Flu, Ace, Nap, and Phe were considered wood straw burning sources, and Ant was related to coal burning. Thus, PC2 could represent the contribution of biomass combustion. PC3 was loaded predominantly by Acy, and Fla. Fla was considered coke oven emissions. Acy also has sources such as leakage of gasoline-related products and vapor evaporation (Farshid, 2015). Natural gas was currently the essential fuel for power generation in the former CIS (Commonwealth of Independent States countries), with a decadal average consumption of 45.32 bcm (billion cubic meters), and has been the preferred energy source in Uzbekistan, while coal used in the country has been increasing for ten consecutive years, making energy use a significant contributor to PAHs in the study region (Bandowe et al., 2010; Zhan et al., 2021). In summary, the sources of PAHs in the Amu Darya River Basin are dominated by high-temperature combustion of petroleum, with a mix of biological and natural gas sources that echoes and complements the diagnostic ratio method.
3.2.2 Composition characteristics and potential source analysis of OCPs
By comparing and analyzing the residual concentrations of OCPs in different rivers worldwide, it can be found that both DDTs and HCHs are the major pollutants, although there are differences in pollution levels (Li et al., 2018b; Shen et al., 2021). HCHs and DDTs are also significant pollutants in this region. HCHs in the environment mainly originated from technical HCHs and lindane. Generally, technical HCHs consist of α-HCH (60%–70%), β-HCH (5%–12%), γ-HCH (10%–15%), and δ-HCH (6%–10%), while Lindane was mainly composed of γ-HCH, accounting for 99% (MacDonald et al., 2000). The ratio of α-HCH/γ-HCH was commonly used in studies to estimate regional HCHs pollution sources (Han and Currell, 2017). The average concentrations of HCH isomers in water in the study area were α-HCH: 55.55%, β-HCH: 23.86%, γ-HCH: 12.51%, δ-HCH: 10.08%, and the ratio of α-HCH/γ-HCH was 1.13–12.09, with an average of 4.51 (Figure 5A). The α-HCH/γ-HCH ratios in the Aral Sea, well, and most of the irrigation drainage water samples were less than 4, indicating that the HCHs in the samples were predominantly of lindane origin. The α-HCH/γ-HCH ratios in the river samples of the Amu Darya River were mainly concentrated between 4 and 7, which were thought to be contaminated by technical HCHs. In addition, γ-HCH could be converted to α-HCH by long-term weathering, including some river samples and two irrigation drainage samples in which HCHs were dominated by the isomeric α-HCH, indicating that the HCHs in these samples mainly originated from the residual contamination of historical technical HCHs. β-HCH was the most stable in the environment, and the other HCH isomers would transform to β-HCH over time (Liu et al., 2021). The detection rate of β-HCH in water was 100%, and the proportion of β-HCH in HCHs ranged from 10.66% to 43.76%, which was higher than that in technical HCHs (5%–12%). This reflects that HCHs in the region were predominantly caused by historical residues (Bao et al., 2012; Khuman et al., 2020). The ratios of DDT and its degradation products, DDD and DDE, were similar to those of HCHs. They have also been used to study the metabolism of OCPS in the environment and analyze pollution sources. DDT can be degraded into DDE and DDD according to aerobic and anaerobic. The (DDE+ DDD)/DDT ratio was generally used as an index to determine whether new pollution sources were entering the water (Ndunda et al., 2018). In this study, the ratios of (DDE + DDD)/DDT was less than 0.5 (mean 0.24, Figure 5B) at most sites, except for the samples from the Aral Sea and the pond near it, suggesting recent DDT input into the aquatic system. The source of DDT was also determined by using the ratio of oˏp'-DDT/pˏp'-DDT according to the different composition of technical DDT (80%–85% pˏp'-DDT, 15%–20% oˏp'- DDT) and dicofol (1.7% pˏp'-DDT and 11.4% oˏp'-DDT). When oˏp'-DDT/pˏp'-DDT ratios <2.5 and >6.7 indicated that the sources were technical DDT and dicofol, respectively (Zhang et al., 2021). We found oˏp'-DDT/pˏp'-DDT ratios yielded a range of 0–5.05, with a mean value of 0.33 in most samples, indicating the main source of technical DDTs, in only one of the irrigation samples, ratios fell within the range of 2.6–6.7, suggesting the existence of mixed sources of dicofol and technical DDT (Xie et al., 2020; Liu et al., 2021).
Endrin is widely used to control agricultural pests and rodenticides, which can be metabolized to endrin aldehyde and endrin ketone (Zhang et al., 2021; Aydin and Albay, 2022). Endrin aldehyde was detected in water bodies in the region at a higher concentration and detection rate (92.5%, mean value 1.26 ± 0.72 ng/L) than endrin (7.5%, mean value 0.55 ± 0.49 ng/L), suggesting that endrin was used extensively in the region historically. Chlordane was once used as a potent, persistent organochlorine insecticide on vegetables, rice, corn, cotton, and other plants (Sah et al., 2020). Technical chlordane available on the international market contains 13% trans-chlordane (TC), 11% cis-chlordane (CC), and 5% heptachlor, resulting in a TC/CC ratio of technical chlordane close to 1.56. The mean values of TC and CC detected in this study were 0.13 ± 0.13 and 0.54 ± 0.57 ng/L, respectively. The TC/CC ratio range was 0–1.54, with a mean value of 0.19, mainly due to the faster degradation rate of TC than CC in the environment. It represents the historical residue of technical chlordane (Vasseghian et al., 2021). The detection rate of endosulfan sulfate in regional samples was 30%, which was higher than that of α- Endosulfan and β-Endosulfan, and the ratio of Endosulfan sulfate/(α-Endosulfan +β-Endosulfan)>1, indicating a high degree of degradation of endosulfan, which is an efficient and broad-spectrum insecticide and acaricide with a good control effect on a variety of crop pests. The oxidation of endosulfan mainly generated the residue of endosulfan sulfate in the region.
The introduction of cotton monoculture in Central Asia during the Soviet era in the 1950s began a 40-year history of intensive use of agricultural chemicals, including in the autonomous republics of Karakalpak stan and Khorezm Oblast, which are adjacent to the Aral Sea in Uzbekistan and are still among the most agriculturally productive regions of the country (Chapagain et al., 2006; Sharov et al., 2016). In the late 1970s, pesticide use in Central Asia was generally between 30 and 35 kg/ha, almost 30 times higher than in the rest of the former Soviet Union during the same period (Snow et al., 2020). It had been found that the contamination levels of DDT and γ-HCH in drinking water in the Karakalpak Stan region were above the standards of Uzbekistan and international recommendations, and HCHs had been detected in food such as onions, potatoes, carrots, and rice. The OCPs residues in fish fat, chicken fat, and egg samples were relatively high, and α-, γ-HCH were almost ubiquitous. The other OCPs, such as heptachlor and methoxychlor were uncommon, and these findings were consistent with the OCPs detected in the water in this study, where OCPs residues in food may have resulted from contact with contaminated water or soil (Muntean et al., 2003; Herbst et al., 2008; Snow et al., 2020). Particularly in the case of the Aral Sea, where the riverbed was constantly absorbing saline substances from the watershed and pesticides and herbicides from agricultural drainage water, the dry riverbed, which was expanding yearly, had become an essential source of local dust and salt aerosols, and pollutants were deposited over large areas through atmospheric transport (Lozowicka et al., 2015; Burr et al., 2019; Yuldasheva et al., 2021).
3.3 Risk assessment
3.3.1 Health risk assessment
OCPs and PAHs in the waters of the study area posed a non-carcinogenic risk to adults and children through the drinking route, as shown in Figure 6. According to USEPA guidelines, the non-carcinogenic risk assessment threshold is 1, above which a potential non-carcinogenic risk exists (Barhoumi et al., 2019; Rodrigues et al., 2019). The range of non-carcinogenic risk values for OCPs and PAHs in waters in the study area was 0–2.07×10−4 and 0–1.3×10−3 for adults and children, respectively, with HI <1 for all compounds, suggesting that non-carcinogenic pollutants may not harm health (Figure 6A). The USEPA evaluates carcinogenic risk values in drinking water in the range of 10−4–10–6, with a potentially significant risk considered when the carcinogenic risk value exceeds 10–4 and a negligible risk if the carcinogenic risk value is less than 10–6. Risk values in the range of 10−6–10–4 indicate some risk to vulnerable groups but are acceptable (Mahmoud et al., 2016; Li et al., 2018a). Carcinogenic risk values for adults in the water of the study area ranged from 10–11 to 10–6, which is below the threshold, but the carcinogenic risk values for α-HCH, BaA, and BbF for children ranged from 10–6 to 10–4, which suggested a certain level of risk for vulnerable groups, such as children (Figure 6B). Children were found to be more susceptible to carcinogenic contamination by OCPs and PAHs compared to adults; previous studies of pollution in Dongting Lake, China (Cao et al., 2021) and the Thamirabarani River, India, reported similar results (Arisekar et al., 2021).
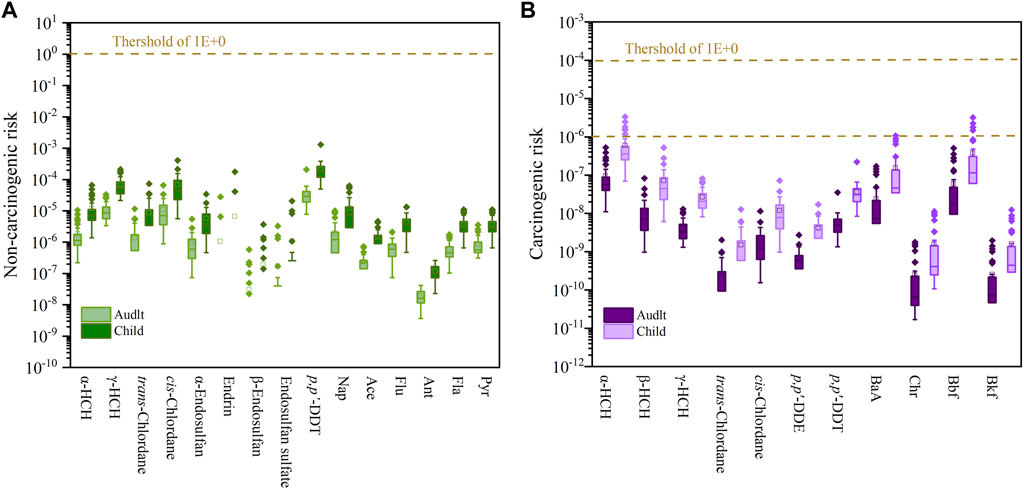
FIGURE 6. Non-carcinogenic risk (A) and carcinogenic risk (B) of OCPs and PAHs in water samples collected in the study area.
3.3.2 Ecological risk assessment
As shown in Figure 7, the RQ values of OCPs in the sampling sites were less than one, mainly at low pollution levels and low ecological risk, except for pˏp'-DDT. In some water sampling sites (16 sites, about 40%), pˏp'-DDT was at medium risk, and the sample sites were mainly concentrated in the pond, irrigation drainage, and river loss to the coast of the Aral Sea. Pyr, BaP, Ace, BkF, and Flu were at medium risk in most water samples (>80%), while Acy, BaA, BbF, Bghip, and IcdP were at high risk with RQMPCs >1. Similar to the distribution of high ecological risk values for OCPs, high ecological risk values for PAHs were mainly concentrated in ponds and river waters along the Amu Darya River, and three samples were at high risk, which could lead to adverse biological effects in the study area. The displacement of the shoreline and the corresponding decline in the groundwater level impeded the exchange of surface water and groundwater, increased evaporation, and led to further deterioration of water quality along the Aral Sea coast (Shibuo et al., 2006; Vitola et al., 2012). The geographic location, climate, and hydrologic conditions make the shoreline waters the most polluted in the study area.
4 Conclusion
As demonstrated in the present study, OCPs and PAHs were detected in different water bodies of the Amu Darya River Basin and the Aral Sea in the Central Asian arid region, with levels in the range of 1.16–22.75 ng/L and 3.18–506.26 ng/L, respectively. The pond waters and the Aral Sea coastal area downstream of the Amu Darya River were identified as the predominant contaminated areas, documenting the potential effects of climatic and hydrological parameters like the water flow direction as well as the surrounding anthropogenic sources. Source apportionment suggested by the isomer ratios and the principal component analysis indicated that PAHs mainly originated from combustion processes, including coal and oil combustion, biomass such as wood straw and industrial coke combustion, showing contributions of 65.2%, 27.2%, and 7.64%, respectively, while most OCPs were mainly from historical usage especially due to the possible large application for monoculture of cotton cultivation. Risk assessment of PAHs and OCPs observed in waters showed no carcinogenic or non-carcinogenic health risk for people around. In contrast, the highly ecological risk induced by Acy, BaA, BbF, BghiP, and IcdP should be emphasized. Therefore, high residues and risks would be induced by such toxic and consistent pollutants during its shrinking crisis because of the concentration effects in the future. More attention needs to be paid to this topic, considering the significant environmental meaning and regional importance of the Aral Sea.
Data availability statement
The original contributions presented in the study are included in the article/Supplementary Material, further inquiries can be directed to the corresponding author.
Author contributions
MJ: Conceptualization, Data curation, Methodology, Software, Writing–original draft. JW: Funding acquisition, Investigation, Methodology, Validation, Writing–review and editing. HZ: Formal Analysis, Visualization. ZZ: Supervision, Validation, Writing–review and editing. MA: Supervision, Validation. RG: Formal Analysis.
Funding
The author(s) declare financial support was received for the research, authorship, and/or publication of this article. The study was supported by the National Natural Science Foundation of China (U2003202 and 41671200) and the Strategic Priority Research Program of Chinese Academy of Sciences, Pan-Third Pole Environment Study for a Green Silk Road (XDA2006030101). This work was funded by the Researchers Supporting Project Number (RSP2023R113) at King Saud University, Riyadh, Saudi Arabia.
Acknowledgments
We thank the CAS Research Center for Ecology and Environment of Central Asia for this work and Huawu Wu and Shuie Zhan for field and laboratory assistance.
Conflict of interest
The authors declare that the research was conducted in the absence of any commercial or financial relationships that could be construed as a potential conflict of interest.
Publisher’s note
All claims expressed in this article are solely those of the authors and do not necessarily represent those of their affiliated organizations, or those of the publisher, the editors and the reviewers. Any product that may be evaluated in this article, or claim that may be made by its manufacturer, is not guaranteed or endorsed by the publisher.
Supplementary material
The Supplementary Material for this article can be found online at: https://www.frontiersin.org/articles/10.3389/feart.2023.1295485/full#supplementary-material
Abbreviations
CCCs, continuous criteria concentrations; CIS, commonwealth of independent states countries; DAD, diode array detector; DDTs, dichlorodiphenyltrichloroethanes; EPA, environmental protection agency; FLD, fluorescence detector; GC-ECD, gas chromatograph- electron capture detector; GC-MS, gas chromatograph-mass spectrometer; HCHs, hexachlorocyclohexanes; HI, hazard index; HMW, high-molecular-weight; HPLC, high-performance liquid chromatography; LOD, limit of detection; LMW, low-molecular-weight; MPCs, maximum permissible concentrations; NCs, negligible concentrations; OCP, organochlorine pesticides; PAHs, polycyclic aromatic hydrocarbons; PCA, principal component analysis; POPs, persistent organic pollutants; RQ, risk quotient; RSDs, relative standard deviations; SPE, solid-phase extraction; USEPA, united states environmental protection agency; WHO, world health organization.
References
Ai, S. H., Gao, X. Y., Wang, X. N., Li, J., Fan, B., Zhao, S. Q., et al. (2021). Exposure and tiered ecological risk assessment of phthalate esters in the surface water of Poyang Lake, China. Chemosphere 262, 127864. doi:10.1016/j.chemosphere.2020.127864
Ali, U., Syed, J. H., Malik, R. N., Katsoyiannis, A., Li, J., Zhang, G., et al. (2014). Organochlorine pesticides (OCPs) in South Asian region: a review. Sci. Total Environ. 476-477, 705–717. doi:10.1016/j.scitotenv.2013.12.107
Arisekar, U., Shakila, R. J., Shalini, R., and Jeyasekaran, G. (2021). Pesticides contamination in the Thamirabarani, a perennial river in peninsular India: the first report on ecotoxicological and human health risk assessment. Chemosphere 267, 129251. doi:10.1016/j.chemosphere.2020.129251
Artikov, H., Kočárek, M., Fraňková, A., Abdrakhmanov, T., Borůvka, L., and Sharipov, U. (2018). Residues of organochlorine pesticides in irrigated sierozem-meadow soils around buried chemicals stock. Soil Water Res. 13 (2), 108–114. doi:10.17221/131/2017-swr
Aydin, F., and Albay, M. (2022). Accumulation of organochlorine pesticide (OCP) residues in surface water and sediment from the İznik Lake in Turkey. Environ. Monit. Assess. 194 (12), 872. doi:10.1007/s10661-022-10505-x
Aziz, F., Syed, J. H., Malik, R. N., Katsoyiannis, A., Mahmood, A., Li, J., et al. (2014). Occurrence of polycyclic aromatic hydrocarbons in the Soan River, Pakistan: insights into distribution, composition, sources and ecological risk assessment. Ecotoxicol. Environ. Saf. 109, 77–84. doi:10.1016/j.ecoenv.2014.07.022
Badawy, M., and Emababy, M. A. (2010). Distribution of polycyclic aromatic hydrocarbons in drinking water in Egypt. Desalination 251 (1-3), 34–40. doi:10.1016/j.desal.2009.09.148
Bai, Y., Ruan, X. H., and van der Hoek, J. P. (2018). Residues of organochlorine pesticides (OCPs) in aquatic environment and risk assessment along Shaying River, China. Environ. Geochem Health 40 (6), 2525–2538. doi:10.1007/s10653-018-0117-9
Bandowe, B. A. M., Shukurov, N., Kersten, M., and Wilcke, M. (2010). Polycyclic aromatic hydrocarbons (PAHs) and their oxygen-containing derivatives (OPAHs) in soils from the Angren industrial area, Uzbekistan. Environ. Pollut. 158 (9), 2888–2899. doi:10.1016/j.envpol.2010.06.012
Bao, L. J., Maruya, K. A., Snyder, S. A., and Zeng, E. Y. (2012). China's water pollution by persistent organic pollutants. Environ. Pollut. 163, 100–108. doi:10.1016/j.envpol.2011.12.022
Barhoumi, B., Beldean-Galea, M. S., Al-Rawabdeh, A. M., Roba, C., Martonos, I. M., Bălc, R., et al. (2019). Occurrence, distribution and ecological risk of trace metals and organic pollutants in surface sediments from a Southeastern European river (Someşu Mic River, Romania). Sci. Total Environ. 660, 660–676. doi:10.1016/j.scitotenv.2018.12.428
Bourgeault, A., and Gourlay-France, C. (2013). Monitoring PAH contamination in water: comparison of biological and physico-chemical tools. Sci. Total Environ. 454-455, 328–336. doi:10.1016/j.scitotenv.2013.03.021
Burr, G. S., Kuzmin, Y. V., Krivonogov, S. K., Gusskov, S. A., and Cruz, R. J. (2019). A history of the modern Aral Sea (central Asia) since the late pleistocene. Quat. Sci. Rev. 206, 141–149. doi:10.1016/j.quascirev.2019.01.006
Cao, F., Li, Z. Z., He, Q., Lu, S. Y., Qin, P., and Li, L. L. (2021). Occurrence, spatial distribution, source, and ecological risk assessment of organochlorine pesticides in Dongting Lake, China. Environ. Sci. Pollut. Res. Int. 28 (24), 30841–30857. doi:10.1007/s11356-021-12743-x
Chapagain, A. K., Hoekstra, A. Y., Savenije, H. H. G., and Gautam, R. (2006). The water footprint of cotton consumption: an assessment of the impact of worldwide consumption of cotton products on the water resources in the cotton producing countries. Ecol. Econ. 60 (1), 186–203. doi:10.1016/j.ecolecon.2005.11.027
Crosa, G., Stefani, F., Bianchi, C., and Fumagalli, A. (2006). Water security in Uzbekistan: implication of return waters on the Amu Darya water quality. Environ. Sci. Pollut. Res. Int. 13 (1), 37–42. doi:10.1065/espr2006.01.007
Farshid, K. (2015). Distribution and sources of polycyclic aromatic hydrocarbons in water and sediments of the Soltan Abad River, Iran. EGYPT J. AQUAT. Res. 41, 227–231. doi:10.1016/j.ejar.2015.06.004
Groll, M., Opp, C., Kulmatov, R., Ikramova, M., and Normatov, I. (2013). Water quality, potential conflicts and solutions—an upstream–downstream analysis of the transnational Zarafshan River (Tajikistan, Uzbekistan). Environ. EARTH Sci. 73 (2), 743–763. doi:10.1007/s12665-013-2988-5
Guo, W., He, M. C., Yang, Z. F., Lin, C. Y., Quan, X. C., and Men, B. (2009). Distribution, partitioning and sources of polycyclic aromatic hydrocarbons in Daliao River water system in dry season, China. J. HAZARD MATER, 164, 1379–1385. doi:10.1016/j.jhazmat.2008.09.083
Gupta, N., Yadav, K. K., Kumar, V., Cabral-Pinto, M. M. S., Alam, M., Kumar, S., et al. (2021). Appraisal of contamination of heavy metals and health risk in agricultural soil of Jhansi city, India. Environ. Toxicol. Pharmacol. 88, 103740. doi:10.1016/j.etap.2021.103740
Han, D. M., and Currell, M. J. (2017). Persistent organic pollutants in China's surface water systems. Sci. Total Environ. 580, 602–625. doi:10.1016/j.scitotenv.2016.12.007
Han, J., Liang, Y. S., Zhao, B., Wang, Y., Xing, F. T., and Qin, L. B. (2019). Polycyclic aromatic hydrocarbon (PAHs) geographical distribution in China and their source, risk assessment analysis. Environ. Pollut. 251, 312–327. doi:10.1016/j.envpol.2019.05.022
Herbst, S., Fayzieva, D., and Kistemann, T. (2008). Risk factor analysis of diarrhoeal diseases in the Aral Sea area (Khorezm, Uzbekistan). Int. J. Environ. Health Res. 18 (5), 305–321. doi:10.1080/09603120701834507
Hijosa-Valsero, M., Becares, E., Fernandez-Alaez, C., Fernandez-Alaez, M., Mayo, R., and Jimenez, J. J. (2016). Chemical pollution in inland shallow lakes in the Mediterranean region (NW Spain): PAHs, insecticides and herbicides in water and sediments. Sci. Total Environ. 544, 797–810. doi:10.1016/j.scitotenv.2015.11.160
Jin, Q. J., Wei, J. F., Yang, Z. L., and Lin, P. R. (2017). Irrigation-induced environmental changes around the Aral Sea: an integrated view from multiple satellite observations. REMOTE SENS-BASEL 9 (9), 900. doi:10.3390/rs9090900
Karthe, D., Abdullaev, I., Boldgiv, B., Borchardt, D., Chalov, S., Jarsjö, J., et al. (2017). Water in Central Asia: an integrated assessment for science-based management. Environ. EARTH Sci. 76 (20), 690. doi:10.1007/s12665-017-6994-x
Khuman, S. N., Vinod, P. G., Bharat, G., Kumar, Y. S. M., and Chakraborty, P. (2020). Spatial distribution and compositional profiles of organochlorine pesticides in the surface soil from the agricultural, coastal and backwater transects along the south-west coast of India. Chemosphere 254, 126699. doi:10.1016/j.chemosphere.2020.126699
Kwach, B. O., and Lalah, J. O. (2009). High concentrations of polycyclic aromatic hydrocarbons found in water and sediments of car wash and Kisat areas of Winam Gulf, Lake Victoria-Kenya. Bull. Environ. Contam. Toxicol. 83 (5), 727–733. doi:10.1007/s00128-009-9859-5
Leng, P. F., Zhang, Q. Y., Li, F. D., Kulmatov, R., Wang, G. Q., Qiao, Y. F., et al. (2021). Agricultural impacts drive longitudinal variations of riverine water quality of the aral Sea basin (Amu Darya and Syr Darya rivers), central Asia. Environ. Pollut. 284, 117405. doi:10.1016/j.envpol.2021.117405
Li, J., and Li, F. D. (2017). Polycyclic aromatic hydrocarbons in the Yellow River estuary: levels,sources and toxic potency assessment. Mar. Pollut. Bull. 116, 479–487. doi:10.1016/j.marpolbul.2016.11.043
Li, Q. F., Lu, Y. L., Wang, P., Wang, T. Y., Zhang, Y. Q., Suriyanarayanan, S., et al. (2018a). Distribution, source, and risk of organochlorine pesticides (OCPs) and polychlorinated biphenyls (PCBs) in urban and rural soils around the Yellow and Bohai Seas, China. Environ. Pollut. 239, 233–241. doi:10.1016/j.envpol.2018.03.055
Li, Q. Y., Wu, J. L., Zhao, Z. H., and Sakiev, K. (2018b). Organochlorine pesticides in soils from the Issyk-Kul region in the western Tian Shan Mountains, Kyrgyzstan: implication for spatial distribution, source apportionment and ecological risk assessment. JMS 15 (7), 1520–1531. doi:10.1007/s11629-018-4963-9
Liu, Q., Zheng, J. P., Guo, Z. L., and Fan, C. N. (2021). Sources and health risk of organochlorine pesticides in surface water from buerhatong River and hunchun river in northeast China. WATER AIR SOIL Poll. 232 (10), 407. doi:10.1007/s11270-021-05329-3
Lozowicka, B., Abzeitova, E., Sagitov, A., Kaczynski, P., Toleubayev, K., and Li, A. (2015). Studies of pesticide residues in tomatoes and cucumbers from Kazakhstan and the associated health risks. Environ. Monit. Assess. 187 (10), 609. doi:10.1007/s10661-015-4818-6
MacDonald, D. D., Ingersoll, C. G., and Berger, T. A. (2000). Development and evaluation of consensus-based sediment quality guidelines for freshwater ecosystems. Arch. Environ. Contam. Toxicol. 39 (1), 20–31. doi:10.1007/s002440010075
Mahmoud, A. F. A., Ikenaka, Y., Yohannes, Y. B., Darwish, W. S., Eldaly, E. A., Morshdy, A. E., et al. (2016). Distribution and health risk assessment of organochlorine pesticides (OCPs) residue in edible cattle tissues from northeastern part of Egypt: high accumulation level of OCPs in tongue. Chemosphere 144, 1365–1371. doi:10.1016/j.chemosphere.2015.10.016
Matlyuba, M., Nekqadam, N., and Nilufar, A. (2019). Results of research on the study of the chemical composition of the Amu Darya River’s water in the dynamics. Am. J. Med. Sci. 9 (7), 270–274. doi:10.5923/j.ajmms.20190907.09
Mojiri, A., Zhou, J. L., Ohashi, A., Ozaki, N., and Kindaichi, T. (2019). Comprehensive review of polycyclic aromatic hydrocarbons in water sources, their effects and treatments. Sci. Total Environ. 696, 133971. doi:10.1016/j.scitotenv.2019.133971
Montuori, P., Auri, S., Garzonio, F., Sarnacchiaro, F., Nardone, A., and Triassi, M. (2016). Distribution, sources and ecological risk assessment of polycyclic aromatic hydrocarbons in water and sediments from Tiber River and estuary, Italy. Sci. Total Environ. 566-567, 1254–1267. doi:10.1016/j.scitotenv.2016.05.183
Muntean, N., Jermini, M., Small, I., Falzon, D., Furst, P., Migliorati, G., et al. (2003). Assessment of dietary exposure to some persistent organic pollutants in the Republic of Karakalpakstan of Uzbekistan. Environ. Health Perspect. 111 (10), 1306–1311. doi:10.1289/ehp.5907
Nagy, A. S., Simon, G., Szabó, J., and Vass, I. (2013). Polycyclic aromatic hydrocarbons in surface water and bed sediments of the Hungarian upper section of the Danube River. Environ. Monit. Assess. 185 (6), 4619–4631. doi:10.1007/s10661-012-2892-6
Ndunda, E. N., Madadi, V. O., and Wandiga, S. O. (2018). Organochlorine pesticide residues in sediment and water from Nairobi River, Kenya: levels, distribution, and ecological risk assessment. Environ. Sci. Pollut. Res. Int. 25 (34), 34510–34518. doi:10.1007/s11356-018-3398-8
Nezlin, N. P., Kostianoy, A. G., and Li, B. L. (2005). Inter-annual variability and interaction of remote-sensed vegetation index and atmospheric precipitation in the Aral Sea region. J. Arid. Environ. 62 (4), 677–700. doi:10.1016/j.jaridenv.2005.01.015
Rodrigues, J. P., Duarte, A. C., Santos-Echeandía, J., and Rocha-Santos, T. (2019). Significance of interactions between microplastics and POPs in the marine environment: a critical overview. TRAC-TREND Anal. Chem. 111, 252–260. doi:10.1016/j.trac.2018.11.038
Sah, R., Baroth, A., and Hussain, S. A. (2020). First account of spatio-temporal analysis, historical trends, source apportionment and ecological risk assessment of banned organochlorine pesticides along the Ganga River. Environ. Pollut. 263, 114229. doi:10.1016/j.envpol.2020.114229
Sarria-Villa, R., Ocampo-Duque, W., Paez, M., and Schuhmacher, M. (2016). Presence of PAHs in water and sediments of the Colombian Cauca River during heavy rain episodes, and implications for risk assessment. Sci. Total Environ. 540, 455–465. doi:10.1016/j.scitotenv.2015.07.020
Schlüter, M., Khasankhanova, G., Talskikh, V., Taryannikova, R., Agaltseva, N., Joldasova, I., et al. (2013). Enhancing resilience to water flow uncertainty by integrating environmental flows into water management in the Amudarya River, Central Asia. Glob. PLANET CHANGE 110, 114–129. doi:10.1016/j.gloplacha.2013.05.007
Schlüter, M., Savitsky, A. G., McKinney, D. C., and Lieth, H. (2005). Optimizing long-term water allocation in the Amudarya River delta: a water management model for ecological impact assessment. Environ. Modell. Softw. 20 (5), 529–545. doi:10.1016/j.envsoft.2004.03.005
Sharov, P., Dowling, R., Gogishvili, M., Jones, B., Caravanos, J., McCartor, A., et al. (2016). The prevalence of toxic hotspots in former Soviet countries. Environ. Pollut. 211, 346–353. doi:10.1016/j.envpol.2016.01.019
Shen, B. B., Wu, J. L., Zhan, S. E., and Jin, M. (2021). Residues of organochlorine pesticides (OCPs) and polycyclic aromatic hydrocarbons (PAHs) in waters of the Ili-Balkhash Basin, arid Central Asia: concentrations and risk assessment. Chemosphere 273, 129705. doi:10.1016/j.chemosphere.2021.129705
Shi, Z., Tao, S., Pan, B., Fan, W., He, X. C., Zuo, Q., et al. (2005). Contamination of rivers in Tianjin, China by polycyclic aromatic hydrocarbons. Environ. Pollut. 134, 97–111. doi:10.1016/j.envpol.2004.07.014
Shibuo, Y., Jarsjö, J., and Destouni, G. (2006). Bathymetry-topography effects on saltwater-fresh groundwater interactions around the shrinking Aral Sea. Water Res. 42 (11). doi:10.1029/2005wr004207
Snow, D. D., Chakraborty, P., Uralbekov, B., Satybaldiev, B., Sallach, J. B., Hampton, L. M. T., et al. (2020). Legacy and current pesticide residues in Syr Darya, Kazakhstan: contamination status, seasonal variation and preliminary ecological risk assessment. Water Res. 184, 116141. doi:10.1016/j.watres.2020.116141
Tao, Y., Bao, A. M., Xu, W. Q., Guo, H., Jiang, L. L., Zheng, G. X., et al. (2019). Exploring variability in landscape ecological risk and quantifying its driving factors in the Amu Darya delta. Int.J.Environ.Res.Public Health 17 (79), 79. doi:10.3390/ijerph17010079
Tongo, I., Ezemonye, L., and Akpeh, K. (2017). Levels, distribution and characterization of polycyclic aromatic hydrocarbons (PAHs) in ovia river, southern Nigeria. J. Environ. Chem. Eng. 5 (1), 504–512. doi:10.1016/j.jece.2016.12.035
Tornqvist, R., Jarsjo, J., and Karimov, B. (2011). Health risks from large-scale water pollution: trends in Central Asia. Environ. Int. 37 (2), 435–442. doi:10.1016/j.envint.2010.11.006
Unger-Shayesteh, K., Vorogushyn, S., Merz, B., and Frede, H. G. (2013). Introduction to “water in central Asia — perspectives under global change”. Glob. PLANET CHANGE 110, 1–3. doi:10.1016/j.gloplacha.2013.09.016
Vasseghian, Y., Hosseinzadeh, S., Khataee, A., and Dragoi, E. N. (2021). The concentration of persistent organic pollutants in water resources: a global systematic review, meta-analysis and probabilistic risk assessment. Sci. Total Environ. 796, 149000. doi:10.1016/j.scitotenv.2021.149000
Vitola, I., Vircavs, V., Abramenko, K., Lauva, D., and Veinbergs, A. (2012). Precipitation and air temperature impact on seasonal variations of groundwater levels. Environ. Clim. Technol. 10 (1), 25–33. doi:10.2478/v10145-012-0022-x
Wegerich, K., Van Rooijen, D., Soliev, I., and Mukhamedova, N. (2015). Water security in the Syr Darya Basin. Water 7 (12), 4657–4684. doi:10.3390/w7094657
Wu, C. F., Luo, Y. M., Gui, T., and Huang, Y. J. (2014). Concentrations and potential health hazards of organochlorine pesticides in (shallow) groundwater of Taihu Lake region, China. Sci. Total Environ. 470-471, 1047–1055. doi:10.1016/j.scitotenv.2013.10.056
Xie, F., Xie, Z. L., Zhou, B., Li, L., Zhou, X. J., Fan, Q. Y., et al. (2020). Characteristics and health risk assessment of organochlorine pesticides (OCPs) residues along sino-Russian boundary river. WATER AIR SOIL Poll. 231 (10), 500. doi:10.1007/s11270-020-04877-4
Yadav, K. K., Kumar, V., Gupta, N., Kumar, S., Rezania, S., and Singh, N. (2019). Human health risk assessment: study of a population exposed to fluoride through groundwater of Agra city, India. Regul. Toxicol. Pharm. 106, 68–80. doi:10.1016/j.yrtph.2019.04.013
Yang, X. W., Wang, N. J., Chen, A. A., He, J., Hua, T., and Qie, Y. F. (2020). Changes in area and water volume of the Aral Sea in the arid Central Asia over the period of 1960–2018 and their causes. Catena 191, 104566. doi:10.1016/j.catena.2020.104566
Yuldasheva, S., Sherimbetov, S., Tulaganov, A., and Khaydarova, K. (2021). Detection of heavy metals and pesticides in the raw materials of Araxacum Officinale Wigg and Taraxacum Kok-saghyz Rodin plants. E3S Web of Conferences 244, 02035. doi:10.1051/e3sconf/202124402035
Zhan, S. E., Wu, J. L., and Jin, M. (2021). Hydrochemical characteristics, trace element sources, and health risk assessment of surface waters in the Amu Darya Basin of Uzbekistan, arid Central Asia. Environ. Sci. Pollut. Res. Int. 29, 5269–5281. doi:10.1007/s11356-021-15799-x
Zhang, K., Liang, B., Wang, J. L., Guan, Y. F., and Zeng, E. Y. (2012). Polycyclic aromatic hydrocarbons in upstream riverine runoff of the Pearl River Delta, China: an assessment of regional input sources. Environ. Pollut., 167, 78–84. doi:10.1016/j.envpol.2012.03.049
Zhang, R. F., Wang, W. L., Shi, X. R., Yu, X. Z., Li, M., Xiao, L., et al. (2011). Health risk of semi-volatile organic pollutants in Wujin river inflow into Taihu Lake. Ecotoxicology 20 (5), 1083–1089. doi:10.1007/s10646-011-0682-5
Keywords: Amu Darya basin, organochlorine pesticides, polycyclic aromatic hydrocarbons, ecotoxicological risk, Aral Sea
Citation: Jin M, Wu J, Zhang H, Zhao Z, Alam M and Guo R (2023) Study on the Aral Sea crisis from the risk assessment of polycyclic aromatic hydrocarbons and organochlorine pesticides in surface water of Amu Darya river basin in Uzbekistan. Front. Earth Sci. 11:1295485. doi: 10.3389/feart.2023.1295485
Received: 16 September 2023; Accepted: 04 December 2023;
Published: 29 December 2023.
Edited by:
Munesh Kumar, Hemwati Nandan Bahuguna Garhwal University, IndiaReviewed by:
Krishna Kumar Yadav, Madhyanchal Professional University, IndiaCarlos German Massone, Pontifical Catholic University of Rio de Janeiro, Brazil
Copyright © 2023 Jin, Wu, Zhang, Zhao, Alam and Guo. This is an open-access article distributed under the terms of the Creative Commons Attribution License (CC BY). The use, distribution or reproduction in other forums is permitted, provided the original author(s) and the copyright owner(s) are credited and that the original publication in this journal is cited, in accordance with accepted academic practice. No use, distribution or reproduction is permitted which does not comply with these terms.
*Correspondence: Jinglu Wu, dy5qaW5nbHVAbmlnbGFzLmFjLmNu