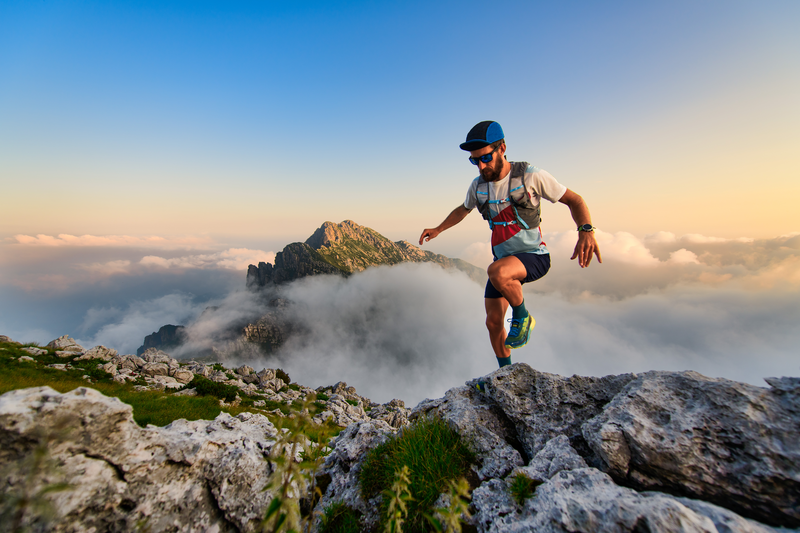
94% of researchers rate our articles as excellent or good
Learn more about the work of our research integrity team to safeguard the quality of each article we publish.
Find out more
ORIGINAL RESEARCH article
Front. Earth Sci. , 09 January 2024
Sec. Structural Geology and Tectonics
Volume 11 - 2023 | https://doi.org/10.3389/feart.2023.1284996
This article is part of the Research Topic Research Progress on the Formation Mechanism, Controlling Factors and Hydrocarbon Accumulation Patterns of Unconventional Oil and Gas Reservoirs Worldwide View all 9 articles
In the Changning block of the southern Sichuan Basin, the shale gas wells in the Upper Ordovician Wufeng Formation to Lower Silurian Longmaxi Formation (Wufeng–Longmaxi) demonstrate significant difference in flowback rate of fracturing fluid, given the same fracturing scale and production time. Based on the P90 curve of flowback rate with time, wells with high flowback rates in this area are categorized, and they are generally defined with the 100-day flowback rate of >40%, the 200-day flowback rate of >50%, and the first-year flowback rate >60%. Through comprehensive research on the geological characteristics of the Changning block, the geological factors that cause high gas well backflow rates have been identified. The results are obtained in four aspects. First, gas wells with high flowback rates generally show higher flow rates for smaller distances to the fault, and the NE faults formed during the late Yanshanian to early Himalayan and with fault throw >200 m have the greatest impact on the flowback rate. Second, plane heterogeneity of water saturation contributes to the high flowback rate of some wells. Third, the proportion of the Middle Ordovician Baota Formation in the horizontal section of wells is positively related to the flowback rate, and the drilling and fracturing of the Baota limestone with high water content can lead to high flowback rates of gas wells to some extent. Fourth, unidirectional linear natural fractures between wells can easily cause inter-well frac-hit, leading to high flowback rates of gas wells. In conclusion, the research results are helpful in predicting areas where high backflow rate gas wells may occur and developing corresponding drainage and production methods in advance.
Shale gas, as a clean and efficient energy source, has become a research hotspot in the energy field in recent years (Ehsan et al., 2019; Yang et al., 2023). The vigorous development of shale gas not only has significant economic and strategic value, but also has important significance in environmental protection (He et al., 2022a; He et al., 2022b). However, shale reservoirs are dense, and commercial development can only be achieved through large-scale volume fracturing. Hydraulic fracturing, as the most effective technology to improve shale gas production and recovery, has been increasingly applied (Khalil Khan et al., 2022; Xu et al., 2022). During hydraulic fracturing in shale gas reservoir, fracturing fluid of (2–5)× 104 m3 is injected into the reservoir and mostly flowed back to the surface (Tan et al., 2015; Wang et al., 2017; Ma and Xie, 2018; Li et al., 2022a; Li et al., 2023a). The backflow rate is generally calculated based on the ratio of the cumulative backflow volume of shale gas wells to the injected fracturing fluid volume. In recent years, with the continuous development of China’s basic geological theory of shale gas and the continuous progress of exploration and development technology, the Silurian Longmaxi Formation in the southern Sichuan Basin has successfully achieved large-scale and efficient development of shale gas (Ma et al., 2020). Through an analysis of production data from shale gas wells in the Changning block, southern Sichuan Basin, it is found that the post-fracturing production performance is negatively correlated with the flowback rate. In other words, given the same fracturing scale and production time, wells with high production rates generally demonstrate low flowback rates, while wells with high flowback rates generally exhibit poor production performance.
The flowback rate of shale gas wells is jointly controlled by geological and engineering factors. The sources and controlling factors of flowback fluids in shale gas wells with high flowback rates can hardly be determined due to the interplay of various geological factors (incl. faults, large-scale natural fractures, and high water-saturation limestone/claystone) and operational factors (hydraulic fracture geometry, fracturing scale, and reservoir stress sensitivity). Many scholars have used analytical and numerical models to clarify the relationship between factors such as fracture network models and flow patterns and the flowback rate of gas wells. Majid et al. constructed the basic diagnostic plots by using flowback data of three horizontal wells, which suggest three separate flowback regions, and then they established an analytical model to compare the pressure/production rate behaviors in the three regions and depicted the fracture network model (Abbasi et al., 2014; Wu et al., 2022a; Wu et al., 2022b). Cao et al. proposed a multiscale-multiphase simulation model and validated it using actual production data in the Barnett shale play, indicating that the dynamic behavior of the water phase in the fracture system significantly affects the flowback rates of shale gas wells (Cao et al., 2017). In an attempt to quantify post-stimulation flowback strategies, Crafton compared observed flowback production histories with multi-phase numerical simulation of flow in multiple fractures of various conductivities and then proposed potential stimulation strategies for the maximization of long-term productivity (Crafton, 2010; Xu and Gao, 2020). Liu et al. proposed a new method for a production dynamic data analysis system based on deconvolution to quantitatively evaluate the differences in fracture characteristics between backflow and production stages. The differences in fracture characteristics between the backflow and production stages can be effectively quantified and evaluated by interpreting the half-length and conductivity of fractures in the two stages through backflow and production data (Liu et al., 2023). Wang et al. (2022) studied the effects of displacement direction, formation pressure, interfacial tension, fracturing fluid viscosity, and proppant wettability on the flowback rate. The results indicate that the final flowback rate of the fracturing fluid decreases with the increase of formation pressure, and stable formation pressure is more conducive to the flowback of the fracturing fluid. The final flowback rate of fracturing fluid increases with the decrease of interfacial tension, but the upward trend gradually slows down and decreases with the increase of liquid viscosity (Wang et al., 2023). However, the causes for gas wells' high flowback rates have rarely been investigated, coupling with geological factors (Zhang et al., 2020; Li et al., 2020; Li et al., 2023b). This paper presents a geological analysis of shale gas wells with high flowback rates in the Changning block, southern Sichuan Basin, to determine the geological factors controlling the high flowback rate. The findings are helpful in predicting areas where high backflow rate gas wells may occur and developing corresponding drainage and production methods in advance.
The Changning block, situated in the low and steep deformation zones of the ancient depression and medium-uplift and the Loushan fold belt in the southern Sichuan Basin, is simple structurally, mainly including three structures: Jianwu syncline, Luochang syncline, and Changning anticline (Shi et al., 2019; Wang et al., 2019; Fan et al., 2020a). The Jianwu syncline, the core structure in the block, is broad and gentle and extends in nearly EW direction. The Changning anticline, the largest structure in the block, extends axially in the NWW direction, with a relatively gentle southwest wing and steep northeast wing. The Luochang syncline axially extends in the NE direction, with several large-scale faults developed (Figure 1).
The study area predominantly features three fault systems (NE, NW, and nearly EW) due to multi-phase tectonic activities. All of these faults are reverse faults, with small to medium faults in dominance, and they mostly diminish in the Silurian strata (Fan et al., 2020b; Li, 2021; Li et al., 2022b). These faults can be classified into four orders by throw: I (throw >300 m), II (throw of 200–300 m), III (throw of 100–200 m), and IV (throw <100 m). In the western part of the Changning block, where the reservoirs are buried at 3,500–4,500 m, there are mainly Order-I and Order-II faults, which segment the strata into fault blocks, resulting in complex preservation conditions under the control of faults. In the central and eastern parts of the block, where the reservoirs are buried at 2000–3,500 m, Order-III and Order-IV faults are predominant.
From surface to basement, the stratigraphic sequence in the Changning block comprises Jurassic, Triassic, Permian, Silurian, Ordovician, Cambrian, and Sinian. The Carboniferous and Devonian are missing, and the Silurian strata in the core of the Changning anticline have been eroded (Jiao, 2019; Zhang et al., 2019). Shale gas wells in the block are produced primarily from the Ordovician Wufeng Formation–Silurian Longmaxi Formation (Wufeng–Longmaxi). According to the block exploration data and previous research results, the Wufeng Formation has a 2–10 m thickness, increasing from east to west and from south to north. The Longmaxi Formation has a 250–350 m thickness, which increases from north to south with the distance to the denudation line and gradually stabilizes (Zhou et al., 2021).
Based on sedimentary cycles, the Longmaxi Formation can be divided into the Long 1 and Long 2 members. Sedimentary cycles are significantly different, with clear boundaries above and below the rhythmite where the greyish black shale at the bottom of Long 2 alternates with the underlying Long 1 black shale–grey silty shale. The Long 1 member represents a progradational inverse cycle that continues to regress, and it can be divided into two sub-members (Long 11 and Long 12) according to secondary cycles and lithological characteristics. Based on petrological, sedimentary structural, paleontological, and electrical properties, Long 11 can be subdivided into four layers, i.e., Long 111, Long 112, Long 113, and Long 114, from bottom to top (Zhao et al., 2015; Zhao et al., 2016; Xie et al., 2017; Wan et al., 2021).
In the Changning block, the interval from Wufeng Formation to Long 11 (Wufeng–Long 11) mainly consists of black carbonaceous shale, siliceous shale, and black shale with silty mudstone. The quality of shale reservoirs is sensitive to various factors, which can be roughly categorized into four groups: gas generation potential, reservoir properties, producibility, and gas-bearing property. For evaluation purposes, four static parameters are selected: total organic carbon (TOC) content, porosity, brittle mineral content, and gas content (Li et al., 2022b). According to core experiment data from coring wells in the Changning block, layers in the Wufeng–Long 11 interval exhibit the average TOC of 2.9%–5.5%, the average porosity of 4.9%–5.8%, the average brittle mineral content of 59.2%–77.8%, and the average gas content of 5.1–7.5 m3/t (Table 1). Overall, the quality of reservoirs in the Changning block is good, especially those in Long 111 and the Wufeng Formation.
TABLE 1. Experimental data of brittle mineral content, TOC, porosity, and gas content for layers in Wufeng–Long 11, Changning block.
Shale reservoirs are tight and can be commercially exploited only through large-scale hydraulic fracturing. Rock mechanics and in-situ stress are primary indicators for assessing shale fracturability (Zhao et al., 2015; Cai, 2020; Li, 2022). Regarding rock mechanics, the Changning block demonstrates high Young’s modulus in Long 111 and Long 112 and low Poisson’s ratio in all layers (Table 2). In terms of in-situ stress, the maximum horizontal principal stress is 57–87.9 MPa, the minimum horizontal principal stress is 44–72.5 MPa, and the horizontal stress difference is 7.5–16.7 MPa, according to the in-situ stress experiment for typical wells in the Changning block (Figure 2).
TABLE 2. Experimental data of Young’s modulus and Poisson’s ratio for layers in Wufeng–Long 11, Changning block.
According to the statistics of production data from over 400 shale gas wells in the Changning block, it is found that wells producing for over 3 years exhibit the flowback rate of 45%–65%, while some wells have the flowback rate of >70% (especially Well B1, which achieves a flowback rate up to 117.07%). To determine wells with high flowback rates in the Changning block and identify their characteristics, the variation of flowback rate with time for shale gas wells in the Changning block was investigated, and the wells with high flowback rate were identified by the P90 curve of flowback rate. Finally, 17 wells, the top 10% of examined wells for flowback rate, are determined as wells with high flowback rate (Figure 3; Table 3).
The 60-day, 100-day, 200-day, and first-year (330 days) flowback rates of the 17 wells with high flowback rates were compared with the average flowback rate of all producing wells in the Changning block. It is found that the differences increase with the production time, and the shale gas wells with high flowback rates are generally characterized by the 100-day flowback rate of >40%, the 200-day flowback rate of >50%, and the first-year flowback rate of >60% (Figure 4). These conditions are the criteria for identifying gas wells with high flowback rates in the Changning block.
FIGURE 4. Flowback rate of gas wells with high flowback rate in Changning block at different production time.
Figure 5 illustrates the relationship between the flowback rate and estimated ultimate recovery (EUR, converted for 1500-m horizontal section) of the 17 wells with high flowback rate. It is seen that EUR is negatively correlated with the flowback rate. This means that, in the Changning block, gas wells with higher flowback rates exhibit poorer production performance. Moreover, the wells with a flowback rate of >100% reveal the EUR not exceeding 1.0 × 108 m3.
Tectonic movements and the resulting faults greatly impact the high flowback rates of shale gas wells. The faults in the study area are classified according to their phases (orientations) and throws, and then their impacts on the high flowback rates of shale gas wells are clarified.
Previous studies have shown a high consistency in their findings regarding the fault development in the Changning block, from surface fracture geological surveys, underground fracture characterization, inclusion of homogeneous temperature measurements, rock acoustic emission experiments, burial history analysis, and Wufeng-Longmaxi fault structure analysis. Their findings suggest that the Changning region has experienced at least three phases of tectonic movements since the Late Ordovician, forming fractures in three periods. Specifically, the first phase of the tectonic movement Middle–Late Yanshanian) induced nearly EW faults; the second phase of the tectonic movement (Late Yanshanian–Early Himalayan) resulted in NE faults; and the third phase of the tectonic movement (Middle Himalayan–the present) created NW faults (Nie et al., 2019; Dong et al., 2020; Zhao et al., 2020; Fan et al., 2022).
The larger the fault throw, the more extensive the contact between shale layers and other strata, resulting in wider fault zones, reduced shale content, and lower fault sealing capacity. This allows for the connectivity between shale layers and other formations. Additionally, large faults can act as pathways for the formation of water migration. They communicate shale layers with high water-content formations, thereby contributing to the phenomenon of high flowback rates of gas wells. A fault throw exceeding the thickness of the regional seal can more likely induce vertical faulting (Li et al., 2006; Huang, 2012; Lan et al., 2021; Zhang et al., 2022). In the western and southern parts of the Changning block, Order-I and Order-II faults are mainly developed, with throw >200 m, and mostly in NE strike. Central and eastern parts primarily have Order-III and Order-IV faults.
According to the statistics of faults near the 17 wells with high flowback rates in the Changning block, it is found that 13 wells correspond to faults nearby. Among the 13 wells, 11 wells (84.6%) are situated near the NE faults formed in the second phase of tectonic movement and with throw >200 m, while only 2 wells (15.4%) are positioned near the NW faults formed in the third phase of tectonic movement (Figure 6; Table 4). It can be preliminarily concluded that the NE faults formed in the second phase of tectonic movement and with throw >200 m have the greatest impact on high flowback rates of gas wells. Such impact is further analyzed.
The NE faults formed in the second phase of tectonic movement are mostly distributed in the western and southern parts of the Changning block. These faults often cut through the Wufeng Formation–Permian, with a throw of >200 m. They penetrate the roof and floor of shale layers to communicate the formation waters in different horizons, which may lead to high flowback rates of gas wells. The current flowback rates of gas wells with high flowback rates cannot be used directly for comparison because of different production time. In this study, a regression analysis was conducted on the nearest distance from shale gas wells to NE faults with throw >200 m based on the 200-day flowback rate (Figure 7). It is found that the distance to fault is negatively correlated with the flowback rate. This indicates that the NE faults formed in the second phase of tectonic movement and with throw >200 m have a certain impact on high flowback rates of shale gas wells—the closer the shale gas well with a high flowback rate is to the fault, the higher the flowback rate.
FIGURE 7. Distance from well with high flowback rate to NE fault (throw >200 m) vs. 200-day flowback rate.
The study also reveals that the correlation between flowback rate and distance to the NE fault becomes more pronounced when the distance from well with high flowback rate to the NE fault is less than 800 m, but weaker when the distance is greater than 800 m. Thus, it is considered that the area within 800 m of the fault has the potential for a high flowback rate, and 800 m is defined as a safe distance for shale gas wells to avoid the influence of faults. To avoid high flowback rates due to faults, deploying gas wells no less than 800 m to the NE faults formed in the late Yanshanian–early Himalayan in the Changning block is recommended.
Water saturation is a crucial parameter that governs the sustained production from shale gas reservoirs, while high initial water content in shale reservoirs may lead to the occurrence of high flowback rates of shale gas wells (Wang et al., 2020; Zhao et al., 2021). Based on the experimental data of water saturation in cores from coring wells in the Changning block, the contour map of water saturation in the Wufeng–Long 11 interval was plotted (Figure 8). It can be seen that the water saturation in the Changning block ranges between 25% and 45%, and there are two areas with low water saturation (<30% generally), which are located near Order-I and Order-II faults in the eastern and western parts of the Changning block.
It is also observed that the gas wells with high flowback rates in the Changning block universally rest in areas with high water saturation. Among the 17 wells with a high flowback rate, 12 wells (70.6%) exhibit a water saturation of >35%. In addition to faults, the plane heterogeneity of water saturation in Wufeng–Long 11 also contributes to a high flowback rate. Neglecting the engineering factors, shale gas wells with high flowback rates are more likely to appear in areas with high water saturation.
The Wufeng–Longmaxi shale in the Changning block is tight, with the tight limestone of the Baota Formation at the bottom, approximately 2–10 m to the major producing layer (Long 111). Select 30 core samples from 5 wells in different horizons of Changning block, and conduct liquid saturation experiments and X-ray diffraction experiments to measure the water saturation and clay mineral content of the core. The results show that the Baota Formation has higher water saturation than the Wufeng–Long 11 interval (Table 5). Among the 17 wells with high flowback rates, 5 wells have horizontal well trajectories penetrating the Baota Formation (Table 6). Further analysis suggests a positive correlation between the proportion of Baota Formation in the horizontal section of the well with a high flowback rate and the flowback rate (Figure 9). In other words, a higher proportion of Baota Formation in the horizontal section corresponds to a higher flowback rate. It is thus inferred that the drilling and fracturing of the Baota limestone with high water content in the horizontal section of the shale gas well will induce a high flowback rate.
TABLE 6. Statistics of horizons encountered by gas wells with high flowback rate in Changning block (as of August 2022).
FIGURE 9. Flowback rate vs. the proportion of Baota Formation in the horizontal section of gas well with a high flowback rate.
The Changning block has undergone multi-phase tectonic movements, such as the Caledonian, Hercynian, Indosinian, and Himalayan, resulting in widespread natural fractures. Natural fractures are essential storage space for shale gas, and their shapes, characteristics, and patterns significantly influence shale gas flow and the formation water. Compared to fractures, natural fractures are often more developed near them but on a smaller scale (Wang et al., 2022; Li et al., 2022c). Utilizing a combination of pre-stack and post-stack seismic fracture prediction techniques, through manual selection and intelligent fusion, the development and spatial distribution of multi-scale fractures were finely depicted. Natural fractures in the Changning block were predicted based on seismic curvature volumes, ant tracking techniques, and the maximum likelihood method. Depending upon the fracture shape, two styles of fractures are identified: polydirectional reticular fractures and unidirectional linear fractures (Figure 10). In terms of fracture density, fractures are well-developed near faults. Regarding the relationship between fracture scale and structural features, NE-striking large-scale fractures prevail in the structural zones, while small-scale fractures dominate in the synclinal zones. Most fractures share the same orientation as the major faults, but multiple polydirectional reticular fractures are hosted within the synclines.
FIGURE 10. Distribution of natural fractures in Wufeng Formation in Changning block predicted by seismic data.
Reticular fractures are microfractures created in multiple directions due to sedimentation and diagenesis. They are mainly distributed within the broad and gentle synclines in the central and eastern parts of the Changning block, often associated with Order-Ⅲ and Order-IV faults. The presence of reticular natural fractures around the wellbore is conducive to forming a complex fracture network after hydraulic fracturing, increasing the contact area between the fractures and the matrix. Comparative analysis of shale gas well production in the Changning block reveals that most high-production wells are located in areas where reticular fractures are well-developed. Therefore, it can be inferred that shale gas wells achieve better production performance in areas with well-developed reticular fractures, and wells with high flowback rates rarely occur.
Unidirectional linear fractures refer to fractures formed in a single direction due to tectonism. They are mainly distributed in the narrow slopes and steep anticlines in the western and northern parts of the Changning block, often controlled by Order-I and Order-II faults (Table 7). When unidirectional linear fractures exist near the shale gas wellbore, the fracturing fluid can easily leak along these natural fractures during fracturing, disabling the effective stimulation of the reservoir. The fracturing fluid also propagates along the unidirectional linear fractures, which may lead to downhole complexities such as frac-hit and casing deformation (Zhao et al., 2018; He et al., 2020; Li, 2023; Zhu et al., 2023).
Some wells demonstrate high flowback rates as a result of frac-hit during neighboring well fracturing operations, due to the presence of unidirectional linear fractures near the wellbores. According to the tracer and microseismic monitoring for shale gas wells in the Changning block, the high flowback rates of 5 wells (B3, B5, B8, B11, and B16) can be attributed to the frac-hit during neighboring well fracturing operations. Specifically, Well B3 has the highest flowback rate—up to 106.35%. Here, this well is an example to analyze the impact of unidirectional linear fractures on high flowback rates of gas wells.
Well B3 exhibits a 100-day flowback rate of 38.34% and a 200-day flowback rate of 46.21%, which do not meet the general characteristics of gas wells with a high flowback rate in the Changning block (i.e., the 100-day flowback rate >40%, the 200-day flowback rate >50%, and the first-year flowback rate >60%). However, its current flowback rate reaches 106.35% due to frac-hit during later neighboring well fracturing, confirming it as a well with a high flowback rate. Figure 11 shows the site and fracture superimposition of Well B3. It can be seen that, during the re-fracturing of Well C1, natural fractures communicate it with Well C2 located 200 m away in the west, making the latter water-invaded. Meanwhile, a unidirectional linear fracture exists between Well C2 and Well B3 located 500 m away in the east, leading to a frac-hit between them. As shown by the drainage test curve of Well B3 (Figure 12), when Well C1 is re-fractured, Well B3 to the north on the same platform displays a dramatic increase in drainage volume, confirming that the presence of unidirectional linear fracture between wells can lead to frac-hit, which will enable a communication with one or more wells. Thus, the unidirectional linear fracture contributes to the high flowback rate of a shale gas well. It is recommended to deploy wells with larger spacing in areas where unidirectional linear fractures exist to avoid a high flowback rate of well due to frac-hit when fracturing fluid propagates along linear natural fractures.
This paper establishes a geological control factor analysis method for the high flowback rate of shale gas wells, analyzing geological characteristics such as faults, reservoir water saturation, and natural fractures and clarifying the geological control factors for the high flowback rate of shale gas wells in Changning Block.
(1) For the gas wells with a high flowback rate in the Changning block, the closer the well is to the fault, the higher the flowback rate. The NE faults formed during the late Yanshanian to early Himalayan and with throw >200 m have the greatest impact on the flowback rate. The area within 800 m to such faults has the potential for a high flowback rate.
(2) The plane heterogeneity of water saturation in Wufeng–Long 11 contributes to the high flowback rate of the gas well. Neglecting the engineering factors, shale gas wells with high flowback rates are more likely to appear in areas with high water saturation.
(3) The proportion of Baota Formation in the horizontal section of a well with a high flowback rate is positively correlated with the flowback rate. The drilling and fracturing of the Baota limestone with high water content in the horizontal section can lead to a high flowback rate of the gas well.
(4) Microfractures between wells are conducive to reservoir fracturing without inter-well frac-hit. However, unidirectional linear fractures between wells can easily induce frac-hit, resulting in high flowback rates of gas wells.
The original contributions presented in the study are included in the article/Supplementary Material, further inquiries can be directed to the corresponding authors.
YL: Data curation, Formal Analysis, Resources, Validation, Visualization, Writing–original draft, Writing–review and editing. SZ: Conceptualization, Data curation, Formal Analysis, Supervision, Writing–original draft, Writing–review and editing. XY: Methodology, Project administration, Resources, Writing–review and editing. BL: Methodology, Resources, Visualization, Writing–original draft, Writing–review and editing. JZ: Investigation, Methodology, Resources, Software, Supervision, Writing–original draft. CJ: Data curation, Funding acquisition, Methodology, Resources, Supervision, Writing–review and editing. SH: Data curation, Methodology, Project administration, Validation, Writing–review and editing. TW: Data curation, Formal Analysis, Investigation, Writing–review and editing. CQ: Formal Analysis, Investigation, Software, Visualization, Writing–review and editing.
The author(s) declare that financial support was received for the research, authorship, and/or publication of this article. This study was supported by the Key Technology Research and Field Test of Efficient Exploitation of Deep Shale Gas—Field Test of Optimization Technology for Deep Shale Gas Development (Task 2) funded by China National Petroleum Corporation Technology R&D Project (2019F-31-02).
Authors YL, SZ, XY, BL, JZ, SH, TW, and CQ were employed by PetroChina Southwest Oil and Gas Field Company.
Author CJ was employed by Sichuan Changning Natural Gas Development Co., Ltd.
All claims expressed in this article are solely those of the authors and do not necessarily represent those of their affiliated organizations, or those of the publisher, the editors and the reviewers. Any product that may be evaluated in this article, or claim that may be made by its manufacturer, is not guaranteed or endorsed by the publisher.
Abbasi, M. A., Ezulike, D. O., Dehghanpour, H., and Hawkes, R. V. (2014). A comparative study of flowback rate and pressure transient behavior in multifractured horizontal wells completed in tight gas and oil reservoirs. J. Nat. Gas. Sci. Eng. 17, 82–93. doi:10.1016/j.jngse.2013.12.007
Cai, M. F. (2020). Key theories and technologies for surrounding rock stability and ground control in deep mining. J. Min. Strata Control Eng. 2 (3), 033037. doi:10.13532/j.jmsce.cn10-1638/td.20200506.001
Cao, P., Liu, J., and Leong, Y. K. (2017). A multiscale-multiphase simulation model for the evaluation of shale gas recovery coupled the effect of water flowback. Fuel 199, 191–205. doi:10.1016/j.fuel.2017.02.078
Crafton, J. W. (2010). “Flowback performance in intensely naturally fractured shale gas reservoirs,” in SPE Unconventional Gas Conference. SPE 131785.
Dong, S., Zeng, L., Lyu, W., Xia, D., Liu, G., Wu, Y., et al. (2020). Fracture identification and evaluation using conventional logs in tight sandstones: a case study in the Ordos Basin, China. Energy Geosci. 1 (3-4), 115–123. doi:10.1016/j.engeos.2020.06.003
Ehsan, M., Gu, H., Ahmad, Z., Akhtar, M. M., and Abbasi, S. S. (2019). A modified approach for volumetric evaluation of Shaly Sand Formations from conventional well logs: a case study from the Talhar Shale, Pakistan. Arab. J. Sci. Eng. 44, 417–428. doi:10.1007/s13369-018-3476-8
Fan, C. H., Li, H., Qin, Q. R., He, S., and Zhong, C. (2020a). Geological conditions and exploration potential of shale gas reservoir in Wufeng and Longmaxi Formation of southeastern Sichuan Basin, China. J. Petrol. Sci. Eng. 191, 107138. doi:10.1016/j.petrol.2020.107138
Fan, C. H., Li, H., Zhao, S. X., Qin, Q. R., Fan, Y., Wu, J. F., et al. (2020b). Formation stages and evolution patterns of structural fractures in marine shale: case study of the Lower Silurian Longmaxi Formation in the Changning area of the southern Sichuan Basin, China. Energy Fuel 34 (8), 9524–9539. doi:10.1021/acs.energyfuels.0c01748
Fan, C. H., Xie, H. B., Li, H., Zhao, S. X., Shi, X. C., Liu, J. F., et al. (2022). Complicated fault characterization and its influence on shale gas preservation in the southern margin of the Sichuan Basin, China. Lithosphere 2022 (S12), 8035106. doi:10.2113/2022/8035106
He, L., Yuan, C. M., and Gong, W. (2020). Influencing factors and preventing measures of intra-well frac hit in shale gas. Res. Eval. Dev. 59 (5), 69–75. doi:10.13809/j.cnki.cn32-1825/te.2020.05.009
He, S., Qin, Q. R., Li, H., and Wang, S. L. (2022b). Deformation differences in complex structural areas in the southern Sichuan Basin and its influence on shale gas preservation: a case study of Changning and Luzhou area. Front. Earth Sci. 9, 818155. doi:10.3389/feart.2021.818155
He, S., Qin, Q. R., Li, H., and Zhao, S. X. (2022a). Deformation differences in complex structural areas in the southern Sichuan Basin and its influence on shale gas preservation: a case study of changning and luzhou areas. Front. Earth Sci. 9, 818543. doi:10.3389/feart.2021.818534
Huang, J. L. (2012). Shale gas accumulation conditions and favorable zones of Silurian Longmaxi Formation in south Sichuan Basin, China. J. China Coal Soc. 37 (5), 782–787. doi:10.13225/j.cnki.jccs.2012.05.005
Jiao, F. Z. (2019). Theoretical insights, core technologies and practices concerning "volume development" of shale gas in China. Nat. Gas. Ind. 39 (5), 525–538. doi:10.1016/j.ngib.2019.05.001
Khalil Khan, H., Ehsan, M., Ali, A., Amer, M. A., Aziz, H., Khan, A., et al. (2022). Source rock geochemical assessment and estimation of TOC using well logs and geochemical data of Talhar Shale, Southern Indus Basin, Pakistan. Front. Earth Sci. 10, 969936. doi:10.3389/feart.2022.969936
Lan, S. R., Song, D. Z., and Li, Z. L. (2021). Experimental study on acoustic emission characteristics of fault slip process based on damage factor. J. Min. Strata Control Eng. 3 (3), 033024. doi:10.13532/j.jmsce.cn10-1638/td.20210510.002
Li, G. Q., and Cao, D. Y. (2006). Analysis of uncertainty factors of fault sealing. J. Oil Gas. Tech. 28 (2), 31–33.
Li, H. (2022). Research progress on evaluation methods and factors influencing shale brittleness: a review. Energy Rep. 8, 4344–4358. doi:10.1016/j.egyr.2022.03.120
Li, H. (2023). Coordinated development of shale gas benefit exploitation and ecological environmental conservation in China: a mini review. Front. Ecol. Evol. 11, 1232395. doi:10.3389/fevo.2023.1232395
Li, H., Zhou, J. L., Mou, X. Y., Guo, H. X., Wang, X. X., An, H. Y., et al. (2022b). Pore structure and fractal characteristics of the marine shale of the Longmaxi Formation in the changning area, southern Sichuan Basin, China. Front. Earth Sci. 10, 1018274. doi:10.3389/feart.2022.1018274
Li, J. J., Qin, Q. R., Li, H., and Wan, Y. F. (2022c). Numerical simulation of the stress field and fault sealing of complex fault combinations in Changning area, Southern Sichuan Basin, China. Energy Sci. Eng. 10, 278–291. doi:10.1002/ese3.1044
Li, J., Li, H., Yang, C., Ren, X. H., and Li, Y. D. (2023a). Geological characteristics of deep shale gas and their effects on shale fracability in the Wufeng–Longmaxi Formations of the southern Sichuan Basin, China. Lithosphere 2023 (1), 4936993. doi:10.2113/2023/4936993
Li, J., Li, H., Yang, C., Wu, Y. J., Gao, Z., and Jiang, S. L. (2022a). Geological characteristics and controlling factors of deep shale gas enrichment of the Wufeng-Longmaxi Formation in the southern Sichuan Basin, China. Lithosphere 2022 (S12), 4737801. doi:10.2113/2022/4737801
Li, Y., Wang, R., Zhao, Q. M., and Xue, Z. J. (2023b). Technological advancement and industrialization path of Sinopec in carbon capture, utilization and storage, China. Energy Geosci., 100107. doi:10.1016/j.engeos.2022.04.003
Li, Y., Zhou, D., Wang, W., Jiang, T., and Xue, Z. (2020). Development of unconventional gas and technologies adopted in China. Energy Geosci. 1 (1-2), 55–68. doi:10.1016/j.engeos.2020.04.004
Li, Y. Z. (2021). Mechanics and fracturing techniques of deep shale from the Sichuan Basin, SW China. Energy Geosci. 2 (1), 1–9. doi:10.1016/j.engeos.2020.06.002
Liu, W. C., Qiao, C. C., and Wang, P. (2023). Study on the differences in fracturing fracture characteristics between shale gas well fracturing fluid flowback and production stage. Chin. J. Theor. Appl. Mech. 55 (6), 1382–1393. doi:10.6052/0459-1879-23-031
Ma, X. H., and Xie, J. (2018). The progress and prospects of shale gas exploration and exploitation in southern Sichuan Basin. Petrol. explor. Dev. 45 (1), 161–169. doi:10.1016/S1876-3804(18)30018-1
Ma, X. H., Xie, J., Yong, R., and Zhu, Y. Q. (2020). Geological characteristics and high production control factors of shale gas reservoirs in Silurian Longmaxi Formation, southern Sichuan Basin, SW China. Petrol. expl. Dev. 47 (5), 901–915. doi:10.1016/s1876-3804(20)60105-7
Nie, H. K., Wang, H., and He, Z. L. (2019). Formation mechanism,distribution and exploration prospect of normal pressure shale gas reservoir:a case study of Wufeng Formation-Longmaxi Formation in Sichuan Basin and its periphery. Acta Petrol. Sin. 40 (2), 131–143. doi:10.7623/syxb201902001
Shi, X. W., Tong, Y. M., and Liu, W. P. (2019). Analysis of seismic-scale fracture system of shale reservoir and its petroleum significance: a case study of well Ning 201 area of Changning Block, Sichuan Basin. Mar. Orig. Petrol. Geol. 24 (4), 97–106. doi:10.3969/j.issn.1672-9854.2019.04.009
Tan, L. Y., Xu, Y., Li, D. H., Zeng, C. L., Wang, F., Zeng, X. L., et al. (2015). Research on the main control factors and models for shale gas accumulation: a case study of Wufeng and Longmaxi Formations in Southeastern Chongqing. Bull. Geol. Sci. Tech. 34 (3), 126–132.
Wan, Y. F., Qin, Q. R., and Fan, Y. (2021). Development characteristics of shale fractures in Longmaxi Formation of Changning anticline and the stage analysis. Spec. Oil Gas. Res. 28 (1), 59–66. doi:10.3969/j.issn.1006-6535.2021.01.008
Wang, G. Q., Xiao, Y. J., and Zhao, H. (2019). Application of microseismic monitoring technology to re-fracturing of shale gas horizontal wells. Geol. Explor. 55 (5), 222–228. doi:10.12134/j.dzykt.2019.05.022
Wang, J., Tan, X. F., Tian, J. C., Luo, C., Ran, T., Chen, Q., et al. (2017). Constraints of hydrocarbon migration in Longmaxi shale in Sichuan Basin on shale gas accumulation. Petrol. Geol. Exp. 39 (6), 755–762. doi:10.11781/sysydz201706755
Wang, J. B., Feng, M. G., and Yan, W. (2020). Influential factors and calculation methods for water saturation in shale gas: examples from Wufeng Formation-Longmaxi Formation, Jiaoshiba block. Nat. Gas. Techn. Econ. 14 (6), 21–28. doi:10.3969/j.issn.2095-1132.2020.06.004
Wang, S. L., Li, H., Lin, L. F., and Yin, S. (2022). Development characteristics and finite element simulation of fractures in tight oil sandstone reservoirs of Yanchang Formation in western Ordos Basin. Front. Earth Sci. 9, 823855. doi:10.3389/feart.2021.823855
Wang, Z., Hao, C., Jin, H., Cui, J., Wu, X., Bo, D., et al. (2023). Geochemical characteristics and hydrocarbon generation potential of main source rocks in the Upper Triassic Xujiahe Formation, Sichuan Basin, China. Front. Earth Sci. 11, 1233959. doi:10.3389/feart.2023.1233959
Wu, Z. H., Tang, M. T., Zuo, Y. J., Lou, Y. L., Wang, W. T., Liu, H., et al. (2022b). Acoustic emission-based numerical simulation of tectonic stress field for tectoclase prediction in shale reservoirs of the northern Guizhou area, China. Energy Geosci. 3 (4), 436–443. doi:10.1016/j.engeos.2021.10.005
Wu, Z. W., Cui, C. Z., Jia, P. F., Wang, Z., and Sui, Y. F. (2022a). Advances and challenges in hydraulic fracturing of tight reservoirs: a critical review. Energy Geosci. 3 (4), 427–435. doi:10.1016/j.engeos.2021.08.002
Xie, J., Zhao, S. X., and Shi, X. W. (2017). Main geological factors controlling high production of horizontal shale gas wells in the Sichuan Basin. Nat. Gas. Ind. 37 (7), 1–12. doi:10.3787/j.issn.1000-0976.2017.07.001
Xu, N. Z., and Gao, C. (2020). Study on the special rules of surface subsidence affected by normal faults. J. Min. Strata. Control Eng. 2 (1), 011007. doi:10.13532/j.jmsce.cn10-1638/td.2020.01.011
Xu, Z., Li, X., Li, J., Xue, Y., Jiang, S., Liu, L., et al. (2022). Characteristics of source rocks and genetic origins of natural gas in deep formations, gudian depression, songliao basin, NE China. ACS Earth Space Chem. 6 (7), 1750–1771. doi:10.1021/acsearthspacechem.2c00065
Yang, L., Wang, H., Xu, H., Guo, D., and Li, M. (2023). Experimental study on characteristics of water imbibition and ion diffusion in shale reservoirs. Geoenergy Sci. Eng. 229, 212167. doi:10.1016/j.geoen.2023.212167
Zhang, C., Song, W. D., Fu, J. X., Li, Y., and Zhang, K. C. (2020). Technology for roadway management of fractured rock masses in a submarine gold mine. J. Min. Strata Control Eng. 2 (3), 033039. doi:10.13532/j.jmsce.cn10-1638/td.20200313.002
Zhang, C. L., Zhang, J., and Li, W. G. (2019). Deep shale reservoir characteristics and exploration potential of Wufeng-Longmaxi Formations in Dazu area, western Chongqing. Nat. Gas. Geosci. 30 (12), 122–132. doi:10.11764/j.issn.1672-1926.2019.08.007
Zhang, P. S., Xu, D. Q., and Fu, X. (2022). Evaluation of hydraulic conductivity based on fault confinement studies. J. Min. Strata Control Eng. 4 (2), 023033. doi:10.13532/j.jmsce.cn10-1638/td.20211215.001
Zhao, J. Z., Ren, L., Shen, C., and Li, Y. (2018). Latest research progresses in network fracturing theories and technologies for shale gas reservoirs. Nat. Gas. Ind. 38 (3), 533–546. doi:10.1016/j.ngib.2018.03.007
Zhao, J. Z., Xu, W. J., and Li, Y. M. (2015). A new method for fracability evaluation of shale-gas reservoirs. Nat. Gas. Geosci. 26 (6), 1165–1172. doi:10.11764/j.issn.1672-1926.2015.06.1165
Zhao, K. K., Jiang, P. F., and Feng, Y. J. (2021). Investigation of the characteristics of hydraulic fracture initiation by using maximum tangential stress criterion. J. Min. Strata Control Eng. 3 (2), 023520. doi:10.13532/j.jmsce.cn10-1638/td.20201217.001
Zhao, S. X., Yang, Y. M., and Zhang, J. (2016). Micro-layers division and fine reservoirs contrast of lower silurian Longmaxi Formation shale, Sichuan Basin, SW China. Nat. Gas. Geosci. 27 (3), 470–487. doi:10.11764/j.issn.1672-1926.2016.03.0470
Zhao, Z. H., Wu, K. D., Fan, Y., Guo, J. C., Zeng, B., and Yue, W. H. (2020). An optimization model for conductivity of hydraulic fracture networks in the Longmaxi shale, Sichuan basin, Southwest China. Energy Geosci. 1 (1–2), 47–54. doi:10.1016/j.engeos.2020.05.001
Zhou, H., Chen, L., Li, X. X., Chen, X., Wang, W. J., Yang, L., et al. (2021). Difference analysis of shale reservoirs of Wufeng Formation and Longmaxi Formation in changning area, southern sichuan. Fault-Block Oil Gas. Field 28 (3), 289–294. doi:10.6056/dkyqt202103001
Zhu, B. Y., Meng, J. H., Pan, R. F., Hu, H. Y., Song, C., Zhu, Z. P., et al. (2023). New insights into the evaluation criteria for high-quality deep marine shale gas reservoirs in the Longmaxi formation: evidence from organic matter pore development characteristics. Front. Ecol. Evol. 11, 1138991. doi:10.3389/fevo.2023.1138991
Keywords: Changning block, shale gas, high flowback rate, FAULT, water saturation, natural fracture
Citation: Liu Y, Zhao S, Yang X, Li B, Zhang J, Ji C, Huang S, Wu T and Qu C (2024) Geological factors controlling high flowback rates of shale gas wells in the Changning area of the southern Sichuan Basin, China. Front. Earth Sci. 11:1284996. doi: 10.3389/feart.2023.1284996
Received: 29 August 2023; Accepted: 21 December 2023;
Published: 09 January 2024.
Edited by:
Shuai Yin, Xi’an Shiyou University, ChinaReviewed by:
Lei Gong, Northeast Petroleum University, ChinaCopyright © 2024 Liu, Zhao, Yang, Li, Zhang, Ji, Huang, Wu and Qu. This is an open-access article distributed under the terms of the Creative Commons Attribution License (CC BY). The use, distribution or reproduction in other forums is permitted, provided the original author(s) and the copyright owner(s) are credited and that the original publication in this journal is cited, in accordance with accepted academic practice. No use, distribution or reproduction is permitted which does not comply with these terms.
*Correspondence: Shengxian Zhao, emhhb3NoZW5neGlhbkBwZXRyb2NoaW5hLmNvbS5jbg==; Bo Li, bGliXzIwMjFAcGV0cm9jaGluYS5jb20uY24=
Disclaimer: All claims expressed in this article are solely those of the authors and do not necessarily represent those of their affiliated organizations, or those of the publisher, the editors and the reviewers. Any product that may be evaluated in this article or claim that may be made by its manufacturer is not guaranteed or endorsed by the publisher.
Research integrity at Frontiers
Learn more about the work of our research integrity team to safeguard the quality of each article we publish.