- 1Chinese Academy of Geological Sciences, Beijing, China
- 2Urban Construction School, Beijing City University, Beijing, China
In this study, a series of unconfined compressive strength tests were conducted to investigate the effect of calcium-based flocculants on the strength and deformation properties of slurry. The test results indicated that the presence of calcium-based flocculants [Ca(OH)2 or CaCl2] significantly enhanced the unconfined compressive strength (qu) of soil. A non-linear relationship was observed between qu and CaCl2 content, revealing that the maximum value of qu is obtained at a CaCl2 concentration of 24.8%. qu exhibited a high increase rate at early curing time in the presence of Ca(OH)2, with a lower increase rate after a longer curing time and high Ca(OH)2 content. The deformation modulus E50 showed an increasing trend with increasing CaCl2 content at 3 and 7 d, followed by a decreasing trend with increasing CaCl2 content at 14 and 28 d. However, the failure strain εf for CaCl2-treated soil ranges from 2.4% to 4.8%, showing no relation with qu. A significant increase in E50 for Ca(OH)2-treated soil in the early curing stages (3 and 7 d) was observed because of the increase in qu. After 14 d of curing, E50 tended to decrease with increasing Ca(OH)2 content. A positive relationship between the degree of vacuum and qu in the presence of calcium-based flocculants was proposed, indicating that a higher qu of treated soil leads to a lower water content after vacuum treatment for the same preloading period, emphasizing that the vacuum treatment efficiency increases with an increase in the qu of calcium-based flocculant-treated soil.
1 Introduction
Flooding is a natural disaster that causes massive economic losses and human casualties annually, particularly in recent decades, owing to global climate change. Therefore, to prevent flood disasters and keep river channels unobstructed, dredging projects have been implemented in most urban cities around the Yangtze and Yellow River regions, generating thousands of dredged soils with high initial water contents annually in China (Zeng et al., 2015, 2021; Bian et al., 2016, 2022a; Liu et al., 2018). These large dredged soils are placed inside a slurry pond and left for several years because of their low permeability and engineering properties (Wang et al., 2019; Min et al., 2020; Xu et al., 2020). Owing to its low cost, large treatment area, and high efficiency, the vacuum-preloading method is often regarded as a suitable technique for accelerating the aforementioned process and reusing dredged materials (Chai et al., 2010; Indraratna et al., 2012; Cai et al., 2019).
Generally, the vacuum-preloading method requires a drainage channel, such as prefabricated vertical drains (PVDs), to allow for vacuum pressure transmission through the PVDs and for water flow out of the slurry. However, fine-grained particles may cause clogging of the PVDs, thereby reducing the transmission rate of the vacuum pressure (Chu et al., 2006; Wang et al., 2016; Cao et al., 2019). Recently, a combination of chemical treatment and vacuum preloading has been introduced to increase the treatment efficiency (Wu et al., 2015; Wang et al., 2017). When additional flocculants were added to the slurry, the flocculation effect triggered a large net-frame structure in the soil, resulting in an increase in the permeability and settlement rate of the slurry, which in turn led to a significant increase in the dewatering capacity of the slurry and enhancement of the effectiveness of vacuum preloading (Mallela et al., 2017; Wang et al., 2019; Moghal et al., 2020). In light of this mechanism, numerous researchers have proposed different chemical agents, such as lignite, polyacrylamide (PAM) FeCl3, or CaO, as flocculants to improve the vacuum preloading efficiency (Mallela et al., 2017; Gao et al., 2019; Wang et al., 2019; He et al., 2020). The change in microstructure due to these chemical agents led to the formation of a flocculated fabric and increased soil strength, which benefited slurry movement during vacuum preloading. However, some lime-based (CaO) agents may induce highly brittle sandy slurry structures, leading to unfavorable engineering properties (Wang et al., 2019). Therefore, investigating the effect of flocculants on slurry strength and vacuum preloading efficiency is important.
In this study, to achieve more optimized results than the vacuum preloading method, two calcium-based flocculants, including Ca(OH)2 and CaCl2, were introduced to improve the slurry strength. Subsequently, the effect of the strength increase on the vacuum-preloading efficiency was analyzed and compared with that reported in previous studies (Yan et al., 2020).
2 Materials and experimental methods
2.1 Materials
BB slurry was collected from a dredging project in the Huaihe River, Eastern China. The liquid and plastic limits in BB slurry were 58% and 26%, respectively. This slurry is classified as high-plasticity clay (CH) according to the Unified Soil Classification System (ASTM D2487). The specific gravity of the soil particles was 2.70 Mg/m3.
Calcium hydroxide (Ca(OH)2) and calcium chloride (CaCl2), manufactured by the Damao Chemical Reagent Factory, Tianjin, China, were used as calcium-based flocculants. Their basic properties have been outlined by Yan et al. (2020) and their basic compositions are summarized in Table 1.

TABLE 1. Basic properties of flocculants [after Yan et al. (2020)].
2.2 Experimental methods
The test programs for the two calcium-based flocculant-treated soils are listed in Table 2. To obtain a homogeneous slurry, a soil slurry with an initial water content that was double the liquid limit was prepared by mixing raw soil with a predetermined amount of water. Then the calcium chloride (0.3%, 0.6%, 1.2%, 2.4%, 4.8% by dry soil weight) and calcium hydroxide (0.3%, 0.6%, 1.2%, 2.4%, 4.8% by dry soil weight) powders were poured into a blender and mixed for approximately 5–10 min to achieve uniformity. The mixture was then transferred to a plastic cylindrical mold with a diameter of 39.1 mm and a height of 80 mm. The cylindrical samples were removed after 24 h. The cylindrical specimens were wrapped separately in plastic bags under 95% relative humidity and at
Unconfined compression tests (UCT) were performed on the specimens at different curing times (3, 7, 14, and 28 d) following ASTM D4219. The vertical displacement rate of the UCTs was 1 mm/min. Triplicate measurements of the unconfined compressive strength (qu) were conducted, and the average value was used. The experimental setup is plotted in Figure 1.
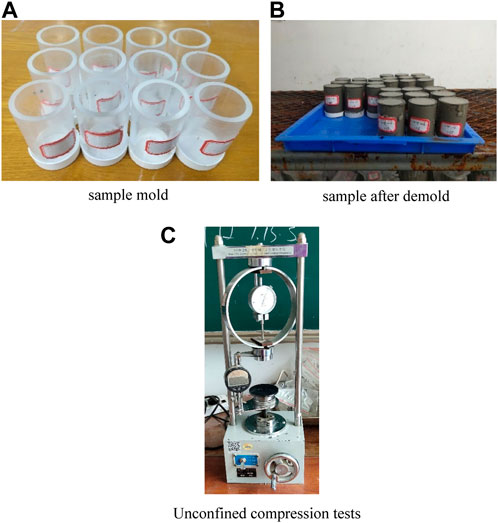
FIGURE 1. Experimental setup. (A) Sample mold. (B) Sample after demold. (C) Unconfined compression tests.
3 Results and discussion
3.1 Stress-strain relationship curve
Figure 2 shows the stress–strain relationship curves of the treated soil at different flocculant contents and curing times. This indicates that the stress of the cured soil increased with strain towards the peak stress, followed by a decrease in stress with strain, showing a typical strain-softening type for all specimens.
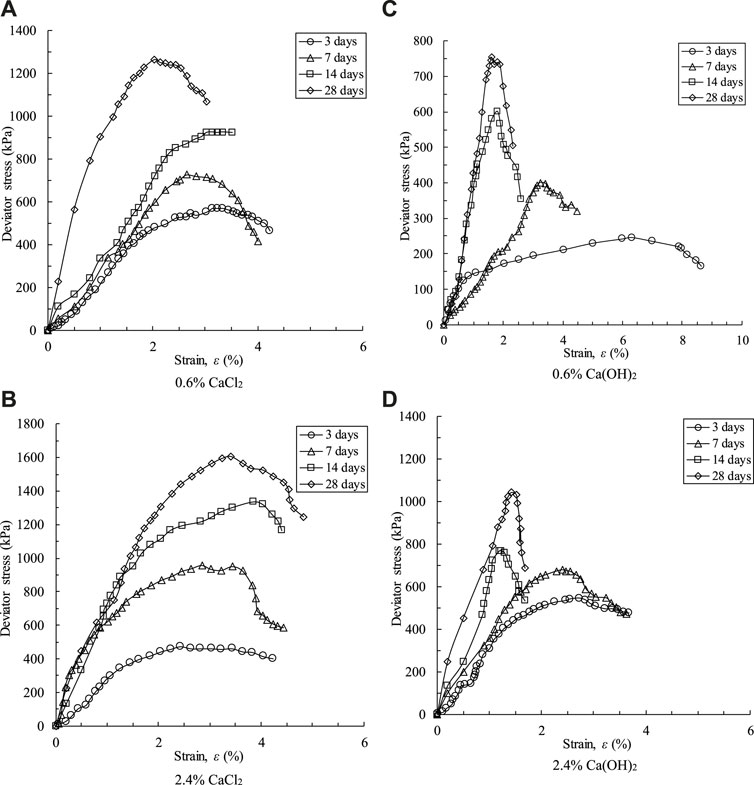
FIGURE 2. Stress-strain relation of different flocculants content. (A) 0.6% CaCl2. (B) 2.4% CaCl2. (C) 0.6% Ca(OH)2. (D) 2.4% Ca(OH)2.
With an increase in curing time, the stress-strain curves tended to move towards the left of the axis, with an increase in the peak stress with curing time. However, the strain at the peak stress decreased with increasing curing time, except for the case of 2.4% CaCl2 with little change in the strain at the peak stress at different curing times. At the same curing times, an increase in the peak stress with increasing flocculant content was observed as the strain of the peak stress increased. This indicates that the addition of flocculants and curing times lead to increased soil brittleness.
3.2 Unconfined compressive strength
Figure 3 shows the variation in the unconfined compressive strength qu with CaCl2 content at different curing times. For a given CaCl2 concentration, the longer the curing time, the greater the value of qu. This behavior indicates a chemical reaction between CaCl2, clay particles, and water that lasts approximately 28 d. As expected, the addition of CaCl2 significantly increased the qu value compared with that of the soil without CaCl2 under the same curing time. qu for soil treated with CaCl2 is close to 1.0 MPa after 14 d of curing and up to 1.5 MPa after 28 d of curing. Moreover, an optimal CaCl2 content corresponding to the highest value of qu was identified. That is, qu increases with an increase in CaCl2 content up to the optimal point, after which it shows a decreasing tendency with a further increase in CaCl2 content. Nevertheless, the addition of CaCl2 led to a significant increase in the strength of the cured soil. The optimal CaCl2 content was primarily attributed to the increased pozzolanic reaction in the treated soil caused by the increased Ca2+ concentration in the pore solution when CaCl2 was added to the slurry. Additionally, the alkaline environment formed by CaCl2 from the hydration reaction accelerates the pozzolanic reaction. Consequently, the increased content of CaCl2 leads to an increase in the strength of the treated soil at the early stage up to the optimal CaCl2 content. When the CaCl2 content exceeded the optimal CaCl2 content, qu decreased because the additional CaCl2 content led to an increase in the Cl− concentration in the pore fluid of the treated soil. At a certain level, Cl− reacts with 3CaO·Al3O3 to form calcium chloroaluminate hydrate (3CaO·Al3O3·3CaCl3·32H2O), which does not belong to the cementitious products and can be expressed as follows:
In other words, the overdosage of CaCl2 content causes a decline in the amount of cementitious products such as 3CaO·2SiO2·3H2O (calcium silicate hydrate, C-S-H) and 3CaO·2SiO2·Ca(OH)2·12H2O (calcium aluminate hydrate, C-A-H), causing a reduction in strength. In summary, the strength of the soil treated with CaCl2 was attributed to the combined effects of Ca2+ and Cl−. Therefore, an optimal concentration of CaCl2 to optimize the strength of the treated soil exists.
The variation of unconfined compressive strength with Ca(OH)2 content is shown in Figure 4. As expected, the qu value and curing time were positively correlated. After 28 d of curing, the maximum value of qu can exceed 1.0 MPa. Unlike CaCl2, the qu of soil treated with Ca(OH)2 shows an increasing trend when Ca(OH)2 content is low and with a slight reduction as the Ca(OH)2 content increases, followed by a further increase in Ca(OH)2 content. Overall, the unconfined compressive strength of the solid treated with Ca(OH)2 was higher than that of the soils without Ca(OH)2. This is mainly attributed to the pozzolanic reaction between Ca(OH)2, SiO2, and Al2O3 in clay minerals, forming cementitious products such as calcium silicate hydrate (C-S-H) and calcium aluminate hydrate (C-A-H), thereby reinforcing the treated soil. Owing to the low solubility of Ca(OH)2 in water, the Ca2+ content in the treated soil did not increase monotonously with increasing Ca(OH)2 content. Therefore, the qu value changing with the Ca(OH)2 content mainly occurs in the low content range, up to 0.6% of Ca(OH)2. When Ca(OH)2 content exceeds 0.6%, the formation of cementitious material covering the soil particles owing to the pozzolanic reaction from the contact between Ca(OH)2 and clay minerals is hindered to a certain extent; therefore, the pozzolanic reaction is slowed down, corresponding to a macroscopic reduction in the strength of the treated soil. Furthermore, as the Ca(OH)2 content increased to 2.4%, a large amount of Ca(OH)2 that did not participate in the pozzolanic reaction with clay minerals reacts with the CO2 in the air to produce water-insoluble calcium carbonate, which produces additional cementitious products, making the microstructure of treated soil denser. Consequently, when Ca(OH)2 content exceeded 2.4%, qu increased. Note that, as qu continuously increased with Ca(OH)2 content, the peak value of qu may correspond to a Ca(OH)2 content higher than 5%. However, the usage of high Ca(OH)2 content will show economic disadvantages. Hence, it is not practical available to implement Ca(OH)2 content higher than 5%. Thus, the range of 1%–5% of Ca(OH)2 content is used in this study.
In addition, because of the solubility of Ca(OH)2, the ionization of Ca(OH)2 dissolved in water produced a lower amount of Ca2+ than that of CaCl2 at the same flocculant concentration. Macroscopically, the increase in the strength of the treated soil caused by Ca(OH)2 was less than that caused by CaCl2.
To further identify the effect of flocculant content on the strength of treated soil, the parameters Δqu are introduced for analysis, where qu0 is the unconfined compressive strength of treated soil without flocculant, qu is the unconfined compressive strength of treated soil with flocculant, and Δqu (=qu-qu0) is the difference between the unconfined compressive strength of cured soil with and without flocculant. The results are shown in Figure 5. When the CaCl2 content increases from 0.3% to 4.8%, Δqu at the same curing time increases from 28.1 to 683.3 kPa. Except for the 0.3%, Δqu increases with the increase in curing time at the same CaCl2 content. For Ca(OH)2, when increasing from 0.3% to 4.8%, Δqu increases from 17.5 to 343.3 kPa. However, unlike CaCl2, most of the increase in strength due to Ca(OH)2 occurs in the early curing period.
In summary, the addition of calcium flocculant is beneficial for increasing the strength of treated soil. However, the amount of flocculant should be designed according to the actual engineering purposes.
3.3 Failure strain
The failure strain εf is an important indicator for characterizing the deformation characteristics of treated soil and its magnitude can reflect the compressibility of treated soil after curing. Typically, the larger the failure strain, the stronger the treated soil; conversely, the smaller the damage strain, the more brittle the treated soil. The strain corresponding to the peak stress on the stress–strain curve of the treated soil is generally considered to be the failure strain. When there is no peak point on the stress–strain curve, a strain of 15% is selected as the failure strain.
According to the stress–strain curves of the cured soils with different CaCl2 and Ca(OH)2 contents, the corresponding failure strains were obtained, as shown in Figure 6. The corresponding damage strains are shown. When the CaCl2 flocculant is used, the presence of CaCl2 in the early curing period (3 and 7 d) led to a decrease in the failure strain of the treated soil with an increase in CaCl2 content. At longer curing times (14 and 28 d), the failure strain was observed to increase with increasing CaCl2 content. This indicates that the brittleness of the soil was reduced at longer curing times in the presence of CaCl2, showing more optimized deformation characteristics of the treated soil for projects such as embankments or foundations. Notably, the failure strain was almost unaffected by the curing time at CaCl2 of 2.4% and 4.8%. When the curing time reached 28 d, the failure strains of the treated soil with different CaCl2 concentrations showed little change, remaining at basically the same magnitude.
Unlike CaCl2, the failure strain of the soil treated with Ca(OH)2 was negatively correlated with the curing time. In addition, the failure strain decreased with increasing Ca(OH)2 content. Compared with the cured soil without flocculant, the addition of Ca(OH)2 caused a significant decrease in the failure strain during the early curing period (3 and 7 d) owing primarily to the enhancement of the early strength of the treated soil by the presence of Ca(OH)2. Subsequently, when the curing time exceeded 7 d, the failure strains of the treated soils with different Ca(OH)2 contents were essentially the same. The addition of Ca(OH)2 has little effect on the long-term deformation and brittleness of the treated soil.
Several researchers proposed that the relationship between failure strain εf and qu of cement-treated soils follows an approximate power function (Bian et al., 2021; Zeng et al., 2021; Bian et al., 2022a; Bian et al., 2022b). Figure 7 shows the relationship between the εf and qu of treated soil at different curing times and different flocculants. For the soil treated with CaCl2, εf basically remains within the range of 2.4% and 4.8% with increasing soil qu and does not show a decreasing pattern. This may be due to the fact that the excessive Cl− disrupts the pozzolanic reaction and leads to the formation of non-cementitious materials calcium chloroaluminate hydrate (3CaO·Al3O3·3CaCl3·32H2O). Therefore, the variation in the failure strain of the soil treated with CaCl2 differed from that of the cement-treated soil. Conversely, the general power relation between εf and qu for soil treated with Ca(OH)2 is observed in Figure 7B. A fair correlation coefficient (R2 = 0.613) suggests that the derived power function can be used as a useful engineering tool for the first-order estimation of failure strain. This is because the mechanisms of strength enhancement, such as pozzolanic reactions, ion exchange, and carbonation for the Ca(OH)2 treated soil were similar to those of cement. Therefore, when the flocculant is Ca(OH)2, the failure strain εf tends to gradually decrease as qu increases.
3.4 Deformation modulus E50
E50 is an important parameter for the quantitative estimation of the undrained settlement of soft ground, which is essential for the design of practical projects (Bian et al., 2021). The deformation modulus E50 can be calculated from the stress–strain curve measured by the unconfined compressive test as follows:
where σ1/2 is the stress corresponding to a vertical strain of half the failure strain.
Figure 8 depicts the deformation modulus E50 of the soil treated with different flocculants; notably, the E50 of the soil treated with CaCl2 increased with an increase in curing time at 3 and 7 d, followed by a decreasing trend with increasing curing time at 14 and 28 d. At early curing times (3 and 7 d), the increase in CaCl2 content caused an increase in E50. Conversely, a decrease in E50 with increasing CaCl2 content was observed at curing times of 14 and 28 d. Moreover, at longer curing times, the E50 of treated soil with CaCl2 contents of 1.2%, 2.4%, and 4.8% were higher than those without flocculant, whereas the E50 at CaCl2 of 0.3% and 0.6% were smaller than those without flocculant. The deformation properties εf and E50 as shown in Figures 7A, 8A illustrate that when CaCl2 flocculant is used to improve the strength and deformation characteristics of treated soil, the appropriate amount of CaCl2 should be selected according to the actual project requirements to meet the requirements of engineering purpose and economy perspective.
Similar results on E50 were observed for Ca(OH)2 treated soil. At early curing times (3 and 7 d), E50 was observed to increase significantly with an increase in Ca(OH)2 content. This was due to the significant enhancement in the early strength of the Ca(OH)2 treated soil as shown in Figure 6. Evidently, E50 for Ca(OH)2 treated soil in the early curing stages (3 and 7 d) was higher than that without flocculants, owing to the increase in qu as mentioned above. When the curing time exceeded 14 d, E50 showed a decreasing tendency with curing time and Ca(OH)2 content and was gradually lower than that without flocculants.
E50 and qu in cement-treated soils are positively correlated ((Bian et al., 2016; 2022a). The relationship between E50 and qu for soils treated with flocculants for different curing times is shown in Figure 9. As expected, E50 increased with increasing qu, which is consistent with previous findings (Bian et al., 2016, 2021; 2022a). The relationship between CaCl2 and Ca(OH)2 treated soil ranges from E50= (20–110) qu. This relationship depends on the flocculant type, flocculant content, and slurry water content. Compared with the results of cemented soil (Kang et al., 2017), this relationship is slightly weak because of the lower level of pozzolanic reaction from the flocculants. The relationship between E50 and qu in this study can provide a first-order estimation of the stiffness of CaCl2 or Ca(OH)2 treated soil. However, detailed determination still needs to be conducted in the field.
3.5 Influence of strength improvement by flocculants in vacuum efficiency
As proposed by Yan et al. (2020), calcium-based flocculants [Ca(OH)2 and CaCl2] significantly influence vacuum transmission within the slurry, thereby improving the vacuum treatment efficiency. Hence, this influence can be inferred to result from the change in flocculant strength, as discussed in the previous sections. Therefore, relating the vacuum transmission with qu at different flocculant contents illustrates the mechanism of improvement in vacuum treatment efficiency with calcium-based flocculants. Figure 10 shows the relationship between the degree of vacuum at the bottom of the model test proposed by Yan et al. (2020) and the strength variation in this study. Evidently, the vacuum degree shows a strong positive relationship with qu of the treated soil in the range of calcium-based flocculants in this study. This behavior indicates that the increase in qu in the presence of calcium-based flocculants significantly improves the vacuum-treatment efficiency, that is, a higher undrained shear strength of the treated soil leads to a lower water content after vacuum treatment at the same preloading period. This is primarily because by adding calcium-based flocculants to the slurry, larger soil aggregates are formed, thus increasing the strength and accelerating soil settlement, consequently easing water extraction from the slurry (Mallela et al., 2017; Wang et al., 2019; Yan et al., 2020).
Furthermore, Figures 3, 4 show that the change in qu with CaCl2 and Ca(OH)2 content did not increase monotonously. This means that the vacuum-treatment efficiency should not linearly increase with the CaCl2 and Ca(OH)2 content. For example, qu decreased with CaCl2 amount exceeding 2.4%. Therefore, an optimal calcium-based flocculant content that corresponds to the highest vacuum-treatment efficiency exists. When the calcium-based flocculant content is lower than the optimal content, larger soil aggregates owing to the flocculation effect of calcium-based flocculants lead to the formation of a large net-frame structure, resulting in a water transmission path, thereby increasing the vacuum transmission rate. Once the optimal calcium-based flocculant content is exceeded, excessively large soil flocs block the drainage materials, reducing the drainage capacity and eventually attenuating the vacuum transmission efficiency (Yan et al., 2020).
These phenomena suggest that when using calcium-based flocculants such as CaCl2 and Ca(OH)2 to improve vacuum efficiency, an adequate dosage is recommended to significantly improve the vacuum treatment efficiency, rather than applying an overdose of calcium-based flocculants to block the drainage materials, thus reducing the vacuum treatment efficiency. Note that, the mechanism of CaCl2 or Ca(OH)2 on the improvement of vacuum efficiency corresponded to the microstructure changes induced by the Ca-based flocculate, which need to be further studied. In addition, the detailed microstructure analysis at pre- and post-vacuum preloading should be conducted to clarify the change in the strength of slurry with the alternation of microstructure at two stages, consequently proposing the optimal treatment combination.
It should also be mentioned that there are some new and environmentally friendly agents such as geopolymer or microbial have emerged to improve the mechanical behavior of soils (Leong et al., 2015; Omoregie et al., 2016). However, these types of agent were mainly dealt with the improvement of soils with water content less than its liquid limit. Hence, the application of geopolymer or microbial in the treatment of slurry with high water content as well as the combination of vacuum preloading method needs to be further studied.
4 Conclusion
This study analyzed and identified the effects of two calcium-based flocculants [Ca(OH)2 and CaCl2] on the strength and deformation properties of slurry. Accordingly, the efficiency of vacuum preloading is discussed based on the relationship between the strength properties after treatment and the vacuum transmission rate. The main results of this study are as follows:
1) Calcium-based flocculants [CaCl2 or Ca(OH)2] increased the unconfined compressive strength (qu). qu increases with increasing CaCl2 content up to 2.4%, then decreases when CaCl2 content exceeds this concentration. However, Ca(OH)2 significantly enhanced qu at an early curing time, decreased slightly up to 2.4% flocculant concentration, and then increased until 4.8% flocculant concentration.
2) For the soil treated with CaCl2, the failure strain εf basically remains within the range of 2.4%–4.8% with no clear correlation with qu. However, the failure strain exhibited a decreasing trend with increasing qu for Ca(OH)2.
3) The E50 of soil treated with CaCl2 shows an increasing trend with increasing curing time at 3 and 7 d, followed by a decreasing trend with increasing curing time at 14 and 28 d. E50 for the Ca(OH)2 treated soil in the early curing stages (3 and 7 d) was higher than that without flocculants owing to the increase in qu as mentioned above. When the curing time exceeded 14 d, E50 tended to decrease with increasing Ca(OH)2 content.
4) The vacuum-treatment efficiency shows a clear increasing trend with the increase in qu in the presence of calcium-based flocculants; that is, a higher undrained shear strength of the treated soil leads to a lower water content after vacuum treatment at the same preloading period.
5) Further studies should be focused on the microstructure analysis of the effect of Ca-based flocculate and vacuum preloading to investigate the mechanism of improvement of vacuum efficiency induced by Ca-based flocculate.
Data availability statement
The raw data supporting the conclusion of this article will be made available by the authors, without undue reservation.
Author contributions
JY: Conceptualization, Data curation, Funding acquisition, Methodology, Software, Writing–original draft, Writing–review and editing. LL: Conceptualization, Data curation, Supervision, Writing–review and editing. YM: Funding acquisition, Resources, Supervision, Writing–review and editing. ZW: Data curation, Investigation, Software, Validation, Writing–review and editing. TR: Data curation, Investigation, Software, Validation, Writing–review and editing.
Funding
The author(s) declare financial support was received for the research, authorship, and/or publication of this article. The authors wish to acknowledge the support of the China Geological Survey Project (Grant No. DD20211314), the Project for the Chinese Academy of Geological Sciences (Nos JKY202122 and HX 2023-05).
Conflict of interest
The authors declare that the research was conducted in the absence of any commercial or financial relationships that could be construed as a potential conflict of interest.
Publisher’s note
All claims expressed in this article are solely those of the authors and do not necessarily represent those of their affiliated organizations, or those of the publisher, the editors and the reviewers. Any product that may be evaluated in this article, or claim that may be made by its manufacturer, is not guaranteed or endorsed by the publisher.
References
Bian, X., Wang, Z. F., Ding, G. Q., and Cao, Y. P. (2016). Compressibility of cemented dredged clay at high water content with super-absorbent polymer. Eng. Geol. 208, 198–205. doi:10.1016/j.enggeo.2016.04.036
Bian, X., Zeng, L. L., Ji, F., Xie, M., and Hong, Z. (2022a). Plasticity role in strength behavior of cement-phosphogypsum stabilized soils. J. Rock Mech. Geotechnical Eng. 14 (6), 1977–1988. doi:10.1016/j.jrmge.2022.01.003
Bian, X., Zeng, L. L., Li, X. Z., and Hong, J. T. (2021). Deformation modulus of reconstituted and naturally sedimented clays. Eng. Geol. 295, 106450. doi:10.1016/j.enggeo.2021.106450
Bian, X., Zhang, W., Li, X. Z., Shi, X. S., Deng, Y. F., and Peng, J. (2022b). Changes in strength, hydraulic conductivity and microstructure of superabsorbent polymer stabilized soil subjected to wetting-drying cycles. Acta Geotech. 17 (11), 5043–5057. doi:10.1007/s11440-022-01573-x
Cai, Y., Xie, Z., Wang, J., Wang, P., and Geng, X. (2019). Reply to the discussion by Mesri and Kane on “New approach of vacuum preloading with booster prefabricated vertical drains (PVDs) to improve deep marine clay strata”. Can. Geotechnical J. 56 (12), 2017. doi:10.1139/cgj-2019-0250
Cao, Y., Xu, J., Bian, X., and Xu, G. (2019). Effect of clogging on large strain consolidation with prefabricated vertical drains by vacuum pressure. KSCE J. Civ. Eng. 23 (10), 4190–4200. doi:10.1007/s12205-019-1884-2
Chai, J., Hong, Z., and Shen, S. (2010). Vacuum-drain consolidation induced pressure distribution and ground deformation. Geotext. Geomembranes 28 (6), 525–535. doi:10.1016/j.geotexmem.2010.01.003
Chu, J., Bo, M. W., and Choa, V. (2006). Improvement of ultra-soft soil using prefabricated vertical drains. Geotext. Geomembranes 24 (6), 339–348. doi:10.1016/j.geotexmem.2006.04.004
Gao, Y. F., Hang, L., He, J., and Chu, J. (2019). Mechanical behaviour of biocemented sands at various treatment levels and relative densities. Acta Geotech. 14 (3), 697–707. doi:10.1007/s11440-018-0729-3
He, J., Gao, Y. F., Gu, Z., Chu, J., and Wang, L. (2020). Characterization of crude bacterial urease for CaCO3 precipitation and cementation of silty sand. J. Mater. Civ. Eng. 32 (5), 04020071. doi:10.1061/(asce)mt.1943-5533.0003100
Indraratna, B., Rujikiatkamjorn, C., Balasubramaniam, A. S., and McIntosh, G. (2012). Soft ground improvement via vertical drains and vacuum assisted preloading. Geotext. Geomembranes 30, 16–23. doi:10.1016/j.geotexmem.2011.01.004
Kang, G., Tsuchida, T., and Kim, Y. (2017). Strength and stiffness of cement-treated marine dredged clay at various curing stages. Constr. Build. Mater. 132, 71–84. doi:10.1016/j.conbuildmat.2016.11.124
Leong, H. Y., Ong, D. E. L., Sanjayan, J. G., and Nazari, A. (2015). A genetic programming predictive model for parametric study of factors affecting strength of geopolymers. RSC Adv. 5 (104), 85630–85639. doi:10.1039/c5ra16286f
Liu, C., Xu, G., and Xu, B. (2018). Field study on the vacuum preloading of dredged slurry with wheat straw drainage. KSCE J. Civ. Eng. 22 (11), 4327–4333. doi:10.1007/s12205-018-1555-8
Mallela, J., Von Quintus, H., Kelly, P. E., and Smith, L. (2017). Consideration of lime-stabilized layers in mechanistic-empirical pavement design.
Moghal, A. A. B., Vydehi, V., Moghal, M. B., Almatrudi, R., AlMajed, A., and Al-Shamrani, M. A. (2020). Effect of calcium-based derivatives on consolidation, strength, and lime-leachability behavior of expansive soil. J. Mater. Civ. Eng. 32 (4), 04020048. doi:10.1061/(asce)mt.1943-5533.0003088
Omoregie, A. I., Senian, N., Li, P. Y., Hei, N. L., Leong, D. O. E., Ginjom, I. R. H., et al. (2016). Ureolytic bacteria isolated from Sarawak limestone caves show high urease enzyme activity comparable to that of Sporosarcina pasteurii (DSM 33). Malays. J. Microbiol., 463–470.
Wang, J., Cai, Y., Ma, J., Chu, J., Fu, H., Wang, P., et al. (2016). Improved vacuum preloading method for consolidation of dredged clay-slurry fill. J. Geotechnical Geoenvironmental Eng. 142 (11), 06016012. doi:10.1061/(asce)gt.1943-5606.0001516
Wang, J., Huang, G., Fu, H., Cai, Y., Hu, X., Lou, X., et al. (2019). Vacuum preloading combined with multiple-flocculant treatment for dredged fill improvement. Eng. Geol. 259, 105194. doi:10.1016/j.enggeo.2019.105194
Wang, J., Ni, J., Cai, Y., Fu, H., and Wang, P. (2017). Combination of vacuum preloading and lime treatment for improvement of dredged fill. Eng. Geol. 227, 149–158. doi:10.1016/j.enggeo.2017.02.013
Wu, D., Xu, W., and Tjuar, R. (2015). Improvements of marine clay slurries using chemical–physical combined method (CPCM). J. Rock Mech. Geotechnical Eng. 7 (2), 220–225. doi:10.1016/j.jrmge.2015.02.001
Xu, G. Z., Yin, J., Feng, X. S., and Ji, F. (2020). An improved method for dewatering sewage sludge using intermittent vacuum loading with wheat straw as vertical drains. KSCE J. Civ. Eng. 24 (7), 2017–2025. doi:10.1007/s12205-020-2216-2
Yan, J. K., Ji, F., Bian, X., Xu, G. Z., Liu, L., Liu, X. L., et al. (2020). Ultra-soft ground treatment of vacuum preloading combining with calcium-based flocculants. Adv. Civ. Eng., 6691565.
Zeng, L. L., Bian, X., Zhao, L., Wang, Y. J., and Hong, Z. S. (2021). Effect of phosphogypsum on physiochemical and mechanical behaviour of cement stabilized dredged soil from Fuzhou, China. Geomechanics Energy Environ. 25, 100195. doi:10.1016/j.gete.2020.100195
Keywords: dredged slurry, calcium-based flocculants, strength, vacuum efficiency, soil treatment
Citation: Yan J, Liu L, Ma Y, Wang Z and Ren T (2023) Strength properties of soils treated with calcium-based flocculants and their impact on vacuum preloading. Front. Earth Sci. 11:1276159. doi: 10.3389/feart.2023.1276159
Received: 11 August 2023; Accepted: 30 October 2023;
Published: 28 December 2023.
Edited by:
Yi Xue, Xi’an University of Technology, ChinaReviewed by:
Arif Ali Baig Moghal, National Institute of Technology Warangal, IndiaDominic E. L. Ong, Griffith University, Australia
Copyright © 2023 Yan, Liu, Ma, Wang and Ren. This is an open-access article distributed under the terms of the Creative Commons Attribution License (CC BY). The use, distribution or reproduction in other forums is permitted, provided the original author(s) and the copyright owner(s) are credited and that the original publication in this journal is cited, in accordance with accepted academic practice. No use, distribution or reproduction is permitted which does not comply with these terms.
*Correspondence: Lei Liu, bGl1bGVpODMwNDEzQHNvaHUuY29t; Yan Ma, eW1hNjQxMTExQDE2My5jb20=