- School of Petroleum Engineering, Yangtze University, Wuhan, China
Steam flooding is an important thermal recovery method for heavy oil reservoirs, and convective heating technology is used to fracture oil shale reservoirs with good results. This paper reviews the main prediction methods, optimization approaches for steam flooding performance, and its application in fractured oil shale reservoirs. The prediction methods include experimental, numerical simulation, and statistical models. These provide insights into steam override, heat transfer, and production dynamics. To optimize steam flooding, parameters like quality, temperature, injection rate, and allocation need to be coordinated based on reservoir conditions and monitoring data. Real-time injection control, economic analysis, and sweep efficiency improvements should also be considered in optimization workflows. Although progress has been made, more field studies are needed to establish systematic optimization practices utilizing advanced technologies. This review summarizes the key developments in steam flooding modeling and optimization, providing a reference for further research and field applications.
1 Introduction
Steam flooding is an important thermal recovery technique that uses heat to mobilize and produce heavy and viscous crude oil. It helps reduce oil viscosity through various mechanisms and improves recovery from depleted or marginal reservoirs. This paper reviews key developments in modeling and optimizing steam flooding performance for conventional heavy oil as well as applications in fractured reservoirs like oil shale. Prediction methods provide insights into complex heat and fluid flow dynamics during steam flooding. Various technical parameters need to be optimized based on reservoir conditions to improve project economics. Convective heating technologies have been applied in fractured reservoirs including oil shale with good results. Further studies on systematic optimization workflows are still needed to maximize the potential of steam flooding (Rao et al., 2022; Xu et al., 2022). This review summarizes progress in this area to provide a reference for future research and field applications.
2 Steam flooding reservoir prediction methods
The main methods for dynamic prediction of steam flooding development are mine-site experimental method (Zhao, 2020), numerical simulation method (Falta et al., 1992; Mozaffari et al., 2013), and statistical model method (Shafiei et al., 2013). The mine-site experimental method mainly summarizes the steam overriding phenomena in the block based on the production situation of the block, combined with geological characteristics, and analyzes some rules of steam overriding (Sander et al., 1991). Lauwerier (1955) was the first scholar to start the theoretical research on steam flooding and make some progress. Through his studies on reservoir heat transfer problems such as injecting steam and other hot fluids, he obtained the laws of reservoir heat transfer in the reservoir and near the injection wells. Langeheim and Marx proposed a classic mathematical model for steam injection and thermal oil recovery by viscosity reduction. This became the theoretical basis for subsequent scholars to study thermal oil recovery by steam. Through Langeheim and Marx’s theory, the speed of heat penetration, cumulative heated area, and the economic limit of injecting heat into an ideal reservoir at a constant temperature rate can be roughly estimated (Marx and Langenheim, 1959).
Ramey (1959) further expanded the above steam flooding theoretical model in 1959. Ramey changed the original fixed steam injection rate to variable steam injection rate, which was Ramey’s major contribution to steam flooding theory. Willman et al. (1961) derived an equation that can roughly estimate the heating radius under a fixed steam injection rate. This method was used to predict the required steam injection rate for a fixed steam volumetric flow rate in radial flow reservoirs.
The research results of the above scholars assume that the temperature distribution in the reservoir is step-wise, dividing the fluid flow regions in the steam flooding process into hot and cold zones. This theoretical assumption was broken by Mandl-Volek (1969)’s innovation on physical models in 1969. The model incorporates heat exchange at the steam front in the steam flooded reservoir, and introduces the concept of “critical time” into the model. Based on Mandl-Volek’s theory, Myhill-Stegemeier (1978) proposed a set of steam front displacement theories through in-depth research. The premise of this method assumes that the oil displacement is equal to the crude oil production. If the calculation is based on this assumption, the results will be biased high.
Through sensitivity analysis of various parameters on the Kern River block, Gomma (1980) established a new steam flooding model. His analysis revealed the relationship between key parameters such as formation thickness, net to gross ratio, and initial oil saturation for this type of steam flooded reservoir. The calculation formula can be used to obtain reservoirs with similar fluid properties or physical similarities to the Kern River block. However, this method is only applicable to reservoirs similar to the Kern River block.
Based on the theoretical foundations of the above models, Jones (1981) proposed a mathematical model for one-dimensional steam flooding. The Jones model combines 3 empirical coefficients from actual field production to predict steam flooding production dynamics. However, the empirical coefficients of this model can only be applied to reservoirs with similar properties to the Kern River field. To apply it to other oilfields, the empirical coefficients must be history matched against actual field data to obtain accurate coefficients.
Considering steam override, Farouq Ali (1982) proposed a steam flooding model based on the Van Lookeren model theory. The most obvious advantage is the use of the principles of conservation of mass and energy. It considers heat loss without relying on empirical data. However, the shortcoming is that some parameters of this model are difficult to obtain, and temperature has a significant impact on relative permeability. Therefore, the experimental results hardly reflect the real situation. To facilitate screening and evaluating reservoirs for suitability of applying steam flooding for improved oil recovery, Aydelotte and Pope (1983) constructed a simplified predictive model for steam flooding. This model expanded the Van Lookeren model and designed a more general computer program.
Neuman C.H. (Neuman, 1975; Newman, 1975; Neuman, 1985) proposed a new model. This model assumes that the steam zone grows vertically and considers steam override. Miller-Leung (Miller and Leung, 1985) divided the reservoir into three zones - the steam zone, condensation zone and cold oil zone, and established a predictive steam flooding model considering steam override. The model established by Torabzadeh-Kumar (Torabzadeh et al., 1990) has standardized the historical dynamics of the same or similar oilfields. However, the relationship between normalized recovery factor and injected steam was fitted using polynomials. Kohei Miura and Jin Wang (Miura and Wang, 2012) proposed a simplified analytical model for predicting cumulative steam-oil ratio during steam assisted gravity drainage process (Figure 1).
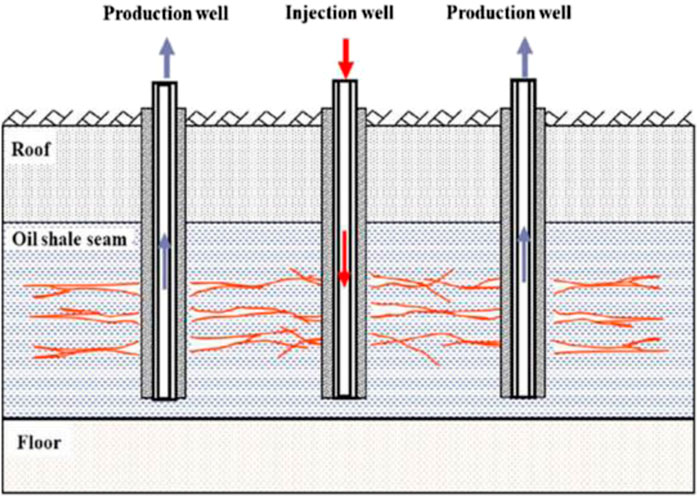
FIGURE 1. Schematic of oil shale in-situ retorting technology by superheated steam injection (MTI) (Kang et al., 2020).
3 Steam flooding production optimization
With the advancement of steam flooding technologies for heavy oil recovery, optimizing steam flooding performance has received increasing attention in both academic research and field applications (Alvarez and Han, 2013; Dong et al., 2019). The key objectives of steam flooding optimization are to maximize heat efficiency, improve sweep efficiency, delay steam breakthrough time, and enhance oil production in an economic manner (Kirmani et al., 2021). This requires optimizing multiple technical parameters throughout the entire process from pilot design to late field development (Huang et al., 2018). Recent studies have proposed various approaches to optimize steam injection parameters based on reservoir conditions (Shin and Polikar, 2007). Dong et al. (2019) optimized quality and temperature of high pressure steam injection for a deep reservoir and achieved higher oil rates than normal pressure. Sun et al. (2017) optimized injection rate to balance heat efficiency and oil production.
With the help of monitoring technologies like distributed temperature sensing (DTS), real-time injection control and optimization has become viable. Patel et al. (2018) designed a closed-loop approach to continuously optimize steam rate based on DTS data and simulator predictions. Teletzke et al. (2010) adjusted injector-producer spacing based on monitoring to mitigate steam override issues. These practices have proven effective in increasing sweep efficiency and reducing steam loss.
To address reservoir heterogeneity, steam allocation optimization has been applied. Yan et al. (2018) optimized zone-based steam allocation with a multi-objective genetic algorithm and improved Net Present Value. Such optimization helps utilize steam energy more efficiently. Economic analysis should be incorporated in steam flooding optimization. Kannah et al. (2021) performed economic evaluation on various steam operational strategies. The optimal strategy balances oil rate, heat efficiency and project life to maximize profitability. Economic optimization helps justify optimization practices and improve overall field development value.
Steam quality is the mass fraction of the vapor to the liquid phase (Guo et al., 2022). It represents a dimensionless number between 0 and 1 indicating the amount of water converted to steam. Steam quality affects displacement efficiency as well as viscosity reduction and other mechanisms, which helps determine optimal flooding conditions (Srochviksit and Maneeintr, 2016). Compared to high injection rates, lower injection rates but higher steam quality can increase recovery to a greater extent (Al Shaibi and Al Abri, 2018). Steam optimization utilizes proper steam allocation to increase oil production in a cost-effective monitoring program, while maintenance, variation in steam quality and restricted steam distribution have negatively impacted steam flooding (Castrup, 2019). Based on these studies, a proper and optimized steam injection scheme can be recommended that activates recovery mechanisms by adjusting steam quality and temperature. This research helps select injection schemes that consider the challenges of steam flooding and maximize total field production from heavy oil reservoirs under economic conditions.
In summary, optimizing steam flooding requires coordinating multiple technical parameters over the entire project life based on continuous monitoring and updated reservoir data. More field studies are still needed to establish robust and systematic optimization workflows with the aid of advanced technologies.
4 Application of steam flooding in fractured oil shale reservoirs
An important technique for developing oil shale reservoirs is convective heating technology, which uses high-temperature fluids to heat the oil shale in situ. Several heating and production wells are first drilled in the oil shale formation, and hydraulic fracturing is used to interconnect the heating and production wells (Wei et al., 2021a; Wei et al., 2021b; Shiming et al., 1305). Then the positions of the production and heating wells are alternated periodically. High-temperature fluids are injected into the oil shale layer through the heating wells, heating up the oil shale formation and thermally cracking kerogen to generate oil and gas. The produced oil and gas are carried out to the surface by the cooler fluids or condensed water through the production wells. The cracking and release of pyrolysis gases from the oil shale generates numerous microfractures, which helps improve the matrix permeability of the oil shale and enhance flow channels (Fan et al., 2010). Compared to electrical heating for in-situ development, the in-situ development of oil shale using fluid heating is more complex, requiring consideration of more factors in numerical simulation. These factors include heat transfer, temperature field, pressure field, fluid flow, and the influence of fractures.
Kang et al. (2008) studied in-situ development techniques for oil shale using steam injection heating, and performed coupled analysis of the fluid flow, temperature, and chemical fields. Li (2017) carried out numerical simulation studies on the temperature field, kerogen concentration, and oil production during steam injection in-situ development of oil shale. Based on hydraulic fracturing to connect the injection and production wells in oil shale formations, Wang (2011) performed simulation analysis of steam injection heating techniques for oil shale. The effects of fracture position on oil shale heating were discussed, but only two-dimensional reservoirs were simulated.
Xue (2007) discovered through numerical simulation that using high temperature and high pressure steam can effectively pyrolyze oil shale and carry away the generated shale oil. High temperature and high-pressure steam can also significantly increase the number of fractures inside the oil shale, improving its permeability. Jiang et al. (2015) performed numerical simulation studies on nitrogen gas injection from production wells for heating and heat transfer. Based on extending the functions of open-source fluid simulation software from Los Alamos National Laboratory in the US, Kelkar (Kelkar et al.) carried out numerical simulation studies of coupled thermal-hydrological-mechanical-chemical processes during in-situ conversion and production, and provided some simple simulation examples. Zhao (2013) performed coupled thermal-flow-solid simulations of the interaction processes between oil and gas components during underground co-gasification of oil shale and coal, obtaining the evolution laws of underground temperature fields and coupling characteristics in the co-gasification process. Li (2017) used CMG numerical simulation software to simulate oil shale pyrolysis experiments, designed the model as a dual-porosity geological model. The kerogen decomposition chemical reactions used an alternative reaction mechanism, simplifying the decomposition process into three chemical reaction equations. Based on IFCD technology, Liu (2019) combined experimental research with numerical simulation to summarize the physical property evolution laws of oil shale during heating. The temperature, stress and strain field variations in the oil shale layer during in-situ development were also analyzed.
5 Results
This review summarizes key developments in modeling and optimizing steam flooding performance. Prediction models provide insights into complex dynamics like steam override and heat efficiency. Optimizing parameters such as quality, temperature, injection rate and allocation can improve performance based on reservoir conditions. Real-time injection control and dynamic optimization enabled by monitoring technologies have been implemented. Economic analysis is vital for justifying optimization practices. Convective heating shows potential in fractured reservoirs like oil shale, by thermally cracking kerogen and enhancing permeability. Further studies are needed to develop systematic optimization workflows using advanced technologies. This review of modeling and optimization advances provides a valuable reference for steam flooding research and applications.
Author contributions
YX: Writing–original draft, Writing–review and editing. ZZ: Conceptualization, Visualization, Writing–review and editing. YZ: Project administration, Supervision, Validation, Writing–original draft, Writing–review and editing.
Funding
The author(s) declare financial support was received for the research, authorship, and/or publication of this article. This study was supported by the Natural Science Foundation of Xinjiang Uygur Autonomous Region (No. 2022D01A330) the National Natural Science Foundation of China (No. 52274030) and “Tianchi Talent” Introduction Plan of Xinjiang Uygur Autonomous Region (2022).
Acknowledgments
The authors thank the support from Yangtze University.
Conflict of interest
The authors declare that the research was conducted in the absence of any commercial or financial relationships that could be construed as a potential conflict of interest.
Publisher’s note
All claims expressed in this article are solely those of the authors and do not necessarily represent those of their affiliated organizations, or those of the publisher, the editors and the reviewers. Any product that may be evaluated in this article, or claim that may be made by its manufacturer, is not guaranteed or endorsed by the publisher.
References
Al Shaibi, R., and Al Abri, A. “Steam optimization topic, injection rate reduction vs. Surface steam quality reduction,” in Proceedings of the SPE EOR Conference at Oil and Gas West Asia, Muscat, Oman, March 2018.
Ali, S. “Steam injection theories-A unified approach,” in Proceedings of the SPE California Regional Meeting, San Francisco, California, March 1982.
Alvarez, J., and Han, S. (2013). Current overview of cyclic steam injection process. J. Petroleum Sci. Res. 2. https://www.academia.edu/26208229/Current_Overview_of_Cyclic_Steam_Injection_Process.
Aydelotte, S. R., and Pope, G. A. (1983). A simplified predictive model for steamdrive performance. J. Petroleum Technol. 35, 991–1002. doi:10.2118/10748-pa
Castrup, S. “Data analytics for steam injection projects,” in Proceedings of the SPE Western Regional Meeting, San Jose, California, USA, April 2019, D031S011R002.
Dong, X., Liu, H., Chen, Z., Wu, K., Lu, N., and Zhang, Q. (2019). Enhanced oil recovery techniques for heavy oil and oilsands reservoirs after steam injection. Appl. energy 239, 1190–1211. doi:10.1016/j.apenergy.2019.01.244
Falta, R. W., Pruess, K., Javandel, I., and Witherspoon, P. A. (1992). Numerical modeling of steam injection for the removal of nonaqueous phase liquids from the subsurface: 1. Numerical formulation. Water Resour. Res. 28, 433–449. doi:10.1029/91wr02526
Fan, Y., Durlofsky, L. J., and Tchelepi, H. A. (2010). Numerical simulation of the in-situ upgrading of oil shale. SPE J. 15, 368–381. doi:10.2118/118958-pa
Gomaa, E. E. (1980). Correlations for predicting oil recovery by steamflood. J. Petroleum Technol. 32, 325–332. doi:10.2118/6169-pa
Guo, X., Wang, Y., Zhang, J., Ci, F., Ding, G., and Chen, Y. (2022). Online fully-automatic high precision steam dryness monitoring system. Measurement 190, 110642. doi:10.1016/j.measurement.2021.110642
Huang, S., Chen, X., Liu, H., Jiang, J., Cao, M., and Xia, Y. (2018). Experimental and numerical study of solvent optimization during horizontal-well solvent-enhanced steam flooding in thin heavy-oil reservoirs. Fuel 228, 379–389. doi:10.1016/j.fuel.2018.05.001
Jiang, P., Sun, Y., Guo, W., and Li, Q. (2015). Heating technology and heat transfer simulation for oil shale of in-situ pyrolysis by fracturing and nitrogen injection. J. Northeast. Univ. Nat. Sci. 36, 1353. doi:10.3969/j.issn.1005-3026.2015.09.029
Jones, J. (1981). Steam drive model for hand-held programmable calculators. J. Petroleum Technol. 33, 1583–1598. doi:10.2118/8882-pa
Kang, Z., Zhao, Y., and Yang, D. (2008). Physical principle and numerical analysis of oil shale development using in-situ conversion process technology. Acta Pet. Sin. 29, 592–595. doi:10.7623/syxb200804022
Kang, Z., Zhao, Y., Yang, D., Tian, L., and Li, X. (2020). A pilot investigation of pyrolysis from oil and gas extraction from oil shale by in-situ superheated steam injection. J. Petroleum Sci. Eng. 186, 106785. doi:10.1016/j.petrol.2019.106785
Kannah, R. Y., Kavitha, S., Karthikeyan, O. P., Kumar, G., Dai-Viet, N. V., Banu, J. R., et al. (2021). Techno-economic assessment of various hydrogen production methods–A review. Bioresour. Technol. 319, 124175. doi:10.1016/j.biortech.2020.124175
Kelkar, S., Pawar, R., and Hoda, N. Numerical simulation of coupled thermal-hydrological-mechanical-chemical processes during in situ conversion and production of oil shale, 1–8.
Kirmani, F. U. D., Raza, A., Gholami, R., Haidar, M. Z., and Fareed, C. S. (2021). Analyzing the effect of steam quality and injection temperature on the performance of steam flooding. Energy Geosci. 2, 83–86. doi:10.1016/j.engeos.2020.11.002
Lauwerier, H. A. (1955). The transport of heat in an oil layer caused by the injection of hot fluid. Appl. Sci. Res. Sect. A 5, 145–150. doi:10.1007/bf03184614
Liu, Z. (2019). Numerical simulation study of coupled processes in in-situ development of oil shale.
Mandl, G., and Volek, C. (1969). Heat and mass transport in steam-drive processes. Soc. Petroleum Eng. J. 9, 59–79. doi:10.2118/2049-pa
Marx, J., and Langenheim, R. (1959). Reservoir heating by hot fluid injection. Trans. AIME 216, 312–315. doi:10.2118/1266-g
Miller, M., and Leung, W. “A simple gravity override model of steamdrive,” in Proceedings of the SPE Annual Technical Conference and Exhibition, Las Vegas, Nevada, United States, September 1985.
Miura, K., and Wang, J. (2012). An analytical model to predict cumulative steam/oil ratio (CSOR) in thermal-recovery SAGD process. J. Can. Petroleum Technol. 51, 268–275. doi:10.2118/137604-pa
Mozaffari, S., Nikookar, M., Ehsani, M. R., Sahranavard, L., Roayaie, E., and Mohammadi, A. H. (2013). Numerical modeling of steam injection in heavy oil reservoirs. Fuel 112, 185–192. doi:10.1016/j.fuel.2013.04.084
Myhill, N., and Stegemeier, G. (1978). Steam-Drive correlation and prediction. JPT 30 (2), 173–182. doi:10.2118/5572-PA
Neuman, C. (1985). A gravity override model of steamdrive. J. petroleum Technol. 37, 163–169. doi:10.2118/13348-pa
Neuman, C. “A mathematical model of the steam drive process applications,” in Proceedings of the SPE California Regional Meeting, Ventura, California, April 1975.
Newman, C. “A mathematical model of the steam drive process-derivation,” in Proceedings of the SPE California Regional Meeting, Ventura, California, April 1975.
Patel, R., Guevara, J. L., and Trivedi, J. “Closed-loop reservoir management using nonlinear model predictive control: A field case study,” in Proceedings of the European Association of Geoscientists & Engineers, Barcelona, Spain, September 2018, 1–25.
Rao, X., Xin, L., He, Y., Fang, X., Gong, R., Wang, F., et al. (2022). Numerical simulation of two-phase heat and mass transfer in fractured reservoirs based on projection-based embedded discrete fracture model (pEDFM). J. Petroleum Sci. Eng. 208, 109323. doi:10.1016/j.petrol.2021.109323
Sander, P. R., Clark, G. J., and Lau, E. C. “Steam-foam diversion process developed to overcome steam override in Athabasca,” in Proceedings of the SPE Annual Technical Conference and Exhibition, October 1991 (Dallas, Texas, United States.
Shafiei, A., Dusseault, M. B., Zendehboudi, S., and Chatzis, I. (2013). A new screening tool for evaluation of steamflooding performance in Naturally Fractured Carbonate Reservoirs. Fuel 108, 502–514. doi:10.1016/j.fuel.2013.01.056
Shiming, W., Yan, J., Jiawei, K., Yang, X., and Botao, L., Reservoir stress evolution and fracture optimization of infill wells during the drilling-fracturing-production process. Acta Pet. Sin. 43, 1305.
Shin, H., and Polikar, M. (2007). Review of reservoir parameters to optimize SAGD and Fast-SAGD operating conditions. J. Can. Petroleum Technol. 46. doi:10.2118/07-01-04
Srochviksit, S., and Maneeintr, K. (2016). Simulation on heavy oil production from steam-flooding. EDP Sci. 68, 07002. doi:10.1051/matecconf/20166807002
Sun, F., Yao, Y., Chen, M., Li, X., Zhao, L., Meng, Y., et al. (2017). Performance analysis of superheated steam injection for heavy oil recovery and modeling of wellbore heat efficiency. Energy 125, 795–804. doi:10.1016/j.energy.2017.02.114
Teletzke, G. F., Wattenbarger, R. C., and Wilkinson, J. R. (2010). Enhanced oil recovery pilot testing best practices. SPE Reserv. Eval. Eng. 13, 143–154. doi:10.2118/118055-pa
Torabzadeh, S. J., Kumar, M., and Hoang, V. T. “Performance correlations for steamflood field projects,” in Proceedings of the SPE California Regional Meeting, Ventura, California, April 1990.
Wei, S., Jin, Y., Liu, X., and Xia, Y. “The optimization of infill well fracturing using an integrated numerical simulation method of fracturing and production processes,” in Proceedings of the Abu Dhabi International Petroleum Exhibition & Conference, Abu Dhabi, UAE, November 2021, D012S136R001.
Wei, S., Kao, J., Jin, Y., Shi, C., and Liu, S. (2021a). A discontinuous discrete fracture model for coupled flow and geomechanics based on FEM. J. Petroleum Sci. Eng. 204, 108677. doi:10.1016/j.petrol.2021.108677
Willman, B., Valleroy, V., Runberg, G., Cornelius, A., and Powers, L. (1961). Laboratory studies of oil recovery by steam injection. J. Petroleum Technol. 13, 681–690. doi:10.2118/1537-g
Xu, Y., Hu, Y., Rao, X., Zhao, H., Zhong, X., Peng, X., et al. (2022). A fractal physics-based data-driven model for water-flooding reservoir (FlowNet-fractal). J. Petroleum Sci. Eng. 210, 109960. doi:10.1016/j.petrol.2021.109960
Xue, J. (2007). Experimental study on physical and mechanical properties of oil shale and unsteady heat conduction mathematical modeling of its in-situ development.
Yan, Z., Sha, J., Liu, B., Tian, W., and Lu, J. (2018). An ameliorative whale optimization algorithm for multi-objective optimal allocation of water resources in Handan, China. Water 10, 87. doi:10.3390/w10010087
Zhao, L. (2013). Study on coupled processes of in-situ pyrolysis of oil shale and underground gasification of coal.
Keywords: fractured oil shale reservoirs, steam flooding, production prediction, schedule optimization, thermal recovery
Citation: Xu Y, Zhang Z and Zhou Y (2023) A brief review of steam flooding and its applications in fractured oil shale reservoirs. Front. Earth Sci. 11:1268103. doi: 10.3389/feart.2023.1268103
Received: 27 July 2023; Accepted: 14 August 2023;
Published: 29 August 2023.
Edited by:
Shiming Wei, China University of Petroleum, Beijing, ChinaReviewed by:
Zhipeng Wang, China University of Petroleum, Beijing, ChinaYan Zhang, China National Petroleum Corporation, China
Copyright © 2023 Xu, Zhang and Zhou. This is an open-access article distributed under the terms of the Creative Commons Attribution License (CC BY). The use, distribution or reproduction in other forums is permitted, provided the original author(s) and the copyright owner(s) are credited and that the original publication in this journal is cited, in accordance with accepted academic practice. No use, distribution or reproduction is permitted which does not comply with these terms.
*Correspondence: Zhiquan Zhang, Nzk5NTcwMjZAcXEuY29t; Yuhui Zhou, emhvdS55dWh1aUBxcS5jb20=