- 1Shandong Construction Engineering Quality Inspection and Testing Center Co., Ltd., Jinan, Shandong, China
- 2School of Emergency Management, Xihua University, Chengdu, Sichuan, China
- 3Key Laboratory of Landslide Risk Early-warning and Control, Ministry of Emergency Management, Chengdu University of Technology, Chengdu, Sichuan, China
- 4State Key Laboratory of Geohazard Prevention and Geoenvironment Protection, Chengdu University of Technology, Chengdu, Sichuan, China
- 5Key Laboratory of Geohazard Prevention of Hilly Mountains, Ministry of Natural Resources, Fuzhou, Fujian, China
Uneven settlement phenomenon is very easy to occur in the sandy pulverized ground stratum, the underground pipe corridor longitudinal and transverse stiffness difference is obvious, so that it is easy to crack and deformation due to uneven settlement in this type of stratum. This paper carries out research on uneven settlement and cracking and deformation of Qihe tube corridor, analyzes the reasons leading to uneven settlement and the factors affecting the deformation and cracking of the tube corridor, and provides guidance for foundation treatment and tube corridor repair and mixing and reinforcement. It was found that the concrete structure of the corridor itself had exposed reinforcement, pockmarks and holes, and that cracks on the structure of the corridor sprouted and expanded from these defects. Defects and damages on the concrete structure of the pipeline corridor are contributing factors to the deformation and cracking of the members, and the uneven settlement of the foundation is the main initiating factor for the cracking and deformation. The analysis of the numerical simulation results of similar underground pipeline corridors is carried out, and it is found that the simulation results are consistent with the deformation characteristics of the Qihe pipeline corridor, which further supports the conclusions of this paper. The main stratigraphic factors contributing to the inhomogeneous settlement were found to be the water-rich and loose bodies in the lower part of the strata, as revealed by the physical means and the experimental verification boreholes. Localized hydraulic effects such as pumping further accelerate the uneven settlement of the foundation.
1 Introduction
Uneven settlement of foundation is the main initiating factor that induces cracking and deformation of the corridor structure, and congenital defects of the corridor structure are factors that promote cracking and deformation. In order to manage and prevent the cracking and deformation of the pipeline corridor, it is necessary to analyze and study the factors that lead to the uneven settlement of the foundation.
Uneven settlement of foundations is one of the main factors leading to damage to building structures, dynamic changes in structural loading conditions and changes in the environment caused by changes in the undercropping of foundations are causing this uneven settlement (Chen and Hanna, 2023; Wu et al., 2022). The degree to which a building is affected by uneven settlement is related to its own characteristics, the greater the stiffness of the building, the poor coordination of deformation, the greater the effect of uneven settlement on it (Chen et al., 2011; Al’ Malul and Michail, 2018; Bao et al., 2020; Wang et al., 2022). Therefore, for structures with large stiffness, the uneven settlement of foundations should be strictly controlled, which is not only reflected in general buildings, but also for the uneven settlement of high-speed railroad foundations, which should be paid more attention to (Zhang et al., 2023; Liu and Moore, 2021; Zhang et al., 2021; Shi et al., 2022). When detecting and analyzing the uneven settlement of building foundations, the traditional detection method has the problems of large error and long time (Liu et al., 2020). Uneven settlement of foundations is one of the common engineering quality problems in wetted loess areas, and it was found that changes in settlement area and settlement volume in wetted loess areas have no significant effect on the interlayer displacement ratio (Bao et al., 2020; Xu et al., 2022; Ho and Kuwano, 2021). Underground pipe corridors are not only affected by uneven settlement but also by ground cracks. It was found that with the increase of ground crack settlement, a stress reduction zone near the ground crack appeared at the bottom of the upper plate wall of the tube corridor structure and revealed localized dehollowing phenomena (Deng et al., 2022; Xie et al., 2020; Bian et al., 2017). Diagnosis of inhomogeneous settlement in regional railroad tunnels using spatial correlation of high-density strain measurement points can effectively diagnose the influence range of inhomogeneous settlement (Liang et al., 2023; Hu et al., 2021). After the highway widens the roadbed, under the effect of differential settlement, the old pavement surface layer and the upper base layer are subjected to tensile stress within about 4 cm, and the base layer reaches the destructive strength first. Below 4 cm of old pavement, the sub-base first reaches breaking strength (Shen et al., 2021; Zhou, 2022; Wang et al., 2023). There is a relationship between the water content of foundation soils and uneven settlement, with a higher degree of uneven settlement and a wider range of effects in foundations with high water content (Ngugi Hannah et al., 2021). It was found that the more permeable sand layer and the upper cohesive soil layer have greater cohesion and can effectively reduce differential settlement (Cong et al., 2021). The joints of underground pipeline corridors are the weakest parts and are highly susceptible to the effects of uneven settlement (Liu et al., 2020; Jiang et al., 2020; Xie et al., 2020; Gebremedhn et al., 2019). Rigidly spliced box girder bridges under the influence of uneven settlement, the spliced section will appear the phenomenon of transverse stress peaks, which will lead to concrete cracking and damage (Xu et al., 2023). Settlement monitoring plays a crucial role in preventing geologic risks and disasters, and stochastic differential equations reliably yield short-term settlement predictions (Guo et al., 2023). The monitoring of the gypsum mine hollow area found that the surface deformation was mainly horizontal displacement, and the direction of the horizontal displacement was overall pointing to the hollow area (Xu et al., 2023). The effect of rainfall on wet submerged loess is very significant and can trigger strong inhomogeneous settlement phenomena (Zhao, 2022).
In summary, uneven settlement is closely related to the characteristics of the stratum in which it occurs. In soft and sensitive strata such as chalky sandy soils, loose bodies and water-rich bodies are likely to occur, and loose bodies are susceptible to the influence of groundwater and rainfall. Exploring the causes of uneven settlement of foundation is the key to prevent and manage uneven settlement, this paper carries out research on uneven settlement of Qihe corridor, analyzes the deformation and cracking characteristics of the corridor, explores the factors causing uneven settlement of the corridor, and provides theoretical guidance for the next foundation treatment and the repair and reinforcement of the corridor.
2 Overview of the pipeline corridor project
Jingyi Road comprehensive pipeline corridor project is located in the northwest of Qihe County, Qihe County, West Area, north of Qishun Street, south to Qi Trade Street, a total length of 300 m. For the open excavation construction of the pipe corridor, the foundation holding layer is a pulverized soil layer with a thickness of 0.6–2.7 m.
2.1 Engineering geological conditions
The stratigraphy of the pipeline corridor site is a sandy chalky soil stratum. The groundwater belongs to the hydrogeological zone of the Yellow River alluvial plain, and the lithology of the aquifer is generally dominated by fine and powdery sand and silt, and the aquifer is mostly distributed horizontally in the form of bands and lenses, and vertically in the direction of staggered distribution of the aquifer and the relative waterproof layer. The type of groundwater dynamics is precipitation infiltration evapotranspiration. Groundwater depth ranges from 0.40 to 2.80m, groundwater level elevation is 20.31m, and annual variation of groundwater level is 1.50 m. Figure 1 shows the engineering geologic profile.
2.2 Deformation characteristics of pipe corridor
The pipeline corridor has experienced two heavy rainfalls, the first of which occurred on 9 August 2022, during which pumps were used to pump the water in the pipeline corridor to the river 30 m away from the east side of the corridor in order to lower the groundwater level of the site and prevent the foundation from settling unevenly. However, on August 16th, the K0+549∼K0+579 section of the corridor still had uneven settlement, the corridor tilted to the south in general, and the maximum settlement occurred in the corridor deformation joint K0+579, the maximum settlement of the corridor on the north side of the deformation joint reached 1.2 m, and the maximum settlement of the corridor on the south side reached 0.1 m, and at the same time, the ground around the cracks appeared. The second heavy rainfall occurred in early October 2022, and the pumping work continued until mid-October, during which the subsidence of the pipe corridor on both sides of the deformation joints increased, and the peripheral backfill collapsed, and the maximum subsidence of the pipe corridor on the north side of the deformation joints at K0+579 reached 2.0m, and the maximum subsidence of the pipe corridor on the south side reached 1.1m, while the peripheral backfill collapsed. The status of uneven settlement at the site of the pipe corridor is shown in Figure 1H, the surface subsidence depth of the upper part of the pipe corridor is higher than that of other places, and a large number of longitudinal tensioned downward misalignment cracks appeared on the surface, and the longitudinal cracks were mainly distributed on the two sides of the pipe corridor, which were caused by the significant subsidence around the pipe corridor. There are also a small number of transverse cracks distributed around the pipe gallery. The duration of the first heavy rainfall was 4 h and the cumulative rainfall was 76mm, which is of heavy rainfall level. The duration of the second heavy rainfall was 3 h and the cumulative rainfall was 83mm, which is of heavy rainfall level.
During the pouring process of the pipe corridor structure, due to the small distance between the formwork and the reinforcement and the incomplete vibration of the concrete, the concrete of the pipe corridor structure has defects and damages, which are mainly manifested in the exposed tendons, pockmarks and holes. Figure 2A, B shows the exposed reinforcement, pockmarked surface and hole defects existing in the roof slab of the pipe corridor at K0+552.5 m and K0+556.5 m. This defects and damage left by the concrete casting will promote the subsequent deformation of the concrete construction damage, in the subsequent uneven settlement, these defects at the hidden danger will be amplified, will be the first in these defective parts of the cracking damage, which evolved to the destruction of the concrete structure as a whole. Such defects and damages left due to concrete pouring belong to the congenital influences on the structural deformation of the pipe corridor.
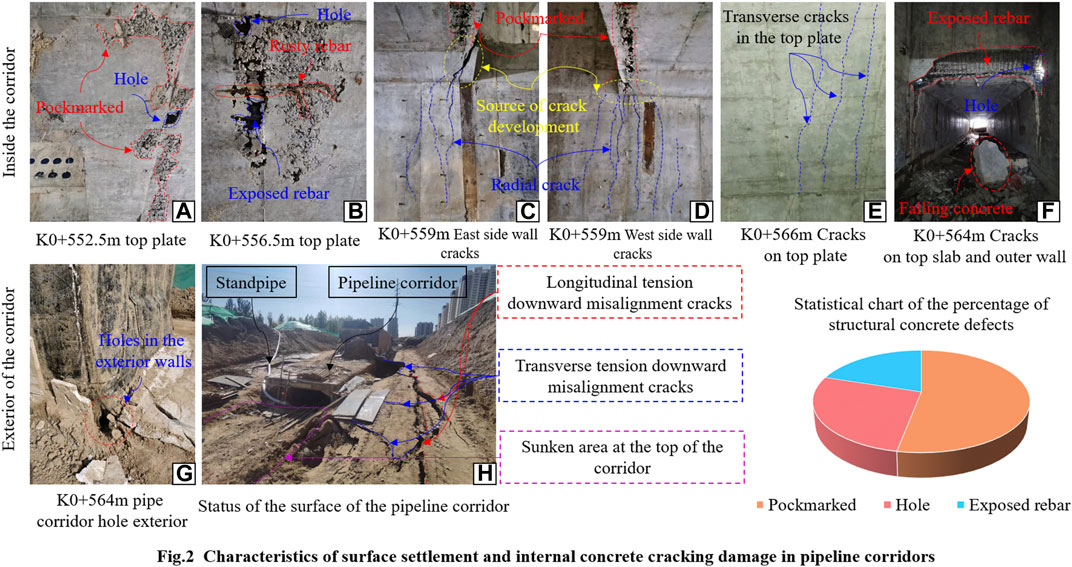
FIGURE 2. Characteristics of surface settlement and internal concrete craking damage in pipeline corridors.
The corridor experienced uneven settlement around the corridor after two heavy rainfall events. Uneven settlement of the foundation leads to unbalanced stress in the corridor structure, and stress concentration occurs at the corridor’s variable cross-section due to the change in cross-sectional area, resulting in tension cracks. There are three cracks in the structure of the pipe corridor, the first crack is located in the east and west sides of the outer wall at K0+559m, the source of cracking is located in the concrete surface, and the cracks are radial expansion from the concrete surface. At K0+559 m there are 7 cracks in the west wall with a maximum crack width of 11.5 mm (shown in Figure 2C) and 5 cracks in the east wall with a maximum crack width of 13.3 mm (shown in Figure 2D). The second crack was located on the top slab at K0+566m, with five cracks distributed transversely and the maximum crack width of 1.24 mm (shown in Figure 2E). The third crack was located in the top slab and exterior wall at K0+564m, where a crack penetrated in the top slab causing the concrete to fall off (shown in Figure 2F) and a hole in the waterproofing layer of the exterior wall (shown in Figure 2G). It can be seen that the uneven settlement of the foundation of the corridor makes the defects of the concrete structure amplified, and the cracks start to sprout from the concrete defects, and expand through under the action of further uneven settlement, leading to structural cracking and damage.
Analyzing the cracking damage characteristics of the pipeline corridor, it is found that the cracks on the concrete structure are mainly sprouted from the exposed reinforcement and pockmarks at the gestation, and penetrate into each other between the concrete holes, and eventually form macroscopic penetrating cracks. It can be seen that inhomogeneous settlement is the main driving factor leading to cracking damage, and original defects in concrete are the main contributing factors leading to cracking damage in concrete structures.
3 Deformation detection methods for pipe corridors
3.1 High-density resistivity methods
In order to investigate the reasons for the uneven settlement of the foundation of the corridor, the high-density resistivity method was used around the corridor to carry out probing on both sides of the corridor and to investigate the physical characteristics of the foundation soil under the corridor. Six measurement lines were arranged on both sides of the pipeline corridor, the lines were 1.0m, 3.0m and 15.0 m away from the outer wall (the distribution of the lines is shown in Figure 3), the spacing of the measurement points was 1.0 m, and the length of the lines was 60 m, and the dipole-dipole device (the on-site arrangement is shown in Figure 3A). The average ground elevation of the 1.0 m measuring line from the outer wall and the 3.0 m measuring line from the outer wall is 18.4m, and the 25# probing point corresponds to its maximum settlement, while the average ground elevation of the 15.0 m measuring line from the outer wall is about 21.4 m, and the 31# probing point corresponds to its maximum settlement.
The maximum transmitting power of the high-density resistance detector is 9000W, the maximum transmitting voltage is ±1500V, the maximum transmitting current is ±6A, the current accuracy is 0.1%, and the working environment temperature is −20–60°C.
3.2 Multi-channel transient surface wave methods
The detection results of the high-density resistivity method indicate the suspected presence of water-rich and loose bodies in the lower part of the pipe corridor, which were eroded by hydraulic forces, resulting in the formation of cavities that led to the uneven settlement of the upper part of the pipe corridor. Due to the diversity of the subsoil, it is difficult to accurately and reliably reflect the real situation of the subsoil with the results obtained by a single means of detection. In order to improve the reliability of the detection information so that the factors leading to inhomogeneous settlement can be accurately identified, the multi-channel transient surface wave method was used to detect the low-resistance region on the basis of the results of the high-density resistivity method. Along the pipeline corridor along the direction of the arrangement of the east and west 2 lines of measurement, each line arrangement of 24 geophones, channel spacing 1.0m, pile number K0 + 579 excitation, offset distance of 1.0 m, detecting the wave velocity of the soil layer below the top plate of the pipeline corridor in the range of 15.0 m, the layout of the measurement line as shown in Figure 3, the distribution of the field detection points as shown in Figure 3B.
The multi-channel transient surface wave detector has a sampling interval of 4–4000 μs, a sampling resolution of 24AD, a dynamic range of more than 110dB, a channel phase error of less than 0.1 ms, a frequency bandwidth of 0.1–5000Hz, and an operating ambient temperature of −20–55°C.
3.3 Standard penetration probe
The results of both the high-density resistivity method and the multi-channel transient surface wave method indicate a high probability of the presence of water-rich and loose bodies in the lower portion of the corridor, and accurately indicate the depth and extent of the subsurface diseased bodies. In order to further verify the authenticity and reliability of the exploration results, based on the results of the high-density resistivity method and multi-channel transient surface wave, drill holes were drilled to verify the results at three points in the area of the suspected sparsity, with hole 1 located in the west side of the pile No. K0+587, hole 2 located in the west side of the pile No. K0+579, and hole 4 located in the east side of the pile No. K0+580. In the area where no abnormality is found, 3# holes (east side pile No. K0+558) are arranged as comparison holes, which are 2.0 m away from the outer walls on both sides of the pipeline corridor, and the arrangement of the holes is shown in Figure 3.
4 Analysis of deformation detection results of pipeline corridor
4.1 Analysis of the results of the high-density resistivity method
The data collected by the high-density resistivity method were inverted and analyzed, and Figure 4 shows a cloud map of the results of the inversion analysis for the six survey lines. The analysis results based on the cloud map show that: 1) along the pipeline corridor direction, pile number K0+578∼ K0+586.5 section, there is an obvious low-resistance area below the bottom plate of the pipeline corridor, the resistivity value is ≤8.0 Ω-m, and initially determined to be the distribution of water-rich and sparse bodies, and the distribution range is shown in Table 1. Water-rich body area due to K0 + 609 south side of the pit pumping and other local hydraulic effects, resulting in the weakening of the soil structure, strength is further reduced, the engineering properties of the soil body deteriorated, endangering the safety of the upper corridor. The hydraulic action triggered by pumping leads to the removal of soil particles from the upper part of the water-rich body, which gradually develops into a cavity and leads to the sinking of the pipe corridor. 2) In the direction of the vertical pipeline corridor axis, it can be seen from Figures 4B–E that the distribution range of the low resistance area is slightly expanded from west to east. As can be seen from Figures 4A, F, the farther away from the centerline of the corridor, the soil below the bottom plate of the corridor as a whole shows that the resistivity of the east side is significantly lower than that of the west side in the same depth range, indicating that the water content of the soil on the east side is significantly higher than that on the west side.
4.2 Analysis of the results of multi-channel transient surface wave method detection
Multi-channel transient surface wave exploration data processing and interpretation are processed by special software, after filtering and de-noising, multi-gun superposition, FK frequency spectrum analysis, frequency dispersion curve extraction, and obtaining V-H velocity depth curves to invert the stratigraphic wave velocity distribution. Figures 5A, B show the depth-velocity curves after data processing, and Figures 5C, D show two-dimensional contour plots of the stratigraphic wave velocity distribution after integrated inversion. Analyzing the change characteristics of the V-H velocity curve, it is found that the wave velocity of the soil layer in the range of 3.0 m below the bottom plate on the east side of the pipeline corridor decreases sharply, the shear wave velocity Vs. decreases from 232 m/s to 147 m/s, and the slope of the dispersion curve changes significantly, and the presence of sparsity is suspected in the range of this depth. The wave velocity value of the soil layer in the range of 5.5 m below the bottom plate of the pipeline corridor on the west side is 155 m/s, and the wave velocity value is obviously low, indicating that there is a suspected loose body.
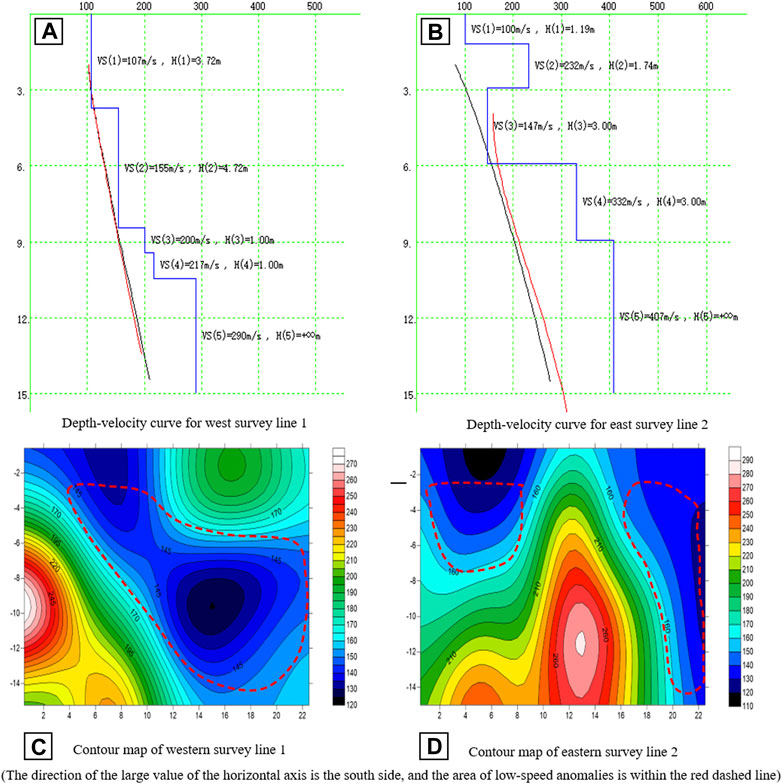
FIGURE 5. Depth-velocity curves versus two-dimensional contour plots of stratigraphic wave velocity distributions.
The wave velocity contour map shows that there exists a low wave velocity zone with gradually increasing depth and width in the west side of the pipeline corridor at pile number K0+572∼K0+590 (horizontal coordinates 4–22), and the depth range is from 0.0 m below the bottom plate to a maximum depth of 12.0 m (red dashed area in Figure 5C). There are two main low-wave velocity areas on the east side of the pipeline corridor (red dashed area in Figure 5D), one of which is pile number K0+568∼K0+577 (horizontal coordinates 0–9), with a depth range of 0.0–5.0 m below the bottom plate of the pipeline corridor. The other one is pile number K0+584∼ K0+590 (horizontal coordinates 16–22), and the depth range is 0.0–12.0 m below the bottom plate of the pipe corridor. These two low-wave velocity areas are suspected to be sparsely populated.
Based on the above analysis, it can be seen that the detection results of the multi-channel transient surface wave method also indicate that there is a high probability of the presence of water-rich and loose bodies in the lower portion of the pipeline corridor, which coincides with the results obtained by the high-density resistivity method. The multi-channel transient surface wave test results corroborate with the high-density resistivity method and further refine the extent and depth of the subsurface diseased body, improving the reliability of the detection information.
4.3 Analysis of results of standardized penetration testing
The results of the standard penetration method show that the standard penetration numbers of the holding layer pulverized soil layer in the range of 2.0 m at the bottom of the foundation of 1#, 2# and 4# verification holes are smaller than those of the corresponding soil layer of 3# comparison holes. The standard penetration number of the silt layer in the depth range of 3.5m–6.5 m below the base of 1#, 2# and 4# verification holes is less than the standard penetration number of the corresponding soil layer of 3# comparison holes. It can be seen that both the chalk and silt layers in the area of the suspected sparsity are more sparse than the other areas where no anomalies were found. Figure 6A, B, D show sample soils from verification holes 1#, 2#, and 4#, respectively, and Figure 6C shows sample soils from comparison hole 3#. Based on the analysis of the soil samples taken from each of the boreholes in Figure 6, the samples from verification boreholes 1#, 2# and 4# have loose soil and high water content, while the sample from comparison borehole 3 has relatively dense soil and low water content. Figure 6 shows photographs of soil samples from the four borings at the site, and Table 2 shows the results of the standard penetration tests.
4.4 Comparative analysis
Table 3 shows the results of the comparative analysis of the two physical means and the standard penetration probing method. Comparing and analyzing the detection results of high-density resistivity method and transient surface wave method, there are suspected water-rich and loose bodies in the soil layer below the bottom plate of the section of pile number K0+572∼K0+590 on the west side of the pipeline corridor. There are suspected water-rich and loose bodies in the soil layer below the bottom plate of the section of pile number K0+568∼K0+590 on the east side of the pipeline corridor. The distribution of spars in the depth range of 1#, 2# and 4# verification holes is basically consistent with the inversion results of the two physical exploration methods. It can be determined that there are water-rich and loose bodies in the lower part of the corridor, and the strong rainfall makes the water content of the loose bodies increase, and the hydraulic effect triggered by pumping leads to the hollowing out of the loose bodies and the formation of cavities, which triggers the upper corridor to undergo inhomogeneous settlement.
5 Analysis and discussion of the chain of causes of pipeline corridor damage
5.1 Causal chain analysis
Based on the results of the above analysis, it is shown that there are two reasons for the cracking and damage of the pipe corridor. One reason is the defects of concrete elements caused by poor vibration and curing during concrete placement, which lay hidden dangers for subsequent cracking and damage of concrete structures. Another reason is the uneven settlement of the foundation of the pipe corridor. The overlying soil layer of the corridor is thin, and the possibility of structural deformation and cracking of the corridor due to the loading of the overlying soil layer is small, so this is not considered as one of the causes of cracking and damage of the corridor. Figure 7 shows the network diagram of the chain relationship between the causes of damage to the pipe corridor.
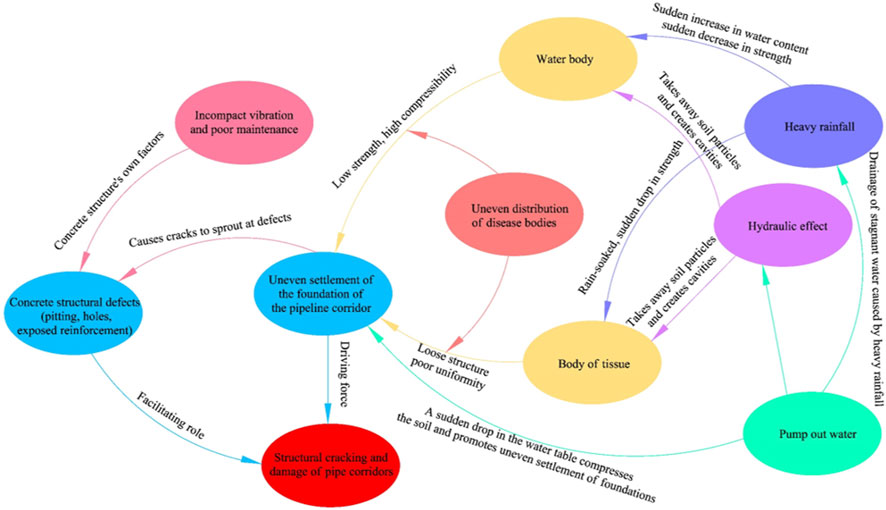
FIGURE 7. Network diagram of chain relationship between causes of cracking and damage in pipe corridors.
Uneven foundation settlement is the main driver of cracking and deformation of concrete structures. Due to the uneven force applied to the concrete structure as a result of the uneven settlement, stress concentration occurs at the variable cross section of the pipe corridor structure and at the concrete defects, and initial cracks start to breed in these places. With the further development of the uneven settlement of the foundation, the cracks on the concrete structure gradually expand and develop, cracks and holes in the concrete structure and pockmarked surface connected to each other through, and eventually formed through the cracks, the structure of the corridor subsequently cracking damage. It can be seen that the uneven settlement of the foundation of the corridor is the main driving factor leading to the deformation and damage of the corridor, which provides the driving force for the cracks on the structure of the corridor to sprout and expand. Defects on concrete members are promoters of deformation cracking, and under the action of uneven settlement, the defects on concrete members are activated, and cracks begin to gestate and sprout at the defects.
Uneven settlement of the foundation becomes the main driving factor for the cracking and deformation of the pipe corridor structure, and the factors leading to the uneven settlement of the foundation are the fundamental factors for the cracking and damage of the pipe corridor. By means of physical exploration to explore the reasons for the uneven settlement of the foundation of the pipe corridor, the results show that the pipe corridor pile number K0+568 ∼ K0+590 section of the bottom plate below the depth range of 0.0 m–12.0 m, the foundation holding layer is suspected to exist in the water-rich body, sparse body, at the same time in the pile number K0+564, K0 + 566, K0 + 559, the pipe corridor due to the uneven settlement of the foundation to produce serious damage. In order to further verify the reliability of the results of the physical exploration, three verification holes and one comparison hole were excavated within the uneven settlement range of the pipeline corridor, and the distribution of loose bodies within the depth range of the 1#, 2# and 4# verification holes exposed basically coincided with the results of the inversion of the two physical exploration methods. By analyzing the results of physical exploration and verification of soil extraction, it was found that the main reasons leading to the uneven settlement of the foundation are the following 3 points.
(1) Several spars and water-rich bodies exist in the depth range of 0–12.0 m below the base plate of the pipeline corridor in this section. Loose bodies are characterized by loose structure and poor homogeneity, and water-rich bodies are characterized by high water content, low strength and high compressibility. Heavy rainfall led to a sudden increase in the water content of the soil around the corridor, the strength of the loose body was significantly reduced by rainwater soaking, the water content of the water-rich body further increased, the strength of the further reduction of its compression at the same time increased. Due to the uneven distribution of loose and water-rich bodies below the subgrade of the corridor, this leads to an uneven settlement of the soil layer below the subgrade. This is the stratigraphic condition in which uneven settlement occurs in the pipeline corridor.
(2) The pumping of water after heavy rainfall caused a rapid decline in the water table, the effective stress of the foundation soil below the base plate of the corridor increased, soil compression occurred, and further contributed to the uneven settlement of the foundation, which is also an important causative factor for the aggravation of the uneven settlement of the foundation.
(3) The pumping operation also triggered localized hydraulic action, and such underground diseased bodies as loose bodies and water-rich bodies were weakened by localized hydraulic action, the soil structure was weakened, the strength was reduced, and the bearing capacity of foundations was decreased. The hydraulic action takes away the soil particles within the loose body and water-rich body, leading to the development of the loose body and water-rich body into a cavity and accelerating the rate of settlement.
(4) The cross-section area of the pipe corridor structure is changing, and under the effect of uneven settlement, the junction of the cross-section change will have a significant stress concentration phenomenon, which will lead to the cracking damage of the pipe corridor structure.
Combined with the analysis of the actual situation on the site, the first time after the rain pumping caused uneven settlement, K0 + 579 position deformation joints staggered, the corridor substrate soil extrusion, surging into the interior of the corridor. The second pumping location at K0+579 deformation seam caused the loss of powdered soil in the holding layer of the base of the pipe corridor, destroying the soil structure of the base and increasing the sinking of the pipe corridor at both ends of the deformation seam.
5.2 Discussion
This paper investigates the cracking and damage characteristics and uneven settlement factors of Qihe pipe corridor. The results of the study show that there are self-factors and external factors in the cracking damage of the pipe corridor, and the self-factors are the self defects such as pockmarks, holes, exposed tendons and other defects caused by the lack of compactness of vibration and poor maintenance during the pouring of concrete. The external factor is the uneven settlement of the foundation of the pipe corridor. There are four reasons for the uneven settlement of the foundation of the pipe corridor: 1) the loose and water-rich bodies distributed in the foundation of the pipe corridor. 2) Heavy rainfall causes a sudden increase in the water content of water-rich and loose bodies in foundations, which reduces strength and increases compressibility. 3) Pumping causes the water table to fall, the effective stress on the foundation soil increases, and the soil is compressed, thus promoting increased uneven settlement. 4) Pumping causes localized hydraulic action, and soil particles in loose and water-rich bodies are carried away under hydraulic action, thus developing into cavities.
In the study of the uneven settlement factors of the foundation of the pipeline corridor, it was found that the localized hydraulic action caused by pumping carried away the soil particles in the loose and water-rich bodies, resulting in uneven settlement of the foundation. The main cause of cracking and damage in pipe corridors is the uneven settlement of the foundation. These two results coincide with the findings of Yang Zhao (Zhao et al., 2021). Yang Zhao analyzed the cracks triggered by the uneven settlement of the comprehensive pipeline corridor of Fuzhou Southeast Expressway and its causes, and used numerical simulation methods to analyze the plastic damage area and crack expansion process of the corridor (shown in Figure 8). Figures 8A, B show water seepage in the deformation joints and misalignment of the deformation joints, respectively, and Figures 8C, D show cracks in the top slab and cracks in the sidewalls; Figure 8E shows the finite element model of the corridor, Figure 8F shows the Y-direction stress cloud diagram of the corridor, Figure 8G shows the curve of variation of the tensile stress of the top slab of the corridor with the length of dehiscence at the bottom of the slab, and Figure 8H shows the on-site drainage from the precipitation wells; Figure 8I shows the rate of decrease in the stiffness of the concrete, Figure 8J shows the degree of tensile damage of the concrete, and Figure 8K shows the results of the analysis of crack extension in the corridor. It was found that the main cause of cracking in integrated pipe corridors is uneven settlement of foundations. The pumped water carries a large number of fine sand particles, and the soil on the outside of the pit is partially hollowed out, leading to uneven settlement and misalignment of the deformation joints in the later stage of the pipe corridor. This is the root cause of cracking in large mileage sections of pipe corridors. Numerical simulation results show that tensile stresses will occur locally in the top slab of the pipe corridor in the event of dehiscence at the bottom of the slab. Near the K3+917 location in the middle of the corridor, the concrete in the top slab and side walls showed a decrease in stiffness and tensile damage zones, indicating that the concrete had cracked. The cracks in the pipe corridor will expand from the top plate to the side wall and center wall, and the crack expansion pattern is more consistent with the actual crack pattern in the field. The uneven settlement of the comprehensive pipeline corridor of Fuzhou Southeast Expressway studied by Yang Zhao is basically consistent with the object of this paper in terms of engineering geological conditions, cross-section shape of the pipeline corridor, pumping operation, and deformation mode of cracking, and the conclusions drawn are also in line with each other. Uneven settlement is the main cause of pipeline corridor cracking, pumping-induced hydraulic action to take away the fine sand particles in the foundation soil, resulting in hollowing out of the soil and dehollowing at the bottom of the slab. The research results obtained by Yang Zhao further support the conclusions of this paper, and his numerical simulation results are also consistent with the cracking of the Qihe pipe corridor, so that the numerical simulation results in Yang Zhao’s paper can well reflect the plastic damage region and the crack extension process of the pipe corridor studied in this paper.
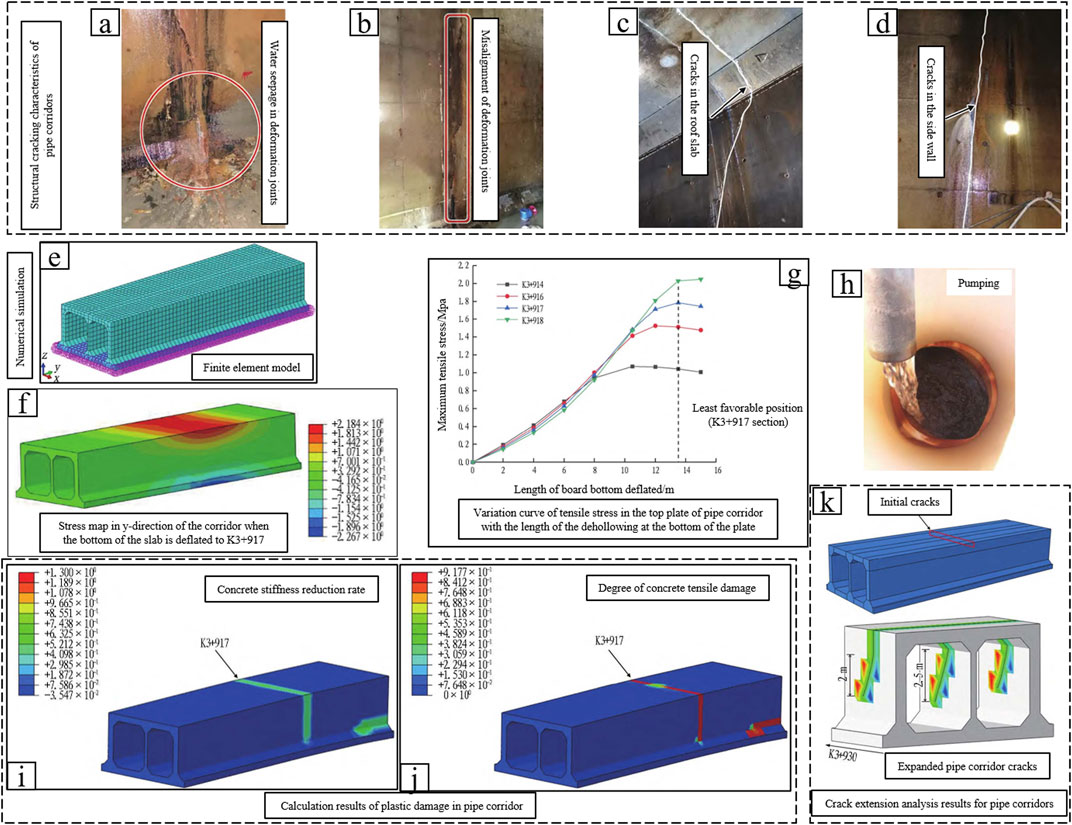
FIGURE 8. Uneven settlement cracking characteristics and Numerical Simulation Analysis of Comprehensive Pipe Corridor in Fuzhou Southeast Expressway (Zhao et al., 2021).
The object of the study is the cracking damage caused by uneven settlement of underground structures in soft ground. Physical exploration methods and standard penetration detection methods are used to reveal the causes of uneven settlement, and numerical simulation methods are used to analyze the cracking process of underground pipe corridors subjected to uneven settlement, and the results of the study provide guidance for the prevention and control of uneven settlement of underground structures. This paper investigates the effect of uneven settlement on the cracking damage of underground pipeline corridors in sandy chalky soil stratum, reveals the main reasons for triggering uneven settlement, and provides guidance for the repair and prevention of underground pipeline corridors. The results of the study are primarily for diseased bodies in foundations, and when foundations without diseased bodies are encountered, the results of this analysis will be unguided. Underground engineering geological conditions are complex and variable, the object studied in this paper is the cracking damage characteristics of underground pipeline corridors triggered by inhomogeneous settlement in soft ground, the research object is relatively single, unable to reflect the deformation characteristics of underground structures under various engineering geological conditions in a holistic and comprehensive manner. Subsequent studies should continue to characterize foundation settlement under different geological conditions and the deformation and damage characteristics of underground structures, so as to provide more comprehensive guiding information for the construction of underground structures and disaster prevention and control.
6 Conclusion
In this paper, the deformation and cracking caused by inhomogeneous settlement of Qihe tube corridor is studied, and the deformation characteristics of the corridor and the reasons for the occurrence of inhomogeneous settlement are analyzed, and the following conclusions are drawn.
(1) The concrete of the pipe corridor structure has exposed reinforcement, pockmarks and holes, and the subsequent deformation cracks start to develop and further expand from these defects and damages. Defects and damages on concrete elements are contributing factors for deformation and cracking of pipe corridors, and uneven settlement is the main initiating factor for deformation and cracking of pipe corridors. The research results are of guiding significance for the prevention and control of cracking damage in underground pipeline corridors.
(2) The detection of high-density resistivity method shows that along the length direction of the pipe corridor, the section of pile number K0+578∼K0+586.5, there is an obvious low-resistance area below the bottom plate of the pipe corridor, with a resistivity value of ≤8.0Ω-m, which is suspected to be the distribution of water-rich and sparse bodies. The results of the multi-channel transient surface wave method of detection are in agreement with the high-density resistivity method.
(3) Through the standard penetration detection, it was found that the distribution of spars in the depth range of 1#, 2# and 4# verification holes was basically consistent with the inversion results of the two physical exploration methods.
(4) Stratigraphic factors that lead to uneven foundation settlement are water-rich and loose bodies in the lower part of the strata, and localized hydraulic effects, such as pumping, are catalysts for accelerating uneven foundation settlement.
(5) The research results reveal the triggering factors of uneven settlement of sandy chalky soil and the intrinsic and extrinsic causes of structural damage of underground pipeline corridors, and the results provide technical guidance for disaster prevention and control in similar projects.
Data availability statement
The original contributions presented in the study are included in the article/Supplementary material, further inquiries can be directed to the corresponding author.
Author contributions
YZ: Writing–original draft, Data curation. LD: Formal Analysis, Writing–original draft. YL: Conceptualization, Writing–original draft. MG: Funding acquisition, Writing–review and editing.
Funding
The author(s) declare that financial support was received for the research, authorship, and/or publication of this article. This work was supported in part by the Opening fund of State Key Laboratory of Geohazard Prevention and Geoenvironment Protection (Chengdu University of Technology) (No. SKLGP 2022K014), the Opening Foundation of Key Laboratory of Landslide Risk Early-warning and Control, Ministry of Emergency Management (Chengdu University of Technology) (No. KLLREC 2022K003), the Opening Foundation of Key Laboratory of Geohazard Prevention of Hilly Mountains, Ministry of Natural Resources (Fujian Key Laboratory of Geohazard Prevention) (Grant No. FJKLGH 2022K005), and the Humanities and Social Sciences Research Project of Ministry of Education (No. 23YJCZH051).
Conflict of interest
Authors YZ, LD, and YL were employed by Shandong Construction Engineering Quality Inspection and Testing Center Co., Ltd.
The remaining authors declare that the research was conducted in the absence of any commercial or financial relationships that could be construed as a potential conflict of interest.
Publisher’s note
All claims expressed in this article are solely those of the authors and do not necessarily represent those of their affiliated organizations, or those of the publisher, the editors and the reviewers. Any product that may be evaluated in this article, or claim that may be made by its manufacturer, is not guaranteed or endorsed by the publisher.
References
Al’ Malul, R., and Michail, G. (2018). The reliability of multistory buildings with the effect of non-uniform settlements of foundation. E3S Web Conf. 33, 02040. doi:10.1051/e3sconf/20183302040
Bao, C., Xu, F. Z., Chen., G., Ma, X. T., and Lim, K. (2020). Seismic response analysis of frame structure with uneven settlement of foundation. Phys. Chem. Earth(prepublish) 120, 102928. doi:10.1016/j.pce.2020.102928
Bian, H., Zhang, X., and Shao, J. (2017). A coupled elastoplastic and visco-plastic damage model for hard clay and its application for the underground gallery excavation. Undergr. Space 1, 60–72. doi:10.1016/j.undsp.2017.03.002
Chen, W., and Hanna, A. (2023). Experimental investigation on structural response of multi-story buildings subjected to differential settlement of its foundations. Int. J. Struct. Integr. 14 (2), 204–228. doi:10.1108/IJSI-09-2022-0115
ChenChuanXin, D. L. R., Liu, R. X., and Li, D. K. (2011). Analysis of the effect of super-structure’s stiffness on foundation’s uneven settlement. Adv. Mater. Res. 374-377, 1690–1693. doi:10.4028/WWW.SCIENTIFIC.NET/AMR.374-377.1690
Cong, S., Tang, L., Ling, X., Geng, L., and Lu, J. (2021). Numerical analysis of liquefaction-induced differential settlement of shallow foundations on an island slope. Soil Dyn. Earthq. Eng. 140, 106453. doi:10.1016/j.soildyn.2020.106453
Deng, B., Pan, L., Xin, L., Jiang, T., and Zhi, B. (2022). Mechanical behavior of underground pipe gallery structure considering ground fissure. J. Mt. Sci. 19 (2), 547–562. doi:10.1007/S11629-021-6867-3
Feng, X., Li, H., Chen., B., and Zhou, J. (2020). Numerical investigations into the failure mode of buried prestressed concrete cylinder pipes under differential settlement. Eng. Fail. Anal. 111, 104492. doi:10.1016/j.engfailanal.2020.104492
Gebremedhn, Z., Qiao, G., and Li, J. (2019). The influence of differential settlement analysis for the single and multi-combined longitudinal precast box culvert. J. Civ. Environ. Eng. 2019, 2.
Guo, W., Song, M., Teng, L., Liao, X., Pei, N., and Chen, X. (2023). Stochastic differential equation modeling of time-series mining induced ground subsidence. Front. Earth Sci. 10, 1026895. doi:10.3389/FEART.2022.1026895
Ho, H. M., and Kuwano, J. (2021). Confined-reinforced earth with various geogrid lengths in reducing differential settlement. Geosynth. Int. 28, 560–573. doi:10.1680/JGEIN.21.00021
Hu, L., Xu, Q., and Liu, Y. (2021). Method for diagnosing the uneven settlement of a rail transit tunnel based on the spatial correlation of high-density strain measurement points. Sustainability 16, 9245. doi:10.3390/SU13169245
Jiang, J., Dong, B., Ding, Z., Gang, W., Zhang, H., and Liao, J. (2020). Dynamic analysis of metro train-monolithic bed track system under tunnel differential settlement. Shock Vib. 2020, 1–12. doi:10.1155/2020/5632180
Liang, X., Qiu, Z., Yang, L., Dong, Y., and Zhou, X. (2023). Rigid spliced box girder bridge under the action of foundation settlement difference analysis of mechanical behavior of splice section. J. Phys. Conf. Ser. 1, 12093. doi:10.1088/1742-6596/2468/1/012093
Liu, H., and Moore, A. (2021). The detection method for uneven settlement of foundation in the area of industrial waste miscellaneous filling. Int. J. Environ. Technol. Manag. 24 (3-4), 135. doi:10.1504/IJETM.2021.116805
Liu, P., Chen, J., Chen, Y., Yang, J., and Tang, Q. (2020). Mechanical model for joints of immersed tunnel considering the influence of joint differential settlement. Int. J. Geosynth. Ground Eng. 6 (4), 57. doi:10.1007/s40891-020-00241-y
Ngugi Hannah, N., Shitote Stanley, M., and Ambassah Nathaniel., (2021). Effect of variation in moisture content on soil deformation and differential settlement of frame structures in nairobi area and its environs. Open Constr. Build. Technol. J. 15, 106–128. doi:10.2174/1874836802115010106
Shen, Q., Lu, Y., Yang, Y., and Guanxu, L. (2021). Research on mechanical response of pavement structure to differential settlement of subgrade on highway widening. Adv. Mater. Sci. Eng. 2021, 1–11. doi:10.1155/2021/4445185
Shi, L., He, J., Huang, Z., Sun, H., and Yuan, Z. (2022). Numerical investigations on influences of tunnel differential settlement on saturated poroelastic ground vibrations and lining forces induced by metro train. Soil Dyn. Earthq. Eng. 156, 107202. doi:10.1016/J.SOILDYN.2022.107202
Wang, B., Liu, Z., and Yuan, S. (2022). Behavior of large shaft sinking headframe subjected to uneven foundation settlement. Front. Earth Sci. 10, 941126. doi:10.3389/FEART.2022.941126
Wang, T., Bui, P., Luo, Q., Liu, K., and Zhang, L. (2023). Three-dimensional physical modeling of load transfer in basal reinforced embankments under differential settlement. Geotext. Geomembranes 51 (2), 330–341. doi:10.1016/J.GEOTEXMEM.2022.12.001
Wu, H., Zhai, K., Fang, H., Wang, F., Xiang, Y., and Li, B. (2022). Bell-and-spigot joints mechanical properties study of PCCP under the uneven settlement of foundation: simulation and full-scale test. Structures 43, 1692–1703. doi:10.1016/J.ISTRUC.2022.07.060
Xie, J., Huang, N., Feng, J., and Zhang, G. (2020). Study on deformation of shallow buried underground pipe gallery. IOP Conf. Ser. Mater. Sci. Eng. 741, 012058. doi:10.1088/1757-899x/741/1/012058
Xu, F., Bao, C., Ma, X., Zhang, Y., Kar, S. L., Zhang, Y., et al. (2022). Shaking table test on seismic response of a planar irregular structure with differential settlements of foundation. Structures 46, 988–999. doi:10.1016/J.ISTRUC.2022.10.090
Xu, Z., Xu, W., Zhu, Z., and Zhao, J. (2023). Research on monitoring and stability evaluation of ground subsidence in gypsum mine goaf. Front. Environ. Sci. 10, 1097874. doi:10.3389/FENVS.2022.1097874
Zhang, J., Zhao, M., and Chang, S. (2021). Early warning limits for uneven settlement of metro tunnels based on vehicle–track interaction simulations. J. Civ. Struct. Health Monit. 11 (2), 507–520. doi:10.1007/S13349-020-00465-6
Zhang, K., Zhang, X., and Zhou, S. (2023). Analysis on dynamic behavior of 400 km/h high-speed train system under differential settlement of subgrade. Eng. Struct. 278, 115521. doi:10.1016/J.ENGSTRUCT.2022.115521
Zhao, B. (2022). Study on the influence of uneven settlement andrainfallinfiltration of loess filling foundation onpile-raftfoundation. Chang’an University.
Zhao, Y., Li, Y., and Yang, R. (2021). Analysis on cracks of cast-in-place utility tunnel induced by uneven settlement: a case study of utility tunnel of Fuzhou Southeast freeway. Tunn. Constr. 41 (12), 2098.
Keywords: sandy chalky soil, uneven settlement, underground pipe corridors, rich water bodies, lax bodies, numerical simulation
Citation: Zhang Y, Duan L, Li Y and Gao M (2024) Study on deformation characteristics of underground pipeline corridors and uneven settlement factors of foundations in sandy chalky soil layer. Front. Earth Sci. 11:1266559. doi: 10.3389/feart.2023.1266559
Received: 25 July 2023; Accepted: 08 November 2023;
Published: 25 January 2024.
Edited by:
Yunhui Zhang, Southwest Jiaotong University, ChinaReviewed by:
Huajin Li, Chengdu University, ChinaChangʼAn Qin, Beijing University of Civil Engineering and Architecture, China
Copyright © 2024 Zhang, Duan, Li and Gao. This is an open-access article distributed under the terms of the Creative Commons Attribution License (CC BY). The use, distribution or reproduction in other forums is permitted, provided the original author(s) and the copyright owner(s) are credited and that the original publication in this journal is cited, in accordance with accepted academic practice. No use, distribution or reproduction is permitted which does not comply with these terms.
*Correspondence: Meiben Gao, gaomb@mail.xhu.edu.cn